- 1Feeds and Nutrition Research Program, Holetta Agricultural Research Center, Holetta, Ethiopia
- 2Ethiopian Institute of Agricultural Research, Livestock Research Directorate, Addis Ababa, Ethiopia
- 3Feed the Future Innovation Lab for Livestock Systems, University of Florida, Gainesville, FL, United States
- 4Department of Animal, Rangeland and Wildlife Sciences, Mekelle University, Mekelle, Ethiopia
- 5Department of Animal Sciences and Food Systems Institute, University of Florida, Institute of Food and Agricultural Science (IFAS), Gainesville, FL, United States
Introduction: Maralfalfa grass (Pennisetum spp.) is known for its high dry matter productivity and nutritive value. However, information on agronomic management practices to improve yield and nutritive value in Ethiopia is sparse.
Materials and method: The experiment consisted of 7 plant densities (33,333 [75 cm × 40 cm]; 26,667[75 cm × 50 cm]; 25,000 [100 cm × 40 cm]; 22,222 [75 cm × 60 cm]; 20,000 [100 cm × 50 cm]; 16,667 [100 cm × 60 cm]; and 13,333 [125 cm × 60 cm] plants per hectare, and arranged in randomized complete block design with three replications.
Results: The results indicated that the year had a significant (P<0.001) effect on measured traits. Plant densities had no significant (P>0.05) effect on the number of nodes and leaves per plant, leaf length, and basal diameters. The number of tillers per plant varied significantly among plant densities only in the first year at the 1st harvest. Plant height in the first year was significantly (P<0.01) greater than in the second year.
Discussion: Annual dry matter production and annual crude protein production of Maralfalfa grass were not significantly affected by plant density. Nutritive value parameters (CP, Ash, NDF, ADF, ADL, and IVDMD) were not significantly (P > 0.05) influenced by plant density. Using a lower plant density could reduce the amount of planting material, transport, and labor costs.
Conclusion: However, further studies on Maralfalfa grass should be conducted in multi-locations of Ethiopia both under rain-fed and irrigated conditions with various agronomic practices.
1 Introduction
Maralfalfa grass (Pennisetum spp.) is a perennial tropical grass that belongs to the Poaceae family. It is a hybrid between napier grass (Pennisetum purpureum) and pearl millet (Pennisetum glaucum) with 2n = 3x = 21 chromosomes (Hanna et al., 1984; Clavero and Razz, 2009). In different studies, maralfalfa is referred to as Pennisetum spp. (Ramirez et al., 2006), with the genotype of Pennisetum purpureum (Marquez et al., 2007) and the giant king grass (Geren and Kavut, 2015). Maralfalfa grass can be grown under a wide range of ecological conditions up to 3,000 m above sea level. Maralfalfa can be grown on a wide range of soil types, but it grows best in deep, well-drained, friable loams with a pH of 4.5 8.2 (Nyambati et al., 2010). It is propagated using stem cuttings, root splits, or rhizomes (Singh et al., 2013). The species is widely cultivated in numerous countries in Latin America (Correa, 2006), sub-tropical regions of Asia (Karforma, 2018), Southern Europe (Clavero and Razz, 2009), and Africa (Obok et al., 2012).
Maralfalfa has a great potential as a forage for ruminant animals, as it has a high level of fodder production and provides 40–60 tons of dry matter ha−1 year−1 (Bolonio et al., 2015). Moreover, due to its fast growth rate, intake potential, and nutritive value, it is the best option to increase feed availability for livestock production (Ramirez et al., 2006; Bolonio et al., 2015). Maralfalfa can reach up to 3–4 m in height and has an acceptable nutritional content for animal feed. It is also tolerant to heat and flooding (Correa et al., 2004). Most importantly, maralfalfa has a 14.4% crude protein (CP) content, which is high for grasses but lower than those of forage legumes, such as alfalfa, which have a CP content ranging from 15% to 22% (Scholtz, 2008).
Studies by Clavero and Rass (2009) and Marquez et al. (2007) indicated that due to its high biomass and nutritive value, maralfalfa grass could provide a considerable amount of quality feed for ruminant livestock producers. Maralfalfa grass can be cut and fed in several forms, such as chopped fresh, without chopping, made into silage, and dried and baled into hay (Santos et al., 2013; Geren and Kavut, 2015). It is best used in a “cut-and-carry” system directly or mixed with other legume forages to achieve the necessary daily nutrient requirement of the animal (Criscioni et al., 2016). Dry matter yield varies depending on genotypes, edaphic and climatic factors, and management practices (Malley et al., 1996). The studies performed by Palacios-Diaz et al. (2013) and Clavero and Razz (2009) suggested that the nutritive value of maralfalfa grass is influenced by management practices.
In Ethiopia, this species was recently introduced from Spain and tested under different agro-ecologies in the country. Then, it was registered as a variety by the Ministry of Agriculture (MOA, 2018). However, despite its introduction and registration, the grass is not well used by livestock producers. Moreover, no detailed studies have been made on the agronomic requirements of maralfalfa grass in different agro-ecological regions of the country. Because of its potential contribution to livestock feed, an understanding of the effects of various management practices on the yield and nutritive value of maralfalfa grass is very important.
Using the right planting density is an important factor in maximizing the herbage yield of Pennisetum species (Danalatos et al., 2007; Tessema, 2008). Plant density is one of the important agronomic factors affecting the yield and quality of forage crops (Tessema, 2008; Ansah et al., 2010; Palacios-Diaz et al., 2013). Currently, the spacing often used for napier grass in Ethiopia is 100-cm inter-row and 50-cm intra-row spacing. Owing to their morphological and genetic differences, understanding the effect of plant density on growth, development, yield, and forage quality will allow for improving management and benefits from potential forage crops such as maralfalfa grass. Therefore, we conducted this study in the central highlands of Ethiopia with the objective of determining the optimal plant densities of maralfalfa grass for dry matter yield (DMY) and nutritive value.
2 Materials and methods
2.1 Description of the study site
The study was performed at Holetta Agricultural Research Center, located in the central highlands of Ethiopia. The center is located at an elevation of 2,400 m above sea level with the coordinates 9° 3′ 47.9′N and 38° 30′ 24.9′E. The long-term (30 years) mean annual rainfall of the area is 1,055 mm, with a bimodal rainfall distribution with the majority (70%) falling in the periods from June to September, whereas the remaining (30%) falls from February to April (EIAR, 2005). The area has an average relative humidity of 60% and mean maximum and minimum temperatures of 22.2°C and 6.1°C, respectively. The weather data during the experimental periods (2019 and 2020) at the Holetta experimental station are indicated in Figure 1. Compared with the long-term records, both 2019 and 2020 had greater annual rainfall with means of 1,344 mm and 1,265 mm, respectively. Comparing the single months of the experimental years, the greatest rainfall was recorded in August with 356 mm and 334 mm in 2019 and 2020, respectively. The mean monthly maximum temperature in April was 17.8°C and 17.1°C for 2019 and 2020, respectively. The mean monthly minimum temperature in October and December was 13.2°C in 2019 and 2020. However, no major differences were observed between the experimental years in mean annual temperatures. The farming area is characterized by a mixed crop–livestock production system.
