- 1Department of Veterinary Medicine and Animal Sciences – DIVAS, University of Milan, Lodi, Italy
- 2Department of Anesthesiology, Clinica Veterinaria Gran Sasso, Milan, Italy
- 3Department of Informatics, University of Milan, Milan, Italy
- 4Department of Surgery, Clinica Veterinaria Gran Sasso, Milan, Italy
- 5Department of Cardiology, Clinica Veterinaria Gran Sasso, Milan, Italy
Introduction: Arterial blood gas analysis is the gold standard for the assessment of oxygenation, ventilation, and metabolic status in dogs; however, its execution is difficult and painful. Therefore, venous blood gas analysis is used in its replacement for the assessment of the metabolic status, but it is not clear whether it can be used to assess respiratory function, too. This study aimed at: 1) comparing jugular and saphenous pH and partial pressure of carbon dioxide (pCO2) with the correspondent arterial pH and pCO2 (paCO2) in healthy dogs during general anesthesia; 2) clarifying whether the arterial-venous relationship is better expressed in jugular or saphenous blood samples; 3) mathematically transforming venous pCO2 (pvCO2) and evaluating whether the calculated values more accurately agree with paCO2.
Methods: Ninety dogs were included and randomly divided into three groups: Group 1 - arterial vs jugular; Group 2 - arterial vs saphenous; Group 3 - arterial vs jugular vs saphenous blood gases. Each group counted 30 dogs. Pearson correlations were calculated. Bland-Altman plots were generated to describe the agreement between venous and arterial values; clinical limits for pH and pCO2 set by the authors were, respectively, ± 0.1 and ± 2.5 mmHg. Univariate linear regression was applied for predicting paCO2 from pvCO2.
Results: Saphenous samples showed strong positive correlations with arterial samples for both pCO2 and pH. Pearson ρ values were stronger for pH than for pCO2. Bland-Altman plots showed good agreement between venous and arterial pH, and poor agreement between pvCO2 and paCO2 for both jugular and saphenous samples. Results suggested that saphenous pvCO2 is preferable with respect to jugular as predictor of paCO2. The transformation of saphenous pvCO2 through univariate linear regression produced a model for predicting paCO2; a Bland-Altman plot assessed the transformed pvCO2 agreement with paCO2.
Discussion: In healthy, anesthetized, mechanically ventilated dogs, variations of pH between venous and arterial values are clinically acceptable. Venous and arterial blood gases cannot be interchanged for the evaluation of pCO2. Saphenous pvCO2 is to be preferable to jugular pvCO2 as predictor of paCO2. A formula for the estimation of predicted paCO2 from saphenous pvCO2 is proposed.
1 Introduction
Arterial blood gas (ABG) analysis is considered the gold standard for the assessment of oxygenation, ventilation, and metabolic status in healthy and ill patients (Razi et al., 2012). However, arterial puncture requires trained personnel and it is painful, therefore it is difficult to be conducted in a conscious animal; moreover, the methodic is associated with an elevated risk of adverse effects such as arterial injury, hemorrhage and infection (Rees et al., 2006; Razi et al., 2012). For these reasons, arterial blood gas (ABG) has been replaced by venous blood gas (VBG) to evaluate the patient’s metabolic status. However, it is not clear whether it can be used to assess the respiratory function, as well; in fact, conditions that affect arterial flow and venous return (i.e. congestive heart failure, circulatory shock, respiratory failure) may influence arteriovenous measures creating a significative difference in values obtained in VBG compared to ABG (Byrne et al., 2014; Chung et al., 2019; Nanjayya et al., 2020). Numerous studies have been conducted in the last few years in human medicine to establish whether there is a relationship between arterial and venous pH and partial pressure of carbon dioxide (pCO2) and how constant and predictable this relationship is, in healthy and ill, conscious and anesthetized patients (Kelly, 2010; Bloom et al., 2014; Byrne et al., 2014). Some authors have even proposed methods to extrapolate arterial values from the VBG analysis (Rees et al., 2006; Lumholdt et al., 2020; Weber and Cave, 2021). Results are different among these studies; however, most of them agree that VBG analysis is a useful tool for the evaluation of pH, since the agreement between arterial and venous values is very good. On the contrary, ABG cannot be replaced by VBG analysis for the assessment of pCO2 since arteriovenous agreement for this parameter is poor and the differences between venous and arterial values are large enough to be of clinical significance (Kelly, 2010; Bloom et al., 2014; Byrne et al., 2014).
In veterinary medicine, only a few studies have been conducted to evaluate the relationship between ABG and VBG analyses in conscious and anesthetized dogs (Ilkiw et al., 1991; Atalan et al., 2008; Pang et al., 2009; Tamura et al., 2015; Kadwa et al., 2022; Shiroshita et al., 2023). Pang et al. in 2009 assessed the suitability of lingual VBG for the determination of pH and pCO2 as an alternative to ABG (collected from the dorsal pedal artery) in anesthetized dogs. The differences between venous and arterial values for pH and pCO2 were deemed as clinically acceptable by the authors (Pang et al., 2009). In contrast, Tamura et al. in 2015 compared VBGs obtained from the jugular vein with ABGs obtained from the dorsal pedal artery in healthy conscious dogs and found that differences in venous and arterial pCO2 and pH were of clinical impact; therefore, the authors discouraged interchangeability between VBG and ABG (Tamura et al., 2015). Similarly, Kadwa et al. compared VBGs from the jugular vein to ABGs from the dorsal pedal artery in anesthetized dogs with respiratory acidosis and concluded that pH measured from the jugular vein is an acceptable alternative to arterial pH, while the same cannot be stated for pCO2 (Kadwa et al., 2022). Finally, Shiroshita et al. compared cephalic and saphenous VBG with ABG (collected from the dorsal pedal artery) after continuously heating the arterial and posterior limbs to 37°C. This procedure has been defined “arterialization”: in human medicine, heating hands is known for making venous blood becoming more similar to arterial blood. Through this procedure, pH showed no significant difference between both the VGBs and ABG. Moreover, pvCO2 collected from the saphenous vein was considered clinically interchangeable with paCO2, unlike pvCO2 collected from the cephalic vein which showed a poor agreement with paCO2 (Shiroshita et al., 2023).
Since veterinary literature, at present, counts different study designs and different applied statistical analyses, the variability of these results could be difficult to interpret. For these reasons, this study aimed at: 1) comparing jugular and saphenous venous blood pH and pCO2 with the correspondent values obtained from arterial blood samples in healthy dogs during general anesthesia; 2) clarifying whether the relationship between venous and arterial values is better expressed in jugular or saphenous blood samples; 3) mathematically transforming venous pCO2 and evaluating whether the calculated values more accurately agree with arterial values.
