- 1School of Agriculture, Food and Ecosystems Sciences, The University of Melbourne, Parkville, VIC, Australia
- 2Department of Jobs, Precincts and Regions, Agrifeed Animal Production, Mill Park, VIC, Australia
- 3School of Agriculture and Food Science, University College Dublin, Dublin, Ireland
- 4Department of Animal Science, Lorestan University, Khorramabad, Iran
- 5Department of Biochemistry, Memorial University of Newfoundland, St. John’s, NL, Canada
- 6Department of Animal Science, University of California, Davis, Davis, CA, United States
- 7INRAE, Université Clermont Auvergne Vetagro Sup, Unité Mixte de Recherche sur les Herbivores, St-Genès-Champanelle, France
- 8Department of Animal Sciences, Faculty of AgriSciences, Stellenbosch University, Matieland, South Africa
Optimising resource use efficiency in animal- agriculture-production systems is important for the economic, environmental, and social sustainability of food systems. Production of foods with increased health enhancing aspects can add value to the health and wellbeing of the population. However, enrichment of foods, especially meat with health enhancing fatty acids (HEFA) increases susceptibility to peroxidation, which adversely influences its shelf life, nutritional value and eating quality. The meat industry has been challenged to find sustainable strategies that enhance the fatty acid profile and antioxidant actions of meat while mitigating oxidative deterioration and spoilage. Currently, by-products or co-products from agricultural industries containing a balance of HEFA and antioxidant sources seem to be a sustainable strategy to overcome this challenge. However, HEFA and antioxidant enrichment processes are influenced by ruminal lipolysis and biohydrogenation, HEFA-antioxidant interactions in rumen ecosystems and muscle biofortification. A deep understanding of the performance of different agro-by-product-based HEFA and antioxidants and their application in current animal production systems is critical in developing HEFA-antioxidant co-supplementation strategies that would benefit modern consumers who desire nutritious, palatable, safe, healthy, affordable, and welfare friendly meat and processed meat products. The current review presents the latest developments regarding discovery and application of novel sources of health beneficial agro-by-product-based HEFA and antioxidants currently used in the production of HEFA-antioxidant enriched ruminant meats and highlights future research perspectives.
1 Introduction
There are many breeds and genotypes within the ruminant animal species of sheep, goats and cattle around the world, each of them is adapted to the different environments they live in and the diets they consume. Ruminant animals obtain their nutrients from fresh forage materials of native rangelands or cultivated pastures, crop residues, cereal grains, preserved forages and agri-food by-products (Ponnampalam et al., 2022), and nutrition and feeding strategies play a major role in the wellness, productivity, and survival of animals. Numerous studies have investigated the effect of feeding systems and feed composition on the fat content, fatty acid (FA) composition and antioxidant status of meat in ruminants (Scollan et al., 2006; Chilliard et al., 2007; Noci et al., 2011; Chauhan et al., 2014; Berthelot and Gruffat, 2018; Vahmani et al., 2020). Dietary proteins, lipids and carbohydrates are vital for ruminant growth and development. Equally, vitamins, minerals and health enhancing fatty acids (HEFA) are crucial for animal health, well-being and product quality (Kaur et al., 2014; Ponnampalam et al., 2021a; Guo et al., 2023; Kearns et al., 2023b). The HEFA include the parent (precursors), their products (derivatives) as well as their biohydrogenation intermediates (BHI). These in turn include alpha-linolenic acid (ALA, C18:3n-3), eicosapentaenoic acid (EPA, C20:5n-3), docosapentaenoic acid (DPA, C22:5n-3), docosahexaenoic acid (DHA, C22:6n-3), linoleic acid (LA, C18:2n-6), rumenic acid (RA, cis(c)9, trans(t)11-18:2), and trans vaccenic acid (TVA, cis(c)9, trans(t)11-18:1). The economic return of animal production systems is largely dependent on the yield, quality, nutritional value and storage stability of the meat produced by the flock or herd.
Red meat, obtained from lamb/mutton, beef, and chevon, is rich in vital nutrients, including a high amount of highly digestible proteins, containing all essential amino acids to meet human requirements, B vitamins (mainly vitamin B12), zinc, iron, and selenium (Klurfeld, 2018). Red meat is one of the most important animal source foods in many countries all over the world. However, epidemiological data from meta-analyses shows that excessive consumption of red meat and processed meat can have deleterious effects, including an increased risk of colorectal cancer and premature death (Schwingshackl et al., 2017; WCRF et al., 2018), leading to recommendations to limit consumption to 500 g/week for red meat and 150 g/week for processed meat (Prache et al., 2022a). North America, Europe and Oceania regions consume large quantities of red and white meat, African regions consume the least amount, and the Asia and Latin America regions consume moderate amounts. The per capita consumption per year of beef-veal, sheep meat (lamb and mutton), poultry meat and pork worldwide, and within major regions of the world is shown in Figure 1. As goat meat intake is very small in quantity around the world and mostly associated with some ethnic groups within countries, it was not shown. The per capita consumption of red meat around the world has increased from 10 to 20 kg of meat between 1961 and 2020 (Ritchie et al., 2019).
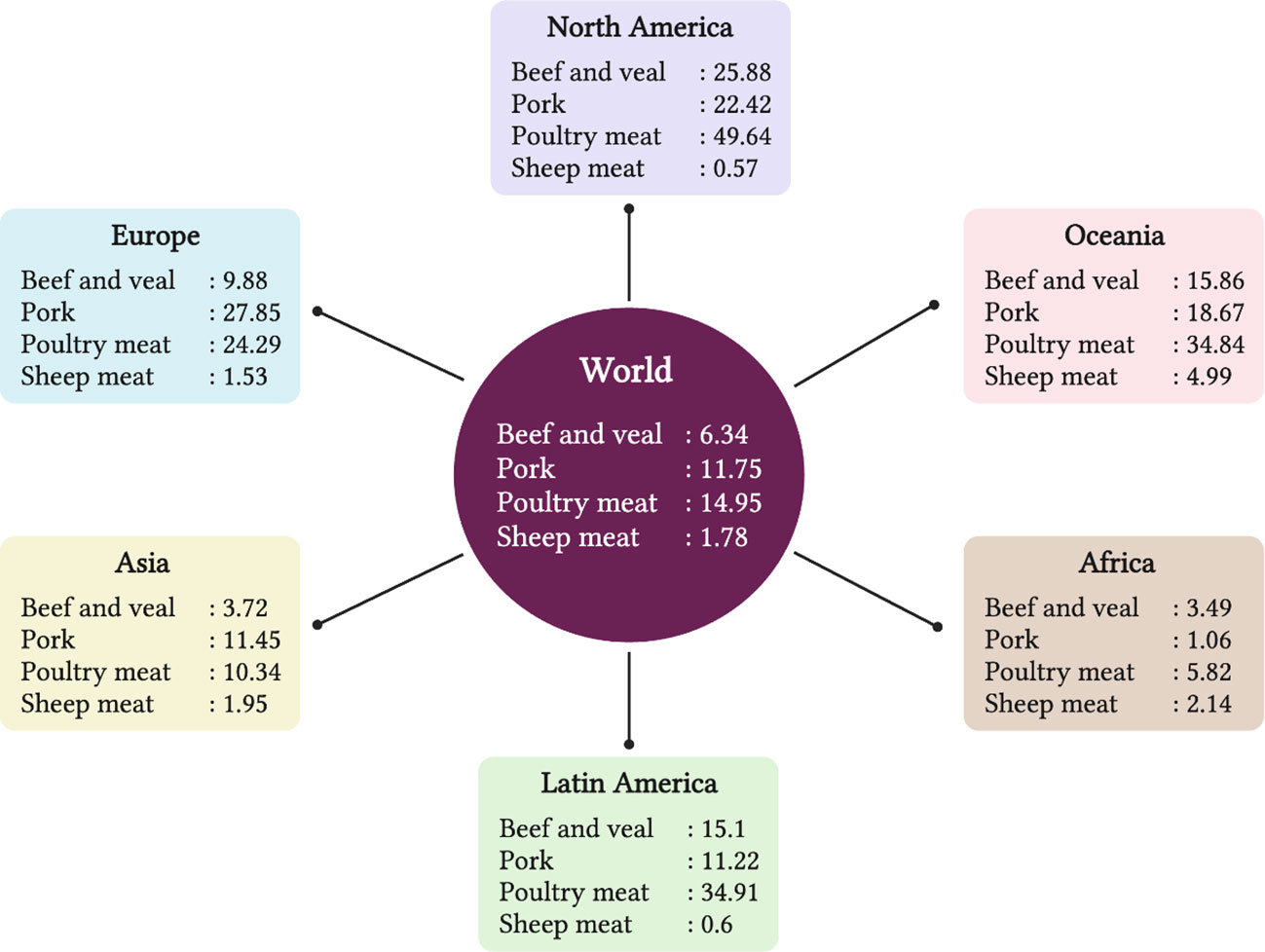
Figure 1 The per capita consumption (kg / year) of beef-veal, sheep meat (lamb and mutton), poultry and pork of world and major regions of world. Source: OECD/FAO (2021), OECD Agriculture statistics (database), http://dx.doi.org/10.1787/agr-outl-dataen.
The mean consumption of unprocessed and processed red meat per person across countries varies due to affordability, availability, and preference. The low-fat content (of particular cuts) relevant to all meats has great importance due to the links found between meat FA consumption and prevalence of chronic disease (Bernstein et al., 2010; Abid et al., 2014). Table 1 below provides some information on consumption of beef-veal, sheep meat (lamb-mutton), poultry meat and pork from developed and developing countries around the world, expressed in retail weight.
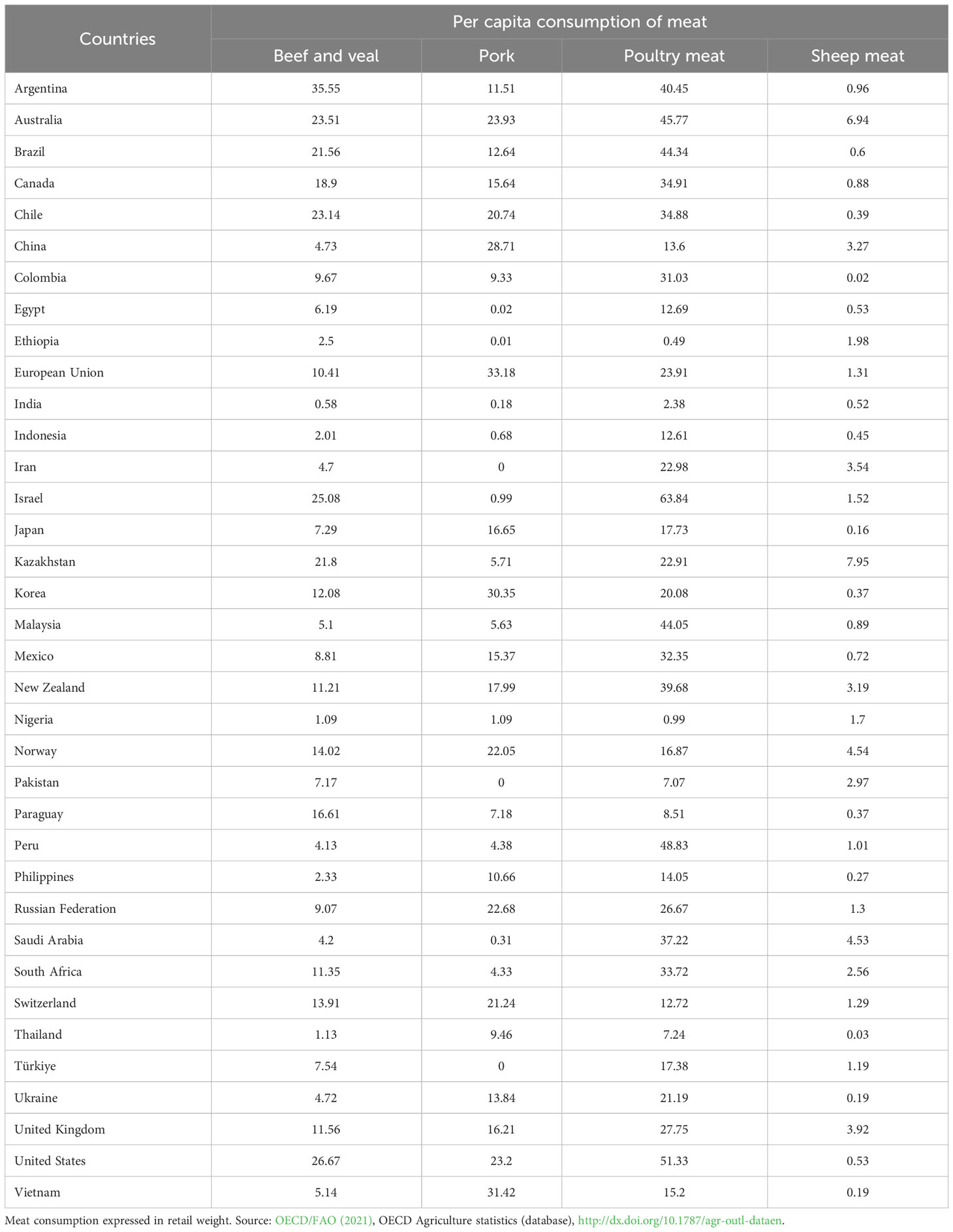
Table 1 Per capita consumption (kg / year) of beef and veal, pork, poultry meat and sheep meat (lamb-mutton) from developed and developing countries around the world.
Ruminant meat is a good source of HEFA. Linoleic acid and ALA are parent (precursor) fatty acids, which cannot be synthesised by the animal and human body and must be obtained from the diet (Kaur et al., 2014; Ponnampalam, 1999). These two FA are vital for the synthesis of their longer chain polyunsaturated fatty acid (PUFA) derivatives and their bioactive intermediates in the rumen and fortification in animal tissues (Ponnampalam et al., 2021b). In addition, ruminant meats contain nutrient antioxidants such as vitamins A, C and E, and the minerals copper, zinc and selenium (Bourre, 2011; Cabrera and Saadoun, 2014), which scavenge the free radicals and reactive oxygen species (ROS) in the cell, thereby mitigating oxidative stress and its consequences in the body (Zehiroglu and Ozturk Sarikaya, 2019). Both HEFA and antioxidants play a crucial role in the maintenance and/or enhancement of animal welfare, meat shelf life and sensory attributes and health of human beings (Scollan et al., 2006; Kurutas, 2015; Lauridsen, 2019; Ponnampalam et al., 2021a; Ponnampalam et al., 2022). Therefore, enriching ruminant meat, which is consumed by the majority of the world population, with HEFA and antioxidants could improve the nutritional value (i.e., health aspects), quality (i.e., sensory), and storage stability (i.e., shelf life) of meat and eventually benefit the health of human beings as illustrated in Figure 2. This review aims to provide an update on aspects of various diets and feeding strategies contributing to the enrichment of HEFA and antioxidants in meat from sheep, goats and cattle, enabling improvement in the nutritional value, quality and health aspects of red meat that in turn may enhance health and well-being of humans upon consumption.
