- Department of Animal Science, Texas A&M University, College Station, TX, United States
Fatty acids are important components of foods derived from livestock species, as they contribute to the healthfulness and benefits of beef and beef products. Oleic acid (18:1n-9) is the most highly regulated and most abundant fatty acid in animal tissue. The greatest risk factor for cardiovascular disease (CVD) is low circulating high-density lipoprotein cholesterol (HDL-C), and consumption of beef naturally enriched with oleic acid increases plasma HDL-C concentrations in men and women. Oleic acid is synthesized by the activity of stearoyl-coenzyme A (CoA) desaturase (SCD). In cattle, SCD activity and SCD1 gene expression are highest in adipose tissue depots, followed by skeletal muscle, intestinal mucosa, and the liver. Early studies demonstrated that the concentration of oleic acid beef contributes to positive flavor attributes, but this finding has been difficult to replicate in more recent studies. Including grain (especially corn) in the finishing diets of cattle is essential for the upregulation of SCD1 expression and activity. The measurement of SCD activity is technically difficult, but quantifying SCD gene expression or the concentration of palmitoleic acid (16:1n-7) in beef often provides insight into SCD activity. DNA polymorphisms in SCD1, the sterol regulatory element binding protein-1, the fatty acid synthase, and the growth hormone are associated with oleic acid concentration in the muscle of Japanese Black cattle, indicating a strong genetic component to the regulation of fatty acid composition of beef.
Introduction
Unlike the essential fatty acids, linoleic acid (18:2n-6) and α-linolenic acid (18:3n-3), the concentration of oleic acid (18:1n-9) can readily be increased in bovine adipose tissue and muscle. Oleic acid is the most abundant fatty acid in the bovine muscle, subcutaneous adipose tissue, and intramuscular adipose tissue (Westerling and Hedrick, 1979; St. John et al., 1987; Sturdivant et al., 1992; May et al., 1993; Smith et al., 1998; Chung et al., 2006; Brooks et al., 2011). Oleic acid is produced by the activity of the Δ9 desaturase, stearoyl-coenzyme A (CoA) desaturase (SCD), and SCD is encoded by stearoyl-CoA desaturase-1 (SCD1), which is expressed in virtually all bovine tissues. This mini-review describes the effects of oleic acid-enriched beef on risk factors for cardiovascular disease (CVD); the relationship between oleic acid and sensory attributes in beef; and dietary and genetic factors regulating SCD1 gene expression and SCD activity in bovine tissues.
Oleic acid and risk factors for CVD
We have demonstrated that the increased consumption of oleic acid-enriched ground beef increases plasma high-density lipoprotein cholesterol (HDL-C) concentration from baseline (Adams et al., 2010; Gilmore et al., 2011; Gilmore et al., 2013). The subjects for Adams et al. (2010) were mildly hypercholesterolemic men; those for Gilmore et al. (2011) were normocholesterolemic men; those for Gilmore et al. (2013) were normocholesterolemic, postmenopausal women; and those for Choi et al. (2018) were normocholesterolemic, postmenopausal women and mildly hypercholesterolemic, older men. Adams et al. (2010) compared the effects of ground beef formulated from lean and fat trim from grass-fed (low oleic acid) and grain-fed (high oleic acid) Wagyu steers and conventional cattle (Table 1). Gilmore et al. (2011) prepared ground beef patties from lean and fat trim from 20-month-old Angus steers raised solely on pasture and hay (low oleic acid) and Angus steers fed a corn-based feedlot diet for 8 months following weaning (high oleic acid). Gilmore et al. (2013) produced patties from retail chub pack ground beef (low oleic acid) and utilized premade ground beef patties formulated from Akaushi (American Red Wagyu) steers (high oleic acid). For Trials 1–3, the high oleic-acid ground beef patties contained 2–3 g more oleic acid per patty than the low oleic-acid patties (Table 1).
