- 1Nofima AS, The Norwegian Institute of Food, Fisheries and Aquaculture Research, Tromsø, Norway
- 2Department of Mechanical Engineering and Technology Management, Norwegian University of Life Sciences, Ås, Norway
- 3Havbruksstasjonen i Tromsø AS, Kårvik, Norway
Introduction: The use of recirculating aquaculture systems (RAS) to produce Atlantic salmon smolts has resulted in exceptionally high fish growth rates. However, there are potential negative trade-offs between fast growth and key physiological processes, such as inadequate smoltification and early sexual maturation, which can both be linked to body energy reserves.
Methods: This study determined the effect of i) dietary fat levels on Atlantic salmon whole-body fat and fatty acids composition, growth performance and smoltification and ii) a previous dietary regime on seawater growth performance and male early sexual maturation. In freshwater RAS, salmon parr (~19g) were fed 3 fat levels (20, 24, and 28%) over a 14-week period at 12°C. Subsequently, in seawater flow-through systems, smolt (~96g) were fed a control diet (26%) for 12 weeks at 2 temperatures (12 and 16°C).
Results: Dietary fat levels resulted in differences in k-factor, fish whole-body fat and fatty acids composition; the low fat diet resulted in fish with a 23% lower whole-body fat when compared to the other two dietary groups at the end of the freshwater phase. These differences in whole-body fat faded at the end of the seawater phase. During the freshwater phase, all three feeds resulted in a comparable growth performance and smoltification indicator values, including k-factor, gill Na+, K+-ATPase, blood serum chloride and smolt index score. In contrast, water temperature resulted in fish body weight differences, where groups reared at 16°C were larger than those reared at 12°C. Nevertheless, both temperature regimes supported a similar thermal growth coefficient. Smolts grown at 16°C showed a higher level of the sex steroids androstenedione compared to those at 12°C. However, 11-ketotestosterone and testosterone levels did not differ despite a trend for higher levels at 16°C. Furthermore, testis histology at the final sampling indicated that some individuals showed initial signs of maturation (stage 3).
Discussion: In conclusion, varying dietary fat levels (20 - 28%) during the freshwater phase did not influence smoltification or male early sexual maturation during the subsequent grow-out phase. However, a temperature increase from 12 to 16°C resulted in larger fish and appeared to stimulate early male maturation in some fish individuals.
1 Introduction
Currently the most common way of producing Atlantic salmon (Salmo salar) consists of a two-phase production cycle matching the salmon’s anadromous lifestyle. First, parrs (juvenile fish) are produced and smoltified in a freshwater hatchery; thereafter, smolts (seawater ready fish) are transferred to sea cages for grow-out (Bergheim et al., 2009). During the past decade freshwater hatcheries have been replaced from conventional flow-through systems to recirculating aquaculture systems (RAS).
RAS are among the most environmentally sustainable systems to culture fish, and in Atlantic salmon production is especially relevant due to the possibility for water temperature control, freshwater reutilization, higher fish densities and larger smolts production (Mota et al., 2022). The production protocols are salmon producer and site specific but generally during the freshwater phase, i.e., first feeding to smolt, the follow production conditions can be observed: water temperature between 12 – 14°C, feeding continuous and to apparent satiation, photoperiod 24 hours light, water exchange rate 400 – 800 L/kg feed and fish densities up to 60 – 80 kg/m3. (V.C. Mota, personal communication, 2024). These RAS controlled production environment results in optimal conditions for fast fish growth and shorter production time. However, there are potential trade-offs between this fast growth and key physiological processes such as inadequate smoltification and early sexual maturation which can ultimately impact the fish health and welfare, and the final product quality including poorer fish fillet quality.
Smoltification or parr-smolt transformation constitutes a suite of physiological, morphological, and behavioural changes such as the development of hypo-osmoregulatory ability that prepares the Atlantic salmon for a life at sea. It is triggered in parr that have passed a size threshold of approximately 12 cm in response to an increasing daylength during spring (Mccormick et al., 2007). The morphological and physiological remodelling is an energy demanding process primarily fuelled by lipolysis (Sheridan and Kao, 1998). The resulting fat mobilization in combination with the action of plasma growth hormone (GH) stimulates an increase in linear growth that becomes visible through a reduced condition factor at the end of smoltification (Mccormick et al., 1995).
Sexual maturation of Atlantic salmon encompasses developmental, behavioural, morphological and physiological processes such as steroidogenesis and gonad development aiming to enhance reproductive capacity (Mobley et al., 2021). In an aquaculture context, early sexual maturation, particularly in male fish, is problematic as it compromises growth, changes fish external appearance, reduces meat quality, and increases mortality at sea leading to high economic loss for the fish farmer (Mcclure et al., 2007). Onset of early sexual maturation of males during the freshwater phase is often the result of fast growth triggered by a combination of 24-h light, relatively high rearing temperatures and energy-rich diets (Good and Davidson, 2016). A reduction in dietary fat levels during spring has been suggested as a possible solution to mitigate early sexual maturation (Good and Davidson, 2016).
A few studies have investigated how smoltification is affected by dietary fat levels and how seawater growth can be linked to dietary history and overall concluded that smolt production may not be impaired by a reduction in dietary fat content (Helland and Grisdale-Helland, 1998; Bendiksen et al., 2003; Berrill et al., 2004). However, these studies were conducted under seminatural conditions, using simulated natural photoperiod, and fluctuating, relatively low temperatures resulting in slow growing fish. In contrast, the intensive rearing conditions in RAS, such as higher temperatures and continuous light and feed promote high metabolic rates and fast growth. Consequently, there is a need to test the interrelationship, and possible interference, between feed containing different levels of dietary fat during smoltification in RAS, subsequent seawater performance and occurrence of early maturation in Atlantic salmon male fish.
The first objective of the present study was to determine the effect of different dietary fat levels on Atlantic salmon whole-body fat and fatty acids (FA) composition, growth performance and smoltification. The second objective was to assess the effects of a previous dietary regime on seawater growth performance and early male sexual maturation at two temperatures.
2 Materials and methods
2.1 Experimental design
Three experimental diet treatments with different fat inclusion were tested in triplicated groups: i) Low fat group - 20%, ii) Control group - 24% and, iii) High fat group - 28% in a Freshwater RAS phase (14 weeks) at 12˚C. This was followed by a Seawater FTS phase (12 weeks) at two temperatures i) 12˚C and ii) 16˚C and, during this period only one diet was used – Control (Figure 1). Due to a pump mechanical failure one Low fat replicate was lost after week 0, therefore N = 3, except for the Low fat group where N = 2, for all data presented in this study except gonad histology and sex steroids data. For these two variables fish was considered the experimental unit (N = 5 - 9).
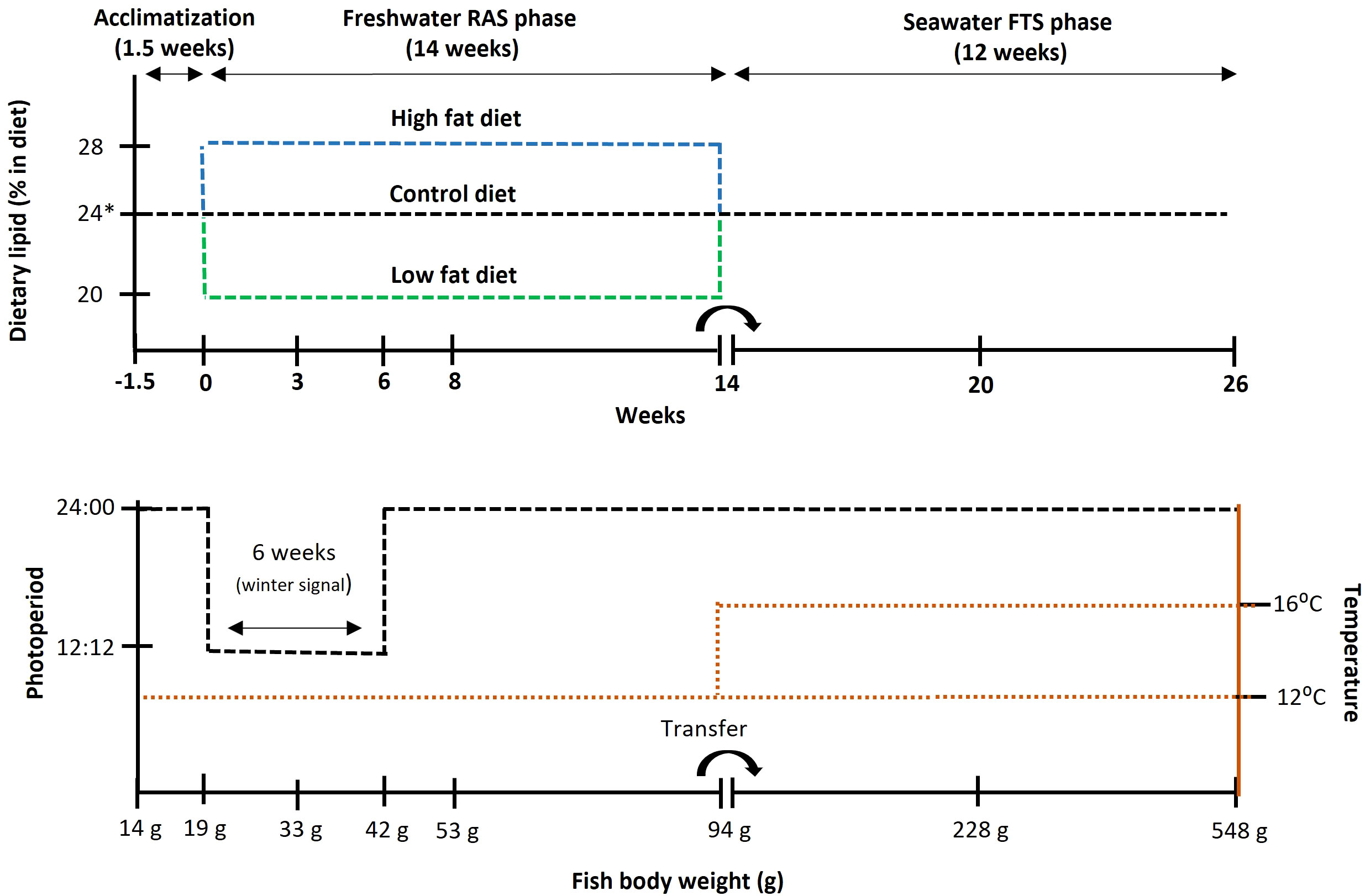
Figure 1 Schematic illustration of the experimental design. * The dietary lipid inclusion during Seawater FTS phase was 26% for all groups.
2.2 Fish
All procedures involving fish were authorized by the Norwegian Food Safety Authority (FOTS) under general husbandry practices and ID24383.
