- Nutrition & Health Division, BASF SE, Ludwigshafen am Rhein, Germany
Vitamin A is essential for poultry health, influencing vision, growth, antioxidant defense, reproduction, and immune function. However, it is highly sensitive to degradation when exposed to light, moisture, heat, and oxygen. To address this, vitamin A is typically formulated as retinyl acetate in small beadlets. These beadlets are commonly included in premixes for industrial poultry diets. However, variations in beadlet formulations among different producers can lead to differences in stability and bioavailability, potentially impacting their effectiveness and biological value for poultry. Understanding the stability and bioavailability of various vitamin A sources is crucial for optimizing poultry feeding strategies and ensuring adequate vitamin A supply. The stability of vitamin A can be evaluated through several methods, including premix stability, stability during pelleting, storage stability in feed, and the stability of pure vitamin A products. Bioavailability, which reflects how effectively vitamin A is absorbed and utilized by the animal, is typically assessed through in vivo studies, with liver retinol levels serving as a key indicator. To enhance poultry production, it is important to select vitamin A sources that offer both high stability and bioavailability. Effective feed management, including the use of stable and readily absorbed forms of vitamin A, can improve poultry health, growth rates, and overall production efficiency.
1 Introduction
Poultry farming is increasingly challenged by the need for sustainable production practices and optimal micronutrient management, driven by significant advancements in performance and metabolic rates over the past three decades (Korver, 2023). Vitamin A, comprising retinol, retinal and retinoic acid, plays a critical role in poultry health, impacting vision, growth, antioxidant status, reproduction, and immune function (Cortes et al., 2006; Shastak and Pelletier, 2023a; Guo et al., 2023). Consequently, it is often considered the most important vitamin by animal and veterinary nutritionists (McDowell, 2000; Silver, 2024; Shastak et al., 2024).
Vitamin A is highly susceptible to degradation when exposed to light, humidity, heat, and oxygen, making it one of the most sensitive vitamins (Allwood, 1982; Shurson et al., 2011; Galli et al., 2024). To mitigate this instability, vitamin A is commonly formulated as retinyl acetate in small solid beadlet particles or, alternatively, in a dispersible spray-dried formulation by various suppliers (Figure 1). These beadlets are universally supplemented via premixes into all industrially produced poultry diets. However, the formulation is unique for each producer, leading to variations in the stability and bioavailability of vitamin A products, which can affect their efficiency and biological value for birds (Hirai et al., 2023; Shastak and Pelletier, 2023a; Table 1).
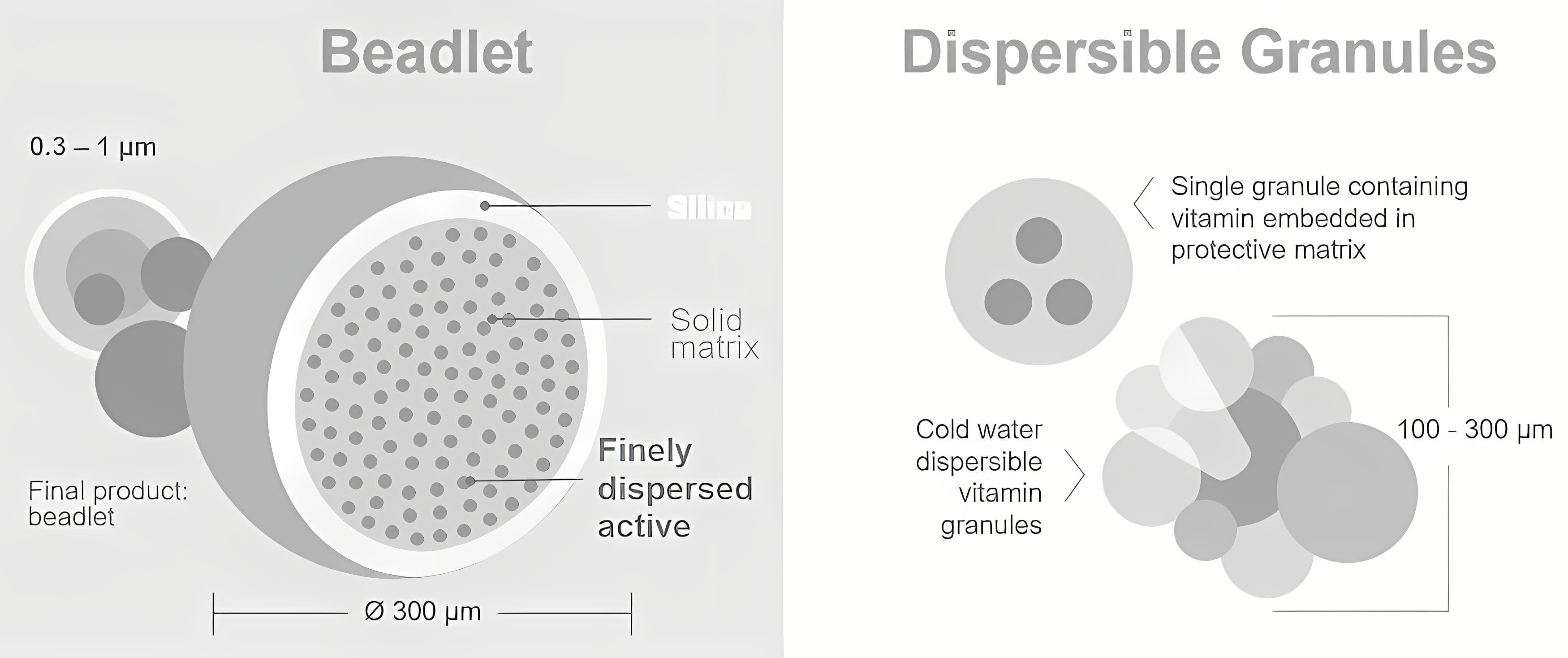
