- 1College of Veterinary Medicine, University of Missouri, Columbia, MO, United States
- 2BSM Research, BSM Partners LLC, Rogers, AR, United States
- 3College of Veterinary Medicine, Washington State University, Pullam, WA, United States
- 4Department of Cardiology, BluePearl Pet Hospital, Overland Park, KS, United States
- 5Lamb Consulting LLC, West St. Paul, MN, United States
Introduction: Diet-associated dilated cardiomyopathy (DCM) has been suspected in breeds that have not been previously noted to have a predisposition to the DCM phenotype. This study hypothesized that over 210 days, dogs fed diets with varying amounts of animal-sourced protein and carbohydrate sources would not be negatively impacted in terms of their cardiac parameters and function.
Methods: Thirty-two purebred beagles and 33 mixed-breed hounds were randomized into four diet groups and studied for 210 days. The diet groups were as follows: the high-animal-protein grain-free (HAGF) group, the low-animal-protein grain-free (LAGF) group, the high-animal-protein grain-inclusive (HAGI), and the low-animal-protein grain-inclusive (LAGI) group. Cardiac-specific biomarkers, endomyocardial biopsies, and linear and volumetric echocardiographic parameters were evaluated.
Results: There was a treatment-by-day-by-breed effect observed for the normalized left ventricular internal diameter at end-diastole (p = 0.0387) and for the normalized left ventricular internal diameter at end-systole (p = 0.0178). On day 210, mixed-breed hounds fed the LAGI diet had a smaller normalized left ventricular internal diameter at end-diastole than on day 90. On day 210, beagles fed the LAGF diet had a larger normalized left ventricular internal diameter at end-systole than those fed the LAGI diet. Fractional shortening for beagles in the LAGF group was significantly lower (p = 0.007) than for those in the HAGI and LAGI groups. Cardiac-specific biomarkers and endomyocardial biopsies were not significantly different between breeds, diets, and various time points.
Discussion: This study did not detect the development of cardiac dysfunction throughout the study period through the echocardiographic parameters measured, select cardiac biomarkers, or endomyocardial biopsies. There were noted interactions of treatment, breed, and time; therefore, isolating a diet association was not possible. Future research should further investigate the other factors that may help to identify the variable(s) and possible mechanisms underlying suspected diet-associated DCM in dogs.
1 Introduction
Dilated cardiomyopathy (DCM) phenotype has been traditionally considered a disease of genetic origin in dogs (primary DCM). The historical prevalence of primary DCM in specific breeds is well recognized. Breed-based predisposition to primary DCM (Dukes-McEwan et al., 2003; Meurs, 2003; Leach et al., 2022) has been reported for Doberman Pinschers (Wess et al., 2010; Beier et al., 2015), Irish Wolfhounds (Vollmar et al., 2013; Vollmar et al., 2019), Great Danes, American Cocker Spaniels, English Bulldogs, Golden Retrievers (Belanger et al., 2005), Newfoundlands (Backus et al., 2003; Fascetti et al., 2003; Backus et al., 2006), Saint Bernards, Portuguese Water Dogs (Sleeper et al., 2002), and Standard and Giant Schnauzers (Harmon et al., 2017). Other documented causes of a DCM phenotype include chronic tachycardia (O’Brien et al., 1990), myocarditis (Santilli et al., 2017), hypothyroidism (Panciera, 1994; Panciera, 2001), hypoadrenocorticism (Phillips and Harkin, 2003; Scott-Moncrieff, 2012), dysautonomia, and known nutritional deficiencies (Fascetti et al., 2003).
Recently, published studies have investigated a potential association between diets rich in pulses, potatoes, and/or sweet potatoes, which have also been referred to as non-traditional diets (Kaplan et al., 2018; Ontiveros et al., 2019; Freid et al., 2021; Walker et al., 2021; Freeman et al., 2022; Owens et al., 2023; Quilliam et al., 2023), and diet-associated DCM. Conversely, there have been studies published that observed no negative impact on cardiac function and parameters associated with such diets (Donadelli et al., 2020; Pezzali et al., 2020; Cavanaugh et al., 2021; Reilly et al., 2021; Singh et al., 2023). Although the body of knowledge continues to grow, there are limitations in the available literature that need to be investigated further (McCauley et al., 2020), which include not controlling for diet parameters, such as the inclusion rate of pulses and/or potatoes in the diets fed to the dogs (Kaplan et al., 2018; Ontiveros et al., 2019; Walker et al., 2021; Freeman et al., 2022; Owens et al., 2023). In addition, although retrospective case series and prospective observational studies have been conducted (Freid et al., 2021), they have uncontrolled variables that could impact their findings, such as not controlling for the diet, environment, and concurrent diseases; varying pharmacotherapy; varying diet changes; the inclusion of breeds known to be predisposed to primary DCM; inconsistent supplementation of taurine and carnitine; and/or small sample sizes (McCauley et al., 2020; Freid et al., 2021; Quilliam et al., 2023).
In response to some of these published studies and other presented information, the United States Food and Drug Administration has investigated a potential link between specific categories of diets and the DCM phenotype (FDA, 2018; FDA, 2019a; FDA, 2019b). Despite reports of an increase in the number of cases of suspected diet-associated DCM, the overall reported incidence rate of the DCM phenotype in dogs diagnosed at the cardiology services of referral veterinary hospitals in the United States has remained stable at 2.53% to 5.65% nationally over the past two decades (Quest et al., 2022). In contrast, a 500% increase in grain-free pet food sales was reported from 2011 to 2019 (Quest et al., 2022). Although there has been increasing research into potential dietary causes for the DCM phenotype in dogs, no clear causation has been established. The objectives of this prospective study were to evaluate any changes in echocardiographic parameters and select cardiac biomarkers, including N-terminal pro-B-type natriuretic peptide (NTproBNP), cardiac troponin-I (cTnI), and endomyocardial biopsies, in dogs eating different custom diets that were formulated to be representative of various grain-free and grain-inclusive dry dog foods currently on the market. It was hypothesized that over a 7-month period, a cohort of purebred and mixed-breed dogs fed diets with varying amounts of animal source protein and carbohydrate sources would not be negatively impacted in terms of their histology and cardiac parameters and biomarkers. Although endomyocardial biopsies are a more invasive diagnostic procedure, early histopathologic changes in cardiac tissue may occur well before echocardiographic changes are noted (Tidholm and Jonsson, 2005).
2 Materials and methods
2.1 Study design
This was a blinded, prospective, longitudinal randomized experimental study. This study was part of a larger study, which also examined other parameters including taurine and carnitine concentrations in the blood, plasma, and skeletal and cardiac muscle tissue (Streeter et al., 2022); the fecal microbiome; digestibility; bile acid metabolites (Clark et al., 2023); plasma amino acid concentrations; foodomics; and metabolomics. The study protocol was approved by the Institutional Animal Care and Use Committee at Pet Food Solutions, Inc (Auxvasse, MO, USA) (020098.01).
2.1.1 Animals and diets
Healthy adult purpose-bred beagles and mixed-breed hounds (n = 65) were evaluated in this study. The dogs were considered healthy based on a complete physical examination and baseline laboratory analysis, including a complete blood count, serum chemistry analysis, and testing for the presence of heartworm, Ehrlichia, Anaplasma, and Lyme disease (SNAP 4DX, IDEXX Laboratories, Westbrook, MN, USA). Sixty-five intact adult dogs were included in the study: 32 beagles [16 males and 16 females, mean (± standard deviation) age 24.2 (± 7.1) months], and 33 mixed-breed hounds [(16 males and 17 females, mean (± standard deviation) age 13.3 (± 0.8) months]. Before the start of the study, each dog was weighed and assigned a body condition score (BCS) on a scale ranging from 1 to 9 (Laflamme, 1997). To ensure that all the dogs were offered enough food to maintain their ideal body weight (BW), the desired kcal/day for each dog was calculated using the maintenance energy requirement for laboratory dogs according to the National Research Council, [kcal/day = 130 × (BW in kg)0.75] (NRC, 2006). The dogs were provided with food once daily at 08:00 and, for those dogs that had not finished their food within 4 hours, the remaining food was weighed and recorded. Then, water was added to the remaining food to entice them to eat. Fresh, clean water was always available. The dogs’ body weight and BCS were recorded weekly, and each dog’s diet was adjusted to maintain their ideal BW and BCS. The ideal BW was calculated for all the dogs based on the American Animal Hospital Association guidelines (Brooks et al., 2014). All the dogs were fed the same baseline diet (Purina Dog Chow®; Nestle-Purina, St. Louis, MO, USA) for at least 3 months before the start of the study.