Atlantic salmon (Salmo salar) eyed eggs (AquaGen Atlantic QTL-innOva PRIME, AquaGen AS, Trondheim, Norway) were hatched and reared in a freshwater flow-through system (Tromsø Aquaculture Research Station, Kårvik, Norway) at ~ 7.5°C under continuous light (LD24:00) photoperiod until approximately 14g. At the end of this phase, all fish (N= 990) were individually PIT-tagged (12.5 mm, GPT12 PLT™, Biomark, USA) and randomly distributed over nine experimental tanks (N = 110/tank). The fish were acclimatized for 1.5 weeks to the rearing units and environmental parameters: dissolved oxygen > 85% saturation, pH = 7 - 7.5, temperature = 11.5 – 12.5°C and salinity = 1 - 2 ppt. Photoperiod was light-dark (LD) 24:00. Fish were fed continuously (approx. 23h day) with an automatic belt feeder over satiation (120%) with an acclimatization diet (control = 24% fat).
After the acclimatization phase, fish (N=110/tank; 18.8 ± 2.3g) were fed the three experimental diets (in triplicates) during a 14-week period, named the Freshwater RAS phase. During this period, fish parr were smoltified using a square wave photoperiodic regime consisting of 6 weeks “winter signal” (LD 12:12), followed by 8 weeks of LD 24:00. The continuous light, i.e. LD 24:00, was used in the current study as it was previously shown to result in the highest growth and it is a common practice in Atlantic salmon industry during the freshwater on-growing phase (Ytrestøyl et al., 2022). The water temperature was 12°C. At week 3, 6, and 8, five fish per replicate group were captured and transferred to a flow-through tank containing full-strength (34 ppt) seawater at 12°C for a 24-hour seawater challenge test (SWCT). At the end of the 14-week period, treatment groups were randomised using a common garden design and fish (N= 27 - 37 per replicate; 95.5 ± 19.3g) were moved to one of two seawater flow-through culture tanks for a subsequent 12-week period, named the Seawater FTS phase. During this phase one culture tank was kept at 12°C whereas the other was kept at 16°C; both tanks were kept at LD 24:00. All fish were fed a control diet resembling a commercial diet containing 26% fat during this phase.
2.3 Rearing units
The experimental Freshwater RAS phase (week 0 to week 14) was conducted in nine replicated RAS units that were described in detail in Mota et al. (2022). Briefly, each individual RAS unit consisted of a cylindro-conical fish tank (V = 0.5 m3); drum filter (micro-screen mesh size = 40 µm); a moving bed bioreactor (V = 0.2 m3, 750 m2/m3 bio-media), a gas exchange unit (CO2-degasser cylinder), low pressure oxygen cone (0.6 Bar) and a temperature control unit. Illumination was provided by a LED light (2358 lm, Pacific LED, Philips, The Netherlands) above each fish tank unit. The total RAS water volume was 0.8 m3, water flow to the fish tank was 1500 L/h.
The experimental Seawater FTS phase (week 14 to week 26) was conducted in two replicated octagonal fish tanks (V = 4 m3) with an average water flow of 3240L/h. Two holding sump tanks (V = 3.3 m3) were used to condition the water for each fish tank, i.e., the temperature was controlled by heat exchangers and the pure oxygen was added through a diffusor. The natural seawater was pre-filtered (drum filter micro-screen mesh size = 60 µm) and UV treated (2200W, WEDECO, ITT Water & Wastewater Herford GmbH, Germany) before flowing into the holding sump tanks.
2.4 Diets
Three experimental diets were formulated and produced at the Aquafeed Technology Centre (Nofima AS, Bergen, Norway) (Tables 1, 2). The control diet was produced in four pellet sizes, i.e., 1.25, 2, 3 and 4.5 mm and the Low Fat and High Fat diets were produced in two pellet sizes i.e., 2 and 3 mm. The diets were used in accordance with increasing fish size as shown in Figure 1, i.e., the Control diet was used for acclimatization, Freshwater RAS phase and Seawater FTS phase and, Low fat and High Fat were used for Freshwater RAS phase only. The control diet was formulated to resemble a commercial diet in terms of fat, protein and energy content to represent a benchmark “standard” for fish performance (Jobling, 2012).
Feed samples from all diet groups and pellet sizes (N = 8) were collected and stored frozen at -80°C until analysis. The chemical composition of the diets was determined via proximate composition analysis according to standard methods described previously by Bou et al. (2017). Briefly, dry matter content of the feeds was determined by gravimetric analysis following drying at 105°C for 16-18 h until the weight remained constant. Ash content was determined based on mass change after combustion in a muffle furnace at 550°C for 16 h. An elemental analyser (Flash 2000 Thermo Fisher Scientific, Cambridge, UK) was used to measure total N2 content which was used to calculate sample protein content based on N × 6.25. Gross energy was determined by isoperibolic oxygen bomb calorimetry (Parr 6200 calorimeter, Parr Instrument Company, Moline, USA).
2.5 Fish sampling and analysis
Seven sampling events took place throughout the experiment at weeks: 0, 3, 6, 8, 14, 20, and 26. Five fish per replicated treatment group were sampled in each event for tissues; except for week 0 where only three fish per group were sampled. Fish were euthanized with a bath overdose of Benzocaine (Benzoak vet, 200 mg/ml, EuroPharma, Leknes, Norway) prior to tissue sampling.
2.5.1 Survival and growth performance
Fish mortality was assessed and recorded daily in each fish tank. During all sampling events, individual weight (g) and fork length (cm) were measured. Condition factor and growth were calculated from these measurements.
Condition factor (K-factor) was calculated as (Equation 1):
Where W is individual weight in grams and L is the length in centimetres.
Thermal growth coefficient (TGC) was calculated as (Equation 2):
Where T is the water temperature in ˚C and Δt is the number of days between time T1 and T2.
Specific growth rate (% per day) was calculated as (Equation 3):
2.5.2 Fat content and fatty acid composition
Total lipids were analysed from the diet and whole-body of fish at the start (week 0), at the end of Freshwater RAS phase (week 14), and at the end of the Seawater FTS phase (week 26) following the method described by Folch et al. (1957). For whole-body analysis, five fish per tank were pooled and total lipids were extracted from the homogenate produced. A sample of 1 ml from the chloroform–methanol phase was used for analysis of FA composition of total lipids using the method described by Mason and Waller (1964). In brief, the extract was dried under N2 gas, and the residual lipid extract was transmethylated overnight with 2’,2’-dimethoxypropane, methanolic HCl and benzene at room temperature. The methyl esters were separated and analysed in a GC (Hewlett Packard 6890; HP) with a split injector, using an SGE BPX70 capillary column (length 60 m, internal diameter 0.25 mm and film thickness 0.25 μm; SGE Analytical Science), flame ionisation detector and HP Chem Station software. The carrier gas was Helium, and the injector and detector temperatures were both 280°C. The oven temperature was raised from 50 to 180°C at the rate of 10°C/min, and thereafter raised to 240°C at a rate of 0.7°C/min. Individual FA methyl esters were identified by reference to well-characterised standards. The relative amount of each FA was expressed as a percentage of the total amount of FA in the analysed sample, and the absolute amount of FA per gram of tissue was calculated using C23:0 methyl ester as the internal standard.
2.5.3 Blood serum chloride concentration
Blood samples were collected from the caudal vessels using Vacuette ® vacuum tubes (Greiner Bio-One, Kremsmunster, Austria) containing a clot activator (for serum). Serum was obtained by centrifugation at 4000 x G for 10 min., which was thereafter stored at −80°C until assayed. Blood serum chloride (Cl-) concentration was measured using a Pentra C400 system (Horiba, CA, USA).
2.5.4 Gill NKA activity
The second gill arch was dissected and placed in SEI buffer (250 mmol/L sucrose, 10 mmol/L Na2-EDTA, 50mmol/L imidazole, pH 7.3), snap frozen in dry ice and stored at −80°C until assayed. Gill NKA activity was assessed by Eurofins Food & Feed Testing Norway AS (Kambo, Norway) according to the method described by (Mccormick, 1993).
2.5.5 Smolt index
Smolt index was visually assessed by individually scoring of body silvering (1-absent to 4-silver), presence of parr marks (1-solid marks to 4-absent) and darkening of the distal edge of the caudal fin (1-absent to 4-black edges).
2.5.6 Gonad histology
Male gonads at the end of the Seawater FTS phase (week 26) were dissected and placed in 10% neutral formalin containers (BiopSafe®, Mermaid Medical, Denmark) and stored at 4 °C until analysis. Sectioning and staining of the tissue samples were done the Norwegian Veterinary Institute in Harstad, Norway. Briefly, the tissue sections were hydrated in water and stained with 1% Alican blue (Alfa Aesar) in 3% acetic acid for 15 min., transferred to 1% periodic acid for 10 min, followed by Schiffs reagent for 15 min, and finally in heamatoxylin for 30 s before dehydration and mounting. The sections were digitised with NanoZoomer S360 (Hamamatsu, Tokoyo, Japan). The maturational stage of the testis and its dominating germ cell score was determined according to a 6-point scoring system modified from Melo et al. (2014) and Ciani et al. (2021). The cell stages were scored as: I) Spermatogonia A un-differentiated and differentiated (SPA) - nuclear diameter ~10 µm, some heterochromatin, foamy appearance of sertoli cells, and presence of tubuli, II) Spermatogonia B (SPB) - nuclear diameter ~6 - 8 µm, high amount of heterochromatin, III) Spermatocytes (SC) – nuclear diameter ~ 3 - 4 µm IV) Spermatides (ST) - densely packed nucleus, ~1.5 - 2 µm, V) Spermatozoa (SZ) - Small densely packed nucleus with tail and VI) SZ Spermatozoa (SPZ) - SPZ dominating, large lumen in tubules (Figure 2).
