- 1Department of Animal Science, The University of Tennessee Institute of Agriculture and AgResearch, Knoxville, TN, United States
- 2Department of Chemistry, University of Tennessee Knoxville, Knoxville, TN, United States
Introduction: A higher estrus-associated temperature (HEAT) is a hallmark feature in sexually active females; however, its functional importance is unclear. Our objective was to examine the relationship between HEAT and the preovulatory follicular fluid metabolome. It was hypothesized that HEAT is functionally important as it affects fertility-related components in the preovulatory follicle.
Methods: Estrus was synchronized in non-lactating Jersey cows. A Thermochron iButton temperature data logger was affixed to blank controlled internal drug release (CIDR) devices and intravaginally inserted after CIDR device removal. The follicular fluid was aspirated 14.9 h + 3.3 h after an animal first stood to be mounted. Regression models were performed using metabolite abundance and HEAT variables. Best-fit models were determined using backward manual selection (p < 0.05).
Results: A total of 86 metabolites were identified in cow follicular fluid samples. The vaginal temperature at first mount and when it was expressed as a change from baseline was positively related to the abundance of four metabolites (i.e., taurine, sn-glycerol 3-phosphate, glycine, and cysteine) and negatively related to one metabolite (i.e., serine). The vaginal temperature at the first standing mount was related to the differential abundance of two metabolites (i.e., jasmonate and N-carbamoyl-L-aspartate). Three metabolites were related to the maximum vaginal temperature (i.e., N-carbamoyl-L-aspartate, uracil, and glycodeoxycholate). When expressed as a change from baseline, the maximum vaginal temperature was related to the differential abundances of uracil, uric acid, and 6-phospho-D-gluconate. The time taken to reach maximum vaginal temperature was related to N-carbamoyl-L-aspartate, glycodeoxycholate, jasmonate, and tricarballylic acid. Pertaining to the combination of HEAT and its duration, the area under the curve associated with the time between the first increase in vaginal temperature and the maximum vaginal temperature was related to 6-phospho-D-gluconate, sulfolactate, guanidoacetic acid, and aspartate. The area under the curve associated with the time between the initial vaginal temperature increase and up to 10 h after a cow first stood to be mounted or when a cow’s temperature returned to baseline was related to the differential abundances of uracil, sn-glycerol 3-phosphate, methionine sulfoxide, and taurodeoxycholate.
Discussion: Our findings support the notion that HEAT is related to changes in the preovulatory follicular fluid metabolites involved in energy metabolism, thermoregulation, and oxidative stress management.
1 Introduction
Although the basis of fertility in cattle is multifaceted, events occurring at or around estrus are essential for reproductive success. Estrus signifies the major endocrine transition in which the preovulatory follicle prepares for ovulation. To this end, the estradiol-induced gonadotropin-releasing hormone (GnRH)—luteinizing hormone (LH) surge (Stumpf et al., 1991) sets the stage for ovulation 24 h to 30 h thereafter (Malhi et al., 2005; Saumande and Humblot, 2005; Ginther et al., 2013). The mural granulosa undergoes luteinization (i.e., the beginnings of corpus luteum formation) and the oocyte contained within the preovulatory follicle undergoes meiotic resumption and progression to metaphase II (Smith et al., 1994; Duggavathi and Murphy, 2009).
Increasing estradiol levels during the follicular phase of the estrous cycle underlie much of the behavioral changes leading up to and during estrus (Lyimo et al., 2000). Female cattle approaching estrus become restless, may walk up to four times more than those not exhibiting estrus, and interact with others forming sexually active groups (Kiddy, 1977; Diskin and Sreenan, 2000; Sveberg et al., 2013). Other secondary signs of estrus activity include, but are not limited to, chin-resting, head butting, and vulva sniffing (Kerbrat and Disenhaus, 2004). The most definitive sign of estrus, however, is the willingness of a female to stand to be mounted by another cow. Interestingly, several studies report a positive relationship between the level of activity in an estrual female and the probability of pregnancy outcome (López-Gatius et al., 2005; Madureira et al., 2015; Madureira et al., 2019; Tippenhauer et al., 2023).
Increased body temperature is another hallmark feature of estrus-active females, even under thermoneutral environmental conditions (Piccione et al., 2003; Sakatani et al., 2016). Higher estrus-associated temperatures (HEAT) may range from 0.3°C to 1.3°C or greater above the baseline (Redden et al., 1993; Kyle et al., 1998; Piccione et al., 2003; Fisher et al., 2008; Suthar et al., 2011; Randi et al., 2018). An estrus-related increase in body temperature is short in duration (5 h to 18 h; Redden et al., 1993; Kyle et al., 1998; Sakatani et al., 2016); it typically occurs around the LH surge and persists for several hours thereafter (Fisher et al., 2008; Miura et al., 2017). In addition to being an informative indicator of estrus (Redden et al., 1993; Sakatani et al., 2016), varying levels of HEAT may be functionally relevant.
In support of HEAT’s functional impact on fertility important events specific to the cumulus–oocyte complex, an acute, but short episode of exposure at 41°C for 4 h to 6 h in vitro, induces germinal vesicle breakdown earlier than in thermoneutral controls (Edwards et al., 2005; Hooper et al., 2015) without compromising embryo development after fertilization (Rowinski et al., 2021). In terms of impacts on other preovulatory follicle components, two transcripts (i.e., calreticulin and serpin family F member 2) in granulosa cells have been shown by others to potentiate ovulation (Tsafriri et al., 1989; Tokuhiro et al., 2015). These transcripts were upregulated by varying degrees of hyperthermia occurring after a pharmacologically induced LH surge (Klabnik et al., 2022). Calreticulin can enhance the binding of bradykinin to its receptor (reviewed in Bedard et al., 2005). Interestingly, higher bradykinin levels were noted in the preovulatory follicular fluid of the same cows exhibiting varying levels of hyperthermia, from which the granulosa samples (Klabnik et al., 2022) were collected (Rispoli et al., 2019). Bradykinin has been shown to potentiate follicular rupture in other species (Yoshimura et al., 1988; Hellberg et al., 1991).
Follicular fluid provides the necessary substrates for follicle growth and progression, oocyte developmental competence, and subsequent embryo viability (Leroy et al., 2011; Sirard, 2011). The differences in follicular fluid metabolome profiles have recently been associated with fertility, follicle maturity, or follicle progression after the onset of estrus (Read et al., 2021; Read et al., 2022; Hessock et al., 2023). Although changes in milk, serum, and urine metabolome profiles have been noted in cattle experiencing elevated body temperature resulting from chronic heat stress (Liao et al., 2018; Yue et al., 2020), it remains unclear whether an acute episode of HEAT is associated with changes in the follicular fluid metabolome of the preovulatory follicle. Thus, the objective of this study was to examine the relationship between HEAT and the preovulatory follicular fluid metabolome.
2 Materials and methods
2.1 Animals and synchronization protocol
Animal use during this study occurred during the month of May. Institutional animal care and use approval at the University of Tennessee, Knoxville, TN, USA, was obtained before the onset of this study. Non-lactating Jersey cows located at a University of Tennessee AgResearch and Education Center grazed the same fescue-based pasture and were provided ad libitum access to minerals (Burkmann Nutrition; Danville, KY, USA). Cows ranged in age from 2.5 years to 7.7 years (5.1 years ± 1.4 years), weighed between 365.6 kg and 585.1 kg (508.3 kg ± 61.6 kg), and had a body condition score ranging from 2.5 to 3.5 (3.1 ± 0.4). Toward the synchronization of a first wave dominant–preovulatory follicle (Figure 1), GnRH was administered (Cystorelin®; 100 µg; i.m.; Boehringer Ingelheim; Ingelheim am Rhein, Germany) and a controlled internal drug release (CIDR) device was placed intravaginally (1.38 g progesterone; Eazi-Breed CIDR; Zoetis Animal Health, Kalamazoo, MI, USA). Nine days later, the CIDR device was removed and prostaglandin F2α (PGF2α; 12.5 mg of dinoprost tromethamine/mL Lutalyse® HighCon; Zoetis Animal Health, Kalamazoo, MI, USA) was administered. GnRH was administered 48 hours after PGF2α (when progesterone levels were < 1 ng/mL; Supplementary Figure 1) and a new CIDR device was inserted. PGF2α was administered 7 days later, after CIDR device removal. The cattle were visually monitored for behavioral signs of estrus; this took place every 4 h after PGF2α administration and continued until a cow displayed estrus activity (~ 31 h after PGF2α). Thereafter, cows were continually monitored by a team of individuals trained in the visual observation of estrus activity. The onset of estrus was defined as the first time a cow was observed to stand to be mounted by another. Of the 16 cows used for this study, 14 exhibited estrus (87.5%).