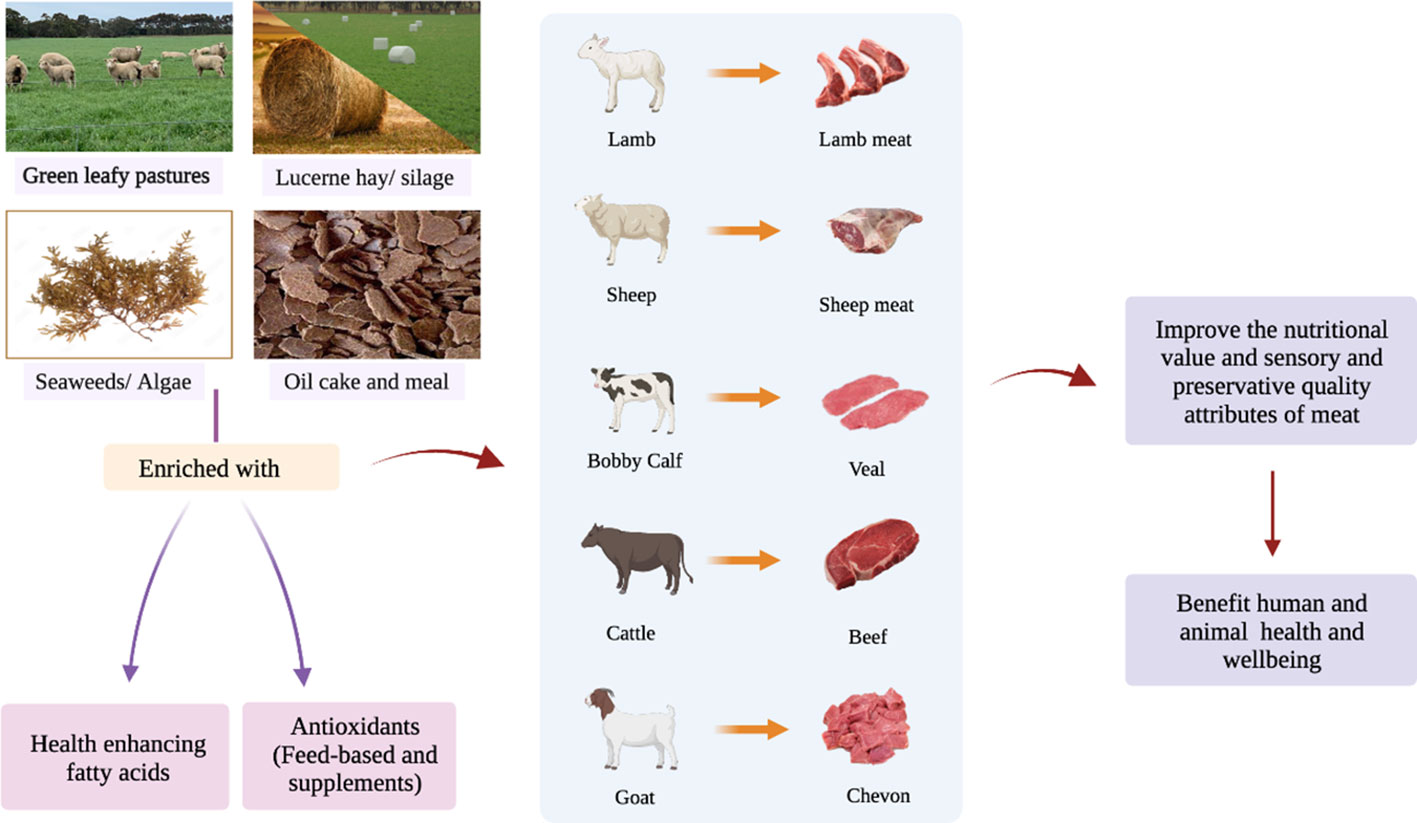
Figure 2 Schematic diagram showing pathways to enrich meat from ruminants with health enhancing FA and antioxidants delivering improved nutritional value and health aspects for consumers.
2 Feed-based enrichment of health enhancing fatty acid concentrations in red meat from sheep, goats and cattle
Meat from ruminant and monogastric animal species contributes important sources of nutrients from childhood to old age in many communities in developed and developing countries (FAO, 2011; Pereira and Vicente, 2013; FAO, 2017). Red meat from ruminants can offer balanced nutrient profiles and promote consumer health in many societies around the world (Pereira and Vicente, 2013), provided it is not consumed in excess (Prache et al., 2022a). Nutrient composition of meat is influenced by the type of diet that the animals consume. For example, feeding grain-based diets to ruminants increases intramuscular fat (i.e., marbling), omega(n)-6 FA concentrations and the n-6:n-3 ratio in meat (Aurousseau et al., 2004; Ponnampalam et al., 2017; Chikwanha et al., 2018, 2007; Berthelot and Gruffat, 2018; Gruffat et al., 2020; Clinquart et al., 2022; Davis et al., 2022). The latter effects are mainly due to variation in diet composition and nutrient availability of feeds affecting the ruminal biohydrogenation process, digestion and absorption of nutrients in the gastro-intestinal tract (GIT) of the host animal and subsequent metabolism in the body, determining the availability of energy and nutrients for the synthesis of biochemical components in meat.
There are many reasons for the variation in HEFA concentrations in meat, mainly determined by the ruminal lipolysis and biohydrogenation process of microbial activity. Lipids/fats in the diet can be categorised as protected or unprotected, depending on their susceptibility to microbial degradation and biohydrogenation in the rumen. Dietary fats can be protected naturally (e.g., feeding whole oilseeds) or by chemical interventions (fat emulsification or encapsulation within proteins) to form calcium soaps or amides, which protect against rumen degradation. Protected fats are conditioned to limit their degradation in the rumen while unprotected fats are subjected to ruminal degradation, whereby PUFA are hydrogenated into SFA and free FAs as a result of ruminal lipolysis by microbes. Biohydrogenation is not comprehensive; a portion of unprotected fats may by-pass degradation in the rumen, thereby becoming available for absorption and deposition in muscle and other tissues.
Lipids are either consumed or synthesised de novo and it should be noted that the digestion and absorption of lipids (or fats) in ruminant and monogastric animals are not similar. This is due to their feeding nature and structure of digestive systems. Ruminant animals accustomed to consuming diets containing 1–4% fat, and lipid supplements fed to ruminants above 5–6% on a dry matter basis may cause negative effects on rumen microbial activity, affecting feed intake and animal productivity. With monogastric animals having a stomach as one organ for temporary storage of diet (fats) in the absence of rumen microbial activity, they can handle greater amounts of lipids in their diet and the fat deposition in meat resembles dietary lipid composition, while this is not the case for ruminants. More details on ruminal lipolysis, biohydrogenation and digestion process of dietary lipids can be found elsewhere (Swanson, 2019; Ponnampalam et al., 2021b).
2.1 Influence of diets on health enhancing fatty acids in lamb and mutton
Levels of HEFA in ruminant meat are largely dependent on the type of dietary fat consumed, the duration of feeding and ruminal biohydrogenation of PUFA. In this regard, a variety of nutritional strategies have been employed to increase HEFA deposition in sheep meat (i.e., lamb and mutton), which have been summarised in Table 2.
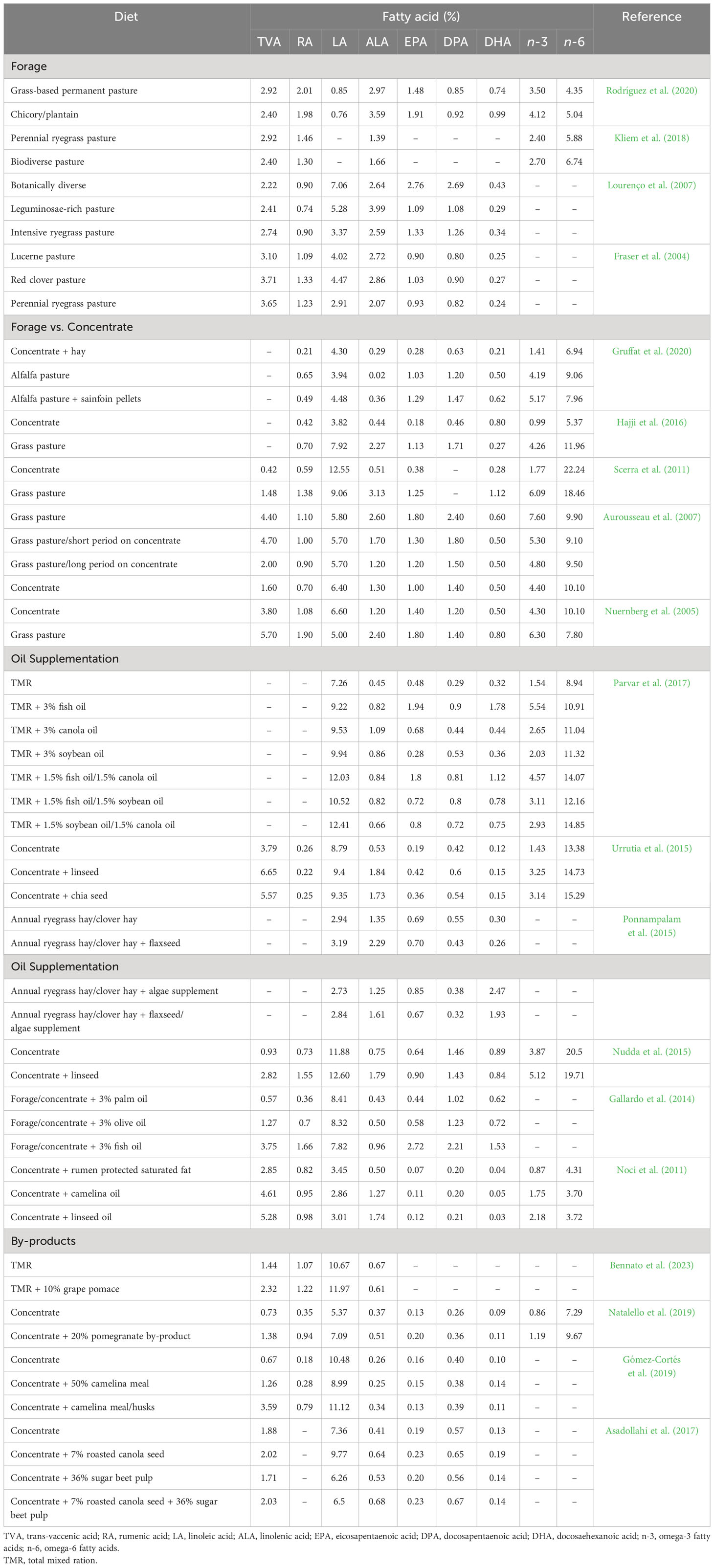
Table 2 Influence of diet on the health enhancing fatty acid composition (% of total fatty acids) of sheep meat.
Meat from pasture-fed animals contains higher n-3 PUFA contents compared to grain-fed animals (Aurousseau et al., 2004; Nuernberg et al., 2005; Hajji et al., 2016, 2007; Berthelot and Gruffat, 2018; Gruffat et al., 2020), due to the naturally high levels of ALA in fresh pasture, a precursor for endogenous production of EPA, DPA and DHA (Ponnampalam et al., 2021b). It also contains a lower proportion of C16:0, which is pro-atherogenic (Berthelot and Gruffat, 2018). Grain supplementation at pasture diminishes these advantages, especially as the quantity of concentrate consumed increases (Montossi et al., 2013; Berthelot and Gruffat, 2018). Prolonged finishing periods on grain post-grazing diminishes n-3 PUFA accumulated in the meat, with lower ALA, EPA and DHA and their BHI such as CLAs (e.g., RA) and TVA levels observed in lamb meat (Aurousseau et al., 2007; Scerra et al., 2011). Conversely, there is a lack of data on the effect of the length of time an animal is finished on pasture pre-slaughter on the HEFA concentration in sheep meat. Pasture type can affect the HEFA composition of meat. Grazing lambs on legumes like lucerne and red clover (Fraser et al., 2004) or white clover (Lourenço et al., 2007) increased ALA levels in meat compared to lambs grazing grasses like perennial ryegrass. Similarly, meat from lambs grazing chicory/arrow leaf clover had higher concentrations of n-3 PUFAs including ALA, EPA and DHA compared to lambs grazing brassica (De Brito et al., 2017). Lambs grazing botanically diverse pastures with phenol-rich plants produce meat richer in PUFA, ALA and LA than lambs grazing intensively managed pastures, with the concentration of these FA in the meat being linearly related to the phenolic concentration of the pasture (Willems et al., 2014). Plant secondary metabolites (polyphenol oxidase, flavonoids, tannins, essential oils and saponins) in legume and forb species can influence microbial biohydrogenation in the rumen, positively affecting beneficial n-3 PUFA and BHI outflow (Campidonico et al., 2016; Chikwanha et al., 2018; Frutos et al., 2020). Moreover, condensed tannins found in certain legumes can improve the lamb flavour by inhibiting the ruminal synthesis of skatole and indole, which are faecal-smelling compounds (Scollan et al., 2008; Girard et al., 2016; Rivaroli et al., 2019). Note here that sheep meat contains more HEFA than beef, due to lower ruminal biohydrogenation and a more selective eating behaviour of the animal (Prache et al., 2022b).