Figure 1. Structure of a beadlet and dispersible spray-dried formulation containing vitamin A acetate.
This mini review aims to summarize the limited published knowledge on the different sources of vitamin A used in poultry diets, their stability, bioavailability, and the factors influencing it. Understanding these key aspects is crucial for developing effective feeding strategies and ensuring optimal vitamin A provision, thereby enhancing poultry health and productivity.
2 Importance of vitamin A in metabolism and dietary recommendations
In poultry, vitamin A plays a critical role in maintaining proper vision and overall health. It serves as a precursor to retinal, which is a key component of rhodopsin, the visual pigment essential for low-light vision (Bridges et al., 1987). Additionally, vitamin A is crucial for protecting the cornea and conjunctiva, preventing dryness, and ensuring adequate tear production by the lacrimal glands (Lima and Souza, 2018). A deficiency in vitamin A can result in night blindness and, in severe cases, complete blindness, impairing a bird’s ability to forage and navigate (Shastak and Pelletier, 2023b).
Vitamin A, sometimes referred to as the “anti-infective” vitamin, is also vital for immune function. It maintains the integrity and functionality of epithelial tissues, which act as the primary barrier against pathogens (Fan et al., 2015). Additionally, vitamin A influences immune responses by promoting the differentiation of regulatory T cells, modulating cytokine production, and enhancing the activity of innate immune cells, thereby contributing to immune homeostasis (Chang and Hou, 2015; Oliveira et al., 2018).
Furthermore, vitamin A is essential for cellular differentiation and tissue development. The active form of vitamin A, retinoic acid, regulates cell cycle progression, particularly during the G1 phase, by activating genes that promote cell division and inhibiting those associated with cell cycle arrest (Qiu et al., 2020; Ma et al., 2023).
Vitamin A also supports the activity of several antioxidant enzymes, including superoxide dismutase and catalase, through its influence on gene expression (Ahlemeyer et al., 2001). In addition, it is crucial for reproductive processes in domestic fowl. In males, vitamin A promotes spermatogenesis by stimulating spermatogonia, while in females, it supports oocyte maturation, ovulation, and transport through the oviducts, all via the retinoic acid signaling pathway (Abdelnour et al., 2019; Shastak and Pelletier, 2023c).
Poultry diets should include sufficient levels of vitamin A, typically achieved through the inclusion of vitamin A supplements via premixes. The recommended level of vitamin A from breeding companies currently ranges between 8,000 and 15,000 IU per kg of feed, depending on species, age, physiological state, and function. For detailed numbers on requirements and recommendations, the reader is referred to the paper by Shastak and Pelletier (2023b).