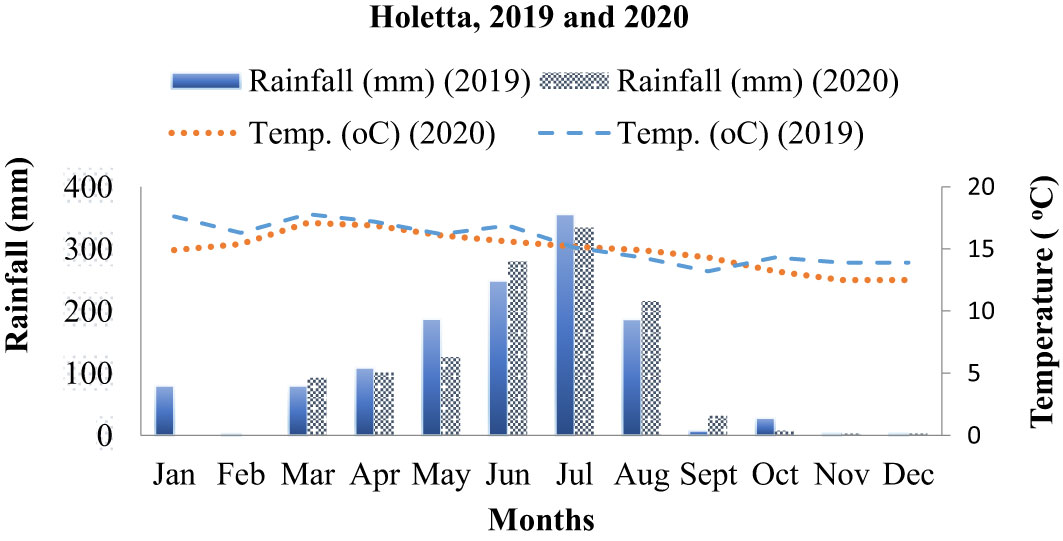
Figure 1 Mean monthly rainfall and temperature during 2019 and 2020 in Holetta. Source: Holetta Agricultural Research Center Metrological data records.
The soil of the area is mainly nitisol with an acidic pH of 4.9, an organic carbon content of 1.8%, total nitrogen of 0.18%, and available phosphorus of 5.6 parts per million (ppm), and with different proportions of clay, sand, and silt (Table 1).
2.2 Experimental treatments, design, and forage establishment
The treatments consisted of seven planting densities, as indicated in Table 2. The experiment was laid out as a randomized complete block design with three replicates, in a total of 21 3.0 m × 7.5 m experimental units spaced 1 m and 1.5 m apart between plots and blocks, respectively. Maralfalfa grass, which was registered as a variety by the Ministry of Agriculture, was planted under rain-fed conditions in mid-July (13 July 2018) in a well-prepared experimental field. We planted the grass in its vegetative form using root splits (Singh et al., 2013). We applied phosphorus fertilizer at the rate of 100 kg/ha to all plots at planting in the form of diammonium phosphate [DAP: 18% N, 20% P, and 1.5% S Urea (46% N)] fertilizer at the rate of 109 kg ha−1. This was applied in split applications, with one-third applied during the short rainy season and the remaining two-thirds at the beginning of the main rainy season at every harvest. Uniform trial management to all treatments, such as hand weeding and hoeing between rows, was carried out during establishment and after every harvest of the grass to facilitate regrowth.
2.3 Data collection, sampling, and measurements
Experimental data were taken for each treatment on morphological, agronomic, and nutritional quality traits. The survival rate and percentage of plot cover were observed at 8 weeks to assess the establishment performance. The number of nodes per plant (NNPP), number of leaves per plant (NLPP), leaf length (LL), number of tillers per plant (NTPP), and basal diameter (BD) were recorded on 10 randomly taken samples plants and their means were recorded for all treatments. The leaf-to-stem ratio (LSR) was measured on five sample plants and partitioned into leaf and stem fractions to determine the ratio of the dry weight of the leaves to the weight of the stems. The plant height (PH) was measured from 10 randomly selected plants in each plot from the ground to the tip of the plant, whereas leaf length (LL) records of flag leaf length were taken from the bottom, middle, and top of the sample plants and their means were used for the analysis (Aklilu and Alemayehu, 2007). For DMY determination, whole plants from each plot size of 22.5 m2 were cut at about 10 cm above ground manually using a sickle, and the fresh yield was measured using a spring balance. A 500 g fresh weight from each plot was taken and chopped into small sizes to facilitate drying. The samples were dried using an oven at 65°C for 72 h (Getnet and Ledin, 2001). The DMY was computed from the samples in each plot. CP yield (CPY) was computed using the formula: CPY (t ha−1) = CP% × DMY divided by 100.
2.3.1 Chemical analysis
Chemical analyses were carried out at the International Livestock Research Institute (ILRI) feed nutrition laboratory. The dried samples were ground in a mill to pass through a 1-mm sieve size used for laboratory analyses. The ground samples were dried overnight at 65°C in an oven to constant moisture. The DM, ash, and organic matter contents of the feed samples were determined following the procedure of AOAC (1990). The total ash content was determined by combusting the samples in a muffle furnace at 550°C for 6 h. The N content of the samples was determined according to the micro-Kjeldahl method AOAC (1990) and the percentage of N was multiplied by 6.25 to get the total CP content. Neutral detergent fiber (NDF), acid detergent fiber (ADF), and acid detergent lignin (ADL) were determined according to the procedure of Van Soest and Robertson (1985). In vitro dry matter digestibility (IVDMD) was made according to Tilley and Terry (1963).
2.4 Statistical analysis
The data were subjected to analysis of variance using SAS software (SAS, 2011). Prior to the analysis we tested the homogeneity of error variances using Bartlett (1947) test. Least square means (ls-means) were calculated, and the significance level was determined as a p-value < 0.05. The following statistical model (Equation 1) was used:
where Yijk is the response variables, μ is the overall mean, Ti is the effect due to plant density ith, Bk is the effect due to block kth, Yj is the effect due to the year (j is 2019 and 2020), (TY)ij is the effect due to the interaction effect between ith treatment and jth year, and eijk is the random error.
3 Results
3.1 Survival rate and plot cover
The survival rate and plot cover of the maralfalfa grass planted in different plant densities are shown in Figure 2. There was no statistical difference (p > 0.05) among the plant densities for survival rate or plot cover of the grass. The survival rate ranged from 90% to 100%, and plot cover ranged from 46% to 68%.
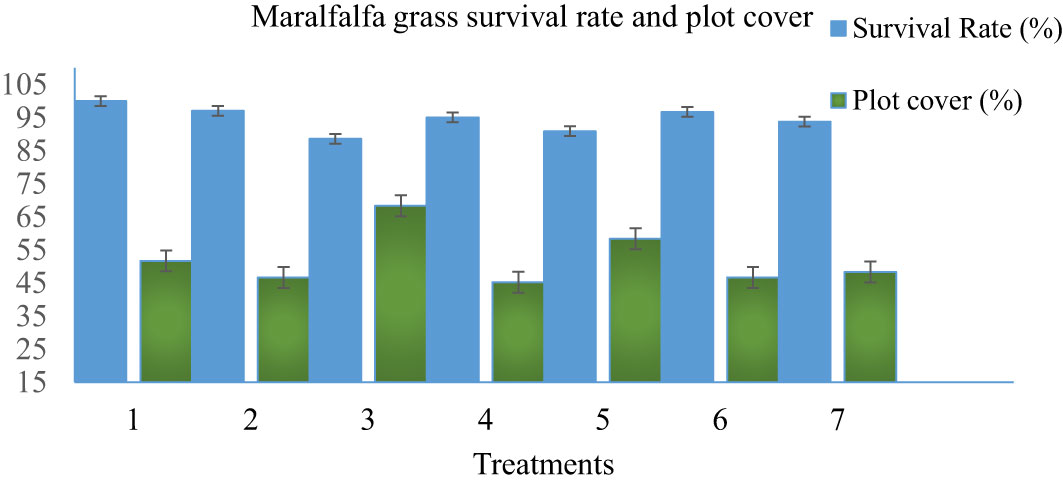
Figure 2 Survival rate and plot cover percentages of maralfalfa grass planted under different plant densities observed 8 weeks after planting.
