- 1Department of Animal Science, University of California, Davis, Davis, CA, United States
- 2Department of Pathology, Microbiology and Immunology, School of Veterinary Medicine, University of California, Davis, Davis, CA, United States
- 3Comparative Pathology Laboratory, School of Veterinary Medicine, University of California, Davis, Davis, CA, United States
- 4Department of Food Science and Technology, Oregon State University, Corvallis, OR, United States
Introduction: Percussive stunning is a widely used and ethically supported method of stunning fish per welfare standards as part of a one- or two-step slaughter process. In this study, the use of a non-penetrative captive bolt (NPCB) gun was evaluated as an effective one-step method of improving welfare for juvenile and adult farmed white sturgeon (Acipenser transmontanus) at slaughter.
Methods: A Jarvis HPS-1 NPCB was operated at pressures of 120, 135, and 145 PSI (827.37, 930.79, and 999.74 kPa, respectively) for juvenile sturgeon (n = 3 sturgeon per operating pressure) and 175, 200, and 225 PSI (1206.58, 1378.95, and 1551.32 kPa, respectively) for adult sturgeon (n = 3 sturgeon per operating pressure). Following a single strike, fish were assessed for jaw relaxation and a somatic response before being exsanguinated and transferred to an ice slurry. An hour after slaughter, fish heads were collected, and a section of cartilage containing the brain was removed and fixed in formalin for histological analysis of brain death. To evaluate fish recovery, juveniles (n = 100) and adult female sturgeon (n = 65) were monitored for two hoursafter a single exposure to 145 and 225 PSI, respectively.
Results: Histology results showed there was an effect between operating pressure and intracranial hemorrhage in juvenile sturgeon (p = 0.024). There was a greater meningeal-to-cerebral hemorrhage at 135 PSI compared to the 120 PSI group (p = 0.020) and a trend towards increased tissue damage from 120 PSI to 145 PSI (p = 0.056). Adults showed no significant difference in meningeal hemorrhage at any operating pressure. When investigating recovery rates, NPCB successfully stunned 100% of juvenile sturgeon at 145 PSI, and 225 PSI stunned 90% of adult sturgeon without recovery.
Discussion: These results demonstrate that the use of an NPCB gun is an improvement in animal welfare compared to repeated strikes, but a single application did not produce histological brain death; further research should be conducted to determine optimal pressures that result in immediate brain death.
1 Introduction
The global sturgeon (Acipenser spps.) farming industry is experiencing significant growth due to the increasing demand for caviar and fish protein (Bronzi et al., 2011; Bronzi et al., 2019). Enhancing the efficiency of fish production and improving fish welfare on-farm, particularly during sturgeon harvest and slaughter, are essential to ensure the sustainability of sturgeon aquaculture. The growing demand for sturgeon caviar production has increased the availability of sturgeon meat (FAO, 2024). However, caviar, fresh fish, and fish by-products are highly perishable and prone to spoilage, primarily caused by microbial growth and oxidation, leading to changes in product odor, flavor, and texture (Buzby et al., 2014; Cheng et al., 2015; Prabhakar et al., 2020; Zhang et al., 2021). The loss of quality of fresh fish products, resulting in reduced shelf life and nutritional value, is a critical cause of food loss and waste in the fish industry. Retailers often discard edible fish and muscle-based products based on appearance or color (Buzby et al., 2014; Love et al., 2014; Aschemann-Witzel et al., 2015). It is estimated that 30% of seafood and fish products (equivalent to 1.9 billion pounds) are lost from the food supply chain at the retail and consumer level, amounting to a fiscal loss of 9.9 billion USD (Buzby et al., 2014).
Enhancing the quality and shelf life of meat products can result in significant economic benefits, as it allows for a longer time to market the product. Pre-harvest factors, such as stress and handling, can profoundly impact fish quality and shelf life by increasing muscle activity (Green, 2010). Reducing stress during harvesting can delay the onset of rigor development, thereby extending the shelf life and maintaining the quality of fresh fish (Poli et al., 2005; Buzby et al., 2014; Braitenbach Cavali et al., 2024) and ultimately reducing food waste. The onset of rigor mortis can have detrimental effects on fish muscle, causing texture deterioration, gaping, discoloration, dehydration, and reduced shelf life (Boggess et al., 1973; Azam et al., 1990; Marx et al., 1997; Robb et al., 2000; Skjervold et al., 2001; Roth et al., 2002; Kiessling et al., 2004; Daskalova, 2019). Typically, rigor mortis starts in bony fish approximately 8 to 24 hours post-mortem, but the onset timing of rigor mortis varies among fish species and can be affected by their age and culture conditions (Berg et al., 1997). Extending the shelf life and improving the quality of fresh and frozen fish products are crucial for reducing economic loss and sustaining the growth and profitability of U.S. fish producers, but these aspects still need to be systematically studied in sturgeon.
Due to the enormous diversity of fish species, variability in anatomy, and size ranges among farmed fish, there is a lack of research and extension materials available for producers to apply best practices concerning slaughter techniques that improve animal welfare for many farmed species. When considering best aquaculture practices for fish slaughter, organizations such as the American Veterinary Medical Association (AVMA) recommend that a humane slaughter protocol ensure that fish are insensible before, or concurrent with, the final termination step and any subsequent invasive processing methods (AVMA, 2016). This slaughter process can be accomplished as either a one-step or a two-step method, depending on the slaughter methods selected for a given species. A single-step method must render the animal insensible at the same time as killing them. In a two-step method, the animal is rendered insensible before the second termination step. Currently, there are a variety of methods approved by the AVMA for the humane slaughter of food fish, including decapitation, cervical transection, manual or automated percussion, including non-penetrative captive bolt, followed by pithing or exsanguination. Electrical stunning is an AVMA-approved method for humane stun and slaughter of fish with or without exsanguination (AVMA, 2016).
Currently, the majority of U.S. sturgeon farms employ a two-step slaughter method, which includes physical damage to the brain to induce unconsciousness followed by exsanguination (J. Gross, personal observation). Manual blunt force trauma is used on most farms across the U.S. However, three of the largest California farms have adopted the use of a non-penetrative bolt gun (NPCB). When performed optimally, both manual stunning and NPCB can render a sturgeon insensible within 1 second of application. Both methods require the removal of the fish from the water and careful placement of the striking stimulus. It can be challenging, especially for adult white sturgeon (A. transmontanus), to manually generate the force needed to cause resulting brain damage due to the sturgeon’s thick, cartilaginous skull compared to the anatomy of bony fish species such as Atlantic salmon (Salmo salar) (Roth et al., 2007), the common carp (Cyrimus carpio) (Retter et al., 2018), and catfish (Clarias gariepinus) (Brijs et al., 2021). To be effective, a large amount of force must be directed to a small area above the brain of the sturgeon. Some brands of NPCB can generate the force required to render a sturgeon insensible, and with training, NPCB can repeatedly produce a consistent, high-pressure concussion, effectively rendering the sturgeon insensible without the need for repeated strikes. To maximize the welfare of these high-value fish, more effective methods must be developed and utilized to meet the minimum requirements for humane slaughter.
This study assessed the efficacy of NPCB stunning as a humane slaughter method for white sturgeon, addressing the need for improved welfare practices in aquaculture across the western United States. White sturgeon, the largest freshwater fish in North America, are predominantly farmed in California and Idaho for meat and caviar (USDA NASS, 2018) and are of particular concern due to their size and cartilaginous anatomy. By examining the effectiveness of NPCB stunning in both juvenile and adult sturgeon, this research aims to highlight potential animal welfare and occupational safety within the aquaculture industry. Additionally, histological examination provides tissue-level detail on the extent of physical damage to brain tissue. The histological examinations validate the challenges of applying NPCB stunning to cartilaginous fish like sturgeon and establish a foundational baseline for advancing humane slaughter practices that meet industry sustainability goals and welfare standards.
2 Materials and methods
2.1 Animal husbandry
Fish utilized in this study (n = 110 juvenile white sturgeon, n = 75 adult white sturgeon) were slaughtered during regular on-farm practices at Sterling Caviar in Elverta, CA, as part of standard operating procedures. Traditionally, juvenile male sturgeon are delivered to the processing plant by truck and dewatered in 91.4 x 121.9 x 91.4 cm totes. Fish are immediately slaughtered by a two-step process of cranial concussion followed by exsanguination by severing the gills and ventral caudal vasculature. Fish are immediately placed in an ice slurry to complete exsanguination and reduce body temperature for a few hours before processing. For adult caviar-ready females, sturgeon are stunned in totes immediately after removal from their tanks by braile net and exsanguinated as described for juvenile fish. Ovaries are immediately removed to produce caviar. Juvenile and adult carcasses are billeted and filleted immediately and either placed on ice for the fish to complete rigor and processed later or sold whole.