3 Measurement of stability
Stability refers to the ability of retinyl acetate in a formulated dry product (such as beadlets or spray-dried powders) to retain its chemical integrity and potency over time under various environmental conditions. Key factors influencing stability include exposure to light, heat, oxygen, oxidizing and reducing agents (e.g., trace elements), pH, moisture, pressure (during processes like pelleting and extrusion) as well as other feed processing parameters, all of which can lead to the degradation and loss of vitamin activity (Riaz et al., 2009; Galli et al., 2024).
The stability of different sources of vitamin A is typically assessed through several approaches:
1. Premix Stability: This involves evaluating the stability of vitamin A in premixes with varying compositions, such as vitamin-only premixes, vitamin-mineral premixes with or without choline chloride, and vitamin-mineral premixes containing organically bound forms of zinc, iron, copper, and manganese (Hirai et al., 2023). The premixes are tested under different ambient temperatures, relative humidity levels, and storage durations (Yang et al., 2021).
2. Pelleting Process Stability: This assesses the retention of retinol activity during the feed pelleting process by comparing the vitamin A content in the pelleted feed to that in the mash feed prior to pelleting (Wang et al., 2022).
3. Storage Stability in Feed: This evaluates the stability of vitamin A in feed during storage under different temperatures and relative humidity conditions (Kostadinovic et al., 2014).
4. Pure Vitamin A Product Stability: This involves testing the stability of pure vitamin A products under elevated temperatures and relative humidity to understand how these conditions affect their longevity and effectiveness (Yang et al., 2022).
Hirai et al. (2023) evaluated the stability of four commercial vitamin A sources in a broiler vitamin-mineral premix, including choline chloride, over 56 days at 35°C and 60–70% r.h. Products A and B lost 51% and 56% of their activity after 28 days, respectively. In contrast, products C and D lost 80% and 96%, showing significantly higher degradation (P < 0.0001). After 56 days, products A and B maintained similar activity levels as at 28 days, while products C and D showed further degradation to 91% and 99% losses, respectively, remaining significantly worse (P < 0.0001) than products A and B.
A parallel evaluation was conducted using the same premixes stored in a refrigerator at 4°C for 56 days. After 28 days, products A, B, and C exhibited a loss of 5%, 5%, and 9% of their activity, respectively. In contrast, product D experienced a significant degradation, with a 58% loss in activity (P < 0.0001). After 56 days, products A, B, and C showed further reductions in activity of 42%, 41%, and 43% compared to their activity at 28 days. Product D, however, lost an additional 12%, demonstrating significantly lower stability (P < 0.0001) compared to products A, B, and C. These results clearly demonstrate that the stability of vitamin A sources in premixes varies among different suppliers, depending on storage temperature and duration. The observed differences are likely due to variations in formulation.
4 Evaluation of bioavailability
The bioavailability of vitamin A pertains to the proportion of ingested retinol, whether in the form of retinyl acetate or other esters, that is absorbed and effectively utilized by the body. In vivo studies are the primary method for evaluating bioavailability (Rana et al., 2021; Liao et al., 2024). Poultry are fed diets supplemented with incremental quantities of retinyl acetate, and liver, blood, or other biological samples are collected after the experimental phase to measure retinol levels (Sacakli et al., 2024a). Generally, higher concentrations of retinol in the liver or blood indicate better bioavailability of the vitamin A source. The liver is considered the gold standard for evaluating vitamin A bioavailability, as it stores approximately 90% of the body’s retinol reserves (Idi et al., 2007; Tanumihardjo et al., 2016).
The statistical calculation of relative bioavailability often employs the slope-ratio method, as outlined by Finney (1964). This method involves comparing the slopes of dose-response curves from different treatments to assess their relative potency. Key aspects of the slope-ratio method include: (1) a linear dose-response relationship, meaning the response increases proportionally with dose, (2) parallelism of slopes, which assumes that treatments act through similar mechanisms and produce comparable responses, differing mainly in potency, (3) the ability to compare slopes to determine relative potency, and (4) precision and reliability, provided that linearity and parallelism assumptions are met.
The depletion-repletion approach is preferred for bioavailability studies because it establishes a deficiency baseline by depleting vitamin A stores, allowing for precise measurement of how effectively different sources replenish vitamin A (Liu et al., 2013). This method directly compares repletion rates and ensures that observed effects are attributable to the vitamin A sources, minimizing confounding factors and providing accurate comparative data.
