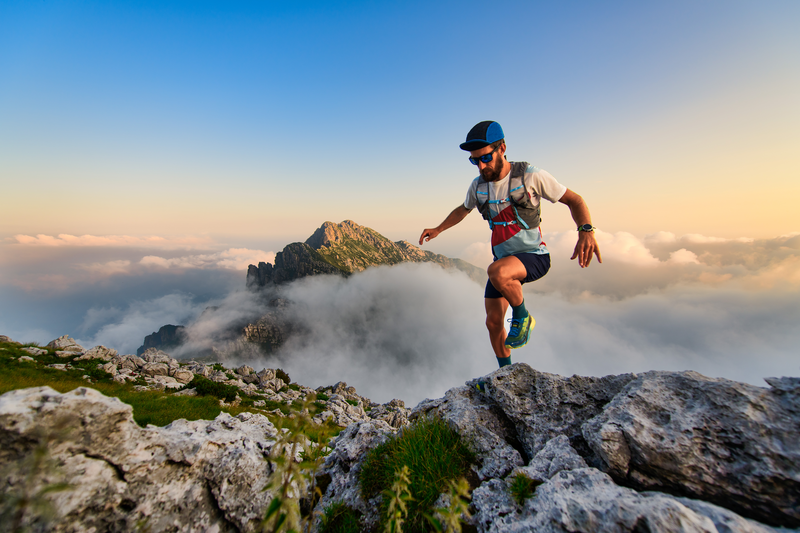
94% of researchers rate our articles as excellent or good
Learn more about the work of our research integrity team to safeguard the quality of each article we publish.
Find out more
ORIGINAL RESEARCH article
Front. Aquac. , 13 February 2024
Sec. Production Biology
Volume 3 - 2024 | https://doi.org/10.3389/faquc.2024.1310429
High larval and fry mortality has been a major challenge to the commercial culture of the African bony-tongue (Heterotis niloticus). Research indicates inadequate feeding to be a possible cause of the high mortality rates of larvae/fry grown in captivity. An experiment was conducted to first describe the developmental stages of H. niloticus eggs and larvae until schooling, 6 days after hatching (DAH), at 26°C. The morphological development, survival, growth (weight gain and length), and swimming behavior of the larvae were monitored. A follow-up feeding trial was conducted with H. niloticus fry from 6 to 27 DAH involving four treatments: feeding with Artemia nauplii; a combined feed of 50% Artemia nauplii and 50% rotifers (w/w); feeding with rotifers; and no feeding. The activities of three digestive enzymes (trypsin, lipase, and amylase) were assessed in fry exposed to the live feed treatments. At hatching, the larvae had a large, vascularized yolk sac filled with yolk platelets, which occupied approximately one-third of the total body length of the abdominal cavity. Yolk platelet reabsorption started at 1 DAH and was complete at 6 DAH. Metamorphosis was completed at 6 DAH, and schooling behavior was observed. For the feeding trial, the fry that received Artemia nauplii underwent the fastest development until 27 DAH. Similarly, the survival rate was considerably higher in fry fed with Artemia nauplii than in those that received a combination of Artemia nauplii and rotifers or only rotifers. Overall, the survival rates for fry fed with Artemia nauplii and larvae co-fed were 72.7% ± 0.9% and 41.2% ± 3.3%, respectively. Fry did not survive until 27 DAH in the rotifer-fed and unfed treatment groups. Digestive enzyme activity differed significantly (p< 0.05) between treatments and sampling dates. All digestive enzymes were detectable at the onset of mouth opening (5 DAH) and fluctuated through the 7 and 9 DAH. Lower enzyme activity was observed when the fry were subjected to starvation or the rotifer treatment. Results indicate that Artemia nauplii is the best live feed organism for H. niloticus larviculture.
The African bony-tongue, Heterotis niloticus, is a freshwater species widely distributed in river basins and freshwater lakes in Sub-Saharan Africa (Moreau, 1982; Levêque et al., 1990) and belongs to the order Osteoglossiformes under the subfamily Arapaiminae (Ferraris, 2003). The potential of the species as suitable for aquaculture was noted in the early 1950s. It constitutes an important component of inland fisheries and is considered an important and highly prized fish by consumers, thus forming a very important component of the catch of fishermen (Frimpong et al., 2011; Akpaglo, 2012). This species is in high demand on the African market and offers vast potential for fish farming because of several advantages, such as its high growth rate, omnivorous diet, large size of up to 5 kg, and high acceptability to consumers (Monentcham et al., 2010). The culture potential of a species depends on several aspects, including ease of fingerling production and thus availability to supply fish culture facilities (Rønnestad et al., 2001; Adite et al., 2005; Adite et al., 2006; Monentcham et al., 2009). However, efforts by fish farmers at captive breeding of H. niloticus have shown marginal success, and farmers mostly resort to obtaining wild fingerlings from fishermen to stock their ponds in polyculture with tilapia and Clarias (Akpaglo, 2012). The rearing of larvae has thus typically resulted in very high mortality rates of 80% to 100% (Moreau, 1982). It has been observed that within a short period of between 5 and 7 days after hatching (DAH), the whole population may die (Reizer, 1964, 1968; Vincke, 1971). Rakotomanampisson (1966) and De Kimpe (1967) also found high mortality rates of between 96% and 82% in various studies on the rearing of H. niloticus larvae. For higher survival rates in most larval species, feeding must be initiated before or soon after depletion of the endogenous energy sources and yolk sac (Kim et al., 2001). Studies have shown that African bony-tongue of all sizes are microphagous or omnivorous. As a result, they eat a variety of foods, from aquatic invertebrates to small seeds, including macrophytes, plant remains, aquatic insects, and fish (Micha, 1973; Moreau, 1982; Mbega, 2004; Adite et al., 2005). In addition, rotifer species have also been observed in their natural habitat based on examinations of samplings of prey items in and around wild nests in the Barekese area in Ghana (author’s personal observations).
Live feed is generally considered the most suitable feed for the first feeding of most fish larvae (Conceição et al., 2010), as this provides energy for growth and physiological functioning (Hamre et al., 2013; Palińska-Żarska et al., 2014; Radhakrishnan et al., 2020). Common live feeds used in the cultivation of finfish and crustaceans include rotifers (Brachionus spp.) and brine shrimp (Artemia spp.) because of their rich nutrient profile, as well as the fact that they are constantly reachable by the larvae and easily digested and assimilated by the larvae (Aragão et al., 2004; Damle and Chari, 2011). The saltwater rotifer Brachionus plicatilis, the freshwater rotifer Brachionus calyciflorus, and unicellular organisms such as Paramecium sp. are used as starter feeds and are subsequently replaced by brine shrimp, Artemia nauplii, at more advanced larval stages (Dhont et al., 2013; Pan et al., 2022). The availability of these live feeds in hatcheries has contributed to the successful fry production of at least 60 marine finfish species and 18 species of crustaceans due to the presence of necessary nutrients, including essential proteins, lipids, carbohydrates, vitamins, minerals, amino acids, and fatty acids. However, the provision of appropriate live food at a proper time plays a major role in achieving maximum growth and survival of juvenile finfish and shellfish (Dhert, 1996; Das et al., 2012).