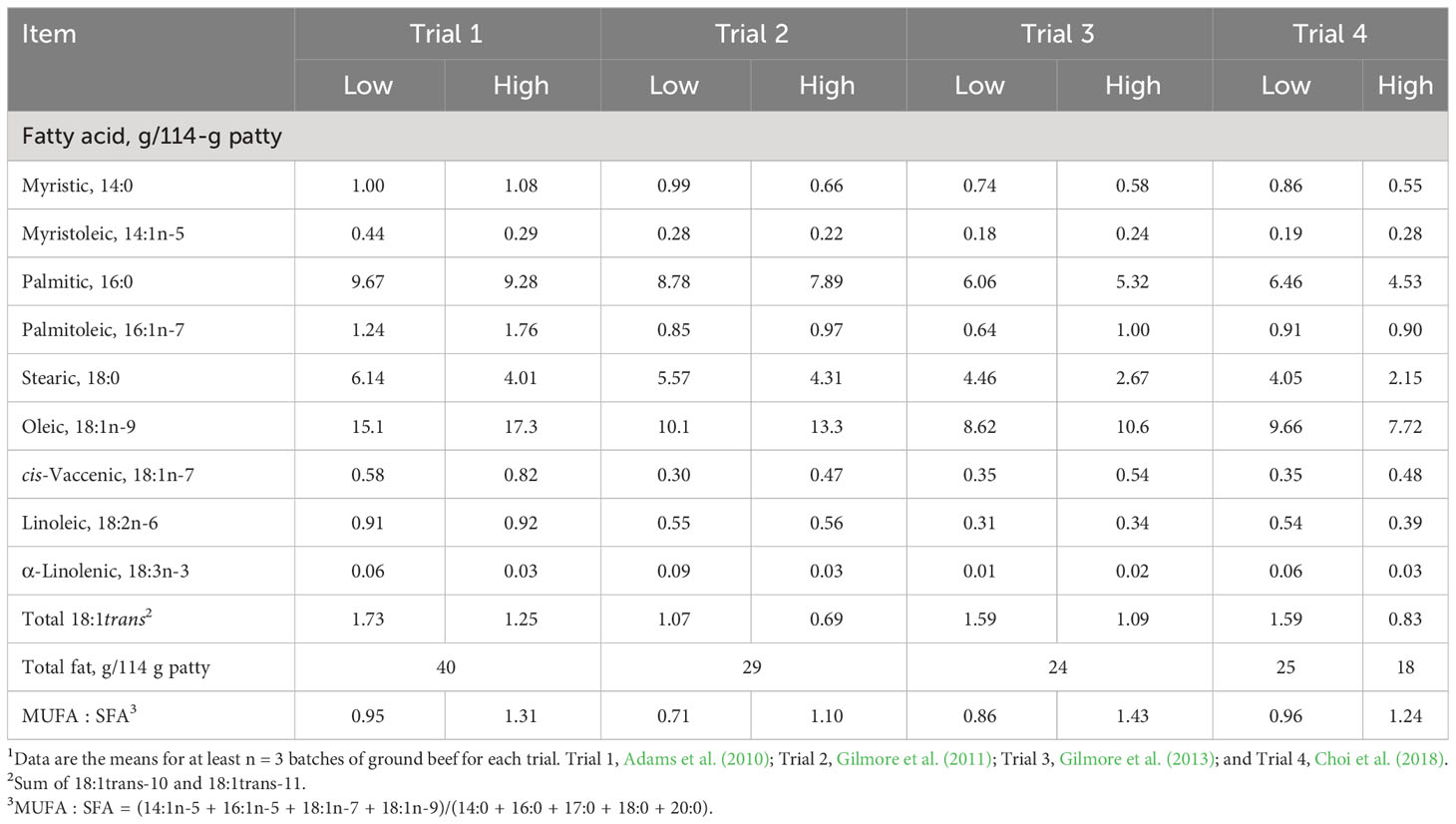
Table 1 Characteristics of low oleic-acid (Low) and high oleic-acid (High) ground beef used in four randomized, controlled trials1.
In an effort to create ground beef patties that contained similar amounts of oleic acid, we formulated patties from chub pack ground beef (low oleic acid; 25% fat) and used premade Akaushi patties (high oleic acid; 20% fat) (Choi et al., 2018). However, after fatty acid analysis of the patties, we found that the chub pack patties contained more oleic acid (9.66 g/patty) than the Akaushi patties (7.72 g oleic acid/patty). We continue to refer to the Akaushi ground beef as high oleic acid because the monounsaturated fatty acid (MUFA):saturated fatty acid (SFA) ratio was higher in Akaushi patties (1.24) than in chub pack patties (0.96).
In Trial 1, men first consumed low oleic-acid ground beef (5 patties/week for 5 weeks) followed by a 3-week washout, during which they returned to their habitual diet. Following the washout period, the men consumed the high oleic-acid ground beef (5 patties/week for 5 weeks). During the first ground beef intervention period, the low oleic-ground beef decreased the plasma HDL-C concentration. During the second ground beef intervention period, the high oleic-acid intervention increased the plasma HDL-C concentration to pretrial levels. Trials 2–4 were randomized crossover trials in which participants consumed low oleic-acid or high oleic-acid ground beef (five 114-g patties/week for 5 weeks) and following a 4-week washout period, consumed the opposite type of ground beef. In Trials 2 and 3, the high oleic-acid ground beef increased plasma HDL-C concentration (Gilmore et al., 2011; Gilmore et al., 2013). Choi et al. (2018) demonstrated that high oleic-acid (Akaushi) ground beef had no effect on HDL-C concentration but increased the plasma concentration of the most buoyant and more healthful low-density lipoprotein cholesterol (LDL-C) fractions, LDL1-C plus LDL2-C, by over 4 mg/dL. On average, Trials 1–4 demonstrated a 3–4 mg/dL increase in HDL-C concentration when men and women consumed high oleic-acid ground beef as compared to conventional ground beef (Smith et al., 2020). A combined analysis of the Framingham Heart Study (1,428 participants), the Lipid Research Clinics Prevalence Mortality Follow-up Study (6,234 participants), the Lipid Research Clinics Coronary Primary Prevention Trial (1,808 participants), and the Multiple Risk Factor Intervention Trial (5,792 participants) concluded that a 1 mg/dL increase in HDL-C was associated with a 2%–3% depression in CVD mortality (Gordon et al., 1989). Therefore, even a relatively small increase in HDL-C concentration might reduce risk for CVD.
In Trial 2, both low and high oleic-acid ground beef decreased HDL2-C and HDL3 particle diameters (Gilmore et al., 2011). Roussell et al. (2012) reported that a Beef in an Optimal Lean Diet (BOLD) depressed HDL-C concentrations in men and women, and Wu et al. (2021) documented that BOLD decreased the abundance of the larger HDL2b lipoprotein particles. Lytle et al. (2023) reported that consumption of lean ground beef (5% fat) decreased HDL-C concentration in men and depressed the abundance of the larger HDL2b and HDL2a lipoprotein particles. The effects of these lean beef interventions might explain the decreased HDL2 and HDL3 particle size reported by Gilmore et al. (2011).