Recent research on broilers suggests that continuous vitamin A supplementation might better match observed data compared to a depletion-repletion approach (Sacakli et al., 2024b). In the study, broilers were fed incremental levels of vitamin A (0, 2000, 4000, or 8000 IU/kg feed) either continuously (days 1-22) or with a depletion-repletion method (depletion days 1-14, repletion days 15-22). On day 23, liver samples from three chicks per pen were analyzed for retinol. The continuous approach had a higher goodness of fit (R² = 0.991) than the depletion-repletion approach (R² = 0.975), indicating a better match. For adult birds like laying hens, a depletion-repletion approach is still preferred due to their ability to store sufficient vitamin A from previous intake.
Data from studies on vitamin A in rats have shown differences in the relative bioavailability of various vitamin A sources, as indicated by liver retinol levels (Rana et al., 2021; Liao et al., 2024). However, similar data for poultry have not yet been generated. Rana et al. (2021) also evaluated the digestibility and retention of different vitamin A sources. While there was a correlation between the bioavailability ranking based on liver retinol levels and the rankings for digestibility and retention, these rankings were not identical.
One challenge in evaluating the digestibility and retention of vitamin A is that unprotected forms, such as retinyl acetate, if not absorbed, can degrade in the digestive tract after being released from the formulation (Buehler, 2001). This degradation can affect the accuracy of measurements of digestibility and retention, potentially leading to overestimation of these values. Therefore, when measuring vitamin A retention or digestibility, it is important to consider the potential degradation of unprotected retinyl esters in the digestive tract and during extraction from feces.
The absorption and utilization of vitamin A and other fat-soluble vitamins are crucial for ensuring their optimal bioavailability. Several factors can impact this process, including species-specific differences, gut health disorders, parasitic infections, imbalances or deficiencies in other nutrients (such as dietary fat and other fat-soluble vitamins), stress, mycotoxins in feed, and certain diseases. These factors can collectively impair the absorption of retinol in the digestive tract (Leeson and Summers, 2001; Shastak and Pelletier, 2023b).
Finally, bioavailability is closely related to another term, bioaccessibility. Bioaccessibility refers to the fraction of vitamin A or other bioactive compounds that is released from its formulation matrix during digestion and becomes available for absorption in the gut (Rodrigues et al., 2022). It measures the potential amount of a micronutrient that the body can absorb and use after consuming a vitamin supplement. The bioaccessibility of a vitamin A product is directly influenced by its formulation; making the product too stable for premix and feed processing steps can reduce its bioavailability to the animal.
5 Practical implications for poultry production
In animal production, the choice of vitamin A sources and their stability and bioavailability have substantial practical implications for the health and efficiency of the livestock (Hu et al., 2020; Hirai et al., 2023; Sacakli et al., 2024a, b). In the end, what truly matters is not just the initial quantity of vitamin A planned for the animal, but rather the actual amount that reaches the animal and the bioavailability of the vitamin form used.
Vitamin A stability varies among different commercial sources due to differences in formulations provided by various suppliers (Shastak et al., 2024). This instability can lead to a significant decrease in the vitamin’s activity over time, posing a risk of deficiencies if not managed properly (Hirai et al., 2023).
Proper storage and handling of premixes and feeds are essential to preserve vitamin A and other vitamins (Galli et al., 2024). Premixes and feeds should be kept in cool, dry environments and protected from light exposure to prevent vitamin breakdown (Menegat et al., 2019). Additionally, incorporating antioxidants into premixes and feed can help stabilize fat-soluble vitamins, mitigating degradation effects (Desbruslais and Wealleans, 2022). Understanding and managing these factors ensure that poultry receive a steady supply of vitamin A, supporting their health and growth.
Bioavailability is another critical factor influencing the effectiveness of vitamin A supplementation due to several reasons:
1. Absorption Efficiency: Bioavailability determines how well vitamin A is absorbed in the animal’s digestive tract (Cerqueira et al., 2017). Even if feed contains sufficient vitamin A, it will not be effective if the vitamin A source is not properly absorbed. Efficient absorption ensures that the birds receive the full nutritional benefit of the supplemented vitamin.
2. Physiological Function Support: Vitamin A is essential for various physiological functions. Adequate bioavailability ensures sufficient active vitamin A is available to support these functions, maintaining the well-being and productivity of the poultry (Alagawany et al., 2020). Poor bioavailability can lead to deficiencies, compromising the birds’ overall health and reducing production efficiency.