2 Materials and methods
2.1 Study design, population, and protocol
This is a preliminary cross-sectional study undertaken at the Clinica Veterinaria Gran Sasso (Milan) in 2023; it was previously approved by the Institutional Ethical Committee for Animal Care at the University of Milan (72/2023). Healthy dogs admitted at the veterinary clinic for elective surgery (ovariectomy via open surgery, orchiectomy, orthopedic implants removal, dental surgery), with no abnormalities on a total blood count and a biochemical analysis as well as on a routine pre-anesthetic echocardiographic examination and a thoracic radiography, were deemed eligible for the study. Laparoscopy was considered an exclusion criterion for this study. A written informed consent was signed by the dogs’ owners. All the dogs were fasted from food for at least 12 hours, and from water for at least 6 hours prior to admission.
Premedication consisted of methadone (0.2 mg kg-1; Semfortan, Dechra Veterinary Products Srl) administered intramuscularly. A cephalic vein was cannulated (Jelco, Smiths Medical International), anesthesia was induced intravenously with propofol to effect (Propomitor, Orion Pharma) and every patient was intubated with cuffed tubes. For each patient, a catheter was positioned into the dorsal pedal artery (Jelco, Smiths Medical International) for the monitoring of the invasive blood pressure during the procedure and for the execution of arterial blood samples.
Anesthesia was maintained with isoflurane (IsoFlo, Zoetis) stabilized at an end-tidal isoflurane concentration of 1.5 and delivered in various percentages of oxygen and medical air, and mechanical ventilation was configured and maintained for the entire surgical procedure (GE Datex-Ohmeda Avance). Volume-controlled ventilation was set, with values of tidal volume and respiratory rate that were tailored on each patient in order to mantain eucapnia throughout the entire surgical procedure. Eucapnia was considered as an end-tidal CO2 (etCO2) of 30-40 mmHg (Teixeira Neto et al., 2002; Hopper and Powell, 2013). Anesthesiologic parameters (heart rate, systolic, diastolic and mean arterial pressure via invasive blood pressure monitoring, oxygen saturation, etCO2, respiratory rate, isofluorane minimum alveolar concentration, and spirometry data) were registered with a multiparameter monitor (GE Datex-Ohmeda S5 Compact) and recorded every five minutes. Dogs that during the surgical procedure and blood sampling developed hyotension and needed treatment to address it were excluded from the study. Hypotension was defined as systolic arterial pressure< 80 mmHg and/or mean arterial pressure< 60 mmHg (Waddell, 2000). In the same way, patients that during surgery developed hypertension due to noxious stimuli were excluded. Hypertension was defined as systolic arterial pressure ≥ 160 mmHg (Acierno et al., 2018).
At the end of surgery, arterial and venous blood samples were simultaneously drawn while patients were still mechanically ventilated (0.5 mL each). Prior to sample acquisition, 0.5 mL of waste blood was drawn from the arterial catheter. Venous blood was collected from the jugular and the saphenous veins via venipuncture. Arterial, jugular and saphenous blood samples were placed into heparinized syringes and analyzed within 10 minutes with a blood gas analyzer machine (Stat Profile pHOx Ultra, Nova Biomedical). For each patient, data about the fraction of inspired O2 (FiO2) and etCO2 were registered from the multiparameter monitor at the time of blood collection, and rectal body temperature was measured; FiO2 and body temperature values were used to calibrate the blood gas analysis machine. The enrolled patients were randomly divided into three groups basing on the site of blood sampling: Group 1 for the analysis of arterial vs jugular blood gases; Group 2 for the analysis of arterial vs saphenous blood gases; Group 3 for the analysis of arterial vs jugular vs saphenous blood gases. For the purposes of our study, Group 3 was divided into three subgroups in order to obtain paired arterial-venous and venous-venous pH and pCO2 values. Hence, subgroups were organized as follows: Group 3a: arterial vs jugular blood gases; Group 3b: arterial vs saphenous blood gases; Group 3c: saphenous vs jugular blood gases. Venous and arterial pH and pCO2 values were then collected.
2.2 Statistical analysis
Statistical analysis was performed using IBM SPSS Statistics 27 [release 27.0.1.0] for macOS and R [release 2021-09-20] for macOS. Distribution of variables was tested for normality using the Shapiro-Wilk test. For normally distributed variables the descriptive analysis of the sample was performed in terms of mean and standard deviation (SD) and variables were compared by the two-sided Student’s t-test (for paired samples when appropriate); for non-normally distributed variables the descriptive analysis was performed in terms of median and interquartile range (IQR) and variables were compared by the Mann-Whitney U test; categorical data were described by frequencies. Linear correlation between variables was investigated by the Pearson ρ correlation coefficient, with the following interpretation: ≤ 0.3 weak correlation, > 0.3 and ≤ 0.7 moderate correlation, > 0.7 strong correlation. A p-value of 0.05 was taken as statistical significance.
Bland-Altman plots were used to describe the degree of agreement between two measurements (Bland and Altman, 1986): the plots show the mean difference (MD), the upper and lower limits of agreement (LOAs) - which are ± 1.96 SDs from the MD, delimiting an interval in which 95% of the differences between the two measures are included, and a 95% confidence interval (CI) for the MD and the LOAs. The 95% LOAs were then compared to upper and lower clinical limits predefined by the authors. The clinical limit width for pCO2 was set from -2.5 mmHg to +2.5 mmHg, and for pH from -0.1 to +0.1. These values were obtained from previously published acceptable clinical limits in human medicine (Ricos et al., 1999; Chung et al., 2019) and were deemed as clinically acceptable by the authors for the everyday practice; therefore, within these limits the two measurements were considered interchangeable.
Univariate linear regression was applied for predicting paCO2 from saphenous pvCO2 after analyzing the results.
3 Results
3.1 Descriptive statistics
The sample consisted of 90 dogs and included 47 females (52.2%), of which 29 (61.7%) intact and 18 (38.3%) sterilized, and 43 males (47.8%), of which 36 (83.7%) intact and 7 (16.3%) neutered. The data set included dogs of different breeds (n. 36). The median weight was 16.0 kg (IQR 20.3), and there wasn’t a statistically significant difference between females (16.0 kg, IQR 20.4) and males (16.4 kg, IQR 20.5). Group 1 was composed of 30 dogs, therefore 30 pairs of arterial and jugular venous samples were obtained; Group 2 counted 30 dogs, for a total of 30 pairs of arterial and saphenous venous samples; finally, Group 3 counted the remaining 30 dogs, in which blood gas analysis was conducted on arterial, jugular, and saphenous blood samples (Group 3a: arterial vs jugular; Group 3b: arterial vs saphenous; Group 3c: saphenous vs jugular). Thus, a total of 60 jugular-arterial (Groups 1 and 3a) and 60 saphenous-arterial (Groups 2 and 3b) blood gases were obtained.