Fatty acids and beef sensory attributes
For over six decades, researchers have attempted to establish a relationship between fatty acid composition and beef palatability. Waldman et al. (1968) investigated the relationship of palatability traits with the percentage of fatty acids in lipids from three adipose tissue depots and the longissimus muscle (LM) but there were no significant associations among fatty acid composition, LM tenderness, juiciness, or flavor. Dryden and Marchello (1970) reported that the percentage of oleic acid was positively correlated with beef flavor, but not juiciness, in LM intramuscular lipid (IML), whereas linoleic acid was negatively correlated with juiciness. Westerling and Hedrick (1979) compared fatty acid composition and the flavor characteristics of steers and heifers fed fescue pasture or fescue pasture plus grain. Beef flavor score increased with time for those fed the grain-based diet and was positively correlated with oleic acid and negatively correlated with palmitic acid (16:0), stearic acid (18:0), and linoleic acid. Melton et al. (1982a) reported a significant, positive correlation with flavor score and oleic acid in beef from grass-finished, forage-grain-finished, and grain-finished steers. Melton et al. (1982b) demonstrated that the percentage of oleic acid was low and that stearic acid was higher in ground beef from steers backgrounded on pasture than in ground beef from steers that had been fed a cracked corn finishing diet. Positive flavor descriptors increased with days on feed and negative flavor descriptors declined with days on feed, and oleic acid was negatively correlated with negative flavor descriptors. Conversely, stearic and α-linolenic acids were positively correlated with negative flavor descriptors. Mandell et al. (1998) compared beef from forage-fed cattle to beef from grain-fed cattle and surmised that higher concentrations of linoleic acid and lower concentrations of oleic acid may have been responsible for the differences in beef flavor.
It has been more difficult to establish a relationship between fatty acid composition and beef flavor attributes when cattle are feedlot-finished the same number of days. Gilbert et al. (2003) fed Brangus steers cracked corn, casein-formaldehyde-protected canola lipid (high in oleic acid), or casein-formaldehyde-protected starch (which also contained canola oil). There was no difference in the oleic acid concentration of subcutaneous or intramuscular adipose tissue or in descriptive meat sensory or flavor attributes among treatments. Blackmon et al. (2015) produced ground beef from beef brisket, flank, and plate primals. Brisket is especially high in oleic acid (Turk and Smith, 2009; Smith et al., 2012; Smith et al., 2020) and the ground beef formulated from the brisket primal contained more oleic acid than ground beef from the flank or plate (Blackmon et al., 2015). Brisket ground beef had greater bloody/serumy and fat-like sensory attributes than ground beef from the flank, but there was no correlation between oleic acid concentration and any sensory panel flavor attributes. Kerth et al. (2015) formulated ground beef containing fat trim from the brisket, chuck, flank, and round. Brisket ground beef contained slightly more oleic acid than ground beef formulated from the other fat trims but there were no differences in consumer sensory traits.
Frank et al. (2016) reported the influence of intramuscular fat, animal feed, and breed type on sensory characteristics, chemical characteristics, and fatty acid composition of Australian Angus and Wagyu cattle. Although they observed several significant relationships between the percentages of IML, sensory attributes, and headspace volatile compounds, Frank et al. (2016) did not report associations among fatty acids and sensory characteristics. Chen et al. (2022) documented muscle fatty acids, beef flavor compounds, and beef flavors in Angus and Xianxi Yellow cattle. Although they reported principal component analysis (PCA) for flavor compounds and flavor attributes, a PCA was not reported for beef fatty acids and flavor attributes. We interpret the results of Frank et al. (2016) and Chen et al. (2022) to mean that there was no relationship between beef fatty acids and any sensory attributes. We conclude that there is little correlation between the concentration of fatty acids and flavor attributes of beef unless there are differences in the production of cattle (e.g., grass feeding vs. grain feeding).
Fatty acid composition, SCD activity, and SCD1 gene expression
As marbling score increases across a broad range of production conditions, the concentration and amount of oleic acid increase, and there is a concomitant decrease in stearic acid in bovine adipose tissue (Waldman et al., 1968; Chung et al., 2006; Brooks et al., 2011; Legako et al., 2015; Frank et al., 2016). In ruminants, dietary oleic acid is largely hydrogenated to stearic acid by ruminal microorganisms before reaching the abomasum (St. John et al., 1991; Ekeren et al., 1992). For bovine tissues, SCD activity is highest in adipose tissues but readily detectable in intestinal mucosal cells (St. John et al., 1991; Chang et al., 1992; Page et al., 1997; Archibeque et al., 2005). We interpret this to mean that duodenal stearic acid is converted in large part to oleic acid before being incorporated into chylomicrons. The primary source of oleic acid in bovine adipose tissue is de novo synthesis. Glucose, acetate, and lactate can be incorporated in fatty acid in bovine subcutaneous and intramuscular adipose tissues (Whitehurst et al., 1978; Smith and Prior, 1981; Smith, 1983; Smith and Crouse, 1984). The fatty acid synthase reaction produces palmitic acid and a small amount of myristic acid (14:0); stearic acid is synthesized by fatty acid elongase; and oleic acid is produced by desaturating stearic acid via SCD. Small amounts of palmitoleic acid (16:1n-7) are also generated by the desaturation of palmitic acid.
Percentages of palmitoleic acid in bovine tissues may provide evidence for current and/or past SCD activity (Smith et al., 1998). Subcutaneous adipose tissue from Japanese Black steers produced in Japan contained 5.2% palmitoleic acid, and subcutaneous adipose tissues from cattle raised in Australia and fed wheat and/or barley contained 1.6% palmitoleic acid (Smith et al., 1998). The proportions of stearic acid for these two groups of steers were 7.6% and 26.1%, respectively. These results illustrate the impact of finishing diets on the fatty acid composition of bovine adipose tissue.
Several studies have documented higher SCD1 gene expression and/or higher SCD activity in subcutaneous adipose tissue is associated with higher concentrations of MUFA (Yang et al., 1999; Archibeque et al., 2005; Chung et al., 2007; Duckett et al., 2009; Brooks et al., 2011). Yang et al. (1999) measured SCD activity in subcutaneous adipose tissue from Australian pasture-finished and feedlot cattle, in which feedlot cattle were fed sorghum-based finishing diets in the absence and presence of rumen-protected cottonseed oil (CSO). Subcutaneous adipose tissue from pasture-finished cattle had higher SCD activity than adipose tissue from grain-finished cattle, and protected CSO strongly depressed SCD activity relative to grain-finished cattle that were not fed CSO. The results of Smith et al. (1998) (which also included Australian grain-finished cattle) and Yang et al. (1999) were quite the opposite of the effects of grain finishing of cattle in the US. Research from the US has consistently demonstrated that MUFA increased over time in adipose tissue from grain-finished steers (Westerling and Hedrick, 1979; Huerta-Leidenz et al., 1996; Chung et al. 2006). SCD1 gene expression is not upregulated until cattle are fed a grain-based diet (Brooks et al., 2011) and SCD1 gene expression increases in LM and most adipose tissue depots the longer the steers are fed a corn-based finishing diet (Martin et al., 1999; Chung et al., 2007; Smith et al., 2012; Li et al., 2018).