2.2 Stunning study
To determine the appropriate NPCB operating pressures (PSI) for juvenile meat fish and adult caviar-ready white sturgeon, we conducted a dose-response study on adult caviar-ready, 6–9-year-old female white sturgeon (n = 9, mean mass = 27.1 kg) and 2–3-year-old juvenile male white sturgeon (n = 10, mean mass = 8.4 kg).
For cranial concussion, a Jarvis HPS-1 pneumatic NPCB (Bunzyl Processing Division Riverside Missouri, USA) with a 2.5 cm striking surface was pressurized by nitrogen gas, and operating pressures were regulated and monitored by a regulator (RADNOR Nitrogen Purge model GP 250–500-580). Properly applied percussion stunning in sturgeon had previously been characterized and is directed at the hindbrain along the midline between the two spiracles (Conte, 2010) (Figure 1). The caviar-ready females were stunned using compressed nitrogen at 175 PSI (1206.58 kPa), 200 PSI (1378.95 kPa), and 225 PSI (1551.32 kPa), and the juvenile sturgeon were stunned at 120 PSI (827.37 kPa), 135 PSI (930.79 kPa), and 145 PSI (999.74 kPa). After the delivery of a single strike, jaw relaxation in each fish was confirmed before exsanguination. Complete jaw relaxation is a generally accepted visual indicator that insensibility is achieved within the sturgeon aquaculture industry (Figure 2). All fish were exsanguinated as a second-step termination method and placed in an ice slurry.
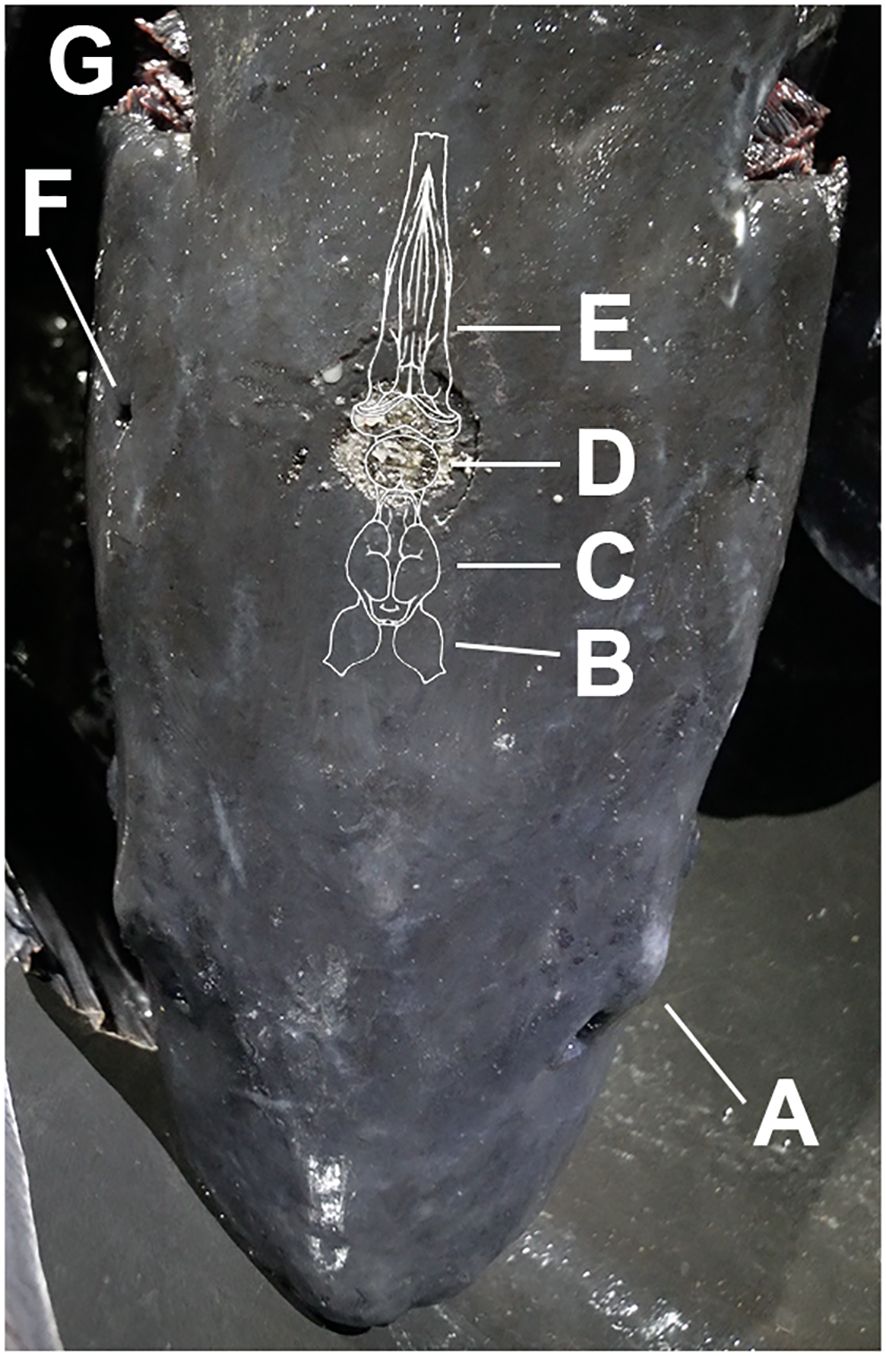
Figure 1 Dorsal image of white sturgeon head with correct midline strike placement between lateral spiracles and brain anatomy. (A) Eye, (B) Olfactory Lobe, (C) Telencephalon, (D) Mesencephalon, (E) Rhombencephalon, (F) Spiracle, (G) Gills.
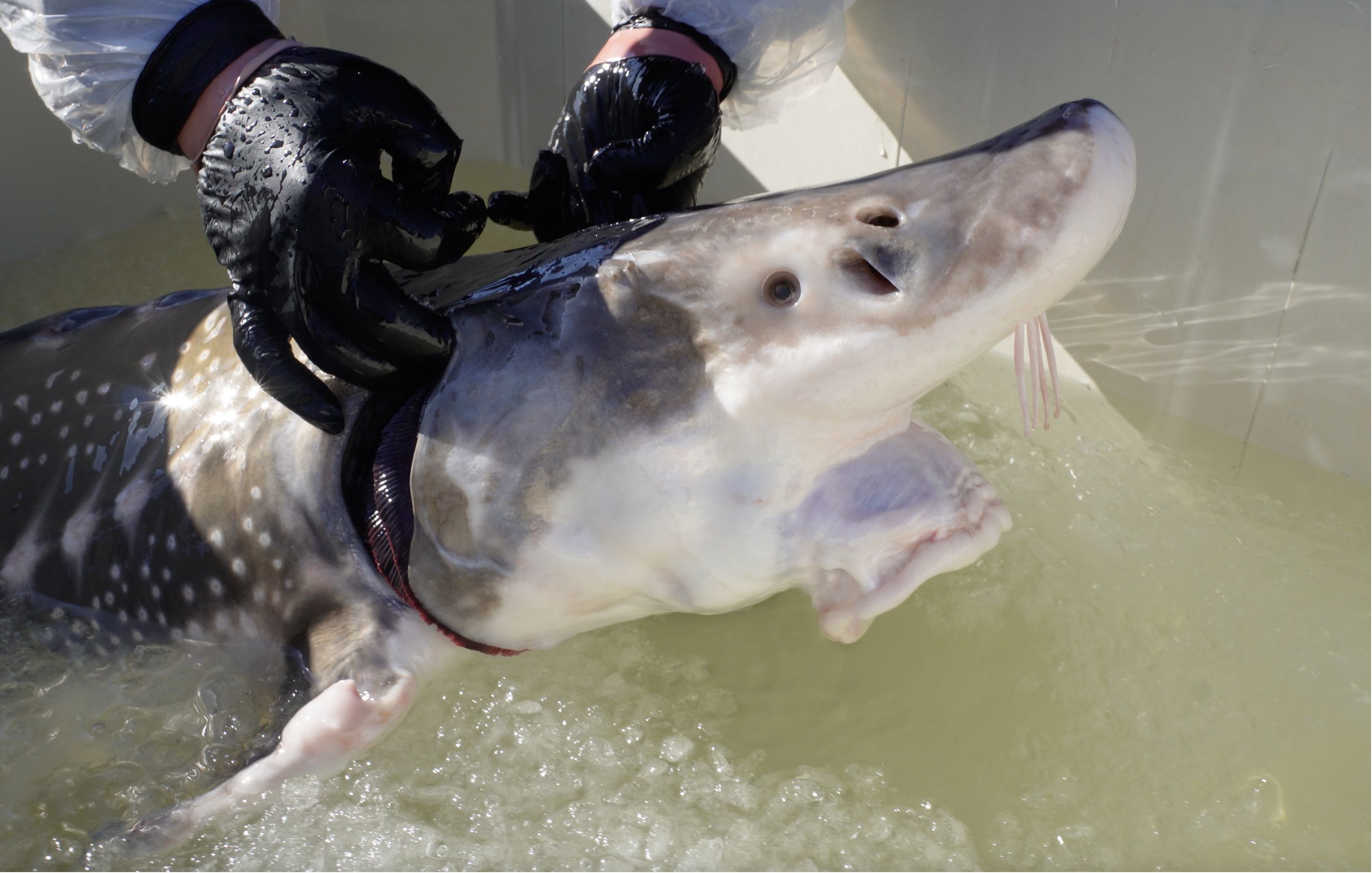
Figure 2 Demonstration of jaw relaxation in adult white sturgeon following a successful strike using a non-penetrating captive bolt gun.
