- 1Department of Experimental Therapeutics, The University of Texas MD Anderson Cancer Center, Houston, TX, United States
- 2Phoenix Biotechnology, Inc., San Antonio, TX, United States
- 3RTI, LLC, Brookings, SD, United States
- 4Departments of Thoracic, Head and Neck Medical Oncology and Veterinary Sciences, The University of Texas MD Cancer Center, Houston, TX, United States
- 5MD Anderson Cancer Center UT Health Graduate School of Biomedical Sciences, Houston, TX, United States
Background: Infection by porcine respiratory and reproductive syncytial virus (PRRSV), swine influenza virus (SIV) and porcine epidemic diarrhea (PEDV) adversely affect worldwide pig production. Because effective control remains elusive the present research was designed to explore the in vitro antiviral activity of oleandrin and an N. oleander extract (PBI-05204) against each porcine virus.
Methods: Monkey kidney (MARK-145) cells, Madin-Darby canine kidney cells (MDCK), and African green monkey kidney cells (VERO 76) were used for in vitro culture systems for PRRSV, SIV and PEDV, respectively. Cytotoxicity was established using serial dilutions of oleandrin or PBI-05204. Noncytotoxic concentrations of each product were used either prior to or at 12 h and 24 h following exposure to corresponding viruses. Infectious virus titers were also determined.
Results: Oleandrin and PBI-05204 demonstrated strong antiviral activity against PRRSV, SIV and PEDV when added prior to or following infection of cells. Determination of viral loads by PCR demonstrated a decline in PRRSV replication reaching 99.57% and 99.79% for oleandrin and PBI-05204, respectively, and decrease of 95.36% and 99.54% in infectivity of progeny virus in PRRSV infected cultures. Similarly, oleandrin tested against SIV and PEDV was effective in near complete inhibition of infectious virus production.
Conclusion: The research demonstrates the potency of oleandrin and PBI-05204 to inhibit infectivity of three important porcine viruses. These data showing non-toxic concentrations of oleandrin as a single common agent for inhibiting infectivity of the three different porcine viruses tested here support further investigation of antiviral efficacy and possible in vivo use.
Introduction
Infectious diseases represent a global threat to humans, livestock, and wildlife animals with potential cross-species transmission (Lerner and Berg, 2015; Andraud and Rose, 2020). Over the last 30 years, diseases caused by emerging swine viruses have acquired particular relevance, more so than in any other species (Liu and Wang, 2021). Therefore, there is an important need to address viral and associated infections in swine as the production of these animals represents approximately one third of meat consumption throughout the world with important consequences on the food chain supply (VanderWaal and Deen, 2018). Beyond their role as a vital food source swine production has increasingly been seen as a problem with respect to the role it plays as intermediate/amplifying hosts for zoonotic viruses with pandemic potential (McLean and Graham, 2022). Infected swine also represent a major economic burden on farmers as adequate control and elimination of viral mediated disease is often difficult and problematic. Three of the most common viruses affecting swine health and commercial production are PRRSV (porcine reproductive and respiratory syndrome virus), SIV (swine influenza virus (SIV), and PEDV (porcine epidemic diarrhea virus).
PRRSV has been indicated as the causative agent of the most economically important disease for the pig industry worldwide (Cornelison et al., 2018; Montaner-Tarbes et al., 2019). The disease, characterized by producing reproductive failure in sows and respiratory problems in growing pigs, appeared in the late 1980s in the United States and Canada. It was not until 1991, however, when the causative agent of this disease, a virus, was isolated in the Netherlands (Wensvoort et al., 1991) and the following year another strain of the same virus (VR-2332) was isolated in the United States (Collins et al., 1992; Ruedas-Torres et al., 2021). Sharing only 55–70% of their nucleotide sequence (Ruedas-Torres et al., 2021), these strains of PRRSV represent enormous antigenic heterogeneity. Regardless of the genetic types of PRRSV, clinical manifestations are similar representing mainly respiratory symptoms in piglets and abortion, reproductive failure, fetal death, and congenital infections in pregnant animals (Chand et al., 2012; Lunney et al., 2016).
PRRSV is a member of the Arteriviridae family of enveloped viruses (Ma et al., 2021; Ruedas-Torres et al., 2021). The viral genome is a single-stranded positive-sense RNA and a great deal of new information has been gained on the pathogenicity of the viral infection that is now known to include deficits in the infected pig innate immune system (Cai et al., 2023). While there exists a commercial vaccine against PRRSV, it offers only modest protection for pigs due in part to the problem of preparing an effective vaccine against all known virus variants (Du et al., 2017; Ma et al., 2021). Specific therapeutic drugs have not reached their desired potential, making the prevention, control, and eradication of PRRSV difficult. Moreover, despite a sustained effort, PRRSV-specific treatment for infected herds, or prevention methods other than vaccines are still unavailable (Du et al., 2017).
Swine influenza (SIV) is an important respiratory disease in pigs which is epidemic in most areas worldwide. It is caused by influenza virus A (IAV) belonging to the family Orthomyzoviridae (Ma, 2020). In pigs, four influenza A virus subtypes (H1N1, H1N2, H3N2 and H7N9) are the most common strains worldwide (Darbeheshti et al., 2021). These are all enveloped RNA viruses. Commercially available whole inactivated virus (WIV) vaccines have been shown to be effective to protect pigs against homologous or genetically similar virus infections and remain widely used (Rahn et al., 2015; Mancera Gracia et al., 2020). However, swine influenza has not been controlled partially due to lack of heterovariant and heterosubtypic protection of WIV vaccines (Ma and Richt, 2010; Ma, 2020). Furthermore, different from influenza A viruses in humans, evolution of IAVs in pigs has shown a unique trend which is subject to geographic locations, depending on the country, region, and even the farm level (Simon et al., 1992; Shao et al., 2017) Therefore, manufacturers of swine influenza vaccines find it difficult to make a “universal” vaccine for all farms in different areas and have to make independent decisions regarding the strains used in their products. As such, swine influenza is still a major challenge and causes significant economic losses to the swine industry (Ma, 2020).
A third virus of economic importance to swine health and commercial production is the porcine epidemic diarrhea virus (PEDV), which is a member of the enveloped RNA coronavirus family, causing deadly watery diarrhea in newborn piglets. The currently available vaccines and drugs are only effective against the classic GI strains that were prevalent prior to 2010, while there is no effective control against the high mortality variant PEDV strains that have emerged and spread globally, having a profound impact on the global swine industry (Zhang et al., 2022). Considering the hazards caused by PEDV, preventive immunization is administered by vaccinating pregnant sows. However, due to the high heterogeneity of PEDV, there are currently no effective and safe vaccines to deal with the threat posed by this virus (Zhang et al., 2023).
An ideal antiviral agent for the control of porcine relevant virally mediated diseases would be safe, effective, and inexpensive. While vaccines are, by design, centric to the particular virus being targeted, there currently exists a need for more effective antiviral tools. Some herbal extracts have been reported to be useful for control of PRRSV, as an example of important swine relevant viruses. Extracts such as those from green tea (epigallocatechin gallate or EGCG) were found to inhibit PRRSV in vitro(Zhao et al., 2014), while additional in vitro studies have shown the utility of herb based chlorogenic acid and scutellarin as those with high anti-PEDV activities (Cheng et al., 2013). We have recently demonstrated that safe, nontoxic concentrations of oleandrin, derived from Nerium oleander, have high efficacy both as broad spectrum prophylactic and therapeutic agents against a number of human viruses (Newman et al., 2020) as well as three of the most important enveloped viruses known to be important in bovine health and wellbeing (Newman et al., 2022). The purpose of the present research was to investigate whether oleandrin and a defined extract (PBI-05204) containing this molecule would serve as a prophylactic as well as a therapeutic agent against each of the three most important viruses known to affect porcine health and husbandry.