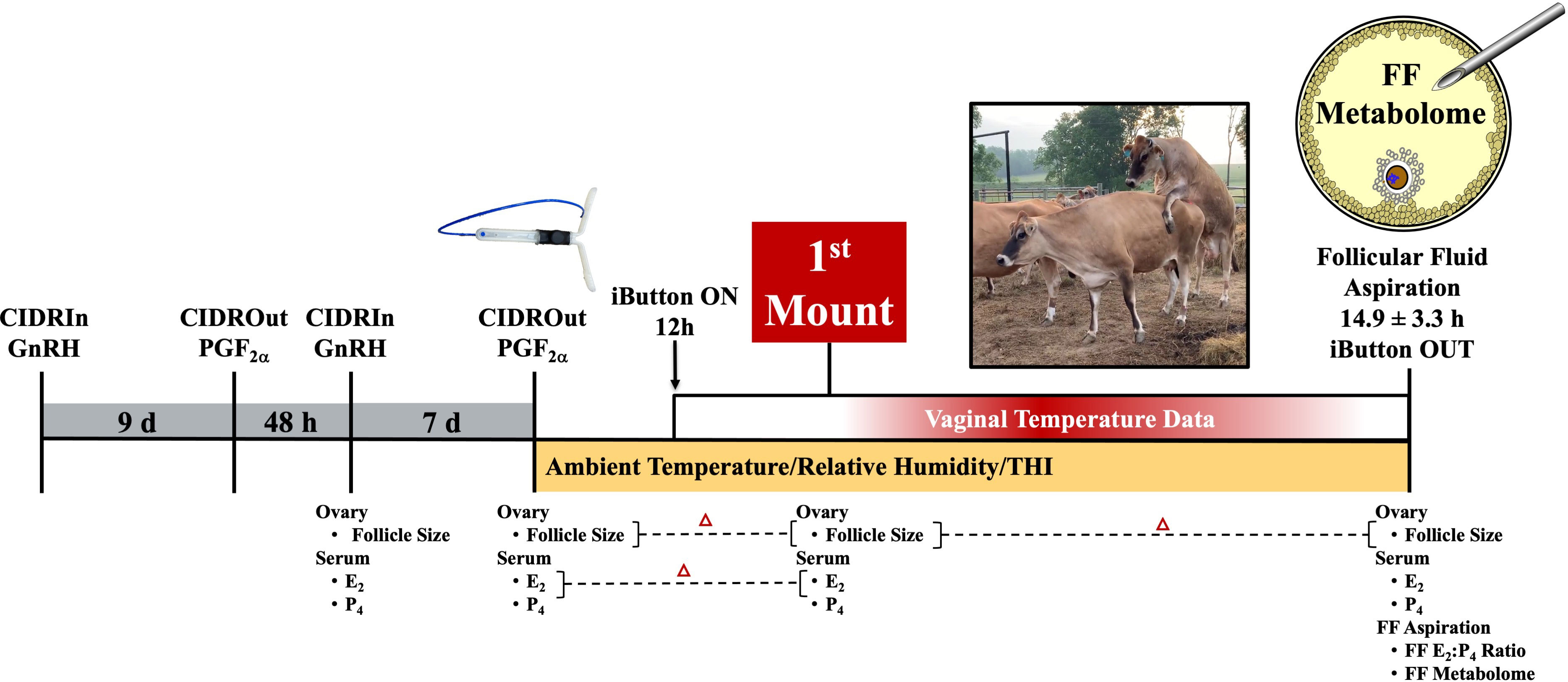
Figure 1 Study schematic depicting synchronization scheme and data collected at each of the different time points relative to when follicle aspiration occurred. GnRH, gonadotropin-releasing hormone; CIDR, controlled internal drug release; PGF2α, prostaglandin F2α; E2, estradiol; P4, progesterone; FF, follicular fluid.
2.2 Vaginal and ambient temperature data
Cows’ vaginal temperature was recorded using a Thermochron iButton™ 1922L data logger (Embedded Data Systems, Lawrenceburg, KY, USA) affixed to a blank CIDR device (i.e., containing no progesterone) consistent with the method described by Burdick et al. (2012). Cows’ vaginal temperature was recorded every 3 min (0.5°C resolution), beginning 12 h after PGF2α administration and continuing until iButton removal, which occurred immediately before preovulatory follicle aspiration. During the time when estrus expression was induced, the ambient temperature and humidity were recorded onsite (hourly) using the HOBO U23 Pro v2 data logger (Onset Computer Corporation, Bourne, MA, USA). Data for the ambient temperature and humidity from the start of the pre-synchronization protocol to PGF2α administration to induce estrus were collected at a local meteorological station and averaged every 2 h. The temperature humidity index (THI) was calculated in accordance with the method described by Abbott et al. (2018).
2.3 Ovarian ultrasound and follicular fluid aspiration
The largest follicle diameter (> 7 mm) was recorded at GnRH, PGF2α, first mount, and follicle aspiration using an IBEX EVO® II ultrasound and eL7 linear probe (E.I. Medical Imaging, Loveland, CO, USA; Figure 1). The neat follicular fluid was successfully collected from the preovulatory follicles of 13 out of the 14 estrus cows through ultrasound-guided transvaginal aspiration (18-g needle; Rispoli et al., 2019; Hessock et al., 2023) using a Samsung HM70A ultrasound and CFA-9 convex probe 14.9 h± 3.3 h after a cow was first observed to stand to be mounted by another cow.
2.4 Serum and follicular fluid hormone assays
The blood samples were collected from the coccygeal vein/artery (Figure 1) and processed in accordance with Hessock et al. (2023). Serum estradiol (E2) was evaluated using a radioimmunoassay (Kirby et al., 1997), with intra- and inter-assay coefficients of variation (CVs) of 3.6% and 6.7%, respectively. The sensitivity of the serum estradiol assay was 1.03 pg/mL. The follicular fluid E2 was analyzed using the DetectX® Serum 17β-Estradiol ELISA Kit (Arbor Assays, Ann Arbor, MI, USA; the sensitivity was 2.21 pg/mL). The intra- and inter-assay CVs for the follicular fluid E2 were 1.7% and 7.3%, respectively. The serum and follicular fluid progesterone (P4) concentrations were measured using ImmuChem™ Double Antibody Radioimmunoassay Kit (MP Biomedicals, LLC, Orangeburg, NY, USA). The intra- and inter-assay CVs for serum P4 were 8.0% and 4.2%, respectively; whereas the follicular fluid progesterone intra- and inter-assay CVs were 4.4% and 5.7%, respectively. The sensitivity of the progesterone assays was 0.11 ng/mL.
2.5 HEAT data—pertinent variables of interest
Vaginal temperature, recorded every 3 min, was averaged every 30 min. The HEAT variables of interest are defined in Table 1 and highlighted in Figure 2. A baseline temperature was calculated for each cow. The baseline temperature was defined as the average vaginal temperature of those recorded between the first temperature, which was taken 12 h after PGF2α, and those recorded over the next 20 h. An increase in vaginal temperature related to HEAT was defined as the first time when the vaginal temperature was 0.3°C higher than baseline, with the increase persisting for 3 h or more (Clapper et al., 1990). Cow vaginal temperature when first observed to stand to be mounted by another cow was noted as first mount. The maximum HEAT vaginal temperature was denoted as VTmax. Both the first mount and VTmax temperature, when expressed as a change from baseline, were also considered. The time to VTmax was the number of hours from the first mount to VTmax. The area under the different portions of the HEAT curves (AUC1: time when vaginal temperature first increased to Vmax; AUC2: time when the vaginal temperature first increased up to 10 h after estrus onset or when the vaginal temperature returned to baseline) was calculated for each cow, in accordance with Pruessner et al. (2003), using the trapezoid formula with the baseline temperature as a lower limit.