3.2 Effects of planting density, year, and their interactions
The mean square for planting density over the years and their interactions with the measured morphological traits are indicated in Table 3. The measured agronomic characteristics were not significantly influenced by planting densities. Similarly, there was no significant (p > 0.05) interaction effect between planting density and year. On the contrary, PH, NTPP, total DMY, and crude protein yield (CPY) were significantly (p < 0.001) affected by the year.
3.3 Year effects on yield and yield components
The overall means of the NTPP, PH, annual DMY, and annual CPY of maralfalfa grass planted at different plant densities during 2019 and 2020 are shown in Table 4. The NTPP, PH, DMY, and CPY were highly different (p < 0.01) across the years. The means of NTPP recorded in year 2 increased by 89.74% compared with those of year 1. The DMY and CPY differed significantly (p < 0.001), and the DMY and CPY in the second year (2020) increased by 65% and 66% compared with those of the first year (2019), respectively.
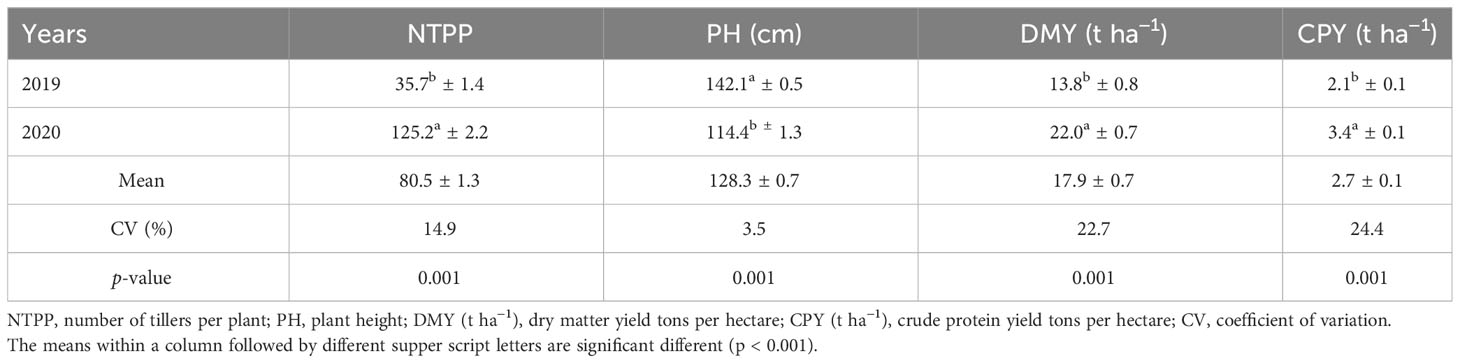
Table 4 Number of tillers per plant, plant height, annual dry matter yield, and annual crude protein yield of maralfalfa grass in 2019 and 2020.
3.4 Planting density effects on the morphological characteristics of maralfafa grass
The morphological characteristics of maralfalfa grass under the different planting densities are shown in Table 5. The planting densities had no significant (p > 0.05) effect on the NNPP, the NLPP, LL, BD, and LSR of maralfalfa grass.
3.5 Plant height at harvest and the number of tillers per plant
The mean PH and mean NTPP of maralfalfa grass under seven planting densities in 2019 and 2020 are indicated in Table 6. There was no significant difference (p > 0.05) among planting densities for plant height in the establishment year, while PH was significantly (p < 0.05) affected by planting densities in the following year. Accordingly, the tallest mean PH was recorded in T7 (125 cm × 60 cm), followed by T6 (100 cm × 60 cm) and T5 (100 cm × 50 cm). The lowest plant height was recorded in T4, but was statistically similar among T1 (75 cm × 40 cm), T2 (75 × 50 cm), and T2 (75 × 60 cm). However, for the 2019 and 2020 cropping seasons, the overall mean PH was not affected (p > 0.05) by planting density. The NTPP increased with the decreasing plant density, and this was pronounced only in the establishment year.
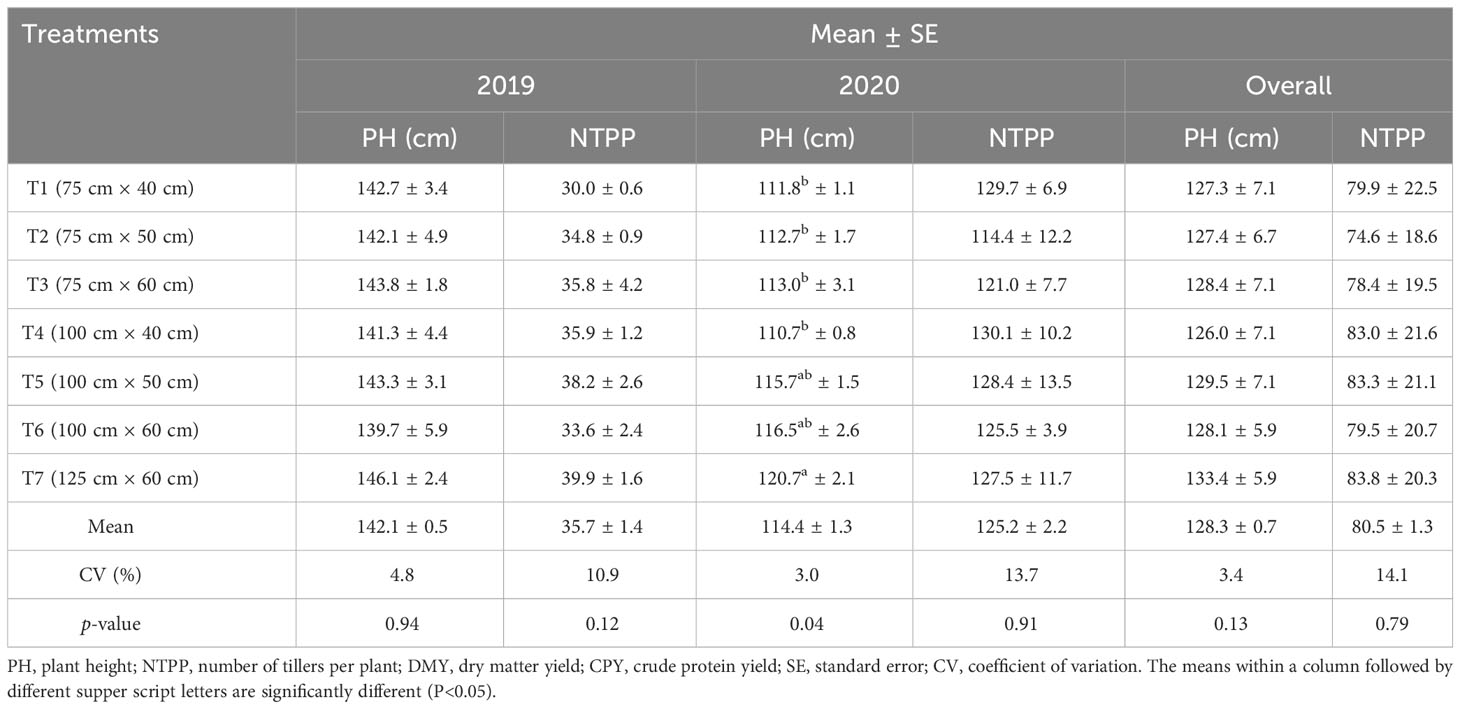
Table 6 Plant height and number of tillers per plant (mean ± SE) of maralfalfa grass under seven planting densities during the 2019 and 2020 cropping years.