Methods
Cells, viruses, and antiviral treatments
Porcine reproductive and respiratory syndrome virus (PRRSV) North American VR 2332 strain, Porcine epidemic diarrhea virus (PEDV) CO isolate, and Swine influenza virus (SIV) Iowa H1N1 strain were obtained from the National Veterinary Services Laboratory (NVSL, Ames, Iowa). Prior to the assays, each virus stock was titrated on susceptible cell line used for the assay. MARC-145, Vero76, and MDCK cells were used for the prophylactic and/or therapeutic assays for PRRSV, PEDV, and SIV respectively. The cells were maintained in a 37°C incubator with 5% CO2 and propagated using Eagle’s Minimum Essential Medium (MEM) (Corning, Manassas, VA) supplemented with 10% Fetal Bovine Serum (PAA GE Healthcare, Westborough, MA), 1× Antibiotic/Antimycotic solution (Ab/Am) (Cytiva, Logan, UT), and 1× L-glutamine solution (Gibco, Grand Island, NY).
PBI-05204 is a supercritical CO2 extract of Nerium oleander leaves and was provided by Phoenix Biotechnology, Inc. (San Antonio, TX). Characterization of PBI-05204 was carried out using an AccuTOF-DART mass spectrometer (Jeol UAS, Peabody, MA). Specific content of the extract was previously reported (Dunn et al., 2011). The extract contains cardiac glycosides, oleandrin (2.99%) and oleandrigenin (3.31%); triterpenoid acids, ursolic and betulinic acids (combined total of 15.29%) and oleanolic acid (0.60%) and odoroside (0.8%); Urs-12-ene-3β, 28-diol/botulin (5.44%), 3β, 3β-hydroxy-12-olean en-28-oic acid (14.26%); 28-nours-12-en-3β-ol (4.94%); and urs-12-en-3β-ol (4.76%) (Dunn et al., 2011). Other triterpenoids present in the extract of Nerium oleander have been reported by others (Siddiqui et al., 2012). A stock solution of PBI-05204 at a concentration of 1 mg/ml in DMSO was diluted based on the oleandrin content as shown in the figures and tables for use in the different assays. A stock solution of oleandrin (PhytoLab, Vestenbergsgreuth, Germany) was dissolved at a concentration of 1 mg/ml in DMSO (Invitrogen, Eugene, OR) and concentrations ranging between 1000 to 5 ng/ml were used in the different assays. The relative cytotoxicity as well as data pertaining to antiviral efficacy of PBI-05204 are reported as equivalent oleandrin concentrations to permit a direct comparison to pure oleandrin. Actual PBI-05204 concentrations ranged from 294 ng/ml to 58,800 ng/ml which were equivalent to 5 ng/ml to 1000 ng/ml of oleandrin, respectively.
Both the pure Oleandrin and PBI-05204 were tested for cytotoxicity and antiviral activity at the Research Technology Innovation laboratories (RTI, LLC, Brookings, SD, USA) as described below.
Cytotoxicity testing of the antiviral products
To determine the safe concentrations of oleandrin and PBI-05204 for susceptible cells, tissue culture plates (96-well) were seeded with 4 × 104 of MARC-145, Vero76 or MDCK cells in 100 µl growth media and cultured in a 37°C/5% CO2 incubator overnight. The following day, the growth media was removed and replaced with 100 µl maintenance media containing either different concentrations of pure oleandrin or PBI-05204 in DMSO along with matching dilutions of DMSO or untreated media to serve as controls. All treatments were added to triplicate wells and cultured in the 37°C/5% CO2 incubator. At either 24- or 48-h post-treatment, the cell viability was assessed by determining the lactate dehydrogenase (LDH) released into the supernatant using the CyQUANT LDH toxicity assay kit (Thermofisher, Waltham, MA) according to the manufacturer’s directions. Absorbance was measured at 490 nm and 680 nm on the Spectramax i3x (Molecular devices, San Jose, CA).
In vitro assays to determine the antiviral activities of oleandrin and PBI-05204
The antiviral activities of different concentrations of oleandrin or PBI-0524 product were tested on susceptible monolayers of respective target cells for each of the three viruses at a concentration of approximately 5 × 105 cells per well in 12-well plates. The antiviral activities were either evaluated prophylactically (pre-challenge) or therapeutically (post challenge) at different time points (12h and 24h) with serial dilutions of oleandrin (5–1000 ng/ml) or PBI-05204 (5–50 ng/ml of oleandrin), selected based on the toxicity assay, along with corresponding dilutions of DMSO controls in fresh maintenance media.
For the pre-challenge treatment, the cells in triplicate wells were incubated with the drug concentrations for 30 min followed by the addition of virus (5 × 103 TCID50 virus units in a volume of 500 µL maintenance media) at a multiplicity of infection (MOI) of 0.01. The infection was allowed to proceed for one hour at 37°C/5% CO2. Subsequently, the cells were washed gently 3 times with phosphate buffered saline (PBS), and 2 ml of maintenance media containing the original concentrations of oleandrin or PBI-05204 in DMSO, or DMSO was added. Samples of cell culture supernatants were collected at 24- and 48-h post infection for determining the amount of virus and its infectivity as shown separately below as “Determination of infectious viral load in the culture supernatants”.
For the post-challenge treatments, the cells in triplicate wells were treated at either 12- or 24-h post-infection with the different concentrations of oleandrin or PBI-05204 in DMSO or matched DMSO-only controls. Samples of cell culture supernatants from the 12-h post-infection treatment were collected at 24- and 48-h post-infection. Similarly, samples of cell culture supernatants from the 24-h post-infection treatment were collected at 48-h post-infection as shown separately below as “Determination of infectious viral load in the culture supernatants”.
Culture supernatant samples collected were either used immediately for determining the infectious virus titer on susceptible cells or stored at −80°C for determining the viral load by polymerase chain reaction (PCR) assay.
Determination of the infectious viral load in the culture supernatants
Culture supernatant samples collected as described above were serially diluted 10-fold in maintenance medium. Diluted samples were added to susceptible cells in triplicate in a volume of 200 µL/well and incubated at 37°C/5% CO2 for 3–4 days for PRRSV and PEDV, and 2days for SIV. After incubation, plates were either checked for cytopathic effect (CPE) as in SIV or fixed with 80% acetone and stained using an appropriate monoclonal antibody specific for each virus (PEDV S1D12, VMRD, Pullman, WA); (PRRSV: SDOW-17, RTI, Brookings, SD). Virus replication was visualized with a goat anti-mouse FITC secondary conjugate (Invitrogen, Carlsbad, CA) and plates were read using a fluorescence microscope. Positive and negative control wells were included and virus titer for each virus was calculated as TCID50/ml using the Spearman-Karber method of estimating 50% end points.