Feeding larvae at the early stages with an artificial diet has been associated with increased mortality and reduced development rates, despite its apparent economic benefits. Furthermore, no dependable recipes for larval diet formulation are available as yet (Lahnsteiner et al., 2023). The lower growth in fish larvae fed with compound diets is associated with low acceptance and attractiveness, inadequate digestion, and poor assimilation compared with live diets (Pan et al., 2022). Although total replacement of live food with a formulated diet is not possible for the larvae of many species, partial replacement of live food with a formulated diet, which is known as co-feeding, may be possible (Hamre et al., 2013).
At the start of external feeding, the digestive system of teleost larvae is undifferentiated (Govoni et al., 1986; Cahu and Zambonino-Infante, 2001). Furthermore, enzyme activity is generally low at the beginning of external feeding and is influenced by the food type provided (Infante and Cahu, 2001). Studies have shown that the basic morphology of the digestive tract of most larval/fry stages coincides with low production of enzymes, hence the requirement for live feed organisms as a source of nutrition (Dabrowski, 1979; Srivastava et al., 2002). The study of the digestive specificities of early larval/fry stages of most fish species is critical in enabling adaption of the nutritional profile and the form in which these nutrients are supplied in a formulated micro diet (Ma et al., 2005). The assessment of the presence and level of activity of certain enzymes is used as a comparative indicator of the rate of development of the larvae and survival rate (Ueberschär, 1993). However, very little is known about the digestive enzyme activity of H. niloticus larvae during their early stages of development. The objectives of this study were first to obtain information on the early morphological development of H. niloticus larvae until 6 DAH in order to determine the optimal time of first feeding, and second to assess the effect of starvation or the provision of different live feed species on larval/fry survival, growth, and digestive enzyme activity. For this study, larvae and fry were fed with Artemia nauplii, a combination of the two live feeds, or rotifers only from 6 to 27 DAH.
Two batches of fertilized eggs and larvae were obtained for the experiments: the first batch was obtained from a pond at a private fish farm and the second batch from the Barekese reservoir in the Ashanti Region, Ghana. The experiments were conducted at the wet laboratory of the Department of Fisheries and Watershed Management, Kwame Nkrumah University of Science and Technology (KNUST), Ghana.
Fertilized eggs of H. niloticus obtained from the breeding nest in a pond at a private farm were transported to the laboratory, where hatching was completed after 24 hours. The newly hatched larvae (9.05 ± 0.09 mm; 12.33 ± 0.6 mg) were counted volumetrically and stocked in triplicate at a density of 17 larvae/L in three transparent 25-L rectangular plastic tanks with 20 L of water. Approximately 50% of the water was replaced daily by siphoning off the waste at the bottom of the tank. The larvae were reared under a constant photoperiod of 12:12 h of light/dark and at 26°C during the 6-day experimental period.
For morphometric analysis, 10 larvae were randomly sampled from each tank every morning to monitor growth and larval development from 0 to 6 DAH. Digital images of individual larvae were taken and analyzed for morphometric development using a camera microscope (LEICA MC190 HD, Leica Microsystems, Wetzlar, Germany) with a scale of 1 mm. The lengths (mm) of the larvae were measured to the nearest 0.01 mm using a digital caliper (Mitutoyo 500-196-20, Mitutoyo, Foshan, China), and body weights were measured to the nearest 0.001 g using a digital scale (UW1020H, Shimadzu, Kyoto, Japan). Samples were collected using methods similar to those described by Siju et al. (2021).
The experiment was conducted in twelve 45-L rectangular plastic tanks filled with 20 L of ground water and provided with gentle aeration to maintain dissolved oxygen at 5 mg/L throughout the experiment and to promote homogeneous distribution of the live feeds. All tanks were kept in a ventilated room under a photoperiod of 12:12 h of light/dark. Fry of H. niloticus from a natural nest at the Barekese reservoir, Ghana, with an initial weight of 12.6 ± 1.58 mg and mean total length of 12.9 ± 0.3 mm, were stocked in 12 tanks at a density of 20 larvae/L. The age of the fry was estimated to be 5 days post hatch based on comparison with H. niloticus larval ontogenic development described in the first part of the article. At this age, their anal and pectoral fins were barely initiated, and their yolk sac was almost diminished. At an age of 6 DAH, H. niloticus are considered to be fry, as they start schooling and resemble fingerlings.
Four treatments were tested in triplicate, each randomly assigned to three of the 12 plastic tanks. In treatment 1, larvae were fed Artemia franciscana nauplii only. In treatment 2, they received a combination of the two live feeds Artemia nauplii and rotifers (co-fed). In treatment 3, they were fed with live rotifers (B. calyciflorus) only. In treatment 4, they were not fed; this treatment was designated as a control. Fry in the fed treatment groups were fed four times daily at 3-hour intervals. In treatments 1 and 3, the live Artemia was added to a final density of 1–2 Artemia nauplii ind./ml (individual dry weight 0.0128 ± 0.0040 mg) and 7–10 rotifer ind./ml (individual dry weight 0.0067 ± 0.0024 mg), respectively. The co-fed treatment consisted of 50% of the density by mass of each live feed (0.5–1 Artemia ind./ml and 4–5 rotifers ind./ml).
Two strains of newly hatched Artemia nauplii were used for the study: a smaller-sized Artemia (AF, 195 × 499 µm) (Inve, Dendermonde, Belgium) and a larger-sized Artemia (EG, 270 × 540 µm) (Inve, Belgium). From 5 to 11 DAH, the fry on the Artemia treatments were fed with AF Artemia nauplii and co-fed with a mixture of AF and EG for 2 days before switching completely to EG Artemia meta-nauplii, as shown in Table 1. Both Artemia and rotifers were enriched with amplified replete algae (Chlorella sp.) at a rate of 100 ppm (1 g/L) and 200 ppm (0.2 g/L), respectively, at 8 to 12 hours before feeding.
Ten larvae/fry from each tank were randomly sampled daily for growth measurements until 11 DAH. Samplings were thereafter discontinued in the rotifer-fed and unfed groups due to total mortality but continued every fourth day for the Artemia nauplii and co-fed groups. The total length (TL) of each larva was measured to the nearest 0.01 mm using a Vernier caliper (Mitutoyo 500-196-20, Foshan, China), and wet body weight (BW) was measured to the nearest 0.001 g using an analytical balance (UW1020H, Shimadzu, Kyoto, Japan). For analysis of digestive enzyme activity, triplicate samples of nine larvae per treatment were randomly collected 2–3 hours after feeding at 5, 7, and 9 DAH. Individuals were rinsed with distilled water and frozen at −80°C until further analysis was conducted. Samples were collected using methods similar to those of Siju et al. (2021).
The growth and survival rates of the larvae were determined as follows:
Digestive enzyme activity was determined by mechanically homogenizing individual larvae/fry in ice-cold Milli-Q water (Merck Millipore, Darmstadt, Germany); this was centrifuged (10 min at 15,800 g), and the supernatant was used to assay enzymatic activity. Amylase activity was determined using a commercial kit (Ultra Amylase Assay kit E33651, Thermo Fisher Scientific, Waltham, MA, USA). Trypsin and lipase were assayed using the methods of Rotllant et al. (2008), modified as described in Goncalves et al. (2021). All enzyme activities are expressed as relative fluorescence units (RFU) per individual. In the case of larvae, the gut was too small to be dissected; hence, the procedure using whole individuals was continued in the case of the fry for purposes of comparison. The results are expressed in the form of specific enzyme activity per individual.
