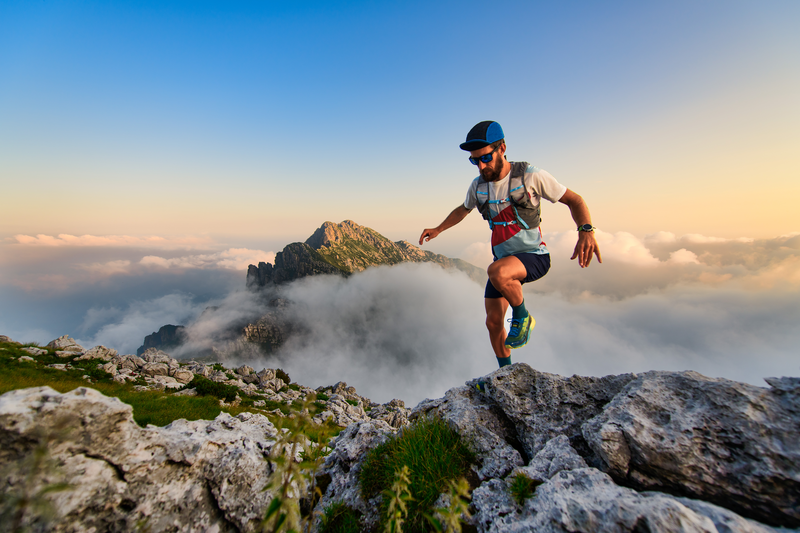
94% of researchers rate our articles as excellent or good
Learn more about the work of our research integrity team to safeguard the quality of each article we publish.
Find out more
ORIGINAL RESEARCH article
Front. Anim. Sci. , 28 January 2025
Sec. Animal Nutrition
Volume 6 - 2025 | https://doi.org/10.3389/fanim.2025.1508660
The environmental impact of livestock waste has driven the need for nutritional strategies to enhance digestive efficiency in pigs, aiming to reduce nutrient excretion and associated emissions of pollutants like ammonia (NH3) and greenhouse gases (GHG). This study investigated the effects of dietary crude protein (CP) reduction by 1.5%, combined with supplementation of a dietary treatment that included feed additives and higher soluble fiber levels, on nutrient digestibility, slurry composition, and emissions across growing and finishing phases. Eighty male pigs were assigned to four different diets in a 13-week trial under a 2 × 2 factorial design: standard protein (SP) and low protein (LP) diets, with or without the dietary treatment. Key measurements included slurry composition, NH3-N and CH4 emissions, and crop yield when slurry was applied as fertilizer. The low-protein diet supplemented with additives (LPA) significantly reduced slurry pH (P ≤ 0.001) and urinary NH3-N excretion (interaction, P = 0.03), improving nutrient digestibility and lowering organic matter content in slurry (P < 0.05). NH3 emissions from the room and slurry pit decreased by over 38%, while CH4 emissions, although higher in LP diets, were mitigated with the LPA diet. Field application of slurry as fertilizer resulted in trends favoring sustainable wheat production, with increased yield and nitrogen use efficiency, alongside reduced CH4 emissions (P < 0.001). These findings underscore the potential of combined dietary strategies to mitigate environmental impacts while enhancing agricultural sustainability.
Continued global demographic growth along with increasing purchasing power in developing countries, mainly in Asia and South America, is generating a growing consumption and demand of animal-based proteins, which is expected to double by 2050 (Rojas-Downing et al., 2017; Zappaterra et al., 2022). The current scenario requires an increase in food production by the agricultural and livestock sector, which must necessarily be accompanied by an improvement in the efficient utilization of nutrients and natural resources. Moreover, the livestock sector is under enormous social pressure for its contribution to environmental pollution and climate change, derived from the excretion of nutrients through manure including nitrogen (N) and phosphorous (P), but also for being an important source of polluting gases. Of the total greenhouse gases (GHG) of anthropogenic origin, it is estimated that livestock farming contributes to 14.5% of its emissions, being methane (CH4) the gas with the highest proportion, with 6.7 gigatons CO2-eq coming from the swine sector (Gerber et al., 2013). In addition, pig farms are an important source of ammonia (NH3) emissions to the atmosphere, coming essentially from animal housing and slurry storage (Philippe et al., 2011). Its emissions have increased by 4.7% in Spain from 1990 to 2020, and of the 479 Kt produced, 96.8% is generated by agriculture and livestock farming (MITECO, 2023).
Dietary manipulation, but especially precision feeding has demonstrated to be a key strategy to improve nutrient efficiency of animals through adjusting the diet composition to animal needs (de la Fuente et al., 2018; Tullo et al., 2019), and thus reducing the excretion of nutrients that are supplied in excess. In this regard, lowering crude protein (CP) content, combined with a balanced crystalline amino acids addition, is a widely adopted measure to reduce N excretion and NH3 emissions (Portejoie et al., 2004; Morazán et al., 2015; Seradj et al., 2018), with reductions of up to 8 to 10% per unit drop in dietary CP (Zhao et al., 2019; Trabue et al., 2020). In addition, dietary supplementation with additives including exogenous enzymes (e.g., phytases or carbohydrases), acidifying agents (e.g., benzoic acid), plant extracted essential oils, and their combination has received special recognition. The use of these products has demonstrated consistently an improved animal’s performance as well as a more efficient nutrient utilization, mainly attributed to their positive effects on animal immune system, gut morphology, microbiota composition and enzyme activity (Torrallardona et al., 2007), thus enhancing the nutrient digestibility and absorption (Balasubramanian et al., 2020; Wang et al., 2020). Likewise, these dietary manipulations can modify the composition and physicochemical characteristics of slurry, directly impacting in the emission of pollutant gases (Wang et al., 2020). In this regard, including higher levels of soluble fiber has been appreciated as a measure towards reducing NH3 emissions from pig slurry by stimulating hindgut fermentation, shifting volatile urinary N excretion to a more stable form in fecal N, and lowering slurry pH (Nguyen et al., 2019).
Furthermore, a proper destination of slurry as organic fertilizer is a concern of producers and a demand of society to reduce the use of mineral fertilizers (Debiase et al., 2016; Geng et al., 2019; Wei et al., 2020). The latter products impact economically but also environmentally on agricultural production systems (Osei et al., 2003; Rotz et al., 2011), negatively influencing the structure and composition of crop soils (Xia et al., 2017). Crops have nutrient requirements for their potential production, however the indiscriminate use of both organic and mineral fertilizers may lead environmental concerns, such as leaching of N and P into soil and water resources (Eghball et al., 1996; Álvaro-Fuentes et al., 2016; Demurtas et al., 2016; Aguilera et al., 2021). In addition, NH3 and N2O emissions from field application should not be underestimated. Therefore, the use of both types of fertilizers has been regulated by recent EU policies (European Environment Agency, 2018; Huygens et al., 2020), and any application of organic products as fertilizers should be tested for their efficacy in crop nutrition and toxicity for soil environment.
The objectives of the present study were to examine the impact of two levels of dietary CP, supplemented or not with a dietary treatment including a blend of feed additives as well as higher levels of soluble fiber in growing and finishing pigs, on slurry composition and gaseous emissions during both pig production cycle and soil application, along with wheat crop production evaluation when slurry was applied as fertilizer.
The current study was carried out at the facilities of the Pig Research Centre (CEP; Torrelameu, Lleida, Spain). All procedures involving animal handling were performed in compliance with the regulations of the Spanish Policy for protection of animals employed in research and other scientific purposes (Real Decreto 53/2013, 2013), which meets the European Union guidelines (Directive 2010/63/EU, 2010) on the protection of animals used for experimentation. Protocols and experimental procedures were approved by the Ethics Committee for Animal Experiments of the University of Lleida, under the Project License Number 11498 (Generalitat de Catalunya).
Eighty intact male Pietrain × (Landrace × Large White) pigs were used in a 13-week experiment, with a mean initial BW of 33.25 ± 0.43 kg (µ ± SE) and 12 weeks of age. Upon arrival at the experimental facilities, the pigs were weighed and randomly distributed into four separate rooms, each corresponding to one of the four diets under study and connected by isolation doors. Twenty pigs were allocated per room (five pigs per pen), with less than 1.5% variability in mean weight and no statistical differences between rooms at the start of the trial. Each room consisted of four pens distributed in two pens on each side of the room, whose slurry was first collected in a single-room slurry pit (145 cm × 150 cm × 40 cm) located underneath. At the end of each feeding phase, the slurry from each experimental diet was drained into a flexible tank of larger capacity. The individual pens (4.2 m2 each) were 55% slat floor and were equipped with a single-space self-feeder in the concrete floor area and a square nipple drinker in the slatted floor area.