Operating pressures for the non-penetrative captive bolt gun were established based on preliminary trials aimed at minimizing the need for additional stunning strikes, thereby enhancing fish welfare. In California, sturgeon aged 2–3 years are sorted by sex via ultrasound, with males directed to protein harvest and females retained for future caviar production. Consequently, the juveniles selected for this study were predominantly male, while the adults were female. A pressure setting of 145 PSI was chosen for the juveniles because it consistently induced jaw relaxation, as observed during initial farm-based experiments (J. Gross, personal observation). Among adults, pressures of 200 and 225 PSI effectively induced jaw relaxation in a preliminary sample of ten white sturgeon, although the specific mass of these fish was not measured. The control pressure for the study was selected as the lowest operational level identified during pilot testing as sufficient to achieve jaw relaxation with a single application using the HPS-1 NPCB (J. Gross, personal observation), which was an essential consideration for animal welfare aimed at reducing the likelihood of requiring multiple strikes.
All replicate strikes at a single gas pressure (n = 3 juveniles per pressure setting; n = 3 adults per pressure setting) were applied continuously for logistical purposes to avoid disrupting staff and causing prolonged delays in fish processing and reducing the time fish were out of water. Individually selected fish received a single strike, affixed with a colored flagging tape, exsanguinated, and placed in an ice slurry. While this procedure was not randomized, applications at the same regulated pressure reduced the potential for variation in operating pressure between fish. Once a series of replicate fish were completed, gas pressure was increased to the next prescribed setting, and the procedure was repeated. Colored flagging tape, representing the low, medium, and high pressures, was labeled to keep track of individuals during fish processing.
Fish heads were retrieved within an hour after slaughter and placed on ice. The cranium was excised en bloc by reciprocating saw and stored individually in 37% formalin for later analysis by the UC Davis Comparative Pathology Laboratory. Sturgeon brains were later removed after fixation for histopathology analysis.
2.3 Histological methods
Coronal sections of mesencephalon and rhombencephalon with surrounding meningeal adipose and hematopoietic tissue were collected (n = 3 juveniles per pressure setting; n = 3 adults per pressure setting). Where present, sections of telencephalon were included. Formalin-fixed brain samples were processed routinely, embedded in paraffin, sectioned at 5μM, and stained with hematoxylin and eosin. Board-certified veterinary anatomic pathologists evaluated tissue sections for the presence of tissue damage (hemorrhage, cartilage necrosis, axonal and neuronal swelling, or edema) that would be consistent with acute traumatic brain injury (Xiong et al., 2013). A semi-quantitative grading system was used to estimate the severity of intracranial hemorrhage: 0 - feature is absent, 1 - mild to moderate hemorrhage in meninges, 2 - mild to moderate hemorrhage in the meninges and extending into the neural parenchyma, 3 - severe hemorrhage, defined as larger pools of hemorrhage effacing regions of the parenchyma or disrupting architecture (Figure 3). A binary grading system was applied to the remaining features of acute brain injury, including cartilage necrosis, axonal and neuronal swelling, and edema.
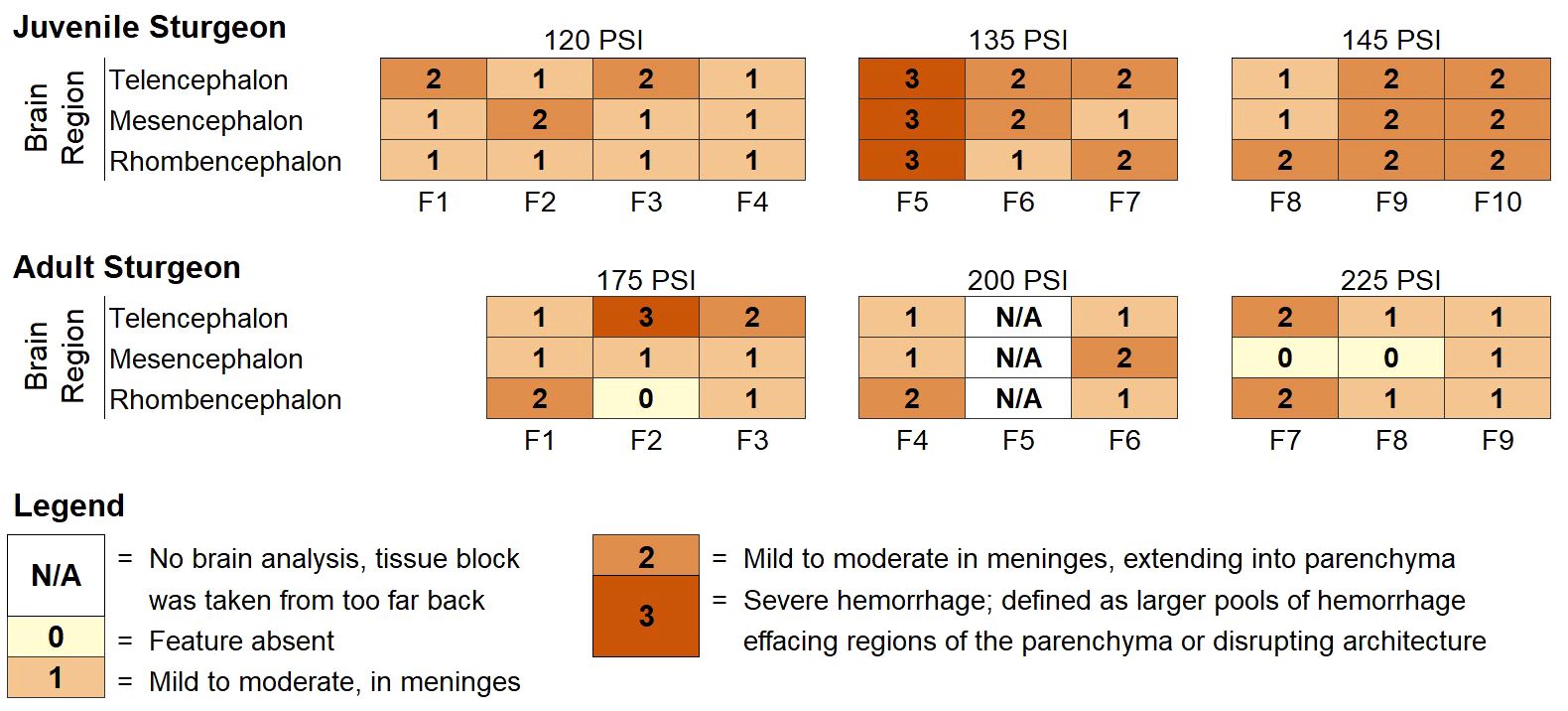
Figure 3 Categorical scores for regionally specific hemorrhage ratings for individual juvenile and adult white sturgeon brains exposed to a single strike using a non-penetrating captive bolt gun.