Genetic regulation of fatty acid composition in beef cattle
Adipose tissue and muscle from Japanese Black, Hanwoo, and Yanbian Yellow cattle (raised in Japan, Korea, and China, respectively) contain an unusually high concentration of oleic acid (Sturdivant et al., 1992; Zembayashi et al., 1995; Smith et al., 2001; Smith et al., 2009; Maharani et al., 2012; Li et al., 2018). Comparisons of beef from Black Wagyu, Akaushi (Red Wagyu), Angus, and commercial beef raised in the US have confirmed that beef from Wagyu and Akaushi cattle fed a grain-based diet contains a higher concentration of MUFA than beef from domestic cattle (May et al., 1993; Chung et al., 2006; Adams et al., 2010; Gilmore et al., 2013; Choi et al., 2018).
Lunt et al. (1993) were the first to compare American Wagyu and Angus steers under the same management conditions. The Wagyu’s subcutaneous adipose tissue contained more MUFA and less SFA (MUFA : SFA ratio = 1.50) than adipose tissue from Angus steers (MUFA : SFA ratio = 1.17) (May et al., 1993). Subsequently, we documented USDA quality grade, fatty acid composition, SCD activity, and SCD1 gene expression in Angus and Wagyu slaughtered at a targeted final body weight of 525 kg and 650 kg (Chung et al., 2006; Chung et al., 2007). The MUFA : SFA ratio increased over time in Wagyu and Angus subcutaneous adipose tissues and was higher in adipose tissues from corn-fed steers than in hay/corn-fed steers (Chung et al., 2006) (Figure 1A). The MUFA : SFA ratio was higher in adipose tissue from Wagyu steers and the slip point (a measure of melting point) was lower in subcutaneous adipose tissue lipids from cattle raised to the Japanese endpoint than in cattle raised to the US endpoint (Chung et al., 2006) (Figure 1B). These findings illustrate the importance of the duration of feeding a finishing diet for adipose MUFA and lipid slip point. SCD activity increased over time in subcutaneous adipose tissue from corn-fed Angus and Wagyu steers and in hay/corn-fed Wagyu steers (Figure 1C) (Chung et al., 2007), and SCD1 expression increased over time in adipose tissue from Wagyu steers (but not Angus steers) fed either the corn or the hay/corn diet (Figure 1D).
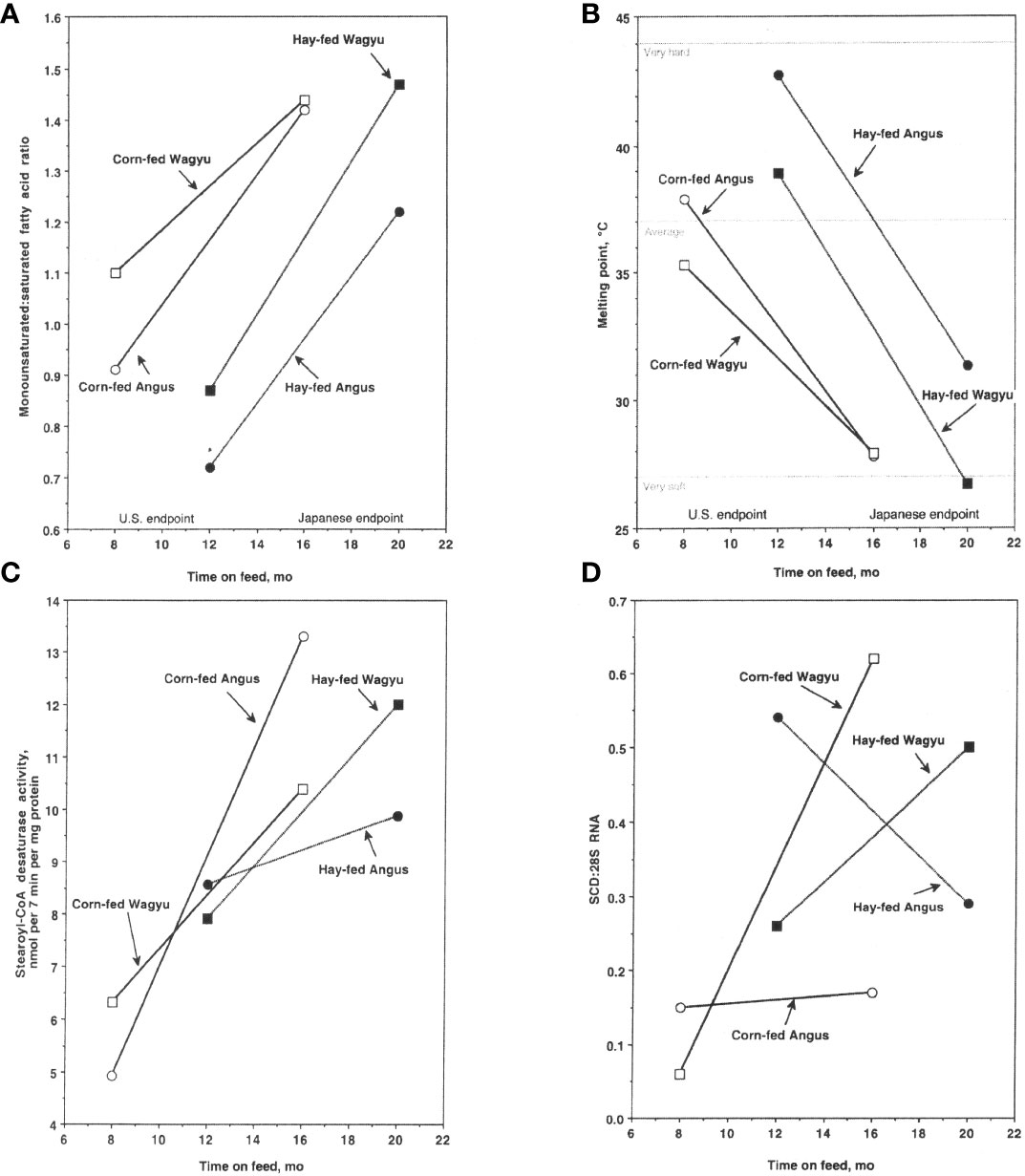
Figure 1 Monounsaturated fatty acid (MUFA):saturated fatty acid (SFA) ratio (A), slip point (B), stearoyl-CoA desaturase activity (C), and SCD1 gene expression (D) in subcutaneous adipose tissues of Angus steers fed a corn-based diet; Angus steers fed a hay/corn diet; Wagyu steers fed a corn-based diet; and Wagyu steers fed a hay/corn diet (n = 4 at all sampling times). All steers were weaned at 8 months of age. The US endpoint steers weighed 525 kg on average at sampling and the Japanese endpoint steers weighed 620 kg on average at sampling. The corn-based diet (Corn-fed) was designed to yield an average daily gain of 1.36 kg/d and the hay-based diet (Hay-fed) was supplemented with the same corn-based diet to yield an average daily gain of 0.9 kg/d. Marbling score was based on USDA marbling standards, and slip point is an estimate of the subcutaneous adipose tissue melting point. Stearoyl-CoA desaturase activity is expressed in nanomoles (nmol) stearic acid converted to oleic acid/(7 min•mg protein) and SCD1 gene expression is the SCD:28S ratio; both were measured in subcutaneous adipose tissues overlying the longissimus muscle. Data were derived from Chung et al. (2006), and Chung et al. (2007).