Generally, ruminants grazing fresh pasture consume higher amounts of n-3 PUFA compared to ruminants fed conserved forages (Ciftci et al., 2010) or total mixed ration (TMR) diets (Aurousseau et al., 2004, 2007; Berthelot and Gruffat, 2018; Gruffat et al., 2020). However, supplementation with n-3 PUFA rich oilseeds, marine- and plant-sourced oils and by-products have sometimes yielded more positive results. For example, addition of flaxseed to lamb diets resulted in increased contents of beneficial n-3 PUFA (i.e., ALA, EPA and DPA) in lamb and mutton (Noci et al., 2011; Ponnampalam et al., 2015; Urrutia et al., 2015; Berthelot and Gruffat, 2018). Diets supplemented with fish/algae oils increased long-chain n-3 PUFA levels such as EPA and DHA in lamb meat (Ponnampalam et al., 2016; Parvar et al., 2017), particularly when fed in an encapsulated form to protect against rumen biohydrogenation (Bessa et al., 2015). Supplementation of forage diets with n-6 rich oils (sunflower/soybean) has been shown to increase proportions of LA, RA and TVA, as well as other CLA isomers in lamb meat when compared to supplementation with n-3 PUFA rich oil sources (Chikwanha et al., 2018). Feeding lambs by-products such as grape/pomegranate pomace, soyabean hull and camelina meal, which are rich in antioxidants (polyphenols, flavonoids, anthocyanidins and tannins) led to enriched levels of ALA and total n-3 PUFA in meat (Gómez-Cortés et al., 2019; Natalello et al., 2019; Bennato et al., 2023). In summary, feeding pasture or high forage diets rich in n-3 PUFA leads to higher contents of health beneficial n-3 PUFA (ALA, EPA and DHA) and their BHI (RA and VA) in lamb meat. When high forage diets are fed along with n-6 PUFA rich sources, high concentrations of RA and VA are observed in meat, as n-6 rich dietary sources are more effective at increasing RA and VA levels compared to n-3 rich dietary sources (Bessa et al., 2015; Chikwanha et al., 2018). Total mixed rations and grain-based diets may require the addition of a n-3 PUFA and forage sources to improve HEFA levels in sheep meat.
Meat from suckling lambs is widely consumed in southern Europe. In this case, lambs are considered as monogastric, and their meat FA profile reflects the feed-milk profile. Feeding ewes on good quality pastures (Joy et al., 2012), particularly biodiverse pastures containing various legume species (Cabiddu et al., 2005) has been shown to improve the HEFA content of meat from their lambs. The inclusion of n-3 PUFA rich oilseeds (Gallardo et al., 2015; Nudda et al., 2015) or marine oils (Gallardo et al., 2014) in ewe diets has also been shown to improve the HEFA content of lamb meat, but the inclusion of grape by-products did not significantly alter the HEFA content of lamb meat (Correddu et al., 2023).
2.2 Influence of diets on health enhancing fatty acids in beef and veal
The FA profile of veal and beef is largely influenced by diet, with forage finishing being the most practical strategy to increase the proportions of PUFAs, particularly n-3 PUFA and their BHIs (mostly RA and TVA), as previously reviewed by numerous authors (Mapiye et al., 2015; Vahmani et al., 2015; Berthelot and Gruffat, 2018). Therefore, only recent studies relating to the dietary manipulation of HEFA in beef have been included in Table 3.
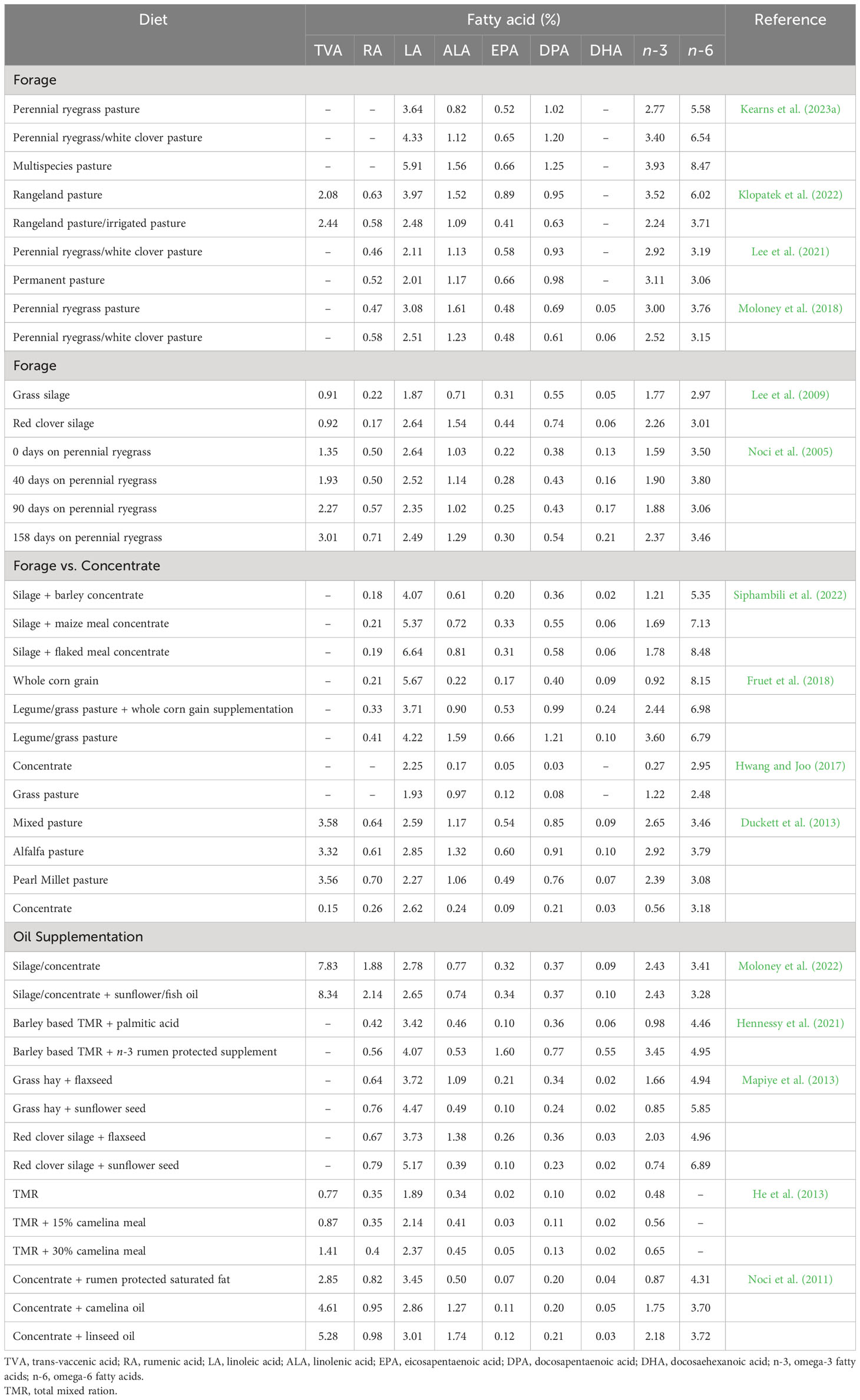
Table 3 Influence of diet on the health enhancing fatty acid composition (% of total fatty acids) of beef from ruminants.
The extent of these changes in beef is mainly dependent on the PUFA content of forage, in particular ALA, which is the predominant PUFA in most forage species (Dierking et al., 2010), and on finishing duration on these forages pre-slaughter (Noci et al., 2005). Noci et al. (2005) observed a linear increase in the n-3 PUFA content of beef up to 158 days of grazing. In general, fresh forages and pastures have higher contents of total lipids and total PUFA compared to conserved forages (i.e., silage and hay). This is mainly due to oxidative degradation of PUFA during haymaking and ensiling processes. In terms of species, legumes generally have higher contents of total FA, total PUFA and n-3 PUFAs, particularly ALA, compared to most grass species. However, grass offers greater levels of ALA than most cereal grains, which are the main sources of n-6 PUFA, mostly LA (Boufaïed et al., 2003). Consequently, beef from cattle grazing pastures containing white or red clover has higher proportions of n-3 PUFAs, along with a lower n-6:n-3 ratio (Lee et al., 2009; Berthelot and Gruffat, 2018; Moloney et al., 2018). Additionally, grazing steers on multispecies pasture consisting of different grasses, legumes and forbs resulted in beef with higher levels of LA, ALA and a higher n-6:n-3 ratio compared to beef from steers grazing perennial ryegrass (Kearns et al., 2023a). Given fresh forages and pastures contain higher concentrations of ALA, grazing is more effective in increasing n-3 PUFA and their respective BHIs while lowering the n-6:n-3 ratio in beef compared to feeding conserved forages and high-grain diets.
Conversely, LA is the predominant FA in grain-based diets which leads to increased n-6 PUFA contents, particularly LA, and higher n-6:n-3 ratios in grain-finished feedlot fed beef (Vahmani et al., 2015; Berthelot and Gruffat, 2018; Klopatek et al., 2022). Despite feedlot beef having a higher n-6:n-3 ratio (5:1 to 8:1) than forage-finished beef (1:1 to 3:1), the ratios are still lower than typical chicken and pork (15:1 to 19:1) (Dugan et al., 2015; Kim et al., 2020). In fact, consuming either forage or grain-finished beef may help to reduce the n-6:n-3 ratio in western diets which is currently estimated to be around 20:1 (DiNicolantonio and O'Keefe, 2021). In addition to forage-finishing strategies, attempts have been made to increase the amount of n-3 PUFAs in beef through supplementing diets with n-3 PUFA rich oils or oilseed meals (e.g., canola oil or canola meal). However, limited n-3 PUFA enrichments can be achieved using this approach because of extensive biohydrogenation of PUFA in the rumen (Vahmani et al., 2015) when compared with supplementing marine-based (algae) or chemically protected n-3 PUFA sources. Moreover, PUFA supplementation, particularly LA in forage diets often results in an increased tissue deposition of BHI, particularly TVA and RA, while supplementation in high-grain diets yields t10-18:1 and t10,c12-CLA (Vahmani et al., 2020; Alves et al., 2021). Given recent studies have shown that ruminant-derived trans FAs such as t10-18:1 (Vahmani et al., 2020) have similar adverse effects to that of industrial trans FAs (Verneque et al., 2022), it would be of importance to determine if the health value of beef enriched with n-3 PUFA is still maintained when different proportions of trans FAs are present.
2.3 Influence of diets on health enhancing fatty acids in chevon
Goat meat (i.e., chevon) has less fat than other red meat from ruminants (James and Berry, 1997), having about 50% less intramuscular fat (IMF) than beef and lamb with similar protein contents (Webb et al., 2005). In general, chevon contains higher ALA, EPA and DPA, but lower undesirable SFA (C14:0, C16:0, C16:1) and n-6:n-3 ratio compared with lamb and beef. Table 4 compares the fatty acid composition of chevon, lamb and beef determined from goat kids, lambs and calves, on % of total fatty acids.
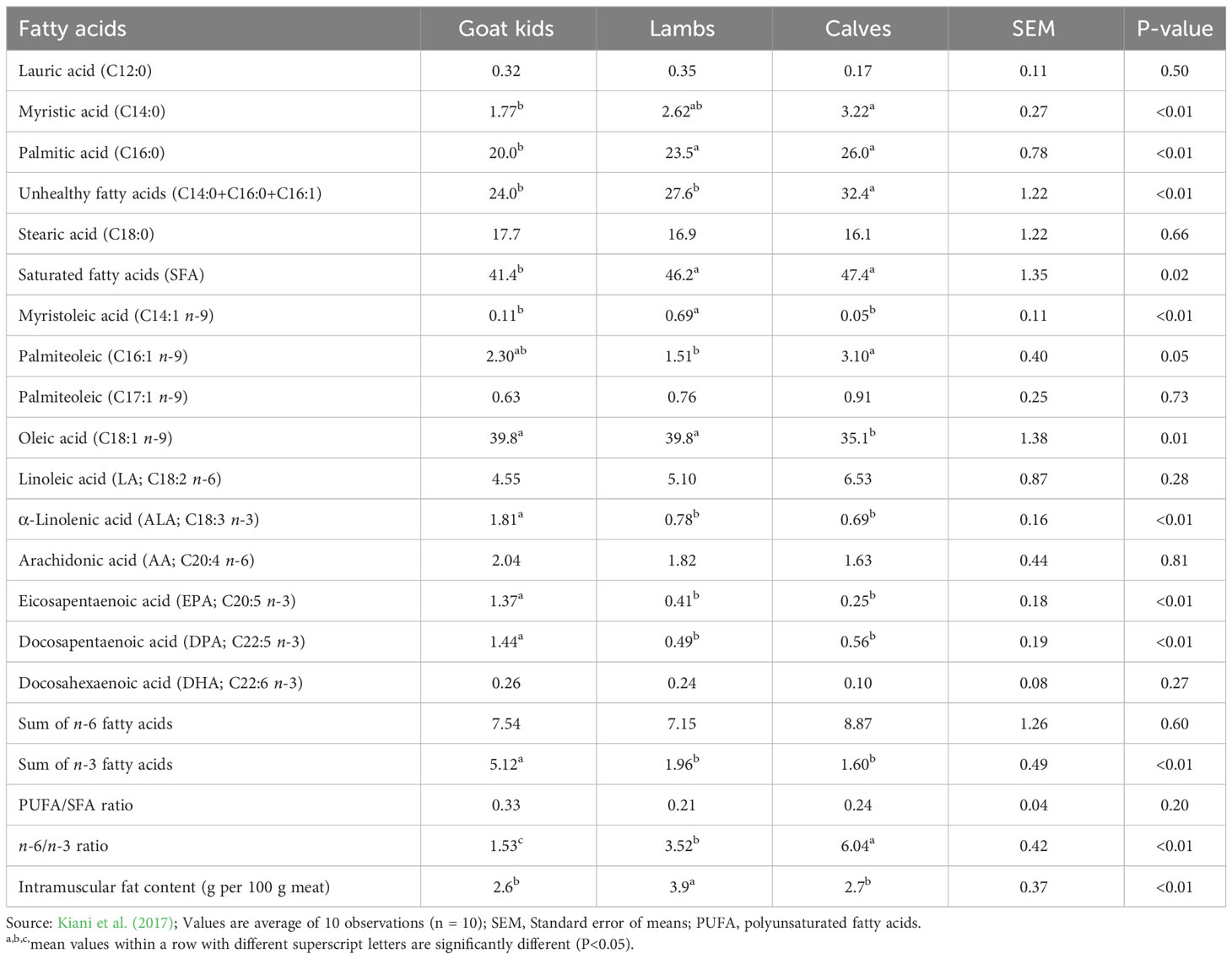
Table 4 Comparison of fatty acid composition (% of total fatty acids) and intramuscular fat (IMF, g per 100 g of meat) of muscle longissimus dorsi from goats (kids), lambs and calves.
Various factors affect the FA composition of goat meat, particularly the diet of the animal. Meat from goats grazing rangeland forages (Liotta et al., 2020) or high-forage diets supplemented with n-3 PUFA sources contain increased contents of n-3 PUFA and the BHIs than those raised intensively on high grain diets (Gagaoua et al., 2023). It has been reported that feeding goats with a grain diet containing threefold C18:1 compared to an alfalfa hay diet increased C18:1 in both IMF (i.e., marbling) and subcutaneous fat. Thus, when East African goats were supplemented with a grain diet (27% sunflower seed cake + 70% maize bran), the proportions of C18:1, TVA and LA in the omental fat increased compared to non-supplemented goats (Gagaoua et al., 2023). The increase in C18:1 in muscle or adipose tissues in response to higher grain levels in the diet could be due to the increase in the Δ9 desaturase enzyme activities necessary for the conversion of C18:0 to C18:1, which can also be seen in sheep and cattle.