At the start of the study, the dogs were stratified based on breed, sex, and body condition score and randomly assigned into one of four dietary treatment groups. All the dogs were housed individually but randomly assigned to one of four housing rooms. The cages were stainless steel with self-spanned polyvinyl chloride-coated expanded metal flooring. The dogs in the study were allowed to exercise for no more than 1 h per day. All the dogs were subjected to 12 hours of light and 12 hours of darkness. The diet food portions were weighed and recorded for each feeding. To control for inclusion rates in the diets fed, the researchers (RMS and SDC) formulated the diets used in the study. The diets were divided into two animal protein groups: high animal protein content (70% of the total protein derived from animal sources) or low animal protein content (45% of the total protein derived from animal sources). Based on previously published data (Kaplan et al., 2018; Ontiveros et al., 2019; Freeman et al., 2022), each animal protein diet was also formulated to be either grain free, that is, containing high-pulse ingredients and potatoes, or grain inclusive, that is, containing no pulses or potatoes. We created four diets using these combinations: a high-animal-protein grain-free diet (HAGF), a high-animal-protein grain-inclusive diet (HAGI), a low-animal-protein grain-free diet (LAGF), and a low-animal-protein grain-inclusive diet (LAGI) (Table 1). The HAGF diet contained 34.5% pulses and 26.8% potatoes, and the LAGF diet contained 66.9% pulses and 1.5% potatoes. Protein, fat, fiber, and ash concentrations were formulated to be the same among all diets and each diet met the American Association of Feed Control Officials (AAFCO) requirements for canine adult maintenance (FDA, 2018). The tested nutrient profile for each raw ingredient was obtained and third-party laboratory testing (Midwest Laboratories, Omaha, NE, USA) was conducted on the finished food prior to the start of the study (Supplementary Table 1). The four test diets were formulated to be as representative as possible of various diets currently on the market. Each of the dogs was fed their respective test diet for 210 days.
2.2 Echocardiography
Complete transthoracic echocardiograms were conducted on all the dogs without sedation at baseline and again on days 81–87, 165–171, and 200–205 of the study. The echocardiographic examinations were conducted using an ultrasound unit (Mindray M7 and M8 Elite, Shenzhen Mindray Bio-Medical Electronics Co. LTD, North Charleston, SC, USA) equipped with several phased-array transducers (1.1 MHz to 7 MHz) matched to the size of the dogs. The dogs were restrained in lateral recumbency for all echocardiograms. All the echocardiograms were conducted by the same registered diagnostic cardiac sonographer. Three board-certified veterinary cardiologists independently reviewed the data from all times points, in addition to a subjective of the echocardiographic images for quality and accuracy. All the echocardiographic studies were considered by the cardiologists to have been of diagnostic quality. Any dog with pre-existing congenital or acquired heart disease identified in the baseline echocardiographic examination was excluded from the study. The dogs with valvular regurgitation that was considered more than mild were excluded from the study (Supplementary Figure 1). Using a previously validated and utilized technique (Muzzi et al., 2003; Chetboul & Tissier, 2012; Li et al., 2019; Liu et al., 2020), mitral regurgitation severity was assessed semiquantitatively using the ratio of the mitral regurgitant maximal jet area to the left atrial area by way of color Doppler, with a result of < 5% considered trace, between 5% and 20% considered mild, between 20% and 50% considered moderate, and of > 50% considered severe. The sonographer and cardiologists were all blinded to the treatment. The cardiologists were also blinded to the time points. All the echocardiographic examinations consisted of two-dimensional, M-mode, color Doppler, and spectral Doppler imaging using standard right parasternal and left apical imaging planes with the dogs placed in right and left lateral recumbency, respectively (Thomas et al., 1993; Isayama et al., 2022). The standard right parasternal views included the long-axis four-chamber view, long-axis five-chamber view, and also the short-axis views at the level of the left ventricular papillary muscles, mitral valve, and aortic valve. The standard left apical views included the four-chamber and five-chamber views. In each view, the visible valves were evaluated using two-dimensional and color Doppler echocardiography to identify any abnormal valve thickening, motion, and regurgitation. Using both pulse-wave and continuous-wave Doppler, the peak transpulmonic flow velocities and peak transaortic flow velocities were interrogated using the right parasternal short-axis view at the level of the aortic root and the left apical five-chamber view, respectively. The echocardiographic measurements were recorded at each time point for the comparison of cardiac size and function. The average from the three cardiac cycles was reported for all measurements. These included M-mode measurements and calculations of the left ventricular internal diameter at end-diastole (LVIDd) and at end-systole (LVIDs), normalized LVIDd and LVIDs (Wess et al., 2021), and left ventricular fractional shortening (FS). Left ventricular end-diastolic volume (EDV), end-systolic volume (ESV), and left ventricular ejection fraction (EF) were calculated using the monoplane Simpson’s method of discs (Wess et al., 2021), and the left atrial-to-aortic root ratio (LA : Ao) was calculated using the Rishniw method (Rishniw and Erb, 2000).
To compare changes in the left ventricular chamber diameters and volume measurements among dogs of various body sizes and conformations, the left ventricular chamber diameters and volume measurements were normalized to each dog’s BW (in kg), and volume measurements were also indexed to each dog’s body surface area (BSA) in meters squared (Visser et al., 2019; British Small Animal Veterinary Association, 2023). To account for the changes in BW and BCS that might occur over the course of the study and to allow for serial comparisons, the left ventricular chamber diameters were also normalized to each dog’s ideal BW and BSA. The BSA was calculated as 0.101 × BW (kg)2/3 using each dog’s BW (actual and ideal) (Laflamme, 1997). Specifically, the LVIDd (cm/kg^0.299) measurements were normalized (LVIDdN) to each dog’s BW (both ideal and actual) using LVIDd/BW^0.299, and the LVIDs (cm/kg^0.387) measurements were normalized (LVIDsN) using LVIDs/BW^0.387. The EDV (mL/m2) and ESV (mL/m2) measurements were normalized (EDVN and ESVN, respectively) to BW using EDV/BW and ESV/BW, respectively. The EDV and ESV were also indexed (EDVI and ESVI, respectively) to BSA using EDV/BSA and ESV/BSA, respectively. In addition, EDV and ESVI were also indexed to BW (actual and ideal). The EDVI (mL/kg) measurements were indexed (EDVI) to BW (kg) using EDV/BW and the ESV (mL/kg) measurements were indexed (ESVI) to BSA using ESV/BW.
2.3 Cardiac biomarkers
The fasted venous blood samples were collected from cephalic or saphenous veins at baseline and at days 81–87 and 165–171. The serum and ethylenediaminetetraacetic acid plasma samples were separated, aliquoted, and stored at −70°C for overnight shipping to an external laboratory (IDEXX Laboratories, Westbrook, MN, USA) for batch analysis of cTnI (Advia Ultra Assay, Siemens Healthcare Diagnostics, Eschborn, Germany) and NTproBNP, respectively. The NTproBNP assay utilized was a commercially available and previously validated second-generation canine-specific sandwich enzyme-linked immunosorbent assay with an upper reported limit of 10,000 pmol/L (Cahill et al., 2015). The cTnI assay is a high-sensitivity assay with a lower limit of detection of 0.006 ng/mL and has previously been validated for use in dogs (Winter et al., 2014).
2.4 Endomyocardial biopsies and light microscopy
Endomyocardial biopsies were obtained from the mixed-breed hounds at baseline and on days 175–184 by a board-certified cardiologist who was blinded to the treatment. In brief, the dogs were anesthetized and endomyocardial biopsy samples were obtained from their right ventricles using a disposable five-French 1.5-mm biopsy forceps through a six-French introducer, placed either percutaneously or via a jugular venous cutdown using a Seldinger technique and with fluoroscopic guidance. The bioptome was directed toward the interventricular septal wall as previously described (Keene et al., 1990). The biopsy samples were visually inspected and weighed. The heaviest biopsy specimen that appeared largely pink and was consistent with the myocardium for each dog was immediately placed and fixed in 10% neutral-buffered formalin and subsequently embedded in paraffin and sectioned. The sections were stained with hematoxylin and eosin and examined under light microscopy by a board-certified veterinary pathologist. The remaining biopsy specimens that were collected from each dog were flash-frozen for a separate research project.
2.5 Statistical analysis
All data were analyzed with commercial software (SAS Institute, Inc., version 9.4, Cary, NC, USA) using repeated measures in the mixed model procedure. The model was run with a fixed effect of diet and breed and a random effect of sex. The day was used as the repeated variable. The interaction of treatment, breed, and day and their main effects were reported using a Fisher-protected least significant difference test with a Tukey adjustment to control for type 1 experiment-wise error. The means were statistically significant at a probability of p < 0.05. The reported standard errors of the means were determined from the MIXED model procedure. The descriptive variables including weight, BCS, and age were reported as the mean [± standard deviation (SD)]. Normality, as evaluated via the Shapiro–Wilk test, demonstrated that all parameters were normally distributed. A power analysis was conducted on ejection fraction to determine the minimum sample size required to test the study’s hypotheses. The results are based on a sample size of 64 to achieve an 83.6% power for detecting a 7.5% effect, at a significance criterion of alpha = 0.05.