Determination of the viral nucleic acid load between treatments
A sample from all treatments and timepoints for each virus was sent to the ADRDL) Animal Disease Research & Diagnostic Laboratory), South Dakota State University (Brookings, SD) for viral nucleic acid extraction and real-time PCR (RT-qPCR) assay. Also, 10-fold serial dilutions of each virus stock were used to create a corresponding standard curve. Viral nucleic acid was extracted from samples using the MagMAX viral RNA isolation kit (Life Technologies, Carlsbad, CA) according to the manufacturer’s instructions. Detection and quantitation of PRRSV or SIV RNA was performed using a commercial EZ-PRRSV™/Flu A MPX 1.0 with ROX, while PEDV RNA quantification was achieved using EZ-PED/TGE/PDCoV MPX 1.1 Master Mix and Enzyme with ROX (Tetracore, Rockville, MD) following the manufacturer’s instruction. The qPCR was performed using ABI 7500 Realtime PCR system. The CT values were converted to TCID50 equivalent/ml by plotting the average CT values for each sample against the standard curve created for each virus.
Results
Relative in vitro cytotoxicity of oleandrin and PBI-05204
Cytotoxicity of oleandrin and PBI-05204 were determined in MARC-145, MDCK, and Vero 76 cells used for infection by PRRSV, SIV, and PEDV, respectively (Table 1).
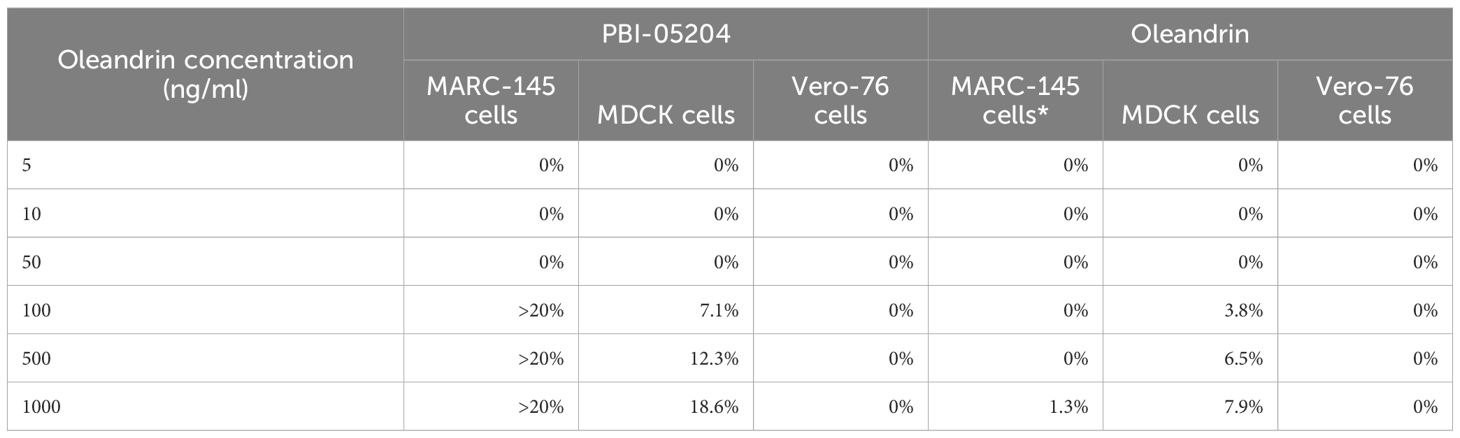
Table 1 Cytotoxicity of oleandrin and PBI-05204 on different target cells used for viral infections.
MARC-145: Monkey Kidney cells used as target cells for PRRSV infection; MDCK: Madin-Darby canine kidney cells used as target cells for SIV infection; Vero-76: African Green Monkey kidney cells used as target cells for PEDV infection. Concentrations of PBI-05204 (at equivalent oleandrin concentrations of 100 to 1000 ng/ml) exhibiting >20% cytotoxicity were not used for prophylactic or therapeutic experiments.
Significant concentration-dependent inhibition of PRRSV production and infectivity by oleandrin and PBI-05204
The MARC-145 cells were treated with different concentrations of oleandrin or PBI-05204 either 30 minutes before or 12h and 24h after infection with PRRSV. Culture supernatants collected at 24h and 48h post-infection were assessed for virus production by RT-PCR (Figure 1A) along with determining the infectivity of the progeny virus on fresh target cells (Figure 1B). Significant concentration-dependent inhibition was observed for virus production at 24h as well as 48h between 50 and 1000 ng/ml concentrations of oleandrin added to cultures either prior to infection with PRRSV or 12h and 24h post-infection. Importantly, when oleandrin was added 12h post-infection, significant inhibition of virus production was observed and was more pronounced at 48h relative to 24h.
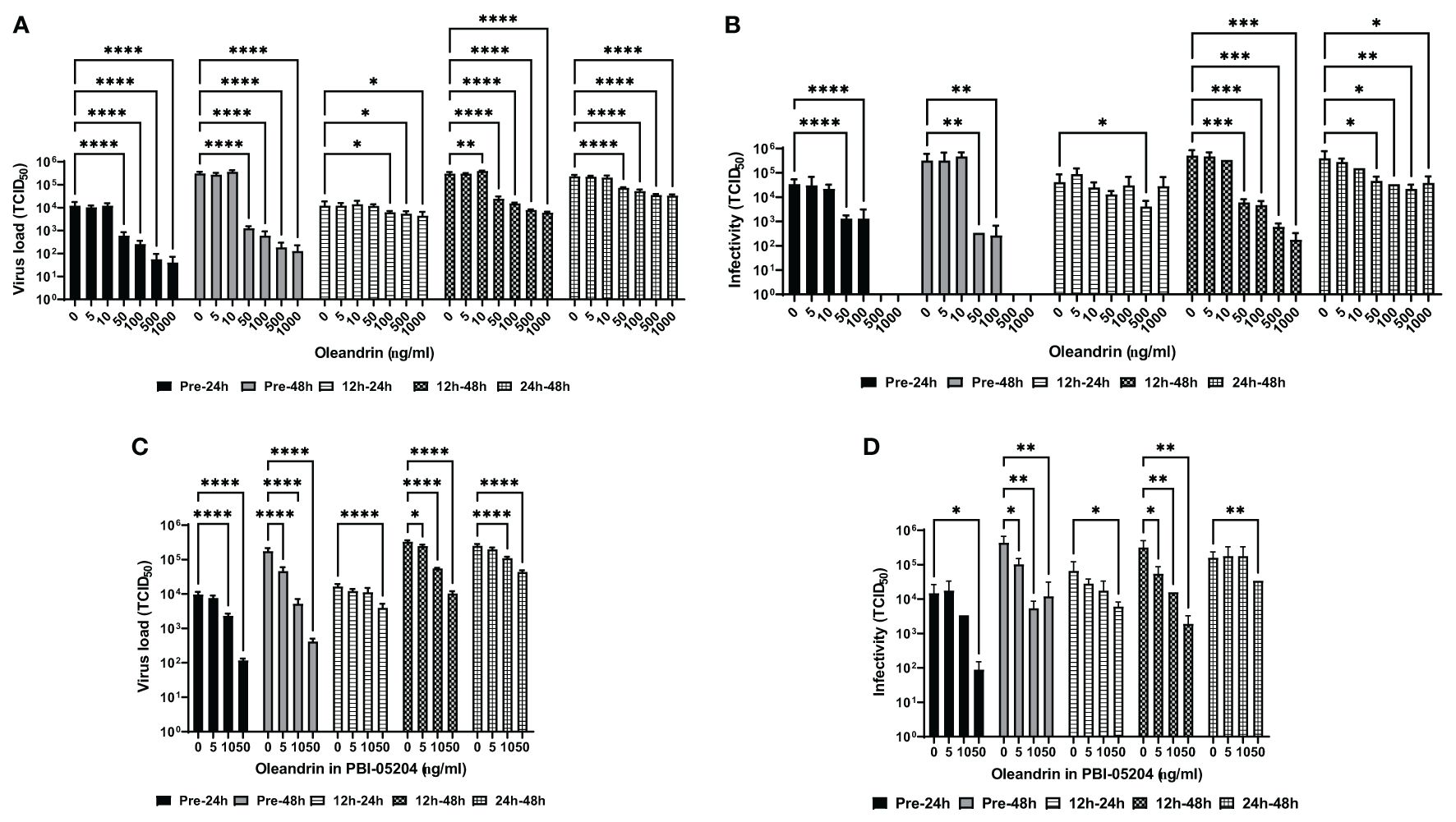
Figure 1 Oleandrin significantly inhibits production and infectivity of PRRSV. The MARC-145 cells were treated with pure oleandrin at concentrations up to 1000 ng/ml or PBI-05204, at matching oleandrin concentrations ranging between 0 and 50 ng/ml either before (Pre-24h and Pre-48h) or 12h and 24h post infection with PRRSV. Culture supernatants collected at 24h and 48h later in each case were analyzed for the virus produced and expressed as virus load (A, C) and the relative infectivity (B, D) in terms of TCID 50 values. Significance of change with each concentration (5 to 1000 ng/ml) of oleandrin relative to no oleandrin (0 ng/ml) was calculated using a 2-way ANOVA with Turkey’s multiple comparisons test. The p values of <0.05 (*), <0.005 (**), <0.0005 (***), and <0.00005 (****) are shown.