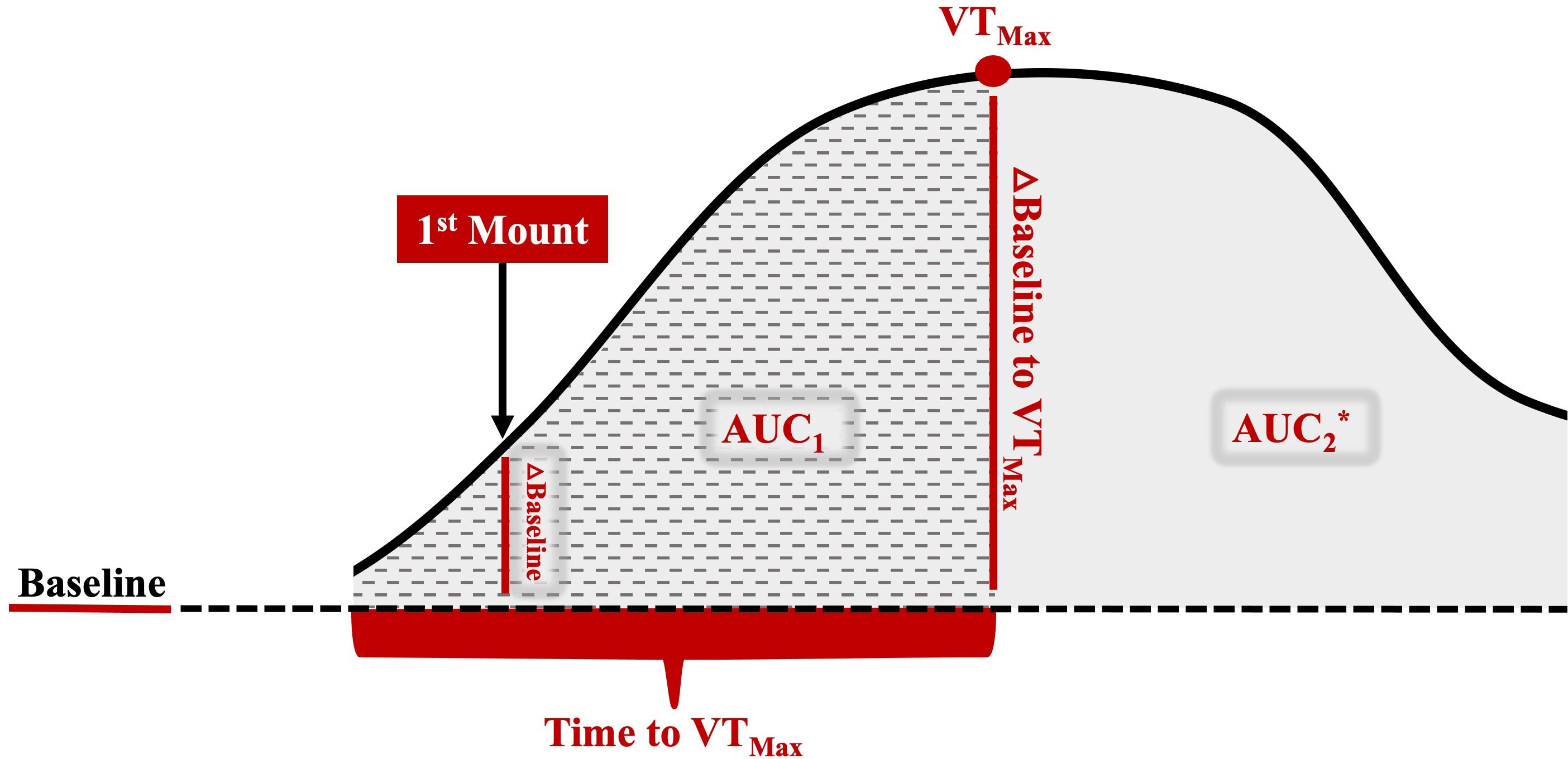
Figure 2 Representative image of the different HEAT variables of interest used to examine the relationship between HEAT and the preovulatory follicular fluid metabolome. The baseline is the average vaginal temperature of those recorded between the first temperature, which was taken 12 h after PGF2α, and those recorded over the next 20 h, which was well before HEAT-related increases. A HEAT-related vaginal temperature increase was defined as the time that the vaginal temperature was 0.3°C higher than the baseline, with this increased temperature observed for 3 or more hours thereafter (Clapper et al., 1990). First mount: the vaginal temperature when the cow was first observed to stand to be mounted by another cow; △ baseline to 1M: the vaginal temperature at first mount minus the baseline; VTMax: the maximum HEAT vaginal temperature; △ baseline to VTMax: the maximum vaginal temperature minus the baseline; time to VTMax: hours from first mount to VTMax; AUC1: area under the curve—time when the vaginal temperature first increased to VTMax; AUC2: area under the curve—time when the vaginal temperature first increased up to 10 h after estrus onset or when the vaginal temperature returned to the baseline; △=change; *AUC2 includes AUC1 area and remaining solid gray shaded area.
2.6 Metabolome profiling of follicular fluid
Ultra-high-performance liquid chromatography–high resolution mass spectrometry (UHPLC-HRMS) was performed at the University of Tennessee Knoxville Biological and Small Molecule Mass Spectrometry Core Facility [Research Resource Identifier (RRID):SCR_021368], as previously described by Horn et al. (2022). In brief, metabolites were extracted from 50 µL of each follicular fluid sample (n = 13) with a solution of 20: 40: 40 water/methanol/acetonitrile with 0.1 M formic acid. The metabolites were then separated on a Synergi Hydro RP, 2.5 μm, 100 mm × 2.0 mm column (Phenomenex, Torrance, CA, USA). The solvents for the mobile phase to elute metabolites were (A) 97: 3 methanol to water with 11 mM tributylamine and 15 mM acetic acid and (B) 100% methanol. The solvent gradient from 0 min to 5 min was (A) 100% and (B) 0%; from 5 min to 13 min, it was (A) 80% and (B) 20%; from 13 min to 15.5 min, it was (A) 45% and (B) 55%; from 15.5 min to19 min, it was (A) 5% and (B) 95%; and from 19 min to 25 min, it was (A) 100% and (B) 0%, with a flow rate of 0.2 μL per min. The mass spectrometry was performed using an Exactive™ Plus Orbitrap™ mass spectrometer (Thermo Fisher Scientific, Waltham, MA, USA) fitted with an electrospray ionization probe operating in negative mode. The scan range was 72000 m/z to 1,000 m/z, with a resolution of 140,000 and an acquisition gain control of 3 × 106 (Greene et al., 2020).
The files generated by HRMS in the Xcalibur® (RAW) format were converted to an open-source mzML format (msconvert; ProteoWizard package) and then processed using the Metabolomic Analysis and Visualization Engine (MAVEN; mzroll software, Princeton University) for an untargeted analysis of the full-scan LC-MS data. A group algorithm for non-linear retention time alignment was used to pick peaks, integrate intensities, and visualize extracted ion chromatograms. The metabolites were identified based on peak shape, signal-to-noise ratio, and retention time. The MAVEN preprocessed peak data were used for further statistical analysis.
2.7 Statistical analyses
The analyses were conducted using R Studio (version 2023.3.0.386; RStudio Team 2020, Boston, MA, USA). Data were checked for normality using the Shapiro–Wilk test and log-transformed when necessary. The outliers identified using the interquartile range (IQR) method were removed. Supplementary Table 1 denotes the metabolites that were log-transformed or had outliers removed for statistical analyses. To examine the relationship between the different features of HEAT (independent variable of interest, e.g., VTMax and other HEAT variables defined and listed in Table 1) with metabolite abundance (dependent variable), hierarchical linear regression models were performed using a backward stepwise approach to derive the best-fit models for each metabolite. The cow and other related independent variables used to derive the best-fit models were utilized as covariates [i.e., variables that may affect response variables but were not of direct interest in our study; these included cow age, weight, body condition score (BCS), proestrus length, serum estradiol and progesterone, follicular fluid E2-to-P4 ratio, follicle size, and THI]. The covariates were included in the model only if they were deemed significant in an initial simple linear regression (p < 0.1). The final models included the HEAT variables of interest (significant if p-value ≤ 0.05) and the covariates significant at a p-value < 0.05.
3 Results
3.1 Proestrus length, follicle size, and ambient conditions
The time from PGF2α to the first observed standing mount (i.e., proestrus length) was 43.1 h ± 8.4 h (range 31.1 h–63.9 h). The preovulatory follicle size at aspiration was 16.2 mm ± 1.3 mm (range 12.9 mm–18.5 mm). At the time of follicle aspiration, serum progesterone and estradiol were 0.13 ng/mL ± 0.02 ng/mL (range 0.1 ng/mL–0.37 ng/mL) and 4.42 pg/mL ± 0.74 pg/mL (range 1.03 pg/mL–9.14 pg/mL), respectively. The ambient temperature, relative humidity, and the THI from the start of the pre-synchronization protocol through to the time of the final follicular fluid aspiration are shown in Figure 3. The estrus-associated vaginal temperature curves for each individual cow (n = 13) are shown in Figure 4.
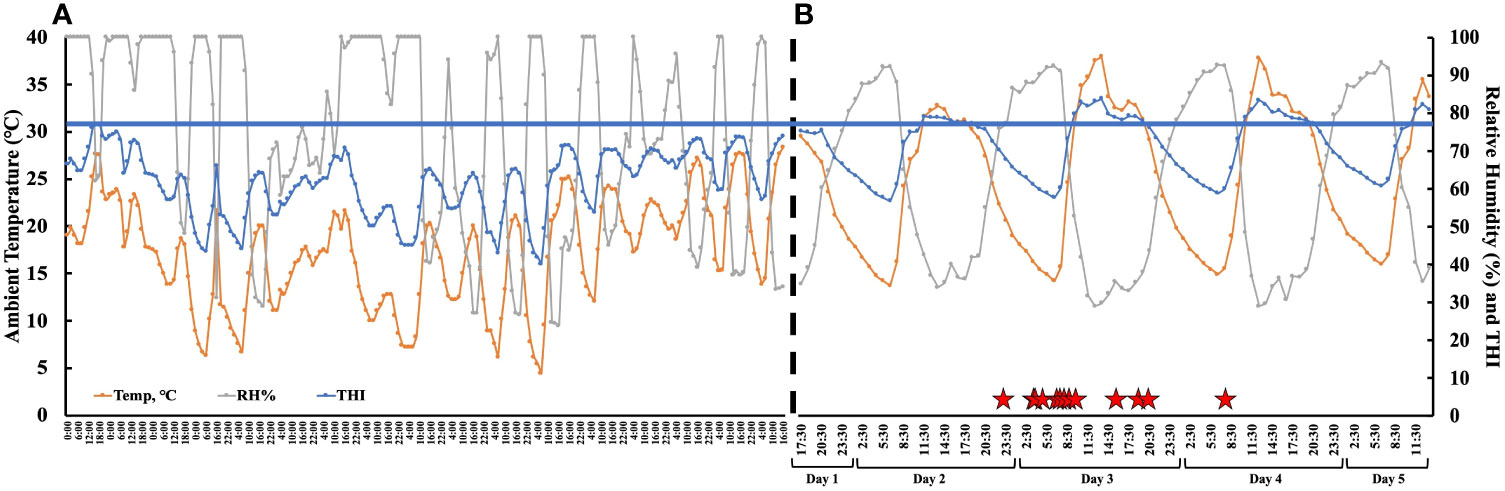
Figure 3 (A) The ambient temperature, relative humidity, and temperature humidity index (THI) collected from a local meteorological station from the start of pre-synchronization protocol to the PGF2α administration to induce estrus. The weather data were averaged every 2 hours. The black dotted line represents the end of the meteorological data and the beginning of the hourly onsite environmental data. (B) The hourly ambient temperature, relative humidity, and temperature humidity index (THI) from PGF2α administration to induce estrus (day 1—17:30) to after final follicle aspiration (day 5—11:30). The red stars denote the time each animal first stood to be mounted. The blue line represents the THI threshold (77) for non-lactating dairy cattle (Ouellet et al., 2021). The THI was calculated as per Abbott et al. (2018).