The tanks were cleaned by siphoning dead fry, waste, and uneaten feed twice daily, and 80% of tank water was replaced daily with fresh water. During the rearing period, the temperature (T; °C), dissolved oxygen (DO; mg/L), total dissolved solids (TDS; mg/L), and pH were recorded daily at 8 a.m. using a multiparameter probe (YSI Professional Plus 18H105806, Yellow Springs, OH, USA). Ammonia nitrogen (mg/L) was determined spectrophotometrically (DS, 1975) weekly in all rearing tanks.
All statistical analyses and graphs were performed and created using GraphPad Prism 5 statistical software. Data on survival and growth parameters were compared between Artemia-fed and co-fed treatments using unpaired t-tests. Data on growth measurement (length) were compared among all four treatments using the Kruskal–Wallis test followed by Dunn’s multiple comparisons test to compare the means. Data on digestive enzyme activity were also analyzed using one-way ANOVA followed by Tukey’s post-test. Results were considered significant at p< 0.05. All data are presented as means ± standard deviation.
A large, yellow, vascularized yolk sac filled with yolk platelets was observed in the abdominal cavity of the larvae at hatching, occupying a major portion of the larvae. The newly hatched larvae were translucent with pigmentation on the back of the yolk sac. On average, approximately 83% of the yolk reserves had been resorbed by the third day, and no yolk platelets were observed after the fourth day (SM Data Sheet 1). The mean body weight increased (~50%) from 12.33 ± 0.57 mg at hatching (0 DAH) to 18.33 ± 4.04 mg by 6 DAH (Figure 1). There were distinct changes in the morphological characteristics of the larvae during larval development from 0 to 6 DAH (SM Data Sheet 1). During the growth period, head length and tail length increased proportionally to TL, whereas pre-anal length and trunk length decreased proportionally to TL. Head length and the post-anal length increased from 14.8% and 31.7% of TL at the yolk sac stage to 20.3% and 42.1% of TL at the pre-flexion stage, respectively. In contrast, pre-anal length and trunk length decreased from 68.3% and 53.5% of TL to 57.9% and 37.6% of TL, respectively.
Figure 1 Growth (length and weight) of Heterotis niloticus from 0 to 6 days after hatching (DAH). Values are means ± SD (n = 10).
At 0–1 DAH, larvae were immobile and only wiggling at the bottom of the tank. However, at 2–3 DAH, some larvae exhibited active vertical movements toward the surface of the water before descending passively to the bottom. Fry engaged in active horizontal movements and started schooling at 6 DAH. Fry were periodically observed gasping for air at the surface of the water when moving in schools. At night and with no lights, all larvae/fry remained dispersed at the bottom of the tanks.
The highest survival and growth rates were observed in fry fed with Artemia. The overall survival rates of fry fed with Artemia and fry co-fed with Artemia and rotifers were 72.7% ± 0.9% and 41.2% ± 3.3%, respectively. Fry fed with rotifers and unfed fry experienced 100% mortality by 11 DAH. The growth data are presented in Table 2.
Table 2 Mean growth and survival rates of Heterotis niloticus larvae cultured from 5 to 27 DAH with different live feeds.
Figure 2 depicts the length increase over time for each treatment. Fry fed with Artemia and fry co-fed survived and grew until the end of the trial at 27 DAH (Figure 2). The highest recorded total length of 25.4 ± 0.5 mm was observed in the group fed with Artemia. Fry reared without food and those provided with rotifers died between 8 and 11 DAH.
Figure 2 Total length (mm ± SD, n = 10) of Heterotis niloticus fry fed with different live diets under experimental conditions.
Digestive enzyme activity (trypsin, amylase, and lipase) was detected in larvae at 5 DAH. Digestive enzyme activity differed significantly (p< 0.05) between treatments and sampling dates. Larval trypsin activity at the start of the trial (5 DAH) was 78.0 ± 25.8 RFU/mg BW for larvae fed with Artemia, 173 ± 137.43 RFU/mg BW for co-fed larvae, and 71.0 ± 49.9 RFU/mg BW for rotifer-fed larvae. From 5 to 7 DAH, enzyme activity decreased slightly for both co-fed and rotifer-fed larvae, but increased significantly for Artemia-fed larvae (146 ± 90.13 RFU/mg BW), reaching a peak 2 days after the start of feeding. There was a marked decrease in enzyme activity with increasing fry age for all treatments. The lowest levels were recorded at 9 DAH (83 ± 13.2 RFU/mg BW) for the Artemia-fed fry, 55 ± 25.9 RFU/mg BW for the co-fed fry, and 19 ± 4.1 RFU/mg BW for the rotifer-fed fry, and very low values (12 ± 3.5 RFU/mg BW) were observed for the unfed control fry, as shown in Figure 3A.
Figure 3 Enzyme activity of (A) trypsin, (B) amylase, and (C) lipase versus age (days after hatching (DAH)) for fry of Heterotis niloticus fed with different live feeds. Data points represent each replicate obtained from whole-body homogenates (N = 9). Activity is expressed as relative fluorescence units (RFU) per individual.
Amylase activity at 5 DAH was lowest for the Artemia-fed larvae (138.3 ± 19.32 RFU/mg BW) and higher for the co-fed larvae (345.5 ± 171.0 RFU/mg BW) and rotifer-fed larvae (237.9 ± 47.79 RFU/mg BW). Amylase activity increased to 266.2 ± 133.88 RFU/mg BW at 7 DAH in Artemia-fed fry, but decreased in the co-fed fry (209.1 ± 59.30 RFU/mg BW) and rotifer-fed fry (100.8 ± 52.36 RFU/mg BW). According to Figure 3B, amylase values at 7–9 DAH were affected by treatment: there were differences between Artemia-fed (173.7 ± 55.4 RFU/mg BW), co-fed (78.1 ± 36.8 RFU/mg BW), rotifer-fed (26.8 ± 38.3 RFU/mg BW), and unfed fry (60.3 ± 52.8 RFU/mg BW).
Lipase activity at DAH 5 was 105.4 ± 74.32 RFU/mg BW for Artemia-fed larvae, 185.2 ± 93.6 RFU/mg BW for co-fed larva, and 132.1 ± 108.01 RFU/mg BW for rotifer-fed larvae. Lipase activity peaked at 7 DAH for all treatments: Artemia-fed fry (320.7 ± 120.0 RFU/mg BW), co-fed fry (377.0 ± 146.5 RFU/mg BW), and rotifer-fed fry (171.0 ± 99.9 RFU/mg BW). It decreased at 9 DAH for Artemia-fed fry (211.9 ± 90.10 RFU/mg BW), co-fed fry (224.0 ± 168.9 RFU/mg BW), rotifer-fed fry (107.5 ± 48.48 RFU/mg BW), and unfed fry (68.4 ± 22.65 RFU/mg BW) at p< 0.02, as shown in Figure 3C.