3. Commercial Value: Vitamin A supplements with higher bioavailability often provide higher commercial value (Shastak and Pelletier, 2023c). Feed producers can use lower doses of more bioavailable forms of vitamin A to achieve the desired physiological vitamin A status, which can potentially reduce feed costs. Alternatively, using a more bioavailable vitamin A source at the same supplementation level can offer a higher safety margin under field conditions, where birds are frequently exposed to increased physiological demands due to higher stress and pathogenic pressures. Conversely, less bioavailable sources may require higher doses, increasing costs and leading to micronutrient waste.
4. Growth and Development: During critical growth phases, adequate vitamin A is essential for optimal development (Cortes et al., 2006; Feng et al., 2019). Bioavailable sources ensure that growing poultry receive necessary micronutrients to reach their full genetic potential, resulting in better growth rates and higher yields. This is particularly important in commercial operations where growth performance directly impacts profitability (Wongnaa et al., 2023).
Thus, to optimize poultry production, it is vital to select vitamin A sources that are both stable and bioavailable. Effective feed management, including the use of stable and easily absorbed forms of vitamin A, enhances poultry health and improves growth rates, ultimately supporting more efficient production systems.
6 Conclusions and perspectives
In conclusion, the stability and bioavailability of vitamin A in poultry nutrition play crucial roles in ensuring optimal health, growth, and productivity. The stability of vitamin A sources can vary among different suppliers, highlighting the importance of selecting reliable sources with proven stability. Proper storage and handling practices are essential to preserve the activity of vitamin A in premixes and feeds (Tavcar-Kalcher and Vengust, 2007; Menegat et al., 2019).
Bioavailability, determined by factors such as absorption efficiency and physiological function support, directly influences the effectiveness of vitamin A supplementation (Combs and McClung, 2017). Choosing vitamin A sources with higher bioavailability can lead to improved commercial value, growth, and development in poultry. It allows for better utilization of the supplemented vitamin A and ensures the provision of adequate levels for physiological needs.
Future research should continue to explore ways to enhance the stability and bioavailability of vitamin A in poultry diets. This includes investigating novel formulations and delivery systems that optimize absorption and utilization (Zhang et al., 2021). Additionally, further studies are needed to assess the impact of vitamin A stability and bioavailability on specific poultry populations, such as broilers, turkeys, ducks, layers, and breeders, to tailor nutritional strategies accordingly.
Overall, understanding and managing the stability and bioavailability of vitamin A in poultry nutrition are crucial for achieving sustainable and efficient production practices (Choi et al., 2023; Shastak and Pelletier, 2023c). By ensuring the availability of stable and bioavailable vitamin A sources, the poultry industry can enhance the health, welfare, and performance of birds, contributing to the production of high-quality and nutritious poultry products.
Author contributions
YS: Conceptualization, Investigation, Writing – original draft, Writing – review & editing. WP: Data curation, Investigation, Validation, Writing – review & editing.
Funding
The author(s) declare that no financial support was received for the research, authorship, and/or publication of this article.
Conflict of interest
Authors YS and WP were employed by the company BASF SE.
Publisher’s note
All claims expressed in this article are solely those of the authors and do not necessarily represent those of their affiliated organizations, or those of the publisher, the editors and the reviewers. Any product that may be evaluated in this article, or claim that may be made by its manufacturer, is not guaranteed or endorsed by the publisher.
References
Abdelnour S. A., Abd El-Hack M. E., Swelum A. A., Saadeldin I. M., Noreldin A. E., Khafaga A. F., et al. (2019). The usefulness of retinoic acid supplementation during in vitro oocyte maturation for the in vitro embryo production of livestock: A review. Animals 9, 561. doi: 10.3390/ani9080561
Ahlemeyer B., Bauerbach E., Plath M., Steuber M., Heers C., Tegtmeier F., et al. (2001). Retinoic acid reduces apoptosis and oxidative stress by preservation of SOD protein level. Free. Radic. Biol. Med. 30, 1067–1077. doi: 10.1016/s0891-5849(01)00495-6
Alagawany M., Elnesr S. S., Farag M. R., Tiwari R., Yatoo M. I., Karthik K., et al. (2020). Nutritional significance of amino acids, vitamins and minerals as nutraceuticals in poultry production and health - a comprehensive review. Vet. Q. 41, 1–29. doi: 10.1080/01652176.2020.1857887
Allwood M. C. (1982). The influence of light on vitamin A degradation during administration. Clin. Nutr. 1, 63–70. doi: 10.1016/0261-5614(82)90006-1
Bridges C. D., Alvarez R. A., Fong S. L., Liou G. I., Ulshafer R. J. (1987). Rhodopsin, vitamin A, and interstitial retinol-binding protein in the rd chicken. Invest. Ophthalmol. Vis. Sci. 28, 613–617. doi: 10.1016/0042-6989(88)90106-x
Buehler V. (2001). Vademecum for vitamin formulations, 2nd revised edition. (Stuttgart, Germany: Wissenschaftliche Verlagsgesellschaft mbH), 144.