3 Results
Seventy-two dogs were initially evaluated, but seven dogs were excluded from the study (Supplementary Figure 1). The dogs were excluded based on the presence of left ventricle dilation [LVIDdN > 1.63 (Visser et al., 2019)] (n = 2) and posterior mitral leaflet tethering (n = 1), as identified in the initial echocardiographic study. In addition, one dog was excluded for having anaplasmosis, two dogs were excluded for having giardia, and one dog was excluded because it had an abscess. A total of 65 intact, adult dogs were enrolled in this study: 32 were purebred beagles (16 males and 16 females) and 33 were mixed-breed hounds (16 males and 17 females). Each diet treatment group contained eight beagles and eight mixed-breed hounds. The distribution of sexes in the groups was even, except for in the HAGI group, which had an extra female mixed-breed hound. At the beginning of the study, the beagles weighed 9.8 kg ± 1.5 kg and had a BCS of 5.3 ± 1.4, and the mixed-breed hounds weighed 27.6 kg ± 3.3 kg and had a BCS of 5.3 ± 1.4. On day 0, the mixed-breed hounds were 13.3 months ± 0.8 months old, and the beagles were 24.2 months ± 7.1 months old. Forty-one out of the 65 dogs (63%) ate 100% of their daily MER throughout the study, with an equal proportion of dogs from each diet treatment group and breed. Seven out of 65 dogs (11%) ate less than 100% of their daily MER for no more than 5 days. Six out of the 65 dogs (9%) ate less than 100% of their daily MER for no more than 10 days throughout the study. Four out of the 65 dogs (6%) ate less than 100% of their daily MER for no more than 20 days throughout the study. Six out of the 65 dogs (9%) ate less than 100% of their daily MER for more than 25 days throughout the study. However, the MER for each dog was adjusted weekly based on their body weight and BCS to ensure that they were not being overfed or underfed. There was a breed-by-day interaction, with the food intake of mixed-breed hounds gradually increasing over time; for beagles, although the weight of food consumed increased, this increase was not significant (Table 2). In addition, this study was part of a larger study, in which all diets were observed to be highly digestible (Clark et al., 2023).
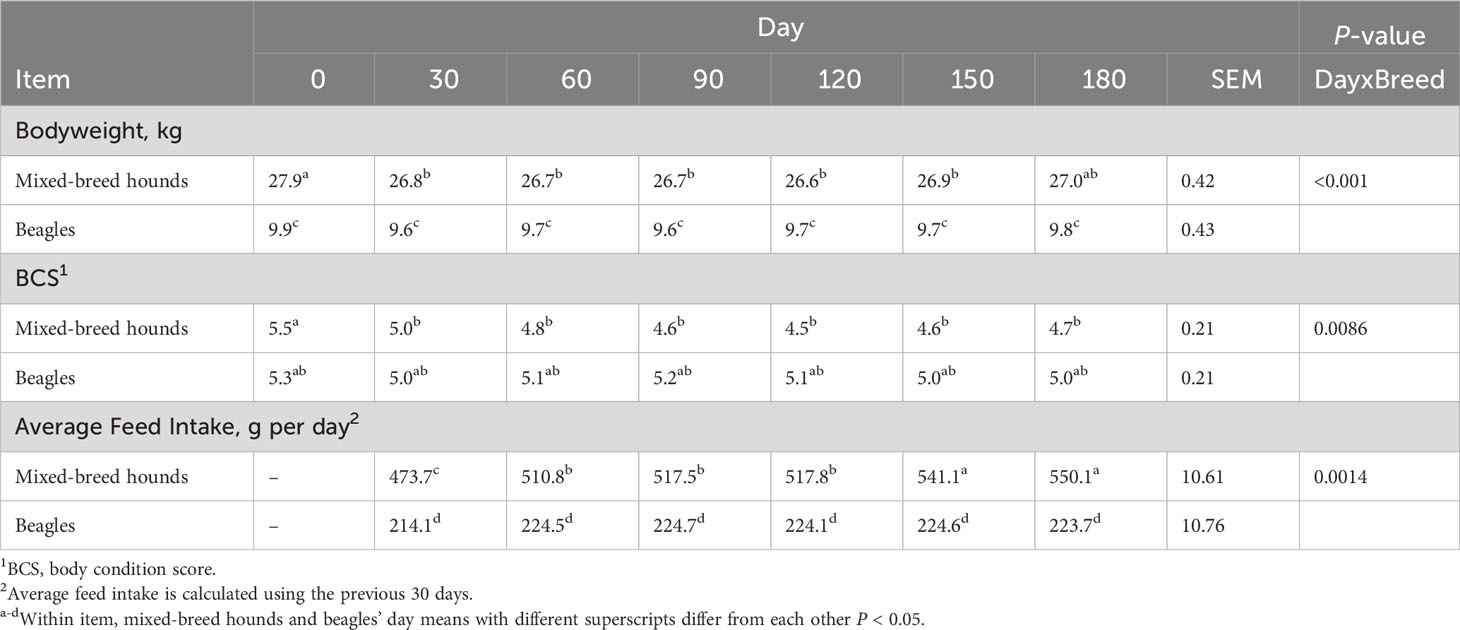
Table 2 Body condition score, body weights, and average feed intake in beagles and mixed-breed hounds.
3.1 Body weight and body condition scores
Over the course of the study, 18 out of 32 beagles lost weight, with 2 kg recorded as the maximum amount of weight lost and with eight dogs (two in each diet group) losing at least 10% of their day 0 body weight. Of the beagles, 28 out of 32 were considered overweight (i.e., they had a BCS > 5) at the beginning of the study. At the end of the study, 11 out of 32 dogs were considered overweight. Despite these results, none of the dogs were considered to be underweight according to their BCS (all dogs had a BCS > 3).
For the mixed-breed dogs, 24 out of 33 mixed-breed dogs lost weight, with 6.7 kg recorded as the maximum amount of weight lost and 18 dogs losing at least 10% of their day 0 body weight. Most of these dogs were in the grain-inclusive diet groups (n = 11). Of the mixed-breed hounds, 23 out of 33 were considered overweight (BCS > 5) at the beginning of the study and 8 out of 33 were considered overweight (BCS > 5) at the end of the study. Three out of 33 had a BCS of 3 at the end of the study. These dogs underwent physical examinations to so that their health could be checked and all were approved by a veterinarian to continue the study.
3.2 Echocardiography
For all cardiac measurements, actual and ideal BW is reported in Supplementary Table 2. The statistical outcomes were compared for actual BW and ideal BW for normalizing measurements and also for normalizing to BSA and volume. The cardiac parameters (LVIDdN, LVIDsN, FS, EF, LA : Ao, EDVI, and ESVI) are reported in Table 3 and are compared to the four test diets across all time points in Figures 1A–D, 2A–D.
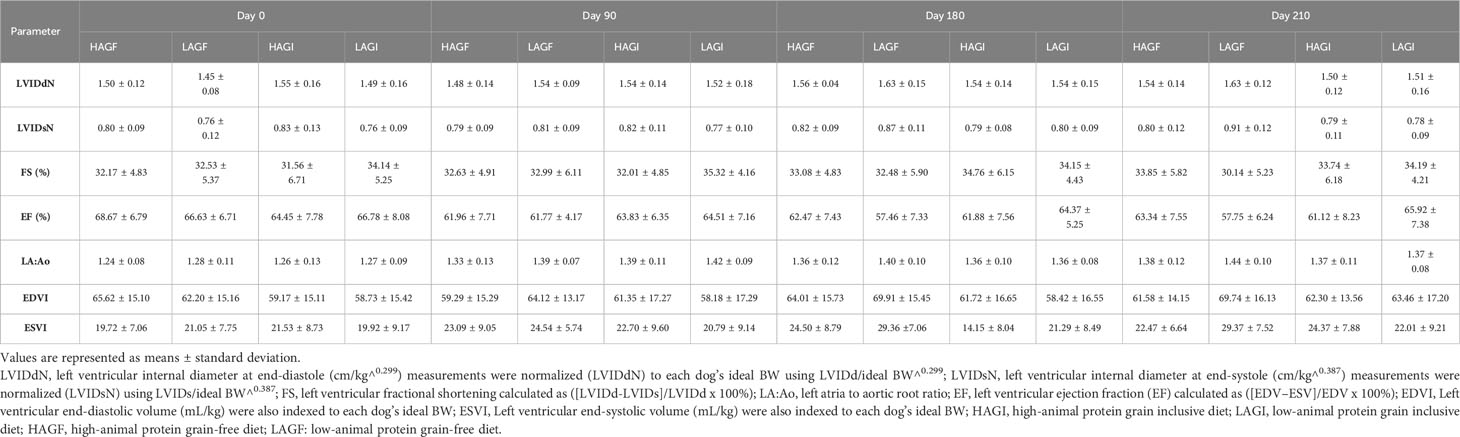
Table 3 Echocardiographic measurements in mixed-breed hounds and beagles fed different dietary treatments.