When goats are fed with oils rich in LA, it can bypass the rumen or is isomerised to RA, which can be bio-hydrogenated to TVA in the rumen increasing their deposition in the muscle tissues. Supplementing goat diets with canola or olive oils (e.g., oils rich in oleic acid) and sunflower or soybean oils (e.g., oils rich in LA) has mainly resulted in an increase in C18:1 and RA in the meat, respectively. On the other hand, supplementation of diets with oils rich in ALA can lead to an increase in the n-3 FA level in chevon. Related to this, the incorporation of 3% canola oil, a good source of ALA with a 2:1 ratio of n-6:n-3, into the goats’ diet has enhanced ALA in the muscle, liver, and kidney fats (Karami et al., 2013). Studies report that supplementation of diets with oils can affect expression levels of genes associated with de novo FA synthesis in goats. A blend of linseed and palm oils (2:1) has reduced both rumen biohydrogenation of ALA and muscle oxidation of ALA in Cashmere goats. Diets supplemented with the blended oil, compared to linseed alone, have reduced the relative abundance of Pseudobutyrivibrio, a bacterial species that hydrogenate dietary ALA in the rumen, increasing n-3 PUFA, leading to a decrease in the n-6:n-3 FA ratio in goat meat. In this regard, a variety of nutritional strategies have been employed to increase HEFA deposition in goat meat, which have been summarised in Table 5.
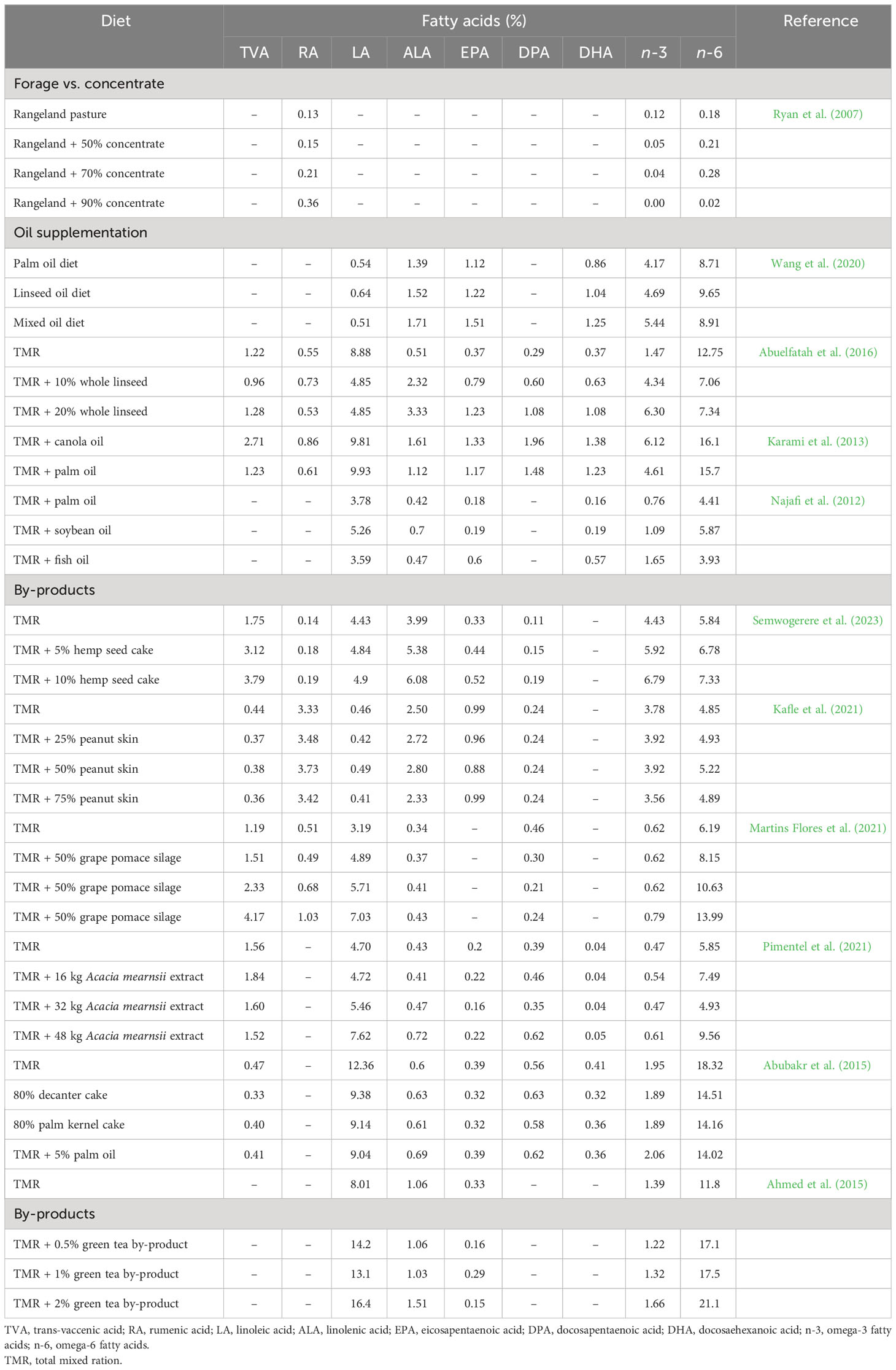
Table 5 Influence of diet on the health enhancing fatty acid composition (% of total fatty acids) of meat from goats.
3 Feed-based enrichment of antioxidants in red meat from sheep, cattle and goats
It is known that diets rich in antioxidants offer better animal health (Ponnampalam et al., 2022), including resistance to heat stress and contribute to a reduction in deteriorative changes in meat quality post mortem by reducing radical formation within the cellular system and reducing the amount of oxidative damage in muscle tissues (Bekhit et al., 2013; Karre et al., 2013; Ponnampalam et al., 2022). Antioxidants reduce the occurrence of oxidative stress and infectious diseases in animals and humans (Lauridsen, 2019; Ponnampalam et al., 2022). Vitamin E (primarily α-tocopherol) is considered an efficient antioxidant functioning compound in a hydrophobic environment. It has been proven from animal and human studies that vitamin E inhibits lipid peroxidation (Gruffat et al., 2020), and by scavenging lipid peroxyl radicals, it prevents or delays the propagation of free radical-mediated chain reactions in the cellular systems. Antioxidants can act synergistically, for example, α-tocopherol and β-carotene can act as an effective ‘radical-scavenging mechanism’ in biological membranes (Kurutas, 2015; Salami et al., 2016; Lauridsen et al., 2021). The inhibition of lipid peroxidation by a combination of the two fat-soluble antioxidants was shown to be greater than the sum of the individual inhibitions (Wrona et al., 2003). Figure 3 illustrates the mechanism of the effect of antioxidants on the quality of meat from ruminant animals (goat is taken as an example in the diagram). The antioxidant action of vitamin E and selenium have been proven in human and animal studies by their fortification in the tissues and defensive effects to reduce oxidation (Nozière et al., 2006). Carotenoids are also regarded as efficient O2 and ROS scavengers operating in cellular lipid bilayers. However, the action of other plant-based antioxidants, namely polyphenols (phenolic acids, tannins, flavonoids), has not been proven through their fortification in animal or human tissues. Flavonoids are thought to have a lower contribution to the direct antioxidative protection in animals and humans, due to their relatively poor absorption and difficulties with storage in animal tissues, even though they are potent scavengers of hydroxyl and superoxide radicals (Fiedor and Burda, 2014).
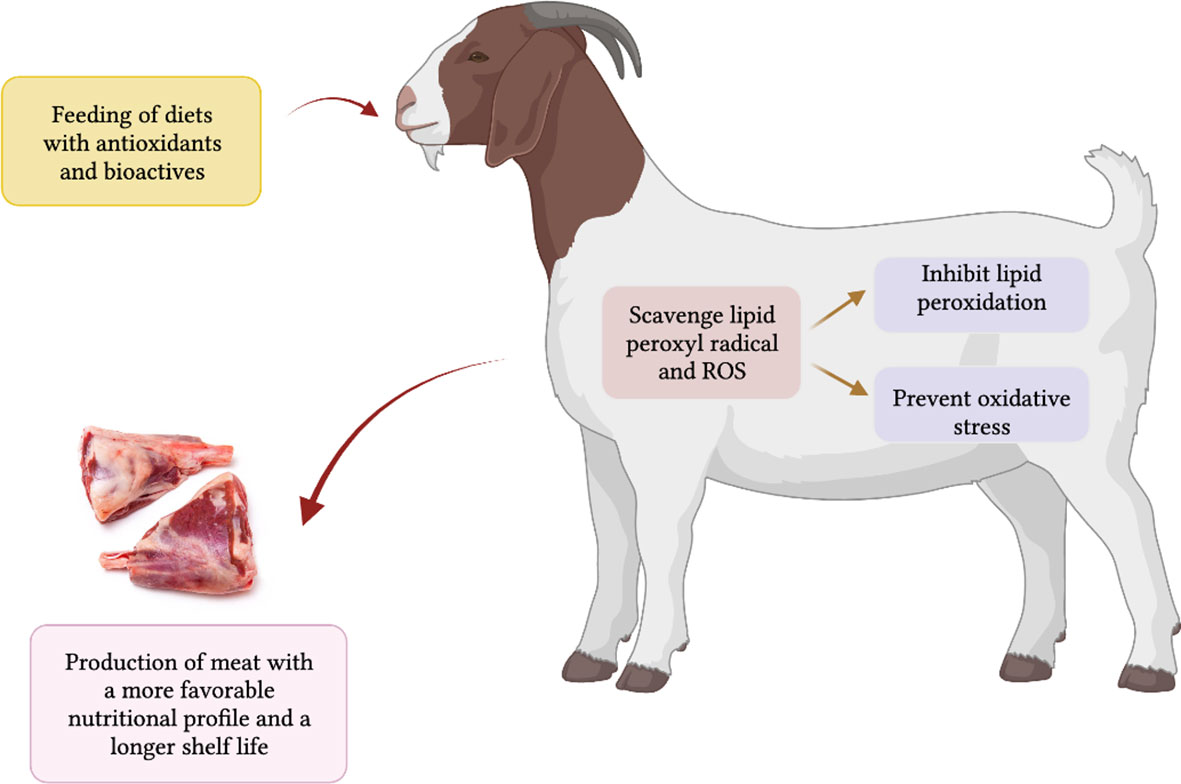
Figure 3 Mechanism of the effect of antioxidants (vitamins and carotenoids) and bioactives enriched feed on the animal health and meat quality of sheep, cattle and goats (goat is taken as an example).
3.1 Influence of diets on antioxidants in lamb and mutton
Modulating the FA profile of lamb and mutton to increase beneficial PUFAs increases susceptibility to lipid oxidation, which can negatively affect meat quality attributes. The presence of natural or supplemented antioxidants in the diet of ruminants can help to reduce oxidation and increase shelf-life (Prache et al., 2022b). Meat from pasture-fed sheep and lambs is characterised by having higher muscle antioxidants levels (mainly α-tocopherol) compared to those fed grains (Zervas and Tsiplakou, 2011; Gruffat et al., 2020), as fresh pasture is a rich source of antioxidants such as tocopherols, carotenoids and phenolic compounds (Prache et al., 2022b). However, variability between breeds in the metabolic fate of ingested carotenoids has been observed (Macari et al., 2017). The presence of different plant species within the pasture can also modulate antioxidant contents in meat, with higher α-tocopherol concentrations reported in the meat of lambs grazing brassica and lucerne/phalaris compared to bladder clover (De Brito et al., 2017). Leguminous species are reported to contain lower levels of α-tocopherol compared to grasses, which may explain higher lipid oxidation values in the meat of lambs grazing alfalfa versus perennial ryegrass (Fraser et al., 2004), however variable results have been reported (Hampel et al., 2021).
The antioxidant content of lamb meat declines with increased inclusion of senesced pasture and TMR in the diet (Ponnampalam et al., 2012, 2017), due to the lower levels of tocopherols, carotenoids and phenolic compounds available in these feed types (Moure et al., 2001). Supplementing feedstuffs containing natural antioxidants to increase concentrations in meat has yielded variable results (Falowo et al., 2014). Supplementing TMR diets with pomegranate silage (Kotsampasi et al., 2014), buckwheat silage (Keles et al., 2018) and increasing levels of Acacia mearnsii leaf-meal (Uushona et al., 2023) increased the antioxidant capacity and phenolic content of lamb meat. Inclusion of oat grain in senesced perennial ryegrass pasture lowered α-tocopherol concentrations in lamb meat compared to senesced perennial lucerne pasture. A reduction in α-tocopherol concentrations and increasing oxidation values have been reported in meat from lambs fed TMR supplemented with algae (Hopkins et al., 2014; Ponnampalam et al., 2016) and camelina hay/meal (Ponnampalam et al., 2021a). Overall, pasture grazing is the most advantageous way to increase the antioxidant content of sheep and lamb meat. Supplementation of senesced pasture and TMR diets with antioxidant rich by-products is important to sufficiently improve the antioxidant content of meat.
3.2 Influence of diets on antioxidants in beef and veal
Use of dietary antioxidants is the most common strategy used to inhibit lipid, protein, and myoglobin oxidation in beef (Estévez, 2021; Petcu et al., 2023) and veal (Skřivanová et al., 2007; D’Agata et al., 2009). It has long been established that forage-based versus grain-based diets are highly endowed with diverse and potent antioxidants, which result in beef and veal with superior antioxidant and oxidative stability profiles (Descalzo and Sancho, 2008; Estévez, 2021). For illustrative purposes, feeding pasture versus grain diets can increase vitamin E content in beef up to 5.9 μg/g of tissue exceeding the ideal concentration of 3.3-3.8 mg/g tissue required to extend oxidative shelf life of beef (Liu et al., 1995; Descalzo and Sancho, 2008). However, the efficacy of these antioxidants in pasture-fed beef depends on dose and type of the antioxidant, plant species and maturity, seasonality, physical form, duration of feeding, amount, and type of pro-oxidants (Ponnampalam et al., 2022; Kearns et al., 2023b).