The average physicochemical parameters were as follows: water temperature, 24.5°C–26.7°C; dissolved oxygen, 4.7–5.0 mg/L; TDS, 26–54 mg/L; pH, 6.0–7.45. Ammonia levels were below 0.01 mg/L (p< 0.05) throughout the trial.
In the early stages of development in most fish species, the growing embryo and newly hatched larvae receive their energy and nutrients from the yolk (Kamler and Kamler, 1992; Rønnestad et al., 1994). Similarly, H. niloticus larvae entirely use up the yolk reserve before their mouths open. According to studies performed by Peña et al. (2003), the yolk sac is endocytosed by a syncytium encircling the yolk sac, providing endogenous nutrition to the larvae. The opening of the mouth is a significant ontogenetic event in the life of a larva because it allows access to exogenous food (Gomes et al., 2010).
Significant changes occurred in the morphology of H. niloticus larvae during the early stage of development from 0 to 6 DAH. Within 6 DAH, the larvae metamorphosed to the fry stage and started schooling. H. niloticus fry exhibited improved swimming abilities at the time of exogenous feeding. These findings are consistent with those of previous studies indicating that the onset of schooling behavior has been observed coincident with or immediately after metamorphosis in other fish species such as anchovy (Engraulis mordax), Atlantic herring (Clupea harengus), and yellowtail (Seriola quinqueradiata) (Nakayama et al., 2003). At night or when kept in dark conditions, however, H. niloticus fry were observed not to organize into well-coordinated schools even after 6 DAH. According to Fukuda et al. (2014), Pacific bluefin tuna (Thunnus orientalis) larvae and young juveniles do not actively swim at night, as they depend on vision to perceive the motion of neighboring fish when schooling. Moreover, according to Emery (1973), fry schools always disintegrate at night, and the fish disperse to lower near-bottom water layers, become inactive, and only change their behavior when an artificial source of light is turned on or when they are frightened. Furthermore, the black coloration observed at the back in H. niloticus larvae after 4 DAH may be attributed to a camouflage strategy to avoid detection by predators from above, which is consistent with the observation of dark pigmentation in the dorsal region of the larvae of sunbleak (Leucaspius delineatus) (Pinder & Gozlan, 2004).
Artemia provides a considerably larger live feed that ranges in size from 200 to 500 μm (Granvil, 2000) compared with rotifers, which range between 50 and 200 μm in size (Pronob et al., 2012). The better growth observed with Artemia compared with rotifers is most likely partly explained by the size of the prey. Use of Artemia likely favored the initial stages of fry, as fry of Heterotis are larger than many other larvae at hatching, with a TL of 9.1 ± 0.1 mm, and hence have a wider mouth gape that can easily handle relatively large prey. Larvae of many fish species are reared at first feeding on rotifers Brachionus spp. (Arimoro, 2006). Rotifers are the most crucial live food organisms as an initial source of nutrition for small fish larvae (Lubzens et al., 2003; Awaiss and Kestemont, 1998). According to Lim and Wong (1997), the freshwater strain B. calyciflorus is among the most suitable species for the larviculture of the freshwater ornamental fish dwarf gourami (Colisa lalia) in Singapore. Shiri et al. (2003) also successfully cultured burbot (Lota lota) larvae, an endangered freshwater fish in Western Europe, with freshwater rotifer, B. calyciflorus, and obtained a survival rate of 69.2% in fry fed with rotifer. Adite et al. (2009) demonstrated in his weaning diet studies the use of zooplankton (freshwater rotifers: Brachionus spp.) collected in a pond and Artemia nauplii as a first feeding diet to maintain Heterotis fry for 2 days before transition to particulate feed. However, the co-occurrence of the total mortality recorded in the rotifer-fed group and unfed treatment group in the present experiment, alongside the higher survival and growth rate for the Artemia-fed treatment relative to the lower survival and growth rate in the co-fed group, indicates some level of starvation and inadequacy for the co-fed treatment and the rotifer-fed treatment. Thus, the most likely cause of the difference in survival and growth observed between the co-feeding group and the Artemia-fed group is the amount of feed provided (0.5–1 Artemia ind./ml vs. 1–2 Artemia ind./ml) and consequently the effect of feed availability and total energy in the Artemia-fed group as compared with the co-fed group. The total mortality observed for the group fed with only rotifers may be a result of the smaller size of rotifers, intake of which therefore did not provide enough energy to support the metabolism and energy requirements of H. niloticus fry, whereas Artemia nauplii have higher energy content compared with rotifers (Darias et al., 2015).
Digestive enzymes are present in some larvae before the start of exogenous feeding (Cahu and Infante, 1994; Ribeiro et al., 1999; Cara et al., 2003), and their presence may influence the rate at which exogenous food will be digested and metabolized (Gawlicka et al., 2000). The ability of fish larvae/fry to digest nutrients from their diet is mostly dependent on the availability of appropriate digestive enzymes, which is influenced by the physical and chemical characteristics of the feed (Cahu and Zambonino-Infante, 2001). The results of this study confirm that the key digestive enzymes are present during first feeding (mouth opening) in H. niloticus fry, demonstrating the importance of these enzymes in the early stages of the development of the species.
The observation of enzymatic activity is considered an indicator of a functional stomach (Moyano et al., 1996). A functional stomach has been observed in early developmental stages (before or during yolk absorption) of some freshwater fish species, such as rainbow trout (Oncorhynchus mykiss; Ershova et al., 2004), Cuban gar (Atractosteus tristoechus; Comabella et al., 2006), Nile tilapia (Oreochromis niloticus; Drossou et al., 2006), and Mexican mojarra (Cichlasoma urophthalmus; López-Ramírez et al., 2011). Thus, the significant increases in specific activities of trypsin, amylase, and lipase observed under the Artemia-fed treatment with age reflect an increase in digestive capacity and larval stomach development (Johnston and Ritar, 2001). In this study, we observed a higher level of activity of enzymes involved in the digestion of proteins, lipids, and carbohydrates compared with studies of other freshwater species, including larvae of the green catfish Mystus nemurus, and in reports on marine species, such as striped bass (Morone saxatilis; Baragi and Lovell, 1986), turbot (Scophthalmus maximus L.; Cousin et al., 1987), and gilthead seabream (Sparus aurata; Moyano et al., 1996). A possible explanation for the higher enzyme activity may be that it results from the analyses of whole larvae/fry, which may have affected results compared with those that would have been obtained if only the gut had been dissected and analyzed. Similar results regarding higher enzyme activity using whole larvae have also been reported in yellowfin seabream (Acanthopagrus latus) by Morshedi et al. (2021).
Trypsin is an important measure of nutritional status that is directly related to protein metabolism. Levels are mostly influenced by the protein content and amino acid profile of the diet (Imentai et al., 2022). The only pancreatic enzyme that can activate both itself and other digestive enzymes is trypsin, which is thus essential for digestion in larvae (Nazemroaya et al., 2015). In the present study, specific trypsin activity in H. niloticus larvae/fry was detected at 5 DAH; this increased significantly and maintained a higher level in the Artemia treatment groups (Artemia-fed and co-fed), whereas it was significantly lower in the rotifer treatment group, and this was similar to what was observed in the unfed treatment group. This clearly points toward starvation in both groups and low acceptance of rotifers as feed by the H. niloticus fry.