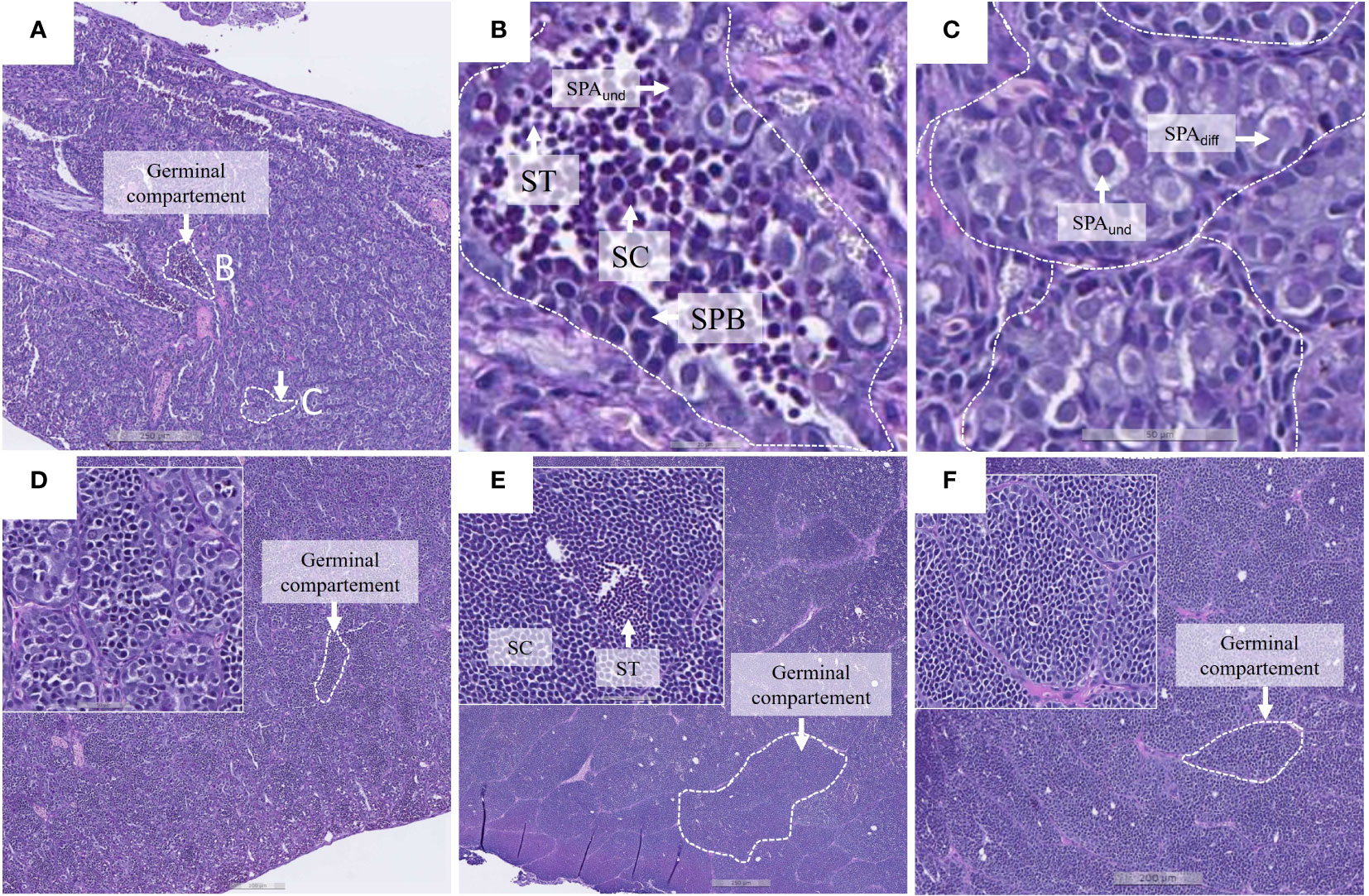
Figure 2 Images of Atlantic salmon testis representing the study population. (A–C) Testis with dominating stage I, and most advanced cell stage IV. Cell stages: I) spermatogonia A un-differentiated (SPAund) and differentiated (SPAdiff), II) spermatogonia B (SPB), III) spermatocytes (SC) and IV) spermatids (ST). (B) Magnification of an enlarged germinal compartment with presence of several germinal cell types. (C) Germinal compartment showing the most dominating germ cells present in the testis. (D–F) Three individuals with dominating cell stage III. Individuals showed large germinal compartments with presence of more advanced germ cell stages. Tissue sections stained with Alcian Blue and PAS.
2.5.7 Sex steroid hormone analysis
The concentration of three sex steroid hormones, i.e., androstenedione (A4), testosterone (T), and 11-ketotestosterone (11-KT), were measured in male serum samples at the end of the Seawater FTS phase (week 26) using a liquid chromatography–tandem mass spectrometry (LC-MS/MS) at the Proteomics and Metabolomics Core Facility (PRiME) at the University of Tromsø (Norway). Briefly, samples were analysed on an Acquity UPLC I-Class interfaced to a Xevo TQ-XS tandem mass spectrometer (Waters, Manchester, UK) with the following settings: ESI positive mode; capillary voltage 1 - kV; desolvation gas temperature - 550°C; source temperature - 150°C; desolvation gas flow – 1000 L/h; cone gas flow – 150 L/h; nebuliser pressure – 7 Bar. The chromatographic separations were done using a Acquity Cortecs T3 1.6 µm 100 x 2.1 mm (Waters, Manchester, UK) column maintained at 50°C with a flow rate of 0.3 mL/min. A linear gradient system composed of 0.1% formic acid in water, and 0.1% formic acid in methanol was used, starting from 40% (v/v) methanol, and increasing to 70% in 8 min., maintaining at 95% for 0.5 min. before returning to the starting conditions. The autosampler temperature was 6°C and the sample injection volume was 4 μl.
2.6 Water quality
Manual measurements and water samples were taken twice a month in the Freshwater RAS phase and daily in the Seawater RAS phase. Dissolved oxygen, pH, conductivity (salinity), and temperature were measured using a portable meter (FDO 925, Sentix 940 and TetraCon 925 sensors connected to a Multi 3630 IDS, WTW, Germany). Carbon dioxide was measured using a portable CO2 sensor (OxyGuard Pacific, OxyGuard, Denmark). Ammonium (NH4-N), nitrite (NO2-N), and nitrate (NO3-N) were measured from water samples using a spectrophotometer (Test Kit 1.14558.001, 1.14776.0001and 1.14942.0001, Spectroquant ®, Merck, Germany). Turbidity was measured from water samples using a (ORION AQ4500, Thermo Scientific®, Thermo Fisher Scientific, USA). All measurements and water samples were obtained from each fish tank outlet.
Hydraulic retention time (HRT; min.), indicator of average time that water was inside the fish tank, was calculated as (Equation 4):
Where V is the culture tank volume in L and Q is the water flow in L/min.
2.7 Statistics
Sex steroid data were acquired and analysed using MassLynx version 4.2 (Waters corporation, USA). Statistical analyses were performed with IBM® SPSS® Statistics V27 (IBM, Corp., USA). The experimental unit considered was the RAS unit (N = 3 or N = 2 for Low fat group) for all parameters with the exception of gonad histology and sex steroids data where fish were considered the experimental unit (N = 5 – 9). The relatively high cost and time-consuming analysis for these two variables resulted in a low fish number per treatment, thus the rationale to use fish as a replicate unit, similarly to other studies (Fjelldal et al., 2018; Ciani et al., 2021). Data in percentage (%) was transformed using arcsin [√ (x + 1)] prior to statistical analysis. Homogeneity of variance was assessed using a Levene’s test and normality using the Shapiro-Wilk test. A one-way ANOVA was used to assess the effect of dietary lipids composition (Diet) on fish and water quality parameters during the Freshwater RAS phase, and was followed by post-hoc Tukey test, if significant. A two-way ANOVA was used to assess the effect of dietary lipids composition (Diet in freshwater), water temperature (Temperature) and their interaction on fish parameters during the Seawater FTS phase, and was followed by post-hoc Tukey test, if significant. An independent samples t-test was used to assess the effect of water temperature on water quality parameters during the Seawater FTS phase. A significant level (α) of 0.05 was used for all analyses. All data are presented as mean ± standard deviation (S.D.).
3 Results
3.1 Survival and growth performance
Overall survival was high (> 99.0%) during both experimental phases. The exception was the Low fat group during the Freshwater RAS phase, where an acute mortality event occurred after sampling at week 8 in one of the replicated tanks, resulting in average treatment group survival of 90.9% (Tables 3, 4). The mortality was likely caused by a hydrogen sulphite release from one water pipe during RAS routine maintenance. Fish growth curves show that the body weight was similar among the dietary groups throughout the 26-week experimental period (Figure 3). In contrast, the body weight in the 16°C groups was significantly larger by 22% and 36% at respectively week 20 (P-value = 0.010), and week 26 (P-value< 0.001) compared to groups grown 12°C. Specific growth rate (SGR) for the Freshwater RAS phase did not differ significantly among the dietary groups, while SGR for the Seawater FTS phase was 19% significantly higher in the groups grown at 16°C when compared to the groups grown at 12°C (P-value< 0.001). The thermal growth coefficient (TGC) did not differ in any of the phases.
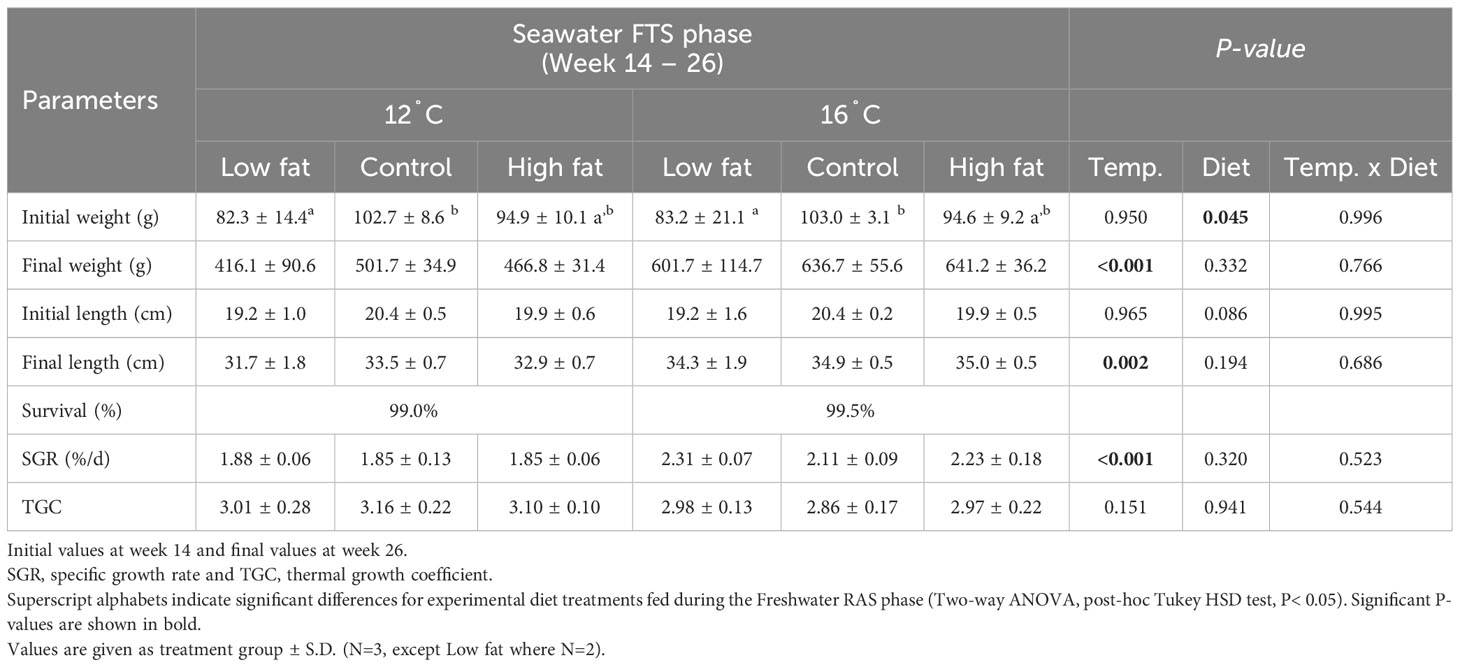
Table 4 Summary of performance parameters of Atlantic salmon during seawater FTS phase (week 14 and week 26).