Low-quality forages and high-grain diets can be supplemented with vitamin E to elevate levels in beef (Descalzo and Sancho, 2008; Petcu et al., 2023) and veal (D’Agata et al., 2009; Franco et al., 2012), subsequently minimizing oxidative deterioration. It has been suggested that vitamin E daily supplementation of 500 IU/head for 126 d or 1300 IU/head for 44 d could meet the ideal vitamin E levels in muscle required to enhance oxidative stability of beef (Liu et al., 1995; Suman et al., 2014). Apart from vitamin E, supplementation of organic selenium appears to somewhat increase oxidative capacity and stability of beef (Rossi et al., 2015; Huang et al., 2023) and veal (Skřivanová et al., 2007; Shabtay et al., 2008). In addition, supplementation of high-grain diets with polyphenolic extracts including flavonoids (Orzuna-Orzuna et al., 2023), a blend of alkaloids, saponins, and phenolic acids (De Zawadzki et al., 2017), resveratrol (Cui et al., 2023), ferulic acid (González-Ríos et al., 2016) and tannic acid (Tabke et al., 2017) enhances the antioxidant capacity and oxidative stability of beef. Similarly, supplementation of polyphenolic fruit by-products such as grape pomace in calves’ (Ianni et al., 2019) and steers’ (Tayengwa et al., 2020) on high-grain diets increased the antioxidant capacity and oxidative stability of meat. Supplementing a blend of essential oils (Rivaroli et al., 2016; Ornaghi et al., 2020) and benzoic acid (Williams et al., 2022) slightly improved lipid stability without affecting colour shelf-life.
The effect of distiller’s grains on the oxidative capacity and lipid stability of beef varies from negative (Suman et al., 2014; De Mello et al., 2018) to neutral (Gill et al., 2008; Depenbusch et al., 2009) and positive (Bloomberg et al., 2011; Merayo et al., 2022) and, thus, merits further investigation. Interestingly, a blend of antioxidant sources tends to either have additive or synergistic effects. For example, a combination of vitamin E with polyphenolic extracts (Gobert et al., 2010; Delosière et al., 2020), essential oils (Fusaro et al., 2021) and distiller’s grains (Bloomberg et al., 2011) was more efficient in increasing antioxidant capacity and oxidative stability than the individual constituents, suggesting synergistic effects with vitamin E. However, high dietary levels of vitamin A suppress tissue deposition of vitamin E reducing antioxidant capacity and oxidative stability of beef (Daniel et al., 2009; Marti et al., 2011). Research should continue to explore synergistic effects of novel nutrient and non-nutrient antioxidants.
3.3 Influence of diets on antioxidants in chevon
Pasture-finished goats differ in colour attributes of meat (L*, a, b, chroma and hue angle values) than goats finished on grain diets (Lee et al., 2008), the same for meat from sheep and cattle. Adding natural antioxidants (Vitamin E, Vitamin C, etc.) and polyphenols, as a nutritional strategy influences the colour stability of goat meat (Karami et al., 2011). For example, pomegranate seed pulp, a cheap source of polyphenols, has improved colour stability in chevon (Emami et al., 2015). Similarly, a dietary green tea by-product (20 g/kg dry matter) has improved the redness and yellowness values of goat meat (Ahmed et al., 2015).
It is noteworthy that supplementing the diet with a high content of n-3 PUFA might increase lipid oxidation and may raise concerns about the impairment of sensory attributes of chevon, similar to that observed with beef and lamb. The inclusion of high doses of n-3 PUFA has produced meat with unusual odours, unpleasant flavours, and a lower overall appreciation of kid meat (Moreno-Indias et al., 2012). A positive association between lipid oxidation and the content of n-3 PUFA in chevon has been reported (Abuelfatah et al., 2016). Intriguingly, liver and muscle (meat) fats from canola oil-fed kids contained fewer lipid oxidative substances compared to those from palm oil-supplemented kids (Karami et al., 2013). The latter study showed that canola oil effectively reduced lipid oxidation both in the blood and muscle tissue of goats. Furthermore, the concern about lipid oxidation of high-PUFA diets could be overcome by dietary supplementation of antioxidant polyphenols, a conclusion that is relevant to all ruminant species.
It has been reported that supplementation of diets containing polyphenols positively improved the FA profile and reduced lipid oxidation in chevon (Cimmino et al., 2018). Similarly, dietary green tea by-products (Ahmed et al., 2015) and extracts from olive mill water waste (Cimmino et al., 2018) reduced lipid oxidation in chevon. Overall, it seems that diets containing phenolic components are positive agents in reducing lipid oxidation in goat meat. These actions may be through synchronised effects with lipids such as unsaturated fatty acids and vitamins (such as vitamin E) at the GIT enterocyte level. However, the dietary biofortification of phytonutrients into tissues such as skeletal muscles is low due to lower rates of digestion and absorption; this is believed to be due to their action as antinutritional factors (causing toxicity to microbes) or binding agents with other nutrients (e.g., protein) leading to complex formation at the GIT level, resulting in reduced microbial degradation.
4 Future directions and gaps
The importance of HEFA and antioxidants is becoming increasingly important, particularly in relation to animal health, as well as the quality and nutritional value of meat, and ultimately human health and wellbeing. Also, there is an increasing trend towards the consumption of red meat compared with other white meats around the world, as global population and affluency increase mainly from Asian and African regions. The social awareness of consumers relating to the quality of meat consumed, the nutrient content of meat that they select, and the health aspects of foods chosen is also expected to change in the coming years as world population become aware of healthy living (Ponnampalam and Holman, 2022). Therefore, addressing the enrichment of red meat with HEFA and antioxidants i.e., improving the nutritional value of meat, has gained much attention. The use of bioactive-rich (vitamins, HEFA, phenolic compounds, tannins, flavonoids) plant by-products for improving the nutritional quality attributes and shelf-life of the meat is under active investigation (Salami et al., 2019), but research is needed to evaluate their effects on other meat quality attributes and animal performance. A point of caution is meat safety, insofar as certain pathogenic agents in plants can be transferred to the meat (Prache et al., 2022a). Although several studies have investigated the effects of feed on the quality and nutritional values of meat, there is a lack of information on the absorption and bioavailability of particular feed-based antioxidants and FA, and their effect in animal- and human-tissues upon deposition. Some authors also point to the gap between food-scale studies and epidemiological studies (Prache et al., 2022a). There is a lack of data linking the FA profile of meat and its antioxidant content (and more generally the way it is produced), to the level of consumption and chronic diseases in humans. This requires a collective effort between research communities working in animal science, meat processing, consumer attitudes, human nutrition and epidemiology (Prache et al., 2022a).
Comparative studies on the consumption of red meat from ruminants versus white meat and their combinations as well as comparing with other sources of animal and plant proteins can provide valuable insights and knowledge into their potential effects on human health. However, this research aspect is lacking and not well proven by human intervention studies, relating it to long term consumption of red meat enriched with HEFA and antioxidants on blood lipid parameters, human health and wellbeing. This may be due to inadequate funding allocated by government bodies around the globe as it requires large funding and intense resources, as well as due to the ethical considerations, regulatory requirements, and recruitment challenges in enrolling adequate numbers of participants (observations) to validate data. Future studies should focus on not only investigating the absorption, bioaccessibility, and bioavailability of different antioxidants and FA through use of in vitro digestion models and in vivo animal studies, but also include human intervention studies undertaken with sound experimental designs covering appropriate replicates and number of observations, comparing the effects of red meat, white meat and other plant and animal protein sources (alone and/or in combination) in human health. Furthermore, animal and human cell lines should be treated (fortified) with plant-based antioxidants, for example phenolic acids or flavonoids, to study their real effects in animal and human tissues as they show antinutritional activities that may lead to lower digestion, absorption and biofortification. Such studies may provide an understanding of the impact of phytonutrients acting as a sole or additive components along with HEFA and other antioxidants (vitamin E, selenium) that are available in the meat to exert a beneficial effect on humans. This, in turn, is key to gaining a deep insight into the mechanism of action of improving human health via enriching red meat with feed-based FA and antioxidants.
5 Conclusions
Regardless of ruminant species, high-quality pasture remains superior in producing meat enriched with HEFA, particularly n-3 PUFA and antioxidants, with some countries already utilising ‘grass-fed’ logos (see Salami et al., 2019; Davis et al., 2022). Low-quality forages and high grain diets require dietary supplementation with n-3 PUFA and antioxidants to raise their contents in meat and extend its shelf life. The importance of some dietary vitamins and minerals in the antioxidant actions and preservative aspects of PUFA in meat has been documented. A combination of nutrient antioxidants, mostly vitamin E and non-nutrient antioxidants, chiefly flavonoids, and carotenoids are speculated to synergistically stabilise high PUFA contents in ruminant meat. Nevertheless, the latter statement needs validation through well-designed in vivo studies undertaken in animal and human populations (with adequate replicates and numbers of observations) along with the quantification of flavonoids or carotenoids in circulatory systems and tissues such as skeletal muscles, and consequently their effects on human health. To this end, research should continue to explore effects of co-feeding of PUFA sources with different mixtures of novel nutrient and non-nutrient antioxidants on animal and human health as well as the underlying mechanisms.
Author contributions
EP: Conceptualization, Writing – original draft, Writing – review & editing. MK: Writing – original draft, Writing – review & editing. AK: Writing – original draft, Writing – review & editing. SS: Writing – original draft, Writing – review & editing. PV: Writing – original draft, Writing – review & editing. SP: Writing – original draft, Writing – review & editing. FM: Writing – original draft, Writing – review & editing. CM: Writing – original draft, Writing – review & editing.
Funding
The author(s) declare that no financial support was received for the research, authorship, and/or publication of this article.
Acknowledgments
The authors are grateful to the support of their corresponding organizations. The first author EP wishes to acknowledge Robyn D. Warner and Brian J. Leury of the University of Melbourne, Australia for their support and collaboration during his career. Their support allows EP to expand his research ideas and hypotheses using field- and laboratory-based investigations with fundamental and applied based research activities.
Conflict of interest
The authors declare that the research was conducted in the absence of any commercial or financial relationships that could be construed as a potential conflict of interest.
The author(s) declared that they were an editorial board member of Frontiers, at the time of submission. This had no impact on the peer review process and the final decision.
Publisher’s note
All claims expressed in this article are solely those of the authors and do not necessarily represent those of their affiliated organizations, or those of the publisher, the editors and the reviewers. Any product that may be evaluated in this article, or claim that may be made by its manufacturer, is not guaranteed or endorsed by the publisher.
References
Abid Z., Cross A. J., Sinha R. (2014). Meat, dairy, and cancer. Am. J. Clin. Nutr. 100, 386S–393S. doi: 10.3945/ajcn.113.071597
Abubakr A., Alimon A. R., Yaakub H., Abdullah N., Ivan M. (2015). Effect of feeding palm oil by-products based diets on muscle fatty acid composition in goats. PloS One 10, e0119756–e0119756. doi: 10.1371/journal.pone.0119756
Abuelfatah K., Zuki A. B. Z., Goh Y. M., Sazili A. Q. (2016). Effects of enriching goat meat with n–3 polyunsaturated fatty acids on meat quality and stability. Small Ruminant Res. 136, 36–42. doi: 10.1016/j.smallrumres.2016.01.001
Ahmed S. T., Lee J. W., Mun H. S., Yang C. J. (2015). Effects of supplementation with green tea by-products on growth performance, meat quality, blood metabolites and immune cell proliferation in goats. J. Anim. Physiol. Anim. Nutr. 99, 1127–1137. doi: 10.1111/jpn.12279
Alves S. P., Vahmani P., Mapiye C., McAllister T. A., Bessa R. J. B., Dugan M. E. R. (2021). Trans-10 18:1 in ruminant meats: A review. Lipids 56, 539–562. doi: 10.1002/lipd.12324
Asadollahi S., Sari M., Erafanimajd N., Kiani A., Ponnampalam E. N. (2017). Supplementation of sugar beet pulp and roasted canola seed in a concentrate diet altered carcass traits, muscle (longissimus dorsi) composition and meat sensory properties of arabian fattening lambs. Small Ruminant Res. 153, 95–102. doi: 10.1016/j.smallrumres.2017.05.012
Aurousseau B., Bauchart D., Calichon E., Micol D., Priolo A. (2004). Effect of grass or concentrate feeding systems and rate of growth on triglyceride and phospholipid and their fatty acids in the M. longissimus thoracis of lambs. Meat Sci. 66, 531–541. doi: 10.1016/S0309-1740(03)00156-6
Aurousseau B., Bauchart D., Faure X., Galot A. L., Prache S., Micol D., et al. (2007). Indoor fattening of lambs raised on pasture: (1) Influence of stall finishing duration on lipid classes and fatty acids in the longissimus thoracis muscle. Meat Sci. 76, 241–252. doi: 10.1016/j.meatsci.2006.11.005
Bekhit A. E. D. A., Hopkins D. L., Fahri F. T., Ponnampalam E. N. (2013). Oxidative processes in muscle systems and fresh meat: Sources, markers, and remedies. Compr. Rev. Food Sci. Food Saf. 12, 565–597. doi: 10.1111/1541-4337.12027
Bennato F., Martino C., Ianni A., Giannone C., Martino G. (2023). Dietary grape pomace supplementation in lambs affects the meat fatty acid composition, volatile profiles and oxidative stability. Foods 12, 1257. doi: 10.3390/foods12061257
Bernstein A. M., Sun Q., Hu F. B., Stampfer M. J., Manson J. E., Willett W. C. (2010). Major dietary protein sources and risk of coronary heart disease in women. Circulation 122, 876–883. doi: 10.1161/CIRCULATIONAHA.109.915165
Berthelot V., Gruffat D. (2018). “Fatty acid composition of muscle,” in INRA Feeding System for Ruminants. Eds. Sauvant D., Delaby L., Nozière P. (Academic Publishers, Wageningen, The Netherlands), 193–202.