Consistent with the reduction in the virus production, a significant loss of infectivity of the progeny virus was observed when treatment with oleandrin was initiated 30 min prior to or 12 and 24h post-infection with PRRSV (Figure 1B). Interestingly, while the amount of virus produced was significantly reduced at the highest two concentrations of oleandrin tested: 500 and 1000 ng/ml, the virus produced in cultures treated with these concentrations completely lacked infectivity.
Similar oleandrin concentration dependent inhibition of virus production (Figure 1C) and infectivity (Figure 1D) of the progeny virus were observed with PBI-05204 at nontoxic cell concentrations up to and including 50 ng/ml.
Optimal oleandrin concentration for the inhibition of PRRSV production and infectivity
A comparison of fold-inhibition values for virus production and infectivity at the different concentrations of oleandrin and PBI-05204 used to treat cells at different times pre- and post-infection with PRRSV, identified 50 ng/ml as the optimum non-toxic concentration to produce the maximal inhibitory effects (Figures 2A, B).
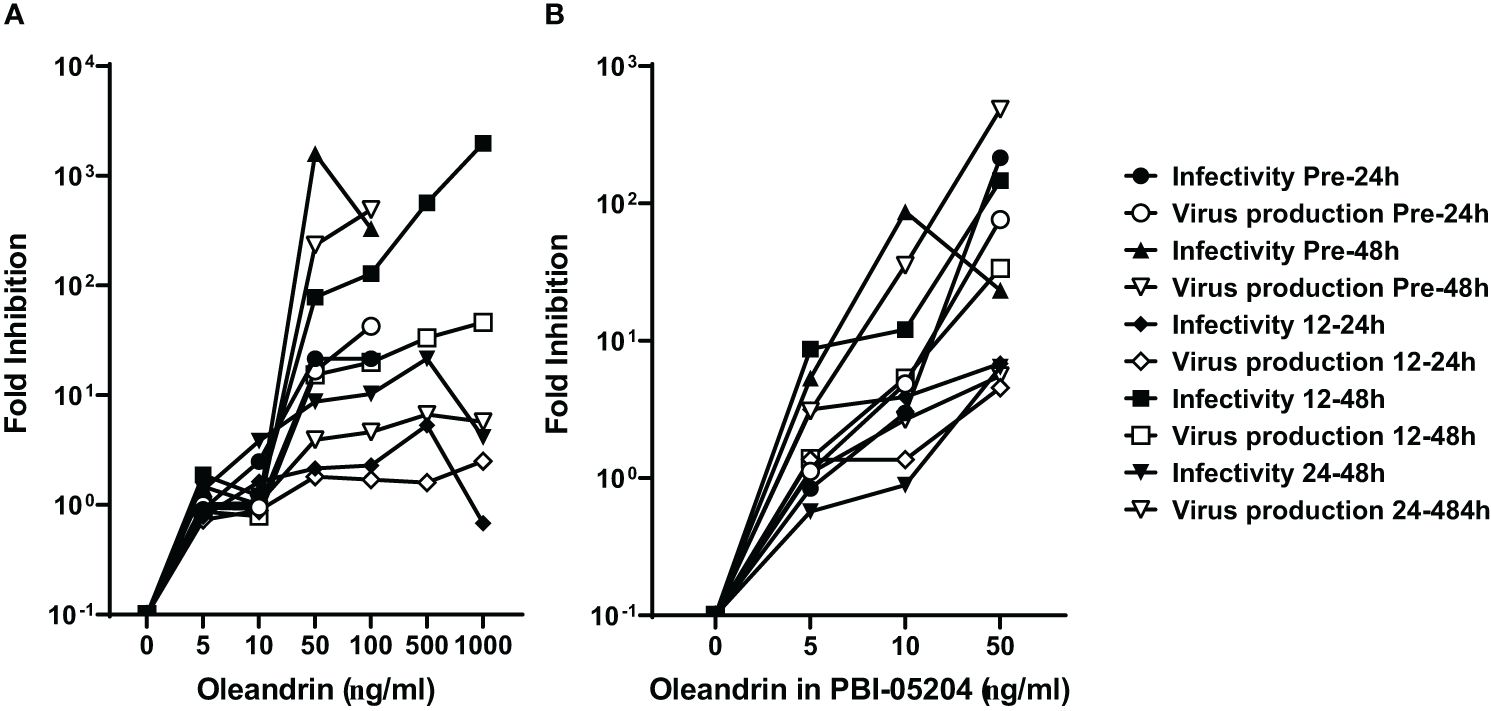
Figure 2 Concentration of oleandrin to exhibit strong inhibition of production as well as infectivity of progeny virus in PRRSV cultures. The amount of virus produced, and its relative infectivity were assessed in culture supernatants of MARC-145 cells treated with different oleandrin concentrations ranging between 0 and 1000 ng/ml (A) or matching oleandrin concentrations between 0 and 50 ng/ml in PBI-05204 (B) either prior to (Pre-24h and Pre-48h) or after (12–24h, 12–48h, and 24–48h) infection with PRRSV. Fold inhibition was calculated for each of the different oleandrin concentrations used relative to no oleandrin (0 ng/ml) and the values plotted.
Both oleandrin and PBI-05204 significantly inhibit production of infectious virus in cultures treated before infection with SIV or PEDV
Based on the results presented in Figure 2 from studies with PRRSV showing 2–3 log-fold reduction in the production of infectious virus at 50 ng/ml oleandrin, we tested oleandrin and PBI-05204 at this optimal non-toxic oleandrin concentration against two other porcine viruses SIV (Figure 3) and PEDV (Figure 4), which are of interest to commercial pig health management. Strong inhibition of virus production ranging between 378- and 8,405-fold was observed for SIV (Figure 3A) paralleling 6.8- to 40.4-fold reduction in infectivity of the progeny virus at 24h and 48h post-infection, respectively (Figure 3B). Similarly, treatment with PBI-05204 at 50 ng/ml resulted in 464- and 77,859-fold reduction in the infectivity of virus produced (Figure 3C).