2.4 Recovery study
To confirm the efficacy of jaw relaxation as an appropriate metric for insensibility by NPCB, juvenile and adult fish were monitored immediately after receiving a single strike and during the exsanguination period during on-farm slaughter activities, which occurred at a later date than the dose-response study. Juvenile fish (n = 100 total juvenile white sturgeon) were individually removed from a dewatered fish tote and exposed to a single strike at 145 PSI on a stainless steel table, exsanguinated in an ice slurry. Only a portion of juvenile sturgeon were evaluated for size (n = 60, mean mass = 8.0 kg, mean length = 100.6 cm) after the monitoring period to avoid interfering with the harvest process. Similarly, 7.5-year-old adult female white sturgeon (n = 65, mean mass = 14.8 kg, mean length = 133 cm) were evaluated during slaughter over a single day. Due to adult fish size, fish were exposed to a single strike at 225 PSI in a dewatered fish tote upon immediate removal from the fish tank and exsanguinated in an ice slurry. All sturgeon were monitored for operculum movement and a somatic evoked response by touching gill rakers immediately after a single strike and then monitored in their respective totes for 2 hrs for coordinated muscle activity during the exsanguination phase in the ice slurry before processing.
2.5 Statistical analysis
The lowest acceptable pressure (120 PSI in juveniles and 175 PSI in adult white sturgeon) was selected and utilized as the control treatment based on pilot experimentation and current farm usage to maintain good animal welfare during fish processing. Stunning efficacy was determined by histopathological evaluation and monitoring during exsanguination. Histopathology was conducted blind to operating pressures and analyzed using GraphPad Prism v8.4.3. Semi-quantitative scores were compared between groups using a Kruskal-Wallis test (non-parametric ANOVA) with Dunn’s multiple comparisons test (p = 0.05).
3 Results
3.1 Stunning study
Intracranial hemorrhage in the meninges, superficial cerebral cortex, and cranial nerves were present in the captive-bolt slaughtered juveniles and market-size females (Figures 3, 4A, B). For juveniles, there was an effect of increased operating pressure on intracranial hemorrhaging (p = 0.024, Kruskal-Wallis ANOVA). Strikes delivered at 135 PSI produced more intracranial hemorrhage than the 120 PSI control (p = 0.020, Dunn’s multiple comparisons), and there was a trend towards significantly greater tissue damage in the 145 PSI group compared to the 120 PSI group (p = 0.056, Dunn’s multiple comparisons) (Figure 5). There was no significant difference in intracranial hemorrhage for the adults between the 175, 200, and 225 PSI groups (p = 0.59, Kruskal-Wallis ANOVA) (Figure 5). When evaluating axonal or neuronal swelling (Figure 4C), no significant differences were observed between operating pressures in the juveniles (p = 0.98, Kruskal-Wallis ANOVA) and adult females (p = 0.83, Kruskal-Wallis ANOVA). No evidence of overt cartilage necrosis or cerebral edema was observed.
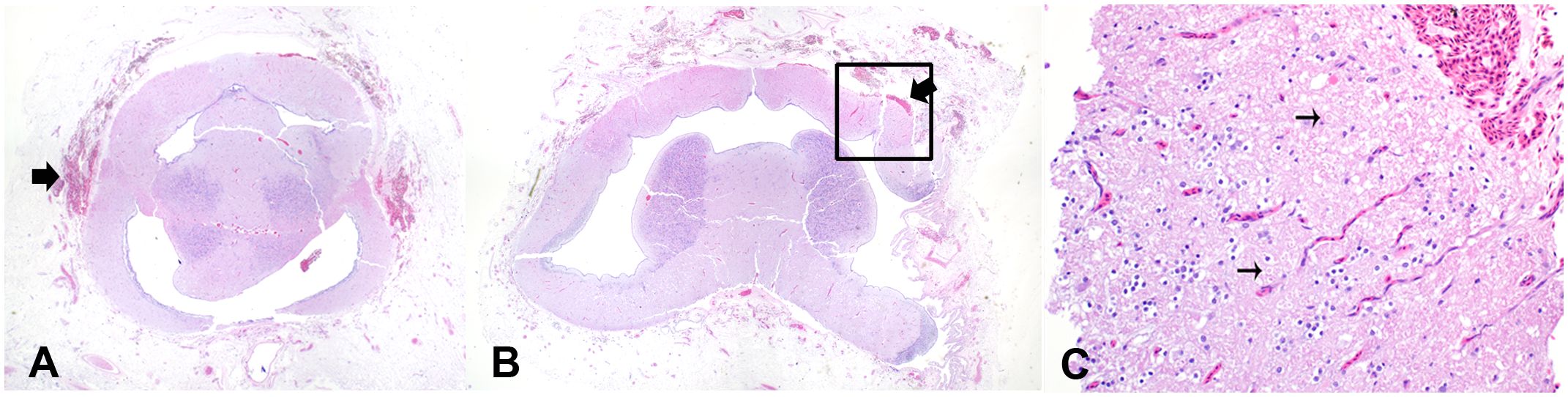
Figure 4 Meningeal and cerebral hemorrhage in white sturgeon after a non-penetrating captive bolt gun strike. (A) Hemorrhage (black arrow) in the meninges. (B) Hemorrhage in meninges and extending into parenchyma. (C) High magnification of the black box demonstrating axonal injury (thin black arrows) associated with hemorrhage.
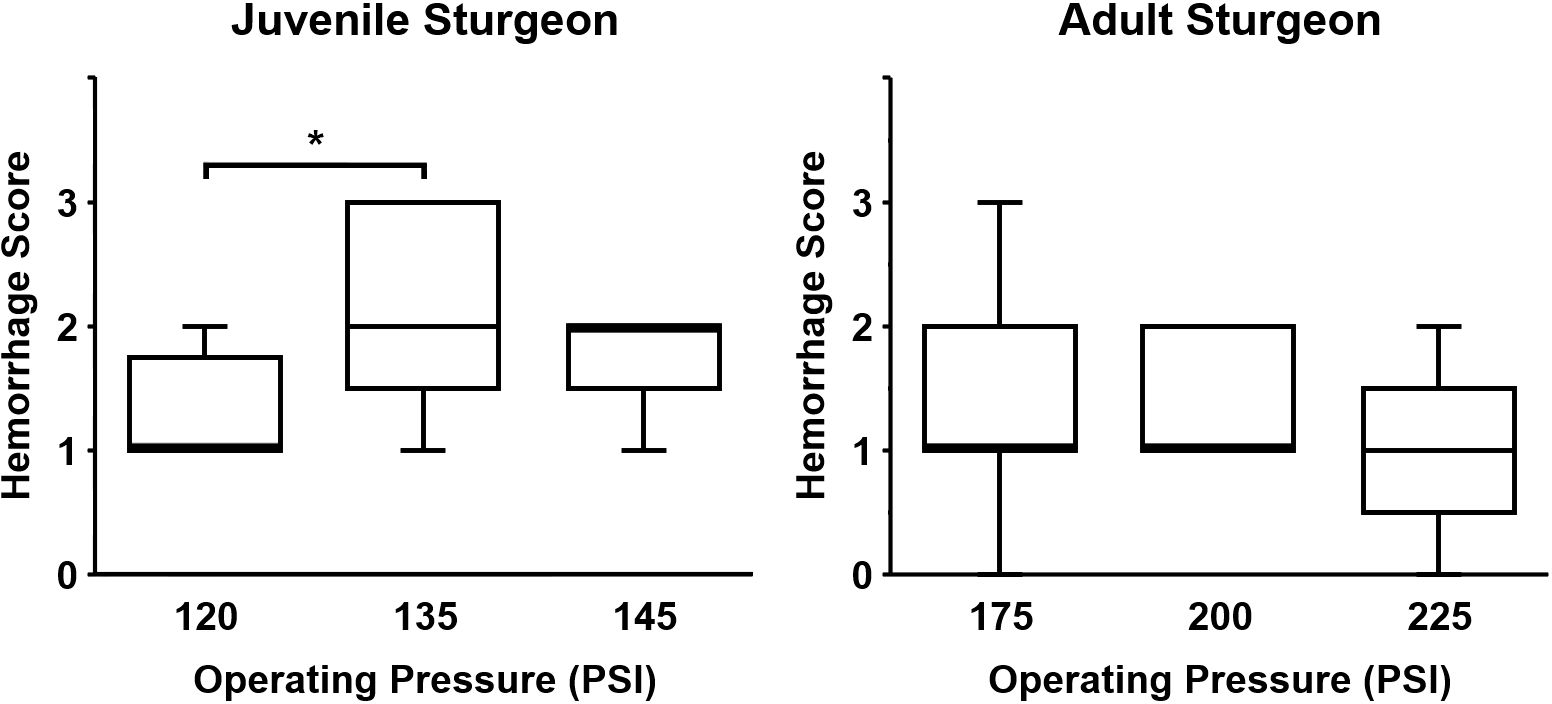
Figure 5 Brain injury in juvenile and adult white sturgeon exposed to a single strike using a non-penetrating captive bolt gun. In juvenile sturgeon, there was a significant effect of operating pressure between 120 PSI and 135 PSI (p < 0.020) with a trend towards greater intracranial hemorrhage scores (p = 0.056) between the 120 and 145 PSI operating pressures. “*” indicates statistical significance. There were no significant differences in intracranial hemorrhage between selected operating pressures in adult sturgeon. Box plots present median scores with minimum and maximum ranges for 3 fish per group (with the exception of 2 fish in the 200 PSI adult sturgeon group).