Energy derived from lipid and carbohydrate sources is crucial for metabolic maintenance and larval growth in order to spare amino acids required for protein synthesis during larval development (Darias et al., 2006). Even though zooplanktonic prey have relatively low carbohydrate content (Dhont and Van Stappen, 2003), some levels of amylase have been discovered in the ontogenetic development of digestive enzymes in some carnivorous fish larvae/fry (Ribeiro et al., 1999; Cahu and Zambonino-Infante, 2001; Martínez-Lagos et al., 2014). The specific activity of amylase in this experiment displayed a bell-shaped pattern (Figure 3), with its peak value occurring at 7 DAH for the best-performing treatment (Artemia-fed) and a decreasing trend for the co-fed and rotifer-fed treatments. The low levels of amylase activity in the Artemia-fed treatment group indicate low levels of carbohydrate ingestion, and suggest that H. niloticus larvae/fry are virtually exclusively carnivorous. According to Nazemroaya et al. (2015), a similar pattern for amylase-specific activity was found in fry of the red porgy, Pagrus pagrus (Suzer et al., 2007b). The decreasing level at 9 DAH for the co-fed and rotifer-fed treatments most likely indicates starvation.
Lipase activity during early larvae ontogeny may affect the utilization of dietary lipids (López-López et al., 2008). In this study, lipase activity measured at mouth opening was considerably higher compared with levels measured in other freshwater fry following mouth opening, including wolf cichlid (Parachromis dovii; Frías-Quintana et al., 2019) and Chinese perch (Siniperca chuatsi; Tang et al., 2021). Adaptation to exogenous nutrition is evidenced by the gradual rise in specific lipase activity after 5 DAH. This is in accordance with studies in other freshwater species such as green catfish fry (M. nemurus; Srichanun et al., 2012), in which the development of a functioning stomach during weaning was found to affect lipase activity.
Water quality variables (temperature, DO, TDS, and pH) minor variation between treatment groups and were within the acceptable ranges for rearing fish larvae (Arimoro, 2007; Ajepe et al., 2014).
In conclusion, the duration of transition between consuming endogenous and exogenous food is a crucial factor in the survival of H. niloticus larvae because, during this time, rapid morphological changes take place in the structural makeup of the organism. Given that feeding with Artemia nauplii was superior to co-feeding with rotifers or feeding with rotifers only in terms of survival and growth, Artemia nauplii can be considered a suitable starter feed for H. niloticus fry, although density and feeding levels should be considered. The findings of this study provide important information for use in curbing the high mortality rates observed in H. niloticus hatcheries. The present study provides knowledge of the presence and levels of the three main enzymes at the onset of mouth opening and offers valuable information on the ability of fry to digest particulate feed and the formulation of such. Studies on the nutritional composition of larval diets and the suitability of particulate diet for the larviculture of H. niloticus as well as the weaning age of H. niloticus fry are required to enhance the commercial culture of the species.
The raw data supporting the conclusions of this article will be made available by the authors, without undue reservation.
The animal studies were approved by Animal Research Ethics Committee (AREC), Kwame Nkrumah University of Science and Technology, Kumasi, Ghana. The studies were conducted in accordance with the local legislation and institutional requirements. Written informed consent was obtained from the owners for the participation of their animals in this study.
PO: Conceptualization, Data curation, Formal analysis, Investigation, Methodology, Project administration, Software, Supervision, Validation, Visualization, Writing – original draft, Writing – review & editing. DA: Conceptualization, Funding acquisition, Investigation, Project administration, Resources, Supervision, Validation, Visualization, Writing – review & editing. RE: Conceptualization, Formal analysis, Funding acquisition, Investigation, Methodology, Project administration, Resources, Supervision, Validation, Visualization, Writing – review & editing. NA: Conceptualization, Funding acquisition, Investigation, Methodology, Project administration, Resources, Supervision, Validation, Visualization, Writing – review & editing. IL: Conceptualization, Formal analysis, Funding acquisition, Investigation, Methodology, Project administration, Resources, Supervision, Validation, Visualization, Writing – review & editing.
The author(s) declare financial support was received for the research, authorship, and/or publication of this article. This research was funded by the Danish International Development Agency (DANIDA) under the project ´Improving the Productivity of Ghanaian Aquaculture’ (DFC No. 18-16-GHA).
The authors wish to thank Seyram Kwadzo Amane-Adjei and Doris Yeboah Baah, both at Kwame Nkrumah University of Science and Technology, Kumasi, Ghana, for assisting with the fieldwork and for analytical support, respectively.
The authors declare that the research was conducted in the absence of any commercial or financial relationships that could be construed as a potential conflict of interest.
All claims expressed in this article are solely those of the authors and do not necessarily represent those of their affiliated organizations, or those of the publisher, the editors and the reviewers. Any product that may be evaluated in this article, or claim that may be made by its manufacturer, is not guaranteed or endorsed by the publisher.
The Supplementary Material for this article can be found online at: https://www.frontiersin.org/articles/10.3389/faquc.2024.1310429/full#supplementary-material
Adite A., Fiogbe E., Accodji J. M. (2009). Effects of weaning age on survival and growth factors of Heterotis niloticus (Cuvier 1829) larvae. Int. J. Biol. Chem. Sci. 3 (6). doi: 10.4314/ijbcs.v3i6.53150
Adite A., Winemiller K. O., Fiogbé E. D. (2005). Ontogenetic, seasonal and spatial variation in the diet of Heterotis niloticus (Osteoglossiformes: Osteoglossidae) in the Soˆ River and Lake Hlan, Benin. West Africa. Environ. Biol. Fishes. 73, 367–378. doi: 10.1007/s10641-004-5563-9
Adite A. K., Winemiller O., Fiogbe E. D. (2006). Population structure and reproduction of the African bonytongue Heterotis niloticus in the Sô River-floodplain system (West Africa): implications for management. Ecol. Freshw. Fishes 15, 30–39. doi: 10.1111/j.1600-0633.2005.00119.x
Ajepe R. G., Hammed A. M., Amosu A. O., Fashina-Bombata H. A. (2014). Comparative study of Artemia (Brine Shrimp) and Ceriodaphnia (Zooplankton) as foods for catfish larvae. Am. J. Exp. Agric. 4 (7), 857. doi: 10.9734/AJEA/2014/6129
Akpaglo P. K. (2012). “The Potential of Heterotis niloticus (African Bonytongue) Aquaculture Candidate in Ghana,” in Master of Philosophy in Aquaculture (Ghana: Kwame Nkrumah University of Science and Technology). Available at: https://ir.knust.edu.gh/items/dedf02cb-0747-49b0-a749-b20f0a0dc448.
Aragão C., Conceição L. E. C., Dinis M. T., Fyhn H.-J. (2004). Amino acid pools of rotifers and artemia under different conditions: nutritional implications for fish larvae. Aquaculture 234, 429–445. doi: 10.1016/j.aquaculture.2004.01.025
Arimoro F. (2007). First feeding in the african catfish clarias anguillaris fry in tanks with the freshwater rotifer brachionus calyciflorus. J. Fish Aquat. Sci. 2 (4), 275–284.
