- 1AQUAM, Animal Science Research Program, Federal University of Rio Grande do Sul – UFRGS, Porto Alegre, RS, Brazil
- 2School of Fisheries, Aquaculture, and Aquatic Science, Auburn University, Auburn, AL, United States
- 3Department of Fisheries and Aquaculture, Federal Rural University of Pernambuco, Recife, PE, Brazil
- 4Southeastern Cooperative Fish Parasite and Disease Laboratory, School of Fisheries, Aquaculture and Aquatic Sciences, College of Agriculture, Auburn University, Auburn, AL, United States
- 5School of Aquaculture and Aquatic Sciences, College of Agriculture, Community, and the Sciences, Kentucky State University, Frankfort, KY, United States
- 6Phibro Animal Health Corporation, Teaneck, NJ, United States
Mycotoxins are fungal secondary metabolites that can adversely affect animals consuming contaminated feeds. This 71-day feeding trial was conducted to assess the effects of dietary deoxynivalenol plus zearalenone (DON+ZEN = 1.6 + 0.3 ppm), and fumonisins (FUM = 15 ppm), and three adsorbent additives on the production performance, hematological parameters, and liver histology of juvenile Nile tilapia. A mycotoxin-free diet (Control) formulated to contain 35% protein and 8% lipid was spiked with either DON+ZEN or FUM using contaminated corn meals replacing portions of non-spiked corn. Subsequently, three out of four DON+ZEN- and FUM-spiked diets were supplemented (0.5%) with an adsorbent. The research was carried out in a recirculating water system (2,500 L) with a controlled temperature of (25.9 ± 1.1°C), feeding was carried out twice a day at rates ranging from 5 to 8%. The experiment included nine treatments with five replications, each experimental unit consisting of an aquarium with a useful volume of 75-L and fifteen juvenile Nile tilapia (average initial weight of 4.0 ± 0.1 g). Mycotoxin-spiked diets without added adsorbent supported lower final biomass and survival of Nile tilapia relative to control and adsorbent containing diets (P ≤ 0.05). Histological examinations revealed liver inflammation evidenced by lymphocytic infiltration adjacent to pancreatic tissue in fish fed mycotoxin-spiked diets without added adsorbent. Mycotoxin contamination significantly increased HSI (hepato somatic index), which was reverted to the Control value or reduced further by adsorbent addition. Lowest and intermediate hematocrit values were observed in groups fed mycotoxin-spiked diets without and with added adsorbents, respectively. Our results reinforce the importance of using adsorbents and the need to investigate the effect of sub-lethal concentrations of mycotoxins in aquaculture feeds.
Introduction
Diet influences the behavior, health, physiological functions, reproduction, and growth of aquatic organisms (National Research Council (NRC), 2011). While maintaining the quality of raw materials and feeds from point of origin to end use is a complex task (Patriarca and Pinto, 2017; Nogueira et al., 2020; Taroncher et al., 2021), evidences of mycotoxin contamination of feedstuffs and aquaculture feeds in recent years have been alarming, drawing increased attention worldwide (Anater et al., 2016; Gonçalves et al., 2020; Oliveira and Vasconcelos, 2020; Bashorun et al., 2023). Heightened concerns over mycotoxin contamination of feed (and food) have coincided with climate changes including higher temperatures, which are expected to favor growth of mycotoxigenic fungi leading to increased contamination of crops (both before harvest and during storage) and finished feeds (Paterson and Lima, 2010; Battilani et al., 2016; Bashorun et al., 2023).
Mycotoxins are toxic secondary metabolites produced by a diversity of fungi that contaminate agricultural crops and feeds (Zychowski et al., 2013; Marroquín-Cardona et al., 2014; Guan et al., 2023). The mycotoxin-producing fungi Aspergillus, Penicillium and Fusarium produce fumonisins (FUM), deoxynivalenol (DON), zearalenone (ZEN) and aflatoxins (AFL), all mycotoxins that are common in aquaculture feeds and that represent the greatest risks for aquaculture (Anater et al., 2016; Nogueira et al., 2020; Taroncher et al., 2021). Research reports on these mycotoxins highlight reduction of protein synthesis, modification of lipid metabolism and mitochondrial activity, and due weakening of the immune system in orally exposed animals (Wang et al., 1991; Döll et al., 2010; Tola et al., 2015; Patriarca and Pinto, 2017; Abdel-Tawwab et al., 2020; Zahran et al., 2020; Ahmed et al., 2022; Smaoui et al., 2023).
Efforts to control feed-driven mycotoxicosis in animals include management strategies that begin before crop establishment and extend to rigorous testing for mycotoxins in feed mills and adoption of mycotoxin mitigation additives for use in feeds (Boudergue et al., 2009; Zhu et al., 2016; Ahmed et al., 2022). Some of the most widely used additives for mycotoxin detoxification of feeds consist of adsorbents based on clays, micronized yeast, plant fibers, and polymers, which alleviate adverse effects of ingested mycotoxins by preventing absorption into blood and organs by complexation. One common adsorbent is bentonite, a clay with a wide variety of industrial uses (Chestnut et al., 1992; Avantaggiato et al., 2005; Hussain et al., 2017). The use of clays is a viable technical and economical solution, as they are abundantly available and can be obtained at reasonable costs. There is considerable interest in validating the efficacy of these products in aquaculture feeds, as well as evaluating the effect of different mycotoxin types on fish health (Nogueira et al., 2020; Oliveira and Vasconcelos, 2020; Smaoui et al., 2023).
In general, warm-water fish are considered more tolerant to dietary mycotoxins than cold-water counterparts, notably rainbow trout (Halver and Goldblatt, 1969; Hooft et al., 2019; Koletsi et al., 2023). However, herbivorous and omnivorous warm water fish are likely more exposed to dietary mycotoxins because are fed diets with higher levels of plant-based ingredients, especially cereal-grain by-products that usually contain higher levels of mycotoxins. In addition, species produced in tropical regions might have higher risks of exposure to mycotoxin contaminated feeds given the more suitable environmental conditions for fungal growth. Tilapia (Oreochromis spp.), particularly the Nile tilapia (O. niloticus), is one of such species largely produced in tropical and temperate regions.
Although Nile tilapia is a hardy fish in many aspects including tolerance to mycotoxins, dietary concentrations of DON/ZEN (< 0.1 to ~ 1 ppm each; Tola et al., 2015) and FUM (40 - 150 ppm; Tuan et al., 2003) have been found to adversely affect growth performance of juvenile fish. Therefore, considering the need for additional information on the potentially harmful effects of dietary mycotoxins on chronically exposed fish, the objectives of this study were to evaluate the responses of juvenile Nile tilapia to practical diets spiked with DON + ZEN or FUM and the potential beneficial effects of mycotoxin adsorbent additives.