The first six weeks of the experiment were considered the growing (GRO) phase and the last seven weeks were defined as the finishing (FIN) phase. In both feeding phases, feed and drinking water were administered ad libitum, and the experiment followed a 2 × 2 factorial design, with the CP level and the inclusion of dietary treatment as the study factors.
The animals were kept under commercial-like production system, where temperature and relative humidity in the four rooms were monitored and recorded every 30 min. The average temperatures and humidity of the four rooms were of 23.7 ± 1.46 °C and 53.9 ± 5.18%, respectively during the GRO phase, and 20.90 ± 1.61 °C and 57.59 ± 6.54%, respectively in the FIN phase (Testo 174 H; Testo AG, Lenzkirch, Germany).
The ingredients and chemical composition of the experimental diets are shown in Table 1. The diets were pelleted and formulated to be isoenergetic for all four groups.
Table 1. Ingredients and chemical composition of the experimental diets of two feeding phases, growing and finishing, fed standard (SP) or low (LP) levels of crude protein, combined with the presence (SPA and LPA) or absence (SPC and LPC) of dietary treatment.
Two CP levels were established for each feeding phase. The standard protein (SP) diets followed FEDNA (2013) recommendations, with CP levels of 16% during the GRO phase and 15% during the FIN phase. In contrast, the low protein (LP) diets had CP levels reduced by 1.5 percentage points compared to SP diets, resulting in CP concentrations of 14.5% and 13.5% for the GRO and FIN phases, respectively. The reduction in CP aimed to investigate the potential impacts of protein sparing on animal performance and nutrient utilization.
In addition to CP concentration, half of the animals in each CP group received a dietary treatment consisting of a blend of feed additives and a higher inclusion level of soluble fiber. The additive blend included the following components: Vitazyme NSP (1000 mg kg-1), a carbohydrase product containing xylanase. VevoVitall® (3000 mg kg-1), composed mainly of benzoic acid. Biotronic® Top 3 (1000 mg kg-1), a combination of organic acids. Digestarom® Finish (150 mg kg-1), a blend of plant extracts designed to promote digestive efficiency.
The dietary treatment also included an elevated level of soluble fiber provided primarily through wheat bran and beet pulp for growing and finishing phases, respectively. Furthermore, all diets included phytase (Ronozyme® HiPhos, Switzerland) at 100 mg kg-1, and the carbohydrases contained in the blend of additives were included using matrix values following the manufacturer recommendations for ME values.
As a result, the study implemented four diets: SPC: Standard protein without dietary treatment; SPA: Standard protein with dietary treatment; LPC: Low protein without dietary treatment; LPA: Low protein with dietary treatment.
Individual BW was obtained every two weeks throughout the experimental period to assess average daily gain (ADG), as well as the weight of feed supplied and refusals (at the pen level) to evaluate average daily feed intake (ADFI) and feed conversion ratio (FCR).
Slurry production was estimated every three weeks considering the volume of the pit, by measuring the depth of the slurry with a meter rule. Then, 1 kg of slurry samples were gathered by choosing three representative sampling points per pit, pooling them to obtain a single sample on which pH was recorded with a portable pH meter (Crison micropH 2000). To minimize N evaporation, the samples were immediately frozen at –20 °C. Dry matter (DM), ash, neutral detergent fiber (NDF) and N were determined using the techniques described by Morazán et al. (2015), and NH3-N was analyzed by direct distillation with Na2B4O7 according to the Kjeldahl method (ref. 976.05; AOAC (1990)). For P content, the samples were processed by high performance microwave digestion (Ethos Up; Milestone, Sorisole, Italy) and analyzed by inductively coupled plasma mass spectrometry (7700x, Agilent).
Two measurements of NH3 and CH4 emissions per feeding phase were performed every three weeks by simultaneous collection of air samples from the slurry pit level and total room gas production, both consisting of a continuous air inlet and outlet flow system. The description of the installation and air collection of both systems is described in detail in Seradj et al. (2018). Briefly, contaminated air was collected from the pit level through portable flow chambers (PFC), so that two PFC were used in each slurry pit, one for NH3 emission and one for CH4 uptake, each operating at different air flow rates. The inlet air was also analyzed to determine the gas concentrations reaching the inside of the PFC. Whereas, the total gaseous emission in the room were collected from the exhaust air outlet at the midpoint of each room, according to the procedure of AMCA (2011). A representative air sample from outside the farm facility was also collected and analyzed.
Ammonia was collected for 48 h using a vacuum air pump (KNF N035.3 AN.18 – IP20, USA) at a flow rate of 3 L min-1 measured by a flowmeter (LZQ-1 0-5 LPM). Following the model proposed by Goldman and Jacobs (1953) and updated by Antezana Julián (2014), the collected air was bubbled in an acid solution (100 mL of H2SO4; 0.5 M) contained in glass impingers trapping the gaseous NH3 in aqueous ammonium (NH4+). After the measurement time elapsed, the acid solution was analyzed by Kjeldahl method (AOAC, 1990) and the NH3 trapped (mg L-1) was determined considering the N concentration, the volume of the solution and the molecular weight of NH3, as well as the flow rate applied during each measurement. The NH3 produced from the slurry was calculated as the difference between the NH3 concentrations of the inlet and outlet air.
As for CH4, all procedures were conducted following Seradj et al. (2018). Briefly, a flow rate of 10 mL min-1 (measured by Alltech electronic gas flowmeter, IL, USA) was generated by a peristaltic pump (Gilson, Minipulse 3, Le Bel Villiers, France) to collect the gas produced, which was stored in separate 20 L inert bags during 24 h. Air samples were taken from each bag using a 15 mL syringe and placed in 12 mL vials (model 039W, Labco, High Wycombe, UK). Samples were analyzed using a 7890A gas chromatograph equipped with a flame ionization detector with a methanizer. An HP-Plot column (30 m long, 0.32 mm diameter) was used, together with a 15 m long pre-column. The injector and furnace temperatures were set at 50 ˚C and 250 ˚C, respectively, while 375 ˚C were set for the methanizer. Hydrogen was used as a carrier gas and N2 as compensatory gas at 35 and 25 mL min-1, respectively. The sample volume injected was 1 mL and the CH4 production was calculated according to (Holland et al., 1999).
The CH4 concentration values (ppm) obtained from gas chromatography were transformed to mass volume-1 concentration (mg (m3)-1) considering its molecular weight, and applying the ideal gas law as follows:
Where Cm is the mass volume-1 concentration (mg (m3)-1), Cv corresponds to the volume volume-1 concentration (ppm), M is the molecular weight of CH4, P is the atmospheric pressure, R is the universal gas constant, and T is the temperature in degrees Kelvin. The absolute CH4 emission was calculated as the difference between inlet and outlet concentration, considering the airflow used during the measurements.
A commercial 7200 m2 irrigated experimental field was set up at the beginning of the experiment in the proximity of the pig facility. An irrigated wheat crop was planted by late November 2021 following standard technological production practices used for this crop. The layout of the experiment was a factorial design containing the application of slurry from animals subjected to the four diets under evaluation explained in the previous chapter on a field-level crop, with three randomized replicates (see Figure 1).
Figure 1. Layout of the 7200 m2 field experiment for agronomical and environmental evaluation of the application of the slurry on a wheat crop in Lleida, Spain. The slurry comes from the four different pig diets, which includes: T1: SPC; T2: SPA; T3: LPC; T4: LPA.
Prior to the start of the experiment, a soil analysis of the commercial plot was conducted to determine soil limiting factors by taking four composite samples from each plot at two depths (0–30 cm and 30–60 cm). The soil resulted to be a clay loamy soil, with a pH of 8.7, electric conductivity of 0.44 dS m-1 (no limiting for salinity), high levels of soil organic matter (25 mg kg-1), high levels of soil fertility in P and K and normal in nitrate concentration (12 ppm NO3–N). The overall result of these analyses is that soil was not a limiting factor for the experiment.
Crop wheat nutrient requirements are about 100–150 kg N ha-1 for a regular yield in these conditions. Due to normal levels of NO3–N concentration in the soil and high organic matter levels, it was decided to apply 100 kg N ha-1 for an expected yield of 5–7 t ha-1. All diets had approximately 5 to 6.5 kg N (m3)-1, then the slurry dosage for each group was calculated depending on this concentration. Slurry was applied as top-dressing fertilization of wheat in February 2022, with a separate tank for each group, which was loaded from the pig facilities.