3.2 Recovery study
No juvenile sturgeon recovered following an NPCB stun at 145 PSI. When stunned at 225 PSI, 59 of the 65 adult sturgeon did not recover following a single NPCB strike. Six adult fish required an immediate second application, while one fish required a third during the 2-hour exsanguination monitoring period.
4 Discussion
This research demonstrates the effectiveness of a single strike by a non-penetrating captive bolt gun to cause brain damage and jaw relaxation during humane slaughter. Jaw relaxation by percussion stunning is generally considered an effective metric for stage 1 stunning across sturgeon aquaculture. Results from this study suggest that the use of the Jarvis HPS-1 non-penetrative captive bolt can be an effective method for stunning juvenile and adult sturgeon. Still, it should not be considered a definitively effective 1st stage terminal slaughter procedure at the tested pressures for the sizes of juvenile and adult fish because the only indicators of percussive injury were mild to moderate hemorrhage in and around the brain. While the histology results suggest that the cartilaginous skeleton protects from brain injury, juvenile and adult sturgeon behavioral responses and lack of recovery confer at least a degree of insensibility, if only temporary. No recovery was observed in juvenile sturgeons when stunned at an operating pressure of 145 PSI, and minimal recovery was seen in adults at 225 PSI, indicating that the selected pressures were acceptable. A recommended minimum operating pressure of 145 PSI (999.74 kPa) for juvenile white sturgeons and 225 PSI (1551.32 kPa) for adult white sturgeon is required to achieve effective percussion stunning with an NPCB with a flat striking surface, followed by exsanguination. While these pressures are effective for juveniles and adults, it may not be the optimal setting for all sturgeon sizes. For larger adult sturgeon (> 45 kg), pilot trials showed that operating pressures ≥ 250 PSI (1723.69 kPa) are generally more effective and should be considered best practice. While sturgeon may exhibit jaw relaxation after a single strike at lower pressure settings and potentially recover during exsanguination (J. Gross, personal observations), using an NPCB for a single strike still significantly improves animal welfare. This method is superior to manually administered strikes and repeated NPCB strikes at the lower pressures typically generated by readily available air compressors.
Currently, NPCB stunning in terrestrial animals is reserved for suckling pigs, neonatal ruminants, and turkeys (AVMA, 2016), and the effectiveness of the stun depends mainly on the size and shape of the skull. In larger livestock, the variation in head size and skull thickness can make it challenging to position the strike such that maximum energy is delivered to the brain (Daly and Whittington, 1989; HSA, 2013). To ensure the maximum force is delivered to the brain, the NPCB should be placed in the area of the head where there is the least distance between the skin and the skull (HSA, 2013). When met with a high-intensity impact, the skull protects the brain by absorbing force and fracturing rather than transferring the energy into the brain cavity (Miller and Mathews, 1974; Mansour, 2008). Brain damage in lambs after penetrating and non-penetrating captive bolt stun found that penetrating bolts caused severe injury at the point of impact, while stunning by a non-penetrating captive bolt caused damage that was less severe but more widespread than focal (Finnie et al., 2000). Analysis of brain activity using electroencephalography (EEG) in sheep following four different captive bolt treatments showed that the bolt’s impact against the skull resulted in an effective stun rather than penetration of the brain tissue (Daly and Whittington, 1989). Similar results were found in an experiment by Roth et al. with Atlantic salmon (Salmo salar), with a flat hammer-shaped bolt resulting in more effective stuns than penetrative bolts (Roth et al., 2007).
Over the last 30 years, as automated percussion stunning system technology has evolved primarily for salmon, additional research has explored the applicability of percussion stunning across various bony fish species (Erikson et al., 1997; Roth et al., 2007; Brijs et al., 2021). In systems designed for salmon, the effectiveness of percussion stunning does not universally apply to other species with different morphologies, as stunning efficacy is highly dependent on the alignment between uniformly applied force and the fish’s skull morphology. While percussive stunning is a widely used and ethically endorsed method in aquaculture, the EFSA (2004) states that percussive stunning can effectively stun/kill many fish species ranging between 0.2 and 14 kg. NPCB is recommended for large finfish (AVMA, 2016) but has been successfully used to stun only a few fish of different sizes (Table 1). However, even a 2-year-old white sturgeon presents a unique challenge due to its size, strength, and distinctive cranial structure. The thick, cartilaginous plate protecting the brain necessitates the application of significant force at a precise focal point to ensure humane slaughter, presenting considerable challenges for conventional percussion stunning methods to render the finfish unconscious.
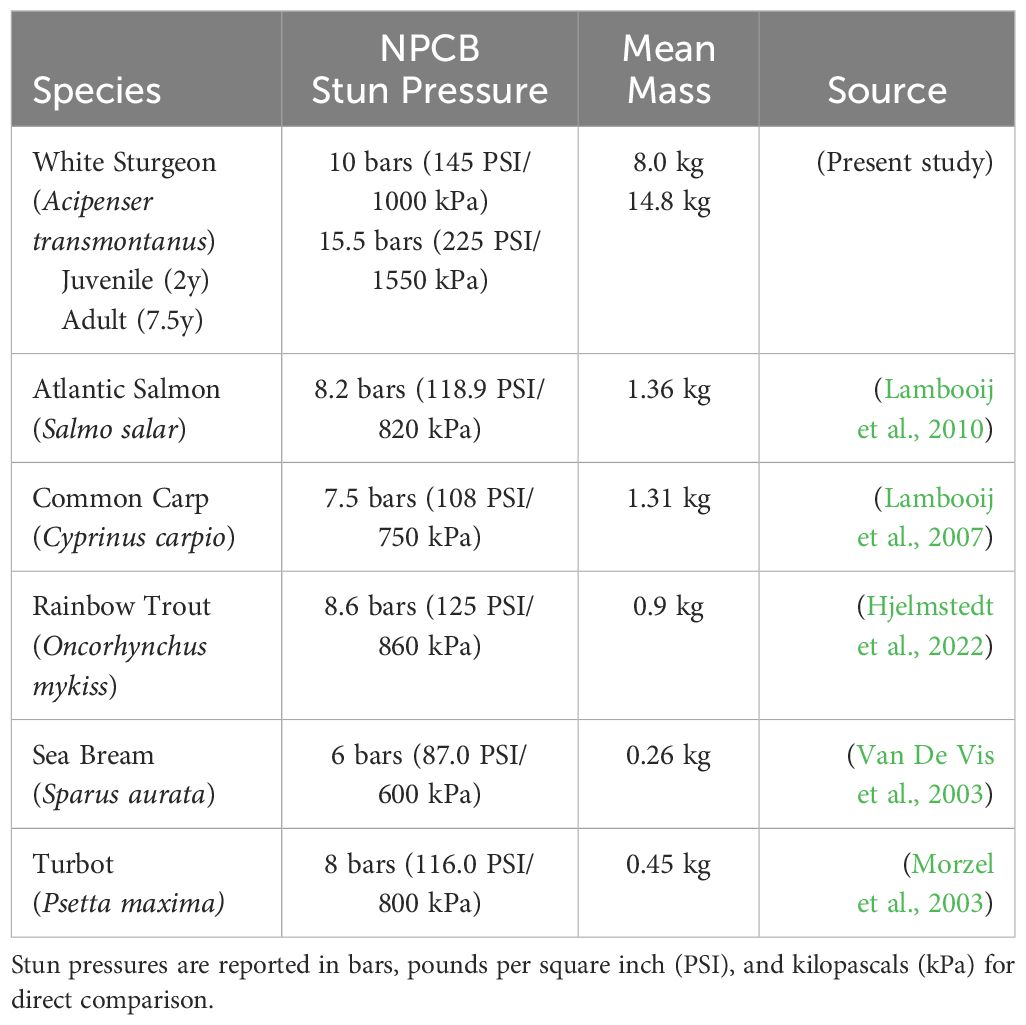
Table 1 Literature review of published effective operating pressures for non-penetrating captive bolt gun (NPCB) utilization for percussion stunning in fish.