Bessa R. J. B., Alves S. P., Santos-Silva J. (2015). Constraints and potentials for the nutritional modulation of the fatty acid composition of ruminant meat. Eur. J. Lipid Sci. Technol. 117, 1325–1344. doi: 10.1002/ejlt.201400468
Bloomberg B. D., Hilton G. G., Hanger K. G., Richards C. J., Morgan J. B., Vanoverbeke D. L. (2011). Effects of vitamin E on color stability and palatability of strip loin steaks from cattle fed distillers grains. J. Anim. Sci. 89, 3769–3782. doi: 10.2527/jas.2011-3843
Boufaïed H., Chouinard P. Y., Tremblay G. F., Petit H. V., Michaud R., Bélanger G. (2003). Fatty acids in forages. I. Factors affecting concentrations. Can. J. Anim. Sci. 83, 501–511. doi: 10.4141/A02-098
Bourre J. M. (2011). Reintroducing beef in a balanced diet. Bull. Académie Vétérinaire France 164, 237–244. doi: 10.4267/2042/48092
Cabiddu A., Decandia M., Addis M., Piredda G., Pirisi A., Molle G. (2005). Managing mediterranean pastures in order to enhance the level of beneficial fatty acids in sheep milk. Small Ruminant Res. 59, 169–180. doi: 10.1016/j.smallrumres.2005.05.005
Cabrera M. C., Saadoun A. (2014). An overview of the nutritional value of beef and lamb meat from South America. Meat Sci. 98, 435–444. doi: 10.1016/j.meatsci.2014.06.033
Campidonico L., Toral P. G., Priolo A., Luciano G., Valenti B., Hervás G., et al. (2016). Fatty acid composition of ruminal digesta and longissimus muscle from lambs fed silage mixtures including red clover, sainfoin, and timothy. J. Anim. Sci. 94, 1550–1560. doi: 10.2527/jas.2015-9922
Chauhan S. S., Celi P., Ponnampalam E. N., Leury B. J., Liu F., Dunshea F. R. (2014). Antioxidant dynamics in the live animal and implications for ruminant health and product (meat/milk) quality: role of vitamin E and selenium. Anim. Production Sci. 54, 1525–1536. doi: 10.1071/AN14334
Chikwanha O. C., Vahmani P., Muchenje V., Dugan M. E. R., Mapiye C. (2018). Nutritional enhancement of sheep meat fatty acid profile for human health and wellbeing. Food Res. Int. 104, 25–38. doi: 10.1016/j.foodres.2017.05.005
Chilliard Y., Glasser F., Ferlay A., Bernard L., Rouel J., Doreau M. (2007). Diet, rumen biohydrogenation and nutritional quality of cow and goat milk fat. Eur. J. Lipid Sci. Technol. 109, 828–855. doi: 10.1002/ejlt.200700080
Ciftci M., Cerci I. H., Kilinc U., Yilmaz O., Gurdogan F., Seven P. T., et al. (2010). Effects of alfalfa (fresh, silage, hay) on the fatty acid and conjugated linoleic acid amounts in lamb muscles and fats. Rev. Médecine Vétérinaire 161, 432–437.
Cimmino R., Barone C. M. A., Claps S., Varricchio E., Rufrano D., Caroprese M., et al. (2018). Effects of dietary supplementation with polyphenols on meat quality in saanen goat kids. BMC Veterinary Res. 14, 181–181. doi: 10.1186/s12917-018-1513-1
Clinquart A., Ellies-Oury M. P., Hocquette J. F., Guillier L., Santé-Lhoutellier V., Prache S. (2022). Review: On-farm and processing factors affecting bovine carcass and meat quality. Animal 16, 100426–100426. doi: 10.1016/j.animal.2021.100426
Correddu F., Caratzu M. F., Lunesu M. F., Carta S., Pulina G., Nudda A. (2023). Grape, pomegranate, olive, and tomato by-products fed to dairy ruminants improve milk fatty acid profile without depressing milk production. Foods 12, 865. doi: 10.3390/foods12040865
Cui Y., Qi J., Li J., Zhang Y., Yang X., Xin L., et al. (2023). Effects of dietary resveratrol supplementation in cattle on the anti-oxidative capacity and meat quality of beef steaks under high oxygen packaging. Meat Sci. 204, 109238. doi: 10.1016/j.meatsci.2023.109238
D’Agata M., Preziuso G., Russo C., Gatta D. (2009). Oxidation and antioxidant status: effects on shelf-life of meat from Limousine cattle fed with supplements of α-tocopherol. Ital. J. Anim. Sci. 8, 405–415. doi: 10.4081/ijas.2009.405
Daniel M. J., Dikeman M. E., Arnett A. M., Hunt M. C. (2009). Effects of dietary vitamin A restriction during finishing on color display life, lipid oxidation, and sensory traits of longissimus and triceps brachii steaks from early and traditionally weaned steers. Meat Sci. 81, 15–21. doi: 10.1016/j.meatsci.2008.07.003
Davis H., Magistrali A., Butler G., Stergiadis S. (2022). Nutritional benefits from fatty acids in organic and grass-fed beef. Foods 11, 646. doi: 10.3390/foods11050646
De Brito G. F., Holman B. W. B., McGrath S. R., Friend M. A., Van De Ven R., Hopkins D. L. (2017). The effect of forage-types on the fatty acid profile, lipid and protein oxidation, and retail colour stability of muscles from White Dorper lambs. Meat Sci. 130, 81–90. doi: 10.1016/j.meatsci.2017.04.001
Delosière M., Durand D., Bourguet C., Terlouw E. C. (2020). Lipid oxidation, pre-slaughter animal stress and meat packaging: Can dietary supplementation of vitamin E and plant extracts come to the rescue? Food Chem. 309, 125668. doi: 10.1016/j.foodchem.2019.125668
De Mello A. S., Jenschke B. E., Senaratne L. S., Carr T. P., Erickson G. E., Calkins C. R. (2018). Effects of finishing diets containing wet distillers’ grains plus solubles on beef quality attributes and fatty acid profile. Meat Sci. 136, 16–22. doi: 10.1016/j.meatsci.2017.10.001
Depenbusch B. E., Coleman C. M., Higgins J. J., Drouillard J. S. (2009). Effects of increasing levels of dried corn distillers grains with solubles on growth performance, carcass characteristics, and meat quality of yearling heifers. J. Anim. Sci. 87, 2653–2663. doi: 10.2527/jas.2008-1496
Descalzo A. M., Sancho A. M. (2008). A review of natural antioxidants and their effects on oxidative status, odor and quality of fresh beef produced in Argentina. Meat Sci. 79, 423–436. doi: 10.1016/j.meatsci.2007.12.006
De Zawadzki A., Arrivetti L. O. R., Vidal M. P., Catai J. R., Nassu R. T., Tullio R. R., et al. (2017). Mate extract as feed additive for improvement of beef quality. Food Res. Int. 99, 336–347. doi: 10.1016/j.foodres.2017.05.033
Dierking R. M., Kallenbach R. L., Grün I. U. (2010). Effect of forage species on fatty acid content and performance of pasture-finished steers. Meat Sci. 85, 597–605. doi: 10.1016/j.meatsci.2010.03.010
DiNicolantonio J. J., O'Keefe J. (2021). The importance of maintaining a low omega-6/omega-3 ratio for reducing the risk of autoimmune diseases, asthma, and allergies. Missouri Med. 118, 453–459.
Duckett S. K., Neel J. P. S., Lewis R. M., Fontenot J. P., Clapham W. M. (2013). Effects of 553 forage species or concentrate finishing on animal performance, carcass and meat quality. J. Anim. Sci. 91, 1454–1467. doi: 10.2527/jas.2012-5914
Dugan M. E. R., Vahmani P., Turner T. D., Mapiye C., Juárez M., Prieto N., et al. (2015). Pork as a source of omega-3 (n-3) fatty acids. J. Clin. Med. 4, 1999–2011. doi: 10.3390/jcm4121956
Emami A., Nasri M. H., Ganjkhanlou M., Zali A., Rashidi L. (2015). Effects of dietary pomegranate seed pulp on oxidative stability of kid meat. Meat Sci. 104, 14–19. doi: 10.1016/j.meatsci.2015.01.016
Estévez M. (2021). Critical overview of the use of plant antioxidants in the meat industry: Opportunities, innovative applications and future perspectives. Meat Sci. 181, 108610. doi: 10.1016/j.meatsci.2021.108610
Falowo A. B., Fayemi P. O., Muchenje V. (2014). Natural antioxidants against lipid–protein oxidative deterioration in meat and meat products: A review. Food Res. Int. 64, 171–181. doi: 10.1016/j.foodres.2014.06.022
FAO (2017). Driving Action Across the 2030 Agenda for Sustainable Development (Rome, ITA). Available at: http://www.fao.org/3/i7454en/i7454en.pdf.
Fiedor J., Burda K. (2014). Potential role of carotenoids as antioxidants in human health and disease. Nutrients 6, 466–488. doi: 10.3390/nu6020466
Franco D., González L., Bispo E., Latorre A., Moreno T., Sineiro J., et al. (2012). Effects of calf diet, antioxidants, packaging type and storage time on beef steak storage. Meat Sci. 90, 871–880. doi: 10.1016/j.meatsci.2011.10.008
Fraser M. D., Speijers M. H. M., Theobald V. J., Fychan R., Jones R. (2004). Production performance and meat quality of grazing lambs finished on red clover, lucerne or perennial ryegrass swards. Grass Forage Sci. 59, 345–356. doi: 10.1111/j.1365-2494.2004.00436.x
Fruet A. P. B., Trombetta F., Stefanello F. S., Speroni C. S., Donadel J. Z., De Souza A. N. M., et al. (2018). Effects of feeding legume-grass pasture and different concentrate levels on fatty acid profile, volatile compounds, and off-flavor of the M. longissimus thoracis. Meat Sci. 140, 112–118. doi: 10.1016/j.meatsci.2018.03.008
Frutos P., Hervás G., Natalello A., Luciano G., Fondevila M., Priolo A., et al. (2020). Ability of tannins to modulate ruminal lipid metabolism and milk and meat fatty acid profiles. Anim. Feed Sci. Technol. 269, 114623. doi: 10.1016/j.anifeedsci.2020.114623
Fusaro I., Cavallini D., Giammarco M., Manetta A. C., Martuscelli M., Mammi L. M. E., et al. (2021). Oxidative status of Marchigiana beef enriched in n-3 fatty acids and vitamin E, treated with a blend of oregano and rosemary essential oils. Front. Veterinary Sci. 8. doi: 10.3389/fvets.2021.662079
Gagaoua M., Alessandroni L., Das A., Lamri M., Bhattacharya D., Kumar Nanda P., et al. (2023). Intrinsic and extrinsic factors impacting fresh goat meat quality: an overview. Meat Technol. 64 (1), 20–40. doi: 10.18485/meattech.2023.64.1.3
Gallardo B., Gómez-Cortés P., Mantecón A. R., Juárez M., Manso T., de la Fuente M. A. (2014). Effects of olive and fish oil ca soaps in ewe diets on milk fat and muscle and subcutaneous tissue fatty-acid profiles of suckling lambs. Anim. (Cambridge England) 8, 1178–1190. doi: 10.1017/S1751731114000238
Gallardo B., Manca M. G., Mantecón A. R., Nudda A., Manso T. (2015). Effects of linseed oil and natural or synthetic vitamin E supplementation in lactating ewes' diets on meat fatty acid profile and lipid oxidation from their milk fed lambs. Meat Sci. 102, 79–89. doi: 10.1016/j.meatsci.2014.12.006
Gill R. K., Vanoverbeke D. L., Depenbusch B., Drouillard J. S., Dicostanzo A. (2008). Impact of beef cattle diets containing corn or sorghum distillers grains on beef color, fatty acid profiles, and sensory attributes. J. Anim. Sci. 86, 923–935. doi: 10.2527/jas.2007-0244
Girard M., Dohme-Meier F., Silacci P., Ampuero Kragten S., Kreuzer M., Bee G. (2016). Forage legumes rich in condensed tannins may increase n-3 fatty acid levels and sensory quality of lamb meat: Forage legumes and sensory quality of lamb meat. J. Sci. Food Agric. 96, 1923–1933. doi: 10.1002/jsfa.7298
Gobert M., Gruffat D., Habeanu M., Parafita E., Bauchart D., Durand D. (2010). Plant extracts combined with vitamin E in PUFA-rich diets of cull cows protect processed beef against lipid oxidation. Meat Sci. 85, 676–683. doi: 10.1016/j.meatsci.2010.03.024
Gómez-Cortés P., Galisteo O. O., Ramírez C. A., Blanco F. P., de la Fuente M. A., Sánchez N. N., et al. (2019). Intramuscular fatty acid profile of feedlot lambs fed concentrates with alternative ingredients. Anim. Production Sci. 59, 914–920. doi: 10.1071/AN17885
González-Ríos H., Dávila-Ramírez J. L., Peña-Ramos E. A., Valenzuela-Melendres M., Zamorano-García L., Islava-Lagarda T. Y., et al. (2016). Dietary supplementation of ferulic acid to steers under commercial feedlot feeding conditions improves meat quality and shelf life. Anim. Feed Sci. Technol. 222, 111–121. doi: 10.1016/j.anifeedsci.2016.10.011
Gruffat D., Durand D., Rivaroli D., Do Prado I. N., Prache S. (2020). Comparison of muscle fatty acid composition and lipid stability in lambs stall-fed or pasture-fed alfalfa with or without sainfoin pellet supplementation. Animal 14, 1093–1101. doi: 10.1017/S1751731119002507
Guo Q., Li T., Qu Y., Liang M., Ha Y., Zhang Y., et al. (2023). New research development on trans fatty acids in food: Biological effects, analytical methods, formation mechanism, and mitigating measures. Prog. Lipid Res. 89, 101199–101199. doi: 10.1016/j.plipres.2022.101199
Hajji H., Joy M., Ripoll G., Smeti S., Mekki I., Gahete F. M., et al. (2016). Meat physicochemical properties, fatty acid profile, lipid oxidation and sensory characteristics from three North African lamb breeds, as influenced by concentrate or pasture finishing diets. J. Food Composition Anal. 48, 102–110. doi: 10.1016/j.jfca.2016.02.011
Hampel V. S., Poli C. H. E. C., Joy M., Tontini J. F., DeVincenzi T., Pardos J. R. B., et al. (2021). Tropical grass and legume pastures may alter lamb meat physical and chemical characteristics. Trop. Anim. Health Production 53, 427–427. doi: 10.1007/s11250-021-02861-6
He M. L., Gibb D., McKinnon J. J., McAllister T. A. (2013). Effect of high dietary levels of canola meal on growth performance, carcass quality and meat fatty acid profiles of feedlot cattle. Can. J. Anim. Sci. 93, 269–280. doi: 10.4141/CJAS2012-090
Hennessy A. A., Kenny D. A., Byrne C. J., Childs S., Ross R. P., Devery R., et al. (2021). Fatty acid concentration of plasma, muscle, adipose and liver from beef heifers fed an encapsulated n-3 polyunsaturated fatty acid supplement. Anim. (Cambridge England) 15, 100039–100039. doi: 10.1016/j.animal.2020.100039
Hopkins D. L., Clayton E. H., Lamb T. A., Van De Ven R. J., Refshauge G., Kerr M. J., et al. (2014). The impact of supplementing lambs with algae on growth, meat traits and oxidative status. Meat Sci. 98, 135–141. doi: 10.1016/j.meatsci.2014.05.016
Huang Q., Wang. S., Yang X., Han X., Liu. Y., Khan N. A., et al. (2023). Effects of organic and inorganic selenium on selenium bioavailability, growth performance, antioxidant status and meat quality of a local beef cattle in China. Front. Veterinary Sci. 10. doi: 10.3389/fvets.2023.1171751
Hwang Y., Joo S. (2017). Fatty acid profiles, meat quality, and sensory palatability of grain-fed and grass-fed beef from hanwoo, american, and Australian crossbred cattle. Korean J. Food Sci. Anim. Resour. 37, 153–161. doi: 10.5851/kosfa.2017.37.2.153
Ianni A., Luca A. D., Martino C., Bennato F., Marone E., Grotta L., et al. (2019). Dietary supplementation of dried grape pomace increases the amount of linoleic acid in beef, reduces the lipid oxidation and modifies the volatile profile. Anim. (Basel) 9, 578. doi: 10.3390/ani9080578
James N. A., Berry B. W. (1997). Use of chevon in the development of low-fat meat products. J. Anim. Sci. 75, 571–577. doi: 10.2527/1997.752571x
Joy M., Ripoll G., Molino F., Dervishi E., Álvarez-Rodriguez J. (2012). Influence of the type of forage supplied to ewes in pre- and post-partum periods on the meat fatty acids of suckling lambs. Meat Sci. 90, 775–782. doi: 10.1016/j.meatsci.2011.11.013
Kafle D., Lee J. H., Min B. R., Kouakou B. (2021). Carcass and meat quality of goats supplemented with tannin-rich peanut skin. J. Agric. Food Res. 5, 100159. doi: 10.1016/j.jafr.2021.100159
Karami M., Alimon A. R., Goh Y. M. (2011). Effect of vitamin E, Andrographis paniculata and turmeric as dietary antioxidant supplementation on lipid and color stability of goat meat. Small Ruminant Res. 97, 67–71. doi: 10.1016/j.smallrumres.2011.02.005
Karami M., Ponnampalam E. N., Hopkins D. L. (2013). The effect of palm oil or canola oil on feedlot performance, plasma and tissue fatty acid profile and meat quality in goats. Meat Sci. 94, 165–169. doi: 10.1016/j.meatsci.2013.02.004
Karre L., Lopez K., Getty K. J. K. (2013). Natural antioxidants in meat and poultry products. Meat Sci. 94, 220–227. doi: 10.1016/j.meatsci.2013.01.007
Kaur N., Chugh V., Gupta A. K. (2014). Essential fatty acids as functional components of foods- a review. J. Food Sci. Technol. 51, 2289–2303. doi: 10.1007/s13197-012-0677-0
Kearns M., Jacquier J., Harrison S. M., Cama-Moncunill R., Boland T. M., Sheridan H., et al. (2023a). Effect of different botanically-diverse diets on the fatty acid profile, tocopherol content and oxidative stability of beef. J. Sci. Food Agric. 103, 4983–4992. doi: 10.1002/jsfa.12633
Kearns M., Ponnampalam E. N., Jacquier J. C., Grasso S., Boland T. M., Sheridan H., et al. (2023b). Can botanically-diverse pastures positively impact the nutritional and antioxidant composition of ruminant meat? – Invited review. Meat Sci. 197, 109055–109055. doi: 10.1016/j.meatsci.2022.109055
Keles G., Kocaman V., Ustundag A. O., Zungur A., Ozdogan M. (2018). Growth rate, carcass characteristics and meat quality of growing lambs fed buckwheat or maize silage. Asian-Australasian J. Anim. Sci. 31, 522–528. doi: 10.5713/ajas.17.0296
Kiani A., Gharoni M. H., Shariati R. (2017). Comparison of the fatty acid composition of the longissimus dorsi muscle of kids, lambs and calves produced under Iranian transhumant production system. Iranian J. Appl. Anim. Sci. 7, 437–443.