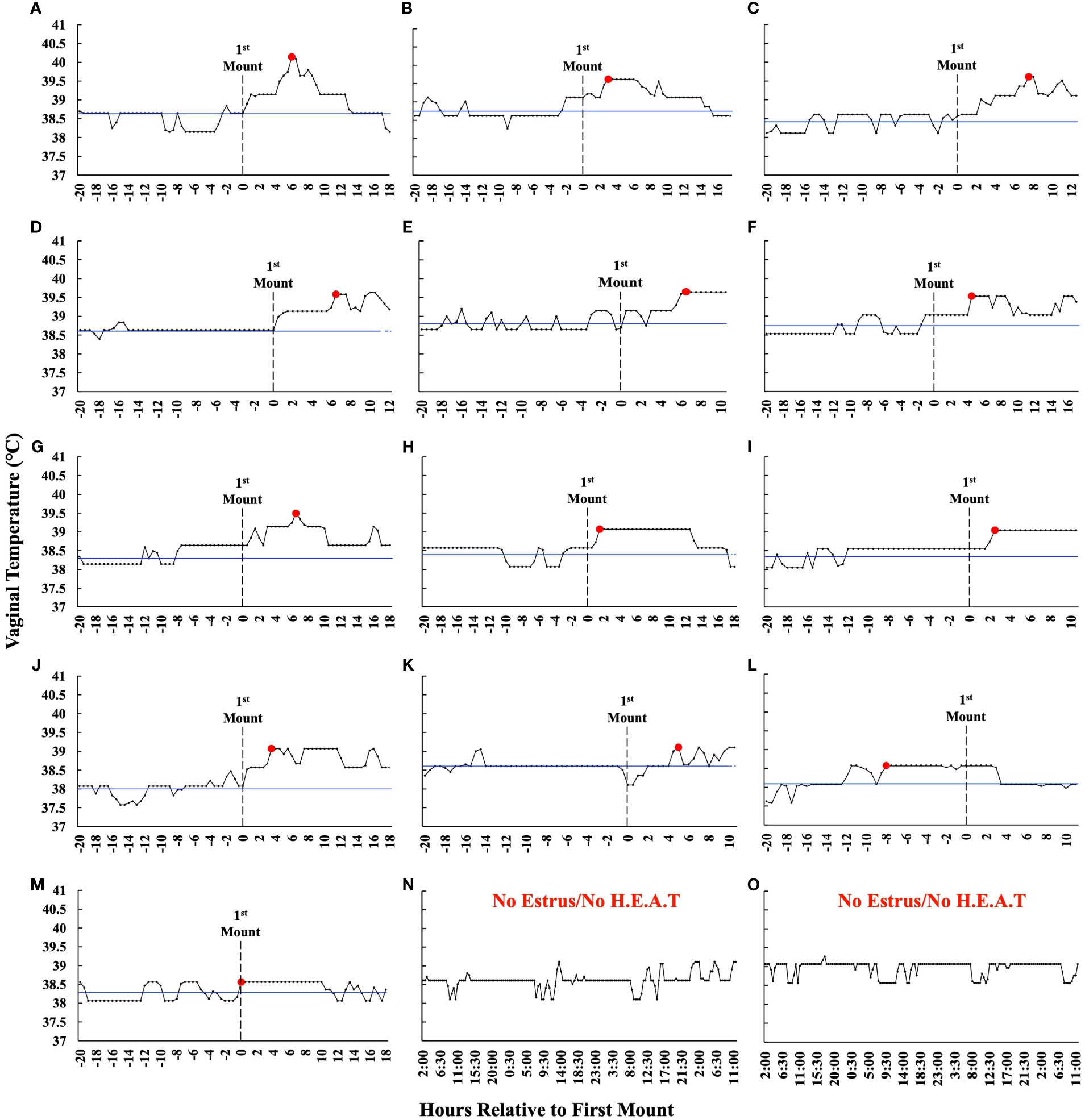
Figure 4 (A–M) Estrus-associated vaginal temperatures for the 13 estrus cows whereby follicular fluid was collected from the preovulatory follicle are presented relative to the time that they first stood to be mounted (hour 0) in order of greatest (A) to least (M) VTMax. The blue line represents an individual animal’s baseline temperature, which was calculated by averaging the vaginal temperature of those recorded between the first temperature, which was taken 12 h after PGF2α, and those over the next 20 h. The red dot depicts VTMax, and the dotted line represents the first time an animal stood to be mounted. The graphs have been truncated for clarity and depict only the first 20 h before estrus and through preovulatory follicle aspiration. (N, O) Representative vaginal temperature graphs of the cows that did not exhibit estrus and did not exhibit a higher estrus-associated vaginal temperature. These animals were not included in the analysis and are displayed for reference only. Data are not shown for the one cow where follicular fluid was not successfully collected.
3.2 Preovulatory follicular fluid metabolites
Eighty-six metabolites were identified in the preovulatory follicular fluid aspirates collected 14.9 h ± 3.3 h after the onset of estrus (Supplementary Table 1). Identified metabolites primarily included amino acids, glucose metabolism and tricarboxylic acid (TCA) cycle derivatives, and nucleosides. The abundances of 17 out of 86 metabolites (19.8%) were related to different aspects of HEAT.
3.3 Follicular fluid metabolites related to HEAT
The vaginal temperature at first standing mount, when expressed as a change from baseline, was associated with the differential abundance of five metabolites. Four of the five metabolites had a positive relationship with the vaginal temperature change from baseline to first mount (i.e., taurine, sn-glycerol 3-phosphate, glycine, and cysteine), and one metabolite, serine, had a negative relationship (Figure 5; Supplementary Table 2). The vaginal temperature at first standing mount was related to the differential abundance of two metabolites. Jasmonate was negatively related to it, and N-carbamoyl-L-aspartate was positively related to it (Figure 6; Supplementary Table 3).
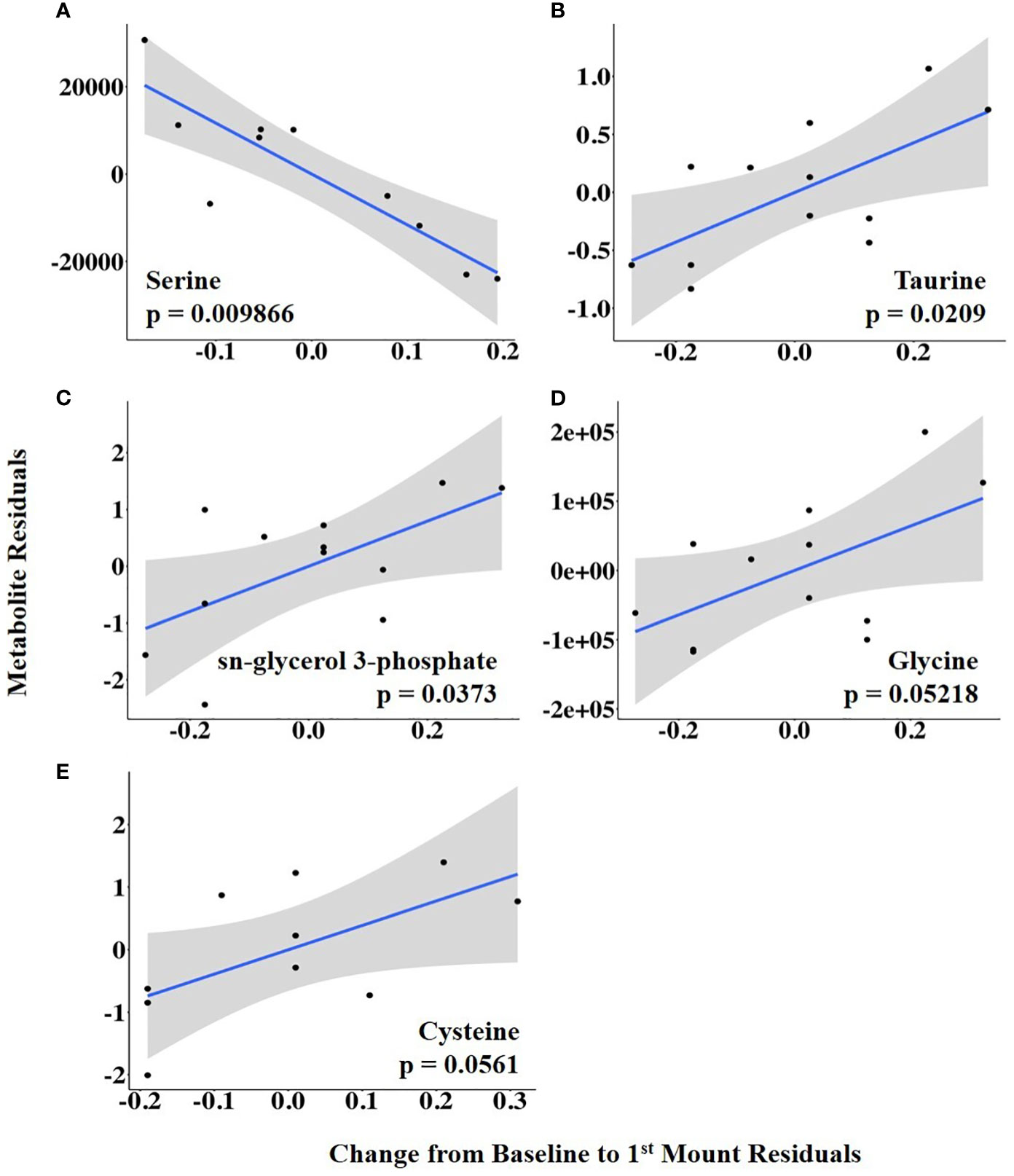
Figure 5 (A–E) Partial regression plots for the final model for each metabolite related to the change in vaginal temperature from the baseline to first mount. The plots depict the relationship between the vaginal temperature change from the baseline to first mount and metabolite abundance while adjusting for additional variables included in the final model.