Descriptive statistics for clinical and blood gas variables of interest in our population are shown in Table 1. All variables showed a normal distribution, except for FiO2 and body temperature. Table 2 summarizes the frequency of occurrence of each surgical procedure within the three groups of interest. None of the dogs experienced any complications either during or after surgery.
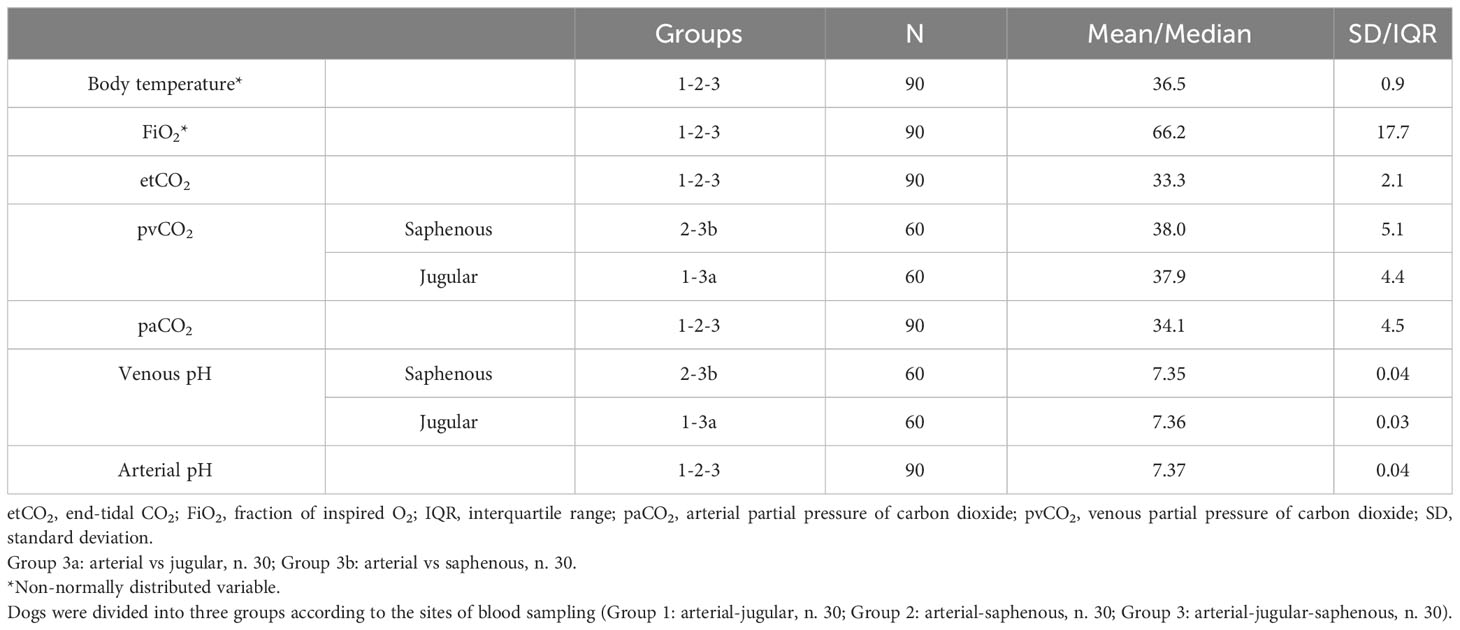
Table 1 Clinical (rectal body temperature), ventilatory (FiO2 and etCO2 registered from the multiparameter monitor at time of blood collection) and blood gas variables (pvCO2, paCO2, venous pH, arterial pH) collected in 90 healthy mechanically ventilated dogs, expressed as mean ± standard deviation or median and interquartile range basing on their distribution (respectively, normal and non-normal).
3.2 Correlations and agreement analysis
Firstly, we analyzed the correlation between jugular vs saphenous and between venous (saphenous or jugular) vs arterial values through the Pearson correlation analysis. Secondly, the agreement between venous (jugular or saphenous) and arterial values was assessed through Bland-Altman plots. Results showed a strong correlation between saphenous and arterial values; however, their agreement was not good, so in a second step we transformed saphenous values through linear regression in order to obtain a better agreement with arterial values.
3.2.1 Pearson correlation
Pearson correlations between jugular vs saphenous values of pCO2 and pH were analyzed in Group 3c, but, while the ρ value was strong for pH (0.74), it was only moderate for pCO2 (0.69). Pearson correlations were then calculated between jugular-arterial (Groups 1 and 3a) and saphenous-arterial pairs (Groups 2 and 3b), and the results are summarized in Table 3: saphenous samples show stronger positive correlations with arterial samples for both pCO2 and pH than jugular samples do. Results also show how ρ values are stronger for pH than for pCO2 in every analysis. All the correlations coefficients were statistically significant (p< 0.001).
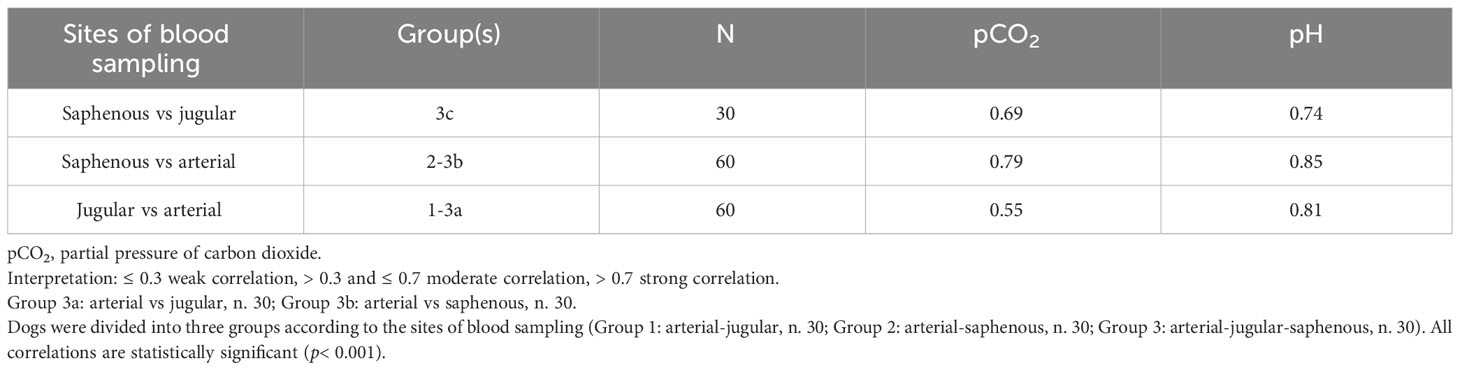
Table 3 Pearson correlations between different pairs of blood samples collected in 90 healthy mechanically ventilated dogs.