Materials and methods
This study followed a completely randomized design including nine dietary treatments with five replicates each (Table 1). Mycotoxin-contaminated corn and mycotoxin adsorbents were sourced from Phibro Animal Health Corporation, Teaneck, NJ, USA.
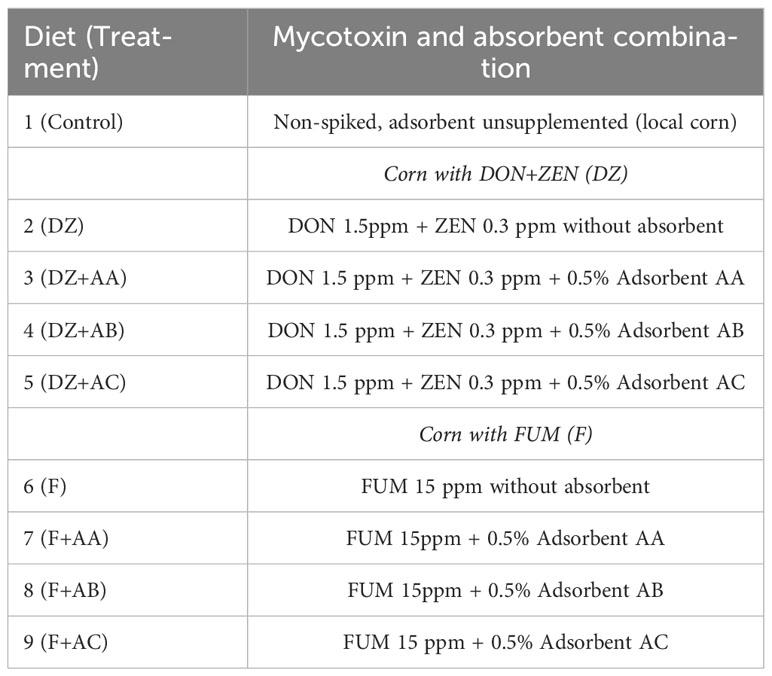
Table 1 Outline of the nine-treatment experimental design evaluating dietary deoxynivalenol + zearalenone (DON+ZEN), fumonisins (FUM), and absorbents AA, AB, and AC.
Experimental diets
All experimental diets were formulated to contain 35% crude protein and 8% lipids and meet the nutritional requirements of Nile tilapia (National Research Council (NRC), 2011; Table 2). Two batches of corn contaminated with mycotoxins were used, one spiked with deoxynivalenol and zearalenone (DON+ZEN; DZ), and another with fumonisins (FUM; F). Inclusion of the contaminated corn in the experimental diets was performed by replacing a portion or the entirety of a locally sourced, non-spiked corn in the overall control (Control) diet. Three different types of commercial adsorbents (designated as adsorbent A (AA), adsorbent B (AB) and adsorbent C (AC)) were evaluated. AA and AB were sodium bentonites containing hydrated calcium aluminosilicates mined from two different locations, while AC consisted of a proprietary blend of adsorbent AB with Saccharomyces cerevisiae beer yeast. Inclusion of mycotoxins and adsorbents in the Control diet originated eight test diets (Table 2): two adsorbent unsupplemented and mycotoxin-contaminated control diets (DZ = 1.5 ppm of DON + 0.3 ppm of ZEN; and F = 15 ppm of FUM), each supplemented with one of the adsorbents evaluated (DZ+AA, AB, or AC; F+AA, AB, or AC). The inclusion levels of DON+ZEN and FUM in the experimental diets were based on reported sub-lethal concentrations for Nile tilapia juveniles (Tuan et al., 2003; Oliveira and Vasconcelos, 2020, respectively), while the adsorbents were included following the manufacturer’s recommended levels.
All diets were prepared at the Auburn University E.W. Shell Fisheries Center, Auburn, AL, USA where the research was conducted. In short, pre-ground dry ingredients and oil were weighed and then mixed in a food mixer (Hobart Corporation, Troy, OH, USA) for 15 min. Hot water was added while mixing for the diet to attain a consistency appropriate for pelleting. Each diet was screw pressed through a 3-mm die plate. After pelleting, diets were dried using standard procedures to a moisture content of <10% and stored at 4 °C until fed.
Levels of mycotoxins in the finished diets (Table 3) were analyzed using liquid chromatography-mass spectrometry (LC/MS) and/or liquid chromatography coupled to sequential mass spectrometry LC/MS/MS following AOAC 2008.02 (Midwest Laboratories in Omaha, Nebraska). As expected, concentrations of DON (1.6 ppm) and ZEN (0.289 to 0.347 ppm) in the DZ diets and of FUM in the F diets were close to the target levels. Trace amounts of DON, FUM, ochratoxin, and ZEN were detected in the Control diet, while AFL was detected in DZ and F diets and FUM in all DZ diets. This was also expected since trace amounts of mycotoxins are usually present in ingredients (specially cereal-grains) used in practical formulations (Tola et al., 2015; Hooft and Bureau, 2017). However, these levels were much lower than those generally considered as safe for farmed animals, including fish (Tuan et al., 2002; Manning et al., 2003; Tuan et al., 2003; Hooft et al., 2019), making potential influences on the responses of the Nile tilapia to the Control and mycotoxin-spiked diets in this study very unlikely.
Fish and rearing conditions
Sex-reversed Nile tilapia were acquired from Spring Genetics, Miami, FL, USA. Following conditioning to local conditions, groups of fifteen tilapia juveniles (mean initial weight 4.0 ± 0.1 g) were stocked into forty-four 75-L aquaria connected to a 2,500-L indoor recirculating system. Rearing water temperature and dissolved oxygen were monitored daily. Water temperature (mean ± standard deviation = 25.9 ± 1.1 oC) was maintained using a submerged 3,600-W heater (Aquatic Eco-Systems Inc., Apopka, FL, USA). An airline connected to a central regenerative blower was used to maintain the dissolved oxygen near saturation (5.94 ± 1.09 mg/L) using air stones in each aquarium and sump tank. The pH (7.93 ± 0.58), total ammonia nitrogen (TAN; 0.089 ± 0.105 mg/L) and nitrite nitrogen (0.063 ± 0.087 mg/L) were measured twice per week and also maintained within optimum ranges for Nile tilapia. Timer-controlled photoperiod was set at 14 h light and 10 h dark. To prevent potential buildups of mycotoxins in the recirculating water, filter backwashes and water exchanges were performed on a regular basis.
During the experimental period (71 days), fish in each aquarium were fed one of the randomly assigned experimental diets twice daily at fixed rates ranging from 5 to 8% of body weight/day. Daily rations were adjusted every 15 days by group weighing fish in each aquarium. The glass aquaria allowed feeding activity to be monitored at each feeding, and no leftover feed was observed during the experimental period.