Arimoro F. O. (2006). Culture of the freshwater rotifer, Brachionus calyciflorus, and its application in fish larviculture technology. Afr. J. Biotechnol. 5 (7), 536–541.
Awaiss A., Kestemont P. (1998). Feeding sequences (rotifer and dry chemical composition of african catfish, (Clarias gariepinus) burchell (Pisces: Clariidae) larvae. Aquaculture Res. 29 (10), 731.
Baragi V., Lovell R. T. (1986). Digestive enzyme activities in striped bass from first feeding through larval development. Trans. Am. Fisheries Soc. 115 (3), 478–484. doi: 10.1577/1548-8659(1986)115<478:DEAISB>2.0.CO;2
Cahu C. L., Infante J. Z. (1994). Early weaning of sea bass (Dicentrarchus labrax) larvae with a compound diet: effect on digestive enzymes. Comp. Biochem. Physiol. Part A: Physiol. 109 (2), 213–222. doi: 10.1016/0300-9629(94)90123-6
Cahu C. L., Zambonino-Infante J. L. (2001). Substitution of live food by formulated diets in marine fish larvae. Aquaculture 200, 161–180. doi: 10.1016/S0044-8486(01)00699-8
Cara J. B., Moyano F. J., Cárdenas S., Fernández-Díaz C., Yúfera M. (2003). Assessment of digestive enzyme activities during larval development of white bream. J. Fish Biol. 63 (1), 48–58. doi: 10.1046/j.1095-8649.2003.00120.x
Comabella Y., Mendoza R., Aguilera C., Carrillo O., Hurtado A., García-Galano T. (2006). Digestive enzyme activity during early larval development of the Cuban gar Atractosteus tristoechus. Fish Physiol. Biochem. 32, 147–157. doi: 10.1007/s10695-006-0007-4
Conceição L. E. C., Yúfera M., Makridis P., Morais S., Dinis M. T. (2010). Live feeds for early stages of fish rearing. Aquaculture Res. 41, 613–640. doi: 10.1111/j.1365-2109.2009.02242.x
Cousin J. C. B., Baudin-Laurencin F., Gabaudan J. (1987). Ontogeny of enzymatic activities in fed and fasting turbot, Scophthalmus maximus L. J. Fish Biol. 30 (1), 15–33. doi: 10.1111/j.1095-8649.1987.tb05728.x
Dabrowski K. (1979). The role of proteolytic enzymes in fish digestion. Cultivation of fish fry and its live food, 4, 107-126.Damle, D. K., & Chari, M. S., (2011). Performance evaluation of different animal wastes on culture of daphnia sp. J. Fish Aquat. Sci. 6 (1), 57.
Damle D. K., Chari M. S. (2011). Performance evaluation of different animal wastes on culture of daphnia sp. J. Fish Aquat. Sci. 6 (1), 57.
Darias M. J., Castro-Ruiz D., Estivals G., Quazuguel P., Fernández-Méndez C., Núñez-Rodríguez J., et al. (2015). Influence of dietary protein and lipid levels on growth performance and the incidence of cannibalism in Pseudoplatystoma punctifer (Castelnau 1855) larvae and early juveniles. J. Appl. Ichthyology 31, 74–82. doi: 10.1111/jai.12978
Darias M. J., Murray H. M., Gallant J. W., Astola A., Douglas S. E., Yúfera M., et al. (2006). Characterization of a partial α-amylase clone from red porgy (Pagrus pagrus): expression during larval development. Comp. Biochem. Physiol. Part B: Biochem. Mol. Biol. 143 (2), 209–218. doi: 10.1016/j.cbpb.2005.11.010
Das P., Mandal S. C., Bhagabati S. K., Akhtar M. S., Singh S. K. (2012). Important live food organisms and their role in aquaculture. Front. aquaculture 5 (4), 69–86. Available at: https://courseware.cutm.ac.in/wp-content/uploads/2020/06/Important-live-feed-and-their-role.pdf.
De Kimpe P. (1967). Heterotis niloticus: Recherche sur la survie des alevins (Bouaké: Centre Technique Forestier Tropical), 18. (miméo).
Dhert P. (1996). “Rotifers,” in Manual on the Production and Use of Live Food for Aquaculture, vol. 361 . Eds. Lavens P., Sorgeloos P. (Rome: FAO), 49–78. FAO Fisheries Technical Paper.
Dhont J., Dierckens K., Støttrup J., Van Stappen G., Wille M., Sorgeloos P. (2013). “Rotifers, Artemia and copepods as live feeds for fish larvae in aquaculture,” in Advances in aquaculture hatchery technology (Woodhead Publishing), 157–202. doi: 10.1533/9780857097460.1.157
Dhont J., Van Stappen G. (2003). Biology, tank production and nutritional value of Artemia. Live feeds Mar. aquaculture 3, 65–121. doi: 10.1002/9780470995143.ch3
Drossou A., Ueberschär B., Rosenthal H., Herzig K. H. (2006). Ontogenetic development of the proteolytic digestion activities in larvae of Oreochromis niloticus fed with different diets. Aquaculture 256 (1-4), 479–488. doi: 10.1016/j.aquaculture.2006.01.038
Emery A. R. (1973). Preliminary comparisons of day and night habits of freshwater fish in ontario lakes. J. Fish. Res. Board Can. 30 (6), 761–774.
Ershova T. S., Volkova I. V., Zaitsev V. F. (2004). Specific features of digestive function development in larvae of some salmonid fish. Russian J. Dev. Biol. 35, 233–237. doi: 10.1023/B:RUDO.0000036714.24271.11
Ferraris J. C. J. (2003). “Arapaimatidae (Bonytongues),” in Checklist of the Freshwater Fishes of South and Central America. Eds. Reis R. E., Kullander S. O., FerrarisJr. J. C. J. (Porto Alegre: Edipucrs, Brasil), 31.
Frías-Quintana C. A., Álvarez-González C. A., Guerrero-Zárate R., Valverde-Chavarría S., Ulloa-Rojas J. B. (2019). Changes in digestive enzymes activities during the initial ontogeny of wolf cichlid, parachromis dovii (Perciformes: Cichlidae). Neotrop. Ichthyol. 17.
Frimpong E. A., Agbo N. W., Amisah S., Anane-Taabeah G., Tettey E., Akpaglo P. (2011). Development and diversification of species for aquaculture in Ghana. Aquanews 26, 401–416. Available at: https://aquafishcrsp.oregonstate.edu/search/google/Development%20and%20Diversification%20of%20Species%20for%20Aquaculture%20in%20Ghana.
Fukuda H., Torisawa S., Takagi T. (2014). Ontogenetic changes in schooling behavior and visual sensitivity during larval and juvenile stages in pacific bluefin tuna, thunnus orientalis. Suisan Sougou Kenkyuu Senta Kenkyuu Houkoku 38, 135–139.