3.6 Dry matter and crude protein yields
The DMYs and CPYs showed insignificant (p > 0.05) differences among planting densities in both years (Table 7). The mean annual DMY in the establishment year under different planting densities was 13.8 t ha−1 ± 0.8 t ha−1, whereas in the annual cumulative yield in the second-year harvest achieved 22.0 t ha−1 ± 0.7 t ha−1. The overall mean of the DMY obtained in 2019 and 2020 under different planting densities was 17.9 t ha−1 ± 0.7 t ha−1. The greater competition associated with greater planting densities did not result in lower dry matter production than lower planting densities. The CPY did not vary significantly among the different planting densities. The mean CPY in the first year was 2.1 t ha−1 ± 0.1 t ha−1, whereas in the following year it was 3.4 t ha−1 ± 0.1 t ha−1. The overall annual mean of CPY attained over the 2019 and 2020 seasons under different planting densities was 2.7 t ha−1 ± 0.1 t ha−1.
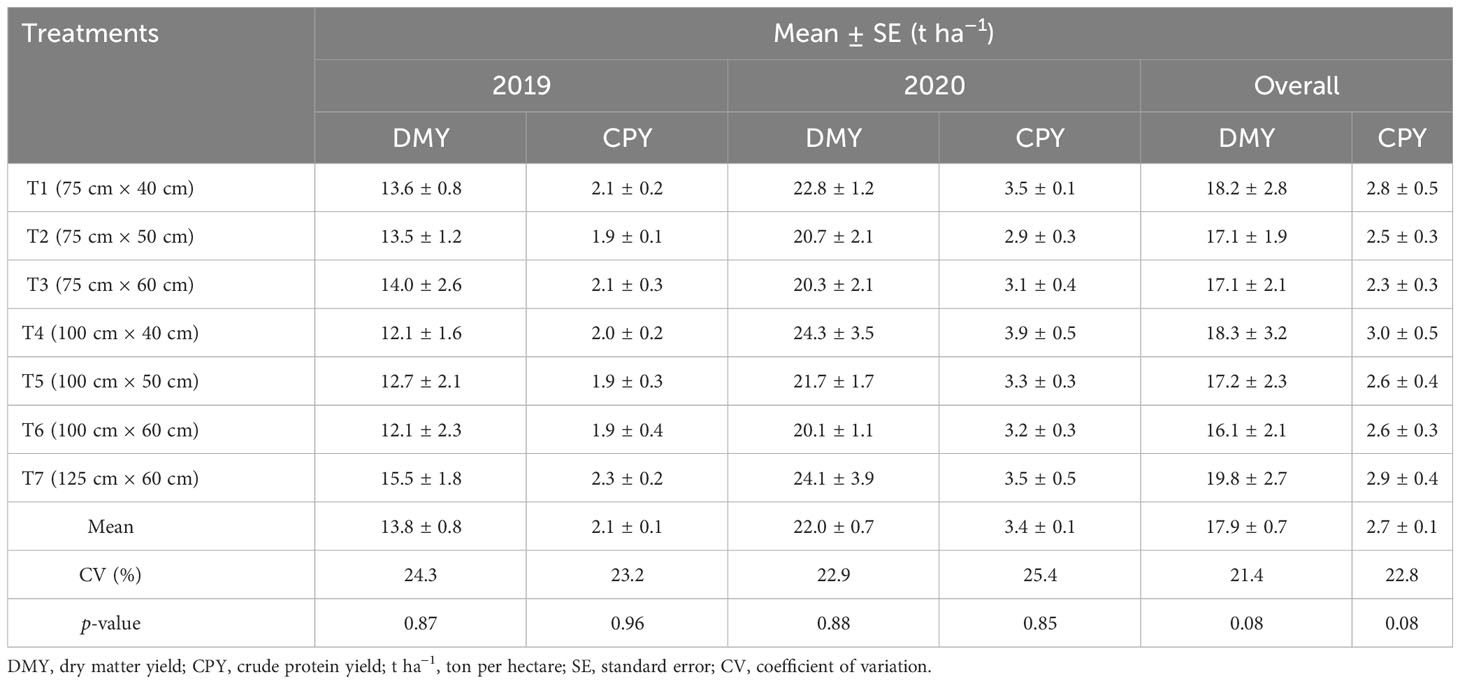
Table 7 Annual dry matter yield and annual crude protein yield (t ha–1) of maralfalfa grass under seven planting densities during the 2019 and 2020 cropping years.
3.7 Trends of dry matter and crude protein yields at harvest
The trends of DM and CPYs of maralfalfa grass under different plant densities during 2019 and 2020 are shown in Figures 3, 4. Three harvests were made in the establishment year, whereas six harvests were achieved in the second year. However, plant density did not affect the DMYs or CPYs at each harvest. During the 2019 cropping season, the DMY at first harvest (DMY1) was slightly increased for T3 (75 cm × 60 cm) relative to T1 (75 cm × 40 cm), T2 (75 cm × 50 cm), and T4 (100 cm × 40 cm) to T7 (125 cm × 60 cm). For harvest 2 (DMY2), the DMY decreased for T3 (75 cm × 60 cm) but slightly increased from T4 to T7. The DMY3 showed curvilinear decreasing trends from T3 (75 cm × 60 cm) to T6 (100 cm × 60 cm). In the second year, DMY1 and DMY2 showed almost similar trends and were greater than DMY4 and DMY6, whereas DMY3 showed inconsistent trends among treatments. In the DMY5 harvest, T1 (75 cm × 40 cm), T4 (100 cm × 40 cm), and T7(125 cm × 60 cm) showed increasing trends as compared with the DMYs achieved in the rest of the treatments. The CPY3 in the first year showed a curvilinear decreasing trend from T3 (75 cm × 60 cm) to T6 (100 cm × 60 cm). In the second year, CPY1 and CPY2 were equivalent but were greater than CPY4 and CPY6, whereas CPY3 showed inconsistent trends among treatment. The CPY consistently followed similar trends as those of DM production in both cropping seasons.
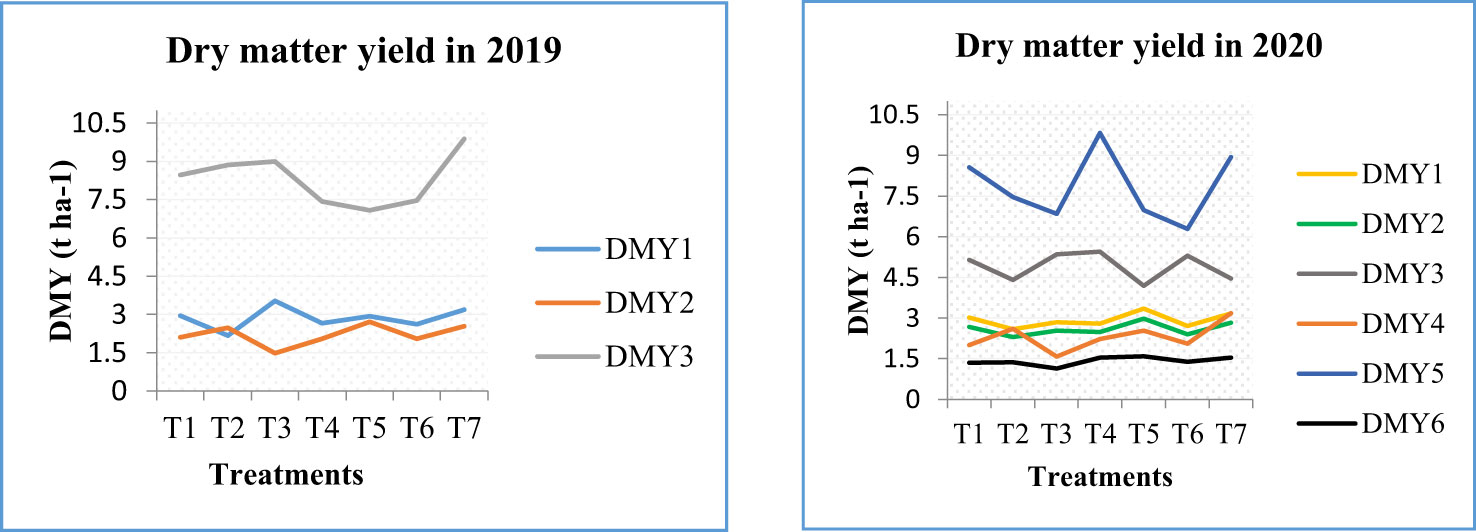
Figure 3 Dry matter yield (DMY) of maralfalfa grass at three or six harvest dates under different planting densities during 2019 and 2020, respectively.