Taniguchi et al. (2004) demonstrated single-nucleotide polymorphisms in the open-reading frame (exon 5) of an SCD1 cDNA generated from Japanese Black cattle, in which valine was replaced with alanine. The VA and AA genotypes contributed to higher MUFA and lower melting points in intramuscular adipose tissue than the more infrequent VV genotype (Hoashi et al., 2007). Matsuhashi et al. (2011), Narukami et al. (2011), and Yokota et al. (2012) subsequently confirmed that these SCD1 polymorphisms contributed to variations in fatty acid composition across populations of Japanese Black cattle. In contrast, Maharani et al. (2012) reported that SCD1 TT, CC, and CT genotypes in exon 5 had no effect on oleic acid or total MUFA in beef from Korean Hanwoo cattle.
Taylor et al. (1998) first reported quantitative trait loci for stearic and oleic acids flanking the region in BTA19 between 28 cM and 51 cM. This region includes growth hormone (GH; 48.8 cM) but Taylor et al. (1998) were unable to identify the other genes in BTA19 responsible for the differences in stearic and oleic acids. Kawaguchi et al. (2021) reported fatty acid synthase (FASN; 51.4 cM) and sterol regulatory element-binding protein 1 (SREBP1; 35.2 cM) are located on BTA19. FASN regulates myristic acid and palmitic acid synthesis and SREPB1 is a transcription factor that regulates expression of FASN and SCD1 (located on BTA26; Campbell et al., 2001). Hoashi et al. (2007) and Matsuhashi et al. (2011) reported that the genotypes of SREPB1 and SCD1 are associated with the concentration of MUFA in beef from Japanese Black cattle, and Matsuhashi et al. (2011) reported that genotypes of FASN and GH are also associated with the concentration of MUFA.
Summary
Oleic-acid-enriched beef may reduce the risk for CVD but the effect of oleic acid on sensory attributes of beef has been difficult to demonstrate. Oleic acid increases in beef if cattle are raised to heavier weights and fed corn-based diets. Stearic acid in adipose tissue samples predicts the lipid melting point and may be negatively associated with beef sensory attributes. Although considerable research has focused on SCD1 gene expression, genetic polymorphisms in GH, FASN, and SREBP1 also contribute significantly to the fatty acid composition of beef.
Author contributions
SS: Conceptualization, Data curation, Formal analysis, Funding acquisition, Investigation, Methodology, Project administration, Resources, Software, Supervision, Validation, Visualization, Writing – original draft, and Writing – review & editing.
Funding
The author(s) declare that no financial support was received for the research, authorship, and/or publication of this article.
Conflict of interest
The author declares that the research was conducted in the absence of any commercial or financial relationships that could be construed as a potential conflict of interest.
Publisher’s note
All claims expressed in this article are solely those of the authors and do not necessarily represent those of their affiliated organizations, or those of the publisher, the editors and the reviewers. Any product that may be evaluated in this article, or claim that may be made by its manufacturer, is not guaranteed or endorsed by the publisher.