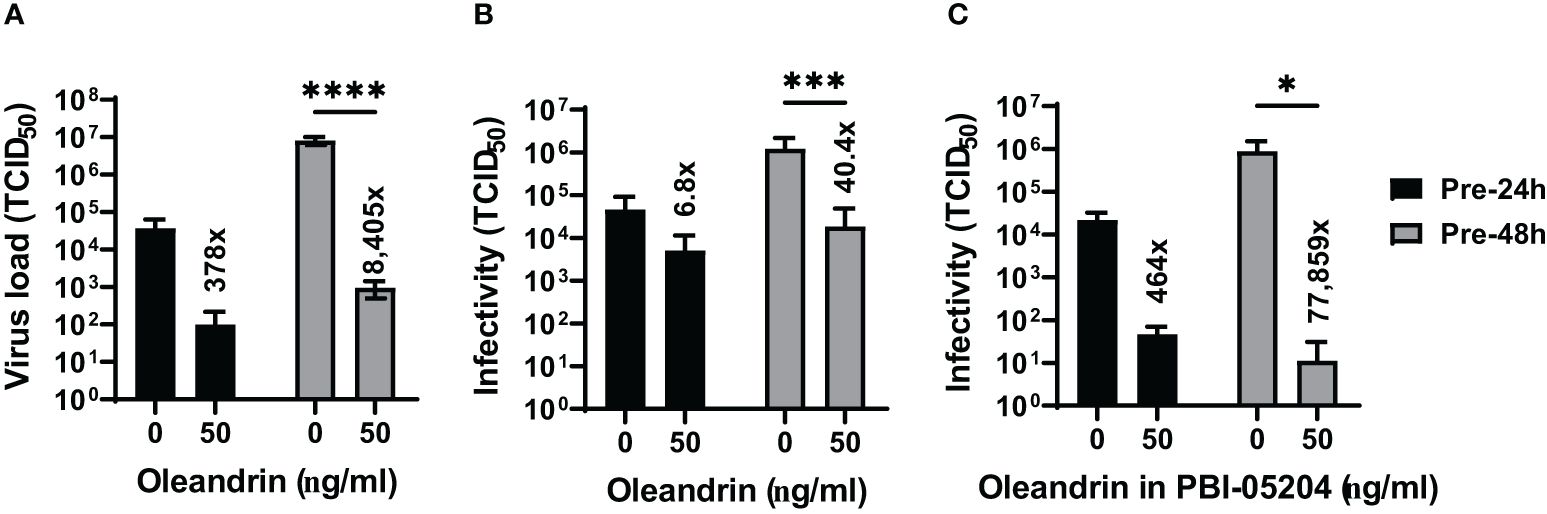
Figure 3 Oleandrin significantly inhibits production and infectivity of PEDV. Vero 76 cells were pretreated with pure oleandrin (A, B) or PBI-05204 (C), both at 50 ng/ml and the culture supernatants were collected at 24h and 48h after infection with PEDV. They were analyzed for virus production (A) and relative infectivity of the virus produced (B, C), and the TCID 50 values were plotted. Significance of change in cultures treated with 50 ng/ml oleandrin, relative to untreated (0 ng/ml) was calculated using a 2-way ANOVA with Turkey’s multiple comparisons test. The p values of <0.05 (*), <0.0005 (***) and <0.00005 (****) are shown. Also shown are fold change values for cultures treated vs untreated.
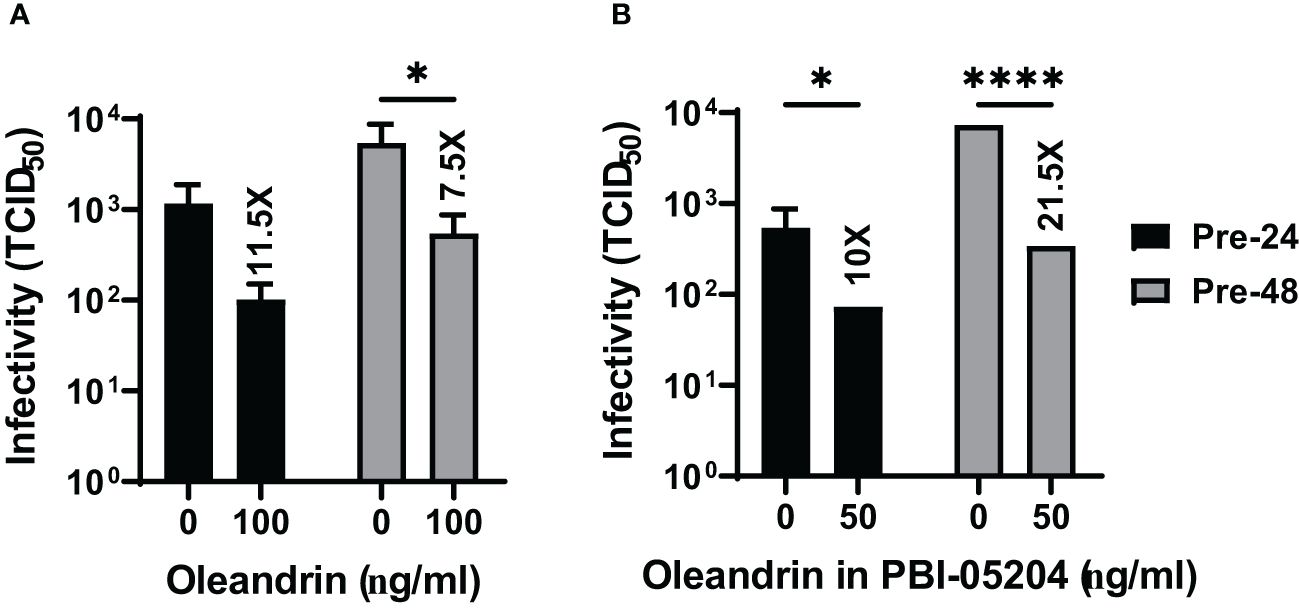
Figure 4 Oleandrin significantly inhibits progeny virus infectivity in PEDV infected cultures. Vero 76 cells were pretreated with pure oleandrin (A) or PBI-05204 (B), both at 50 ng/ml. The culture supernatants collected at 24h and 48h after infection with PEDV were analyzed for relative infectivity of the virus produced, and the TCID 50 values were plotted. Significance of change in cultures treated with 50 ng/ml oleandrin, relative to no oleandrin (0 ng/ml) was calculated using a 2-way ANOVA with Turkey’s multiple comparisons test. The p values of <0.05 (*) and <0.00005 (****) are shown. Also shown are fold change values for cultures treated vs untreated. Thus, oleandrin effectively inhibited infection of three different viruses of interest to porcine health.
We also observed that both oleandrin and PBI-05204 were effective in inhibiting the progeny virus infectivity in the cultures of PEDV infected cells (Figures 4A, B) resulting in 11.5- and 7.5-fold inhibition at 24h and 10- and 21.5-fold at 48h, respectively.