Sampling and data acquisition
Production performance metrics
At the end of the growth trial all fish from each aquarium were counted and group weighed after an overnight fasting to determine biomass gain, weight gain, survival, and feed conversion ratio using the following equations:
Biomass gain (g) = final fish biomass – initial fish biomas;
Weight gain (%) = [(final body weight – initial body weight)/(initial body weight)] × 100;
Survival (%) = final population/initial population × 100;
Feed conversion ratio (FCR) = feed fed/weight gain.
Additionally, four fish per aquarium were randomly sampled and sedated using 100 ppm buffered tricaine methanesulfonate (MS-222) for the collection of blood, and determination of length and weight of each fish. After this procedure, fish were euthanized and their livers dissected and weighed. The liver weight was used to compute hepatosomatic index (HSI) as follows:
HSI (%) = [liver weight/body weight] × 100.
Hematological analyses
Blood from four fish per aquarium was drawn from the caudal vasculature using 1-mL syringes with 21-gauge needles, then aliquoted into two 3-mL microtubes. The first aliquot was collected with 10% EDTA anticoagulant to measure hematocrit, as described by Reitman and Frankel (1957). The second aliquot, without anticoagulant, was centrifuged at 3,000 × g for 10 min and the serum was collected into 1.5 mL microtubes and stored at -80°C pending the following analyses performed using a VetScan Diagnostic Profile II (Abaxis, Inc., Union City, CA): alkaline phosphatase (ALP); alanine aminotransferase (ALT); bile acids (BA); total bilirubin (TBIL); albumin (ALB); urea nitrogen (BUN), and cholesterol (CHOL).
Liver histology
The intact liver of one fish per aquarium was excised immediately after spinal severance. After fixation in 10% neutral buffered formalin for 48 h, the dissected livers were transferred to 70% ethanol until processed for histological examination. A 0.5 cm long portion of liver tissue was cut from each sample to fit into tissue processing cassettes, yielding 44 portions of tissue that were rinsed in distilled water for 2 h, dehydrated in an ethanol, sectioned at 4 µm, and stained with Gill’s 2 hematoxylin and eosin (Luna, 1968). Ten slides with approximately 20 sections per slide were cut from each block, yielding >8,800 sections on 440 slides. Five slides per block were evaluated for pathology. Slides were evaluated by comparing histological features of the treatment groups to that of the Control diet-fed groups.
Statistical analysis
Due to discrepant production performance relative to the other four replicates, data from one replicate each of treatments DZ+AA and DZ+AB were flagged as outliers and were excluded from the analyzed data. All remaining data were subjected to an one-way ANOVA. When significant (P < 0.05) treatment effects were detected, the Student-Newman-Keuls multiple comparison test was used to identify differences among treatment means. All statistical analyses were conducted using the Statistical Analysis System for windows (SAS Institute, Cary, NC).
Results
Production performance
There were significant differences for biomass gain, percentage survival and HSI among fish in different dietary treatments (Table 4). Survival and biomass gain significantly decreased in fish fed the DZ diet when compared to the Control-fed fish. Addition of the adsorbents AA, AB and AC to DZ supported biomass gain and survival similar to the Control-fed fish (P > 0.05). Feeding the DZ diet significantly increased HSI of Nile tilapia relative to Control-fed fish, whereas a significant reduction in HSI was observed when adsorbent AC was included in the DZ diet. Inclusion of FUM in the F diet significantly reduced biomass gain and survival of the Nile tilapia relative to Control-fed groups, whereas addition of the adsorbents AB and AC, or all absorbents to the F diet supported biomass gain and survival similar to Control-fed groups, respectively (P > 0.05). The HSI of tilapia fed the F+AC diet was significantly lower than groups fed other F diets and the Control. The final weight, weight gain and FCR of Nile tilapia were unaffected by the experimental diets.
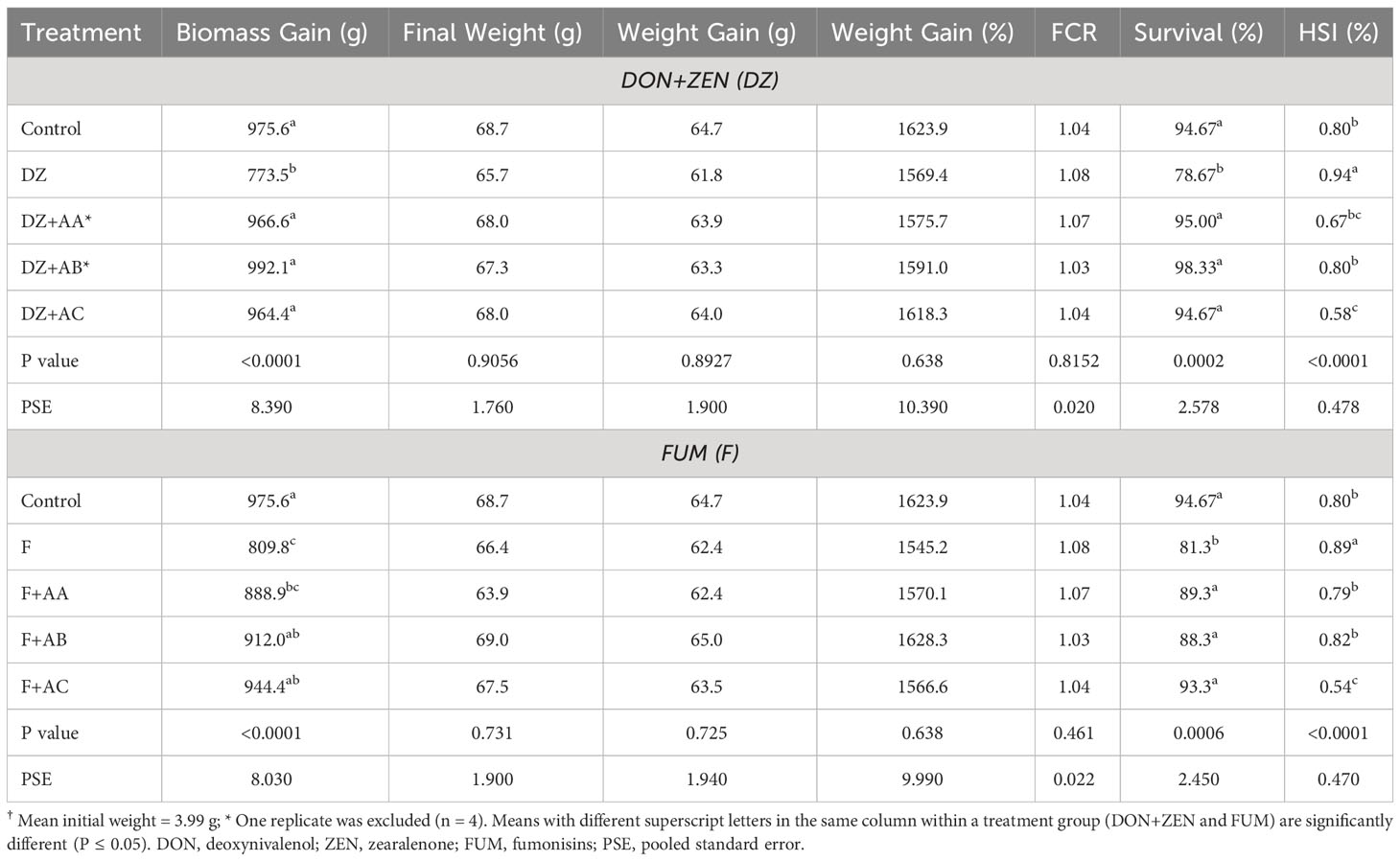
Table 4 Production performance and hepatosomatic index (HSI) of the Nile tilapia† fed the experimental diets for 71 days.