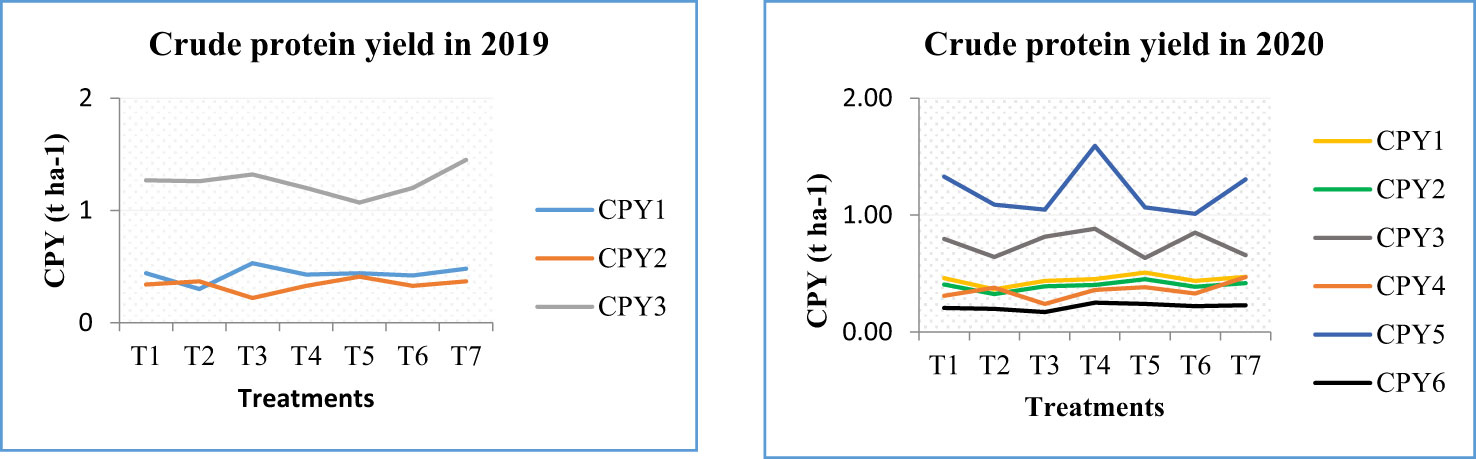
Figure 4 Crude protein yield (CPY) of maralfalfa grass at three or six harvest dates under different planting densities during 2019 and 2020, respectively.
3.8 Chemical composition and in vitro dry matter digestibility
The nutritional contents and in vitro DM digestibility of maralfalfa grass under different planting densities are shown in Table 8. The contents of DM, ash, CP, neutral detergent fiber (NDF), acid detergent fiber (ADF), and in vitro dry matter digestibility (IVDMD) of maralfalfa grass were not influenced by planting density. The overall mean was 15.3 ± 0.2 for CP%, and 58.2 ± 0.4 and 60.4 ± 0.3 for NDF and IVDMD %, respectively.
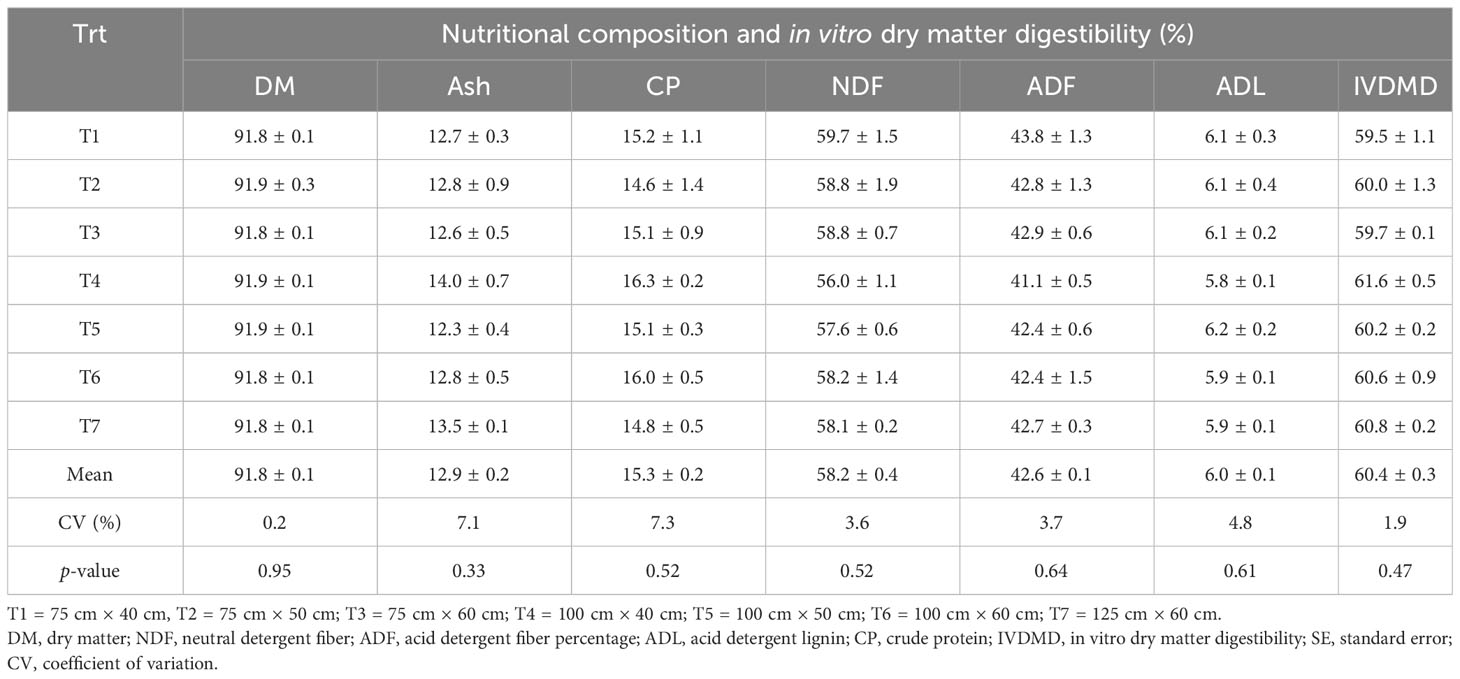
Table 8 Nutritional contents and in vitro dry matter digestibility of maralfalfa grass under different planting densities.