Discussion
Over the last 30 years, diseases caused by emerging swine viruses have acquired great relevance, more so than in any other species. Changes in virulence (mutation, reassortment, recombination) of the agents in the host, particularly RNA and single strand viruses that have a high mutation rate provide a difficult challenge for effective vaccine development (Shao et al., 2017). This, in turn, dictates the need for additional effective prophylactic and therapeutic treatment options beyond vaccine development. While there has been an increasing focus of the relative ability of cardenolide compounds such as oleandrin and digoxin to serve as potential antiviral therapeutic agents against viruses of importance to human health (Dunn et al., 2011; Newman et al., 2020) relatively few studies have examined the potential efficacy of cardenolides against viruses of importance to commercial animal health. We have previously reported the prophylactic and therapeutic efficacy of oleandrin against three pathogenic viruses affecting cattle production (Newman et al., 2022) but to the best of our knowledge only a single study of the antiviral efficacy of cardenolides including oleandrin has been reported against porcine transmissible gastroenteritis virus (TEGV) that affects all ages and categories of pigs, with mortality rates close to 100% in young pigs (Perfumo et al., 2020). The research presented here has demonstrated that oleandrin and PBI-05204, a defined extract of N. oleander plant leaves containing this molecule, are highly effective as antiviral agents against PRRSV, SIV and PEDV that are among the most important pathogenic viruses affecting pig health and porcine production worldwide (Ma, 2020; Perfumo et al., 2020; Ruedas-Torres et al., 2021; McLean and Graham, 2022).
The current research on porcine viruses replicates data from earlier studies with HIV that we previously reported in which oleandrin was shown to reduce viral infectivity as suggested by a reduced formation of a key viral envelope glycoprotein content in newly formed HIV viral particles as a potential mechanism for the antiviral activity (Singh et al., 2013). Future studies testing the effect of these two agents on the porcine viruses could include investigation testing for potential antiviral mechanisms. Since that report, numerous antiviral mechanisms of oleandrin and related cardenolide compounds have been reported (Yang et al., 2017; Reddy et al., 2020). These include interaction with the endoplasmic reticulum stress response that facilitates virally infected cells to undergo apoptosis (Li et al., 2020), inhibition of viral protein translation (Wong et al., 2018), inhibition of viral pre-RNA splicing (Wong et al., 2013; Grosso et al., 2017), inhibition of viral gene expression (Wong et al., 2013; Grosso et al., 2017; Reddy et al., 2020), inhibition of viral entry through ATP-mediated Src signaling and decreased content of viral envelope leading to greatly reduced progeny virus infectivity (Hutchison et al., 2019). A recent study has also demonstrated that cardenolides suppress coronaviral replication by downregulating JAK1 via a Na+/K+-ATPase independent proteolysis (Yang et al., 2020).
In addition to its ability to inhibit viral load and infectivity of progeny virus there are other pharmacologic attributes of oleandrin that may significantly impact animal health especially in the face of viral infection. Viral infections contribute to morbidity and mortality in part through an increase in inflammation and reduced immune response in humans and host animals. Oleandrin has been reported to block tumor necrosis factor (TNF)-induced activation of NF-κβ in a concentration- and time-dependent manner as well as provide inhibition of damaging oxidation reactions (Manna et al., 2000; Sreenivasan et al., 2003; Yang et al., 2020). This effect was mediated through inhibition of phosphorylation and degradation of Iκβα, an inhibitor of NF-κβ. Oleandrin also blocked AP-1 activation induced by tumor necrosis factor (TNF) and other agents and inhibited the TNF-induced activation of c-Jun NH2-terminal kinase (Sreenivasan et al., 2003; Sreenivasan et al., 2006). In an evaluation of the role of both oleandrin and PBI-05204 to affect the human innate immune system, we used an in vitro model of human peripheral blood mononuclear cells to document effects under three different culture conditions: normal, challenged with the viral mimetic polyinosinic:polycytidylic acid Poly I:C, and inflamed by lipopolysaccharide (LPS). Both PBI-05204 and oleandrin directly activated Natural Killer (NK) cells and monocytes and triggered increased production of cytokines. Under viral mimetic challenge, PBI-05204 and oleandrin enhanced the Poly I:C-mediated immune activation of monocytes and NK cells and enhanced production of IFN-γ. Under inflammatory conditions, many cytokines were controlled at similar levels as in cultures treated with PBI-05204 and oleandrin without inflammation (Jensen et al., 2023). The results show that PBI-05204 and oleandrin directly activate innate immune cells, enhance anti-viral immune responses through NK cell activation and IFN-γ levels, and modulate immune responses under inflamed conditions.
A limitation to the present study is that only a single representative variant of each virus was investigated. The data suggest, however, that due to multiple mechanisms of antiviral activity as well that the data may be applicable to additional variants present currently or those to arise in the future. Vaccines are targeted to specific viral variants and rarely afford broad protection against a broad array of viral species. Fortunately, the broader antiviral potential of selected plants and their isolated natural compounds is now appreciated (Akram et al., 2018; Ben-Shabat et al., 2020; Adhikari et al., 2021; de Oliveira et al., 2022). The broad antiviral activities of oleandrin against viruses affecting bovine health as well as those demonstrating adverse health of humans would suggest that it, and appropriately defined and characterized extracts containing this molecule, might very well offer broad viral variant protection.
Like most compounds that are used for therapeutic effect, CGs including oleandrin can be toxic at certain concentrations or doses. While in vivo pilot studies of PBI-05204 and related products prepared from N. oleander in pigs have yet to be carried out, human clinical trials with PBI-05204 have shown that it can be safely administered to cancer patients without serious adverse consequences (Hong et al., 2014; Roth et al., 2020). The documented plasma concentrations of oleandrin achieved in these clinical trials believed to be important for elimination of malignant cell proliferation are significantly higher than the low concentrations shown in the present study to be effective for virus control. It is of obvious importance to carry out full toxicology studies in appropriate pig populations including piglets and sows. Such studies are currently being planned.
Data availability statement
The raw data supporting the conclusions of this article will be made available by the authors, without undue reservation.
Ethics statement
Ethical approval was not required for the studies on animals in accordance with the local legislation and institutional requirements because only commercially available established cell lines were used.
Author contributions
RN: Conceptualization, Formal analysis, Funding acquisition, Resources, Supervision, Writing – original draft, Writing – review & editing. KA: Formal analysis, Investigation, Methodology, Visualization, Writing – review & editing, Writing – original draft. RB: Conceptualization, Formal analysis, Methodology, Writing – original draft, Writing – review & editing, Supervision. KS: Conceptualization, Formal analysis, Methodology, Writing – original draft, Writing – review & editing.
Funding
The author(s) declare financial support was received for the research, authorship, and/or publication of this article. This work was supported by Phoenix Biotechnology Inc.
Acknowledgments
We gratefully acknowledge the support and helpful comments from Sonja Van Holland and Christopher C.L. Chase, PhD.
Conflict of interest
Author RN serves as a Director of Phoenix Biotechnology, Inc., the company that provided funds to do the research. Author JS is a paid consultant to Phoenix Biotechnology, Inc. providing expertise in antiviral research.
The remaining authors declare that the research was conducted in the absence of any commercial or financial relationships that could be construed as a potential conflict of interest.
Publisher’s note
All claims expressed in this article are solely those of the authors and do not necessarily represent those of their affiliated organizations, or those of the publisher, the editors and the reviewers. Any product that may be evaluated in this article, or claim that may be made by its manufacturer, is not guaranteed or endorsed by the publisher.