To assess the effect of the different diets on the soil and crop, a composite sample of three or four soil cores was taken from each plot three times (prior to sowing, before top dressing application of slurry and after harvest) and at two depths (0–30 cm, 30–60 cm). Soil water content was determined by gravimetric method. Soil NH4+-N and NO3–N contents were quantified by extracting 20 g of fresh soil with 100 mL of 1 M KCl by means of a continuous flow autoanalyzer (Seal Autoanalyzer 3, Seal Analytical, Norderstedt, Germany). To determine crop N content, two plant samples per plot were taken at harvest, and N content was determined by DUMAS combustion (Tru Spec CN; Leco Corporation, St. Joseph, MI, USA; ISO (2008)) for the grain and for the rest of the plant.
Each plot was harvested in July 2022 with 10% grain moisture in the field. Grain yield of each plot was measured by an automatic trolley equipment with a weighting scale. To determine resource use efficiencies, indicators of water use efficiency (WUE) for grain yield (Equation 1) and N use efficiency (NUE) for grain yield (Equation 2) were calculated:
Where water use (WU) is calculated as the difference between soil water content at planting and at harvest, plus the cumulative rainfall and irrigation received between these two dates.
During the crop growth cycle, 15 samplings were done by a set of 24 non-steady-state chambers (Hutchinson and Mosier, 1981) to determine the concentration of three GHG: CH4, N2O and CO2. During the winter months (November to March), gas measurements were conducted at 3-week intervals. Gas samples of 15 mL each were collected at intervals of 0, 20, and 40 min following chamber closure and were subsequently stored in 12 mL Exetainer® borosilicate vials (model 038 W, Labco, High Wycombe, UK). During the warmer seasons (April to July), soil gas fluxes were measured every two weeks. However, when the land underwent fertilization, the sampling frequency was intensified: samples were collected 24 h before fertilization and then at 2, 24, 48, and 72 h after fertilization.
Samples were analyzed by a gas chromatography system (7890A, Agilent, Santa Clara, CA, United States) equipped with a flame ionization detector coupled to a methanizer to determine CO2, an electrical conductivity detector and a KRCIAES column (IA KRCIAES 6017: 250°C, 45 m long, 0.32 μm of section and 10 μm; Ingeniería Analítica., Barcelona, Spain) with a 15 m long pre-column of the same characteristics.
All data from the pig farm were analyzed using SAS System® software (SAS Institute Inc., Cary, NC, USA), employing an ANOVA mixed analysis model of repeated measures (PROC MIXED). The experimental design followed a randomized complete block design. The model consisted of fixed factors such as PH that represents the feeding phase (GRO and FIN) and considered as repeated measures, PL, representing the protein content of the diets (standard and low protein content), ADD represents the use of the dietary treatment with additives in the diets (with or without), and all possible interactions of the fixed factors as outlined below:
In cases (e.g., gas emissions) where the data did not conform to a normal distribution, a Log10 transformation was conducted prior to statistical analysis. Mean comparisons were performed using Tukey’s test with a significance level of P < 0.05. Trends were considered with P values between 0.05 and 0.10.
Statistical analyses of field results were performed using JMP pro 15 (SAS Institute Inc, 2020) statistical software. Cumulative soil CH4, CO2 and N2O emissions were quantified on a mass basis using the trapezoidal rule (Levy et al., 2017). The data were checked for normality, homoscedasticity and serial independence by Shapiro-Wilk, Bartlett, and Durbin-Watson test, respectively. Data were transformed when necessary to pass these tests. Outliers were checked using the Grubb’s test with a statistical confidence level of 95%. Repeated measures analysis of variance (ANOVA) was performed with each protein level, additives use and their interactions as effects. When significant differences among diets were identified at 0.05 probability level of significance with Tukey HSD test, a mean separation was carried out.
In this study, nutritional strategies were evaluated for their ability to modify pig digestive physiology to reduce enteric CH4 emissions, as well as slurry features to minimize the emission of pollutant gases from its excretion and on-farm storage to crop application. Therefore, the combined effect of lowering dietary CP level and a dietary treatment including a blend of feed additives, which have previously shown scientific evidence to reduce NH3 and CH4 emissions, was investigated.
Pig performance was within the expected results (see Supplementary Table S1). The reduction of dietary CP level, together with an appropriate supply of essential amino acids, allows to reduce costs and environmental impact (Wang et al., 2018) without altering growth performance (Wang et al., 2023), which was fully confirmed in our results. Regarding the use of the dietary treatment with feed additives, pigs fed the SPC diet in the FIN phase had higher ADG than the other groups, however, the analysis of the overall results showed little relevance of the interaction.
Regarding to the chemical composition of the slurry, the most relevant difference between the slurry produced in the two feeding phases was the DM content, which was much higher in the GRO phase in all diets, as can be seen in Table 2. These results can be explained by the increase in water consumption and subsequent urine production as the animals matured.
Table 2. Chemical composition (g (100 g DM)-1) of slurry from pigs of two feeding phases, growing and finishing, fed standard (SP) or low (LP) levels of crude protein, combined with the presence (SPA and LPA) or absence (SPC and LPC) of the dietary treatment.
Dietary CP level had a significant effect on slurry pH in both feeding phases (P < 0.001), showing a lower pH when pigs were fed the LP diets, as has been widely described in the existing literature (Morazán et al., 2015; Trabue et al., 2021; Le Dinh et al., 2022). Lower CP content reduces the excess of excreted N, mainly in urine but also in feces (Wang et al., 2020), with NH4+ levels being the main alkalinizing factor. However, in the present study, no differences in total N concentration were found between SP and LP diets. A discrete reduction in the dietary CP (i.e., 1.5%) should not be excluded as the reason for the lack of significant differences in this parameter, as well as a collateral increase in fecal N excretion due to the higher fiber content in the LP diets. However, a higher NH3 emission by pigs fed the SP diet, especially in the FIN phase, is also proposed and discussed below.
The incorporation of the aforementioned dietary treatment also had an effect on reducing the pH of the slurry, this was true in the FIN phase (P = 0.001) and in the LP group of the GRO phase, whereas young pigs fed the SPA diet showed the opposite response (significant interaction, P < 0.001). The decrease in pH may be explained by i) the presence of benzoic acid in the additive blend, whose hepatic conjugation with glycine leads to hippuric acid excretion in the urine, thus lowering the urine and slurry pH (Halas et al., 2010; Humphrey et al., 2022); and ii) the slightly higher fiber content of SPA and LPA diets in both feeding phases (see Table 1). Higher levels of fiber may reduce slurry pH either by improving the production of volatile fatty acids or by reducing urinary NH3-N (and the NH3-N alkalizing effect) in favor of fecal bacterial N (Kerr et al., 2006; Jarret et al., 2012), as was found especially in the slurry of LPA fed pigs in the FIN phase (significant interaction P = 0.03). As previously mentioned, the effect of the used dietary treatment was not as clear in the more immature piglet hindgut, and therefore this point needs to be further investigated.
In addition, feed acidifiers were also associated with an improvement in nutrient digestibility, as reported in several studies (Liu et al., 2018). This effect is explained by the improvement of intestinal morphology, the increase in the activity of some digestive enzymes such as pepsin, lipase or sucrose, and the modulation of the gut microbiota, making it more beneficial for the animal (Torrallardona et al., 2007; Halas et al., 2010; Xu et al., 2018), as well as an increased N retention (Humphrey et al., 2022). Our pH reductions in additive-supplemented diets agree well with those reported by Kerr et al. (2006), who used an in vitro dynamic system with continuous slurry addition.
Xylanases were included in the additive blend mainly to improve the digestibility of non-starch polysaccharides provided by the higher fiber level within the SPA and LPA diet and also to reduce the viscosity of the intestinal content. Moreover, xylanases might improve the availability of other nutrients such as amino acids retained in the fiber fraction (Woyengo et al., 2008). Consequently, the digestible energy content was also improved (Nortey et al., 2007), which explains the lower fat content required in SPA and LPA diets to obtain iso-energetic diets. In this sense, the reduction of OM content in the slurry of pigs fed the additive-supplemented diets in both feeding phases (P ≤ 0.05) may be associated with improved nutrient digestibility (Boontiam et al., 2022). The increased availability of shorter oligosaccharides due to the action of xylanases on long arabinoxylan chains explains the compositional modulation in the intestinal microbiota (Boontiam et al., 2022); also associated with a great prebiotic potential. In this regard, it has been described that essential oils and other plant extracts can create a favorable intestinal environment for beneficial microbes, either through their antioxidant activity or by improving nutrient digestibility (Liu et al., 2018).