References
Adams T. H., Walzem R. L., Smith D. R., Tseng S., Smith S. B. (2010). Hamburger high in total, saturated and trans-fatty acids decreases HDL cholesterol and LDL particle diameter, and increases plasma TAG, in mildly hypercholesterolaemic men. Br. J. Nutr. 103, 91–98. doi: 10.1017/S0007114509991516
Archibeque S. L., Lunt D. K., Tume R. K., Smith S. B. (2005). Fatty acid indices of stearoyl Co-A desaturase activity do not reflect actual stearoyl Co-A desaturase enzyme activity in adipose tissues of beef steers finished with corn-, flaxseed-, or sorghum-based diets. J. Anim. Sci. 83, 1153–1166. doi: 10.2527/2005.8351153x
Blackmon T., Miller R. K., Kerth C., Smith S. B. (2015). Ground beef patties prepared from brisket, flank and plate have unique fatty acid and sensory characteristics. Meat Sci. 103, 46–53. doi: 10.1016/j.meatsci.2015.01.004
Brooks M. A., Choi C. W., Lunt D. K., Kawachi H., Smith S. B. (2011). Subcutaneous and intramuscular adipose tissue stearoyl-coenzyme A desaturase gene expression and fatty acid composition in calf- and yearling-fed Angus steers. J. Anim. Sci. 89, 2556–2570. doi: 10.2527/jas.2010-3369
Campbell E. M. G., Gallagher D. S., Davis S. K., Taylor J. F., Smith S. B. (2001). Rapid communication: Mapping of the bovine stearoyl-coenzyme A desaturase (SCD) gene to BTA26. J. Anim. Sci. 79, 1954–1955. doi: 10.2527/2001.7971954x
Chang J. H. P., Lunt D. K., Smith S. B. (1992). Fatty acid composition and fatty acid elongase and stearoyl-CoA desaturase activities in tissues of steers fed high oleate sunflower seed. J. Nutr. 122, 2074–2080. doi: 10.1093/jn/122.11.2074
Chen D., Wang X., Guo Q., Deng H., Luo J., Yi K., et al. (2022). Muscle fatty acids, meat flavor compounds and sensory characteristics of Xiangxi Yellow cattle in comparison to Aberdeen Angus. Anim. (Basel) 12, 1161. doi: 10.3390/ani12091161
Choi S. H., Gharahmany G., Walzem R. L., Meade T. H., Smith S. B. (2018). Ground beef high in total fat and saturated fatty acids decreases x receptor signaling targets in peripheral blood mononuclear cells of men and women. Lipids 53, 279–290. doi: 10.1002/lipd.12028
Chung K. Y., Lunt D. K., Choi C. B., Chae S. H., Rhoades R. D., Adams T. L., et al. (2006). Lipid characteristics of subcutaneous adipose tissue and M. longissimus thoracis of Angus and Wagyu steers fed to U.S. and Japanese endpoints. Meat Sci. 73, 432–441. doi: 10.1016/j.meatsci.2006.01.002
Chung K. Y., Lunt D. K., Kawachi H., Yano H. (2007). Lipogenesis and stearoyl-CoA desaturase gene expression and enzyme activity in adipose tissue of short- and long-fed Angus and Wagyu steers fed corn- or hay-based diets. J. Anim. Sci. 85, 380–387. doi: 10.2527/jas.2006-498
Dryden F. D., Marchello J. A. (1970). Influence of total lipid and fatty acid composition upon the palatability of three bovine muscles. J. Anim. Sci. 31, 36–41. doi: 10.2527/jas1970.31136x
Duckett S. K., Pratt S. L., Pavan E. (2009). Corn oil or corn grain supplementation to steers grazing endophyte-free tall fescue. II. Effects on subcutaneous fatty acid content and lipogenic gene expression. J. Anim. Sci. 87, 1120–1128. doi: 10.2527/jas.2008-1420
Ekeren P. A., Smith D. R., Lunt D. K., Smith D. R. (1992). Ruminal biohydrogenation of fatty acids from high-oleate sunflower seeds. J. Anim. Sci. 70, 2574–2580. doi: 10.2527/1992.7082574x
Frank D., Ball A., Hughes J., Krishnamurthy R., Piyasiri U., Stark J., et al. (2016). Sensory and flavor chemistry characteristics of Australian beef: Influence of intramuscular fat, feed, and breed. J. Agric. Food Chem. 64, 4299–4311. doi: 10.1021/acs.jafc.6b00160
Gilbert C. D., Lunt D. K., Miller R. K., Smith S. B. (2003). Carcass, sensory, and adipose tissue traits of Brangus steers fed casein-formaldehyde-protected starch and/or canola lipid. J. Anim. Sci. 81, 2457–2468. doi: 10.2527/2003.81102457x
Gilmore L. A., Crouse S. F., Carbuhn A., Klooster J., Calles J. A. E., Meade T., et al. (2013). Exercise attenuates the increase in plasma monounsaturated fatty acids and high-density lipoprotein but not high-density lipoprotein 2b cholesterol caused by high-oleic ground beef in women. Nutr. Res. 33, 1003–1011. doi: 10.1016/j.nutres.2013.09.003
Gilmore L. A., Walzem R. L., Crouse S. F., Smith D. R., Adams T. H., Vaidyananthan V., et al. (2011). Consumption of high-oleic acid ground beef increases HDL cholesterol concentration but both high- and low-oleic acid ground beef decrease HDL particle diameter in normocholesterolemic men. J. Nutr. 141, 1188–1194. doi: 10.3945/jn.110.136085
Gordon D. J., Probstfield J. L., Garrison R. J., Neaton J. D., Castelli W. P., Knoke J. D., et al. (1989). High-density lipoprotein cholesterol and cardiovascular disease: Four prospective American studies. Circulation 79, 8–15. doi: 10.1161/01.cir.79.1.8
Hoashi S., Ashida N., Ohsaki H., Utsugi T., Sasazaki S., Taniguchi M., et al. (2007). Genotype of bovine sterol regulatory element binding protein-1 (SREBP-1) is associated with fatty acid composition in Japanese Black cattle. Mamm Genome 18, 880–886. doi: 10.1007/s00335-007-9072-y