3.2.2 Bland-Altman plots for the agreement between venous (jugular or saphenous) and arterial values
The Bland-Altman plots graphically describe the agreement between venous and arterial samples. Figure 1 shows the agreement between jugular-arterial (A) and saphenous-arterial (B) pCO2. For the paired jugular-arterial pCO2 (Groups 1 and 3a), the MD was 3.42 mmHg (95% CI: 2.31-4.52 mmHg), with 95% LOAs of -4.94 mmHg (95% CI: -6.86 to -3.03 mmHg) to 11.79 mmHg (95% CI: 9.88-13.70 mmHg). For the paired saphenous-arterial pCO2 (Groups 2 and 3b), the MD was 4.18 mmHg (95% CI: 3.37-4.98 mmHg), with 95% LOAs of -1.90 mmHg (95% CI: -3.29 to -0.51 mmHg) to 10.26 mmHg (95% CI: 8.87-11.65 mmHg).
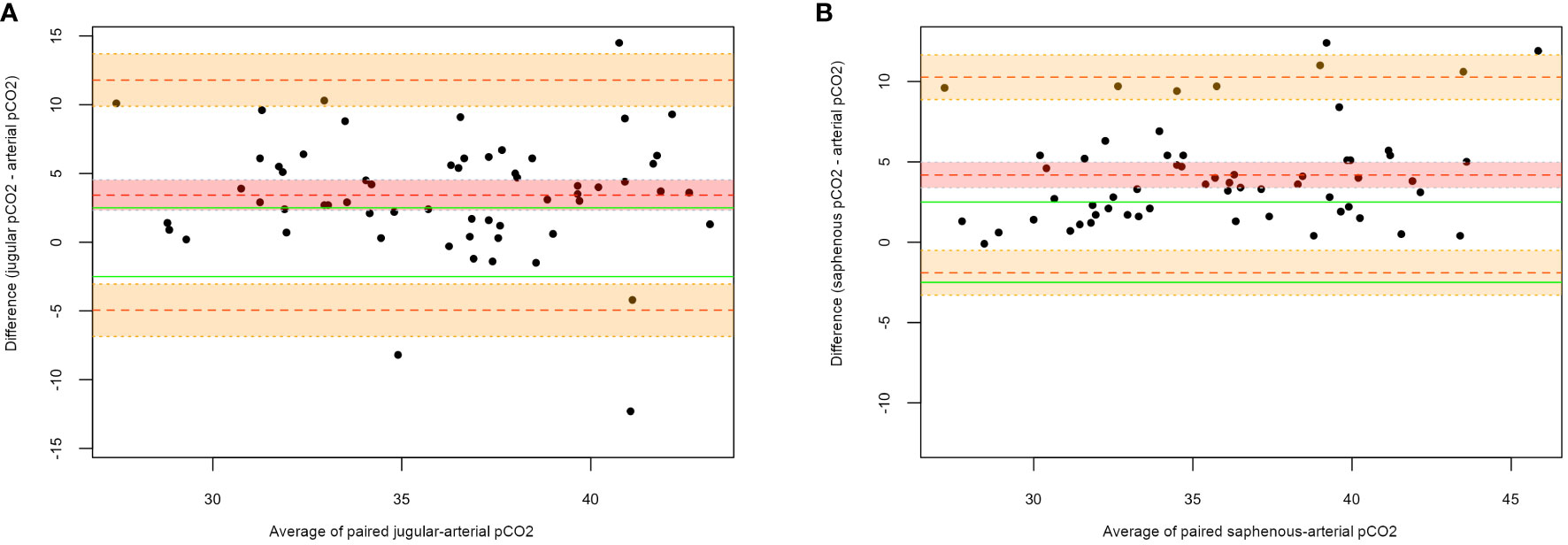
Figure 1 Bland-Altman plots for jugular-arterial and saphenous-arterial pCO2. (A) Jugular-arterial pairs; (B) Saphenous-arterial pairs. On the X-axis the average of the paired measures is represented, while on the Y-axis the difference between the two measures is reported. The central dotted red line corresponds to the MD; the upper and lower orange dotted lines correspond to the upper and lower LOAs, respectively; the green lines correspond to the clinical limits predefined by the authors (± 2.5 mmHg); the red shadowed area outlines a 95% CI for the mean difference; the orange shadowed areas outline a 95% CI for the upper and lower limits of agreement.
Figure 2 shows the agreement between jugular-arterial (A) and saphenous-arterial (B) pH. For the paired jugular-arterial pH (Groups 1 and 3a), the MD was -0.015 (95% CI: -0.02 to -0.01), with 95% LOAs of -0.05 (95% CI: -0.06 to -0.04) to 0.02 (95% CI: 0.01-0.03). For the paired saphenous-arterial pH (Groups 2 and 3b), the MD was -0.021 (95% CI: -0.026 to -0.015), with 95% LOAs of -0.06 (95% CI: -0.07 to -0.05) to 0.02 (95% CI: 0.008-0.026).