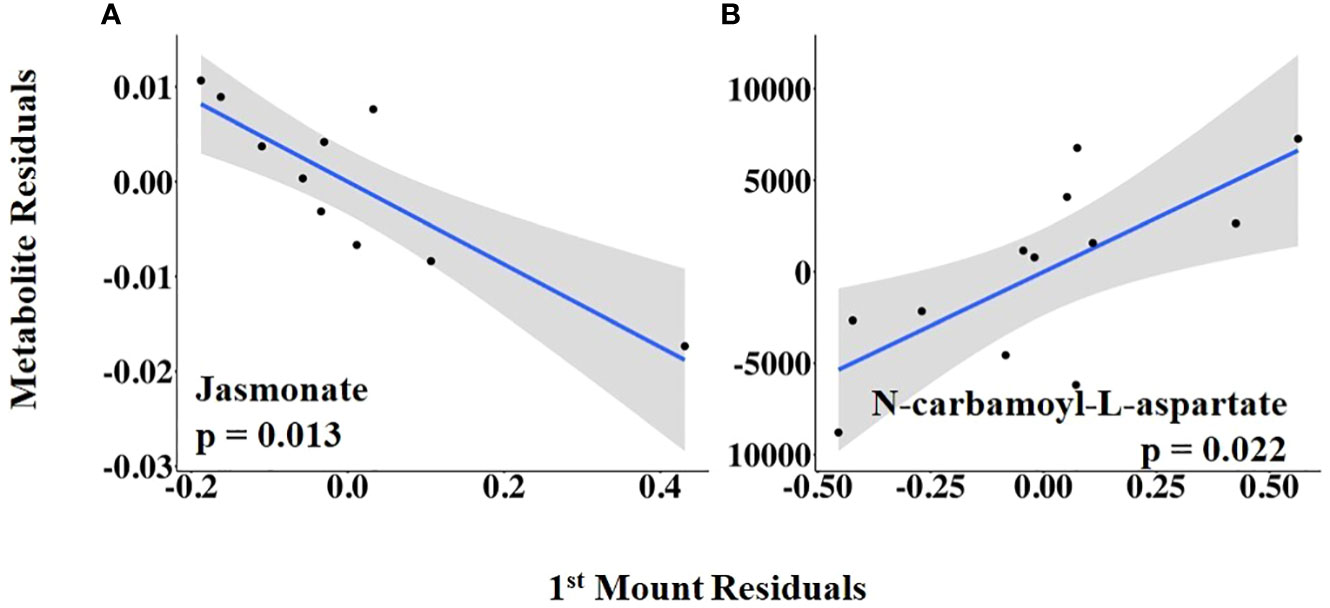
Figure 6 (A, B) Partial regression plots for the final model for each metabolite related to the vaginal temperature at first standing mount. The plots depict the relationship between vaginal temperature at first standing mount and metabolite abundance while adjusting for the additional variables included in the final model.
The abundances of three follicular fluid metabolites at 14.9 h ± 3.3 h after the onset of estrus were related to VTMax: N-carbamoyl-L-aspartate was positively related to it, whereas uracil and glycodeoxycholate were negatively related to it (Figure 7; Supplementary Table 4). When VTMax was expressed as a change from baseline, uric acid and 6-phospho-D-gluconate were positively related to it, whereas uracil was negatively related to it (Figure 8; Supplementary Table 5).
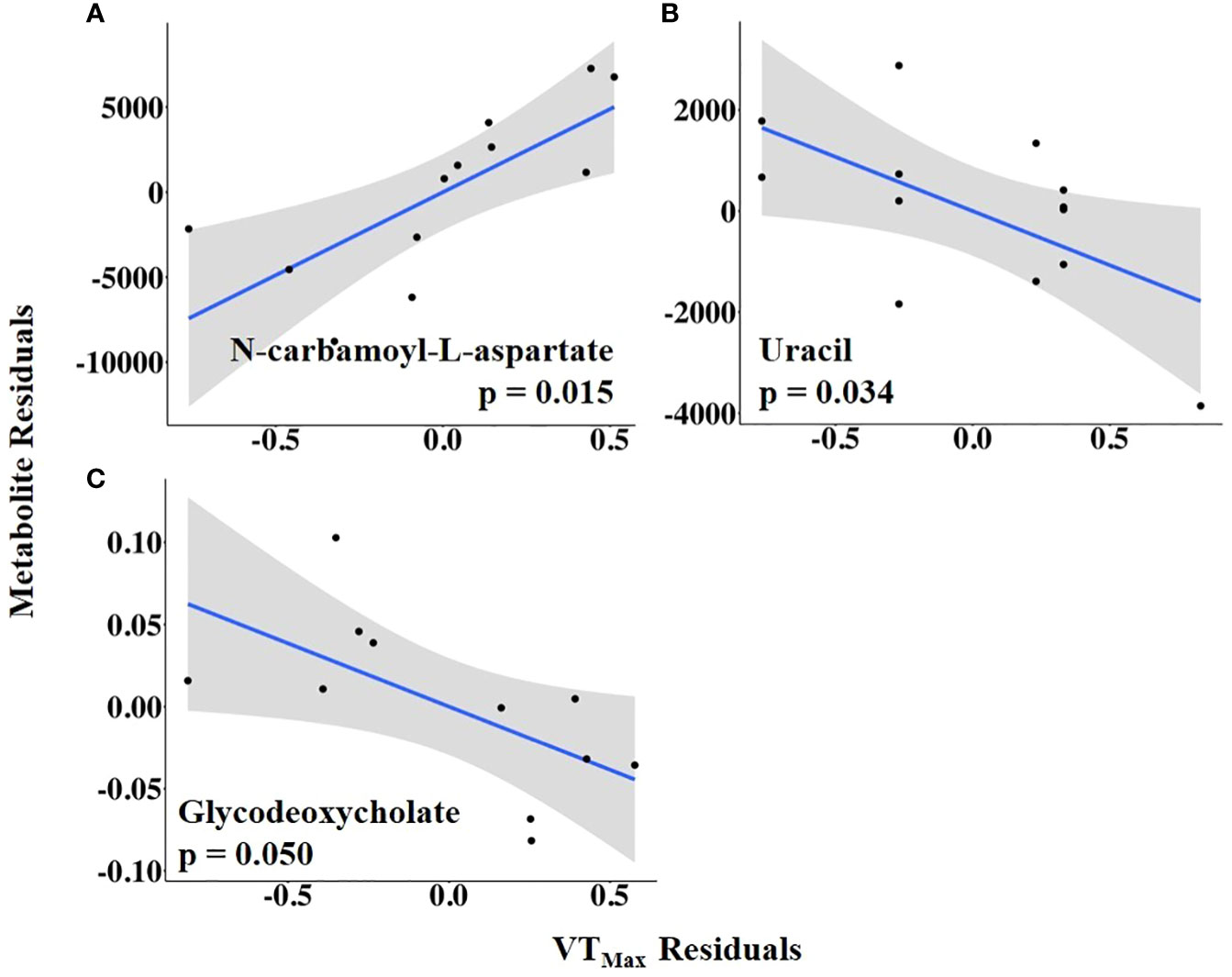
Figure 7 (A–C) Partial regression plots for the final model for each metabolite related to maximum vaginal temperature (VTMax). The plots depict the relationship between VTMax and metabolite abundance while adjusting for additional variables included in the final model.