References
Adhikari B., Marasini B. P., Rayamajhee B., Bhattarai B. R., Lamichhane G., Khadayat K., et al. (2021). Potential roles of medicinal plants for the treatment of viral diseases focusing on COVID-19: A review. Phytother. Res. 35, 1298–1312. doi: 10.1002/ptr.6893
Akram M., Tahir I. M., Shah S. M. A., Mahmood Z., Altaf A., Ahmad K., et al. (2018). Antiviral potential of medicinal plants against HIV, HSV, influenza, hepatitis, and coxsackievirus: A systematic review. Phytother. Res. 32, 811–822. doi: 10.1002/ptr.6024
Andraud M., Rose N. (2020). Modelling infectious viral diseases in swine populations: a state of the art. Porcine Health Manag 20, 6:22. doi: 10.1186/s40813-020-00160-4
Ben-Shabat S., Yarmolinsky L., Porat D., Dahan A. (2020). Antiviral effect of phytochemicals from medicinal plants: Applications and drug delivery strategies. Drug Delivery Transl. Res. 10, 354–367. doi: 10.1007/s13346-019-00691-6
Cai H., Zhang H., Cheng H., Liu M., Wen S., Ren J. (2023). Progress in PRRSV infection and adaptive immune response mechanisms. Viruses 15, 1442. doi: 10.3390/v15071442
Chand R. J., Trible B. R., Rowland R. R. (2012). Pathogenesis of porcine reproductive and respiratory syndrome virus. Curr. Opin. Viro. 2, 256–263. doi: 10.1016/j.coviro.2012.02.002
Cheng J., Sun N., Zhao X., Niu L., Song M., Sun Y., et al. (2013). In vitro screening for compounds derived from traditional Chinese medicines with antiviral activities against porcine reproductive and respiratory syndrome virus. J. Microbiol. Biotechnol. 23, 1076–1083. doi: 10.4014/jmb
Collins J. E., Benfield D. A., Christianson W. T., Harris L., Hennings J. C., Shaw D. P., et al. (1992). Isolation of swine infertility and respiratory syndrome virus (isolate ATCC VR-2332) in North America and experimental reproduction of the disease in gnotobiotic pigs. J. Vet. Diagn. Invest. 4, 117–126. doi: 10.1177/104063879200400201
Cornelison A. S., Karriker L. A., Williams N. H., Haberl B. J., Stalder K. J., Schulz L. L., et al. (2018). Impact of health challenges on pig growth performance, carcass characteristics, and net returns under commercial conditions. Transl. Anim. Sci. 2, 50–61. doi: 10.1093/tas/txx005
Darbeheshti F., Mahdiannasser M., Uhal B. D., Ogino S., Gupta S., Rezaei N. (2021). Interindividual immunogenic variants: Susceptibility to coronavirus, respiratory syncytial virus and influenza virus. Rev. Med. Virol. 31, e2234. doi: 10.1002/rmv.2234
de Oliveira J. R., Antunes B. S., do Nascimento G. O., Kawall J. C. S., Oliveira J. V. B., Silva K. G. D. S., et al. (2022). Antiviral activity of medicinal plant-derived products against SARS-CoV-2. Exp. Biol. Med. (Maywood) 247, 1797–1809. doi: 10.1177/15353702221108915
Du T., Nan Y., Xiao S., Zhao Q., Zhou E. M. (2017). Antiviral strategies against PRRSV infection. Trends Microbiol. 25, 968–979. doi: 10.1016/j.tim.2017.06.001
Dunn D. E., He D. N., Yang P., Johansen M., Newman R. A., Lo D. C. (2011). In vitro and in vivo neuroprotective activity of the cardiac glycoside oleandrin from Nerium oleander in brain slice-based stroke models. J. Neurochem. 119, 805–814. doi: 10.1111/j.1471-4159.2011.07439.x
Grosso F., Stoilov P., Lingwood C., Brown M., Cochrane A. (2017). Suppression of adenovirus replication by cardiotonic steroids. J. Virol. 91, e01623–e01616. doi: 10.1128/JVI.01623-16
Hong D. S., Henary H., Falchook G. S., Naing A., Fu S., Moulder S., et al. (2014). First-in-human study of pbi-05204, an oleander-derived inhibitor of akt, fgf-2, nf-κB and p70s6k, in patients with advanced solid tumors. Invest. New Drugs 32, 1204–1212. doi: 10.1007/s10637-014-0127-0
Hutchison T., Yapindi L., Malu A., Newman R. A., Sastry K. J., Harrod R. (2019). The botanical glycoside oleandrin inhibits human T-cell leukemia virus type-1 infectivity and env-dependent virological synapse formation. J. Antivir Antiretrovir 11, 184. doi: 10.35248/1948-5964
Jensen G. S., Yu L., Iloba I., Cruickshank D., Matos J. R., Newman R. A. (2023). Differential activities of the botanical extract PBI-05204 and oleandrin on innate immune functions under viral challenge versus inflammatory culture conditions. Molecules 28, 4799. doi: 10.3390/molecules28124799
Lerner H., Berg C. (2015). The concept of health in One Health and some practical implications for research and education: what is One Health? Infect. Ecol. Epidemiol. 5, 25300. doi: 10.3402/iee.v5.25300
Li X. X., Wang D. Q., Sui C. G., Meng F. D., Sun S. L., Zheng J., et al. (2020). Oleandrin induces apoptosis via activating endoplasmic reticulum stress in breast cancer cells. BioMed. Pharmacother. 124, 109852. doi: 10.1016/j.biopha.2020.109852
Liu Q., Wang H. Y. (2021). Porcine enteric coronaviruses: an updated overview of the pathogenesis, prevalence, and diagnosis. Vet. Res. Commun. 45, 75–86. doi: 10.1007/s11259-021-09808-0
Lunney J. K., Fang Y., Ladinig A., Chen N., Li Y., Rowland B., et al. (2016). Porcine reproductive and respiratory syndrome virus (PRRSV): pathogenesis and interaction with the immune system. Annu. Rev. Anim. Biosci. 4, 129–154. doi: 10.1146/annurev-animal-022114-111025
Ma W. (2020). Swine influenza virus: Current status and challenge. Virus Res. 288, 198118. doi: 10.1016/j.virusres.2020.198118
Ma J., Ma L., Yang M., Wu W., Feng W., Chen Z. (2021). The function of the PRRSV-host interactions and their effects on viral replication and propagation in antiviral strategies. Vaccines (Basel). 9, 364. doi: 10.3390/vaccines9040364
Ma W., Richt J. A. (2010). Swine influenza vaccines: current status and future perspectives. Anim. Health Res. Rev. 11, 81–96. doi: 10.1017/S146625231000006X
Mancera Gracia J. C., Pearce D. S., Masic A., Balasch M. (2020). Influenza A virus in swine: epidemiology, challenges and vaccination strategies. Front. Vet. Sci. 7, 647. doi: 10.3389/fvets.2020.00647
Manna S. K., Sah N. K., Newman R. A., Cisneros A., Aggarwal B. B. (2000). Oleandrin suppresses activation of nuclear transcription factor-kappaB, activator protein-1, and c-Jun NH2-terminal kinase. Cancer Res. 60, 3838–3847.