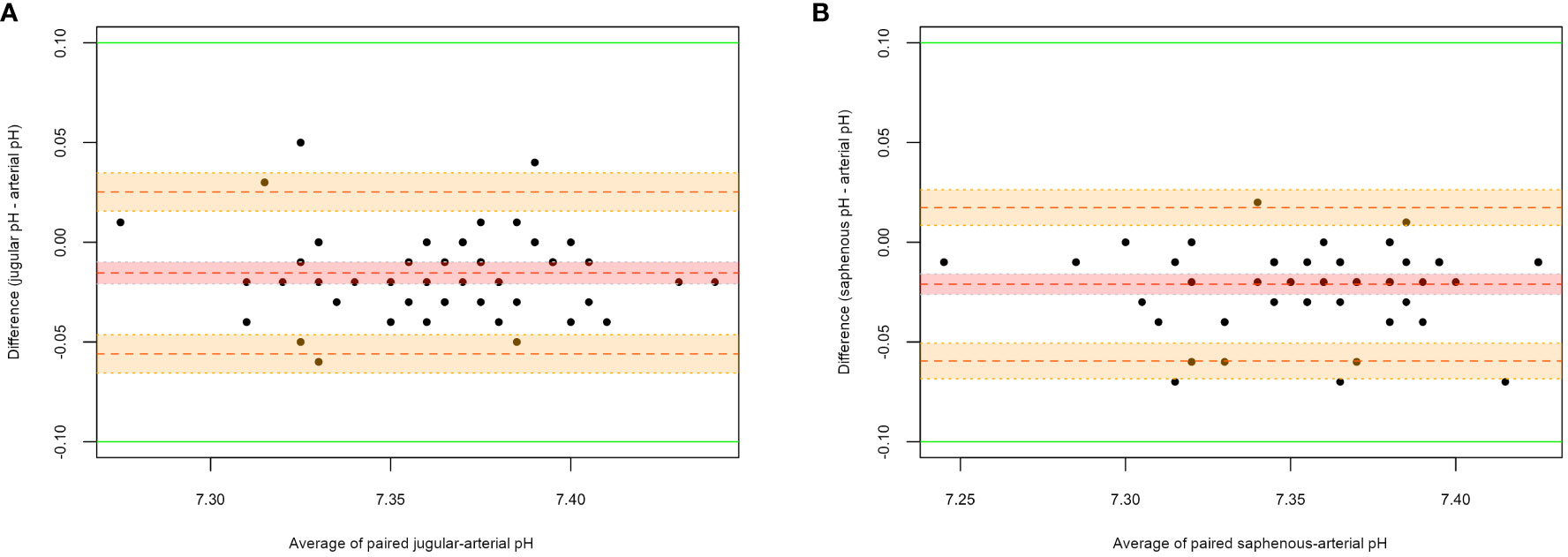
Figure 2 Bland-Altman plots for jugular-arterial and saphenous-arterial pH. (A) Jugular-arterial pairs; (B) Saphenous-arterial pairs. On the X-axis the average of the paired measures is represented, while on the Y-axis the difference between the two measures is reported. The central dotted red line corresponds to the MD; the upper and lower orange dotted lines correspond to the upper and lower LOAs, respectively; the green lines correspond to the clinical limits predefined by the authors (± 0.1); the red shadowed area outlines a 95% CI for the mean difference; the orange shadowed areas outline a 95% CI for the upper and lower limits of agreement.
3.2.3 Bland-Altman plot for the agreement between transformed saphenous and arterial pCO2
Both the correlation and the agreement analyses suggested that saphenous values of pCO2 were preferable to jugular ones as predictors of arterial values. In fact, as shown in Table 3, the correlation between saphenous pvCO2 and paCO2 was 0.79. However, the Bland-Altman plot showed a bias since the mean difference was 4.18 mmHg. For these reasons, we mathematically transformed the saphenous pvCO2: to do so, we applied univariate linear regression (independent variable: saphenous pvCO2; dependent variable: paCO2) to produce a model for predicting paCO2 from saphenous pvCO2.
Through the univariate linear regression, we produced a simple linear regression model which can be explained with the following equation:
The model that we produced is reported in Table 4 and it explained the 63% of the variability of data (R-squared = 0.63).

Table 4 Linear regression model for the estimation of paCO2 from saphenous pvCO2, obtained in 60 healthy mechanically ventilated dogs (Group 2: arterial-saphenous, n. 30; Group 3b: arterial- saphenous, n. 30).
Basing on this model, this is the formula that we obtained for the estimation of the predicted paCO2 from the saphenous pvCO2:
The Bland-Altman plot was subsequently generated to compare predicted values to the arterial correspondents. Figure 3 shows the agreement between predicted saphenous and arterial pCO2 (Groups 2 and 3b). The MD was 0.00 mmHg (95% CI: -0.70 to 0.70 mmHg), with 95% LOAs of –5.2 mmHg (95% CI: -6.40 to -4.00 mmHg) to 5.2 mmHg (95% CI: 4.00-6.40 mmHg). In order to evaluate the suitability of this model for our purposes, we should also take into account that residuals within the clinical predefined range (± 2.5 mmHg) are an acceptable approximation of paCO2. Results showed that the predicted arterial value was outside of these limits in the 33% of cases: 15% underestimating, 18% overestimating the true arterial value (Figure 3). In comparison, Figure 1B on untransformed values of saphenous pvCO2 shows that the residuals are outside the clinical limits in the 65% of cases, always overestimating the true paCO2.
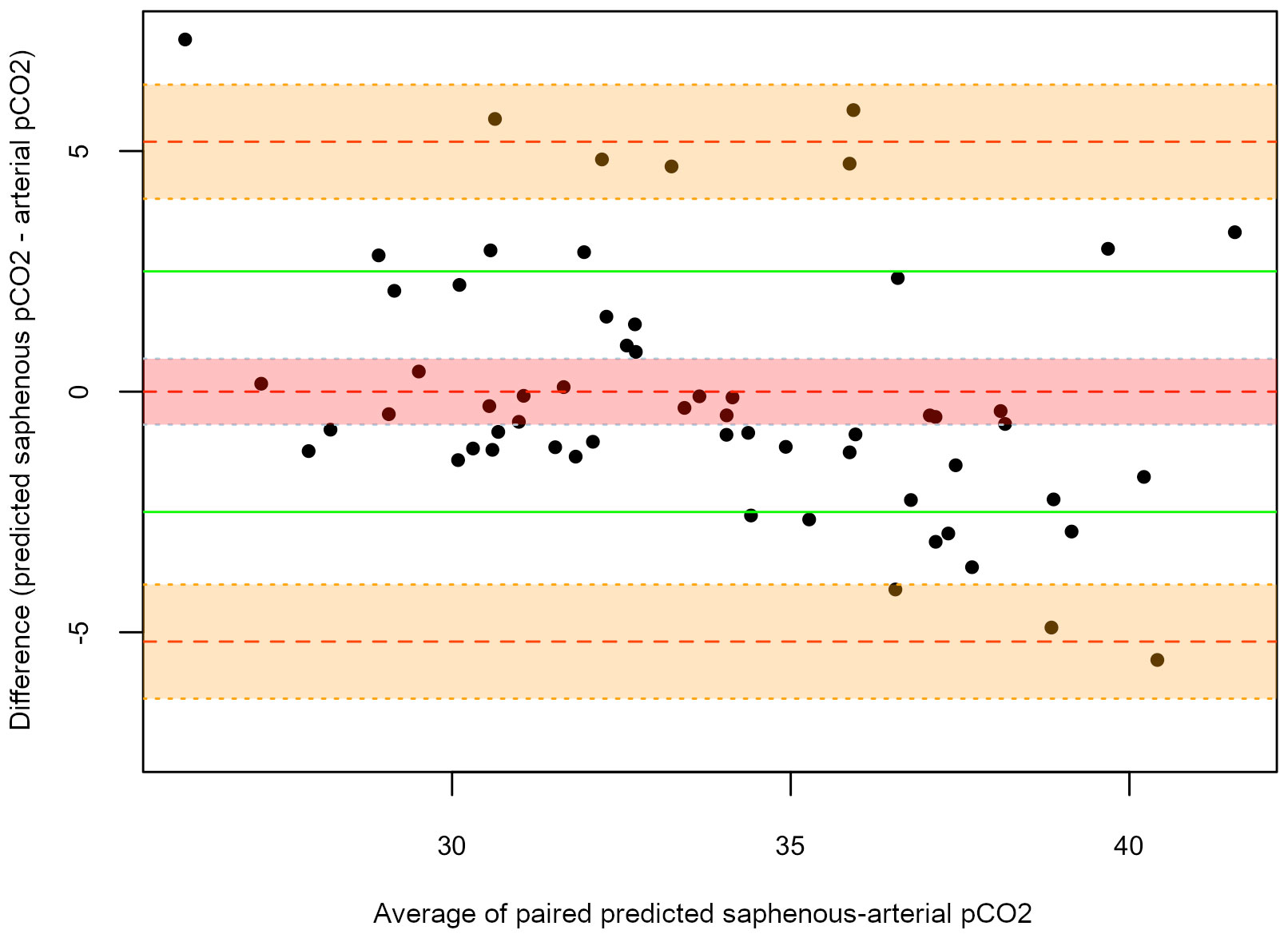
Figure 3 Bland-Altman plots for predicted-arterial pCO2. On the X-axis the average of the paired measures is represented, while on the Y-axis the difference between the two measures is reported. The central dotted red line corresponds to the MD; the upper and lower orange dotted lines correspond to the upper and lower LOAs, respectively; the green lines correspond to the clinical limits predefined by the authors (± 2.5 mmHg); the red shadowed area outlines a 95% CI for the mean difference; the orange shadowed areas outline a 95% CI for the upper and lower limits of agreement.
4 Discussion
4.1 Pearson correlation vs Bland-Altman plot analysis