Cerqueira M., Pinheiro A. P. R., do Carmo A. C. B., Duarte C. V. S., da Cunha C. M. M., Vicente M., et al. (2017). “Nanostructured biobased systems for nutrient and bioactive compounds delivery,” in Nutrient Delivery (New York, United States: Elsevier Inc.), 43–85. doi: 10.1016/b978-0-12-804304-2.00002-0
Chang H.-K., Hou W.-S. (2015). Retinoic acid modulates interferon-γ Production by hepatic natural killer T cells via phosphatase 2A and the extracellular signal-regulated kinase pathway. J. Interferon Cytokine Res. 3, 200–212. doi: 10.1089/jir.2014.0098
Choi J., Kong B., Bowker B. C., Zhuang H., Kim W. K. (2023). Nutritional strategies to improve meat quality and composition in the challenging conditions of broiler production: A review. Animals 13, 1386. doi: 10.3390/ani13081386
Combs G. F., McClung J. P. (2017). “Sources of the Vitamins,” in The Vitamins. Eds. Combs G. F., McClung J. P. (Academic Press, London, UK), 501–530. doi: 10.1016/B978-0-12-802965-7.00020-4
Cortes P. L., Tiwary A. K., Puschner B., Crespo R. M., Chin R. P., Bland M., et al. (2006). Vitamin A deficiency in Turkey poults. J. Vet. Diagn. Invest. 18, 489–494. doi: 10.1177/104063870601800514
Desbruslais A., Wealleans A. L. (2022). Oxidation in poultry feed: impact on the bird and the efficacy of dietary antioxidant mitigation strategies. Poultry 1, 246–277. doi: 10.3390/poultry1040022
Fan X., Liu S., Liu G., Zhao J., Jiao H., Wang X., et al. (2015). Vitamin A deficiency impairs mucin expression and suppresses the mucosal immune function of the respiratory tract in chicks. PloS One 10, e0139131. doi: 10.1371/journal.pone.0139131
Feng Y. L., Xie M., Tang J., Huang W., Zhang Q., Hou S. S. (2019). Effects of vitamin A on growth performance and tissue retinol of starter White Pekin ducks. Poult. Sci. 98, 2189–2192. doi: 10.3382/ps/pey571
Finney D. J. (1964). Statistical Method in Biological Assay. 2nd ed (London, UK: Charles Griffin & Co.), 101.
Galli G. M., Andretta I., Martinez N., Wernick B., Shastak Y., Gordillo A., et al. (2024). Stability of vitamin A at critical points in pet-feed manufacturing and during premix storage. Front. Vet. Sci. 11. doi: 10.3389/fvets.2024.1309754
Guo S., He L., Zhang Y., Niu J., Li C., Zhang Z., et al. (2023). Effects of vitamin A on immune responses and vitamin A metabolism in broiler chickens challenged with necrotic enteritis. Life 13, 1122. doi: 10.3390/life13051122
Hirai R. A., De Leon D., Randig-Biar M., Silva A., Sanchez E., McElro,y A. P., et al. (2023). “Evaluation of the stability of vitamin A acetate concentrates mixed in a vitamin-trace mineral premix over a 56-day high temperature and humidity storage stress,” in The International Poultry Scientific Forum 2023, Atlanta, Georgia, USA. (Lincoln, Nebraska, USA: Southern Poultry Science Society), 112.