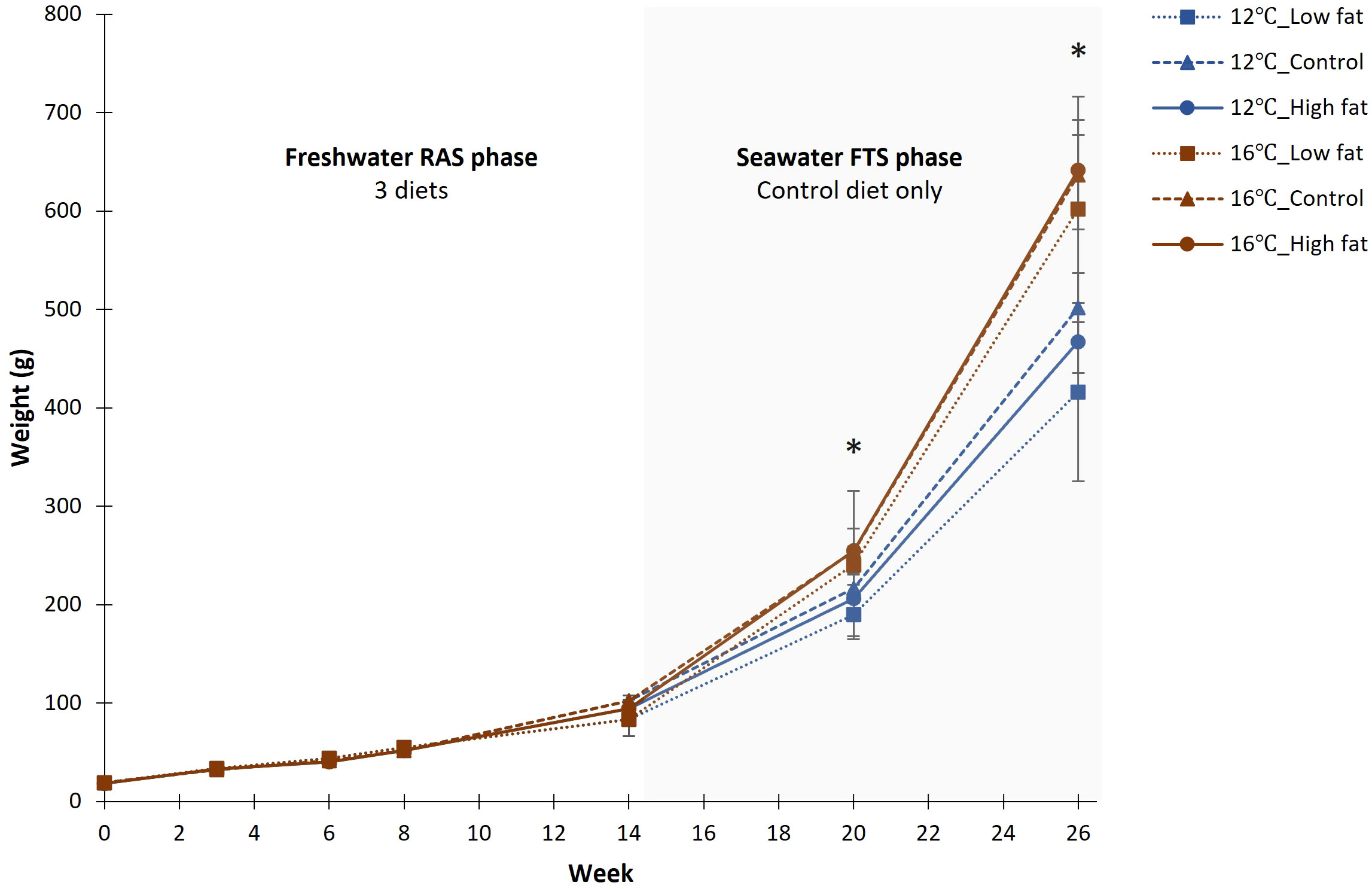
Figure 3 Changes in fish body weight (g) throughout the 26-week experimental period (N = 3, except N = 2 for Low fat group). Two periods are shown: Freshwater RAS (0 - 14 week) and Seawater FTS (14 – 26 week). * Indicate significant differences between the two temperature groups (P-value< 0.05).
3.2 Fat content and fatty acid composition
The whole-body fat at the start of the experiment (week 0) did not differ among the three dietary groups (11.3 ± 0.3%; Figure 4). In contrast, at the end of the Freshwater RAS phase (week 14), the whole-body fat from the Low fat group had decreased to 9.0 ± 0.7% and was significantly lower (↓ 23%; P-value = 0.009), when compared with the Control (11.1 ± 0.7%) and High fat groups (12.3 ± 0.7%). At the end of the Seawater FTS phase (week 26), the whole-body fat was not significantly affected by the dietary history of the fish. On the other hand, fish grown at 12°C had significantly lower (↓8%; P-value = 0.017) whole-body fat (14.4 ± 0.1%) when compared to the fish groups grown at 16°C (15.6 ± 0.4%).
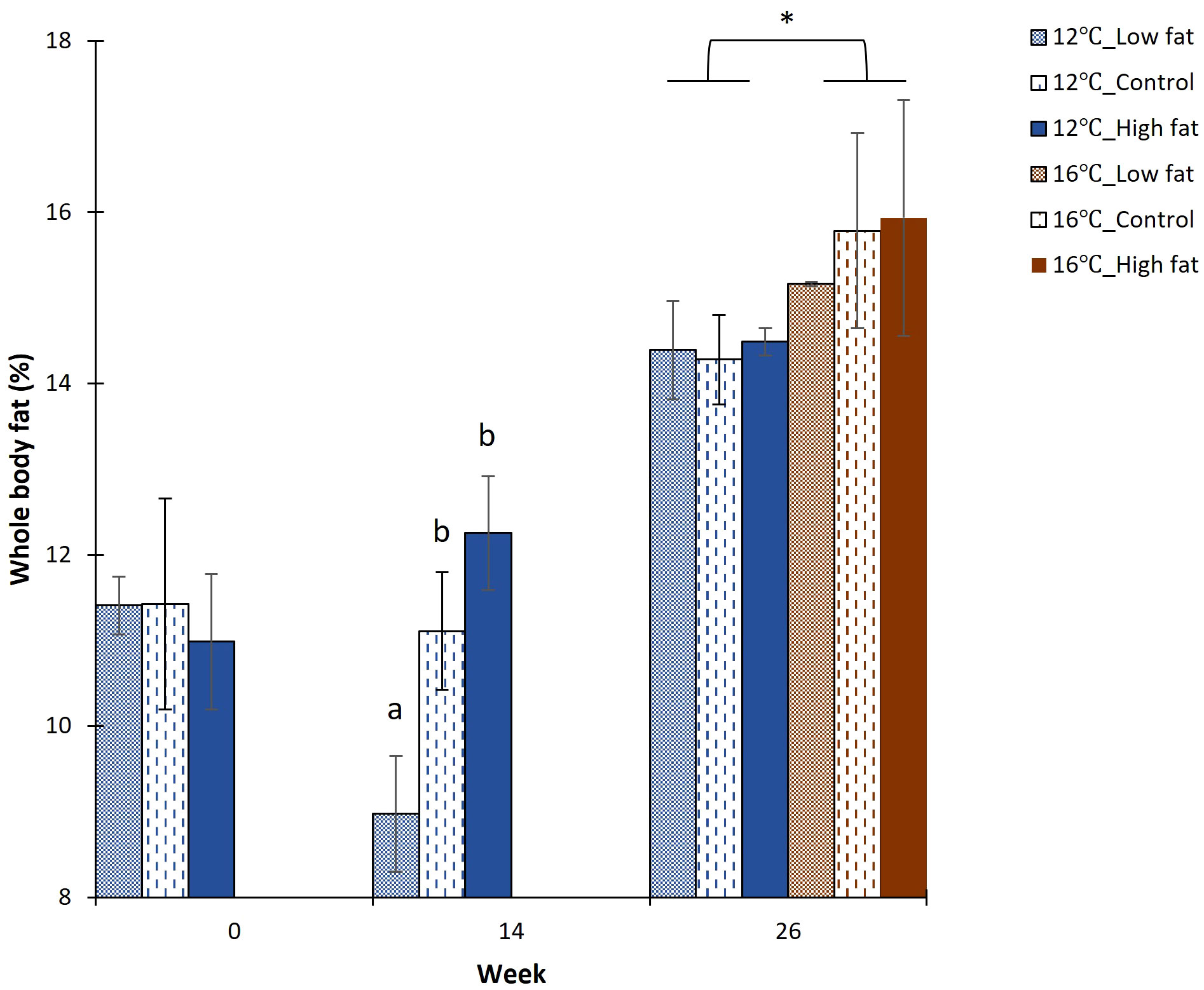
Figure 4 Fish whole-body fat content (%) at week 0, 14 and 26. Values are given as treatment group mean ± S.D. (N = 3, except Low fat where N = 2). Superscript alphabets indicate significant differences among diet groups and * indicates significant differences between temperature groups (P-value< 0.05).
The whole-body fish FA composition at the end of the Freshwater RAS phase (week 14) differed among some specific FA, including sums of omega-6 (P-value< 0.001) (Table 5). Nevertheless, no differences among the three groups were observed in the sums of saturated fatty acids (SFA), monounsaturated fatty acids (MUFA) and omega-3.