In order to assess the agreement between two variables, correlation coefficient is often calculated. However, the coefficient measures the strength of a relation between two variables and estimates the linear correlation, not their agreement. Furthermore, correlation coefficient cannot explain whether any difference between two measurements is systematic or random. Finally, it is dependent on the range of variation of the variable (Bland and Altman, 1986; Van Stralen et al., 2008). Hence, Bland and Altman proposed a different method to assess agreement that goes into more detail in the investigation of correlation (Bland and Altman, 1986). The plot they designed is a scatter plot in which the difference between the paired measurement is plotted against their mean value and this allows assessing any systematic or random difference between values. Furthermore, the plot shows the variation of the values in respect of the upper and lower limits of agreement (95% LOAs), which are MD ± 1.96 SDs. Clinical limits, however, should be decided prior to the analysis and then confronted to the obtained LOAs. If these limits include the 95% LOAs, the two analyzed methods can be considered interchangeable (Bland and Altman, 1986; Van Stralen et al., 2008; Chung et al., 2019).
4.2 pH
Pearson correlation coefficients between venous pH, both jugular and saphenous, and arterial pH are strong (0.81 and 0.85, respectively). The Bland-Altman plots show good agreement between venous and arterial pH, which is comparable between jugular and saphenous values, since the LOAs are of the same width. Furthermore, compared with the clinical limits predefined by the authors in this study (± 0.1), the LOAs being included inside those limits suggests the interchangeability of venous - whether jugular or saphenous - and arterial pH. Results from this work agree with most of the studies that have been conducted in human (Bloom et al., 2014; Byrne et al., 2014; Nanjayya et al., 2020) and veterinary (Pang et al., 2009; Kadwa et al., 2022; Shiroshita et al., 2023) medicine. Variations of pH between venous and arterial values are not of clinical impact in healthy, anesthetized, mechanically ventilated dogs.
4.3 pCO2
Pearson correlation coefficients between pvCO2 and paCO2 are strong for saphenous sampling (0.79) but only moderate for jugular sampling (0.55). Furthermore, the Bland-Altman plots show that the venous and arterial values cannot be considered interchangeable since the mean difference is outside the clinical limits (± 2.5 mmHg), and pvCO2 tends to overestimate paCO2 (MD = 3.42 mmHg for jugular pvCO2; MD = 4.18 mmHg for saphenous pvCO2). Furthermore, LOAs are too wide for the interchangeability to be acceptable (jugular-arterial: -4.94 mmHg to 11.79 mmHg; saphenous-arterial: -1.90 mmHg to 10.26 mmHg). Results from this work reflect what has been reported in both human (Bloom et al., 2014; Byrne et al., 2014; Chung et al., 2019; Nanjayya et al., 2020) and veterinary (Tamura et al., 2015; Kadwa et al., 2022; Shiroshita et al., 2023) medicine, where the reported MD between venous and arterial pCO2 ranges between 4.15 mmHg (Byrne et al., 2014) and 4.41 mmHg (Bloom et al., 2014) in humans, and between 2.00 mmHg (Kadwa et al., 2022) and 5.80 mmHg (Tamura et al., 2015) in dogs, values that are superimposable to the ones that we found in our population.
On the other hand, the agreement between transformed saphenous pvCO2 and paCO2 shows an improvement, with a MD = 0.00 mmHg and narrower LOAs (–5.2 mmHg to 5.2 mmHg). The formula hereby proposed to transform saphenous pvCO2 has only a moderate predictive power (R-squared = 0.63), in fact only in the 67% of cases the mathematic transformation provides a transformed value of pvCO2 that agrees with paCO2 (since it is included inside the clinical acceptance interval of ± 2.5 mmHg). Basing on these results, authors suggest that, at present, the formula should be used with caution, since it has been derived from data acquired on healthy, anesthetized, mechanically ventilated patients, and its utility cannot be assessed yet. Nevertheless, this study could open the doors to further research with larger samples, and with the application of different statistical methods that are currently used to create prediction models, like neural networks or support vector machines. Indeed, the utilization of a formula could be an in-clinic easy, quick and useful instrument for the estimation of paCO2, especially in hospitalized patients that require a frequent monitoring of the respiratory function.