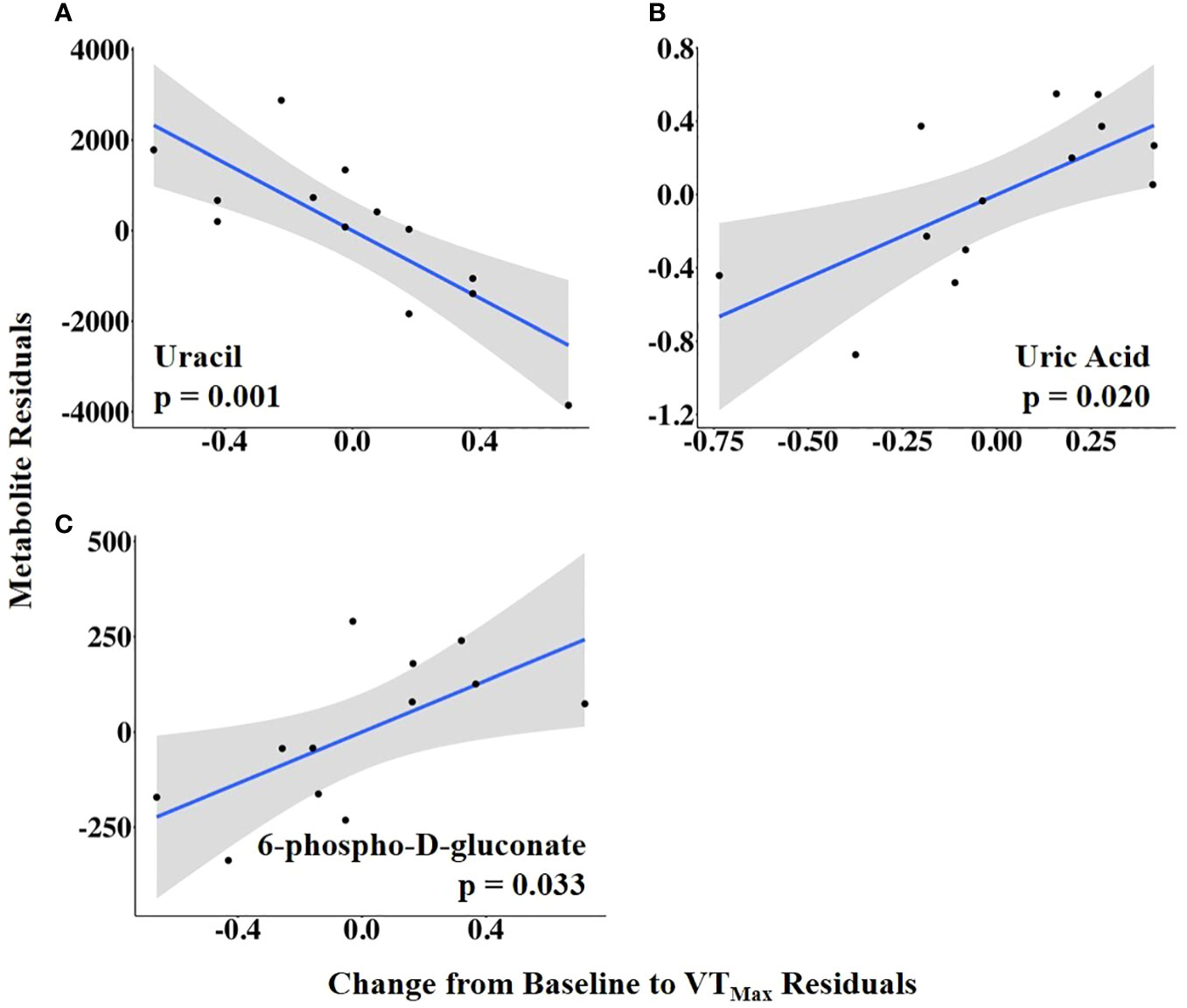
Figure 8 (A–C) Partial regression plots for the final model for each metabolite related to the vaginal temperature change from the baseline to the maximum vaginal temperature (VTMax). The plots depict the relationship between vaginal temperature change from the baseline to VTMax and metabolite abundance while adjusting for additional variables included in the final model.
To examine the relationship between HEAT duration and the preovulatory follicular fluid metabolome, time to VTMax was used as an independent variable. Time to VTMax was positively associated with the abundance of N-carbamoyl-L-aspartate but negatively related to the abundances of glycodeoxycholate, jasmonate, and tricarballylic acid (Figure 9; Supplementary Table 6).
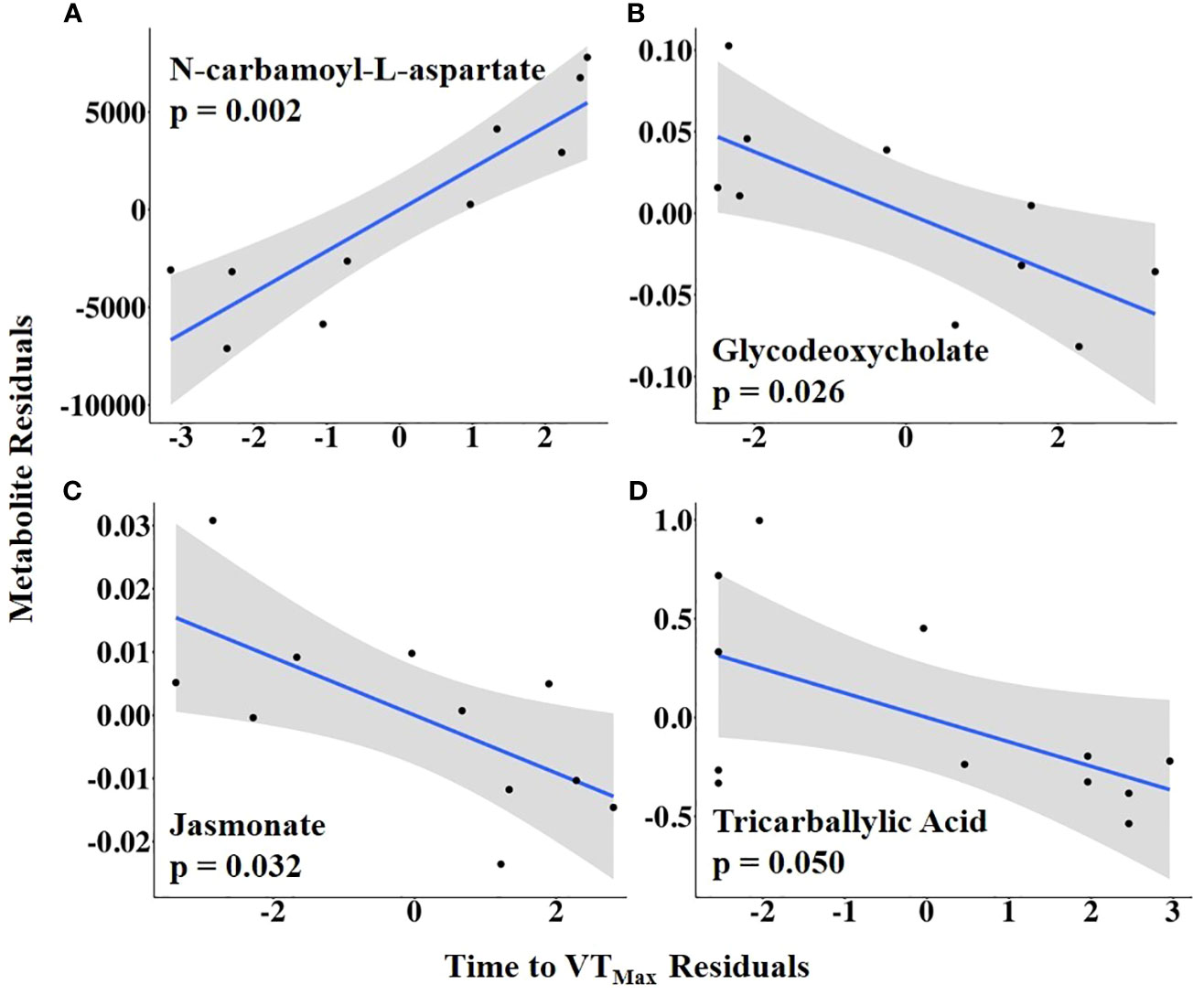
Figure 9 (A–D) Partial regression plots for the final model for each metabolite related to time from first temperature increase to maximum vaginal temperature (VTMax). The plots depict the relationship between the time from the first temperature increase to VTMax and metabolite abundance while adjusting for additional variables included in the final model.
Combining HEAT level with duration, AUC1 and AUC2 were tested as independent variables of interest. Sulfolactate, 6-phospho-D-gluconate, and aspartate levels were positively related to AUC1, whereas guanidoacetic acid abundance was negatively related to AUC1 (Figure 10; Supplementary Table 7). In contrast, AUC2 was positively associated with the abundance of methionine sulfoxide, taurodeoxycholate, and sn-glycerol 3-phosphate but negatively related to uracil abundance (Figure 11; Supplementary Table 8).