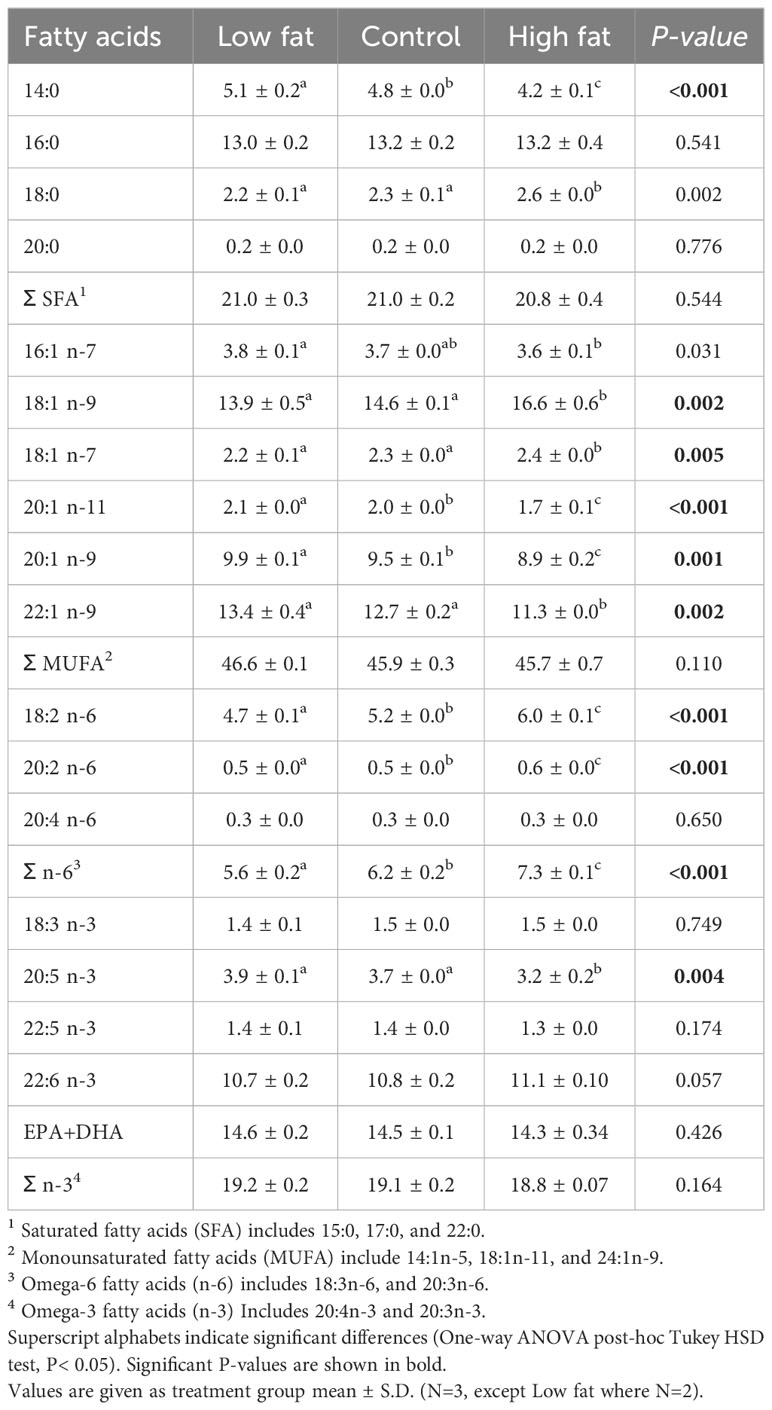
Table 5 Fatty acid composition (% of total fatty acids) in the whole-body of Atlantic salmon at the end of Freshwater RAS phase (week 14).
3.3 Smoltification indicators – freshwater RAS phase
The K-factor was constant between week 0 and week 8 ranging between 1.24 - 1.39, whereas at week 14 it had declined to a range between 1.12 – 1.20 (Figure 5A). Additionally, at week 14 the k-factor of the Low fat group was significantly lower (↓5%) when compared to Control and High fat groups (P-value = 0.001).
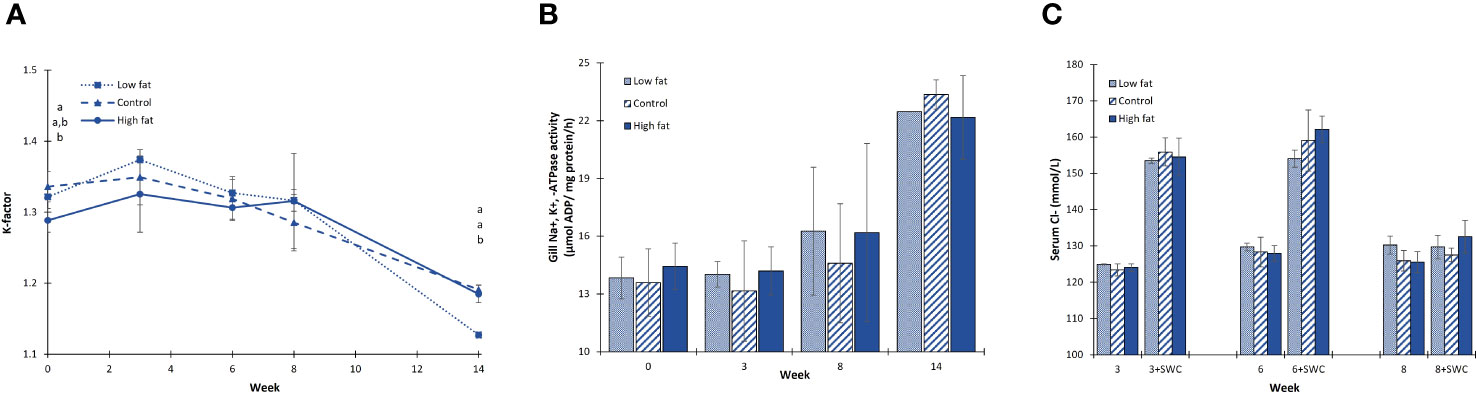
Figure 5 Fish smoltification parameters during the Freshwater RAS phase. (A) fish K-factor, (B) gill Na+, K+, -ATPase activity (µmol ADP/mg protein/h) and (C) serum Cl- (mmol/L). Values are given as treatment group mean ± S.D. (N = 3, except N = 2 for Low fat group all and N = 1 for Low fat group at gill Na+, K+, -ATPase activity week 14). Superscript alphabets indicate significant differences among diet groups (P-value< 0.05). SWC = fish exposed to a seawater (34 ppt) challenge for 24 h.
Gill NKA activity did not differ among the dietary groups (Figure 5B). Moreover, it appeared constant between week 0 and week 8 (7.9 - 18.5 µmol ADP/mg protein/h) with a subsequent peak at week 14 (19.7 – 24.2 µmol ADP/mg protein/h).
Serum chloride ions (Cl-) of fish exposed to a 24h seawater challenge (SWC) did not differ among the dietary groups (Figure 5C). Additionally, at week 3 and week 6 serum Cl- increased in response to a SWC by 30-31 mmol/L (↑23-25%), whereas this response was absent at week 8, two weeks after onset of smoltification.
Smolt index score did not differ among the dietary groups (Figure 6), except for parr marks score at week 8, where Control presented a significantly lower score 4 when compared to Low fat and High fat (P-value = 0.020). A maximum smolt appearance, i.e., score 4, was observed at week 6 for silver colour (Figure 6A) and week 14 for both parr marks absence (Figure 6B) and black edges of the caudal fin (Figure 6C).
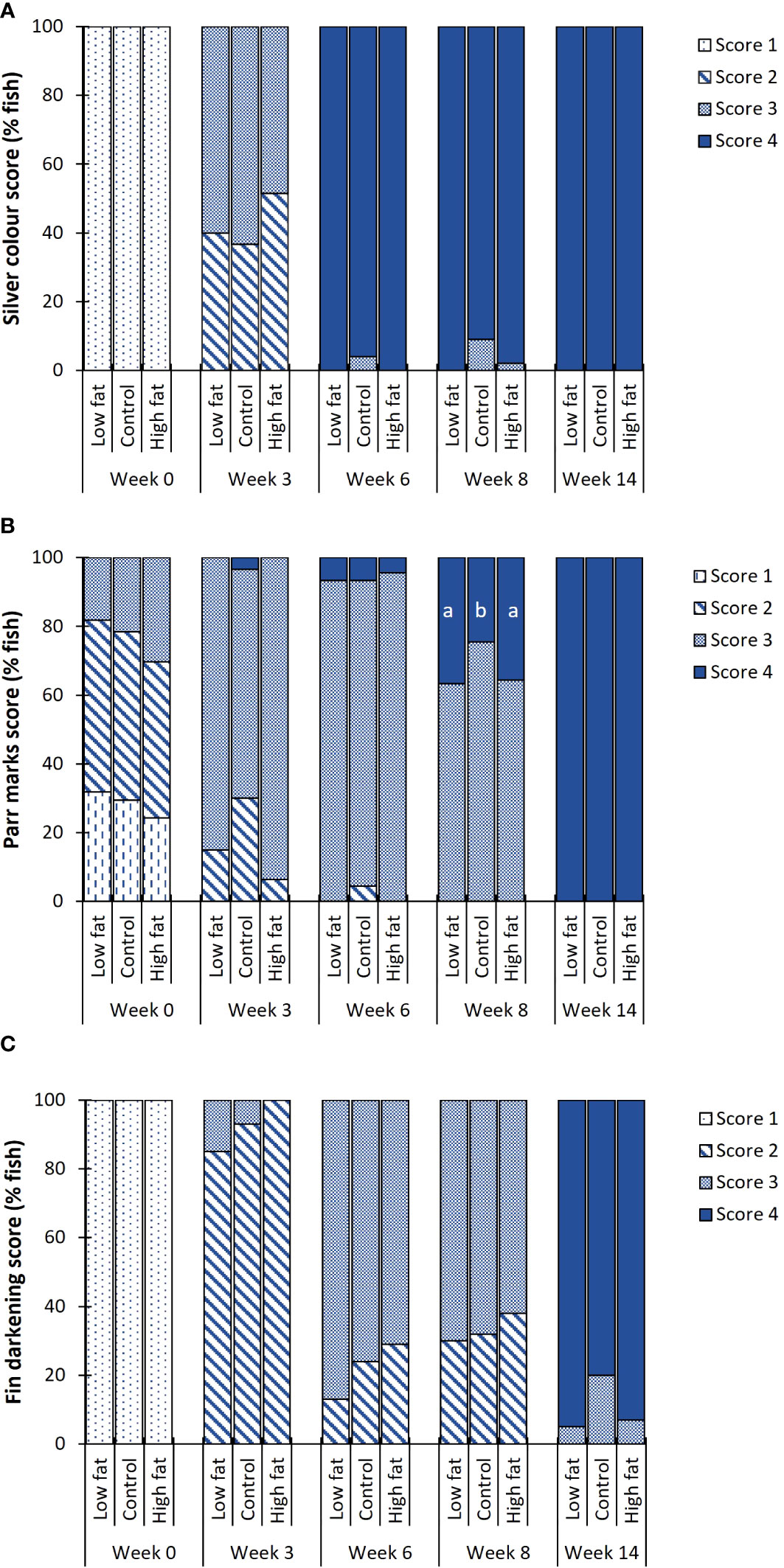
Figure 6 Fish external smoltification index score (% of fish) during the Freshwater RAS phase. (A) Body silvering (1-absent to 4-silver), (B) presence of parr marks (1-solid marks to 4-absent marks) and (C) darkening of the distal edge of the caudal fin (1-absent to 4-black edges). Values are given as treatment group mean (N = 3, except N = 2 for Low fat group). Superscript alphabets indicate significant differences among diet groups (P-value< 0.05).