Kim H. J., Kim H. J., Jeon J., Nam K. C., Shim K. S., Jung J. H., et al. (2020). Comparison of the quality characteristics of chicken breast meat from conventional and animal welfare farms under refrigerated storage. Poultry Sci. 99, 1788–1796. doi: 10.1016/j.psj.2019.12.009
Kliem K. E., Thomson A. L., Crompton L. A., Givens D. I. (2018). Effect of selected plant species within biodiverse pasture on in vitro fatty acid biohydrogenation and tissue fatty acid composition of lamb. Anim. (Cambridge England) 12, 2415–2423. doi: 10.1017/S1751731118000265
Klopatek S. C., Xu Y., Yang X., Oltjen J. W., Vahmani P. (2022). Effects of multiple grass- and grain-fed production systems on beef fatty acid contents and their consumer health implications. ACS Food Sci. Technol. 2, 712–721. doi: 10.1021/acsfoodscitech.2c00021
Klurfeld D. M. (2018). What is the role of meat in a healthy diet? Anim. Front. 8, 5–10. doi: 10.1093/af/vfy009
Kotsampasi B., Christodoulou V., Zotos A., Liakopoulou-Kyriakides M., Goulas P., Petrotos K., et al. (2014). Effects of dietary pomegranate byproduct silage supplementation on performance, carcass characteristics and meat quality of growing lambs. Anim. Feed Sci. Technol. 197, 92–102. doi: 10.1016/j.anifeedsci.2014.09.003
Kurutas E. B. (2015). The importance of antioxidants which play the role in cellular response against oxidative/nitrosative stress: Current state. Nutr. J. 15, 71–71. doi: 10.1186/s12937-016-0186-5
Lauridsen C. (2019). From oxidative stress to inflammation: Redox balance and immune system. Poultry Sci. 98, 4240–4246. doi: 10.3382/ps/pey407
Lauridsen C., Matte J. J., Lessard M., Celi P., Litta G. (2021). Role of vitamins for gastro-intestinal functionality and health of pigs. Anim. Feed Sci. Technol. 273, 114823. doi: 10.1016/j.anifeedsci.2021.114823
Lee M. R. F., Evans P. R., Nute G. R., Richardson R. I., Scollan N. D. (2009). A comparison between red clover silage and grass silage feeding on fatty acid composition, meat stability and sensory quality of the M. longissimus muscle of dairy cull cows. Meat Sci. 81, 738–744. doi: 10.1016/j.meatsci.2008.11.016
Lee J. H., Kouakou B., Kannan G. (2008). Chemical composition and quality characteristics of chevon from goats fed three different post-weaning diets. Small Ruminant Res. 75, 177–184. doi: 10.1016/j.smallrumres.2007.10.003
Lee M. R. F., McAuliffe G. A., Tweed J. K. S., Griffith B. A., Morgan S. A., Rivero M. J., et al. (2021). Nutritional value of suckler beef from temperate pasture systems. Anim. (Cambridge England) 15, 100257–100257. doi: 10.1016/j.animal.2021.100257
Liotta L., Chiofalo V., Lo Presti V., Chiofalo B. (2020). Effect of production system on growth performances and meat traits of suckling messinese goat kids. Ital. J. Anim. Sci. 19, 245–252. doi: 10.1080/1828051X.2020.1726832
Liu Q., Lanari M. C., Schaefer D. M. (1995). A review of dietary vitamin E supplementation for improvement of beef quality. J. Anim. Sci. 73, 3131–3140. doi: 10.2527/1995.73103131x
Lourenço M., Van Ranst G., De Smet S., Raes K., Fievez V. (2007). Effect of grazing pastures with different botanical composition by lambs on rumen fatty acid metabolism and fatty acid pattern of longissimus muscle and subcutaneous fat. Anim. (Cambridge England) 1, 537–545. doi: 10.1017/S1751731107000316
Macari S., Graulet B., Andueza D., Prache S. (2017). Nitrogen stable isotope and carotenoid pigments signatures in the meat as tools to trace back the diet: Comparison between two sheep breeds. Small Ruminant Res. 153, 107–113. doi: 10.1016/j.smallrumres.2017.05.013
Mapiye C., Aalhus J. L., Turner T. D., Rolland D. C., Basarab J. A., Baron V. S., et al. (2013). Effects of feeding flaxseed or sunflower-seed in high-forage diets on beef production, quality and fatty acid composition. Meat Sci. 95, 98–109. doi: 10.1016/j.meatsci.2013.03.033
Mapiye C., Vahmani P., Mlambo V., Muchenje V., Dzama K., Hoffman L. C., et al. (2015). The trans-octadecenoic fatty acid profile of beef: Implications for global food and nutrition security. Food Res. Int. 76, 992–1000. doi: 10.1016/j.foodres.2015.05.001
Marti S., Realini C. E., Bach A., Pérez-Juan M., DeVant M. (2011). Effect of vitamin A restriction on performance and meat quality in finishing Holstein bulls and steers. Meat Sci. 89, 412–418. doi: 10.1016/j.meatsci.2011.05.003
Martins Flores D. R., da Fonseca P., Franco A., Schmitt J., José Tonetto C., Rosado Junior A. G., et al. (2021). Lambs fed with increasing levels of grape pomace silage: Effects on meat quality. Small Ruminant Res. 195, 106234. doi: 10.1016/j.smallrumres.2020.106234
Merayo M., Rizzo S. A., Rossetti L., Pighin D., Grigioni G. (2022). Effect of aging and retail display conditions on the color and oxidant/antioxidant status of beef from steers finished with DG-supplemented diets. Foods 11, 884. doi: 10.3390/foods11060884
Moloney A. P., McGettrick S., Dunne P. G., Shingfield K. J., Richardson R. I., Monahan F. J., et al. (2022). Supplementation with Sunflower/Fish oil-containing concentrates in a grass-based beef production system: Influence on fatty acid composition, gene expression, lipid and colour stability and sensory characteristics of longissimus muscle. Foods 11, 4061. doi: 10.3390/foods11244061
Moloney A. P., O'Riordan E. G., Schmidt O., Monahan F. J. (2018). The fatty acid profile and stable isotope ratios of C and N of muscle from cattle that grazed grass or grass/clover pastures before slaughter and their discriminatory potential. Irish J. Agric. Food Res. 57, 84–94. doi: 10.1515/ijafr-2018-0009
Montossi F., Font-i-Furnols M., del Campo M., San Julián R., Brito G., Sañudo C. (2013). Sustainable sheep production and consumer preference trends: Compatibilities, contradictions, and unresolved dilemmas. Meat Sci. 95, 772–789. doi: 10.1016/j.meatsci.2013.04.048
Moreno-Indias I., Sanchez-Macias D., Martinez-De La Puente J., Morales-Dela Nuez A., Hernandez-Castellano L. E., Castro N., et al. (2012). The effect of diet and DHA addition on the sensory quality of goat kid meat. Meat Sci. 90, 393–397. doi: 10.1016/j.meatsci.2011.08.004
Moure A., Cruz J. M., Franco D., Domiínguez J. M., Sineiro J., José Núñez M. A., et al. (2001). “Natural antioxidants from residual sources,” in Food Chemistry (Elsevier Ltd, Oxford). doi: 10.1016/S0308-8146(00)00223-5
Najafi M. H., Zeinoaldini S., Ganjkhanlou M., Mohammadi H., Hopkins D. L., Ponnampalam E. N. (2012). Performance, carcass traits, muscle fatty acid composition and meat sensory properties of male mahabadi goat kids fed palm oil, soybean oil or fish oil. Meat Sci. 92, 848–854. doi: 10.1016/j.meatsci.2012.07.012
Natalello A., Luciano G., Morbidini L., Valenti B., Pauselli M., Frutos P., et al. (2019). Effect of feeding pomegranate byproduct on fatty acid composition of ruminal digesta, liver, and muscle in lambs. J. Agric. Food Chem. 67, 4472–4482. doi: 10.1021/acs.jafc.9b00307
Noci F., Monahan F. J., French P., Moloney A. P. (2005). Fatty acid composition of muscle fat and subcutaneous adipose tissue of pasture-fed beef heifers: Influence of the duration of grazing. J. Anim. Sci. 83, 1167–1178. doi: 10.2527/2005.8351167x
Noci F., Monahan F. J., Moloney A. P. (2011). The fatty acid profile of muscle and adipose tissue of lambs fed camelina or linseed as oil or seeds. Animal 5, 134–147. doi: 10.1017/S1751731110001485
Nozière P., Graulet B., Lucas A., Martin B., Grolier P., Doreau M. (2006). Carotenoids for ruminants: From forages to dairy products. Anim. Feed Sci. Technol. 131, 418–450. doi: 10.1016/j.anifeedsci.2006.06.018
Nudda A., Battacone G., Bee G., Boe R., Castanares N., Lovicu M., et al. (2015). Effect of linseed supplementation of the gestation and lactation diets of dairy ewes on the growth performance and the intramuscular fatty acid composition of their lambs. Anim. (Cambridge England) 9, 800–809. doi: 10.1017/S175173111400305X
Nuernberg K., Nuernberg G., Ender K., Dannenberger D., Schabbel W., Grumbach S., et al. (2005). Effect of grass vs. concentrate feeding on the fatty acid profile of different fat depots in lambs. Eur. J. Lipid Sci. Technol. 107, 737–745. doi: 10.1002/ejlt.200501141
OECD/FAO (2021). “OECD-FAO agricultural outlook,” in OECD agriculture statistics (database). Paris: OECD Publishing. doi: 10.1787/agr-outl-dataen
Ornaghi M. G., Guerrero A., Vital A. C. P., De Souza K. A., Passetti R. A. C., Mottin C., et al. (2020). Improvements in the quality of meat from beef cattle fed natural additives. Meat Sci. 163, 108059. doi: 10.1016/j.meatsci.2020.108059
Orzuna-Orzuna J. F., Dorantes-Iturbide G., LarA-Bueno A., Chay-Canul A. J., Miranda-Romero L. A., Mendoza-Martínez G. D. (2023). Meta-analysis of flavonoids use into beef and dairy cattle diet: Performance, antioxidant status, ruminal fermentation, meat quality, and milk composition. Front. Veterinary Sci. 10. doi: 10.3389/fvets.2023.1134925
Parvar R., Ghoorchi T., Shargh M. S. (2017). Influence of dietary oils on performance, blood metabolites, purine derivatives, cellulase activity and muscle fatty acid composition in fattening lambs. Small Ruminant Res. 150, 22–29. doi: 10.1016/j.smallrumres.2017.03.004
Pereira P. M. d. C. C, Vicente A. F. d. R. B. (2013). Meat nutritional composition and nutritive role in the human diet. Meat Sci. 93, 586–592. doi: 10.1016/j.meatsci.2012.09.018
Petcu C. D., Mihai O. D., Tăpăloagă D., Gheorghe-Irimia R. A., Pogurschi E. N., Militaru M., et al. (2023). Effects of plant-based antioxidants in animal diets and meat products: A Review. Foods 12, 1334. doi: 10.3390/foods12061334
Pimentel P. R. S., Pellegrini C. B., Lanna D. P. D., Brant L. M. S., Ribeiro C. V. D. M., Silva T. M., et al. (2021). Effects of Acacia mearnsii extract as a condensed-tannin source on animal performance, carcass yield and meat quality in goats. Anim. Feed Sci. Technol. 271. doi: 10.1016/j.anifeedsci.2020.114733
Ponnampalam E. N., Burnett V. F., Norng S., Hopkins D. L., Plozza T., Jacobs J. L. (2016). Muscle antioxidant (vitamin E) and major fatty acid groups, lipid oxidation and retail colour of meat from lambs fed a roughage based diet with flaxseed or algae. Meat Sci. 111, 154–160. doi: 10.1016/j.meatsci.2015.09.007
Ponnampalam E. N., Burnett V. F., Norng S., Warner R. D., Jacobs J. L. (2012). Vitamin e and fatty acid content of lamb meat from perennial pasture or annual pasture systems with supplements. Anim. Production Sci. 52, 255–262. doi: 10.1071/AN11054
Ponnampalam E. N. (1999). Nutritional modification of muscle long chain omega-3 fatty acids in lamb: effects on growth and composition and quality of meat. [master's thesis]. Melbourne, Australia: The University of Melbourne. 1999.