The impact against the cartilaginous skull may be a key reason why non-penetrative captive bolt stunning is more effective for sturgeon than other larger livestock. Sturgeon notochord cartilage resembles avian or mammalian hyaline, also known as articular cartilage (Miller and Mathews, 1974). When impacted, there is no time for fluid to flow into the collagen matrix, and, as a result, the cartilage acts as an elastic but incompressible solid (Mansour, 2008), resulting in a greater transfer of energy into the brain cavity and increasing the probability of an effective stun. Failure to produce insensibility via non-penetrative captive bolt stunning can be largely attributed to the animal’s anatomy (HSA, 2013). In comparison of skull anatomy between eastern and western Pacific sturgeon, white and green sturgeon reportedly have small to completely absent fontanels as well as filled interfrontal space when compared to eastern Pacific Chinese (A. sinensis) or Danbry sturgeon (A. dabryanus) and as such may require greater percussive force (Artyukhin et al., 2007). In this study, histopathologic findings of intracranial hemorrhage and axonal/neuronal swelling were consistent with acute observations in animal models of weight-drop traumatic brain injury (Xiong et al., 2013).
Ideally, the number of re-strikes needed during the harvest of any animal would be none, but realistically, there will always be variability in effectiveness. Standardization of strike pressure and placement by trained professionals can reduce this risk. Still, there are limits, especially regarding the accuracy and precision of the strike when fish are dewatered. Furthermore, as white sturgeon grow larger and stronger, the target becomes smaller, and the sturgeon becomes a challenge to restrain. In the recovery assessment study, even when operators focused on precision instead of speed, several miss-strikes were recorded, with five adult fish requiring a second strike to achieve jaw relaxation. Moreover, the strike’s effectiveness is compromised when the sturgeon’s heads are not laid flush against a surface, as elevation or submersion in water leads to the dispersion of the striking force. This likely led to the observed increase in variability of intracranial hemorrhaging among juvenile fish exposed to 135 and 145 PSI (Figures 3, 5), as staff, during meat fish processing, placed a higher priority on speed rather than the positioning of the fish. To mitigate this potential confounder and ensure the full impact assessment of the strike, the heads of the juvenile sturgeon in the recovery assessment study were positioned with their ventral surfaces in direct contact with a steel table, while the heads of adult females were laid flush against the dense plastic bottom of the fish tote. Proper positioning of the fish and ensuring that the bolt head is flush to the fish’s head is crucial for the strike to deliver its intended effect efficiently (Figure 6).
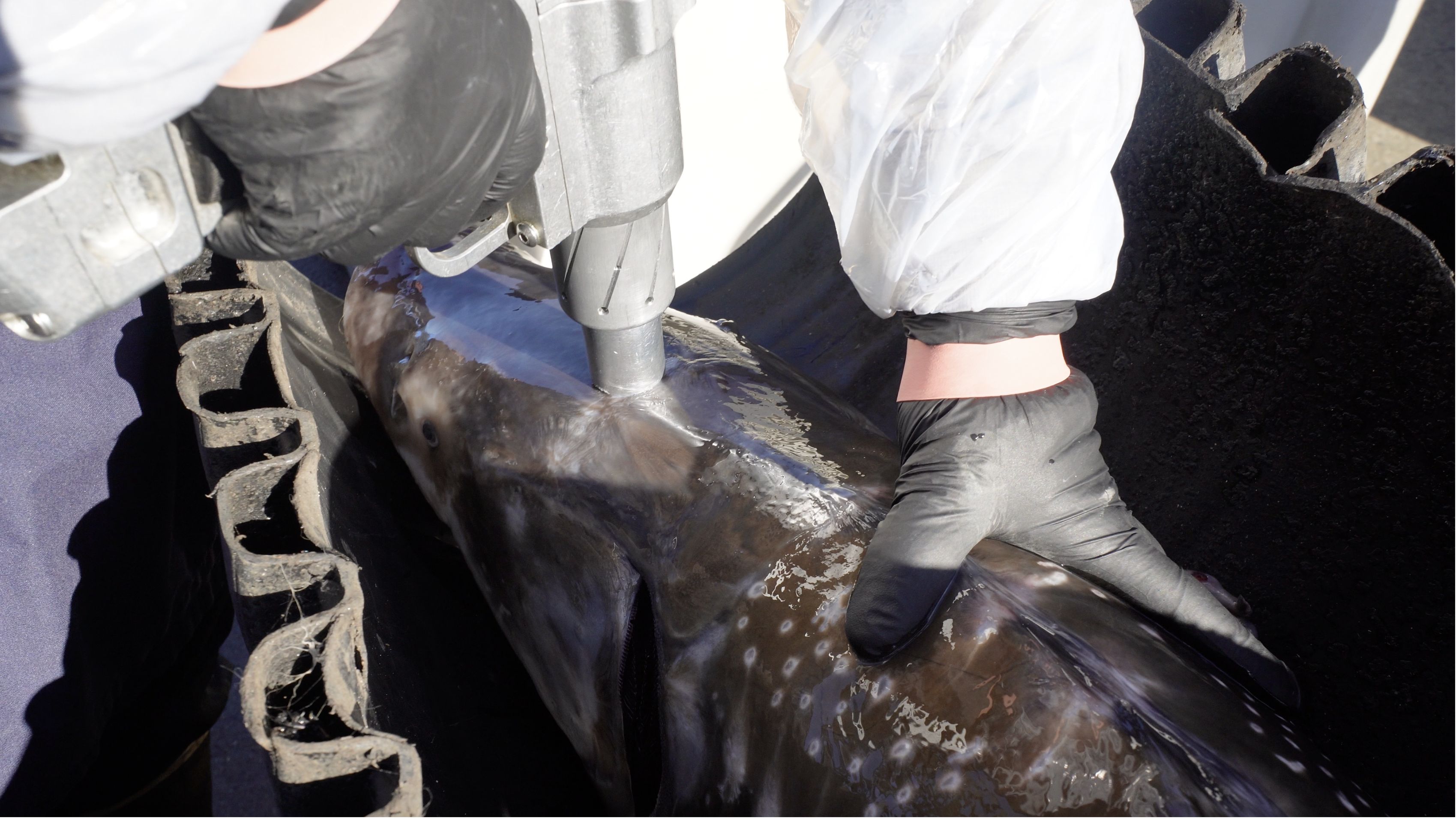
Figure 6 A demonstration of the correct positioning for the stunning process: the ventral surface of the fish’s head must be flush against the table, and the bolt head should be precisely positioned flush and medially between the two spiracles. This alignment is crucial to ensure that the percussive strike is delivered efficiently and achieves its intended effect.