McLean R. K., Graham S. P. (2022). The pig as an amplifying host for new and emerging zoonotic viruses. One Health 1, 100384. doi: 10.1016/j.onehlt.2022.100384
Montaner-Tarbes S., Del Portillo H. A., Montoya M., Fraile L. (2019). Key gaps in the knowledge of the porcine respiratory reproductive syndrome virus (PRRSV). Front. Vet. Sci. 6, 38. doi: 10.3389/fvets.2019.00038
Newman R. A., Chase C. C. L., Matos J. R., Abdelsalam K., Buterbaugh R., Van Holland S., et al. (2022). Efficacy of oleandrin and PBI-05204 against bovine viruses of importance to commercial cattle health. Antivir Chem. Chemother. 30, 20402066221103960. doi: 10.1177/20402066221103960
Newman R. A., Sastry K. J., Arav-Boger R., Cai H., Matos R., Harrod R. (2020). Antiviral effects of oleandrin. J. Exp. Pharmacol. 12, 503–515. doi: 10.2147/JEP.S273120
Perfumo C. J., Pereda A., Jongkaewwattana A., Chen Z., Perez D. R., Ma J. (2020). Editorial: emerging swine viruses. Front. Vet. Sci. 7, 132. doi: 10.3389/fvets.2020.00132
Rahn J., Hoffmann D., Harder T. C., Beer M. (2015). Vaccines against influenza A viruses in poultry and swine: Status and future developments. Vaccine 33, 2414–2424. doi: 10.1016/j.vaccine.2015.03.052
Reddy D., Kumavath R., Barh D., Azevedo V., Ghosh P. (2020). Anticancer and antiviral properties of cardiac glycosides: A review to explore the mechanism of actions. Molecules 25, 3596. doi: 10.3390/molecules25163596
Roth M. T., Cardin D. B., Borazanci E. H., Steinbach M., Picozzi V. J., Rosemury A., et al. (2020). Single-arm, open-label, bayesian adaptive efficacy and safety study of PBI-05204 in patients with stage IV metastatic pancreatic adenocarcinoma. Oncologist 25, e1446–e1450. doi: 10.1634/theoncologist.2020-0440
Ruedas-Torres I., Rodríguez-Gómez I. M., Sánchez-Carvajal J. M., Larenas-Muñoz F., Pallarés F. J., Carrasco L., et al. (2021). The jigsaw of PRRSV virulence. Vet. Microbiol. 260, 109168. doi: 10.1016/j.vetmic.2021.109168
Shao W., Li X., Goraya M. U., Wang S., Chen J. L. (2017). Evolution of influenza A virus by mutation and re-assortment. Int. J. Mol. Sci. 18, 1650. doi: 10.3390/ijms18081650
Siddiqui B. S., Khatoon N., Begum S., Farooq A. D., Qamar K., Bhatti H. A., et al. (2012). Flavonoid and cardenolide glycosides and a pentacyclic triterpene from the leaves of Nerium oleander and evaluation of cytotoxicity. Phytochemistry 77, 238–244. doi: 10.1016/j.phytochem.2012.01.001
Simon M. A., Chalifoux L. V., Ringler D. J. (1992). Pathologic features of SIV-induced disease and the association of macrophage infection with disease evolution. AIDS Res. Hum. Retroviruses 8, 327–337. doi: 10.1089/aid.1992.8.327
Singh S., Shenoy S., Nehete P. N., Yang P., Nehete B., Fontenot D., et al. (2013). Nerium oleander derived cardiac glycoside oleandrin is a novel inhibitor of HIV infectivity. Fitoterapia 84, 32–39. doi: 10.1016/j.fitote.2012.10.017
Sreenivasan Y., Raghavendra P. B., Manna S. K. (2006). Oleandrin-mediated expression of Fas potentiates apoptosis in tumor cells. J. Clin. Immunol. 26, 308–322. doi: 10.1007/s10875-006-9028-0
Sreenivasan Y., Sarkar A., Manna S. K. (2003). Oleandrin suppresses activation of nuclear transcription factor-kappa B and activator protein-1 and potentiates apoptosis induced by ceramide. Biochem. Pharmacol. 66, 2223–2239. doi: 10.1016/j.bcp.2003.07.010
VanderWaal K., Deen J. (2018). Global trends in infectious diseases of swine. Proc. Natl. Acad. Sci. U.S.A. 115, 11495–11500. doi: 10.1073/pnas.1806068115
Wensvoort G., Terpstra C., Pol J. M., ter Laak E. A., Bloemraad M., de Kluyver E. P., et al. (1991). Mystery swine disease in The Netherlands: the isolation of Lelystad virus. Vet. Q 13, 121–130. doi: 10.1080/01652176.1991.9694296
Wong R. W., Balachandran A., Ostrowski M. A., Cochrane A. (2013). Digoxin suppresses HIV-1 replication by altering viral RNA processing. PloS Pathog. 9, e1003241. doi: 10.1371/journal.ppat.1003241
Wong R. W., Lingwood C. A., Ostrowski M. A., Cabral T., Cochrane A. (2018). Cardiac glycoside/aglycones inhibit HIV-1 gene expression by a mechanism requiring MEK1/2-ERK1/2 signaling. Sci. Rep. 8, 850. doi: 10.1038/s41598-018-19298-x
Yang C. W., Chang H. Y., Hsu H. Y., Lee Y. Z., Chang H. S., Chen I. S., et al. (2017). Identification of anti-viral activity of the cardenolides, Na+/K+-ATPase inhibitors, against porcine transmissible gastroenteritis virus. Toxicol. Appl. Pharmacol. 332, 129–137. doi: 10.1016/j.taap.2017.04.017
Yang C. W., Hsu H. Y., Chang H. Y., Lee Y. Z., Lee S. J. (2020). Natural cardenolides suppress coronaviral replication by downregulating JAK1 via a Na+/K+-ATPase independent proteolysis. Biochem. Pharmacol. 180, 114122. doi: 10.1016/j.bcp.2020.114122
Zhang Y., Chen Y., Zhou J., Wang X., Ma L., Li J., et al. (2022). Porcine epidemic diarrhea virus: an updated overview of virus epidemiology, virulence variation patterns and virus-host interactions. Viruses 14, 2434. doi: 10.3390/v14112434
Zhang H., Zou C., Peng O., Ashraf U., Xu Q., Gong L., et al. (2023). Global dynamics of porcine enteric coronavirus PEDV epidemiology, evolution, and transmission. Mol. Biol. Evol. 40, msad052. doi: 10.1093/molbev/msad052
Keywords: Nerium oleander, porcine viruses, antiviral, in vitro, control
Citation: Newman RA, Abdelsalam K, Buterbaugh R and Sastry KJ (2024) Efficacy of oleandrin and PBI-05204 against viruses of importance to commercial pig health management. Front. Anim. Sci. 5:1359681. doi: 10.3389/fanim.2024.1359681
Received: 21 December 2023; Accepted: 23 May 2024;
Published: 05 June 2024.
Edited by:
Pasquale De Palo, University of Bari Aldo Moro, ItalyReviewed by:
Pradip Kumar Das, West Bengal University of Animal and Fishery Sciences, IndiaShouwen Du, Guangdong Academy of Agricultural Sciences, China
Copyright © 2024 Newman, Abdelsalam, Buterbaugh and Sastry. This is an open-access article distributed under the terms of the Creative Commons Attribution License (CC BY). The use, distribution or reproduction in other forums is permitted, provided the original author(s) and the copyright owner(s) are credited and that the original publication in this journal is cited, in accordance with accepted academic practice. No use, distribution or reproduction is permitted which does not comply with these terms.
*Correspondence: Robert A. Newman, bmV3bWFuc2NpZW50aWZpY2NvbnN1bHRpbmdAZ21haWwuY29t
†ORCID: Robert A. Newman, orcid.org/0000-0001-5661-5846
K. Jagannadha Sastry, orcid.org/0000-0002-6987-4422
Karim Abdelsalam, orcid.org/0000-0001-6747-257X