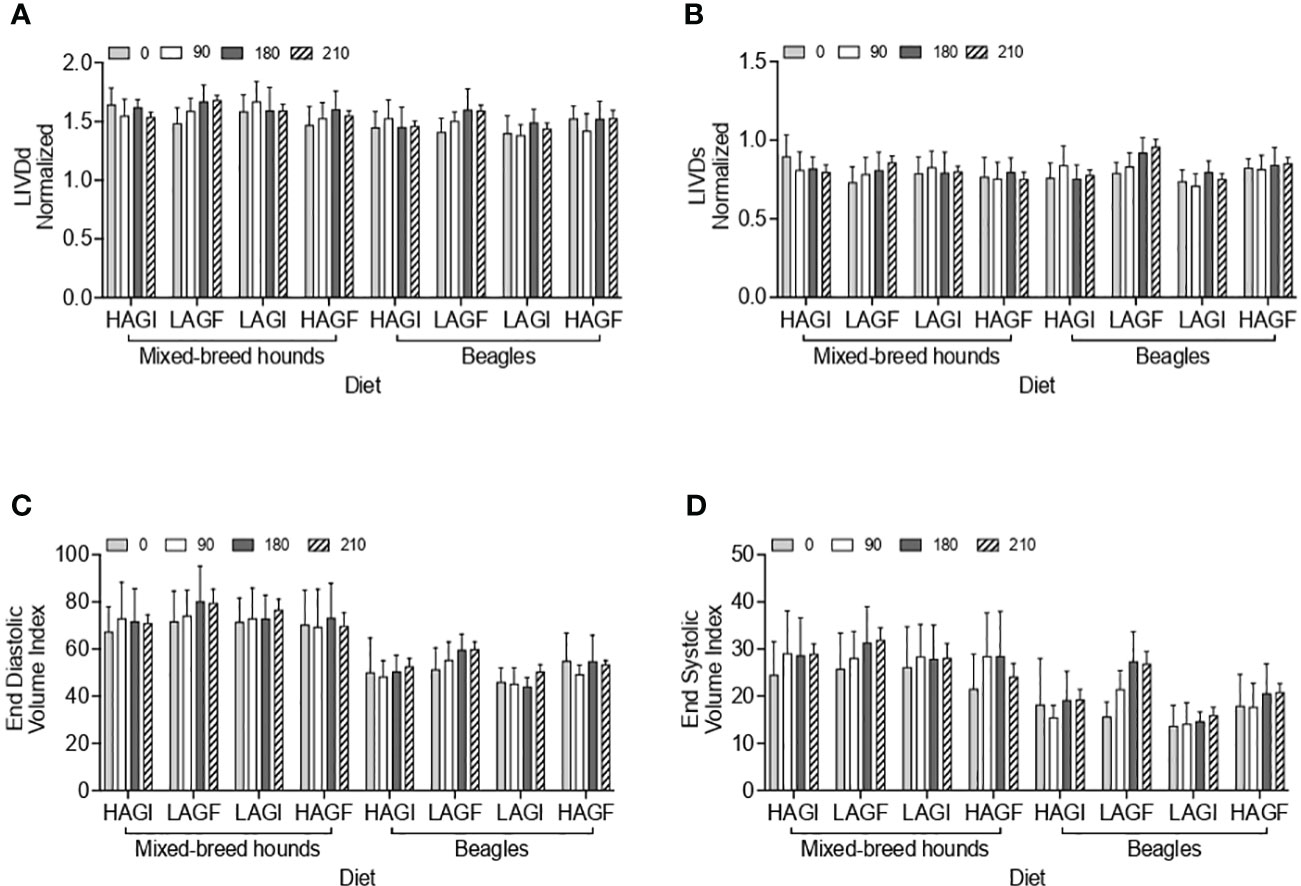
Figure 1 Echocardiographic variables including LVIDdN (A), LVIDsN (B), EDVI (C), and ESVI (D). LVIDAN, left ventricular internal diameter in diastole normalized to ideal body weight; LVIDsN, left ventricular internal diameter in systole normalized to ideal body weight; EDVI, end-diastolic volume index; ESVI, end-diastolic volume index; HAGI, high animal protein grain-inclusive diet; LAGF, low animal protein grain-free diet; LAGI, low animal protein grain-inclusive diet; HAGF, high animal protein grain-free diet. A treatment-by-day-by-breed was observed for LVIDdN and LVIDsN (P = 0.0387, P = 0.0178, respectively); A breed effect was observed for EDVI (P < 0.0001); A breed and a day effect was observed for ESVI (P < 0.0001; P = 0.0003, respectively).
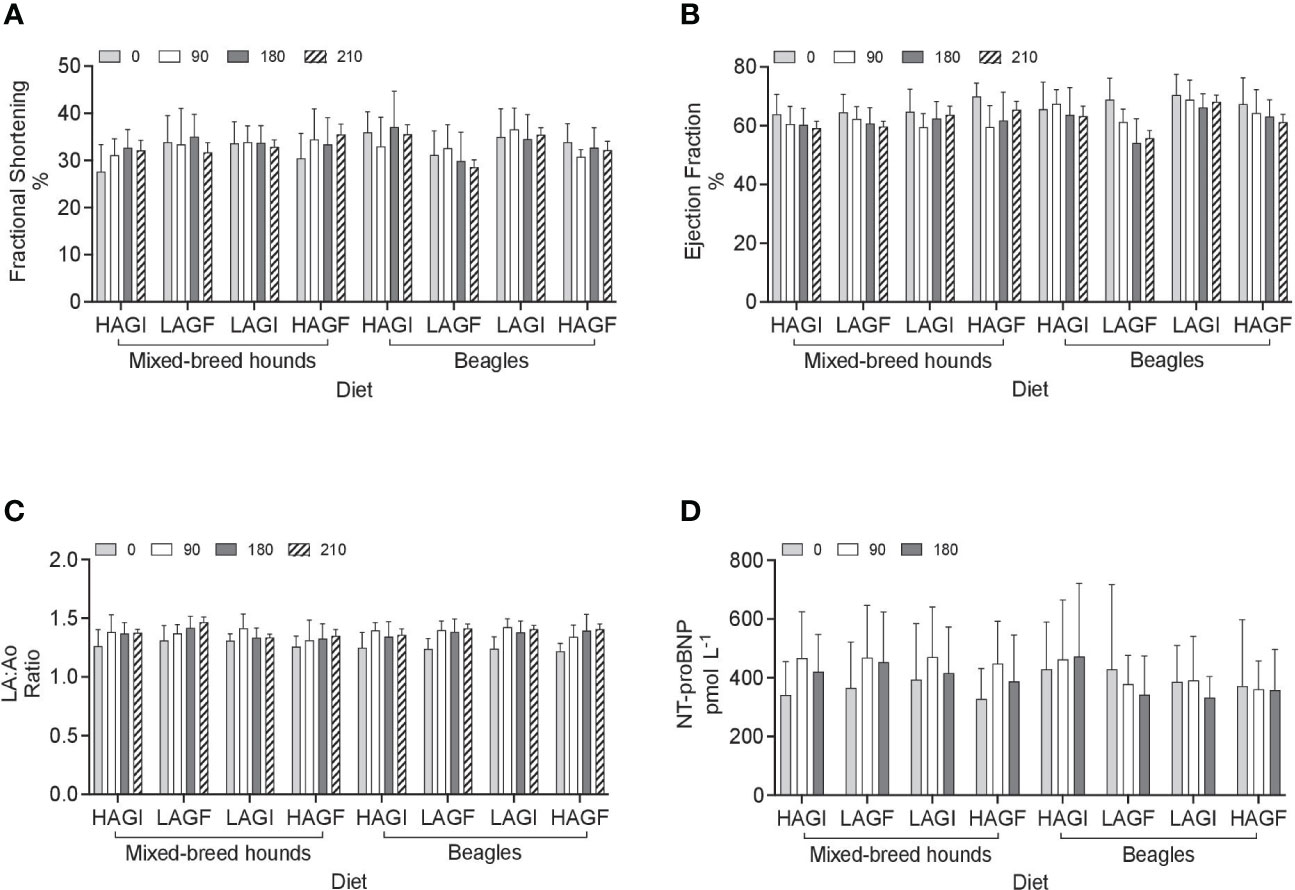
Figure 2 Echocardiographic variables including fractional shortening (A), ejection fraction (B), LA:Ao ratio (C), and NTproBNP (D). LA:Ao, left atrial to aortic root ratio; NT-proBNP, N-terminal pro-B-type natriuretic peptide; Fractional shortening had a treatment-by-breed effect (P = 0.007). Ejection fraction had a day effect and treatment effect (P < 0.0001, P = 0.02, respectively). LA:Ao ratio had a day effect (P < 0.0001). No significance was observed for NT-proBNP.
A breed effect was observed for ESVI, in that it was significantly higher (p < 0.0001) in mixed-breed hounds (27.5 mL/m2) than in beagles (18.5 mL/m2). In addition, there was a day effect observed for ESVI, which was significantly lower for all dogs at baseline (20.1 mL/m2) (p = 0.0003) than at the other three time points: day 90 (22.7 mL/m2), day 180 (24.7 mL/m2), and day 210 (24.4 mL/m2). Similarly, EDVI had a breed effect (p < 0.0001), and was significantly higher in mixed-breed hounds (72.7 mL/m2) than in beagles (51.1 mL/m2). For EDVI and ESVI, no treatment effect was observed (p > 0.05).
The mean LVIDdN was <1.53 cm/m2 for each diet group, which is below the cited value (≤ 1.63 cm/m2) (Visser et al., 2019). There was a significant treatment-by-day-by-breed effect (p = 0.0387) for LVIDdN. The mixed-breed hounds fed the LAGI diet had a significantly lower LVIDdN on day 0 (0.789 cm/m2) than the mixed-breed hounds at day 90 (0.828 cm/m2). For LVIDsN, the average for each treatment group was < 0.83 cm/m2, which is below the cited value (≤ 0.92 cm/m2) (Visser et al., 2019). There was a significant treatment-by-day-by-breed effect (p = 0.0178) for LVIDsN. At day 210, beagles in the LAGF group (0.958 cm/m2) had a significantly higher LVIDsN than beagles fed the LAGI diet on day 210 (0.75 cm/m2).