Regarding P concentration, the results indicate that despite the fact that the same amount of digestible P and phytase was provided in all experimental diets, pigs fed feed additive mixture had a lower excretion, especially in the FIN phase (P = 0.005), where the decrease of P was 12% in SP diets and up to 24% in LP diets. Several studies have demonstrated the positive effect of the addition of organic acids such as benzoic acid and essential oils (Sauer et al., 2009; Xu et al., 2018) on the apparent digestibility of P in growing pigs. The reduced pH by organic acids may have enhanced phytase activity on phytate P, while increasing mineral absorption through its association with acid anions (Nahm, 2004; Suiryanrayna and Ramana, 2015).
Emissions of NH3 and CH4 were analyzed at 2 different measurement locations in the same room, at the slurry pit level just below the animals through the PFCs, and total room emissions. A summary of these gas emissions for the GRO and FIN phases is presented in Table 3.
Table 3. Gas emissions (g per animal per day) measured at the slurry pit level and total room gas production in the growing and finishing phases of pigs fed diets with two levels of crude protein, including or not a dietary treatment.
The recorded NH3 emissions, especially those from total room production, were within the range reported by other authors (Philippe et al., 2007; Hansen et al., 2014; Le Dinh et al., 2022) for the GRO and FIN phases in pigs reared on slatted floors. In addition, these same NH3 emissions increased numerically with pig maturation, as previously reported by Seradj et al. (2018). It was assumed that total room emissions correspond to those generated in the slurry pit plus those evaporated in the soiled portion of the floor and in the slat by direct contact of urine and feces prior draining into the slurry pit. This mixture leads to urea hydrolysis through the enzymatic action of ureases from fecal bacteria and the subsequent and rapid volatilization from NH4+ (Philippe et al., 2011; Fuertes et al., 2021). Accordingly, these latter NH3 emissions should be numerically higher compared to those obtained in the slurry pit, where NH3 formation and release occur in the long-term period and are exclusively subjected to the air-slurry interface, which limits the potential volatilization area. In the latter fraction, NH3 emissions from the degradation of undigested proteins must also be considered, although they are proportionally much less important (Aarnink and Verstegen, 2007).
The dietary CP level had important effects on NH3 emissions, which decreased in the rooms where LP diets were offered. This response was observed in the total room emissions of the GRO and FIN phases, with an overall reduction of 38% (P = 0.025) and 46% (P < 0.001), respectively, and also at the slurry pit level of the FIN phase, with a noticeable reduction of 85% (P < 0.001). The reduction of NH3 emissions by lowering dietary CP levels has been widely reported in previous studies (Galassi et al., 2010; Hernández et al., 2011; Seradj et al., 2018; Le Dinh et al., 2022), associated with lower N excretion, mainly in the form of urine (Philippe et al., 2011; Wang et al., 2018). In this sense, the lack of significant differences in total N concentration between the two CP levels could be attributed to the higher NH3 volatilization in the SP-fed pigs. In addition, the important effect on slurry pH discussed earlier may directly contribute to the lower conversion of manure NH4+ to NH3 in the LP-fed pigs, thus reducing its emissions (Aarnink and Verstegen, 2007; Le Dinh et al., 2022). This effect was even more pronounced in pigs receiving the combination of the LP diet and the dietary treatment (i.e., LPA), which resulted in a reduced NH3-N concentration especially in the FIN phase (significant interaction P = 0.03). It is well known that the reduction of NH3-N in swine slurry reduces NH3 volatilization, which in turn is a consequence of the reduced pH (van der Peet-Schwering et al., 1999).
Until recently, few studies have measured the emission of pollutant gases at the slurry pit level. In the present study, we observed that the overall reduction in NH3 emissions by lowering the CP level was more intense at the slurry pit, up to almost 60%, than the total room emission, which was reduced by 43%. Likewise, in a recent study by Le Dinh et al. (2022), in which NH3 emissions were measured at four heights, 1 and 10 cm above the slurry surface, and at floor and room level, they obtained more intense reductions in NH3 emissions at locations closer to the volatilization surfaces when dietary CP was reduced by 2%.
In accordance with the above, the use of the dietary treatment under study also had significant effects on NH3 emissions, although some differences were observed between both feeding phases. In the GRO phase, total room NH3 emissions decreased significantly in the presence of the dietary treatment, by 36% in the SP and 38% in the LP group (P < 0.029). However, significant interactions were found in the FIN phase in both measurement locations (P < 0.05). While NH3 emissions decreased significantly with the dietary treatment in the LP group, up to 89% at the slurry pit level and 51% in the total room emissions, no differences were found in the SP group, or even numerically increased. This slight increase could be consistent with the high values of NH3-N in the slurry presented in Table 2. Furthermore, the greater reduction in total room NH3 emissions shows that the inclusion of such dietary treatment may play a greater role when urine and feces are mixed prior to falling into the slurry pit, rather than the long-term emissions produced at the air-slurry interface of the slurry pit.
In line with the beneficial effects associated with the inclusion of feed additives on the dietary treatment discussed above, the meta-analysis by Ti et al. (2019), which evaluated several mitigation practices to reduce NH3 emissions, concluded that the highest efficacy of feed additives, beyond reducing CP levels, can be explained by their effects on modifying the microbial population of the digestive tract, the nutrient composition, and the physicochemical properties of the slurry (e.g., pH), and thus gas emissions from both the digestive tract and the slurry.
Partitioning of emissions between the slurry pit level and total room emissions refers only to NH3 evaporation. In the case of CH4, the slurry pit emission is generated by anaerobic fermentation of slurry material, while the total CH4 room emissions (excluding slurry pit emissions) correspond to the enteric CH4 generated in the hindgut and eliminated by flatulence. Indeed, the CH4 generated by the fermentation of fecal material during its transit toward the pit is considered negligible. Therefore, the results are presented and discussed in this context. Methane emissions showed considerable differences between the feeding phases, both at the slurry pit level and in total room emissions, which may be influenced by the different fiber source between phases. Emissions were higher in the FIN phase than in the GRO phase, in agreement with previous values from our group (Seradj et al., 2018). This is explained by a more developed and abundant microbial population in the hindgut of mature pigs (Sarri et al., 2021), resulting in a higher fermentative capacity and enteric production of CH4 (Le Goff et al., 2002). However, it also coincides with higher dietary fiber content. Overall, CH4 emissions showed a greater rate of variability between days of analysis compared to NH3 emissions. This variability in the results led to a lack of statistical differences in most of the analyses performed for this gas.
Although in previous research (Seradj et al., 2018, 2020) we did not find differences in CH4 emissions as a function of dietary CP variations, in the present study certain differences were obtained, especially in the GRO phase. Overall, CH4 emissions increased by 6–8 times in the LP group of the GRO phase and by 2–3 times in the FIN phase, which was mainly due by a high rise in LPC diet, being significant in the total room emissions of the GRO phase (P = 0.049). However, this result was necessarily explained by its interaction with the presence of the dietary treatment (significant interaction P = 0.013). It should be noted that the fiber content in LP diets was 3% higher than in SP diets (Table 1), and therefore an increase in CH4 emissions would have been expected. However, when the dietary treatment was included in the LP diets (i.e., LPA diet), an important reduction in CH4 emissions was obtained in the total room emissions, reaching values close to those of the SP diet, even though the dietary treatment involved a 15% higher fiber content. These results suggest that the inclusion of additives may have helped to digest and absorb certain nutrients that would otherwise have been fermented in the hindgut, promoting both enteric and slurry pit CH4 production (Balasubramanian et al., 2020). The specific effect of inclusion of additives seemed to be stronger in the enteric fermentation rather than the slurry pit emission, suggesting that it may target non-digestible but highly fermentable material that might be more available to the animal after enzymes action before reaching the hindgut.
On the other hand, the inclusion of feed additives in the dietary treatment could not involve a clear trend, as its effect varied with respect to control diets among phases and sampling locations. A previous study also using benzoic acid at 0.3% (Humphrey et al., 2022) showed no differences in CH4 emissions when this acid was included in the diet. Moreover, investigations carried out by the Guide of emission reduction techniques in swine and poultry livestock (MAGRAMA, 2014), regarding the use of acidifiers in feed, indicate an 11% increase in CH4 compared to control groups, despite being no significant, as in our case. Again, it has to be considered that the dietary treatment included a higher fiber content compared to control diets. This could mean that other components of the blend of additives could have a greater effect on the reduction of CH4.