Hu Y., Zhang L., Zhang Y., Xiong H., Wang F., Wang Y., et al. (2020). Effects of starch and gelatin encapsulated vitamin A on growth performance, immune status and antioxidant capacity in weaned piglets. Anim. Nutr. 6, 30–133. doi: 10.1016/j.aninu.2020.01.005
Idi A., Permin A., Jensen S., Murrell K. (2007). Effect of a minor Vitamin A deficiency on the course of infection with ascaridia galli (Schrank 1788) and the resistance of chickens. Helminthologia 44, 3–9. doi: 10.2478/s11687-006-0047-4
Korver D. R. (2023). Review: Current challenges in poultry nutrition, health, and welfare. Animal 17, 100755. doi: 10.1016/j.animal.2023.100755
Kostadinović L., Teodosin S., Lević J., Čolović R., Banjac V., Vukmirović Đ., et al. (2014). Effect of pelleting and expanding processes on vitamin A stability in animal feeds. J. Proc. Energy Agric. 18, 44–46.
Leeson S., Summers J. D. (2001). “Commercial Poultry Nutrition,” in Scott’s Nutrition of the Chicken, 3rd ed. Ed. Leeson S. (Nottingham University Press, Nottingham, UK), 398.
Liao Y., Sun L., Fan D., Xu W., Zelikman M. V., Qiao P. (2024). Enhancing the solubility, stability, and bioavailability of retinol acetate through mechanochemistry. Biomacromolecules 25, 3831–3839. doi: 10.1021/acs.biomac.4c00440
Lima H., Souza L. (2018). Vitamin A in the diet of laying hens: Enrichment of table eggs to prevent nutritional deficiencies in humans. World’s Poult. Sci. J. 74, 619–626. doi: 10.1017/s004393391800065x
Liu Y., Shaw J. J., Swaisgood H. E., Allen J. C. (2013). Bioavailability of oil-based and β -lactoglobulin-complexed vitamin A in a rat model. ISRN Nutr. 2013, 270580. doi: 10.5402/2013/270580
Ma Y., Wu W., Zhang Y., Wang X., Wei J., Guo X., et al. (2023). The synchronized progression from mitosis to meiosis in female primordial germ cells between layers and broilers. Genes 14, 781. doi: 10.3390/genes14040781
McDowell L. R. (2000). “Vitamin A,” in Vitamins in Animal and Human Nutrition. Ed. McDowell L. R. (Iowa State University Press, USA), 5–90. doi: 10.1002/9780470376911
Menegat M. B., Goodband R. D., DeRouchey J. M., Tokach M. D., Woodworth J. C., Dritz S. S. (2019). Kansas State University swine nutrition guide: vitamin sources for swine diets. Available online at: https://www.asi.k-state.edu/extension/swine/swinenutritionguide/pdf/KSU%20Vitamin%20Sources%20for%20Swine%20Diets%20fact%20sheet.pdf (Accessed June 29, 2024).
Oliveira L. M., Teixeira F. M. E., Sato M. N. (2018). Impact of retinoic acid on immune cells and inflammatory diseases. Mediators Inflamm. 2018, 1–17. doi: 10.1155/2018/3067126
Qiu J., Nordling S., Vasavada H. H., Butcher E. C., Hirschi K. K. (2020). Retinoic acid promotes endothelial cell cycle early G1 state to enable human hemogenic endothelial cell specification. Cell Rep. 33, 108465. doi: 10.1016/j.celrep.2020.108465
Rana S., Arora S., Gupta C., Bodemala H., Kapila S. (2021). Evaluation of in-vivo model for vitamin A bioavailability from vitamin A loaded caseinate complex. Food Biosc. 42, 101174. doi: 10.1016/j.fbio.2021.101174
Riaz M. N., Asif M., Ali R. (2009). Stability of vitamins during extrusion. Crit. Rev. Food Sci. Nutr. 49, 361–368. doi: 10.1080/10408390802067290
Rodrigues D. B., Marques M. C., Hacke A., Filho P. S., Cazarin C. B. B., Mariutti L. R. B. (2022). Trust your gut: Bioavailability and bioaccessibility of dietary compounds. Curr. Res. Food Sci. 5, 228–233. doi: 10.1016/j.crfs.2022.01.002
Sacakli P., Ramay M. S., Harijaona J. A., Shastak Y., Pelletier W., Gordillo A., et al. (2024a). “Does the latest NASEM vitamin A requirement estimate support optimal growth in broiler chickens?,” in Proceedings of the 16th European Poultry Congress. (Valencia, Spain: Spanish branch of WPSA), 24–28 June 2024.