Fractional shortening had a treatment-by-breed effect (p = 0.007): fractional shortening in beagles in the LAGF (29.7%) group was significantly lower than that in beagles in the HAGI (35.4%) group and in beagles in the LAGI (35.4%) group. However, the same was not observed in mixed-breed hounds. The average FS for all diet treatment groups in all the dogs at all time points was > 25%, which was considered normal (Visser et al., 2019). The ejection fraction had a significant (p < 0.0001) day effect, with that at day 0 (66.93%), being significantly higher (p < 0.001) than those at days 90, 180, and 210 (63.1%, 61.5%, and 62.0%, respectively) in all the dogs regardless of dietary treatment groups. There was also a significant treatment effect (p = 0.03), with the EF in the LAGF (60.96%) group being significantly lower (p = 0.02) than that in the LAGI (65.60%) group for all the dogs. There was also a day effect for LA : Ao ratio. On day 0, the LA : Ao ratio was significantly lower (p < 0.0001) than at all other time points across all dogs and all diet groups.
3.3 Cardiac biomarkers
No significant differences (p > 0.05) were observed in the cTnI and NTproBNP concentrations among treatments, breeds, and time points. All samples collected had a cTnI concentration of < 0.2 ng/mL for all the dogs at all the time points and in all the treatment groups. The NTproBNP concentration for all the dogs in all diet treatment groups for all time points was normal at < 900 pmol/L, except for three mixed-breed dogs at various time points. Two dogs had NTproBNP values of 1,087 pmol/L and 1,593 pmol/L at day 0, but were within the normal range on days 90 and 180. One dog in the LAGI group had a NTproBNP value of 1,422 pmol/L on day 180; however, when echocardiographic measurements were reviewed for both left ventricle dimensions and systolic function, they were within the cited reference intervals.
3.4 Endomyocardial biopsies and light microscopy
The mean number of endomyocardial biopsy samples obtained from the mixed-breed hounds during acclimation was six (range 4–12 samples, SD ± 1.72 samples). The mean weight of the endomyocardial biopsy samples used for histopathology at day 0 was 1.18 μg (range 0.26–2.92 μg, SD ± 0.60 μg). The mean number of endomyocardial biopsy samples obtained per dog at days 175–184 was five (range 3–8 samples, SD ± 1.12 samples). The mean weight of the endomyocardial biopsy samples used for histopathology at day 180 was 0.74 μg (range 0.22–1.97 μg, SD ± 0.42 μg).
Histopathologic evaluation of the right ventricular endomyocardial biopsies revealed normal tissue architecture in all samples from all dogs at both time points (Supplementary Figure 2). No inflammatory or fatty infiltrates, no interstitial fibrosis, and no myocyte attenuation were identified in any of the samples. There were minimal crush artifacts, which likely came from the sampling technique and sectioning. This is consistent with prior reports of high diagnostic yields of 92% to 99% and adequacy for pathologic interpretation in people (Braunlin et al., 1998; Daly et al., 2012). Direct visualization of the gross biopsy specimens in the catheterization laboratory may also improve the diagnostic yield (Daly et al., 2012). The design (Stibbs, 2023) of newer bioptomes may be superior in providing clean-cut surfaces and minimizing crush artifacts when compared with older generations of bioptomes, and a recent report indicated that the Jawz bioptome used in this paper is superior to the Cordis bioptome. In addition, disposable bioptomes are also likely to have sharper cutting surfaces than reusable bioptomes.
4 Discussion
The current study did not identify a detectable DCM phenotype throughout the study period of 7 months, with echocardiographic parameters measured for DCM phenotypes based on the criteria of LVDdn ≥ 1.63, LVDsn ≥ 0.92, FS ≤ 20.7%, and EF ≤ 46.7% (Visser et al., 2019; Wess et al., 2021; Bonagura and Visser, 2022), and on the basis of select cardiac biomarkers or endomyocardial histopathology. However, this research observed an interaction between treatment, breed, and day for LVIDsN and LVIDdN. The differences observed in a three-way interaction suggest that this could be a multifactorial disease that depends on the breed of dog, the diet they are eating, and the study duration.
4.1 Echocardiography
Overall, the beagles in the LAGF group had a higher LVIDsN at day 210 than the beagles in the LAGI group at day 210, but a similar LVIDsN to those in the HAGF and HAGI groups. Furthermore, significance was not observed in the mixed-breed hounds.
Recently, clinical studies have evaluated a potential link between grain-free diets, which are commonly rich in pulse ingredients and/or potatoes, and DCM (Kaplan et al., 2018; Ontiveros et al., 2019; Walker et al., 2021; Freeman et al., 2022; Owens et al., 2023). In each of these retrospective studies, the dogs were categorized by diet type, namely traditional (grain-inclusive) vs. non-traditional (pulse-rich) diets on the basis of pet owner-provided histories. In recent prospective observational studies (Freeman et al., 2022; Owens et al., 2023), statistical differences in LVIDdN or LVIDsN were noted between dogs with pulses or peas in their diet and dogs eating grain-inclusive diets. These studies concluded that some dogs eating non-traditional diets were more likely to develop the DCM phenotype, but that when switched to a traditional diet, their cardiac function improved (Kaplan et al., 2018; Ontiveros et al., 2019; Walker et al., 2021; Freeman et al., 2022). Furthermore, Freeman et al. (2022) noted that over a 9-month study, an improvement in the LVIDdN and LVIDsN of all dogs in the two study groups was observed, possibly due to all dogs receiving cardiac medication, and some receiving taurine supplementation (Freeman et al., 2022), and this can make isolating variables difficult. Another limitation of these previous studies was their failure to account for changes in BW that would alter any of the echocardiographic parameters normalized to BW. In a recent study (Partington et al., 2022), it was observed that overweight dogs can experience negative cardiac changes, such as systemic hypertension, cardiac remodeling, and systolic and diastolic dysfunction, and that, although wall dimensions can improve during weight loss, cardiac function was not observed to improve (Partington et al., 2022). To address this concern, we proposed using a calculated ideal BW and BSA for normalization and indexing of the left ventricular chamber and volume measurements in addition to the actual BW and BSA.
The current study was unable to isolate diet as a variable among the measured echocardiographic parameters of left ventricular size and systolic function, and there were no differences noted in the cardiac biomarkers among the four diet groups. Another potential explanation for the varying observations between the current study and past studies is the previous studies’ reliance on pet owner-provided diet histories, which may be inaccurate or incomplete. One study has noted large inaccuracies related to pet owners’ underestimation and overestimation of food allocations even when using various measuring devices (Coe et al., 2019). In the study by Kaplan et al. (2018), most of the dogs were found to be consuming only up to 62% of their predicted MER, and the other studies did not investigate if the dogs were meeting their caloric requirements. Other studies have shown that diet histories given by pet owners could influence the accuracy and completeness of the data collected depending on how the questions are designed and asked (MacMartin et al., 2015; Coe et al., 2020). Similarly, the World Small Animal Veterinary Association (WSAVA) provides a standardized diet history form (World Small Animal Veterinary Associations, 2013) for veterinarians; however, it is not explicitly stated if this was utilized in previous studies. It would be prudent for practitioners to share findings of clinical cases and search for etiologies of previously unknown disease causes. However, caution should be exercised when the categorization of dogs into research study groups is solely based on pet owner-provided dietary histories.
In contrast to previous literature, one study evaluated cardiac health in Siberian huskies eating grain-free diets with varying inclusion rates (15%, 30%, and 45%) of pulse ingredients plus a grain-inclusive control diet over 5 months (Singh et al., 2023). The results of this study showed that there were no significant differences in left ventricular diameter measurements, left ventricular volume measurements, EF, FS, sphericity index, cTnI, or NTproBNP among diet treatments at the end of the study. Another study evaluated the cardiac parameters in privately owned dogs of varying breeds, including mixed-breed dogs eating a vegan diet for 12 weeks (Cavanaugh et al., 2021). In this study, the LVIDd and LVIDdN measurements in the dogs eating the vegan diet were increased at baseline compared with at the end of the study; however, there was no significant difference in either measurement when compared with a control group eating a traditional diet over the same period. The current study’s diets were formulated to represent pulse and potato types that are currently being utilized in pet food at higher proportions (> 65%) than in commercially available food. Notably, the test diets were formulated to be the same on macronutrients, but during laboratory testing, some nutrients were not exact, such as amino acids, which were not controlled for, and this is a limitation of this study. With the interaction of breed, diet, and study duration observed in the current study and the inconsistent conclusion seen in the literature, further studies should investigate additional factors, such as genetics, age, and a longer duration of feeding trial, that may be involved in cardiac health.