Hematological parameters
Of all hematological analyses performed in the experimental fish, significant dietary effects were only found for hematocrit (Table 5). While all test diets supported lower hematocrit in comparison to the Control diet, the addition of the adsorbents in DZ and F diets significantly increased it from 34.9 and 35.1% to as high as 38.8%, respectively.
Liver histology
Histological sections of livers from all Nile tilapia from the Control groups (Figure 1A) displayed normal histological features (Ferguson, 2006) and served as references for comparison to the treatment groups. Contrastingly, fish fed all F diets (regardless of adsorbent inclusion) and the DZ diet, displayed liver inflammation characterized by lymphocytic infiltration adjacent to exocrine pancreatic tissues (Figure 1B). No lympoocytic infiltration or any pathological changes were observed in fish fed the Control and DZ diets with added adsorbents, showing similar histological appearance to Control-fed groups (Figure 1A).
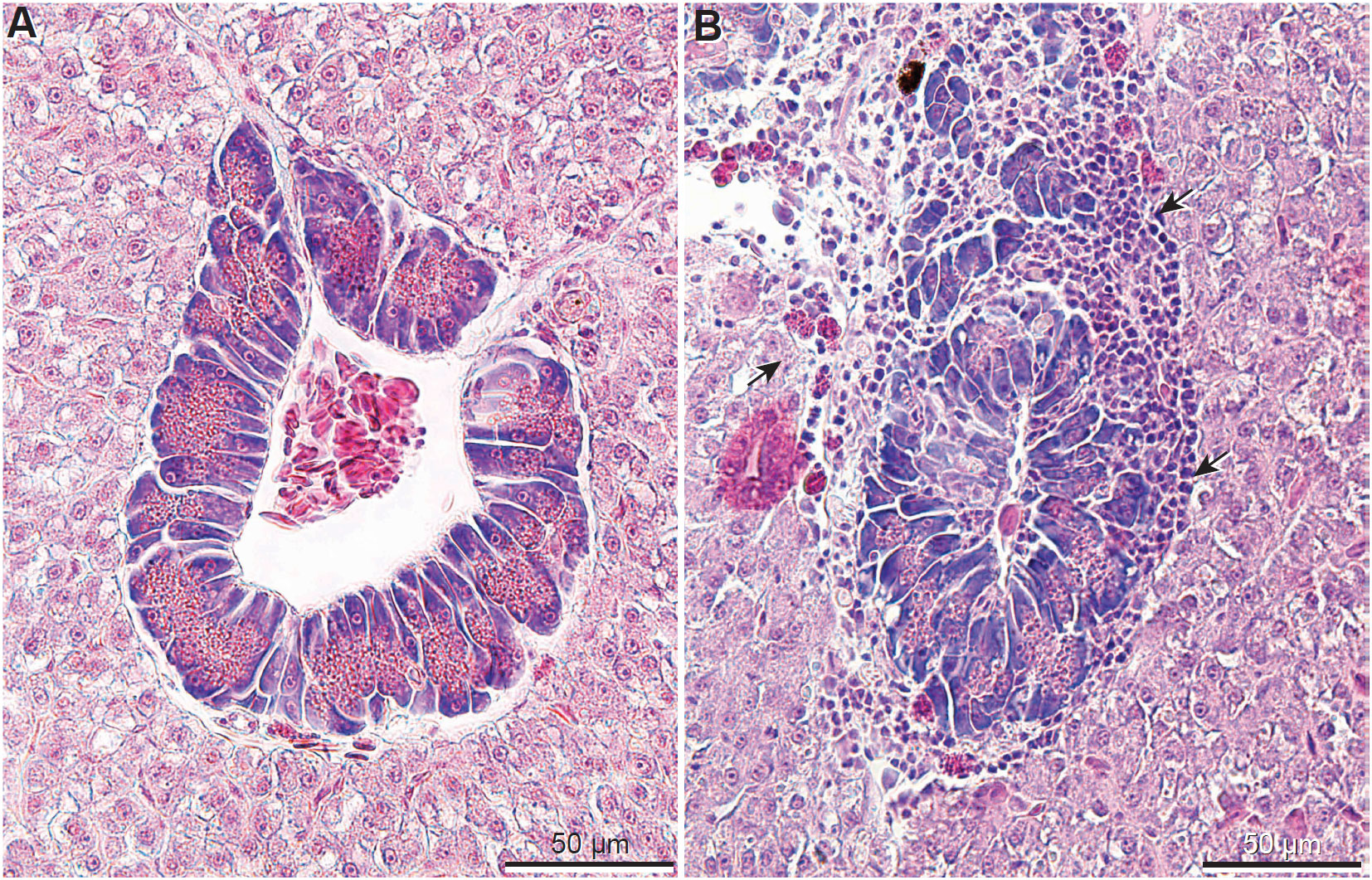
Figure 1 Liver histological sections (hematoxylin and eosin) of the Nile tilapia: (A) Liver from fish fed the Control diet showing normal structure of hepatocytes and exocrine pancreatic tissue; (B) Example of liver from fish fed DZ (DON 1.5 ppm + ZEN 0.3 ppm, without absorbent) and F (FUM = 15 ppm, without absorbent; shown above) diets showing lymphocytes adjacent exocrine pancreatic tissue (arrows).