The first thing to be considered regarding the application of slurry on crop production and environmental assessment, is that this type of fertilization technology has low effect during the first year, and it is more relevant to study the cumulative effect over the years as well as the seasonal effect. Hence, the results obtained in this study can miss some of the long-term potential of using low CP diets on crop fertilization, limiting in this way the ability to assess the full scope of benefits. However, similar to what has been observed in other short-term studies (Franco-Otero et al., 2012; Jin et al., 2022), it remains valuable to identify trends in this particular matter that indicate the direction for further exploration in future studies.
Grain yield and grain protein content in the trial were similar to those obtained in the surrounding area (Zhang et al., 1998; Lloveras et al., 2004; Aranguren et al., 2021), with yields exceeding 5 t ha-1, regardless of the diet, and an average protein content of 9.25%. Differences in crop yield and grain protein content for irrigated wheat among diets were not statistically significant, whether comparing protein levels, additives, or their interaction (Table 4). Therefore, the results should be interpreted as trends. In this sense, there was a positive trend towards increased wheat crop yield with the presence of feed additives, observed in both SP and LP diets. In terms of grain protein content, the slurry from pigs fed the dietary treatment led to a slightly higher protein content when combined with the LP diet, compared to when no dietary treatment was used. These results may suggest that LPA slurry had more accessible N, which could favor a faster incorporation of protein into the grain. Conversely, the opposite trend was observed at the SP level (Table 4).
Table 4. Crop performance for wheat crop yield, grain protein content, water use efficiency (WUE) and N use efficiency (NUE) after slurry application.
The WUE in the wheat crop was below the area’s average for irrigated conditions (Zhang et al., 1998; Katerji et al., 2008). The main hypotheses for this phenomenon revolve around the coincidence of grain filling stages with moderate temperatures and adequate soil moisture content during the tillering and flowering processes (Zahedi and Jenner, 2003). In this case, the results obtained should also be interpreted as trends. The primary observation was a better WUE when using the slurry from pigs supplemented with the dietary treatment, and a lower WUE values in the LPC slurry. Moreover, the NUE, considered as crop production vs. applied N was above 50 kg of grain per kg N ha-1, which is considered a medium-high level of efficiency of the application of the slurry. Results were again not significant, despite it can be appreciated a trend towards a better NUE with the inclusion of the dietary treatment under study, in both SP and LP diets.
The GHG derived from slurry application to the field under study are shown for the four different diets in Table 5. In all of the three GHG analyzed (CH4, CO2 and N2O) the level of the accumulated emission is consistent with the obtained in our Mediterranean conditions with irrigated wheat crop (Alhajj Ali et al., 2017; Oliveira et al., 2021).
Table 5. Greenhouse gases accumulated emissions for CH4, CO2 and N2O from the experimental field under the different diets of the study.
The only GHG that showed statistical significance was CH4, with values close to zero or even reaching negative balance in the emissions. This suggests that methanotrophic activity in the soil surpassed methanogenic activity due to the presence of aerobic conditions (Guo et al., 2022). This negative balance pattern is commonly observed in experiments conducted in the region under similar conditions (Franco-Luesma et al., 2019; Fernández-Ortega et al., 2024). In this way, some differences were found between groups. Diets including the dietary treatment significantly reduced CH4 emissions when comparing with control (P < 0.001), as well as with the use of LP diets (P < 0.001), with a significant interaction between both parameters under study (P < 0.001). Considering the low emissions of CH4, it is remarkable that possibly the LP diet combined with the dietary treatment could be contributing to lower CH4 emissions added to a fixation of this as C in the soil.
No statistical differences were found for CO2 between the four groups. That is consistent with the normal behavior of CO2 emissions that are not usually affected by the application of fertilizers in a short period of one year, such as in this study.
Regarding N2O, soils are the dominating source for atmospheric N2O (Butterbach-Bahl et al., 2013). Deposited NH3 can act as an indirect source of this gas, as it can be transformed into N2O through nitrification-denitrification processes (IPCC, 2006). In our case, N2O did not present significance for any treatment under study; nevertheless, its emissions showed a trend to be increased in LPA diets. Differences on the emission by diets may be associate to a more intense microbiological activity. Emission integration of CO2, N2O and CH4 indicates the accumulated CO2 emission equivalent and therefore its global warming potential. This GHG accumulation is presented in Figure 2, and despite there were no significant differences, numerically higher accumulated GHG emission in LPA diets can be appreciated (P > 0.05), mainly due to the aforementioned higher N2O emissions. This may highlight the importance of considering the impact of N2O, given its significant warming potential (EPA, 2023). On the other hand, the numerically lowest accumulated GHG emissions were found when the dietary treatment and the SP level were combined.
All human activity has an impact on GHG emission, but they are included in the natural cycles of the planet; therefore, any activity with a biological component produces emissions of CO2, N2O and CH4. In this case, the best crop yields were observed with diets that included the dietary treatment, both for standard and low protein level. The numerically higher N2O field emissions in the LPA slurry could be related to its lower emission of NH3 during the animal trial, with a lower NH3-N concentration in slurry (Table 2). Nevertheless, it may be possible that this slurry could still present a relevant organic N concentration, which may lead to higher N2O emissions during field application (Dambreville et al., 2008). However, this effect needs to be further investigated in repeated slurry application as fertilizer in longer-term studies to better understand the interaction between N excretion forms and the subsequent GHG emissions when slurry is applied as fertilizer.
Results suggest that CP level in pigs can be reduced at some extent (1.5%) to reduce NH3 emissions at the slurry pit level but also in the total room emissions, without compromising animal performance. Moreover, the use of feed additives combined with a higher soluble fiber content to mitigate NH3 emissions led to significant reductions from slurry in both pit and room without affecting pig performance. Reduction was more effective in the FIN phase and especially in LP diets, where the inclusion of this dietary treatment led to a NH3 reduction of more than 90% in the slurry pit and more than 50% at the room level. LP diets showed higher levels of CH4 in both pit and room, although most of analysis weren´t conclusive for the high variability in the results. Regarding field application, LPA slurry had positive effects on the efficiency and yield of the crop despite not showing significance, suggesting some beneficial tendencies with the combination of LP and the dietary treatment with additives. Moreover, based on GHG emissions and global warming potential, LPA diets showed no significant negative effects of the application of the resulting slurry as top-dressing fertilization in the wheat crop.
The original contributions presented in the study are included in the article/Supplementary Material. Further inquiries can be directed to the corresponding authors.
The manuscript presents research on animals that do not require ethical approval for their study.
EF: Formal analysis, Investigation, Methodology, Visualization, Writing – original draft, Writing – review & editing. LS: Conceptualization, Investigation, Methodology, Supervision, Writing – original draft, Writing – review & editing. RC: Data curation, Formal analysis, Investigation, Methodology, Writing – original draft, Writing – review & editing. EP-C: Funding acquisition, Project administration, Supervision, Visualization, Writing – original draft, Writing – review & editing. ÁC: Conceptualization, Data curation, Formal analysis, Supervision, Writing – original draft, Writing – review & editing. JB: Conceptualization, Funding acquisition, Resources, Supervision, Writing – original draft, Writing – review & editing. AS: Conceptualization, Methodology, Resources, Supervision, Writing – original draft, Writing – review & editing. CC-M: Formal analysis, Investigation, Methodology, Supervision, Writing – original draft, Writing – review & editing. JF-O: Formal analysis, Investigation, Methodology, Visualization, Writing – original draft, Writing – review & editing. GD: Conceptualization, Investigation, Project administration, Supervision, Writing – original draft, Writing – review & editing.
The author(s) declare financial support was received for the research, authorship, and/or publication of this article. Funding was provided by DSM-Firmenich. EF was the recipient of a grant from Agencia de Gestión de Ayudas Universitarias y de Investigación of Spain (FI-SDUR 2020/00031), and together with LS are currently receiving Investigo funding (2022 INV-1 00010).
The authors would like to acknowledge the staff of the Centre d’Estudis Porcins (CEP, Spain).
EP-C and AC were employed by DSM- Firmenich.
The remaining authors declare that the research was conducted in the absence of any commercial or financial relationships that could be construed as a potential conflict of interest.
The author(s) declare that no Generative AI was used in the creation of this manuscript.
All claims expressed in this article are solely those of the authors and do not necessarily represent those of their affiliated organizations, or those of the publisher, the editors and the reviewers. Any product that may be evaluated in this article, or claim that may be made by its manufacturer, is not guaranteed or endorsed by the publisher.