Huerta-Leidenz N. O., Cross H. R., Savell J. W., Belk K. E., Lunt D. K., Baker J. F., et al. (1996). Fatty acid composition of adipose tissues of male calves at different stages of growth. J. Anim. Sci. 74, 1256–1264. doi: 10.2527/1996.7461256x
Kawaguchi F., Kakiuchi F., Oyama K., Mannen H., Sasazaki S. (2021). Effect of five polymorphisms on percentage of oleic acid in beef and investigation of linkage disequilibrium to confirm the locations of quantitative trait loci on BTA19 in Japanese Black cattle. Life (Basel). 11, 597. doi: 10.3390/life11070597
Kerth C. R., Harbison A. L., Smith S. B., Miller R. K. (2015). Consumer sensory evaluation, fatty acid composition, and shelf-life of ground beef with subcutaneous fat trimmings from different carcass locations. Meat Sci. 104, 30–36. doi: 10.1016/j.meatsci.2015.01.014
Legako J. F., Dinh T. T., Miller M. F., Brooks J. C. (2015). Effects of USDA beef quality grade and cooking on fatty acid composition of neutral and polar lipid fractions. Meat Sci. 100, 246–255. doi: 10.1016/j.meatsci.2014.10.013
Li X. Z., Yan C. G., Gao Q. S., Yan Y., Choi S. H., Smith S. B. (2018). Adipogenic/lipogenic gene expression and fatty acid composition in chuck, loin, and round muscles in response to grain feeding of Yanbian Yellow cattle. J. Anim. Sci. 96, 2698–2709. doi: 10.1093/jas/sky161
Lunt D. K., Riley R. R., Smith S. B. (1993). Growth and carcass characteristics of Angus and American Wagyu steers. Meat Sci. 34, 327–334. doi: 10.1016/0309-1740(93)90081-R
Lytle J. R., Price T., Crouse S. F., Smith D. R., Walzem R. L., Smith S. B. (2023). Consuming high-fat and low-fat ground beef depresses high-density and low-density lipoprotein cholesterol concentrations, and reduces small, dense low-density lipoprotein particle abundance. Nutrients 15, 337. doi: 10.3390/nu15020337
Maharani D., Jung Y., Jung W. Y., Jo C., Ryoo S. H., Lee S. H., et al. (2012). Association of five candidate genes with fatty acid composition in Korean cattle. Mol. Biol. Rep. 39, 6113–6121. doi: 10.1007/s11033-011-1426-6
Mandell I. B., Buchanan-Smith J. G., Campbell C. P. (1998). Effects of forage vs grain feeding on carcass characteristics, fatty acid composition, and beef quality in Limousin-cross steers when time on feed is controlled. J. Anim. Sci. 76, 2619–2630. doi: 10.2527/1998.76102619x
Martin G. S., Lunt D. K., Britain K. G., Smith S. B. (1999). Postnatal development of stearoyl coenzyme A desaturase gene expression and adiposity in bovine subcutaneous adipose tissue. J. Anim. Sci. 77, 630–636. doi: 10.2527/1999.773630x
Matsuhashi T., Maruyama S., Uemoto Y., Kobayashi N., Mannen H., Abe T., et al. (2011). Effects of bovine fatty acid synthase, stearoyl-coenzyme A desaturase, sterol regulatory element-binding protein 1, and growth hormone gene polymorphisms on fatty acid composition in Japanese Black Cattle. J. Anim. Sci. 89, 12–22. doi: 10.2527/jas.2010-3121
May S. G., Sturdivant C. A., Lunt D. K., Miller R. K., Smith S. B. (1993). Comparison of sensory characteristics and fatty acid composition between Wagyu crossbred and Angus steers. Meat Sci. 35, 289–298. doi: 10.1016/0309-1740(93)90034-F
Melton S. L., Amiri M., Davis G. W., Backus W. R. (1982a). Flavor and chemical characteristics of ground beef from grass-, forage-grain and grain-finished steers. J. Anim. Sci. 55, 71–87. doi: 10.2527/jas1982.55177x
Melton S. L., Black J. M., Davis G. W., Backus W. R. (1982b). Flavor and selected chemical components of ground beef from steers backgrounded on pasture and fed corn up to 140 days. J. Food Sci. 47, 699–704. doi: 10.1111/j.1365-2621.1982.tb12694.x
Narukami T., Sasazaki S., Oyama K., Nogi T., Taniguchi M., Mannen H. (2011). Effect of DNA polymorphisms related to fatty acid composition in adipose tissue of Holstein cattle. Anim. Sci. J. 82, 406–411. doi: 10.1111/j.1740-0929.2010.00855.x
Page A. M., Sturdivant C. A., Lunt D. K., Smith S. B. (1997). Dietary whole cottonseed depresses lipogenesis but has no effect on stearoyl coenzyme desaturase activity in bovine subcutaneous adipose tissue. Comp. Biochem. Physiol. B Biochem. Mol. Biol. 118, 79–84. doi: 10.1016/s0305-0491(97)00027-8
Roussell M. A., Hill A. M., Gaugler T. L., West S. G., Heuvel J. P., Alaupovic P. (2012). Beef in an Optimal Lean Diet study: effects on lipids, lipoproteins, and apolipoproteins. Am. J. Clin. Nutr. 95: 9–16. doi: 10.3945/ajcn.111.016261
Smith S. B. (1983). Contribution of the pentose cycle to lipogenesis in bovine adipose tissue. Arch. Biochem. Biophys. 221, 46–56. doi: 10.1016/0003-9861(83)90120-0
Smith S. B., Crouse J. D. (1984). Relative contributions of acetate, lactate and glucose to lipogenesis in bovine intramuscular and subcutaneous adipose tissue. J. Nutr. 114, 792–800. doi: 10.1093/jn/114.4.792
Smith S. B., Go G. W., Johnson B. J., Chung K. Y., Choi S. H., Sawyer J. E., et al. (2012). Adipogenic gene expression and fatty acid composition in subcutaneous adipose tissue depots of Angus steers between 9 and 16 months of age. J. Anim. Sci. 90, 2505–2514. doi: 10.2527/jas.2011-4602