Discussion
In recent years, contamination of feedstuffs and aquaculture feeds with mycotoxins and their effects in farmed fish have received considerable attention (Vila-Donat et al., 2018; Gonçalves et al., 2020; Oliveira and Vasconcelos, 2020), and so have ways of attenuating their harmful effects (Olokkaran and Mathew, 2020; Zahran et al., 2020). Despite great research efforts worldwide, several aspects of mycotoxin contaminations and strategies for preventing/mitigating mycotoxicosis in farmed fish, such as upper dietary limits for different mycotoxins and effectiveness of mycotoxin mitigating additives, remain undefined for several species. Nevertheless, it is a reality that mycotoxin-contaminated diets can adversely affect the production performance and health of fish (Greeff-Laubscher et al., 2020), which is corroborated by the findings of the present study demonstrating significant reductions in final biomass and survival of Nile tilapia fed diets contaminated with sub-lethal levels of some of the most economically relevant mycotoxins, especially in the absence of adsorbent additives.
Adverse effects of dietary DON+ZEN on the performance and of Nile tilapia were observed in the present study. Accordingly, Gonçalves et al. (2018) reported decreases in growth performance of rainbow trout (Oncorhynchus mykiss) fed diets containing 1.1 and 2.7 ppm of DON for 50 days or 0.3 ppm of DON for 168 days. Döll et al. (2010) also found that dietary DON concentrations above 0.3 ppm negatively affected the performance of Atlantic salmon (Salmo salar). Hooft et al. (2019) tested the effects of DON at concentrations of 0.1, 0.7 and 1.3 ppm in diets for Nile tilapia and rainbow trout, reporting deleterious effects on trout performance at all concentrations but only at 1.3 ppm for Nile tilapia. As with other warm-water species such as channel catfish (Ictalurus punctatus; Jantrarotai and Lovell, 1990), Nile tilapia displays relatively higher tolerance to dietary mycotoxins (Tuan et al., 2002; Hooft et al., 2019) than rainbow trout (Halver and Goldblatt, 1969; Hooft et al., 2011).
Similarly to the effects of DON+ZEN, the responses of Nile tilapia fed FUM-contaminated diets in this study are in agreement with previous findings by Tuan et al. (2003), who verified negative effects in performance parameters of Nile tilapia fry fed diets containing FUM at 10 ppm. Similar effects were observed in other fish species including channel catfish (Ictalurus punctatus) and common carp (Cyprinus carpio), whereby dietary FUM at ~ 10 and 20 ppm (respectively) adversely affected performance (Lumlertdacha et al., 1995). The adverse effects of FUM in animals has been associated with alterations in sphingolipid biosynthesis. The mechanism of action seems to be linked to the enzyme N-acyltransferase which, when inhibited by FUM in the endoplasmic reticulum, leads to the toxic accumulation of free sphinganine and sphingosine in cells (Wang et al., 1991).
Adsorbents directly bind mycotoxins decreasing their absorption in the gastrointestinal tract (Huwig et al., 2001), but adsorbent efficiency varies among different adsorbing materials as well as among different mycotoxins. Inorganic and/or yeast-based mycotoxin sequestering additives show relatively lower efficiency against DON when compared to the adsorption of AFL, ZEA, and FUM (Avantaggiato et al., 2005; Kong et al., 2014; Holanda and Kim, 2021). However, even when inorganic adsorbents were tested in DON contaminated feeds, improvements in growth, feed intake, and feed efficiency were observed in pigs (Holanda and Kim, 2021), corroborating the results of this study. In addition, it is possible that a more efficient adsorption of ZEA in the DZ diets contributed to the positive effects observed in Nile tilapia in this study. Overall, our findings indicate that the adsorbents attenuated the negative effects of these mycotoxins on juvenile Nile tilapia, in agreement with findings of Zychowski et al. (2013) and Hussain et al. (2017) when a clay-based adsorbent was tested in diets contaminated with AFL-B1. The efficacy of mycotoxin adsorbents have also been demonstrated in other animals and in vitro assays, showing an overall decrease in adverse effects (Solfrizzo et al., 2001; Avantaggiato et al., 2005; Di Gregorio et al., 2014; Selim et al., 2014; Vila-Donat et al., 2018). Further studies covering economic analyses of the economic and environmental impacts of using mycotoxin adsorbents in commercial feeds are warranted.
The HSI is a somatic index commonly used to indicate differences in dietary nutrient/energy partitioning, as well as adverse effects including liver inflammation caused by mycotoxins (Mommsen, 2001). Accordingly, in this study we found a greater HSI in tilapia fed the DZ and F diets, whereas the addition of the adsorbents in both diets lowered HSI values, especially for adsorbent AC (Table 4). Tuan et al. (2003) found that after eight weeks feeding Nile tilapia (2.7 g) FUM-containing diets, concentrations above 40 ppm caused substantial changes in the liver. Lumlertdacha et al. (1994) also observed altered livers in catfish after 10 weeks of exposure to dietary FUM at 20 ppm. In the present study, changes in the liver of Nile tilapia were found after 71 days of consuming the DZ (DON+ZEN = 1.6 ppm and 0.3 ppm, respectively) and F (FUM = 15 ppm) diets. However, addition of any of the adsorbents in the DZ diets prevented noticeable pathological changes in the livers based on the liver histology of Control-fed fish, indicating the effectiveness of these additives in reducing/preventing absorption of mycotoxins in the gut.
As with other animals, hematological and biochemical parameters are widely used in the evaluation of nutritional and health status, as well as adaptation to challenging environmental conditions (Fazio et al., 2013; Faggio et al., 2014; Abdel-Tawwab et al., 2015; Sehonova et al., 2018; Burgos-Aceves et al., 2019). In the present study, neither dietary mycotoxins nor adsorbents affected the assessed hematological parameters (P>0.05), but the observed differences in hematocrit indicate deleterious effects of the dietary mycotoxins. Similar observations of reduced hematocrit in Nile tilapia after receiving diets containing FUM and moniliformin for eight weeks were reported by Tuan et al. (2003). Abdel-Tawwab et al. (2020) also reported decreased hematocrit values in European sea bass (Dicentrarchus labrax) fed diets with ZEN. The mycotoxin toxicity might have induced a hypochromic macrocytic anemia, which inhibits red blood cell enlargement (Abdel-Tawwab et al., 2020). Other parameters, such as BUN analysis, are mainly associated with gill and liver diseases (Stoskopf, 1993), but there were no statistical differences between the treatments in this experiment.
In the present study, the dietary concentrations of mycotoxins were well above those reported for commercial feeds for both DON+ZEN and FUM. Pietsch et al. (2014) reported a wide variation of DON (0.066 to 0.825 ppm) and ZEN (0.003 to 0.051 ppm), and ~3.42 ppm of FUM in commercial feeds. Therefore, our study not only highlight the deleterious effects of the evaluated mycotoxins, but also demonstrate the potential benefits of using commercially available additives for prevention of potential mycotoxicosis. In addition, adverse effects arising from chronic exposure to relatively lower levels of dietary mycotoxin may also occur as aspects such as health status, environmental conditions, and exposure period to contaminated feeds may influence the fish’s responses to the toxins (Gonçalves et al., 2020; Oliveira and Vasconcelos, 2020).