In fish, insensibility is generally determined using visual indicators of unconsciousness. Indicators can include loss of righting reflex (equilibrium), vestibulo-ocular reflex (eye compensation for tilt), and ventilation. However, these indicators can be incredibly difficult to discern in a farm setting due to the pace at which large numbers of animals are processed, and have yet to be validated for sturgeon (Clemente et al., 2023). Following a stun, sturgeon are exsanguinated in a large container with a high density of fish, making indicators like loss of vestibulo-ocular reflex and loss of ventilation difficult to observe in individual fish. Additionally, the loss of righting reflex is one of the last indicators to return following insensibility for these fish (J. Gross, personal observations), and in many sturgeon species, stressed fish will lay inverted at the water surface or at the bottom of the tank, often observed during longer handling activities such as sexing or when assessing egg readiness. While not a reliable indicator of unconsciousness on its own, complete jaw relaxation has been used as an anecdotal indicator of brain dysfunction incompatible with consciousness in cattle (Grandin, 2002; Gregory and Grandin, 2007; Oliveira et al., 2017) and water buffalo (Gregory et al., 2009). As jaw relaxation can also be seen in conscious animals (Gregory et al., 2009), it should be used in conjunction with other visual indicators to determine if an animal is insensible. In this study, operculation readily informed the processor that another strike was required. Following a percussive stun, gills were gently touched while operators watched for a physical response. A lack of response indicated an effective stun before exsanguination via cutting the gills. Future research should be conducted using electroencephalography to study the relationship, if any, between brain activity and jaw relaxation following a stunning method.
Data availability statement
The raw data supporting the conclusions of this article will be made available by the authors, without undue reservation.
Ethics statement
The requirement of ethical approval was waived by UC Davis Institutional Animal Care and Use Committee for the studies involving animals because animals were owned and slaughtered during daily farming activities by the staff at Sterling Caviar, Elverta, California, United States. The studies were conducted in accordance with the local legislation and institutional requirements. Written informed consent was obtained from the owners for the participation of their animals in this study.
Author contributions
JG: Conceptualization, Funding acquisition, Investigation, Methodology, Project administration, Supervision, Writing – original draft, Writing – review & editing, Visualization. JB: Data curation, Project administration, Validation, Visualization, Writing – original draft, Writing – review & editing. DI: Formal analysis, Methodology, Validation, Visualization, Writing – original draft, Writing – review & editing. TW: Formal analysis, Methodology, Writing – original draft, Writing – review & editing. TD: Investigation, Writing – original draft, Writing – review & editing. SB: Writing – original draft, Writing – review & editing. RM: Funding acquisition, Writing – original draft, Writing – review & editing. XY: Funding acquisition, Resources, Writing – original draft, Writing – review & editing.
Funding
The author(s) declare financial support was received for the research, authorship, and/or publication of this article. This project was supported by the Western Regional Aquaculture Center Grant nos. 2018–38500-28889 and 2020–38500-32561.
Acknowledgments
The authors would like to thank Sterling Caviar (Elverta, California, USA) for their generous cooperation and the pivotal role they play in promoting and facilitating improved welfare practices that extend beyond their operations, contributing to the betterment of sturgeon aquaculture worldwide. We dedicate this publication to the memory of Dr. Fred Conte, whose pioneering contributions to animal welfare in aquaculture have profoundly influenced this research. Dr. Conte was a trailblazer in our field and a cherished mentor who inspired us with their dedication, insight, and passion for improving the well-being of aquatic animals. Their legacy continues to guide and inspire our work, and we are deeply grateful for the path they forged and the wisdom they imparted.
Conflict of interest
The authors declare that the research was conducted in the absence of any commercial or financial relationships that could be construed as a potential conflict of interest.
Publisher’s note
All claims expressed in this article are solely those of the authors and do not necessarily represent those of their affiliated organizations, or those of the publisher, the editors and the reviewers. Any product that may be evaluated in this article, or claim that may be made by its manufacturer, is not guaranteed or endorsed by the publisher.
References
Artyukhin E. N., Vecsei P., Peterson. D. L. (2007). Morphology and ecology of Pacific sturgeons. Environ. Biol. Fishes 79, 369–381. doi: 10.1007/s10641-006-9157-6
Aschemann-Witzel J., De Hooge I., Amani P., Bech-Larsen T., Oostindjer M. (2015). Consumer-related food waste: causes and potential for action. Sustainability 7, Article 6. doi: 10.3390/su7066457
AVMA (2016). AVMA Guidelines for the Humane Slaughter of Animals: 2016 Edition (American Veterinary Medical Association), (Schaumburg, IL, USA ), 64. Available at: https://www.avma.org/sites/default/files/resources/Humane-Slaughter-Guidelines.pdf.
Azam K., Strachan N. J. C., Mackie I. M., Smith J., Nesvadba P. (1990). Effect of slaughter method on the progress of rigor of rainbow trout (Salmo gairdneri) as measured by an image processing system. Int. J. Food Sci. Technol. 25, 477–482. doi: 10.1111/j.1365-2621.1990.tb01107.x
Berg T., Erikson U., Nordtvedt T. S. (1997). Rigor mortis assessment of Atlantic salmon (Salmo salar) and effects of stress. J. Food Sci. 62, 439–446. doi: 10.1111/j.1365-2621.1997.tb04402.x
Boggess T. S., Heaton E. K., Beuchat L. R. (1973). A procedure for processing smoked channel catfish (Ictalurus punctatus). J. Food Prot. 36, 469–473. doi: 10.4315/0022-2747-36.9.469
Braitenbach Cavali J., Vargas Baldi S. C., Coutinho Marques Rocha A. S., Eloy da Silva E., Taveira Nunes C., Soares E. C., et al. (2024). Pre-slaughter stunning methods influence the meat quality of Arapaima gigas fillets. Animals 14, Article 8. doi: 10.3390/ani14081155
Brijs J., Sundell E., Hjelmstedt P., Berg C., Senčić I., Sandblom E., et al. (2021). Humane slaughter of African sharptooth catfish (Clarias gariepinus): Effects of various stunning methods on brain function. Aquaculture 531, 735887. doi: 10.1016/j.aquaculture.2020.735887
Bronzi P., Chebanov M., Michaels J. T., Wei Q., Rosenthal H., Gessner J. (2019). Sturgeon meat and caviar production: Global update 2017. J. Appl. Ichthyol. 35, 257–266. doi: 10.1111/jai.13870
Bronzi P., Rosenthal H., Gessner J. (2011). Global sturgeon aquaculture production: An overview. J. Appl. Ichthyol. 27, 169–175. doi: 10.1111/j.1439-0426.2011.01757.x
Buzby J. C., Farah-Wells H., Hyman J. (2014). The estimated amount, value, and calories of postharvest food losses at the retail and consumer levels in the United States. SSRN Electronic J. 121, 39 .doi: 10.2139/ssrn.2501659
Cheng J.-H., Sun D.-W., Zeng X.-A., Liu D. (2015). Recent advances in methods and techniques for freshness quality determination and evaluation of fish and fish fillets: A review. Crit. Rev. Food Sci. Nutr. 55, 1012–1225. doi: 10.1080/10408398.2013.769934
Clemente G. A., Tolini C., Boscarino A., Lorenzi V., Dal Lago T. L., Benedetti D., et al. (2023). Farmed fish welfare during slaughter in Italy: Survey on stunning and killing methods and indicators of unconsciousness. Front. Vet. Sci. 10. doi: 10.3389/fvets.2023.1253151
Conte F. (2010). Animal welfare: Growing factor in international aquaculture - Responsible Seafood Advocate (Global Seafood Alliance). Available at: https://www.globalseafood.org/advocate/animal-welfare-growing-factor-in-international-aquaculture/.
Daly C. C., Whittington P. E. (1989). Investigation into the principal determinants of effective captive bolt stunning of sheep. Res. Vet. Sci. 46, 406–408. doi: 10.1016/S0034-5288(18)31189-5
Daskalova A. (2019). Farmed fish welfare: stress, post-mortem muscle metabolism, and stress-related meat quality changes. Int. Aquat. Res. 11, 113–124. doi: 10.1007/s40071-019-0230-0
EFSA (2004). Scientific Opinion of the Panel on Animal Health and Welfare (AHAW) on a request from the Commission related to welfare aspects of the main systems of stunning and killing the main commercial species of animals. The EFSA Journal, 45, 1–29.
Erikson U., Beyer A., Sigholt T. (1997). Muscle high-energy phosphates and stress affect K-values during ice storage of Atlantic salmon (Salmo salar). J. Food Sci. 62, 43–47. doi: 10.1111/j.1365-2621.1997.tb04365.x
FAO (2024). FAO Fisheries and Aquaculture - FishStatJ - Software for Fishery and AquacultureStatistical Time Series. In: FAO Fisheries and Aquaculture Division [online]. Rome. Available at: https://www.fao.org/fishery/en/statistics/software/fishstatj.