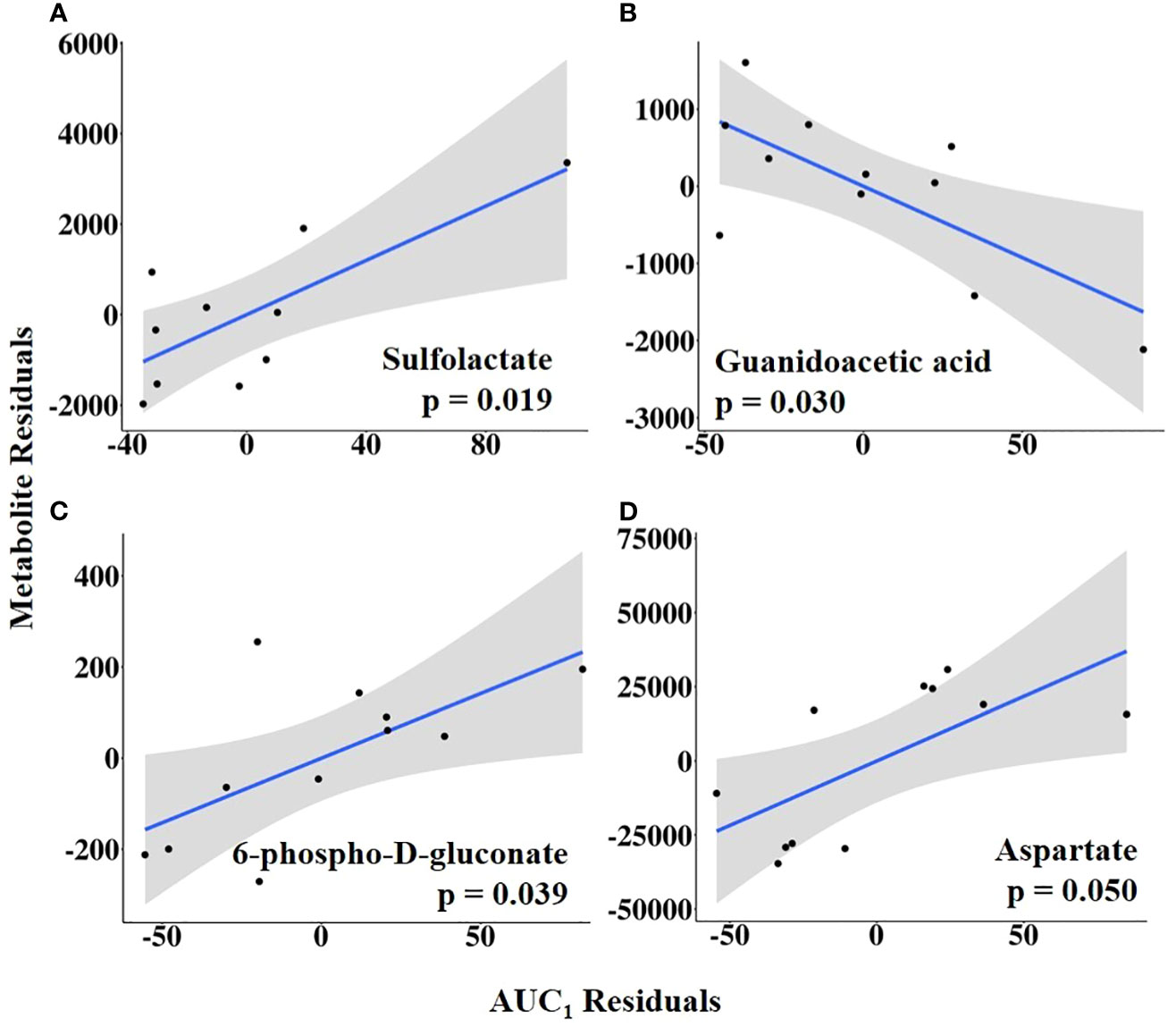
Figure 10 (A–D) Partial regression plots for the final model for each metabolite related to the area under the curve from the first increase to the maximum vaginal temperature (AUC1). The plots depict the relationship between AUC1 and metabolite abundance while adjusting for additional variables included in the final model.
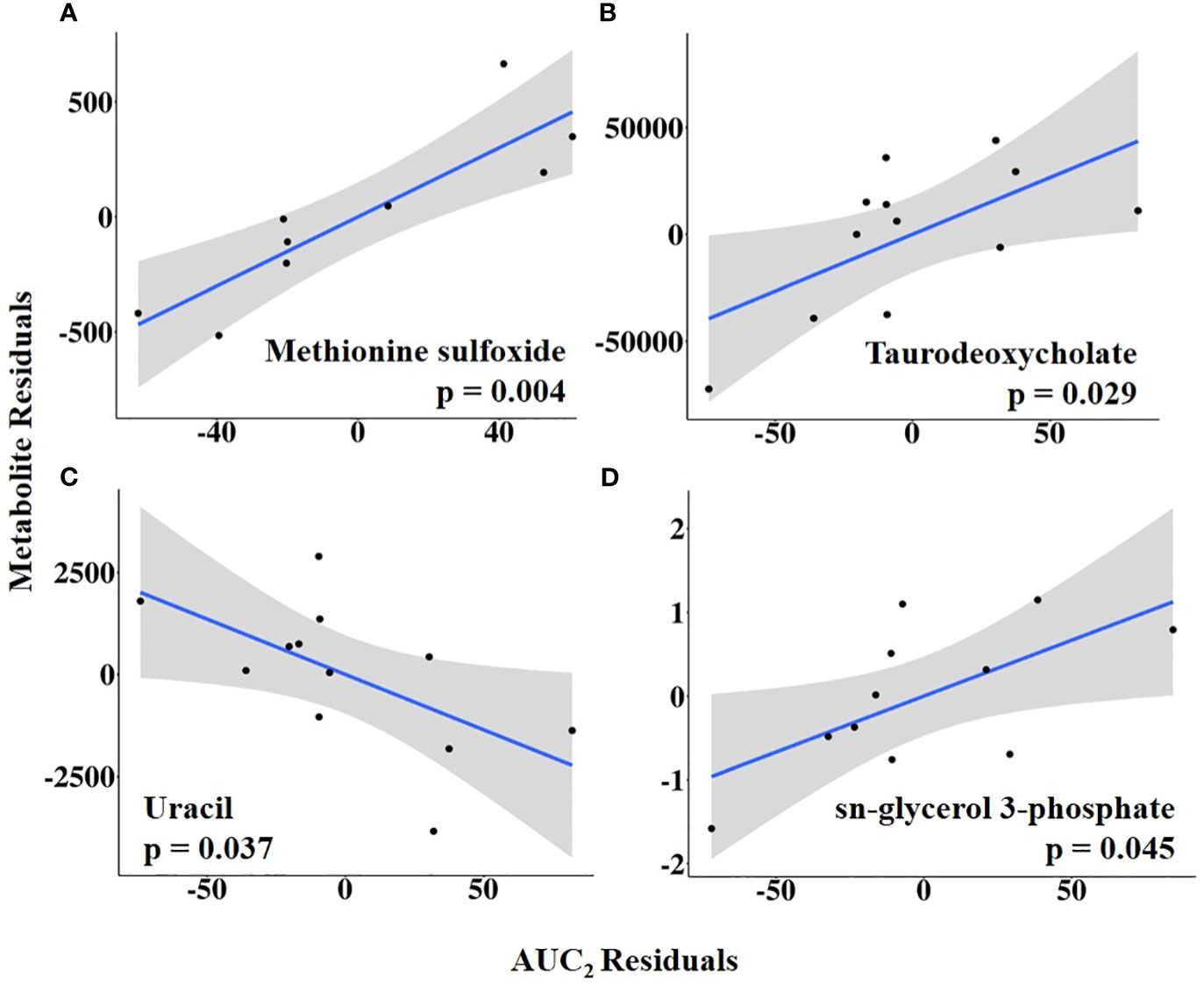
Figure 11 (A–D) Partial regression plots for the final model for each metabolite related to area under the curve from the first temperature increase to 10 h post-estrus onset, or the completion of the temperature curve (AUC2). The plots depict the relationship between AUC2 and metabolite abundance while adjusting for additional variables included in the final model.
3.4 Metabolites relating to multiple HEAT variables
Of the 17 metabolites (35.3%) significantly related to different aspects of HEAT, 6 were related to two or more HEAT variables (Figure 12). Uracil and N-carbamoyl-L-aspartate were related to three HEAT variables (uracil: VTMax, vaginal temperature change from baseline to VTMax, and AUC2; N-carbamoyl-L-aspartate: first mount, VTMax, and time to VTMax; Figure 12). The abundances of jasmonate, glycodeoxycholate, 6-phospho-D-gluconate, and sn-glycerol 3-phosphate were related to two HEAT variables (Figure 12). Jasmonate was related to both vaginal temperature at first standing mount and time to VTMax. Glycodeoxycholate was related to both VTMax and time to VTMax. Neither AUC1 nor AUC2 were related to any common differentially abundant metabolites; however, 6-phospho-D-gluconate was differentially abundant in analyses of AUC1 and temperature change from baseline to VTMax, while sn-glycerol 3-phosphate abundance was related to AUC2 and temperature change from baseline to first standing mount (Figure 12).