In summary, exposure of juvenile Nile tilapia to dietary DON+ZEN and FUM at 1.6 + 0.3 ppm and 15 ppm, respectively, during a 71-day period adversely affects production performance and survival of the fish. Inclusion of adsorbent additives in the diets may help in attenuating/preventing adverse effects driven by mycotoxin contamination.
Data availability statement
The raw data supporting the conclusions of this article will be made available by the authors, without undue reservation.
Ethics statement
The animal study was approved by School of Fisheries, Aquaculture, and Aquatic Science, Auburn University, complying with regulations on the use of animals (approval code 20183337). The study was conducted in accordance with the local legislation and institutional requirements.
Author contributions
DF: Conceptualization, Data curation, Formal Analysis, Investigation, Methodology, Project administration, Resources, Software, Writing – original draft, Writing – review & editing. SP: Conceptualization, Data curation, Formal Analysis, Methodology, Writing – review & editing. SK: Conceptualization, Formal Analysis, Investigation, Writing – review & editing. SB: Conceptualization, Data curation, Investigation, Methodology, Writing – review & editing. WR: Conceptualization, Methodology, Writing – review & editing. DN: Funding acquisition, Methodology, Resources, Supervision, Writing – review & editing. DD: Conceptualization, Data curation, Investigation, Methodology, Project administration, Resources, Supervision, Validation, Writing – review & editing.
Funding
The author(s) declare financial support was received for the research, authorship, and/or publication of this article. This research was funded by the Alabama Agricultural Experiment Station, the Hatch program (ALA016-08027) of the National Institute of Food and Agriculture, U.S. Department of Agriculture and Phibro Animal Health Corporation. Scholarships for DF and SP were provided by the National Council for Scientific and Technological Development (CNPq; CNPq-204884/2018-7) and the Brazilian Federal Foundation for Support and Evaluation of Graduate Education (CAPES; CAPES-PRINT-88887.368344/2019-00), respectively.
Acknowledgments
The authors thank those who have taken time to critically review this manuscript as well as those who helped support research at the E.W. Shell Research Station, School of Fisheries, Aquaculture and Aquatic Sciences, Auburn University. Special thanks to students and staff who helped maintain the daily management during the trials. Mention of trademark or proprietary product does not constitute an endorsement of the product by Auburn University and does not imply its approval to the exclusion of other products that may also be suitable.
Conflict of interest
Author DN was employed by the company Phibro Animal Health Corporation, who assisted in sourcing mycotoxin loaded ingredients.
The remaining authors declare that the research was conducted in the absence of any commercial or financial relationships that could be construed as a potential conflict of interest.
Publisher’s note
All claims expressed in this article are solely those of the authors and do not necessarily represent those of their affiliated organizations, or those of the publisher, the editors and the reviewers. Any product that may be evaluated in this article, or claim that may be made by its manufacturer, is not guaranteed or endorsed by the publisher.
References
Abdel-Tawwab M., Khalifa E., Diab A. M., Khallaf M. A., Abdel-Razek N., Khalil R. H. (2020). Dietary garlic and chitosan alleviated zearalenone toxic effects on performance, immunity, and challenge of European sea bass, Dicentrarchus labrax, to Vibrio alginolyticus infection. Aquacult. Int. 28, 493–510. doi: 10.1007/s10499-019-00477-0
Abdel-Tawwab M., Sharafeldin K. M., Mosaad M. N. M., Ismaiel N. E. M. (2015). Coffee bean in common carp, Cyprinus carpio L. diets: effect on growth performance, biochemical status, and resistance to waterborne zinc toxicity. Aquaculture 448, 207–213. doi: 10.1016/j.aquaculture.2015.06.010
Ahmed S. A. A., Nada H. S., Elsheshtawy H. M., Ibrahim S. M., Fahmy E. M., Khedr M. H. E., et al. (2022). Comparative antitoxic potency of honey and natamycin-supplemented diets against aflatoxicosis and their influences on growth, serum biochemistry, immunohistochemistry, and residual deposition in Nile tilapia (Oreochromis niloticus). Aquaculture 551, 01–09. doi: 10.1016/j.aquaculture.2022.737934
Anater A., Manyes L., Meca G., Ferrer E., Luciano F. B., Pimpão C. T., et al. (2016). Mycotoxins and their consequences in aquaculture: A review. Aquaculture 451, 1–10. doi: 10.1016/j.aquaculture.2015.08.022
Avantaggiato G., Solfrizzo M., Visconti A. (2005). Recent advances on the use of adsorbent materials for detoxification of fusarium mycotoxins. Food Addit. Contam 22, 379–388. doi: 10.1080/02652030500058312
Bashorun A., Hassan Z. U., Al-Yafei M. A., Jaoua S. (2023). Fungal contamination and mycotoxins in aquafeed and tissues of aquaculture fishes and their biological control. Aquaculture 576, 01–08. doi: 10.1016/j.aquaculture.2023.739892
Battilani P., Toscano P., van der Fels-Klerx H. J., Moretti A., Leggieri Camardo M., Brera C., et al. (2016). Aflatoxin B1 contamination in maize in Europe increases due to climate change. Sci. Rep. 6, 24328. doi: 10.1038/srep24328
Boudergue C., Burel C., Dragacci S., Favrot M.-C., Fremy J.-M., Massimi C., et al. (2009). Review of mycotoxin-detoxifying agents used as feed additives: mode of action, efficacy and fee/food safety Vol. 6 (European Union: EFSA Supporting Publication), 192. EN-22.
Burgos-Aceves M. A., Lionetti L., Faggio C. (2019). Multidisciplinary haematology as prognostic device in environmental and xenobiotic stress-induced response in fish. Sci. Total Environ. 670, 1170–1183. doi: 10.1016/j.scitotenv.2019.03.275
Chestnut A. B., Anderson P. D., Cochran M. A., Fribourg H. A., Gwinn K. D. (1992). Effects of hydrated sodium calcium aluminosilicate on fescue toxicosis and mineral absorption. J. Anim. Sci. 70, 2838–2846. doi: 10.2527/1992.7092838x
Di Gregorio M. C., de Neeff D. V., Jager A. V., Corassin C. H., de Pinho Carão A. C., de Albuquerque R., et al. (2014). Mineral adsorbents for prevention of mycotoxins in animal feeds. Toxin Rev. 33, 125–135. doi: 10.3109/15569543.2014.905604
Döll S., Baardsen G., Koppe W., Stubhaug I., Dänicke S. (2010). “Effects of increasing concentrations of the mycotoxins deoxynivalenol, zearalenone or ochratoxin A in diets for Atlantic salmon (Salmo salar) on growth performance and health,” in The 14th International Symposium on Fish Nutrition and Feeding, (Qingdao, China). 120.