4.2 Cardiac biomarkers
Cardiac troponin I is a non-specific indicator of myocardial injury and its presence in increased concentrations has been noted in a variety of heart diseases (Langhorn and Willesen, 2015). All cTnI concentrations throughout the study for all the dogs were low and reported to be less than 0.2 ng/mL. Natriuretic peptides are endogenous hormones released in response to increased myocardial stretch and stress, and NTproBNP has been researched extensively as a biomarker for identifying heart disease in dogs (Oyama et al., 2013; Kanno et al., 2016). NTproBNP concentrations of < 735 pmol/L suggest that significant cardiac disease is unlikely based on the assay used in this study, although biological variability has been shown in both healthy dogs and dogs with heart disease (Winter et al., 2017). One notable finding in our study was a NTproBNP value of 1,422 pmol/L on day 180 for one dog in the LAGI group; on days 0 and 90, we found that the NTproBNP concentrations were 600 pmol/L and 724 pmol/L for this dog, respectively. When the echocardiographic parameters LVIDdN, LVIDsN, EF, and FS were evaluated for this dog, at day 180, they were 1.61, 0.81, 69.5%, and 37.9%, respectively. On day 210, the same parameters were 1.66, 0.9, 60.8%, and 34.3%, respectively, thus suggesting that the dog’s cardiac function and left ventricular measurements were considered normal at the end of the study despite the NTproBNP value measured on day 180.
4.3 Limitations
Even though the dogs were acclimated to their housing assignments a minimum of 3 months prior to the start of the study and were socialized throughout the study, one major limitation of this study was that the purpose-bred dogs used in this study may not have been as acclimated to socialization or human interaction as privately owned dogs and may therefore have experienced surges in sympathetic tone during the evaluations. A second limitation could be the variable hydration status of the dogs during each evaluation. All the dogs had ad libitum access to water; however, little could be done to ensure that all dogs had the same hydration status during each evaluation. Even mild fluid deficits have been previously shown to have demonstrable hemodynamic effects and alter echocardiographic parameters (Fine, 2010). Another limitation of this study was that endomyocardial biopsy samples were obtained from the right ventricle, whereas DCM most commonly affects the left ventricle with varying degrees of right ventricular involvement. Access to the left ventricle would require a more invasive approach, entailing either a carotid arterial cutdown or a trans-septal puncture through the atrial septum. To reduce procedural times and the number of complications, the right ventricle was chosen as it is easily accessed via the jugular vein with a minimally invasive approach. In addition, more extensive testing of the endomyocardial biopsies could have been considered (Sabbah, 2000; Schipke et al., 2017). Our power analysis was based on a 7.5% change in ejection fraction; however, recognized variability occurs and was minimized with single-observer measurements (Visser et al., 2019). In addition to the echocardiographic parameters discussed, other possible cardiac measures, such as E-point-to-septal separation and the sphericity index, were not evaluated, and more advanced echocardiographic imaging tools such as strain, strain rate, and tissue Doppler, were not utilized. Another limitation is that it is also unknown if a study duration of 7 months is sufficient for the comparison of cardiac parameters in dogs eating different categories of diets, although this is the longest prospective study to date evaluating diet and cardiac function. Furthermore, this study did not take into account infrequent events that may be observed in a larger population of dogs. This study captured data relating only to a specific cohort of dogs fed diets with specific parameters and, although it was unable to identify any dietary correlation to DCM, additional research is clearly warranted.
4.4 Conclusion
There was a significant treatment effect among treatments for EF; however, all EFs were above the reference interval (≤ 46.7%). In addition, we did not identify a treatment effect for all other parameters and biomarkers in the dogs eating any of the four diets over the course of this study. This study did not detect the development of cardiac dysfunction throughout its duration through the echocardiographic parameters measured, select cardiac biomarkers, or endomyocardial biopsies. Future research should focus on isolating individual cases to identify the variable(s) and possible mechanisms underlying suspected diet-associated DCM in dogs.
Data availability statement
The original contributions presented in the study are included in the article/Supplementary Material. Further inquiries can be directed to the corresponding author.
Ethics statement
The animal study was approved by The IACUC Committee of Pet Food Solutions. The study was conducted in accordance with the local legislation and institutional requirements.
Author contributions
SC: writing—review and editing; writing—original draft; validation; supervision; project administration; methodology; investigation; funding acquisition; data curation; and conceptualization. SL: writing—review and editing; writing—original draft; methodology; investigation; formal analysis; and data curation. RB: writing—review and editing; validation; investigation; and data curation. SM: writing—review and editing; project administration; methodology; investigation; funding acquisition; formal analysis; and conceptualization. JT: writing—review and editing; validation; investigation; and data curation. RS: writing—review and editing; project administration; methodology; investigation; funding acquisition; data curation; and conceptualization. CZ: writing—review and editing; software; and formal analysis. KL: writing—review and editing; software; and formal analysis. BQ: writing—review and editing; writing—original draft; supervision; project administration; methodology; investigation; funding acquisition; data curation; and conceptualization.
Funding
The authors declare financial support was received for the research, authorship, and/or publication of this article. The study was funded by BSM Research LLC, with other financial support received from individuals, veterinarians, retailers, distributors, ingredient suppliers, manufacturers, and brands. Individuals and organizations that granted money to BSM Research LLC were not involved in the study design, study implementation, data analysis, manuscript writing, or publication submission. Organizations that granted money to BSM Research LLC included both those that were not referenced in the United States Food and Drug Administration’s communications regarding non-hereditary canine dilated cardiomyopathy and those that were.
Conflict of interest
SL, RB, and JT acknowledge receiving a donation from BSM Research LLC to the University of Missouri, Washington State University, and Kansas State University’s veterinary research accounts, respectively. SL and JT have worked as paid consultants for BSM Research LLC and SC, SM, RS, CZ, and BQ are all employees of BSM Partners LLC. BSM Partners LLC is a full service pet health industry consulting and research firm.
The remaining author declares that the research was conducted in the absence of any commercial or financial relationships that could be construed as a potential conflict of interest.
Publisher’s note
All claims expressed in this article are solely those of the authors and do not necessarily represent those of their affiliated organizations, or those of the publisher, the editors and the reviewers. Any product that may be evaluated in this article, or claim that may be made by its manufacturer, is not guaranteed or endorsed by the publisher.
Supplementary material
The Supplementary Material for this article can be found online at: https://www.frontiersin.org/articles/10.3389/fanim.2023.1271202/full#supplementary-material
References
Backus R. C., Cohen G., Pion P. D., Good K. L., Rogers Q. R., Fascetti A.J. (2003). Taurine deficiency in Newfoundlands fed commercially available complete and balanced diets. J. Am. Vet. Med. Assoc. 223 (8), 1130–1136. doi: 10.2460/javma.2003.223.1130
Backus R. C., Ko K. S., Fascetti A. J., Kittleson M. D., MacDonald K. A., Maggs D. J., et al. (2006). Low plasma taurine concentration in Newfoundland dogs is associated with low plasma methionine and cysteine concentrations and low taurine synthesis. J. Nutr. 136 (10), 2525–2533. doi: 10.1093/jn/136.10.2525
Beier P., Reese S., Holler P. J., Simak J., Tater G., Wess G. (2015). The role of hypothyroidism in the etiology and progression of dilated cardiomyopathy in Doberman Pinschers. J. Vet. Intern. Med. 29 (1), 141–149. doi: 10.1111/jvim.12476
Belanger M. C., Ouellet M., Queney G., Moreau M. (2005). Taurine-deficient dilated cardiomyopathy in a family of golden retrievers. J. Am. Anim. Hosp. Assoc. 41 (5), 284–291. doi: 10.5326/0410284
Bonagura J. D., Visser L. C. (2022). Echocardiographic assessment of dilated cardiomyopathy in dogs. J. Vet. Cardiol. 40, 15–50. doi: 10.1016/j.jvc.2021.08.004
Braunlin E. A., Shumway S. J., Bolman R. M., McDonald K. M., Ring W. S., Olivari M. T., et al. (1998). Usefulness of surveillance endomyocardial biopsy after pediatric cardiac transplantation. Clin. Transplant. 12, 184–189.
British Small Animal Veterinary Association. (2023). Available at: https://www.bsavalibrary.com/content/formulary/backmatter/canine-and-felinebodyweightbwtobodysurfaceareabsaconversiontables.