The reason why saphenous pvCO2 better correlates and agrees with paCO2 when compared to jugular pvCO2 has not been investigated in this study. In human literature, it has been demonstrated that peripheral venous blood for blood gas and acid-base assessment, especially peripheral venous pCO2, is influenced by local metabolism and tissue perfusion (Rang et al., 2002; Byrne et al., 2014). The authors’ main hypothesis is that the saphenous vein is more distal than the jugular vein, hence, the saphenous vein drains tissues with lower metabolism (especially in dogs under general anesthesia) when compared to the jugular vein. Therefore, as already reported in literature, saphenous pvCO2 could resemble paCO2 more than jugular pvCO2 (Shiroshita et al., 2023).
The timing of blood sampling and dogs’ recumbency were not considered in the statistical analysis of the present study. None of the patients included in the study experienced hypotension or hypertension during surgical procedures; hence, venous and arterial samples were obtained on well-perfused patients. In human medicine, the venous-to-arterial carbon dioxide partial pressure difference, defined as pCO2 gap, is considered a marker of tissue hypoperfusion and, in normal condition, is reported to range from 2 to 5 mmHg (Scheeren et al., 2018). The MDs between venous and arterial pCO2 reported in the present study were 3.42 mmHg for jugular and 4.18 mmHg for saphenous pvCO2-paCO2, respectively: MDs were included in the pCO2 gap normality range reported in human medicine (Scheeren et al., 2018). Therefore, this supports the fact that the dogs included in the study were not experiencing tissue hypoperfusion when blood sampling was performed. Nevertheless, LOAs were wide for both jugular and saphenous samples, suggesting that some dogs had pCO2 gaps higher than the normal reference range. However, our LOAs values are superimposable to those reported in Shiroshita et al. (2023) study; dogs included in their research were well-perfused, and all were positioned in lateral recumbency (Shiroshita et al., 2023). Hence, similarities among the results of the two studies suggest that individual differences could affect the pCO2 gap in well-perfused dogs, regardless of recumbency and timing of blood sampling.
4.4 Limitations
This study has some limitations. The small number of paired ABG-VBGs was the main limit, since it may have prevented the computing of a more precise formula though the univariate linear regression. Furthermore, the division of the population into three different groups did not allow to establish the relationship between jugular and saphenous pvCO2, since this analysis could only be conducted on 30 dogs. Samplings have been conducted on healthy, anesthetized, mechanically ventilated dogs, so the results hereby described are not yet applicable to awake patients, nor to patients affected by respiratory or cardiovascular diseases that could alter their alveolar gas exchange and their hemodynamic status. Lastly, the present study did not consider the effects of timing of blood sampling, the type of surgical procedure, and the animal positioning. Exploring their impact on venous and arterial blood gas variables could increase knowledge on this topic in veterinary medicine.
5 Conclusion
In conclusion, this preliminary study tried to assess the interchangeability of venous (both jugular and saphenous) and arterial pH and pCO2 in anesthetized, mechanically ventilated, healthy dogs. Venous blood gas, whether jugular or saphenous, can be used for the estimation of arterial pH since the differences between venous and arterial values are not of clinical impact. On the other hand, VBG cannot be used to reliably replace ABG in the analysis of pCO2. Saphenous pCO2 better correlates with paCO2, so sampling from this site is to be preferred; furthermore, the authors provide a conversion formula to obtain a predicted pCO2 which better agrees with paCO2. At the moment, the formula – applied on saphenous pCO2 – delivers a transformed value that agrees with paCO2 only in the 67% of cases in our dataset and is applicable to anesthetized, mechanically ventilated, healthy dogs. Nevertheless, this study could open the doors to further research to be conducted in larger samples, non-anesthetized dogs, and patients affected by different diseases. The formula could be, indeed, an in-clinic easy, quick and useful instrument for the estimation of paCO2, especially in hospitalized patients that require frequent monitoring.
Data availability statement
The raw data supporting the conclusions of this article will be made available by the authors, without undue reservation.
Ethics statement
The animal studies were approved by Institutional Ethical Committee for Animal Care at the University of Milan (72/2023). The studies were conducted in accordance with the local legislation and institutional requirements. Written informed consent was obtained from the owners for the participation of their animals in this study.
Author contributions
SG: Writing – review & editing, Data curation, Investigation, Writing – original draft, Methodology. DGa: Data curation, Investigation, Writing – review & editing, Methodology. AZ: Data curation, Writing – review & editing, Formal analysis, Methodology. PB: Writing – review & editing, Supervision, Validation. AC: Investigation, Data curation, Writing – review & editing. PD: Investigation, Data curation, Writing – review & editing. MB: Investigation, Data curation, Writing – review & editing. DGh: Investigation, Data curation, Writing – review & editing. CR: Investigation, Data curation, Writing – review & editing. DC: Investigation, Data curation, Writing – review & editing. CB: Conceptualization, Methodology, Supervision, Validation, Writing – review & editing. GR: Conceptualization, Methodology, Supervision, Writing – review & editing.
Funding
The author(s) declare financial support was received for the research, authorship, and/or publication of this article. The authors acknowledge the University of Milan for the publication of this research which was covered by the APC fund.
Conflict of interest
The authors declare that the research was conducted in the absence of any commercial or financial relationships that could be construed as a potential conflict of interest.
Publisher’s note
All claims expressed in this article are solely those of the authors and do not necessarily represent those of their affiliated organizations, or those of the publisher, the editors and the reviewers. Any product that may be evaluated in this article, or claim that may be made by its manufacturer, is not guaranteed or endorsed by the publisher.
References
Acierno M. J., Brown S., Coleman A. E., Jepson R. E., Papich M., Stepien R. L., et al. (2018). ACVIM consensus statement: Guidelines for the identification, evaluation, and management of systemic hypertension in dogs and cats. J. Vet. Intern. Med. 32, 1803–1822. doi: 10.1111/jvim.15331
Atalan G., Gunes V., Uzlu E., Yapar K. (2008). Comparison of arterial and venous blood gas values in conscious dogs and dogs under anesthesia induced by ketamine. Revue. Med. Vet. 159, 288–292.