The Supplementary Material for this article can be found online at: https://www.frontiersin.org/articles/10.3389/fanim.2025.1508660/full#supplementary-material
Aarnink A. J. A., Verstegen M. W. A. (2007). Nutrition, key factor to reduce environmental load from pig production. Livestock Sci. 109, 194–203. doi: 10.1016/j.livsci.2007.01.112
Aguilera E., Reyes-Palomo C., Díaz-Gaona C., Sanz-Cobena A., Smith P., García-Laureano R., et al. (2021). Greenhouse gas emissions from Mediterranean agriculture: Evidence of unbalanced research efforts and knowledge gaps. Global Environ. Change 69, 102319. doi: 10.1016/j.gloenvcha.2021.102319
Alhajj Ali S., Tedone L., Verdini L., De Mastro G. (2017). Effect of different crop management systems on rainfed durum wheat greenhouse gas emissions and carbon footprint under Mediterranean conditions. J. Clean. Product. 140, 608–621. doi: 10.1016/j.jclepro.2016.04.135
Álvaro-Fuentes J., Arrúe J. L., Cantero-Martínez C., Isla R., Plaza-Bonilla D., Quílez D. (2016). Fertilization scenarios in sprinkler-irrigated corn under Mediterranean conditions: effects on greenhouse gas emissions. Soil Sci. Soc. America J. 80, 662–671. doi: 10.2136/sssaj2015.04.0156
AMCA International (2011). Field performance measurement of fan systems (Arlington Heights, IL, USA: The International Authority on Air Systems Components).
Antezana Julián W. O. (2014). Optimización de la medición de emisiones de NH3 de purines por el método de trampas ácidas húmedas in vitro (Valencia, Spain: Universitat Politècnica de València).
AOAC (1990). Association of Official Analytical Chemists. 15th ed. Ed. Helrich K. (Arlington, VA, USA: Association of Official Analytical Chemists, Inc).
Aranguren M., Castellón A., Aizpurua A. (2021). Wheat grain protein content under Mediterranean conditions measured with chlorophyll meter. Plants 10, 374. doi: 10.3390/plants10020374
Balasubramanian B., Park J. H., Shanmugam S., Kim I. H. (2020). Influences of enzyme blend supplementation on growth performance, nutrient digestibility, fecal microbiota and meat-quality in grower-finisher pigs. Animals 10, 386. doi: 10.3390/ani10030386
Boontiam W., Phaenghairee P., Van Hoeck V., Vasanthakumari B. L., Somers I., Wealleans A. (2022). Xylanase impact beyond performance: effects on gut structure, fecal volatile fatty acid content and ammonia emissions in weaned piglets fed diets containing fibrous ingredients. Animals 12, 3043. doi: 10.3390/ani12213043
Butterbach-Bahl K., Baggs E. M., Dannenmann M., Kiese R., Zechmeister-Boltenstern S. (2013). Nitrous oxide emissions from soils: how well do we understand the processes and their controls? Philos. Trans. R. Soc. B 368, 20130122. doi: 10.1098/rstb.2013.0122
Dambreville C., Morvan T., Germon J. C. (2008). N2O emission in maize-crops fertilized with pig slurry, matured pig manure or ammonium nitrate in Brittany. Agric. Ecosyst. Environ. 123, 201–210. doi: 10.1016/J.AGEE.2007.06.00
Debiase G., Montemurro F., Fiore A., Rotolo C., Farrag K., Miccolis A., et al. (2016). Organic amendment and minimum tillage in winter wheat grown in Mediterranean conditions: Effects on yield performance, soil fertility and environmental impact. Eur. J. Agron. 75, 149–157. doi: 10.1016/j.eja.2015.12.009
de la Fuente G., Seradj A. R., Balcells J. (2018). Un paso adelante en la alimentación de precisión. Suis 146, 20–27.
Demurtas C. E., Seddaiu G., Ledda L., Cappai C., Doro L., Carletti A., et al. (2016). Replacing organic with mineral N fertilization does not reduce nitrate leaching in double crop forage systems under Mediterranean conditions. Agricult. Ecosyst. Environ. 219, 83–92. doi: 10.1016/j.agee.2015.12.010
Directive 2010/63/EU (2010). Directive 2010/63/EU of the European Parliament and of the Council of 22 September 2010 on the protection of animals used for scientific purposes., Pub. L. No. Off. J. Eur. Union L276 33. Available at: https://eur-lex.europa.eu/eli/dir/2010/63/oj/eng.
Eghball B., Binford G. D., Baltensperger D. D. (1996). Phosphorus movement and adsorption in a soil receiving long-term manure and fertilizer application. J. Environ. Qual. 25, 1339–1343. doi: 10.2134/jeq1996.00472425002500060024x
EPA (2023). Understanding global warming potentials. Available at: https://www.epa.gov/ghgemissions/understanding-global-warming-potentials.
European Environment Agency (2018). “Environmental indicator report 2018,” in In support to the monitoring of the Seventh Environment Action Program (Publications Office of the European Union, Copenhagen, Denmark). doi: 10.2800/180334
FEDNA (2013). Necesidades nutricionales para ganado porcino. 2nd ed. Eds. Blas C., Gasa J., Mateos G. G. (Madrid, Spain: Normas FEDNA).
Fernández-Ortega J., Álvaro-Fuentes J., Cantero-Martínez C. (2024). Double-cropping, tillage and nitrogen fertilization effects on soil CO2 and CH4 emissions. Agricult. Ecosyst. Environ. 359, 108758. doi: 10.1016/J.AGEE.2023.108758
Franco-Luesma S., Álvaro-Fuentes J., Plaza-Bonilla D., Arrúe J. L., Cantero-Martínez C., Cavero J. (2019). Influence of irrigation time and frequency on greenhouse gas emissions in a solid-set sprinkler-irrigated maize under Mediterranean conditions. Agric. Water Manage. 221, 303–311. doi: 10.1016/J.AGWAT.2019.03.042
Franco-Otero V. G., Soler-Rovira P., Hernández D., López-de-Sá E. G., Plaza C. (2012). Short-term effects of organic municipal wastes on wheat yield, microbial biomass, microbial activity, and chemical properties of soil. Biol. Fertil. Soils 48, 205–216. doi: 10.1007/s00374-011-0620-y
Fuertes E., Seradj A. R., Maynegre J., Villalba D., de la Fuente G., Balcells J. (2021). Annual nitrogen balance from dairy barns, comparison between cubicle and compost-bedded pack housing systems in the northeast of Spain. Animals 11, 2136. doi: 10.3390/ani11072136
Galassi G., Colombini S., Malagutti L., Crovetto G. M., Rapetti L. (2010). Effects of high fiber and low protein diets on performance, digestibility, nitrogen excretion and ammonia emission in the heavy pig. Anim. Feed Sci. Technol. 161, 140–148. doi: 10.1016/j.anifeedsci.2010.08.009
Geng Y., Cao G., Wang L., Wang S. (2019). Effects of equal chemical fertilizer substitutions with organic manure on yield, dry matter, and nitrogen uptake of spring maize and soil nitrogen distribution. PloS One 14, e0219512. doi: 10.1371/journal.pone.0219512
Gerber P. J., Steinfeld H., Henderson B., Mottet A., Opio C., Dijkman J., et al. (2013). Tackling climate change through livestock - A global assessment of emissions and mitigation opportunities (Rome: Food and agriculture organization of the United Nations (FAO). Available at: www.fao.org/publications (Accessed May, 2022).
Goldman F. H., Jacobs M. B. (1953). Chemical methods in industrial hygiene. J. AOAC Int. 37, 558. doi: 10.1093/JAOAC/37.2.558
Guo L., Shi J., Lin W., Liang J., Lu Z., Tang X., et al. (2022). Soil bacteria mediate soil organic carbon sequestration under different tillage and straw management in rice-wheat cropping systems. Agric. (Switzerland) 12, 1552. doi: 10.3390/agriculture12101552
Halas D., Hansen C. F., Hampson D. J., Kim J. C., Mullan B. P., Wilson R. H., et al. (2010). Effects of benzoic acid and inulin on ammonia-nitrogen excretion, plasma urea levels, and the pH in feces and urine of weaner pigs. Livestock Sci. 134, 243–245. doi: 10.1016/j.livsci.2010.06.153
Hansen M. J., Nørgaard J. V., Adamsen A. P. S., Poulsen H. D. (2014). Effect of reduced crude protein on ammonia, methane, and chemical odorants emitted from pig houses. Livestock Sci. 169, 118–124. doi: 10.1016/j.livsci.2014.08.017
Hernández F., Martínez S., López C., Megías M. D., López M., Madrid J. (2011). Effect of dietary crude protein levels in a commercial range, on the nitrogen balance, ammonia emission and pollutant characteristics of slurry in fattening pigs. Animal 5, 1290–1298. doi: 10.1017/S1751731111000115
Holland E. A., Robertson G. P., Greenberg J., Groffman P. M., Boone R. D., Gosz J. R. (1999). “Soil CO2, N2O, and CH4 exchange,” in Standard soil methods for long-term ecological research. Eds. Robertson G. P., Bledsoe C. S., Coleman D. C., Sollins P. (Oxford University Press, New York, USA), 185–201.