4 Discussion
The survival rate of maralfalfa grass was not influenced by the plant densities. Similarly, the plot cover measured at 8 weeks after the establishment date was not affected by planting density. The insignificant contribution of different planting densities of the maralfalfa grass on the survival rate and plot canopy cover might be due to the good establishment and tillering performances of the grass in the study area. A study done by Kebede et al. (2016) suggested that the establishment performance of the same species or variety varied across different locations due to soil and climatic variations. The establishment and survival rate are strongly related to the rooting ability of seedlings. This indicated that maralfalfa grass is well established and highly adapted to nitisol soil types consisting of clay soil texture and tolerant to acidic soils. The results of this study agree with Geren and Kavut (2015), who noted that maralfalfa grass can adapt to a wide range of soil particles, from sandy to clay, and a pH range of very acidic to alkaline in Ethiopia.
The significant effect of years on PH, NTPP, DM, and CPY may be due to the variation in weather effects (Tessema, 2005); however, it is more likely related to cumulative time from establishment. Thus, significant differences were observed in yield-related traits across the years. Similar to the current study, Habte et al. (2020) indicated that year had a significant (p<0.01) effect on the NTPP, PH, and the LSR of napier grass. Our finding also agrees with Geren and Kavut (2015), who reported that the interactions of year and planting density of maralfalfa were not significant.
The year effect on the measured traits of maralfalfa grass could be due to new tillers emerging from the mother plants with more developed root systems in terms of depth and width in the soil profile and utilizing nutrient reserves available in the soil. Geren and Kavut (2015) indicated that the average number of tillers in the first year (44 tillers) of maralfalfa grass was significantly lower than the second year (62 tillers). Tessema (2008) also reported that the number of tillers of napier grass attained in the second year was approximately seven times higher than in the first year. The DM production also varied over the year, and this might have been due to the regeneration of stems and a greater number of tillers and developed roots to take up available water and nutrients from the soil (Tegami and Mello, 2007; Wijitphan et al., 2009). Tessema (2005) reported that due to its perennial nature, as the pasture period increases, napier grass produces more tillers and greater vegetative growth of napier grass accessions in the north-western parts of Ethiopia. The DMY obtained in the first year was almost similar (13.06 t ha−1) to the first-year DMY of napier grass accessions planted at 100 cm × 50 cm spacing reported by Gezahagn et al. (2017).
In contrast to the present study, Karanja (1984) indicated that narrow spacing resulted in a greater number of, and longer, internodes than wider spacing in napier grass. The study done by Tessema (2008) indicated that plant densities affected leaf length, basal circumference, and nodes per plant in the second year. The presence of a greater proportion of leaves is a good nutritive value indicator, but the planting density in the current study did not significantly influence the LSR of maralfalfa grass. The LSR of plants mainly depends on the number of leaves per plant, leaf length, and thickness of the stem. The nutritive value of any forage crop is highly influenced by leafiness, leaf length, and the nature of the stem. In contrast to the present study, Tessema and Baars (2003) and Taye (2004) found that the LSR of napier grass planted at 100 cm × 50 cm spacing was greater than that planted at 50 cm × 50 cm, with values of 1.91 and 1.49, respectively.
The NTPP was not influenced by plant densities in our study, although one may hypothesize that because plants with wider spacing in the establishment season may have reduced inter-tiller competition for space, moisture, and nutrients during early growth. Although different from the current study, Geren and Kavut (2015) reported that the planting density significantly affected the NTPP. Similarly, Tessema (2008) reported that planting density significantly influenced the NTPP from napier grass: he reported 62.3 tillers from wider rows (175 cm × 25 cm) versus 24.3 tillers from narrower (50 cm × 75 cm) rows.
In agreement with Tessema (2008), there was no significant effect on the PH of napier grass due to planting density in the first year but there was in the second year. The PH range obtained in the first and second years of the present study lies between the reported heights (100 cm to 150 cm) of napier grass (Muia et al., 1999; Fekede et al., 2005; Kariuki et al., 2016).
The DMY difference across the years might have been due to improving vegetative growth as the pasture period increased in perennial grass (Ndikumana, 1996). The greater DMYs obtained at the third harvest (DMY3) during the establishment year and DMY5 during the following year might have been due to the harvests in September and August during the main rainy seasons when rainfall was sufficient for growth. In agreement with the present finding, Berihun (2005) reported that an insignificant difference in DMY of napier grass was attained under different plant densities. In contrast to the present study, Wijitphan et al. (2009) reported that napier grass in different planting spaces (50 cm × 40 cm, 50 cm × 60 cm, 50 cm × 80 cm, and 50 cm × 100 cm) had a significant effect on DM yield, and the greatest total DMY (70.84 t ha−1) was obtained from 3 years’ cumulative harvest from a 50 cm × 40 cm row spacing in Thailand. A study done by Tessema (2008) on napier grass under different planting densities indicated that the DM yield increased as plant density increased. Generally, the variations of DMY at different harvest times may have been due to harvesting at different growth periods. The trends of CPY were consistent with similar trends for DMY as the CPY was computed from the DMY and because the CP content was relatively non-varying.
The insignificant differences in the chemical analysis and IVDMD of maralfalfa grass at different plant densities is likely because all the treatments were harvested at similar growth stages with similar LSRs. The nutritional quality of forages is influenced by the environment (climate), management factors, soil characteristics, and age at harvest (Getnet and Ledin, 2001; Tessema et al., 2011; Keba et al., 2013). Tessema (2008) and Wijitphan et al. (2009) reported that the DM, NDF, ADF, CP, and ADL contents of napier grass were not influenced by plant densities. Marafalfa grass is known to have a high CP content and the present study also indicated that the grass had an overall treatment mean value of 15.3 ± 0.2 CP% content, which is above the minimum CP level of 7% required for optimum rumen function for ruminant animals (Van Soest, 1984). The grass is categorized as a medium protein source of forage (Lonsdale, 1989). Except for the CP of T1 and T3 plant densities, the CP of the planting densities can satisfy the minimum CP content (150 g/kg DM) required for lactation and growth of cattle (Norton,1982). However, compared with high nutritional quality grasses, such as Kikuyu grass (Pennisetum clandestinum) with 21.9% CP and 27.4% ADF, maralfalfa grass had lower CP and higher fiber contents (Echavarria et al., 2020). The mean values of the NDF content of maralfalfa under different plant densities lie between 45% and 65%, a range that is recommended as medium-quality roughage (Singh and Oosting, 1992). The IVDMD value of maralfalfa in the current study was almost similar to the digestibility of tropical grasses, which lies between 50% and 60% (Owen and Jayasuria, 1989).
5 Conclusion
Plant densities had no significant effect on the measured morphological traits, DM production, and nutritional quality of maralfalfa grass. It is recommended that the farmers should consider the availability of planting materials, labor, and transportation cost for the planting materials without compromising the DM production. Thus, using 125 cm × 60 cm inter- and intra-row spacing with 13,333 plant population ha−1 can be an appropriate planting density for an optimum DM production in the study area and similar agro-ecologies of the country. However, further studies on maralfalfa grass should be conducted in multiple locations in Ethiopia under both rain-fed and irrigated conditions and with various agronomic practices.
Data availability statement
The raw data supporting the conclusions of this article will be made available by the authors, without undue reservation.
Author contributions
GM: Data curation, Writing – original draft, Formal analysis, Methodology. GK: Conceptualization, Writing – review & editing. MF: Methodology, Writing – review & editing. FF: Funding acquisition, Project administration, Writing – review & editing. KM: Data curation, Writing – review & editing. AK: Formal analysis, Supervision, Writing – review & editing. DG: Resources, Writing – review & editing. MM: Conceptualization, Data curation, Methodology, Writing – review & editing. MB: Funding acquisition, Project administration, Writing – review & editing. ER: Data curation, Visualization, Writing – review & editing. KB: Data curation, Writing – review & editing. JD: Methodology, Writing – review & editing. AA: Project administration, Resources, Writing – review & editing.