3.4 Sexual maturation indicators - seawater FTS phase
At the end of the Seawater FTS phase (week 26), no differences were observed among the dietary groups regarding blood serum sex steroid levels of sampled males (Figures 7A–C). In contrast, with respect to temperature androstenedione (A4) levels were significantly higher (P-value = 0.041) in the 16°C groups (0.6 – 24.7 ng/ml) compared to 12°C groups (0.1 – 11.4 ng/ml), while testosterone (0.0 – 3.1 ng/ml) and 11-ketotestosterone (0.0 – 1.9 ng/ml) concentrations did not differ.
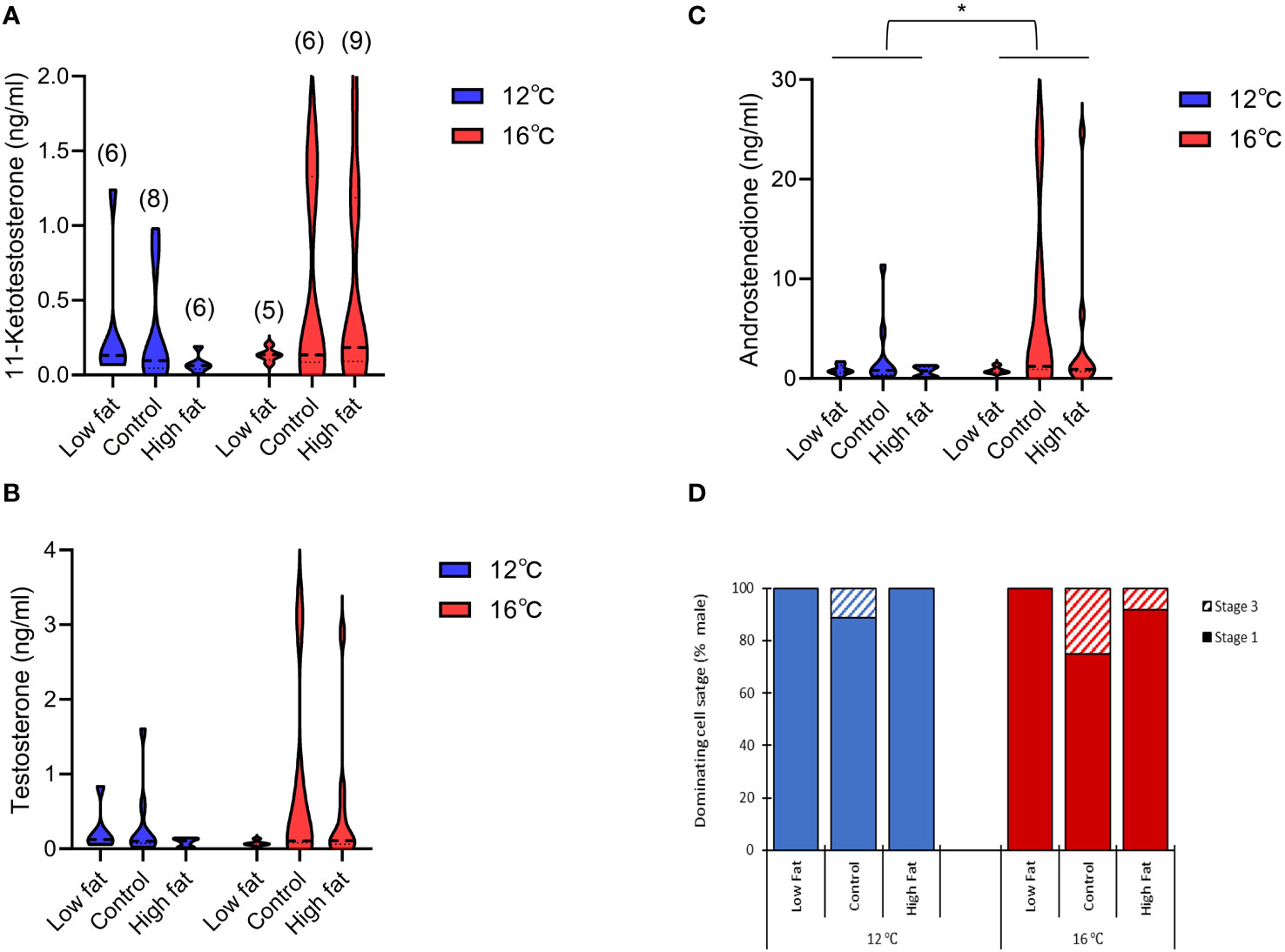
Figure 7 The violin plots of the blood sex steroid levels (ng/ml) of Atlantic salmon males at the end of the Seawater FTS phase (week 26): (A) Testosterone, (B) 11-ketotestosterone and (C) Androstenedione. The thick dashed line represents the median value and the thin dashed line represent quartiles. (D) The histogram of the dominating cell stage (% males). The number in brackets shown the n of males for each group. * Indicates significant differences between temperature groups (P-value< 0.05).
Histological analysis of the testis showed no differences in dominating cell stage between diet or temperature at the end of the Seawater FTS phase (week 26). Overall, the testis scored stage I with some individuals in Control (12 and 16°C) and High fat (16°C only) groups scoring stage III – maturing testis (Figure 7D).
3.5 Water quality
The results of the water quality parameters measured during the two experimental phases are summarized in Table 6. Most of the measured and calculated parameters did not significantly differ among the dietary treatments, with the exception of NH4-N/NH3-N and NO2-N at Freshwater RAS phase and temperature at Seawater FTS phase. The High fat group presented significantly lower NH4-N and NH3-N compared to the Control and Low fat groups, and significantly lower NO2-N compared to Low fat group.
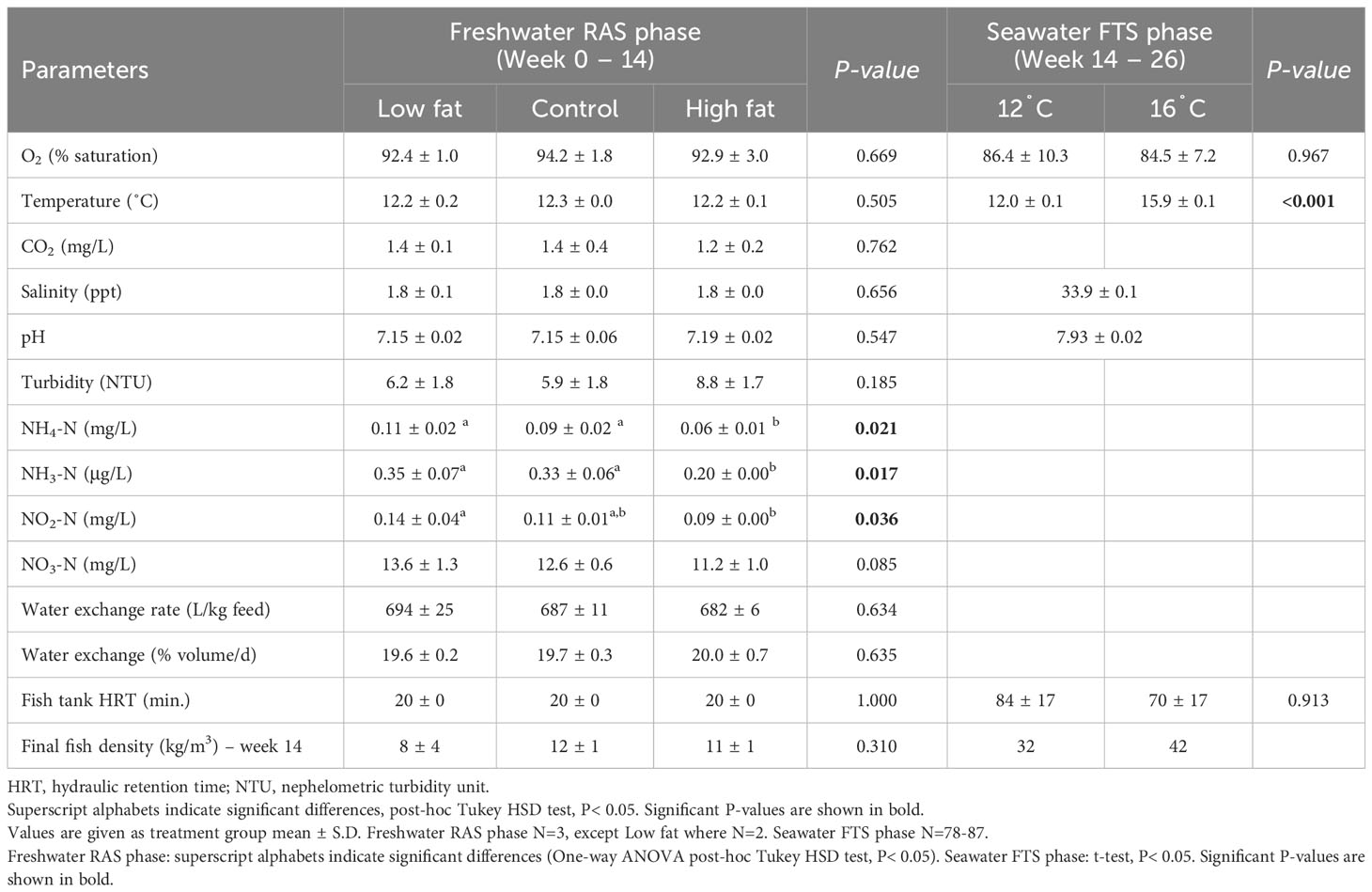
Table 6 Summary of water quality parameters measured at the fish tank effluent throughout the 26-week experimental period.
4 Discussion
Continuous feeding, constant light and relatively high temperatures applied in current day RAS facilities to produce smolts may interfere with smoltification and promote precocious maturation. In the present study, we investigated the influence of different dietary fat levels (Low fat 20%, Control 24% and High fat 28%) on smoltification in RAS, and subsequently, their long-term effects on outgrowth and sexual maturation in seawater FTS. It was found that k-factor, whole-body fat and fatty acids composition differed significantly among groups, whereas growth, NKA activity, Cl- and smoltification indicators were unaffected by the diets.
Testes histology showed most testes as immature (stage I) with some testes maturing (stage III) without clear effect of diets or temperature. However, fish body weight and sex steroid androstenedione were positively affected by temperature but not by diets at the end of the Seawater FTS phase.