Bland J. M., Altman D. G. (1986). Statistical methods for assessing agreement between two methods of clinical measurement. Lancet. 1, 307–310. doi: 10.1016/S0140-6736(86)90837-8
Bloom B. M., Grundlingh J., Bestwick J. P., Harris T. (2014). The role of venous blood gas in the emergency department: a systematic review and meta-analysis. Eur. J. Emerg. Med. 21, 81–88. doi: 10.1097/MEJ.0b013e32836437cf
Byrne A. L., Bennett M., Chatterji R., Symons R., Pace N. L., Thomas P. S. (2014). Saphenous venous and arterial blood gas analysis in adults: are they comparable? A systematic review and meta-analysis. Respirology. 19, 168–175. doi: 10.1111/resp.12225
Chung P. A., Scavone A., Ahmed A., Kuchta K., Bellam S. K. (2019). Agreement and correlation of arterial and venous blood gas analysis in a diverse population. Clin. Med. Iinsights Trauma Intensive Med. 10, 1–6. doi: 10.1177/1179560319845869
Hopper K., Powell L. L. (2013). Basics of mechanical ventilation for dogs and cats. Vet. Clin. North. Am. Small. Anim. Pract. 43, 955–969. doi: 10.1016/j.cvsm.2013.03.009
Ilkiw J. E., Rose R. J., Martin I. C. (1991). A comparison of simultaneously collected arterial, mixed venous, jugular venous and cephalic venous blood samples in the assessment of blood-gas and acid-base status in the dog. J. Vet. Intern. Med. 5, 294–298. doi: 10.1111/j.1939-1676.1991.tb03136.x
Kadwa A. R., Boustead K. J., Zeiler G. E. (2022). Agreement between arterial and jugular venous blood pH and its contributing variables in anaesthetized dogs with respiratory acidosis. Vet. Anaesth Analg. 49, 299–303. doi: 10.1016/j.vaa.2022.02.001
Kelly A. M. (2010). Review article: Can venous blood gas analysis replace arterial in emergency medical care. Emerg. Med. Australas. 22, 493–498. doi: 10.1111/j.1742-6723.2010.01344.x
Lumholdt M., Damgaard K. A., Christensen E. F., Leutscher P. D. C. (2020). Correction to: Mathematical arterialisation of saphenous venous blood gas for obtainment of arterial blood gas values: a methodological validation study in the clinical setting. J. Clin. Monit. Comput. 34, 387. doi: 10.1007/s10877-019-00314-y
Nanjayya V. B., McCracken P., Vallance S., Board J., Kelly P. J., Schneider H. G., et al. (2020). Arterio-VENouS Intra Subject agreement for blood gases within intensive care: The AVENSIS study. J. Intensive Care Soc 21, 64–71. doi: 10.1177/1751143719840259
Pang D. S. J., Allaire J., Rondenay Y., Kaartinen J., Cuvelliez S. G., Troncy E. (2009). The use of lingual venous blood to determine the acid-base and blood-gas status of dogs under anesthesia. Vet. Anaesth. Analg. 36, 124–132. doi: 10.1111/j.1467-2995.2008.00438.x
Rang L. C. F., Murray H. E., Wells G. A., Macgougan C. K. (2002). Can peripheral venous blood gases replace arterial blood gases in emergency department patients? CJEM. 4, 7–15. doi: 10.1017/s1481803500006011
Razi E., Nasiri O., Akbari H., Razi A. (2012). Correlation of arterial blood gas measurements with venous blood gas values in mechanically ventilated patients. Tanaffos. 11, 30–35.
Rees S. E., Toftegaard M., Andreassen S. (2006). A method for calculation of arterial acid-base and blood gas status from measurements in the saphenous venous blood. Comput. Methods Programs. Biomed. 81, 18–25. doi: 10.1016/j.cmpb.2005.10.003
Ricos C., Alvarez V., Cava F., Garcia-Lario J. V., Hernandez A., Jimenez C. V., et al. (1999). Current databases on biological variation: pros, cons and progress. Scand. J. Clin. Lab. Invest. 59, 491–500. doi: 10.1080/00365519950185229
Scheeren T. W. L., Wicke J. N., Teboul J. L. (2018). Understanding the carbon dioxide gaps. Curr. Opin. Crit. Care 24, 181–189.24. doi: 10.1097/MCC.0000000000000493
Shiroshita Y., Tanaka R., Shimizu M., Yamane Y. (2023). Cephalic and saphenous venous blood collected by continuous heating of the paws compared with arterial blood for measurement of blood gas values in well-perfused dogs. J. Vet. Intern. Med. 37, 1376–1389. doi: 10.1111/jvim.16745
Tamura J., Itami T., Ishizuka T., Fukui S., Miyoshi K., Sano T., et al. (2015). Jugular venous blood gas and acid-base status in conscious dogs and cats. J. Vet. Med. Sci. 77, 865–869. doi: 10.1292/jvms.14-0503
Teixeira Neto F. J., Carregaro A. B., Mannarino R., Cruz M. L., Luna S. (2002). Comparison of a sidestream capnograph and a mainstream capnograph in mechanically ventilated dogs. J. Am. Vet. Med. Assoc. 221, 1582–1585. doi: 10.2460/javma.2002.221.1582
Van Stralen K. J., Jager K. J., Zoccali C., Dekker F. W. (2008). Agreement between methods. Kidney Int. 74, 1116–1120. doi: 10.1038/ki.2008.306
Waddell L. S. (2000). Direct blood pressure monitoring. Clin. Tech. Small. Anim. Pract. 15, 111–118. doi: 10.1053/svms.2000.18292
Keywords: arterial blood gas, venous blood gas, respiratory function, metabolic status, dogs
Citation: Ghilardi S, Gamba D, Zanaboni AM, Brambilla PG, Casarrubea A, Drummer P, Balsamino M, Ghezzi D, Ricci C, Caristi D, Bussadori CM and Ravasio G (2024) Arterial pCO2 prediction using saphenous pCO2 in healthy mechanically ventilated dogs. Front. Anim. Sci. 4:1291233. doi: 10.3389/fanim.2023.1291233
Received: 08 September 2023; Accepted: 15 December 2023;
Published: 10 January 2024.
Edited by:
Mohan Mondal, ICAR-National Dairy Research Institute, Kalyani, IndiaReviewed by:
Dario Floriano, University of Pennsylvania, United StatesChiara Del Prete, University of Naples Federico II, Italy
Chiara De Gennaro, University of Florida, United States
Copyright © 2024 Ghilardi, Gamba, Zanaboni, Brambilla, Casarrubea, Drummer, Balsamino, Ghezzi, Ricci, Caristi, Bussadori and Ravasio. This is an open-access article distributed under the terms of the Creative Commons Attribution License (CC BY). The use, distribution or reproduction in other forums is permitted, provided the original author(s) and the copyright owner(s) are credited and that the original publication in this journal is cited, in accordance with accepted academic practice. No use, distribution or reproduction is permitted which does not comply with these terms.
*Correspondence: Paola G. Brambilla, cGFvbGEuYnJhbWJpbGxhQHVuaW1pLml0
†These authors have contributed equally to this work and share first authorship