Funding
The author(s) declare financial support was received for the research, authorship, and/or publication of this article. This work was funded in whole or part by the United States Agency for International Development (USAID) Bureau for Resilience and Food Security under Agreement # AID-OAA-L-15-00003 as part of Feed the Future Innovation Lab for Livestock Systems. Additional funding was received from Bill & Melinda Gates Foundation OPP#1175487. Any opinions, findings, conclusions, or recommendations expressed here are those of the authors alone. Under the grant conditions of the Foundation, a Creative Commons Attribution 4.0 Generic License has already been assigned to the Author Accepted Manuscript version that might arise from this submission.
Acknowledgments
We gave many thanks to researchers, and technical and field assistants of forage and pasture research program, Holetta Agricultural Research Center for their continuous dedication and commitment to data collection. Our special thanks to International Livestock Research Institute (ILRI), animal nutrition laboratory technicians for their collaborations, and valuable assistance in the laboratory analysis work.
Conflict of interest
The authors declare that the research was conducted in the absence of any commercial or financial relationships that could be construed as a potential conflict of interest.
The handling editor GD declared a shared affiliation with the authors MB, ER, KB, JD, AA at the time of review.
The reviewer TB declared a shared parent affiliation with the authors GM, GK, MD, FF, KM, AK, DG, MM to the handling editor at the time of review.
Publisher’s note
All claims expressed in this article are solely those of the authors and do not necessarily represent those of their affiliated organizations, or those of the publisher, the editors and the reviewers. Any product that may be evaluated in this article, or claim that may be made by its manufacturer, is not guaranteed or endorsed by the publisher.
References
Aklilu M., Alemayehu M. (2007). Measurements in pasture and forage cropping systems. Technical manual 18 (Addis Ababa, Ethiopia: Ethiopian Agricultural Research Institute (EIAR)).
Ansah T., Osafo E. L. K., Hansen H. H. (2010). Herbage yield and chemical composition of four varieties of Napier (Pennisetum purpureum) grass harvested at three different days after planting. USA: Agriculture and Biology Journal of North America.
AOAC. (1990). Methods of analysis. Association of Official Analytical Chemists. 15th ed (International, Arlington, Virginia, USA: AOAC).
Berihun M. (2005). Effect of planting patterns and harvesting days on yield and quality of Bana grass [Pennisetum Purpureum (L.) x Pennisetum Americanum (L.)]. MSc Thesis (Haramaya, Ethiopia: Haramaya University).
Bolonio D., Neu P. M., Schober S., Mittelbach M., Canoira L. (2015). International Congress and Expo on Biofuels & Bioenergy. (Valencia, Spain).
Bray R. H., Kurtz L. T. (1945). Determination of total, organic, and available forms of phosphorus in soils. Soil Sci. 59 (1), 39–46. doi: 10.1097/00010694-194501000-00006
Clavero T., Razz R. (2009). Nutritive value of Maralfalfa grass (Pennisetum purpureum× Pennisetum glaucum) under defoliation conditions. Rev. la Facultad Agronomía Universidad del Zulia 26 (1), 78–87.
Correa H. J. (2006). Calidad nutricional del pasto maralfalfa (Pennisetum sp) coseChado a dos edades de rebrote. Livestock Res. Rural Dev. 18, 326–335, 6.
Correa H., Cerón J., Arroyave H., Henao Y., López A. (2004). Pasto Maralfalfa: mitos y realidades. IV seminario internacional Competitividad en carne y leche (Medellín, Colombia: Cooperativa Colanta), 231–274.
Criscioni P., Marti J. V., Pérez-Baena I., Palomares J. L., Larsen T., Fernández C. (2016). Replacement of alfalfa hay (Medicago sativa) with maralfalfa hay (Pennisetum sp.) in diets of lactating dairy goats. Anim. Feed Sci. Technol. 219, 1–12. doi: 10.1016/j.anifeedsci.2016.05.020
Danalatos N. G., Archontoulis S. V., Mitsios I. (2007). Potential growth and biomass productivity of Miscanthus x giganteus as affected by plant density and N-fertilization in central Greece. Biomass and Bioenergy 31, 145–152. doi: 10.1016/j.biombioe.2006.07.004
Echavarria D. M., Giraldo L. A., Marín A. (2020). In vitro fermentation of Pennisetum clandestinum Hochst Ex. Chiov increased methane production with ruminal fluid adapted to crude glycerol. Trop. Anim. Health Prod. 52 (2), 565–571. doi: 10.1007/s11250–019–02043–5
EIAR (Ethiopian Institute of Agricultural Research) (2005). Holetta Agricultural Research Center/HARC/progress report 2005/06 (Holetta, Ethiopia: EIAR/HARC).
Fekede F., Getnet A., Lulseged G., Muluneh M., Tadesse T. (2005). “Evaluation of Napier grass-vetch mixture to improve total herbage yield in the central highlands,” in Proceedings of the 13th annual conference of the Ethiopian Society of Animal Production (ESAP) (Addis Ababa, Ethiopia: Ethiopian Society of Animal Production (ESAP)).
Geren H., Kavut Y. (2015). Effect of different plant densities on the yield and some silage quality characteristics of giant king grass (Pennisetum hybridum) under Mediterranean climatic conditions. Turkish J. Field Crops 20 (1), 85–91. doi: 10.17557/.08771
Getnet A., Ledin I. (2001). Effect of variety, soil type, and fertilizer on the establishment, growth, forage yield, quality, and voluntary intake by cattle of oats and vetches cultivated in pure stands and mixtures. Anim. feed Sci. Technol. 92 (1-2), 95–111. doi: 10.1016/S0377-8401(01)00242-5
Gezahagn K., Fekede F., Getnet A., Mengistu A., Alemayehu M., Aemiro K., et al. (2017). Agronomic performance, dry matter yield stability and herbage quality of Napier grass (Pennisetum purpureum (L.) Schumach) accessions in different agroecological zones of Ethiopia. J. Agric. Crop Res. 5 (4), 49–65.
Habte E., Muktar M. S., Abdena A., Hanson J., Sartie A. M., Negawo A. T., et al. (2020). Forage performance and detection of marker trait associations with potential for Napier grass (Cenchrus purpureus) improvement. Agronomy 10 (4), 542. doi: 10.3390/agronomy10040542
Hanna W. W., Gaines T. P., Onzales B. G., Monson W. G. (1984). Effects of ploid on yield and quality of pearl millet x Napier grass hybrids. Agron. J. 76, 669–971. doi: 10.2134/agronj1984.00021962007600060024x
Karanja G. M. (1984). The effects of frequency and height of cutting on tillering, herbage yield and nutritive value of four varieties of napier grass (Nairobi, Kenya: Diss. University of Nairobi).
Karforma J. (2018). “Hybrid Napier (napier bajra hybrid),” in Forage Crops of the World, Volume I: Major Forage Crops (New York: Apple Academic Press), 159–170.
Kariuki I. W., Mwendia S. W., Muyekho E. N., Ajanga S. I., Omayio D. O. (2016). Biomass production and forage quality of head-smut disease Resistant Napier grass accessions. Afr. Crop Sci. J. 24, 157–165. doi: 10.4314/acsj.v24i1.18S
Keba H. T., Madakadze I. C., Angassa A., Hassen A. (2013). Nutritive value of grasses in semi-arid rangelands of Ethiopia: local experience-based herbage preference evaluation versus laboratory analysis. Asian-Australasian J. Anim. Sci. 26 (3), 366. doi: 10.5713/ajas.2012.12551
Kebede G., Feyissa F., Assefa G., Alemayehu M., Mengistu A., Kehaliew A., et al. (2016). Chemical composition and in-vitro organic matter digestibility of napier grass (Pennisetum purpureum (L.) schumach) accessions in the mid and highland areas of Ethiopia. Int. J. Livestock Res. 6 (4), 41–59. doi: 10.5455/ijlr.20160317124016
Lonsdale C. (1989). Raw Materials for Animal Feeds Compounders and Farmers (Britain: Chalcombe Publications Great Britain), 17–47.