Humphrey D. C., Bergstrom J. R., Calvo E. P., Trabue S. L., Scoggin K. D., Greiner L. L. (2022). The effect of benzoic acid with or without a direct-fed microbial on the nutrient metabolism and gas emissions of growing pigs. J. Anim. Sci. 100, 1-11. doi: 10.1093/jas/skac296
Hutchinson G. L., Mosier A. R. (1981). Improved soil cover method for field measurement of nitrous oxide fluxes. Soil Sci. Soc. America J. 45, 311–316. doi: 10.2136/sssaj1981.03615995004500020017x
Huygens D., Orveillon G., Lugato E., Tavazzi S., Comero S., Jones A., et al. (2020). “[amp]]hellip;,” in Technical proposals for the safe use of processed manure above the threshold established for Nitrate Vulnerable Zones by the Nitrates Directive (91/676/EEC) (Luxembourg: Publications Office of the European Union). doi: 10.2760/984729
IPCC (2006). Guidelines for national greenhouse gas inventories. 4th ed. Eds. Eggleston S., Buendia L., Miwa K., Ngara T., Tanabe K. (Hayama, Japan: IGES).
ISO (2008). “Food products- Determination of the total nitrogen content by combustion according to the Dumas principle and calculation of the crude protein content,” in ISO 16634-1, 1st ed (International Organization for Standardization, Geneva, Switzerland). Available at: https://www.iso.org/standard/46328.html (Accessed November, 2020).
Jarret G., Cerisuelo A., Peu P., Martinez J., Dourmad J. Y. (2012). Impact of pig diets with different fiber contents on the composition of excreta and their gaseous emissions and anaerobic digestion. Agricult. Ecosyst. Environ. 160, 51–58. doi: 10.1016/j.agee.2011.05.029
Jin H., Zhang D., Yan Y., Yang C., Fang B., Li X., et al. (2022). Short-term application of chicken manure under different nitrogen rates alters structure and co-occurrence pattern but not diversity of soil microbial community in wheat field. Front. Microbiol. 13. doi: 10.3389/fmicb.2022.975571
Katerji N., Mastrorilli M., Rana G. (2008). Water use efficiency of crops cultivated in the Mediterranean region: review and analysis. Eur. J. Agron. 28, 493–507. doi: 10.1016/j.eja.2007.12.003
Kerr B. J., Ziemer C. J., Trabue S. L., Crouse J. D., Parkin T. B. (2006). Manure composition of swine as affected by dietary protein and cellulose concentrations. J. Anim. Sci. 84, 1584–1592. Available at: https://academic.oup.com/jas/article-abstract/84/6/1584/4776970.
Le Dinh P., van der Peet-Schwering C. M. C., Ogink N. W. M., Aarnink A. J. A. (2022). Effect of diet composition on excreta composition and ammonia emissions from growing-finishing pigs. Animals 12, 229. doi: 10.3390/ani12030229
Le Goff G., Dubois S., Van Milgen J., Noblet J. (2002). Influence of dietary fiber level on digestive and metabolic utilization of energy in growing and finishing pigs. Anim. Res. 51, 245–259. doi: 10.1051/animres:2002019
Levy P. E., Cowan N., van Oijen M., Famulari D., Drewer J., Skiba U. (2017). Estimation of cumulative fluxes of nitrous oxide: uncertainty in temporal upscaling and emission factors. Eur. J. Soil Sci. 68, 400–411. doi: 10.1111/ejss.12432
Liu Y., Espinosa C. D., Abelilla J. J., Casas G. A., Lagos L. V., Lee S. A., et al. (2018). Non-antibiotic feed additives in diets for pigs: a review. Anim. Nutr. 4, 113–125. doi: 10.1016/j.aninu.2018.01.007
Lloveras J., Manent J., Viudas J., López A., Santiveri P. (2004). Seeding rate influence on yield and yield components of irrigated winter wheat in a Mediterranean climate. Agron. J. 96, 1258–1265. doi: 10.2134/agronj2004.1258
MAGRAMA (2014). Evaluación de técnicas de reducción de emisiones en ganadería. Sectores de porcino y avicultura de carne y puesta (Madrid, Spain: Secretaría General Técnica Centro de Publicaciones).
MITECO (2023). Inventory informative report (informe de inventario de emisiones de contaminantes a la atmósfera). Available online at: https://www.miteco.gob.es/content/dam/miteco/es/calidad-y-evaluacion-ambiental/temas/sistema-espanol-de-inventario-sei-/es_iir_edicion2023_tcm30-560375.pdf (Accessed March, 2024).
Morazán H., Álvarez-Rodríguez J., Seradj A. R., Balcells J., Babot D. (2015). Trade-offs among growth performance, nutrient digestion and carcass traits when feeding low protein and/or high neutral-detergent fiber diets to growing-finishing pigs. Anim. Feed Sci. Technol. 207, 168–180. doi: 10.1016/j.anifeedsci.2015.06.003
Nahm K. H. (2004). Additives to reduce phosphorus excretion and phosphorus solubility in poultry and swine manure. Aust. J. Exp. Agric. 44, 717–728. doi: 10.1071/EA02143
Nguyen Q. H., Le P. D., Chim C., Le N. D., Fievez V. (2019). Potential to mitigate ammonia emission from slurry by increasing dietary fermentable fiber through inclusion of tropical byproducts in practical diets for growing pigs. Asian-Australas J. Anim. Sci. 32 (4), 574–584. doi: 10.5713/ajas.18.0481
Nortey T. N., Patience J. F., Sands J. S., Zijlstra R. T. (2007). Xylanase supplementation improves energy digestibility of wheat by-products in grower pigs. Livestock Sci. 109, 96–99. doi: 10.1016/j.livsci.2007.01.092
Oliveira M., Castro C., Coutinho J., Trindade H. (2021). Grain legume-based cropping systems can mitigate greenhouse gas emissions from cereal under Mediterranean conditions. Agricult. Ecosyst. Environ. 313, 107406. doi: 10.1016/j.agee.2021.107406
Osei E., Gassman P. W., Hauck L. M., Jones R., Beran L., Dyke P. T., et al. (2003). Environmental benefits and economic costs of manure incorporation on dairy waste application fields. J. Environ. Manage. 68, 1–11. doi: 10.1016/S0301-4797(02)00226-8
Philippe F.-X., Cabaraux J.-F., Nicks B. (2011). Ammonia emissions from pig houses: influencing factors and mitigation techniques. Agricult. Ecosyst. Environ. 141, 245–260. doi: 10.1016/j.agee.2011.03.012
Philippe F. X., Laitat M., Canart B., Vandenheede M., Nicks B. (2007). Comparison of ammonia and greenhouse gas emissions during the fattening of pigs, kept either on fully slatted floor or on deep litter. Livestock Sci. 111, 144–152. doi: 10.1016/j.livsci.2006.12.012
Portejoie S., Dourmad J. Y., Martinez J., Lebreton Y. (2004). Effect of lowering dietary crude protein on nitrogen excretion, manure composition and ammonia emission from fattening pigs. Livestock Product. Sci. 91, 45–55. doi: 10.1016/j.livprodsci.2004.06.013
Real Decreto 53/2013 (2013). Real Decreto 53/2013, de 1 de febrero, por el que se establecen las normas básicas aplicables para la protección de los animales utilizados en experimentación y otros fines científicos, incluyendo la docencia., 1 de febrero §. Available at: https://www.boe.es/eli/es/rd/2013/02/01/53.