Finnie J. W., Blumbergs P. C., Manavis J., Summersides G. E., Davies R. A. (2000). Evaluation of brain damage resulting from penetrating and non-penetrating captive bolt stunning using lambs. Aust. Vet. J. 78, 775–778. doi: 10.1111/j.1751-0813.2000.tb10451.x
Grandin T. (2002). Return-to-sensibility problems after penetrating captive bolt stunning of cattle in commercial beef slaughter plants. JAVMA 221, 1258–1261. doi: 10.2460/javma.2002.221.1258
Green D. P. (2010). “Sensory evaluation of fish freshness and eating qualities,” in: Alasalvar C, Shahidi F, Miyashita K, Wanasundara U, editors Handbook of Seafood Quality, Safety and Health Applications. (Oxford (UK): Blackwell Publishing Ltd.), 29–38. doi: 10.1002/9781444325546.ch3
Gregory N. G., Grandin T. (2007). “Stunning and slaughter,” in Animal Welfare and Meat Production (CABI), (Wallingford, Oxfordshire OX10 8DE, UK), 191–212. Available at: https://www.google.com/books/edition/Animal_Welfare_and_Meat_Production/RTeyiR0rSEwC?hl=en&gbpv=1&bsq=jaw. doi: 10.1079/9781845932152.0000
Gregory N. G., Spence J. Y., Mason C. W., Tinarwo A., Heasman L. (2009). Effectiveness of poll stunning water buffalo with captive bolt guns. Meat Sci. 81, 178–182. doi: 10.1016/j.meatsci.2008.07.016
Hjelmstedt P., Sundell E., Brijs J., Berg C., Sandblom E., Lines J., et al. (2022). Assessing the effectiveness of percussive and electrical stunning in rainbow trout: Does an epileptic-like seizure imply brain failure? Aquaculture 552, 738012. doi: 10.1016/j.aquaculture.2022.738012
HSA (2013). Captive-Bolt Stunning of Livestock (Humane Slaughter Association), (The Old School, Brewhouse Hill, Wheathampstead, Herts), 34. Available at: https://www.hsa.org.uk/downloads/publications/captiveboltstunningdownload.pdf.
Kiessling A., Espe M., Ruohonen K., Mørkøre T. (2004). Texture, gaping and colour of fresh and frozen Atlantic salmon flesh as affected by pre-slaughter iso-eugenol or CO2 anaesthesia. Aquaculture 236, 645–657. doi: 10.1016/j.aquaculture.2004.02.030
Lambooij E., Grimsbø E., van de Vis J. W., Reimert H. G. M., Nortvedt R., Roth B. (2010). Percussion and electrical stunning of Atlantic salmon (Salmo salar) after dewatering and subsequent effect on brain and heart activities. Aquaculture 300, 107–112. doi: 10.1016/j.aquaculture.2009.12.022
Lambooij E., Pilarczyk M., Bialowas H., van den Boogaart J. G. M., van de Vis J. W. (2007). Electrical and percussive stunning of the common carp (Cyprinus carpio L.): Neurological and behavioural assessment. Aquacultural Eng. 37, 171–179. doi: 10.1016/j.aquaeng.2007.04.004
Love D. C., Fry J. P., Genello L., Hill E. S., Frederick J. A., Li X., et al. (2014). An international survey of aquaponics practitioners. PloS One 9, e102662. doi: 10.1371/journal.pone.0102662
Mansour J. M. (2008). “Biomechanics of cartilage,” in Kinesiology: The mechanics and Pathomechanics of Human Movement. Ed. Oatis C. A. (Lippincott Williams & Wilkins, PA, USA), 69–83.
Marx H., Brunner B., Weinzierl W., Hoffmann R., Stolle A. (1997). Methods of stunning freshwater fish: Impact on meat quality and aspects of animal welfare. Z. Für Lebensmitteluntersuchung Und-Forschung 204, 282–286. doi: 10.1007/s002170050078
Miller E. J., Mathews M. B. (1974). Characterization of notochord collagen as a cartilage-type collagen. Biochem. Biophys. Res. Commun. 60(1), 424–430. doi: 10.1016/0006-291x(74)90221-6
Morzel M., Sohier D., Van De Vis H. (2003). Evaluation of slaughtering methods for turbot with respect to animal welfare and flesh quality. J. Sci. Food Agric. 83, 19–28. doi: 10.1002/jsfa.1253
Oliveira S. E. O., Gregory N. G., Dalla Costa F. A., Gibson T. J., Paranhos da Costa M. J. R. (2017). Efficiency of low versus high airline pressure in stunning cattle with a pneumatically powered penetrating captive bolt gun. Meat Sci. 130, 64–68. doi: 10.1016/j.meatsci.2017.04.007
Poli B. M., Parisi G., Scappini F., Zampacavallo G. (2005). Fish welfare and quality as affected by pre-slaughter and slaughter management. Aquac. Int. 13, 29–49. doi: 10.1007/s10499-004-9035-1
Prabhakar P. K., Vatsa S., Srivastav P. P., Pathak S. S. (2020). A comprehensive review on freshness of fish and assessment: Analytical methods and recent innovations. Food Res. Int. (Ottawa Ont.) 133, 109157. doi: 10.1016/j.foodres.2020.109157
Retter K., Esser K.-H., Lüpke M., Hellman J., Steinhagan D., Jung-Schoers V. (2018). Stunning of common carp: Results from a field and laboratory study. BMC Vet. Res. 14, e205. doi: 10.1186/s12917-018-1530-0
Robb D. H., Wotton S. B., McKinstry J. L., Sørensen N. K., Kestin S. C. (2000). Commercial slaughter methods used on Atlantic salmon: Determination of the onset of brain failure by electroencephalography. Vet. Rec. 147, 298–303. doi: 10.1136/vr.147.11.298
Roth B., Moeller D., Veland J., Imsland A., Slinde E. (2002). The effect of stunning methods on rigor mortis and texture properties of Atlantic salmon (Salmo salar). J. Food Sci. 67, 1462–1466. doi: 10.1111/j.1365-2621.2002.tb10306.x
Roth B., Slinde E., Robb D. H. F. (2007). Percussive stunning of Atlantic salmon (Salmo salar) and the relation between force and stunning. Aquacultural Eng. 36, 192–197. doi: 10.1016/j.aquaeng.2006.11.001
Skjervold P. O., Fjæra S. O., Østby P. B., Einen O. (2001). Live-chilling and crowding stress before slaughter of Atlantic salmon (Salmo salar). Aquaculture 192, 265–280. doi: 10.1016/S0044-8486(00)00447-6
USDA NASS (2018). 2017 Census of Agriculture, (2017 Census of Agriculture) (USDA National Agricultural Statistics Service). Available at: www.nass.usda.gov/AgCensus.
Van De Vis H., Kestin S., Robb D., Oehlenschläger J., Lambooij B., Münkner W., et al. (2003). Is humane slaughter of fish possible for industry? Aquac. Res. 34, 211–220. doi: 10.1046/j.1365–2109.2003.00804.x
Xiong Y., Mahmood A., Chopp M. (2013). Animal models of traumatic brain injury. Nat. Rev. Neurosci. 14, 128–142. doi: 10.1038/nrn3407
Keywords: animal welfare, sturgeon, percussion stunning, insensibility, humane slaughter, aquaculture, fish farming
Citation: Gross JA, Bowman JR, Imai DM, Wong TS, Duarte TL, Boles SE, McGorrin RJ and Yang X (2024) Evaluation of non-penetrative captive bolt stunning as a method of slaughter for white sturgeon (Acipenser transmontanus). Front. Anim. Sci. 5:1405554. doi: 10.3389/fanim.2024.1405554
Received: 23 March 2024; Accepted: 20 May 2024;
Published: 03 June 2024.
Edited by:
Eva Voslarova, University of Veterinary and Pharmaceutical Sciences Brno, CzechiaReviewed by:
Francesca Conte, University of Messina, ItalyDaniel Mota-Rojas, Metropolitan Autonomous University, Mexico
Copyright © 2024 Gross, Bowman, Imai, Wong, Duarte, Boles, McGorrin and Yang. This is an open-access article distributed under the terms of the Creative Commons Attribution License (CC BY). The use, distribution or reproduction in other forums is permitted, provided the original author(s) and the copyright owner(s) are credited and that the original publication in this journal is cited, in accordance with accepted academic practice. No use, distribution or reproduction is permitted which does not comply with these terms.
*Correspondence: Jackson A. Gross, amFncm9zc0B1Y2RhdmlzLmVkdQ==