Malley O., Grattapaglia D. M., Chaparro D., Wilcox J. X., Amerson P. L., Liu H. V. (1996). “Molecular markers, forest genetics, and tree breeding,” in Genomes of plants and animals (Boston, MA: Springer), 87–102.
Márquez F. J., Sánchez D., Urbano and Dávila C. (2007). Evaluation of the cutting frequency and fertilization types on three elephant grass genotypes (Pennisetum purpureum) on Yield and protein content. Rendimiento y contenido proteína. ZootecniaTrop 25 (4), 253–259.
MOA (Ministry of Agriculture) (2018). Plant Variety Release, Protection and Seed Quality Control Directorate, Crop Variety Register Issue No.21 (Addis Ababa Ethiopia).
Muia J. M., Tamminga S., Mbugua P. N., Kariuki J. N. (1999). Optimal stage of maturity for feeding Napier grass (Pennistetum purpureum) to dairy cows in Kenya. (Naivasha, Kenya).
Ndikumana J. (1996). Multi-locational evaluation of Napier and Pennisetum hybrids: Highlights of from the Addis Ababa. AFRNET Newslett. 6, 1–11.
Norton B. W. (1982). “Differences between species inforage in forage,” in Proceedings of international symposium held at St. Luica, Queeensland, Australia, (Queensland, Australia: Nutritional limits to animal production from pastures). 24-29 Sep. 1981.
Nyambati E. M., Muyekho F. N., Onginjo E., Lusweti C. M. (2010). Production, characterization and nutritional quality of Napier grass [Pennisetum purpureum (Schum.)] cultivars in Western Kenya. Afr. J. Plant Sci. 4, 496–502.
Obok E. E., Akenrsquo M. E., Iwo G. A. (2012). Forage potentials of interspecific hybrids between elephant grass selections and cultivated pearl millet genotypes of Nigerian origin. J. Plant Breed. Crop Science. 4 (8), 136–143.
Okalebo J. R., Gathua K. W., Woomer P. L. (1993). Laboratory methods for soil and plant analysis: A working manual (Nairobi, Kenya: Tropical Soil Biology and Fertility Programme).
Owen E., Jayasuriya M. C. N. (1989). Use of crop residues as animal feeds in developing countries: a review. Res. Dev. Agriculture. 6 (3), 129–138.
Palacios-Díaz M. P., Mendoza-Grimón V., Fernández-Vera J. R., Hernández-Moreno J. M. (2013). Effects of defoliation and nitrogen uptake on forage nutritive values of Pennisetum sp. Anim. Plant Sci. 23 (2), 566–574.
Ramirez R., Londoño I., Alonso J., Morales M. (2006). Evaluación del pasto Maralfalfa (ractices sp.) como recuperador de un andisol degradado por ractices agrícolas.
Santos R. J. C. D., Lira M. D. A., Guim A., Santos M. V. F. D., Junior J. C. B. D., Mello A. C. D. L. D. (2013). Elephant grass clones for silage production. Sci. Agric. 70 (1), 6–11. doi: 10.1590/S0103-90162013000100002
Scholtz G. D. J. (2008). A grading system for Medicago sativa hay in South Africa (Doctoral dissertation (South Africa: University of the Free State).
Singh G. P., Oosting S. J. (1992). A model for describing the energy value of straws. Indian dairyman XLI, 322–327.
Singh B. P., Singh H. P., Obeng E. (2013). Biofuel Crops: Production, Physiology and Genetics; 13th section: Elephant grass. Ed. Singh B. P. (United Kingdom: CAB International), 271–291.
Taye B. (2004). Effect of intercropping Napier grass with Desmodim intortum or Lablab purpureus on forage yield and nutritional quality. MSc Thesis (Haramaya, Ethiopia: Haramaya University).
Tegami Â, Mello S. (2007). Avaliação da produtividade e qualidade do capim paraíso (Pennisetum hybridum), em função de diferentes doses de nitrogênio em cobertura e freqüência de corte. Nucleus 4 (1-2), 9–12.
Tessema Z. (2005). Variation in growth, yield, chemical composition and in vitro dry matter digestibility of Napier grass accessions (Pennisetum purpureum). Trop. Sci. 45, 93–99. doi: 10.1002/ts.51
Tessema Z. (2008). Effect of plant density on morphological characteristics, yield and chemical composition of napier grass (Pennisetum purpureum (L.) Schumach). East Afr. J. Sci. 2 (2), 55–61. doi: 10.4314/eajsci.v2i1.40365
Tessema Z., Baars R. M. T. (2003). Effect of cutting height of Napier grass on rumen degradation and invitro dry matter digestibility. Trop. Sci. 43, 125–121. doi: 10.1002/ts.102
Tessema Z. K., De Boer W. F., Baars R. D., Prins H. H. T. (2011). Changes in soil nutrients, vegetation structure and herbaceous biomass in response to grazing in a semi-arid savanna of Ethiopia. J. Arid Environments 75 (7), 662–670. doi: 10.1016/j.jaridenv.2011.02.004
Tilley J. M. A., Terry D. R. (1963). A two-stage technique for the in vitro digestion of forage crops. Grass Forage Sci. 18 (2), 104–111. doi: 10.1111/j.1365-2494.1963.tb00335
Van Soest P. J., Robertson J. B. (1985). “Analysis of Forages and Fibrous Feeds,” in Laboratory Manual for Animal Science, vol. 613. (New York, USA: Cornell University).
Walkley A. J., Black I. A. (1934). Estimation of soil organic carbon by the chromic acid titration method. Soil Sci. 37, 29–38.
Keywords: establishment, plant spacing, management practices, maralfalfa grass, yield, quality
Citation: Mengistu G, Kebede G, Faji M, Feyissa F, Mohammed K, Kehaliew A, Geleti D, Minta M, Balehegn M, Rios EF, Adesogan AT, Dubeux JCB Jr and J. Boote K (2024) Morphological characteristics, dry matter yield, and nutritive value of maralfalfa grass (Pennisetum spp.) grown under different planting densities in the central highlands of Ethiopia. Front. Anim. Sci. 4:1308911. doi: 10.3389/fanim.2023.1308911
Received: 07 October 2023; Accepted: 18 December 2023;
Published: 15 January 2024.
Edited by:
Geoffrey E. Dahl, University of Florida, United StatesReviewed by:
Elio Romano, Centro di ricerca per l’Ingegneria e le Trasformazioni agroalimentari (CREA-IT), ItalyBimrew Asmare, Bahir Dar University, Ethiopia
Tolossa Ameyu Bedessa, Ethiopian Institute of Agricultural Research (EIAR), Ethiopia
Copyright © 2024 Mengistu, Kebede, Faji, Feyissa, Mohammed, Kehaliew, Geleti, Minta, Balehegn, Rios, Adesogan, Dubeux and J. Boote. This is an open-access article distributed under the terms of the Creative Commons Attribution License (CC BY). The use, distribution or reproduction in other forums is permitted, provided the original author(s) and the copyright owner(s) are credited and that the original publication in this journal is cited, in accordance with accepted academic practice. No use, distribution or reproduction is permitted which does not comply with these terms.
*Correspondence: Gezahegn Mengistu, gezemen19@gmail.com