Faggio C., Fedele G., Arfuso F., Panzera M., Fazio F. (2014). Haematological and biochemical response of Mugil cephalus after acclimation to captivity. Cah. Biol. Mar. 55, 31–36.
Fazio F., Marafioti S., Torre A., Sanfilippo M., Panzera M., Faggio C. (2013). Haematological and serum protein profiles of Mugil cephalus: effect of two different habitats. Ichthyol. Res. 60, 36–42. doi: 10.1007/s10228-012-0303-1
Ferguson H. W. (2006). “Liver,” in Systemic pathology of fish, 2nd edition. Ed. Ferguson H. W. (London: Scotian Press), 201–216.
Gonçalves R. A., Navarro-Guillén C., Gilannejad N., Dias J., Schatzmayr D., Bichl G., et al. (2018). Impact of deoxynivalenol on rainbow trout: growth performance, digestibility, key gene expression regulation and metabolism. Aquaculture 490, 362–372. doi: 10.1016/j.aquaculture.2018.03.001
Gonçalves R. A., Schatzmayr D., Albalat A., Mackenzie S. (2020). Mycotoxins in aquaculture: feed and food. Aquaculture 12, 145–175. doi: 10.1111/raq.12310
Greeff-Laubscher M. R., Beukes I., Marais G. J., Jacobs K. (2020). Aflatoxin detoxification using microorganisms and enzymes. Mycology. 11, 105–117. doi: 10.1080/21501203.2019.1604575
Guan Y., Chen J., Nepovimova E., Long M., Wu W., Kuca K. (2023). Mycotoxin production by three different toxigenic fungi genera on formulated abalone feed and the effect of an aquatic environment on fumonisins. Toxins 13 (46), 01–17. doi: 10.3390/toxins13010046
Halver J. E., Goldblatt L. A. (1969). “Aflatoxicosis and trout hepatoma,” in Aflatoxin: scientific background, control, and implications (New York, New York, USA: Academic Press), 265–306.
Holanda D. M., Kim S. W. (2021). Mycotoxin occurrence, toxicity, and detoxifying agents in pig production with emphasis on deoxynivalenol. Toxins 13, 171. doi: 10.3390/toxins13020171
Hooft J. M., Bureau D. P. (2017). Evaluation of the efficacy of a commercial feed additive against adverse effects of feed-borne deoxynivalenol (DON) on the performance of rainbow trout (Oncorhynchus mykiss). Aquaculture 473, 237–245. doi: 10.1016/j.aquaculture.2017.02.019
Hooft J. M., Elmor A. E. H. I., Encarnaçao P., Bureau D. P. (2011). Rainbow trout (Oncorhynchus mykiss) is extremely sensitive to the feed-borne Fusarium mycotoxin deoxynivalenol (DON). Aquaculture 311, 224–232. doi: 10.1016/j.aquaculture.2010.11.049
Hooft J. M., Wu P., Powell C. D., Lou Y., Squires E. J., Cant J. P., et al. (2019). A comparative investigation of the effects of feed-borne deoxynivalenol (DON) on growth performance, nutrient utilization and metabolism of detoxification in rainbow trout (Oncorhynchus mykiss) and Nile tilapia (Oreochromis niloticus) fed diets containing different levels of digestible carbohydrates. Aquaculture 505, 306–318. doi: 10.1016/j.aquaculture.2019.02.019
Hussain D., Mateen A., Gatlin D. M. (2017). Alleviation of aflatoxin B1 (AFB1) toxicity by calcium bentonite clay: Effects on growth performance, condition indices and bioaccumulation of AFB1 residues in Nile tilapia (Oreochromis niloticus). Aquaculture 475, 8–15. doi: 10.1016/j.aquaculture.2017.04.003
Huwig A., Freimund S., Käppeli O., Dutler H. (2001). Mycotoxin detoxication of animal feed by different adsorbents. Toxicol. Lett. 122, 179–188. doi: 10.1016/s0378-4274(01)00360-5
Jantrarotai W., Lovell R. T. (1990). Acute and subchronic toxicity of cyclopiazonic acid to channel catfish. J Aquat Anim Health 2, 255–260. doi: 10.1577/1548-8667(1990)002<0255:AASTOC>2.3.CO;2
Koletsi P., Graat E. A. M., Lyons P., Wiegertjes G. F. (2023). Are the effects of Deoxynivalenol (DON) on performance, liver and gastrointestinal tract health of rainbow trout (Oncorhynchus mykiss) influenced by dietary composition? Aquaculture. 32, 01–14. doi: 10.1016/j.aqrep.2023.101740
Kong C., Shin S. Y., Kim B. G. (2014). Evaluation of mycotoxin sequestering agents for aflatoxin and deoxynivalenol: an in vitro approach. SpringerPlus 3, 346. doi: 10.1186/2193-1801-3-346
Lumlertdacha S., Lovell R. T., Shelby R. A., Lenz S. D., Kemppainen B. W. (1994). Growth, hematology, and histopathology of channel catfish, Ictalurus punctatus, fed toxins from Fusarium moniliforme. Aquaculture 130, 201–218. doi: 10.1016/0044-8486(94)00219-E
Luna L. G. (1968). Manual of histologic staining methods of the armed forces institute of pathology, third ed (New York: McGraw–Hill).
Manning B. B., Ulloa R. B., Li M. H., Robinson E. H., Rottinghaus G. E. (2003). Ochratoxin A fed to channel catfish (Ictalurus punctatus) causes reduced growth and lesions of hepatopancreatic tissue. Aquaculture 219, 739–750. doi: 10.1016/S0044-8486(03)00033-4
Marroquín-Cardona A. G., Johnson N. M., Phillips T. D., Hayes A. W. (2014). Mycotoxins in a changing global environment: A review. Food Chem. Toxicol. 69, 220–230. doi: 10.1016/j.fct.2014.04.025
Mommsen T. P. (2001). Paradigms of growth in fish. Comp. Biochem. Physiol. 129, 207–219. doi: 10.1016/S1096-4959(01)00312-8
National Research Council (NRC) (2011). Nutrient requirements of fish and shrimp (Washington D.C., USA: National Academies Press).