Rojas-Downing M. M., Nejadhashemi A. P., Harrigan T., Woznicki S. A. (2017). Climate change and livestock: impacts, adaptation, and mitigation. Climate Risk Manage. 16, 145–163. doi: 10.1016/j.crm.2017.02.001
Rotz C. A., Kleinman P. J. A., Dell C. J., Veith T. L., Beegle D. B. (2011). Environmental and economic comparisons of manure application methods in farming systems. J. Environ. Qual. 40, 438–448. doi: 10.2134/jeq2010.0063
Sarri L., Balcells J., de la Fuente G., Tor M., Gómez-Arrue J., Seradj A. R. (2021). Evolution of viscera and muscle fractional protein synthesis rate in lean meat selected hybrids and castrated Duroc pigs fed under moderate crude protein restriction. Animal 15, 100220. doi: 10.1016/j.animal.2021.100220
Sauer W., Cervantes M., Yanez J., Araiza B., Murdoch G., Morales A., et al. (2009). Effect of dietary inclusion of benzoic acid on mineral balance in growing pigs. Livestock Sci. 122, 162–168. doi: 10.1016/j.livsci.2008.08.008
Seradj A. R., Balcells J., Morazan H., Alvarez-Rodriguez J., Babot D., De la Fuente G. (2018). The impact of reducing dietary crude protein and increasing total dietary fiber on hindgut fermentation, the methanogen community and gas emission in growing pigs. Anim. Feed Sci. Technol. 245, 54–66. doi: 10.1016/J.ANIFEEDSCI.2018.09.005
Seradj A. R., Balcells J., Sarri L., Fraile L. J., de la Fuente G. (2020). The impact of producing type and dietary crude protein on animal performances and microbiota together with greenhouse gases emissions in growing pigs. Animals 10, 1–16. doi: 10.3390/ani10101742
Suiryanrayna M. V. A. N., Ramana J. V. (2015). A review of the effects of dietary organic acids fed to swine. J. Anim. Sci. Biotechnol. 6, 1–11. doi: 10.1186/s40104-015-0042-z
Ti C., Xia L., Chang S. X., Yan X. (2019). Potential for mitigating global agricultural ammonia emission: A meta-analysis. Environ. pollut. 245, 141–148. doi: 10.1016/j.envpol.2018.10.124
Torrallardona D., Badiola I., Broz J. (2007). Effects of benzoic acid on performance and ecology of gastrointestinal microbiota in weanling piglets. Livestock Sci. 108, 210–213. doi: 10.1016/j.livsci.2007.01.062
Trabue S. L., Kerr B. J., Scoggin K. D., Andersen D., van Weelden M. (2020). Swine diets impact manure characteristics and gas emissions: part I protein level. Sci. Total Environ. 755, 142528. doi: 10.1016/j.scitotenv.2020.142528
Trabue S. L., Kerr B. J., Scoggin K. D., Andersen D., van Weelden M. (2021). Swine diets impact manure characteristics and gas emissions: Part I protein level. Sci. Total Environ. 755, 142528. doi: 10.1016/J.SCITOTENV.2020.142528
Tullo E., Finzi A., Guarino M. (2019). Review: environmental impact of livestock farming and precision livestock farming as a mitigation strategy. Sci. Total Environ. 650, 2751–2760. doi: 10.1016/J.SCITOTENV.2018.10.018
van der Peet-Schwering C. M. C., Aarnink A. J. A., Rom H. B., Dourmad J. Y. (1999). Ammonia emissions from pig houses in the Netherlands, Denmark and France. Livestock Product. Sci. 58, 265–269. doi: 10.1016/S0301-6226(99)00017-2
Wang D., Chen G., Li W., Chai M., Zhang H., Su Y. (2023). Effects of low protein diet on production performance and intestinal microbial composition in pigs. Vet. Sci. 10, 655. doi: 10.3390/vetsci10110655
Wang H., Long W., Chadwick D., Velthof G. L., Oenema O., Ma W., et al. (2020). Can dietary manipulations improve the productivity of pigs with lower environmental and economic cost? A global meta-analysis. Agricult. Ecosyst. Environ. 289, 106748. doi: 10.1016/j.agee.2019.106748
Wang Y., Zhou J., Wang G., Cai S., Zeng X., Qiao S. (2018). Advances in low-protein diets for swine. J. Anim. Sci. Biotechnol. 9, 60. doi: 10.1186/s40104-018-0276-7
Wei Z., Ying H., Guo X., Zhuang M., Cui Z., Zhang F. (2020). Substitution of mineral fertilizer with organic fertilizer in maize systems: a meta-analysis of reduced nitrogen and carbon emissions. Agronomy 10, 1149. doi: 10.3390/agronomy10081149
Woyengo T. A., Sands J. S., Guenter W., Nyachoti C. M. (2008). Nutrient digestibility and performance responses of growing pigs fed phytase- and xylanase-supplemented wheat-based diets. J. Anim. Sci. 86, 848–857. doi: 10.2527/jas.2007-0018
Xia L., Lam S. K., Yan X., Chen D. (2017). How does recycling of livestock manure in agroecosystems affect crop productivity, reactive nitrogen losses and soil carbon balance? Environ. Sci. Technol. 51, 7450–7457. doi: 10.1021/acs.est.6b06470
Xu Y. T., Liu L., Long S. F., Pan L., Piao X. S. (2018). Effect of organic acids and essential oils on performance, intestinal health and digestive enzyme activities of weaned pigs. Anim. Feed Sci. Technol. 235, 110–119. doi: 10.1016/j.anifeedsci.2017.10.012
Zahedi M., Jenner C. (2003). Analysis of effects in wheat of high temperature on grain filling attributes estimated from mathematical models of grain filling. J. Agric. Sci. 141, 203–212. doi: 10.1017/S0021859603003411
Zappaterra M., Catillo G., Lo Fiego D. P., Belmonte A. M., Padalino B., Davoli R. (2022). Describing backfat and semimembranosus muscle fatty acid variability in heavy pigs: analysis of non–genetic factors. Meat Sci. 183, 108645. doi: 10.1016/j.meatsci.2021.108645
Zhang H., Oweis T. Y., Garabet S., Pala M. (1998). Water-use efficiency and transpiration efficiency of wheat under rain-fed conditions and supplemental irrigation in a Mediterranean-type environment. Plant Soil 201, 295–305. doi: 10.1023/A:1004328004860
Zhao Y., Tian G., Chen D., Zheng P., Yu J., He J., et al. (2019). Effect of different dietary protein levels and amino acids supplementation patterns on growth performance, carcass characteristics and nitrogen excretion in growing-finishing pigs. J. Anim. Sci. Biotechnol. 10, 75. doi: 10.1186/s40104-019-0381-2
Keywords: ammonia emission, crude protein, feed additives, field application, slurry, swine
Citation: Fuertes E, Sarri L, Carnicero R, Pérez-Calvo E, Calderón Á, Balcells J, Seradj AR, Cantero-Martínez C, Fernández-Ortega J and de la Fuente G (2025) From feed to field: effect of dietary protein level and use of a blend of feed additives on gaseous emissions from growing-finishing pig slurry. Front. Anim. Sci. 6:1508660. doi: 10.3389/fanim.2025.1508660
Received: 09 October 2024; Accepted: 09 January 2025;
Published: 28 January 2025.
Edited by:
David L. Harmon, University of Kentucky, United StatesReviewed by:
Ravikanth Reddy Poonooru, University of Missouri, United StatesCopyright © 2025 Fuertes, Sarri, Carnicero, Pérez-Calvo, Calderón, Balcells, Seradj, Cantero-Martínez, Fernández-Ortega and de la Fuente. This is an open-access article distributed under the terms of the Creative Commons Attribution License (CC BY). The use, distribution or reproduction in other forums is permitted, provided the original author(s) and the copyright owner(s) are credited and that the original publication in this journal is cited, in accordance with accepted academic practice. No use, distribution or reproduction is permitted which does not comply with these terms.
*Correspondence: Laura Sarri, bGF1cmEuc2FycmlAdWRsLmNhdA==; Gabriel de la Fuente, Z2FicmllbC5kZWxhZnVlbnRlQHVkbC5jYXQ=
‡ORCID: Estefania Pérez-Calvo, orcid.org/0000-0002-1007-7250
Joaquim Balcells, orcid.org/0000-0002-2126-7375
Disclaimer: All claims expressed in this article are solely those of the authors and do not necessarily represent those of their affiliated organizations, or those of the publisher, the editors and the reviewers. Any product that may be evaluated in this article or claim that may be made by its manufacturer is not guaranteed or endorsed by the publisher.
Research integrity at Frontiers
Learn more about the work of our research integrity team to safeguard the quality of each article we publish.