Brooks D., Churchill J., Fein K., Linder D., Michel K. E., Tudor K., et al. (2014). AAHA weight management guidelines for dogs and cats. J. Am. Anim. Hosp. Assoc. 50, 1–11. doi: 10.5326/JAAHA-MS-6331
Cahill R. J., Pigeon K., Strong-Townsend M. I., Drexel J. P., Clark G. H., Buch J. S. (2015). Analytical validation of a second generation immunoassay for the quantification of N-terminal pro-B-type natriuretic peptide in canine blood. J. Vet. Diagn. Invest. 27, 61–67. doi: 10.1177/1040638714562826
Cavanaugh S. M., Cavanaugh R. P., Gilbert G. E., Leavitt E. L., Ketzis J. K., Vieira A. B. (2021). Short-term amino acid, clinicopathologic, and echocardiographic findings in healthy dogs fed a commercial plant-based diet. PlosOne 16 (10), 1–15. doi: 10.1371/journal.pone.0258044
Chetboul V., Tissier R. (2012). Echocardiographic assessment of canine degenerative mitral valve disease. J. Vet. Cardiol. 14, 127–148. doi: 10.1016/j.jvc.2011.11.005
Clark S. D., Hsu C., McCauley S. R., de Godoy M. R. C., He F., Streeter R. M., et al. (2023). The impact of protein source and grain inclusion on digestibility, fecal metabolites, and fecal microbiome in adult canines. J. Anim. Sci. 101, 1–18. doi: 10.1093/jas/skad268
Coe J. B., O’Connor R. E., MacMartin C., Verbrugghe A., Janke K. A. (2020). Effects of three diet history questions on the amount of information gained from a sample of pet owners in Ontario, Canada. JAVMA 256, 469–478. doi: 10.2460/javma.256.4.469
Coe J. B., Rankovic A., Edwards T. R., Parr J. M. (2019). Dog owner’s accuracy measuring different volumes of dry dog food using three different measuring devices. Vet. Rec. 185 (19), 599–604. doi: 10.1136/vr.105319
Daly K. P., Marshall A. C., Vincent J. A., Zuckerman W. A., Hoffman T. M., Canter C. E. (2012). Endomyocardial biopsy and selective coronary angiography are low risk procedures in pediatric heart transplant recipients: results of a multicenter experience. J. Heart Lung Tranplant. 31, 398–409. doi: 10.1016/j.healun.2011.11.019
Donadelli R. A., Pezzali J. G., Oba P. M., Swanson K. S., Coon C., Varney J., et al. (2020). A commercial grain-free diet does not decrease plasma amino acids and taurine status but increases bile acid excretion when fed to Labrador retrievers. Trans. Anim. Sci. 4 (3), 1–12. doi: 10.1093/tas/txaa141
Dukes-McEwan J. M., Borgarelli A., Tidholm A., Vollmar C., Haggstrom J. (2003). Cardiomyopathy. Proposed guidelines for the diagnosis of canine idiopathic dilated cardiomyopathy. J. Vet. Cardiol. 5 (2), 7–19. doi: 10.1016/S1760-2734(06)70047-9
Fascetti A. J., Reed J. R., Rogers Q. R., Backus R. C. (2003). Taurine deficiency in dogs with dilated cardiomyopathy: 12 cases, (1997-2001). J. Am. Vet. Med. Assoc. 223 (8), 1137–1141. doi: 10.2460/javma.2003.223.1137
FDA. (2018). “Drug administration,” in FDA Investigating potential connection between diet and cases of canine heart disease (FDA). Available at: https://wayback.archive-it.org/7993/20201222194256/https://www.fda.gov/animal-veterinary/cvm-updates/fda-investigating-potential-connection-between-diet-and-cases-canine-heart-disease.
FDA. (2019a). FDA investigation into potential link between certain diets and canine dilated cardiomyopathy (FDA). Available at: https://www.fda.gov/animal-veterinary/outbreaks-and-advisories/fda-investigation-potential-link-between-certain-diets-and-canine-dilated-cardiomyopathy.
FDA. (2019b). “Vet-LIRN Update on Investigation into Dilated Cardiomyopathy,” in Vet-LIRN (FDA). Available at: https://www.fda.gov/animal-veterinary/science-research/vet-lirn-update-investigation-dilated-cardiomyopathy.
Fine D. M. (2010). Echocardiographic assessment of hemodynamic changes produced by two methods of inducing fluid deficit in dogs. J. Vet. Intern. Med. 24, 348–353. doi: 10.1111/j.1939-1676.2009.0448.x
Freeman L. M., Rush J. A., Adin D., Weeks K., Antoon K., Brethel S., et al. (2022). Prospective study of dilated cardiomyopathy in dogs eating nontraditional or traditional diets and in dogs with subclinical cardiac abnormalities. J. Vet. Intern. Med. 36 (2), 451–463. doi: 10.1111/jvim.16397
Freid K. J., Freeman L. M., Rush J. E. (2021). Retrospective study of dilated cardiomyopathy in dogs. J. Vet. Intern. Med. 35, 58–67. doi: 10.1111/jvim.15972
Harmon M. W., Leach S. B., Lamb K. E. (2017). Dilated cardiomyopathy in standard Schnauzers: Retrospective study of 15 cases. J. Am. Anim. Hosp. Assoc. 53, 38–44. doi: 10.5326/JAAHA-MS-6506
Isayama N., Uchimura Y., Sasaki K., Maeda E., Takahashi T., Watanabe M. (2022). Reference values for M-mode echocardiographic parameter in adult toy breed dogs. Front. Vet. Sci. 9. doi: 10.3389/fvets.2022.918457
Kanno N., Hori Y., Hidaka Y., Chikazawa S., Kanai K., Hoshi F., et al. (2016). Plasma atrial natriuretic peptide and N-terminal pro B-type natriuretic peptide concentrations in dogs with right-sided congestive heart failure. J. Vet. Med. Sci. 78 (4), 535–542. doi: 10.1292/jvms.15-0435
Kaplan J. L., Stern J. A., Fascetti A. J., Larsen J. A., Skolnik H., Peddle G. D., et al. (2018). Correction: Taurine deficiency and dilated cardiomyopathy in golden retrievers fed commercial diets. PlosOne 13 (12), e0210233. doi: 10.1371/journal.pone.0210233
Keene B. W., Kittleson M. E., Atkins C. E., Rush J. E., Eicker S. W., Pion P., et al. (1990). Modified transvenous endomyocardial biopsy technique in dogs. Am. J. Vet. Res. 51, 1169–1172.
Laflamme D. P. (1997). Development and validation of a body condition score system for dogs. Canine Pract. 22, 10–15.
Langhorn R., Willesen J. L. (2015). Cardiac troponins in dogs and cats. J. Vet. Internal Med. 30, 36–50. doi: 10.1111/jvim.13801
Leach S. B., Briggs M., Hansen L., Johnson G. S. (2022). Prevalence, geographic distribution, and impact on lifespan of a dilated cardiomyopathy-associated RNA-binding motif protein 20 variant in genotyped dogs. J. Vet. Cardiol. 40, 119–125. doi: 10.1016/j.jvc.2021.05.002
Li Q., Heaney A., Langenfeld-McCoy N., Boler B. V., Laflamme D. P. (2019). Dietary intervention reduces left atrial enlargement in dogs with early preclinical myxomatous mitral valve disease: A blinded randomized controlled study in 36 dogs. BMC Vet. Res. 15, 425. doi: 10.1186/s12917-019-2169-1
Liu B., Leach S. B., Pan W., Zheng F., Jia L., Zhou X., et al. (2020). Preliminary outcome of a novel edge-to-edge closure device to manage mitral regurgitation in dogs. Front. Vet. Sci. 17. doi: 10.3389/fvets.2020.597879
MacMartin C., Wheat H. C., Coe J. B., Adams C. L. (2015). Effect of question design on dietary information solicited during veterinarian-client interactions in companion animal practice in Ontario, Canada. J. Vet. Med. Assoc. 248, 1203–1214. doi: 10.2460/javma.246.11.1203
McCauley S. R., Clark S. D., Quest B., Streeter R., Oxford E. (2020). Review of canine dilated cardiomyopathy in the wake of diet-associated concerns. J. Anim. Sci. 98 (6), 1–20. doi: 10.1093/jas/skaa209
Meurs K. M. (2003). “Myocardial disease,” in Handbook of small animal practice. Eds. Morgan R., Bright R., Swartout M. (Philadelphia: WB Saunders), 101–102.
Muzzi R. A., de Araújo R. B., Muzzi L. A., Pena J. L., Silva E. F. (2003). Regurgitant jet area by Doppler color flow mapping: quantitative assessment of mitral regurgitation severity in dogs. J. Vet. Cardiol. 5, 33–38. doi: 10.1016/S1760-2734(06)70050-9
NRC. (2006). Nutrient requirements of dogs and cats. 10th ed (Washington, DC: The National Academy Press).
O’Brien P. J., Ianuzzo S. C., Moe G. W., Stopps T. P., Armstrong P. W. (1990). Rapid ventricular pacing of dogs to heart failure: biochemical and physiological studies. Can. J. Physiol. Pharmacol. 68 (1), 34–39. doi: 10.1139/y90-004
Ontiveros E. S., Whelchel B., Yu J., Kaplan J., Sharpe A., Fousse S., et al. (2019). Development of plasma and whole blood taurine reference ranges and identification of dietary features associated with taurine deficiency and dilated cardiomyopathy in golden retrievers: A prospective, observational study. PlosOne 15 (5), 1–25. doi: 10.1371/journal.pone.0233206
Owens E. J., LeBlanc N. L., Freeman L. M., Scollan K. F. (2023). Comparison of echocardiographic measurements and cardiac biomarkers in healthy dogs eating nontraditional or traditional diets. J. Vet. Intern. Med. 37, 37–46. doi: 10.1111/jvim.16606
Oyama M. A., Boswood A., Connolly D. J., Ettinger S. J., Fox P. R., Gordon S. G., et al. (2013). Clinical usefulness of an assay for measurement of circulating N-terminal pro-B-type natriuretic peptide concentration in dogs and cats with heart disease. J. Am. Vet. Med. Assoc. 243 (1), 71–82. doi: 10.2460/javma.243.1.71
Panciera D. L. (1994). Hypothyroidism in dogs: 66 cases (1987-1992). J. Am. Vet. Med. Assoc. 204 (5), 761–767.