Nutrient requirements studies have shown that high lipid diets increase growth and improve feed utilization in adult Atlantic salmon. Consequently lipid content of commercial Atlantic salmon diets have increased from about 17% to 35% (Torstensen et al., 2001). Interestingly the origin of the lipids, i.e., fish oil, plant oil (rapeseed) or algae oil (Schizochytrium sp.) in balanced diets seems to not affect Atlantic salmon performance, feed intake, gut health, histology, welfare, pigmentation, or fillet quality (Zatti et al., 2023). However, in parr-smolt Atlantic salmon fed ad libitum, dietary lipid inclusion has been found to have no or only minor effects on fish growth, although it was found to influence fish whole body fat levels (Grisdale-Helland and Helland, 1997; Berrill et al., 2004). In the current study, parr-smolt were fed ad libitum with three diets with fat levels ranging from 20 to 28% during the freshwater-RAS phase. Similarly, to the previous studies (Berrill et al., 2004) growth rate did not significantly differ among the diet treatments, however, the lower fat diet resulted in smolt with a significantly lower whole-body fat and lower k-factor. Reduction in body fat can result from lipolysis during smoltification, a high energy demanding process (Helland and Grisdale-Helland, 1998), which can be visible in a lower condition factor of smolts when compared to parr. In the current study, it is likely that the lower fat diet could not replenish the body lipid reserves that were being recruited for the smoltification process, which resulted in a significantly lower whole-body fat level and k-factor compared to the other two diets at the end of freshwater-RAS phase. All diet groups showed similar whole-body fat at the end of the seawater-FTS phase, regardless the earlier phase differences. This finding suggests that smolts that were initially low on body fat level were able to regain the fat level and thereafter maintain it similarly to the other two diet groups at a maximum lipid load determined by a lipostatic mechanism as it has been postulated previously for salmonids (Jobling and Johansen, 1999). Yet, feed intake was not monitored in this study, and possible differences in energy intake due to different feeding activity cannot be ruled out.
Temperature was previously shown to influence fat accumulation, where lower temperatures (5/6°C vs 12°C) result in a higher accumulation, namely in the liver (Ruyter et al., 2006; Sissener et al., 2017). Low temperatures are suggested to lead to an accumulation of fat in the liver, probably due to a selective deposition (Ruyter et al., 2006) and reduced activity of enzymes (Sissener et al., 2017). These mechanisms seem to be reduced or ceased at temperatures above 10°C (Liland et al., 2013; Sissener et al., 2017), which may justify the current study results, where it was found that at the end of the seawater phase post-smolt grown at 16°C had a significantly higher whole-body fat when compared to fish grown at 12°C. Concurrently higher temperature also resulted in post-smolts being 36% heavier than 12°C grown fish. Previous studies found that Atlantic salmon appetite increases with increasing water temperatures (Imsland et al., 2014; Martinez et al., 2022) resulting in larger and fatter fish when water temperatures are on the high end of the optimum range. The whole-body fish FA composition had some minor differences among groups, but the total FA classes, i.e., SFA, MUFA and omega-3, were not different among the three diet groups. These results suggest that despite different fat level in the diets during the freshwater phase, the content in FAs likely was within the fish requirements, and therefore no major differences were observed at the fish body composition level.
Dietary effects on smoltification have been studied in fish fed different diets through the development from parr to smolt (Helland and Grisdale-Helland, 1998; Berrill et al., 2004). However, high-energy diets did not seem to affect the plasma ion (Cl- and Na+) regulation during seawater challenge (Redell et al., 1988). Another study found that variations in protein-to-energy ratio did not affect plasma ion (Cl-), K-factor or whole-body nutrient levels, but increasing dietary lipid level increased K-factor and Hepato-somatic index (Nordgarden et al., 2002). Berrill et al. (2004) studied the influence of dietary lipid inclusion during freshwater production of parr-smolt and found that fish whole body lipid composition increases in high dietary lipid inclusion but fish smoltification markers, such as NKA activity and condition factor, were not affected by dietary lipid levels. However, the previous studies only followed the fish development until approximately 40g and the current production practices, smoltification protocols and freshwater production systems have changed considerably since these two studies, with the adoption of RAS and larger smolt production. For example, a study comparing the performance of Atlantic salmon smolt reared in RAS and FTS found branchial gene expression of key transporters (e.g. NKA) significantly different in RAS raised fish relative to fish from the FTS at the seawater transfer (Kolarevic et al., 2014). In the current study, fish were smoltified and grown in RAS units until approximately 90g and most smoltification markers measured indicate that fish had smoltified independently of their fat diet regime. The smolt status markers gill NKA activity, serum Cl- response to a seawater challenge and smolt index score did not differ in response to different fat dietary levels. The exception was a lower k-factor on the Low Fat diet group. The reduction of the k-factor is a typical result of the increase length growth relative to body mass during smoltification (Mccormick et al., 2013), whether this observation in the Low Fat group is a result of the lower diet fat content or a sign of strong smoltification is not clear.
In the seawater phase of Atlantic salmon aquaculture average mortality is 16-17% (Sommerset et al., 2021). A high proportion of this mortality occurs the first months after smolt have been transferred to sea cages. A recent study investigated if parr fed diets varying in amino acids, methionine, and B-vitamins during the freshwater stage could improve smolt growth performance and survival in the seawater stage (Sissener et al., 2021). These authors found no positive effects on the robustness, survival and growth of the tested dietary improvements compared to the control diet (similar to a commercial feed), indicating that the control diet likely contained all necessary nutrients. Similarly, in the current study fish fed during the freshwater to diets varying on fat composition performed equally well in the seawater phase, with no mortality, similar growth rate and final whole body fat composition. These findings support earlier findings that poor performance after seawater transfer may not be the result of current commercial diets.
Maturation in Atlantic salmon is known to be influenced by factors such as photoperiod, water temperature, exercise, genetic strain, rearing water quality and feed, with water temperature and photoperiod appearing to be the most critical factors for the decision to start of delay maturation (Good and Davidson, 2016). Imsland et al. (2014) suggested that photoperiod is the main signal for the start of maturation, whereas it is temperature that controls the magnitude of the photoperiod effect. For example, a recent study comparing two rearing temperatures has found that Atlantic salmon had similar production performance but the prevalence of maturation was lower at the 12°C compared to 14°C (Crouse et al., 2022). Similarly, Martinez et al. (2022) found that Atlantic salmon fed to satiation grown at 18 °C from 23 g to 600 g resulted in almost 100% maturation in contrast to 12.5 °C and 8°C, that resulted in 40% and 0% maturing males, respectively, and lowed final body weight (200 – 400g). In the present study, no differences in male precocious maturation were observed among the dietary groups, assessed by blood serum sex steroid levels or histological analysis of the testis. In contrast, males reared at 16°C resulted in higher level of the sex steroids androstenedione compared to 12°C. Androstenedione serves as an intermediate in the biosynthesis of for example 11-ketotestosterone. However, 11-ketotestosterone and testosterone levels did not differ, despite a trend for higher levels at 16°C. Further, testis histology at the final sampling (week 26) indicate that some individuals showed initial signs of maturation (testis cell stage 3), although no clear differences were found between groups with respect to diet or temperature. The fish body weight in the 16°C groups was significantly larger by 36% week 26 compared to groups grown 12°C, 601.7- 636.7g and 416.1-501.7g respectively. Other studies where fish grew larger, the maturation of Atlantic salmon males seemed to appear more evident when the fish reached a body weight of 851-908 g (Crouse et al., 2022) and ~1000 g (Skjold et al. submitted). One could speculate whether the fish grown at 16°C for a longer period in the current study would have resulted in a clear sign of early sex maturation in males. This topic should be further studied for Atlantic salmon since early sex maturation incidence is recurrent in commercial conditions and poses important economic losses.
5 Conclusion
The current study showed no effects on smoltification, seawater performance and maturation of a low (20%) and high (28%) diet fat levels compared to a control (24%) fat diet (similar to a commercial feed). In contrast, whole-body fat and FA composition differed among dietary groups in the Freshwater RAS phase. Moreover, fish grown in a seawater FTS at 12 or 16°C for 12-weeks until approximately 416 - 637g did not result in a difference in maturation in the male gonad histology evaluation, despite some maturing testes. However, the sex steroid androstenedione levels may indicate an onset of maturation of some fish culture at 16°C. Overall, these results indicate that no positive effects may arise from changing modern diets fat levels on smoltification, seawater performance and maturation, but this study’s results also show that it may not be advisable to culture Atlantic salmon at high temperatures due to the risk of male early sex maturation.
Data availability statement
The raw data supporting the conclusions of this article will be made available by the authors, without undue reservation.
Ethics statement
The animal study was approved by the Norwegian Food Safety Authority (FOTS) under general husbandry practices and ID24383. The study was conducted in accordance with the local legislation and institutional requirements.
Author contributions
VM: Conceptualization, Data curation, Formal analysis, Funding acquisition, Investigation, Methodology, Project administration, Software, Writing – original draft, Resources, Writing – review & editing. GV: Data curation, Investigation, Methodology, Writing – review & editing. AS: Conceptualization, Data curation, Investigation, Methodology, Writing – original draft, Writing – review & editing. EL: Conceptualization, Data curation, Investigation, Methodology, Writing – review & editing. JD: Conceptualization, Writing – review & editing, Methodology. LS: Conceptualization, Data curation, Formal analysis, Investigation, Methodology, Writing – original draft, Writing – review & editing. EB: Conceptualization, Data curation, Formal analysis, Funding acquisition, Investigation, Methodology, Project administration, Writing – original draft, Writing – review & editing. MB: Conceptualization, Data curation, Investigation, Methodology, Writing – original draft, Writing – review & editing.
Funding
The author(s) declare financial support was received for the research, authorship, and/or publication of this article. This work was funded by The Research Council of Norway, Strategic Institute Research Nofima AS, project nr 12878 “Atlantic salmon biology in focus: for a robust fish in a shifting aquaculture industry”.
Acknowledgments
The authors would like to thank Jan-Eirik Jensen, Rune Olsen, Morten Marienborg and Astrid-Elisabeth Chr. Hanssen at the Tromsø Aquaculture research station (Havbruksstasjonen i Tromsø AS) for support with the fish experimental trial. Thanks to Odd Helge Romarheim for the formulation of the experimental diets and Gunhild Johansson, Hanne Brenne, Ragnhild Berg for the sampling of fish tissues and laboratory analysis at Nofima AS. Thanks to Sietske Grijseels, Ole Martin Fuskevåg, Giovanni Allaoui for help with the sex hormone analysis at PRiME, the Proteomics and Metabolomic Core Facility.
Conflict of interest
The authors declare that the research was conducted in the absence of any commercial or financial relationships that could be construed as a potential conflict of interest.
Publisher’s note
All claims expressed in this article are solely those of the authors and do not necessarily represent those of their affiliated organizations, or those of the publisher, the editors and the reviewers. Any product that may be evaluated in this article, or claim that may be made by its manufacturer, is not guaranteed or endorsed by the publisher.