Smith S. B., Kawachi H., Choi C. B., C. Choi C. W., Wu G., Sawyer J. E. (2009). Cellular regulation of bovine intramuscular adipose tissue development and composition. J. Anim. Sci. 87 (E Suppl.), E72–E82. doi: 10.2527/jas.2008-1340
Smith S. B., Lunt D. K., Smith D. R., Walzem R. L. (2020). Producing high-oleic acid beef and the impact of ground beef consumption on risk factors for cardiovascular disease: A review. Meat Sci. 163, 108076. doi: 10.1016/j.meatsci.2020.108076
Smith S. B., Prior R. L. (1981). Evidence for a functional ATP-citrate lyase:NADP-malate dehydrogenase pathway in bovine adipose tissue: enzyme and metabolite levels. Arch. Biochem. Biophys. 211, 192–201. doi: 10.1016/0003-9861(81)90444-6
Smith S. B., Yang A., Larsen T. W., Tume R. K. (1998). Positional analysis of triacylglycerols from bovine adipose tissue lipids varying in degree of unsaturation. Lipids 33, 197–207. doi: 10.1007/s11745-998-0196-8
Smith S. B., Zembayashi M., Lunt D. K., Sanders J. O., Gilbert C. D. (2001). Carcass traits and microsatellite distributions in offspring of sires from three graphical regions of Japan. J. Anim. Sci. 79, 3041–3051. doi: 10.2527/2001.79123041x
St. John L. C., Lunt D. K., Smith S. B. (1991). Fatty acid elongase and desaturase activities in bovine liver and adipose tissue microsomes. J. Anim. Sci. 69, 1064–1073. doi: 10.2527/1991.6931064x
St. John L. C., Young C. R., Knabe D. A., Schelling G. T., Grundy S. M., Smith S. B. (1987). Fatty acid profiles and sensory and carcass traits of tissues from steers and swine fed an elevated monounsaturated fat diet. J. Anim. Sci. 64, 1441–1447. doi: 10.2527/jas1987.6451441x
Sturdivant C. A., Lunt D. K., Smith S. B. (1992). Fatty acid composition of longissimus muscle and subcutaneous and intramuscular adipose tissues of Japanese Wagyu cattle. Meat Sci. 32, 449–458. doi: 10.1016/0309-1740(92)90086-J
Taniguchi M., Utsugi T., Oyama K., Mannen H., Kobayashi M., Tanabe Y., et al. (2004). Genotype of stearoyl-coA desaturase is associated with fatty acid composition in Japanese Black cattle. Mamm Genome 15, 142–148. doi: 10.1007/s00335-003-2286-8
Taylor J. F., Coutinho L. L., Herring K. L., Gallagher D. S. Jr, Brenneman R. A., Burney N., et al. (1998). Candidate gene analysis of GH1 for effects on growth and carcass composition of cattle. Anim. Genet. 29, 194–201. doi: 10.1046/j.1365-2052.1998.00317.x
Turk S. N., Smith S. B. (2009). Carcass fatty acid mapping. Meat Sci. 81, 658–663. doi: 10.1016/j.meatsci.2008.11.005
Waldman R. C., Suess G. G., Brungardt J. M. (1968). Fatty acids of certain bovine tissues and their association with growth, carcass and palatability traits. J. Anim. Sci. 48, 1368–1379. doi: 10.2527/jas1968.273632x
Westerling D. B., Hedrick H. B. (1979). Fatty acid composition of bovine lipids as influenced by diet, sex and anatomical location and relationship to sensory characteristics. J. Anim. Sci. 48, 1343–1348. doi: 10.2527/jas1979.4861343x
Whitehurst G. B., Beitz D. C., Pothoven M. A., Ellison W. R., Crump M. H. (1978). Lactate as a precursor of fatty acids in bovine adipose tissue. J. Nutr. 108, 1806–1811. doi: 10.1093/jn/108.11.1806
Wu X., Roussell M. A., Hill A. M., Kris-Etherton P. M., Walzem R. L. (2021). Baseline insulin resistance is a determinant of the small, dense low-density lipoprotein response to diets differing in saturated fat, protein, and carbohydrate contents. Nutrients 13, 4328. doi: 10.3390/nu13124328
Yang A., Larsen T. W., Smith S. B., Tume R. K. (1999). Δ9 desaturase activity in bovine subcutaneous adipose tissue of different fatty acid composition. Lipids 34, 971–978. doi: 10.1007/s11745-999-0447-8
Yokota S., Sugita H., Ardiyanti A., Shoji N., Nakajima H., Hosono M., et al. (2012). Contributions of FASN and SCD gene polymorphisms on fatty acid composition in muscle from Japanese Black cattle. Anim. Genet. 43, 790–792. doi: 10.1111/j.1365-2052.2012.02331.x
Keywords: oleic acid, SCD, HDL-C, cardiovascular risk, genetic regulation of SCD
Citation: Smith SB (2024) Oleic acid concentration in bovine adipose tissues: impact on human health, sensory attributes, and genetic regulation. Front. Anim. Sci. 5:1332861. doi: 10.3389/fanim.2024.1332861
Received: 03 November 2023; Accepted: 03 January 2024;
Published: 29 January 2024.
Edited by:
Jeff Wood, University of Bristol, United KingdomReviewed by:
Stefaan De Smet, Ghent University, BelgiumJerrad Legako, Texas Tech University, United States
Copyright © 2024 Smith. This is an open-access article distributed under the terms of the Creative Commons Attribution License (CC BY). The use, distribution or reproduction in other forums is permitted, provided the original author(s) and the copyright owner(s) are credited and that the original publication in this journal is cited, in accordance with accepted academic practice. No use, distribution or reproduction is permitted which does not comply with these terms.
*Correspondence: Stephen B. Smith, c2JzbWl0aEB0YW11LmVkdQ==