Panciera D. L. (2001). Conditions associated with canine hypothyroidism. Vet. Clin. North Am. Small Anim. Pract. 31 (5), 935–950.
Partington C., Hodgkiss-Geere H., Woods G. R. T., Dukes-McEwan J., Flanagan J., Biourge V., et al. (2022). The effect of obesity and subsequent weight reduction on cardiac structure and function in dogs. BMC. Vet. Res. 18, 351. doi: 10.1186/s12917-022-03449-4
Pezzali J. G., Acuff H. L., Henry W., Alexander C., Swanson K. S., Aldrich C. G. (2020). Effects of different carbohydrate sources on taurine status in healthy beagle dogs. J. Anim. Sci. 98, 1–9. doi: 10.1093/jas/skaa010
Phillips D. E., Harkin K. R. (2003). Hypothyroidism and myocardial failure in two Great Danes. J. Am. Anim. Hosp. Assoc. 39 (2), 133–137. doi: 10.5326/0390133
Quest B. W., Leach S. B., Garimella S., Konie A., Clark S. D. (2022). Incidence of canine dilated cardiomyopathy diagnosed at referral institutes and grain-free pet food store sales: A retrospective survey. Front. Anim. Sci. 3 (3846227). doi: 10.3389/fanim.2022.846227
Quilliam C., Reis L. G., Ren Y., Ai Y., Weber L. P. (2023. Effects of a 28-day feeding trial of grain-containing versus pulse-based diets on cardiac function, taurine levels and digestibility in domestic dogs. PlosOne,18(5) e0285381. doi: 10.1371/journal.pone.0285381
Reilly L. M., He F., Clark L., de Godoy M. R. C. (2021). Longitudinal assessment of taurine and amino acid concentration in dogs fed a green lentil diet. J. Anim. Sci. 99 (11), 1–16. doi: 10.1093/jas/skab315
Rishniw M., Erb H. N. (2000). Evaluation of four 3-dimensional echocardiographic methods of assessing left atrial size in dogs. J. Vet. Intern. Med. 14, 429–435. doi: 10.1111/j.1939-1676.2000.tb02252.x
Sabbah H. N. (2000). Apoptotic cell death in heart failure. Cardio. Res. 45, 704–712. doi: 10.1016/S0008-6363(99)00348-X
Santilli R. A., Battaia S., Perego M., Tursi M., Grego E., Marzufero C., et al. (2017). Bartonella-associated inflammatory cardiomyopathy in a dog. J. Vet. Cardiol. 19 (1), 74–81. doi: 10.1016/j.jvc.2016.07.005
Schipke J., Brandenberger C., Rajces A., Manninger M., Alogna A., Post H., et al. (2017). Assessment of cardiac fibrosis: A morphometric method comparison for collagen quantification. J. Appl. Physiol. 122, 1019–1030. doi: 10.1152/japplphysiol.00987.2016
Scott-Moncrieff J. C. (2012). Thyroid disorders in the geriatric veterinary patient. Vet. Clin. Small Anim. Pract. 42 (4), 707–725. doi: 10.1016/j.cvsm.2012.04.012
Singh P., Banton S., Raheb S., Templeman J. R., Saunders-Blades J., Kostiuk D., et al. (2023). The pulse of it: The effects of zero to forty-five percent pulse inclusion in canine diets on cardiac health. J. Nutr. 153 (5), 1461–1475. doi: 10.1016/j.tjnut.2023.03.027
Sleeper M. M., Henthorn P. S., Vijayasarathy C., Dambach D. M., Bowers T., Tijskens P., et al. (2002). Dilated cardiomyopathy in juvenile Portuguese Water Dogs. J. Vet. Intern. Med. 16 (1), 52–62. doi: 10.1111/j.1939-1676.2002.tb01606.x
Stibbs P. (2023). ). Jawz Endomyocardial biopsy forceps from argon medical: A review of safety and efficacy based on literature review and bench model comparison With the cordis bioptome. Sci. Open Posters. doi: 10.14293/S2199-1006.1.SOR-.PPEZMK7.v1
Streeter R. M., Clark S. D., McCauley S. R., Leach S. B., Quest B. (2022). “Evaluation of concurrent plasma, whole blood, and cardiac muscle taurine and carnitine concentrations in a group of purpose-bred mongrel dogs,” in American Academy of Veterinary Nutrition (AAVN)’s 22nd Annual Clinical Nutrition & Research Symposium, Austin, Texas (American Academy of Veterinary Nutrition).
Thomas W. P., Gaber C. E., Jacob G. J., Kaplan P. M., Lombard C. W., Moise N. S., et al. (1993). Recommendations for standards in transthoracic two-dimensional echocardiography in the dog and cat. J. Vet. Intern. Med. 7, 247–252. doi: 10.1111/j.1939-1676.1993.tb01015.x
Tidholm A., Jonsson L. (2005). Histologic characterization of canine dilated cardiomyopathy. Vet. Pathol. 42, 1–8. doi: 10.1354/vp.42-1-1
Visser L. C., Ciccozzi M. M., Sintov D. J., Sharpe A. N. (2019). Echocardiographs quantitation of left heart size and function in 122 healthy dogs: A prospective study proposing reference intervals and assessing repeatability. J. Vet. Int. Med. 33 (5), 1909–1920. doi: 10.1111/jvim.15562
Vollmar A. C., Fox P. R., Servet E., Biourge V. (2013). Determination of the prevalence of whole blood taurine in Irish Wolfhound dogs with and without echocardiographic evidence of dilated cardiomyopathy. J. Vet. Cardiol. 15 (3), 189–196. doi: 10.1016/j.jvc.2013.03.005
Vollmar C., Vollmar A., Keene B. W., Fox P. R., Reese S., Kohn B. (2019). Dilated cardiomyopathy in 151 Irish Wolfhounds: Characteristic clinical findings, life expectancy and causes of death. Vet. J. 245, 15–21. doi: 10.1016/j.tvjl.2018.12.018
Walker A. L., DeFrancesco T., Bonagura J., Keene B., Meurs K., Tou S., et al. (2021). Association of diet with clinical outcomes in dogs with dilated cardiomyopathy and congestive heart failure. J. Vet. Cardiol. 40, 99–109. doi: 10.1016/j.jvc.2021.02.001
Wess G. A., Bauer A., Kopp A. (2021). Echocardiographic reference intervals for volumetric measurements of the left ventricle using Simpson’s method of discs in 1331 dogs. J. Vet. Intern. Med. 35, 724–738. doi: 10.1111/jvim.16089
Wess G., Schulze A., Butz V., Simak J., Killich M., Keller L. J., et al. (2010). Prevalence of dilated cardiomyopathy in Doberman Pinschers in various age groups. J. Vet. Intern. Med. 24 (3), 533–538. doi: 10.1111/j.1939-1676.2010.0479.x
Winter R. L., Saunders A. B., Gordon S. G., Buch J. S., Miller M. W. (2017). Biological variability of N-terminal pro-brain natriuretic peptide in healthy dogs and dogs with myxomatous mitral valve disease. J. Vet. Cardiol. 19, 124–131. doi: 10.1016/j.jvc.2016.11.001
Winter R. L., Saunders A. B., Gordon S. G., Miller M. W., Sykes K. T., Suchodolski J. S., et al. (2014). Analytical validation and clinical evaluation of a commercially available high-sensitivity immunoassay for the measurement of troponin I in humans for use in dogs. J. Vet. Cardiol. 16, 81–89. doi: 10.1016/j.jvc.2014.03.002
World Small Animal Veterinary Associations. (2013). Available at: https://wsava.org/wp-content/uploads/2020/01/Diet-History-Form.pdf.
Keywords: dilated cardiomyopathy, grain-free, natriuretic peptide, nutrition, troponin
Citation: Leach SB, Clark SD, Baumwart RD, McCauley SR, Thomason JD, Streeter RM, Zumbaugh CA, Lamb K and Quest BW (2023) Prospective evaluation of echocardiographic parameters and cardiac biomarkers in healthy dogs eating four custom-formulated diets. Front. Anim. Sci. 4:1271202. doi: 10.3389/fanim.2023.1271202
Received: 01 August 2023; Accepted: 12 September 2023;
Published: 06 October 2023.
Edited by:
Anna Katharine Shoveller, University of Guelph, CanadaReviewed by:
Britta Dobenecker, Ludwig Maximilian University of Munich, GermanyDennis E. Jewell, Kansas State University, United States
Sonja Fonfara, University of Guelph, Canada
Mark Rishniw, Cornell University, United States
Copyright © 2023 Leach, Clark, Baumwart, McCauley, Thomason, Streeter, Zumbaugh, Lamb and Quest. This is an open-access article distributed under the terms of the Creative Commons Attribution License (CC BY). The use, distribution or reproduction in other forums is permitted, provided the original author(s) and the copyright owner(s) are credited and that the original publication in this journal is cited, in accordance with accepted academic practice. No use, distribution or reproduction is permitted which does not comply with these terms.
*Correspondence: Stephanie D. Clark, drclark@bsmpartners.net