References
Bendiksen E., Arnesen A., Jobling M. (2003). Effects of dietary fatty acid profile and fat content on smolting and seawater performance in Atlantic salmon (Salmo salar L.). Aquaculture 225, 149–163. doi: 10.1016/S0044-8486(03)00286-2
Bergheim A., Drengstig A., Ulgenes Y., Fivelstad S. (2009). Production of Atlantic salmon smolts in Europe—current characteristics and future trends. Aquacult. Eng. 41, 46–52. doi: 10.1016/j.aquaeng.2009.04.004
Berrill I. K., Porter M. J., Bromage N. R. (2004). The influence of dietary lipid inclusion and daily ration on growth and smoltification in 1+ Atlantic salmon (Salmo salar) parr. Aquaculture 242, 513–528. doi: 10.1016/j.aquaculture.2004.08.029
Bou M., Berge G. M., Baeverfjord G., Sigholt T., Østbye T.-K., Romarheim O. H., et al. (2017). Requirements of n-3 very long-chain PUFA in Atlantic salmon (Salmo salar L): effects of different dietary levels of EPA and DHA on fish performance and tissue composition and integrity. Br. J. Nutr. 117, 30–47. doi: 10.1017/S0007114516004396
Ciani E., Von Krogh K., Nourizadeh-Lillabadi R., Mayer I., Fontaine R., Weltzien F.-A. (2021). Sexual maturation in Atlantic salmon male parr may be triggered both in late summer and early spring under standard farming conditions. Aquaculture 544, 737086. doi: 10.1016/j.aquaculture.2021.737086
Crouse C., Davidson J., Good C. (2022). The effects of two water temperature regimes on Atlantic salmon (Salmo salar) growth performance and maturation in freshwater recirculating aquaculture systems. Aquaculture 553, 738063. doi: 10.1016/j.aquaculture.2022.738063
Fjelldal P. G., Schulz R., Nilsen T. O., Andersson E., Norberg B., Hansen T. J. (2018). Sexual maturation and smoltification in domesticated Atlantic salmon (Salmo salar L.)–is there a developmental conflict? Physiol. Rep. 6, e13809. doi: 10.14814/phy2.13809
Folch J., Lees M., Sloane Stanley G. H. (1957). A simple method for the isolation and purification of total lipids from animal tissues. J. Biol. Chem. 226, 497–509. doi: 10.1016/S0021-9258(18)64849-5
Good C., Davidson J. (2016). A review of factors influencing maturation of Atlantic salmon, Salmo salar, with focus on water recirculation aquaculture system environments. J. World Aquac. Soc 47, 605–632. doi: 10.1111/jwas.12342
Grisdale-Helland B., Helland S. (1997). Replacement of protein by fat and carbohydrate in diets for Atlantic salmon (Salmo salar) at the end of the freshwater stage. Aquaculture 152, 167–180. doi: 10.1016/S0044-8486(97)00003-3
Helland S., Grisdale-Helland B. (1998). The influence of replacing fish meal in the diet with fish oil on growth, feed utilization and body composition of Atlantic salmon (Salmo salar) during the smoltification period. Aquaculture 162, 1–10. doi: 10.1016/S0044-8486(98)00206-3
Imsland A. K., Handeland S. O., Stefansson S. O. (2014). Photoperiod and temperature effects on growth and maturation of pre-and post-smolt Atlantic salmon. Aquac. Int. 22, 1331–1345. doi: 10.1007/s10499-014-9750-1
Jobling M. (2012). National Research Council (NRC): Nutrient requirements of fish and shrimp. Aquac. Int. 20, 601–602. doi: 10.1007/s10499-011-9480-6
Jobling M., Johansen S. (1999). The lipostat, hyperphagia and catch-up growth. Aquac. Res. 30, 473–478. doi: 10.1046/j.1365-2109.1999.00358.x
Kolarevic J., Baeverfjord G., Takle H., Ytteborg E., Reiten B. K. M., Nergård S., et al. (2014). Performance and welfare of Atlantic salmon smolt reared in recirculating or flow through aquaculture systems. Aquaculture 432, 15–25. doi: 10.1016/j.aquaculture.2014.03.033
Liland N. S., Espe M., Rosenlund G., Waagbø R., Hjelle J. I., Lie Ø., et al. (2013). High levels of dietary phytosterols affect lipid metabolism and increase liver and plasma TAG in Atlantic salmon (Salmo salar L.). Br. J. Nutr. 110, 1958–1967. doi: 10.1017/S0007114513001347
Martinez E. P., Balseiro P., Stefansson S. O., Kaneko N., Norberg B., Fleming M. S., et al. (2022). Interaction of temperature and feed ration on male postsmolt maturation of Atlantic salmon (Salmo salar L.). Aquaculture 562, 738877. doi: 10.1016/j.aquaculture.2022.738877
Mason M. E., Waller G. R. (1964). Dimethoxypropane induced transesterification of fats and oils in preparation of methyl esters for gas chromatographic analysis. Anal. Chem. 36, 583–586. doi: 10.1021/ac60209a008
Mcclure C. A., Hammell K. L., Moore M., Dohoo I. R., Burnley H. (2007). Risk factors for early sexual maturation in Atlantic salmon in seawater farms in New Brunswick and Nova Scotia, Canada. Aquaculture 272, 370–379. doi: 10.1016/j.aquaculture.2007.08.039
Mccormick S. D. (1993). Methods for nonlethal gill biopsy and measurement of Na+, K+-ATPase activity. Can. J. Fish. Aquat. Sci. 50, 656–658. doi: 10.1139/f93-075
Mccormick S., Björnsson B. T., Sheridan M., Eilerlson C., Carey J., O'dea M. (1995). Increased daylength stimulates plasma growth hormone and gill Na+, K+-ATPase in Atlantic salmon (Salmo salar). J. Comp. Physiol. B. 165, 245–254. doi: 10.1007/BF00367308
Mccormick S. D., Regish A. M., Christensen A. K., Björnsson B. T. (2013). Differential regulation of sodium–potassium pump isoforms during smolt development and seawater exposure of Atlantic salmon. J. Exp. Biol. 216, 1142–1151. doi: 10.1242/jeb.080440
Mccormick S. D., Shrimpton J. M., Moriyama S., Björnsson B. T. (2007). Differential hormonal responses of Atlantic salmon parr and smolt to increased daylength: a possible developmental basis for smolting. Aquaculture 273, 337–344. doi: 10.1016/j.aquaculture.2007.10.015
Melo M. C., Andersson E., Fjelldal P. G., Bogerd J., França L. R., Taranger G. L., et al. (2014). Salinity and photoperiod modulate pubertal development in Atlantic salmon (Salmo salar). J. Endocrinol. 220, 1–15. doi: 10.1530/JOE-13-0240
Mobley K. B., Aykanat T., Czorlich Y., House A., Kurko J., Miettinen A., et al. (2021). Maturation in Atlantic salmon (Salmo salar, Salmonidae): a synthesis of ecological, genetic, and molecular processes. Rev. Fish Biol. Fish. 31, 523–571. doi: 10.1007/s11160-021-09656-w
Mota V. C., Striberny A., Verstege G. C., Difford G. F., Lazado C. C. (2022). Evaluation of a recirculating aquaculture system research facility designed to address current knowledge needs in Atlantic salmon production. Front. Anim. Sci. 26. doi: 10.3389/fanim.2022.876504
Nordgarden U., Hemre G.-I., Hansen T. (2002). Growth and body composition of Atlantic salmon (Salmo salar L.) parr and smolt fed diets varying in protein and lipid contents. Aquaculture 207, 65–78. doi: 10.1016/S0044-8486(01)00750-5
Redell L. A., Rottiers D. V., Lemm C. A. (1988). Lack of dietary effects on the timing of smoltification in Atlantic salmon. Prog. fish. c. 50, 7–11. doi: 10.1577/1548-8640(1988)050<0007:ALODEO>2.3.CO;2
Ruyter B., Moya-Falcón C., Rosenlund G., Vegusdal A. (2006). Fat content and morphology of liver and intestine of Atlantic salmon (Salmo salar): effects of temperature and dietary soybean oil. Aquaculture 252, 441–452. doi: 10.1016/j.aquaculture.2005.07.014
Sheridan M. A., Kao Y.-H. (1998). Regulation of metamorphosis-associated changes in the lipid metabolism of selected vertebrates. Am. Zoologist 38, 350–368. doi: 10.1093/icb/38.2.350
Sissener N., Hamre K., Fjelldal P., Philip A., Espe M., Miao L., et al. (2021). Can improved nutrition for Atlantic salmon in freshwater increase fish robustness, survival and growth after seawater transfer? Aquaculture 542, 736852. doi: 10.1016/j.aquaculture.2021.736852
Sissener N., Torstensen B., Owen M., Liland N., Stubhaug I., Rosenlund G. (2017). Temperature modulates liver lipid accumulation in Atlantic salmon (Salmo salar L.) fed low dietary levels of long-chain n-3 fatty acids. Aquac. Nutr. 23, 865–878. doi: 10.1111/anu.12453
Sommerset I., Jensen B. B., Bornø G., Haukaas A., Brun E. (2021). Fiskehelserapporten 2020 (Technical Rapport 41a/2021, in Norwegian with Abstract in English). Norwegian Veterinary Institute. Available at: https://www.vetinst.no/rapporter-og-publikasjoner/rapporter/2021/fiskehelserapporten-2020 (accessed on 18 January 2024).
Torstensen B., Lie Ø., Hamre K. (2001). A factorial experimental design for investigation of effects of dietary lipid content and pro-and antioxidants on lipid composition in Atlantic salmon (Salmo salar) tissues and lipoproteins. Aquac. Nutr. 7, 265–276. doi: 10.1046/j.1365-2095.2001.00184.x
Ytrestøyl T., Hjelle E., Kolarevic J., Takle H., Rebl A., Afanasyev S., et al. (2022). Photoperiod in recirculation aquaculture systems and timing of seawater transfer affect seawater growth performance of Atlantic salmon (Salmo salar). J. World Aquac. Soc. 53, 73–95. doi: 10.1111/jwas.12880
Keywords: smoltification, early sexual maturation, seawater performance, dietary fat levels, temperature, recirculating aquaculture systems (RAS)
Citation: Mota VC, Verstege GC, Striberny A, Lutfi E, Dessen J-E, Sveen L, Burgerhout E and Bou M (2024) Smoltification, seawater performance, and maturation in Atlantic salmon (Salmo salar) fed different fat levels. Front. Aquac. 3:1323818. doi: 10.3389/faquc.2024.1323818
Received: 18 October 2023; Accepted: 12 January 2024;
Published: 25 January 2024.
Edited by:
Tiago Hori, Atlantic Aqua Farms Ltd, CanadaReviewed by:
Daniel Matulić, University of Zagreb, CroatiaPeggy Biga, University of Alabama at Birmingham, United States
Copyright © 2024 Mota, Verstege, Striberny, Lutfi, Dessen, Sveen, Burgerhout and Bou. This is an open-access article distributed under the terms of the Creative Commons Attribution License (CC BY). The use, distribution or reproduction in other forums is permitted, provided the original author(s) and the copyright owner(s) are credited and that the original publication in this journal is cited, in accordance with accepted academic practice. No use, distribution or reproduction is permitted which does not comply with these terms.
*Correspondence: Vasco C. Mota, dmFzY28ubW90YUBubWJ1Lm5v