Ponnampalam E. N., Butler K. L., Muir S. K., Plozza T. E., Kerr M. G., Brown W. G., et al. (2021a). Lipid oxidation and colour stability of lamb and yearling meat (Muscle longissimus lumborum) from sheep supplemented with camelina-based diets after short, medium, and long-term storage. Antioxidants 10, 1–19. doi: 10.3390/antiox10020166
Ponnampalam E. N., Holman B. W. B. (2022). “Sustainable animal production and meat processing,” in Lawrie´s meat science textbook– edition 9. Ed. Toldrá F. (Cambridge, United States: Elsevier Publisher of Scientific Books and Journals).
Ponnampalam E. N., Kiani A., Santhiravel S., Holman B. W. B., Lauridsen C., Dunshea F. R. (2022). The importance of dietary antioxidants on oxidative stress, meat and milk production, and their preservative aspects in farm animals: antioxidant action, animal health, and product quality-Invited Review. Animals 12, 3279. doi: 10.3390/ani12233279
Ponnampalam E. N., Lewandowski P. A., Fahri F. T., Burnett V. F., Dunshea F. R., Plozza T., et al. (2015). Forms of n-3 (ALA, C18:3n-3 or DHA, C22:6n-3) Fatty Acids Affect Carcass Yield, Blood Lipids, Muscle n-3 Fatty Acids and Liver Gene Expression in Lambs. Lipids 50, 1133–1143. doi: 10.1007/s11745-015-4070-4
Ponnampalam E. N., Plozza T., Kerr M. G., Linden N., Mitchell M., Bekhit A. E. D. A., et al. (2017). Interaction of diet and long ageing period on lipid oxidation and colour stability of lamb meat. Meat Sci. 129, 43–49. doi: 10.1016/j.meatsci.2017.02.008
Ponnampalam E. N., Sinclair A. J., Holman B. W. B. (2021b). The sources, synthesis and biological actions of omega-3 and omega-6 fatty acids in red meat: An overview. Foods 10, 1358. doi: 10.3390/foods10061358
Prache S., Adamiec C., Astruc T., Baéza-Campone E., Bouillot P. E., Clinquart A., et al. (2022a). Review: Quality of animal-source foods. Animal 16, 100376–100376. doi: 10.1016/j.animal.2021.100376
Prache S., Schreurs N., Guillier L. (2022b). Review: Factors affecting sheep carcass and meat quality attributes. Animal 16, 100330–100330. doi: 10.1016/j.animal.2021.100330
Ritchie H., Rosado P., Roser M. (2019). Meat and dairy production. Available at: https://ourworldindata.org/meat-production.
Rivaroli D. C., Guerrero A., Velandia Valero M., Zawadzki F., Eiras C. E., Campo M. D. M., et al. (2016). Effect of essential oils on meat and fat qualities of crossbred young bulls finished in feedlots. Meat Sci. 121, 278–284. doi: 10.1016/j.meatsci.2016.06.017
Rivaroli D., Prunier A., Meteau K., do Prado I. N., Prache S. (2019). Tannin-rich sainfoin pellet supplementation reduces fat volatile indoles content and delays digestive parasitism in lambs grazing alfalfa. Animal 13, 1883–1890. doi: 10.1017/S1751731118003543
Rodríguez R., Alomar D., Morales R. (2020). Milk and meat fatty acids from sheep fed a plantain-chicory mixture or a grass-based permanent sward. Anim. (Cambridge England) 14, 1102–1109. doi: 10.1017/S1751731119002611
Rossi C. S., Compiani R., Baldi G., Bernardi C. E. M., Muraro M., Marden J. P., et al. (2015). The effect of different selenium sources during the finishing phase on beef quality. J. Anim. Feed Sci. 24, 93–99. doi: 10.22358/jafs/65633/2015
Ryan S. M., Unruh J. A., Corrigan M. E., Drouillard J. S., Seyfert M. (2007). Effects of concentrate level on carcass traits of Boer crossbred goats. Small Ruminant Res. 73, 67–76. doi: 10.1016/j.smallrumres.2006.11.004
Salami S. A., Guinguina A., Agboola J. O., Omede A. A., Agbonlahor E. M., Tayyab U. (2016). Review: In vivo and postmortem effects of feed antioxidants in livestock: A review of the implications on authorization of antioxidant feed additives. Anim. (Cambridge England) 10, 1375–1390. doi: 10.1017/S1751731115002967
Salami S. A., Luciano G., O'Grady M. N., Biondi L., Newbold C. J., Kerry J. P., et al. (2019). Sustainability of feeding plant by-products: A review of the implications for ruminant meat production. Anim. Feed Sci. Technol. 251, 37–55. doi: 10.1016/j.anifeedsci.2019.02.006
Scerra M., Luciano G., Caparra P., Foti F., Cilione C., Giorgi A., et al. (2011). Influence of stall finishing duration of Italian Merino lambs raised on pasture on intramuscular fatty acid composition. Meat Sci. 89, 238–242. doi: 10.1016/j.meatsci.2011.04.012
Schwingshackl L., Schwedhelm C., Hoffmann G., Lampousi A., Knüppel S., Iqbal K., et al. (2017). Food groups and risk of all-cause mortality: A systematic review and meta-analysis of prospective studies. The American. J. Clin. Nutr. 105, 1462–1473. doi: 10.3945/ajcn.117.153148
Scollan N. D., Dannenberger D., Schreurs N. M., Lane G. A., Tavendale M. H., Barry T. N., et al. (2008). Pastoral flavour in meat products from ruminants fed fresh forages and its amelioration by forage condensed tannins. Anim. Feed Sci. Technol. 146, 193–221. doi: 10.1016/j.anifeedsci.2008.03.002
Scollan N., Hocquette J.-F. O., Nuernberg K., Dannenberger D., Richardson I., Moloney A. (2006). Innovations in beef production systems that enhance the nutritional and health value of beef lipids and their relationship with meat quality. Meat Sci. 74, 17–33. doi: 10.1016/j.meatsci.2006.05.002
Semwogerere F., Chikwanha O. C., Katiyatiya C. L. F., Marufu M. C., Mapiye C. (2023). Health value and keeping quality of chevon from goats fed finisher diets containing hemp (Cannabis sativa L.) seed cake. Meat Sci. 198, 109114–109114. doi: 10.1016/j.meatsci.2023.109114
Shabtay A., Eitam H., Tadmor Y., Orlov A., Meir A., Weinberg P., et al. (2008). Nutritive and antioxidative potential of fresh and stored pomegranate industrial byproduct as a novel beef cattle feed. J. Agric. Food Chem. 56, 10063–10070. doi: 10.1021/jf8016095
Siphambili S., Moloney A. P., O'riordan E. G., Mcgee M., Harrison S. M., Monahan F. J. (2022). Partial substitution of barley with maize meal or flaked meal in bovine diets: Effects on fatty acid and α-tocopherol concentration and the oxidative stability of beef under simulated retail display. Anim. Production Sci. 62, 182–190. doi: 10.1071/AN20627
Skřivanová E., Marounek M., De Smet. S., Raes K. (2007). Influence of dietary selenium and vitamin E on quality of veal. Meat Sci. 76, 495–500. doi: 10.1016/j.meatsci.2007.01.003
Suman S. P., Hunt M. C., Nair M. N., Rentfrow G. (2014). Improving beef color stability: practical strategies and underlying mechanisms. Meat Sci. 98, 490–504. doi: 10.1016/j.meatsci.2014.06.032
Swanson K. C. (2019). “Small intestinal anatomy, physiology, and digestion in ruminants,” in Reference Module in Food Science (Elsevier, Amsterdam, The Netherlands).
Tabke M. C., Sarturi J. O., Galyean M. L., Trojan S. J., Brooks J. C., Johnson B. J., et al. (2017). Effects of tannic acid on growth performance, carcass characteristics, digestibility, nitrogen volatilization, and meat lipid oxidation of steers fed steam-flaked corn–based finishing diets. J. Anim. Sci. 95, 5124–5136. doi: 10.2527/jas2017.1464
Tayengwa T., Chikwanha O. C., Gouws P., Dugan M. E. R., Mutsvangwa T., Mapiye C. (2020). Dietary citrus pulp and grape pomace as potential natural preservatives for extending beef shelf life. Meat Sci. 162, 108029. doi: 10.1016/j.meatsci.2019.108029
Urrutia O., Soret B., Insausti K., Mendizabal J. A., Purroy A., Arana A. (2015). The effects of linseed or chia seed dietary supplementation on adipose tissue development, fatty acid composition, and lipogenic gene expression in lambs. Small Ruminant Res. 123, 204–211. doi: 10.1016/j.smallrumres.2014.12.008
Uushona T., Chikwanha O. C., Katiyatiya C. L. F., Strydom P. E., Mapiye C. (2023). Fatty acid and oxidative shelf-life profiles of meat from lambs fed finisher diets containing Acacia mearnsii leaf-meal. Meat Sci. 201, 109190–109190. doi: 10.1016/j.meatsci.2023.109190
Vahmani P., Mapiye C., Prieto N., Rolland D. C., McAllister T. A., Aalhus J. L., et al. (2015). The scope for manipulating the polyunsaturated fatty acid content of beef: A review. J. Anim. Sci. Biotechnol. 6, 29–29. doi: 10.1186/s40104-015-0026-z
Vahmani P., Ponnampalam E. N., Kraft J., Mapiye C., Bermingham E. N., Watkins P. J., et al. (2020). Bioactivity and health effects of ruminant meat lipids. Invited Review. Meat Sci. 165, 108114–108114. doi: 10.1016/j.meatsci.2020.108114
Verneque B. J. F., MaChado A. M., De Abreu Silva L., Lopes A. C. S., Duarte C. K. (2022). Ruminant and industrial trans-fatty acids consumption and cardiometabolic risk markers: A systematic review. Crit. Rev. Food Sci. Nutr. 62, 2050–2060. doi: 10.1080/10408398.2020.1836471
Wang X., Martin G. B., Wen Q., Liu S., Li Y., Shi B., et al. (2020). Palm oil protects α-linolenic acid from rumen biohydrogenation and muscle oxidation in cashmere goat kids. J. Anim. Sci. Biotechnol. 11, 100–100. doi: 10.1186/s40104-020-00502-w
WCRF, AICR, CUP Expert report (2018). Meat, fish and dairy products and the risk of cancer (London, UK: WCRF International).
Webb E. C., Casey N. H., Simela L. (2005). Goat meat quality. Small Ruminant Res. 60, 153–166. doi: 10.1016/j.smallrumres.2005.06.009
Willems H., Kreuzer M., Leiber F. (2014). Alpha-linolenic and linoleic acid in meat and adipose tissue of grazing lambs differ among alpine pasture types with contrasting plant species and phenolic compound composition. Small Ruminant Res. 116, 153–164. doi: 10.1016/j.smallrumres.2013.11.002
Williams M. S., Mandell I. B., Wood K. M., Bohrer B. M. (2022). The effects of feeding benzoic acid and/or active dry yeast (Saccharomyces cerevisiae) on fatty acid composition, sensory attributes, and retail shelf-life of beef longissimus thoracis. Trans. Anim. Sci. 7, 161. doi: 10.1093/tas/txac161
Wrona M., Korytowski W., Różanowska M., Sarna T., Truscott T. G. (2003). Cooperation of antioxidants in protection against photosensitized oxidation. Free Radical Biol. Med. 35, 1319–1329. doi: 10.1016/j.freeradbiomed.2003.07.005
Zehiroglu C., Ozturk Sarikaya S. B. (2019). The importance of antioxidants and place in today’s scientific and technological studies. J. Food Sci. Technol. 56, 4757–4774. doi: 10.1007/s13197-019-03952-x
Keywords: red meat, dietary means, forages, phytonutrients, conjugated linoleic acid, omega-3 fatty acids, antioxidants, muscle fortification
Citation: Ponnampalam EN, Kearns M, Kiani A, Santhiravel S, Vahmani P, Prache S, Monahan FJ and Mapiye C (2024) Enrichment of ruminant meats with health enhancing fatty acids and antioxidants: feed-based effects on nutritional value and human health aspects – invited review. Front. Anim. Sci. 5:1329346. doi: 10.3389/fanim.2024.1329346
Received: 28 October 2023; Accepted: 02 February 2024;
Published: 01 March 2024.
Edited by:
Ian Givens, University of Reading, United KingdomReviewed by:
Nigel Scollan, Queen’s University Belfast, United KingdomSandra Sofia Quinteiro Rodrigues, Centro de Investigação de Montanha (CIMO), Portugal
Copyright © 2024 Ponnampalam, Kearns, Kiani, Santhiravel, Vahmani, Prache, Monahan and Mapiye. This is an open-access article distributed under the terms of the Creative Commons Attribution License (CC BY). The use, distribution or reproduction in other forums is permitted, provided the original author(s) and the copyright owner(s) are credited and that the original publication in this journal is cited, in accordance with accepted academic practice. No use, distribution or reproduction is permitted which does not comply with these terms.
*Correspondence: Eric N. Ponnampalam, ZXBvbm5hbXBhbGFtQHVuaW1lbGIuZWR1LmF1; Michelle Kearns, bWljaGVsbGUua2Vhcm5zQHVjZGNvbm5lY3QuaWU=; Cletos Mapiye, Y21hcGl5ZUBzdW4uYWMuemE=