Nogueira W. V., de Oliveira F. K., Marimón Sibaja K. V., de Oliveira Garcia S., Kupski L., de Souza M. M., et al. (2020). Occurrence and bioacessibility of mycotoxins in fish feed. Food Addit. Contam. Part B 13, 244–251. doi: 10.1080/19393210.2020.1766577
Oliveira M., Vasconcelos V. (2020). Occurrence of mycotoxins in fish feed and its effects: a review. Toxins 12, 160. doi: 10.3390/toxins12030160
Olokkaran R., Mathew S. (2020). In vitro detoxification of aflatoxin B1 by calcium bentonite clay supplementation in aflatoxigenic mould contaminated feeds for Nile tilapia, Oreochromis niloticus (Linnaeus 1758). Asian J. Fish. Aquat. Res. 10, 1–18. doi: 10.9734/ajfar/2020/v10i130169
Paterson R. R. M., Lima N. (2010). How will climate change affect mycotoxins in food? Food Res. Int. 43, 1902–1914. doi: 10.1016/j.foodres.2009.07.010
Patriarca A., Pinto V. F. (2017). Prevalence of mycotoxins in foods and decontamination. Curr. Opin. Food Sci. 14, 50–60. doi: 10.1016/j.cofs.2017.01.011
Pietsch C., Michel C., Kersten S., Valenta H., Dänicke S., Schulz C., et al. (2014). In vivo effects of deoxynivalenol (DON) on innate immune responses of carp (Cyprinus carpio L.). Food Chem. Toxicol. 68, 44–52. doi: 10.1016/j.fct.2014.03.012
Reitman S., Frankel S. (1957). In vitro determination of transaminase activity in serum. Am. J. Clin. Pathol. 28, 56–63. doi: 10.1093/ajcp/28.1.56
Sehonova P., Svobodova Z., Dolezelova P., Vosmerova P., Faggio C. (2018). Effects of water borne antidepressant son non-target animals living in the aquatic environment: a review. Sci. Total Environ. 631, 789–794. doi: 10.1016/j.scitotenv.2018.03.076
Selim K. M., El-hofy H., Khalil R. H. (2014). The efficacy of three mycotoxin adsorbents to alleviate aflatoxin B1 - induced toxicity in Oreochromis niloticus. Aquacult. Int. 22, 523–540. doi: 10.1007/s10499-013-9661-6
Smaoui S., D´Amore T., Agriopoulou S., Khaneghah A. M. (2023). Mycotoxins in seafood: occurrence, recent development of analytical techniques and future challenges. Separations 22, 523–540. doi: 10.3390/separations10030217
Solfrizzo M., Carratu M. R., Avantaggiato G., Galvano F., Pietri A., Visconti A. (2001). Ineffectiveness of activated carbon in reducing the alteration of sphingolipid metabolism in rats exposed to fumonisin contaminated diets. Food Chem. Toxicol. 10, 217. doi: 10.1016/s0278-6915(00)00160-5
Stoskopf M. K. (1993). “Clinical pathology,” in Fish medicine. Ed. Stoskopf M. K. (Philadelphia: W.B. Saunders Company), 113–131.
Taroncher M., Rodríguez-Carrasco Y., Aspevik T., Kousoulaki K., Barba F. J., Ruiz M. J. (2021). Cytoprotective effects of fish protein hydrolysates against H2O2-induced oxidative stress and mycotoxins in caco-2/TC7 cells. Fish Physiol. Biochem. 47, 515–532. doi: 10.1007/s10695-021-00929-6
Tola S., Bureau D. P., Hooft J. M., Beamish F. W. H., Sulyok M., Krska R., et al. (2015). Effects of wheat naturally contaminated with Fusarium mycotoxins on growth performance and selected health in dices of red tilapia (Oreochromis niloticus × O. mossambicus). Toxins 7, 1929–1944. doi: 10.3390/toxins7061929
Tuan N. A., Grizzle J. M., Lovell R. T., Manning B. B., Rottinghaus G. E. (2002). Growth and hepatic lesions of Nile tilapia (Oreochromis niloticus) fed diets containing aflatoxin B1. Aquaculture 212, 311–319. doi: 10.1016/S0044-8486(02)00021-2
Tuan N. A., Manning B. B., Lovell R. T., Rottinghaus G. E. (2003). Responses of Nile tilapia (Oreochromis niloticus) fed diets containing different concentrations of moniliformin or fumonisin B1. Aquaculture 217, 515–528. doi: 10.1016/S0044-8486(02)00268-5
Vila-Donat P., Marín S., Sanchis V., Ramos A. J. (2018). A review of the mycotoxin adsorbing agents, with an emphasis on their multi-binding capacity, for animal feed decontamination. Food Chem. Toxicol. 114, 246–259. doi: 10.1016/j.fct.2018.02.044
Wang E., Norred W. P., Bacon C. W., Riley R. T., Merril A. H. Jr. (1991). Inhibition of sphingolipid biosynthesis by fumonisins; Implications for diseases associated with Fusarium moniliforme. J. Biol. Chem. 226, 1486–1490. doi: 10.1016/S0021-9258(18)98712-0
Zahran E., Risha E., Hamed M., Ibrahim T., Palić D. (2020). Dietary mycotoxicosis prevention with modified zeolite (Clinoptilolite) feed additive in Nile tilapia (Oreochromis niloticus). Aquaculture 515, 734562. doi: 10.1016/j.aquaculture.2019.734562
Zhu Y., Hassan Y. I., Watts C., Zhou T. (2016). Innovative technologies for the mitigation of mycotoxins in animals feed and ingredients – A review of recent patents. Anim. Feed Sci. Technol. 2016, 19–29. doi: 10.1016/j.anifeedsci.2016.03.030
Keywords: fumonisins, deoxynivalenol, zearalenone, adsorbents, productive performance
Citation: Fornari DC, Peixoto S, Ksepka SP, Bullard SA, Rossi W, Nuzback DE and Davis DA (2023) Effects of dietary mycotoxins and mycotoxin adsorbent additives on production performance, hematological parameters, and liver histology in juvenile Nile tilapia (Oreochromis niloticus). Front. Anim. Sci. 4:1281722. doi: 10.3389/fanim.2023.1281722
Received: 04 September 2023; Accepted: 26 October 2023;
Published: 13 November 2023.
Edited by:
Samad Rahimnejad, University of Murcia, SpainReviewed by:
Heba Mahboub, Zagazig University, EgyptMansour Torfi Mozanzadeh, South Iran Aquaculture Research Center, Iran
Copyright © 2023 Fornari, Peixoto, Ksepka, Bullard, Rossi, Nuzback and Davis. This is an open-access article distributed under the terms of the Creative Commons Attribution License (CC BY). The use, distribution or reproduction in other forums is permitted, provided the original author(s) and the copyright owner(s) are credited and that the original publication in this journal is cited, in accordance with accepted academic practice. No use, distribution or reproduction is permitted which does not comply with these terms.
*Correspondence: Darci Carlos Fornari, ZGFyY2kucGVpeGVnZW5AZ21haWwuY29t; D. Allen Davis, ZGF2aXNkYUBhdWJ1cm4uZWR1