Gawlicka A., Parent B., Horn M. H., Ross N., Opstad I., Torrissen O. J. (2000). Activity of digestive enzymes in yolk-sac larvae of Atlantic halibut (Hippoglossus hippoglossus): indication of readiness for first feeding. Aquaculture 184 (3-4), 303–314. doi: 10.1016/S0044-8486(99)00322-1
Gomes M. L. M., Matta S. L. P., Araujo V. A., Silva G. M. F., Zacaro A. A. (2010). Larval ontogeny and morphology of giant trahira Hoplias lacerdae. J. Fish Biol. 76 (4), 852–861. doi: 10.1111/j.1095-8649.2010.02540.x
Goncalves R., Lund I., Gesto M. (2021). Interactions of temperature and dietary composition on juvenile European lobster (Homarus gammarus, L.) energy metabolism and performance. Comp. Biochem. Physiol. Part A: Mol. Integr. Physiol. 260, 111019. doi: 10.1016/j.cbpa.2021.111019
Govoni J. J., Boehlert G. W., Watanabe Y. (1986). The physiology of digestion in fish larvae. Environ. Biol. Fishes 16, 59–77. doi: 10.1007/BF00005160
Granvil T. D. (2000). Artemia production for marine larval fish culture (Vol. 702). (Stoneville, Mississippi: Southern Regional Aquaculture Center), pp 1–8.
Hamre K., Yúfera M., Rønnestad I., Boglione C., Conceição L. E., Izquierdo M. (2013). Fish larval nutrition and feed formulation: knowledge gaps and bottlenecks for advances in larval rearing. Rev. Aquaculture 5, S26–S58. doi: 10.1111/j.1753-5131.2012.01086.x
Imentai A., Gilannejad N., Martínez-Rodríguez G., López F. J. M., Martínez F. P., Pěnka T., et al. (2022). Effects of first feeding regime on gene expression and enzyme activity in pikeperch (Sander lucioperca) larvae. Front. Mar. Sci. 9, 864536. doi: 10.3389/fmars.2022.864536
Infante J. Z., Cahu C. L. (2001). Ontogeny of the gastrointestinal tract of marine fish larvae. Comp. Biochem. Physiol. Part C: Toxicol. Pharmacol. 130 (4), 477–487.
Johnston D. J., Ritar A. (2001). Mouthpart and foregut ontogeny in phyllosoma larvae of the spiny lobster Jasus edwardsii (Decapoda: Palinuridae). Mar. Freshw. Res. 52 (8), 1375–1386. doi: 10.1071/MF01105
Kamler E., Kamler E. (1992). “Endogenous feeding period,” in Early life history of fish: An energetics approach. (London: Chapman & Hall), 107–175.
Kim B. G., Divakaran S., Brown C. L., Ostrowski A. C. (2001). Comparative digestive enzyme ontogeny in two marine larval fishes: Pacific threadfin (Polydactylus sexfilis) and bluefin trevally (Caranx melampygus). Fish Physiol. Biochem. 24 (3), 225–241.
Lahnsteiner F., Lahnsteiner E., Duenser A. (2023). Suitability of different live feed for first feeding of freshwater fish larvae. Aquaculture J. 3 (2), 107–120. doi: 10.3390/aquacj3020010
Levêque C., Paugy D., Teugels G. G. (1990). Faunes des Poissons d’Eaux Douces et Saumâtres de l’Afrique de l’Ouest. Tome 1. Paris (FRA); Tervuren: ORSTOM; MRAC, 384 p. (Faune Tropicale; 28).
Lim L. C., Wong C. C. (1997). Use of the rotifer, brachionus calyciflorus pallas, in freshwater ornamental fish larviculture. Hydrobiologia 358 (1-3), 269–273.
López-López S., Nolasco H., Vega-Villasante F. (2008). Characterization of digestive gland esterase-lipase activity of juvenile redclaw crayfish Cherax quadricarinatus. Comp. Biochem. Physiol. B 135 (2), 337–347. doi: 10.1016/S1096-4959(03)00087-3
López-Ramírez G., Cuenca-Soria C. A., Alvarez-González C. A., Tovar-Ramírez D., Ortiz-Galindo J. L., Perales-García N., et al. (2011). Development of digestive enzymes in larvae of mayan cichlid cichlasoma urophthalmus. Fish Physiol. Biochem. 37, 197–208.
Lubzens E., Zmora O., Stottrup J., McEvoy L. (2003). Production and nutritional value of rotifers. Live feeds Mar. aquaculture 2, 300–303. doi: 10.1002/9780470995143.ch2
Ma H., Cahu C., Zambonino J., Yu H., Duan Q., Le Gall M. M., et al. (2005). Activities of selected digestive enzymes during larval development of large yellow croaker (Pseudosciaena crocea). Aquaculture 245 (1-4), 239–248.
Martínez-Lagos R., Tovar-Ramírez D., Gracia-López V., Lazo J. P. (2014). Changes in digestive enzyme activities during larval development of leopard grouper (Mycteroperca rosacea). Fish Physiol. Biochem. 40 (3), 773–785. doi: 10.1007/s10695-013-9884-5
Micha J. C. (1973). Study of the ubangui fish populations and attempts to select and adapt a few species to the fishpond (Nogent-sur-Marne: Centre Technique Forestier Tropical), 110.
Monentcham S. E., Kouam J., Pouomogne V., Kestemont P. (2009). Biology and prospect for aquaculture of African bonytongue, Heterotis niloticus (Cuvier, 1829): A review. Aquaculture 289 (3-4), 191–198. doi: 10.1016/j.aquaculture.2009.01.019
Monentcham S. E., Pouomogne V., Kestemont P. (2010). Influence of dietary protein levels on growth performance and body composition of African bonytongue fingerlings, Heterotis niloticus (Cuvier 1829). Aquaculture. Nutr. 16, 144–152. doi: 10.1111/j.1365-2095.2008.00646.x
Moreau J. (1982). Exposé synoptique des données biologiques sur Heterotis niloticus (Cuvier 1829). FAO Synop. Pêches 131, 45.
Morshedi V., Hamedi S., Pourkhazaei F., Mozanzadeh M. T., Tamadoni R., Ebadi M., et al. (2021). Larval rearing and ontogeny of digestive enzyme activities in yellowfin seabream (Acanthopagrus latus, Houttuyn 1782). Comp. Biochem. Physiol. Part A: Mol. Integr. Physiol. 261, 111044. doi: 10.1016/j.cbpa.2021.111044
Moyano F. J., Diaz M., Alarcón F. J., Sarasquete M. C. (1996). Characterization of digestive enzyme activity during larval development of gilthead seabream (Sparus aurata). Fish Physiol. Biochem. 15, 121–130. doi: 10.1007/BF01875591
Nakayama S., Masuda R., Shoji J., Takeuchi T., Tanaka M. (2003). Effect of prey items on the development of schooling behavior in chub mackerel Scomber japonicus in the laboratory. Fisheries Sci. 69 (4), 670–676. doi: 10.1046/j.1444-2906.2003.00673.x
Nazemroaya S., Yazdanparast R., Nematollahi M. A., Farahmand H., Mirzadeh Q. (2015). Ontogenetic development of digestive enzymes in Sobaity sea bream Sparidentex hasta larvae under culture condition. Aquaculture 448, 545–551. doi: 10.1016/j.aquaculture.2015.06.038
Palińska-Żarska K., Żarski D., Krejszeff S., Nowosad J., Biłas M., Trejchel K., et al. (2014). Dynamics of yolk sac and oil droplet utilization and behavioural aspects of swim bladder inflation in burbot, Lota lota L., larvae during the first days of life, under laboratory conditions. Aquaculture Int. 22, 13–27. doi: 10.1007/s10499-013-9663-4
Pan Y. J., Dahms H. U., Hwang J. S., Souissi S. (2022). Recent trends in live feeds for marine larviculture: a mini review. Front. Mar. Sci. 9, 864165. doi: 10.3389/fmars.2022.864165
Peña R., Dumas S., Villalejo-Fuerte M., Ortız-Galindo J. L. (2003). Ontogenetic development of the digestive tract in reared spotted sand bass paralabrax maculatofasciatus larvae. Aquaculture 219 (1-4), 633–644.