Sacakli P., Ramay M. S., Harijaona J. A., Shastak Y., Pelletier W., Gordillo A., et al. (2024b). “A comparison of two methodological approaches for evaluating the effect of vitamin A supplementation in broiler chickens,” in The International Poultry Scientific Forum 2024, P 317, Atlanta, Georgia, USA. (Lincoln, Nebraska, USA: Southern Poultry Science Society), 99.
Shastak Y., Pelletier W. (2023a). The role of vitamin A in non-ruminant immunology. Front. Anim. Sci. 4. doi: 10.3389/fanim.2023.1197802
Shastak Y., Pelletier W. (2023b). Delving into vitamin A supplementation in poultry nutrition: current knowledge, functional effects, and practical implications. Worlds Poult. Sci. J. 80, 109–131. doi: 10.1080/00439339.2023.2250327
Shastak Y., Pelletier W. (2023c). Nutritional balance matters: assessing the ramifications of vitamin A deficiency on poultry health and productivity. Poultry 2, 493–515. doi: 10.3390/poultry2040037
Shastak Y., Pelletier W., Kuntz A. (2024). Insights into analytical precision: understanding the factors influencing accurate vitamin A determination in various samples. Analytica 5, 54–73. doi: 10.3390/analytica5010004
Shurson G. C., Salzer T. M., Koehler D. D., Whitne M. H. (2011). Effect of metal specific amino acid complexes and inorganic trace minerals on vitamin stability in premixes. Anim. Feed Sci. Technol. 163, 200–206. doi: 10.1016/j.anifeedsci.2010.11.001
Silver R. J. (2024). Liquid A Drops. Technical report for veterinarian use only. VBS Direct Ltd. 1 Mill View Close, Bulkeley, Cheshire, SY14 8DB. Available online at: https://vbsdirect.co.uk/files/Vitamin_A_Tech_Report2.pdf (Accessed February 10, 2024).
Tanumihardjo S. A., Russell R. M., Stephensen C. B., Gannon B. M., Craft N. E., Haskell M. J., et al. (2016). Biomarkers of nutrition for development (BOND)-vitamin A review. J. Nutr. 146, 1816S–1848S. doi: 10.3945/jn.115.229708
Tavčar-Kalcher G., Vengušt A. (2007). Stability of vitamins in premixes. Anim. Feed Sci. Technol. 132, 148–154. doi: 10.1016/j.anifeedsci.2006.03.001
Wang H., Li L., Zhang N., Zhang T., Ma Y. (2022). Effects of pelleting and long-term high-temperature stabilization on vitamin retention in swine feed. Animals 12, 1058. doi: 10.3390/ani12091058
Wongnaa C. A., Mbroh J., Mabe F. N., Abokyi E., Debrah R., Dzaka E. (2023). Profitability and choice of commercially prepared feed and farmers’ own prepared feed among poultry producers in Ghana. J. Agric. Food Res. 12, 100611. doi: 10.1016/j.jafr.2023.100611
Yang P., Wang H., Li L., Zhang N., Ma Y. (2021). The stability of vitamin A from different sources in vitamin premixes and vitamin-trace mineral premixes. Appl. Sci. 11, 3657. doi: 10.3390/app11083657
Yang H., Xu L., Hou L., Xu T. C., Ye S. H. (2022). Stability of vitamin A, E, C and thiamine during storage of different powdered enteral formulas. Heliyon 8, e11460. doi: 10.1016/j.heliyon.2022.e11460
Keywords: vitamin A, retinol, stability, bioavailability, bioaccessibility, poultry
Citation: Shastak Y and Pelletier W (2024) Vitamin A source variability: a mini review on stability and bioavailability in poultry. Front. Anim. Sci. 5:1484262. doi: 10.3389/fanim.2024.1484262
Received: 21 August 2024; Accepted: 10 September 2024;
Published: 07 October 2024.
Edited by:
Vincenzo Lopreiato, University of Messina, ItalyReviewed by:
Isaac Oluseun Adejumo, University of Ibadan, NigeriaCopyright © 2024 Shastak and Pelletier. This is an open-access article distributed under the terms of the Creative Commons Attribution License (CC BY). The use, distribution or reproduction in other forums is permitted, provided the original author(s) and the copyright owner(s) are credited and that the original publication in this journal is cited, in accordance with accepted academic practice. No use, distribution or reproduction is permitted which does not comply with these terms.
*Correspondence: Yauheni Shastak, eWF1aGVuaS5zaGFzdGFrQGJhc2YuY29t