Pinder A. C., Gozlan R. E. (2004). Early ontogeny of sunbleak. J. Fish Biol. 64 (3), 762–775. doi: 10.1111/j.1095-8649.2004.00346.x
Pronob D., Mandal S. C., Bhagabati S. K., Akhtar M. S., Singh S. K. (2012). Important live food organisms and their role in aquaculture. Front. Aquac. 5(4), 69–86.
Radhakrishnan K. D., AkbarAli I., Schmidt B. V., John E. M., Sivanpillai S., Thazhakot Vasunambesan S. (2020). Improvement of nutritional quality of live feed for aquaculture: An overview. Aquaculture Res. 51, 1–17. doi: 10.1111/are.14357
Rakotomanampisson A. (1966). Premiers résultats de l’acclimatation de heterotis niloticus à madagascar (Tananarive: Direction des forêts), 32. (miméo).
Reizer C. (1964). Comportement et reproduction de Heterotis niloticus en petits étangs. Bois For. Trop. 95, 49–60.
Reizer C. (1968). Influence et distribution de la nourriture artificielle sur la mortalité des alevins, la croissance préadulte, et la maturité sexuelle chez heterotis niloticus. FAO Fish. Rep. 3 (44), 326–358.
Ribeiro L., Zambonino-Infante J. L., Cahu C., Dinis M. T. (1999). Development of digestive enzymes in larvae of Solea Senegalensis, Kaup 1858. Aquaculture 179 (1-4), 465–473. doi: 10.1016/S0044-8486(99)00180-5
Rønnestad I., Koven W. M., Tandler A., Harel M., Fyhn H. J. (1994). Energy metabolism during development of eggs and larvae of Gilthead Sea bream (Sparus aurata). Mar. Biol. 120, 187–196. doi: 10.1007/BF00349678
Rønnestad I., Rojas Garcia C. R., Kamisaka Y., Koven W., Barr Y., Fyhn H. J., et al. (2001). “Ontogeny of digestive function of marine fish larvae,” in LARVI‘01-fish and shellfish larviculture symposium (European Aquaculture Society, Special Publication), 514–516.
Rotllant J., Liu D., Yan Y. L., Postlethwait J. H., Westerfield M., Du S. J. (2008). Sparc (Osteonectin) functions in morphogenesis of the pharyngeal skeleton and inner ear. Matrix Biol. 27 (6), 561–572. doi: 10.1016/j.matbio.2008.03.001
Shiri A. H., De Charleroy D., Auwerx J., Van Slycken J., Dhert P., Sorgeloos P. (2003). Larval rearing of burbot (Lota lota l.) using brachionus calyciflorus rotifer as starter food. J. Appl. Ichthyol. 19 (2), 84–87.
Siju R., Anil M. K., Sawant P. B., Babitha Rani A. M., Ambarish G. P., Gomathi P., et al. (2021). Ontogenetic development of digestive tract and enzymes activity in hatchery-reared pink ear emperor, lethrinus lentjan larvae. Turkish J. Fisheries Aquat. Sci. 23 (5), 1–14. doi: 10.4194/TRJFAS21151
Srichanun M., Tantikitti C., Vatanakul V., Musikarune P. (2012). Digestive enzyme activity during ontogenetic development and effect of live feed in green catfish larvae (Mystus nemurus Cuv. & Val.). Songklanakarin J. Sci. Technol. 34 (3).
Srivastava A. S., Kurokawa T., Suzuki T. (2002). mRNA expression of pancreatic enzyme precursors and estimation of protein digestibility in first feeding larvae of the japanese flounder, paralichthys olivaceus. Comp. Biochem. Physiol. Part A Mol. Integr. Physiol. 132 (3), 629–635.
Suzer C., Kamaci H. O., Coban D., Saka Ş., Firat K., Özkara B., et al. (2007b). Digestive enzyme activity of the red porgy (Pagrus pagrus, L.) during larval development under culture conditions. Aquaculture Res. 38 (16), 1778–1785. doi: 10.1111/j.1365-2109.2007.01841.x
Tang S., Liang X., He S., Zhang Y., Peng D., Feng H. (2021). Development of digestive enzymatic activity and gene expression during the early ontogeny of Chinese perch (Siniperca chuatsi). (Research Square Company, a CC BY 4.0 License), pp 2–17. doi: 10.21203/rs.3.rs-141784/v1
Ueberschär B. (1993). “Measurement of proteolytic enzyme activity: significance and application in larval fish research,” in Physiological and biochemical aspects of fish development (Norway: Univ. of Bergen), 233–239.
Keywords: hatching, yolk sac, Heterotis niloticus, Artemia, rotifer and larval survival
Citation: Ofori-Darkwah P, Adjei-Boateng D, Edziyie RE, Agbo NW and Lund I (2024) Early ontogeny of the African bony-tongue (Heterotis niloticus) and the effect of Artemia and rotifer live feeds on larval digestive enzyme activity and performance. Front. Aquac. 3:1310429. doi: 10.3389/faquc.2024.1310429
Received: 09 October 2023; Accepted: 15 January 2024;
Published: 13 February 2024.
Edited by:
Youji Wang, Shanghai Ocean University, ChinaReviewed by:
Tran Nguyen Duy Khoa, Can Tho University, VietnamCopyright © 2024 Ofori-Darkwah, Adjei-Boateng, Edziyie, Agbo and Lund. This is an open-access article distributed under the terms of the Creative Commons Attribution License (CC BY). The use, distribution or reproduction in other forums is permitted, provided the original author(s) and the copyright owner(s) are credited and that the original publication in this journal is cited, in accordance with accepted academic practice. No use, distribution or reproduction is permitted which does not comply with these terms.
*Correspondence: Prince Ofori-Darkwah, UHJpbmNlZGFya3dhaDU4QGdtYWlsLmNvbQ==; cHJpbmNlZGFya3dhaEB5YWhvby5jb20=
Disclaimer: All claims expressed in this article are solely those of the authors and do not necessarily represent those of their affiliated organizations, or those of the publisher, the editors and the reviewers. Any product that may be evaluated in this article or claim that may be made by its manufacturer is not guaranteed or endorsed by the publisher.
Research integrity at Frontiers
Learn more about the work of our research integrity team to safeguard the quality of each article we publish.