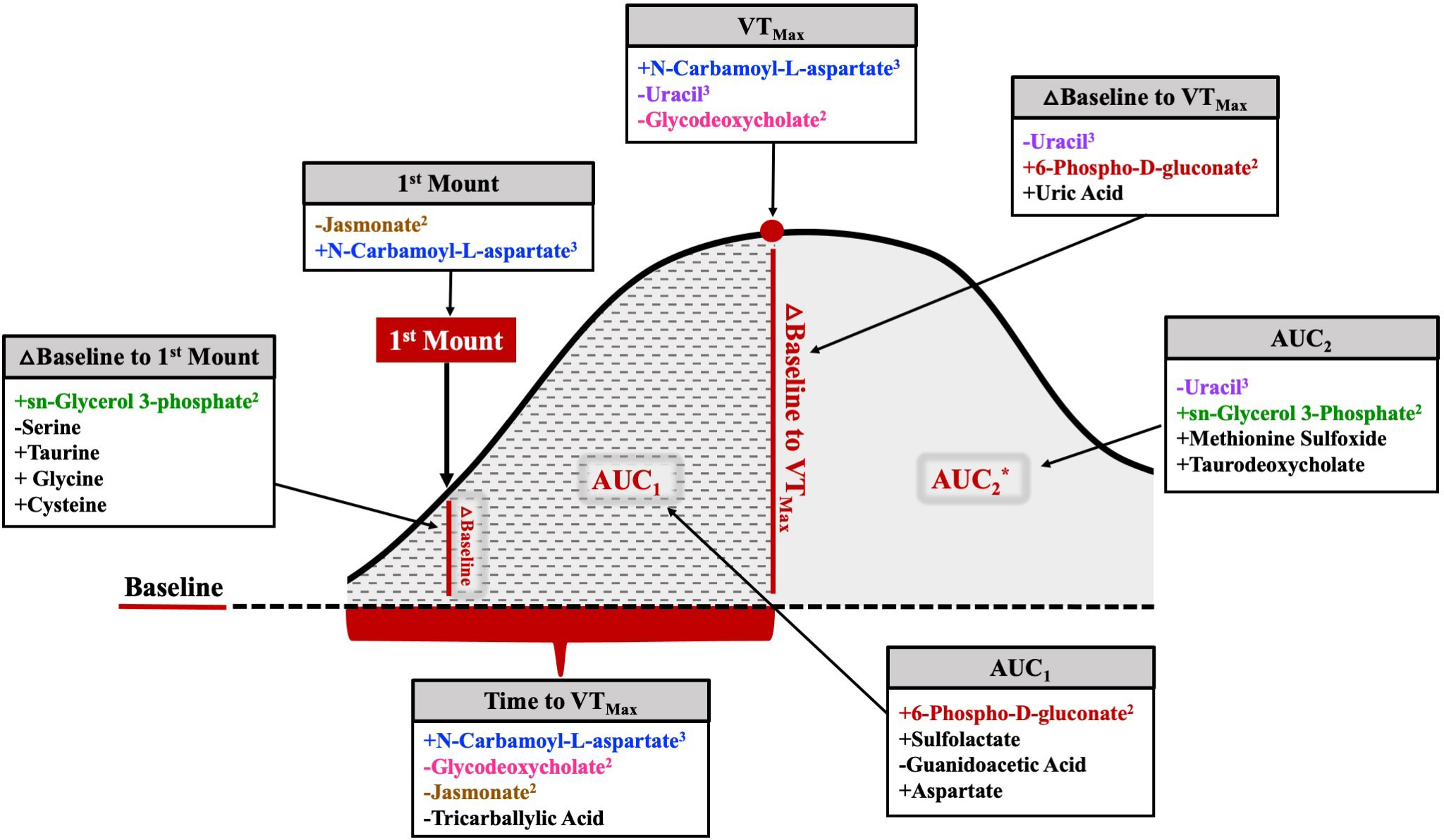
Figure 12 Comprehensive figure depicting metabolites related to each HEAT variable of interest. The metabolites listed in color were related to at least one other temperature variable. The metabolites listed in black were solely related to a specific temperature variable. Positive or negative relationships between metabolite abundance and each variable are depicted by the symbol preceding the metabolite name. Superscript following metabolite name denotes the number of HEAT variables to which the metabolite was significantly related. △=change; *AUC2 includes AUC1 area and remaining solid gray shaded area.
4 Discussion
The novel findings described in this study highlight the relationship of varying levels of HEAT with the abundance of multiple metabolites in the preovulatory follicle fluid (19.8% of 86 total) ~ 14 h after the onset of estrus. Interestingly, the majority of affected metabolites were positively related to HEAT (11/17; 65%). This finding is especially interesting because intra-follicular changes in the preovulatory follicle in the final hours leading up to the LH surge are important for promoting oocyte competence for embryo development (Atkins et al., 2013; Jinks et al., 2013) and impacting cumulus–oocyte complex metabolism (Read et al., 2021; Moorey et al., 2022; Read et al., 2022) in addition to affecting subsequent luteal function (Perry et al., 2005) and pregnancy outcome (Lamb et al., 2001; Perry et al., 2005; Atkins et al., 2013).
Regardless of species, sex, or reproductive status, heightened levels of activity for as few as 45 min to 1 hour increase body temperature (Murray and Yeates, 1967; Vajrabukka and Thwaites, 1984; Gleeson, 1998). Estrus-induced or not, resultant increases in body temperature are likely a by-product of increased metabolic activity, increased blood flow, and or muscle strain due to elevated activity (Gleeson, 1998). Higher estrus-associated temperatures in our study fell within a range reported by others (Lewis and Newman, 1984; Redden et al., 1993; Kyle et al., 1998; Fisher et al., 2008), with one cow exceeding 40°C. Although the THI during the final week of our study approached the upper limit of thermoneutral conditions (77 THI for non-lactating Holsteins; Ouellet et al., 2021), the varying levels of HEAT likely reflect those attributable to changes in estrus activity. Increases in vaginal temperature were most notable after a cow first stood to be mounted, which is not surprising when using non-lactating Jersey cows, as they are more thermotolerant (Seath and Miller, 1947; Harris et al., 1960; Muller and Botha, 1993; Lim et al., 2021). In addition, the calculated baseline body temperature for each individual cow fell within the range of normal body temperatures for cattle not experiencing heat stress (Gaalaas, 1945). It is also important to note that estrus onset occurred in the majority of cows when the ambient conditions were thermoneutral.
Although instances of chronic elevations in body temperature because of disease or heat stress are detrimental to reproductive success, short-term acute increases in temperature may in fact be beneficial. At the cellular level, Hoshino (2018) demonstrated that increased oocyte intracellular temperature during maturation may be an indicator of oocyte quality and developmental competence. Fallon (1962) reported rectal temperatures ranging from 98.8°F to 105°F in dairy cows undergoing artificial insemination (AI) the morning (a.m.) after estrus was first observed the preceding afternoon (p.m.). In this otherwise synchronized set of females, cows with rectal temperatures ranging from 101.7°F to 105.0°F had higher fertility (73.5%) than those with lower rectal temperatures (98.8°F to 101.6°F). Fallon speculated that cows with a lower rectal temperature may have been out of estrus or were too close to ovulation when AI was performed. However, because the pregnancy outcomes (60.2%) in the cows with a lower rectal temperature were similar to those in other reported cow groupings observed in estrus and bred at different times, it is also plausible that HEAT was functionally relevant and impactful. The positive benefits of elevated body temperature are not limited to dairy cattle. Recently, our laboratory reported that a higher rectal temperature at AI in beef cattle subjected to a fixed-time AI protocol was related to a higher likelihood of pregnancy (Liles et al., 2022).
Nonetheless, for HEAT-related changes to be related to estrus activity, Laitano et al. (2010) reported that whole-body heat stress-induced oxidative stress in humans. When hyperthermia was combined with moderate-intensity exercise, however, a parallel increase in antioxidant defense compensated for hyperthermia-induced oxidative stress. Laitano et al. (2010) further speculated that elevated body temperature in combination with exercise likely enhances antioxidant defense by avoiding redox imbalance, thereby preventing cellular damage. Antioxidants are essential components within mammals, as they maintain oxidative homeostasis within the body (Mulla et al., 2018). Glutathione, an important antioxidant, is a major component of animal cells (Wu et al., 2004) and has properties that have been deemed essential to successful reproduction through maintaining reactive oxygen species levels (ROS; De Matos et al., 1997; Edwards et al., 2001; Zuelke et al., 2003).
Pertaining to our study, HEAT was positively related to different glutathione precursors (cysteine and glycine). Interestingly, cysteine alleviates the negative consequences of prolonged exposure of bovine oocytes to elevated temperature through the stimulation of glutathione production (De Matos et al., 1996; Luberda, 2005; Nabenishi et al., 2012). Glutathione levels fluctuate throughout the estrous cycle, with the highest concentrations at or around meiotic maturation (Sutovsky and Schatten, 1997; Brad et al., 2003; Zuelke et al., 2003). Not only is glutathione involved in events related to meiotic maturation and subsequent development, but its levels have also been linked to thermal responses. When a glutathione inhibitor was administered, the thermotolerance of various cell types decreased (Mitchell et al., 1983; Russo et al., 1984; Harris et al., 1991). Given that cold stress depletes glutathione levels and the inhibition of glutathione results in decreased thermotolerance, metabolite abundance changes associated with HEATs are more likely the result of a combination of activity-induced elevations in temperature.
Serine was the only metabolite that had a negative association with vaginal temperature at the onset of estrus when expressed as a change from the baseline. This metabolite is a non-essential amino acid that serves as a precursor for numerous molecules, including glutathione, taurine, and cysteine (Kalhan and Hanson, 2012). Turathum et al. (2021) suggested that oxidative stress is managed during oocyte maturation through the utilization of serine for glutathione synthesis within the developing oocyte. In mice, serine uptake within the oocyte reaches its maximum during the latter stages of metaphase I and then rapidly decreases once the oocyte reaches metaphase II (Zhang et al., 2020). Given its role in mediating ROS accumulation in the maturing oocyte and that elevated temperatures are known to promote ROS production, in retrospect, it is not surprising that HEAT may be negatively related to serine abundance in the follicular fluid of the preovulatory follicle.
The increased bioavailability of other metabolites highlights other potential HEAT-related consequences on intrafollicular metabolism important to support oocyte maturation and maintain oxidative balance. The amino acid taurine was positively associated with the vaginal temperature at estrus onset when expressed as a change from the baseline. Taurine is present in many mammalian tissues and is involved in numerous cellular functions. Specifically, it is a cytoprotective molecule with antioxidant properties that is also thought to be involved in the thermoregulatory process (Schaffer and Kim, 2018; Page et al., 2019). Toward this end, when humans engaged in intense exercise, the oral administration of taurine resulted in increased exercise endurance and temperature regulation in hotter environments (Page et al., 2019). While the impacts of taurine supplementation before the LH surge and during oocyte maturation are not clear, it is interesting to note that taurine positively impacts bovine embryo development under thermoneutral conditions (Dumoulin et al., 1992; Takahashi and Kanagawa, 1998).
The generation of heat is a natural and normal byproduct of cellular function and processes (Holtzclaw, 2001). In our study, during times of elevated body temperature, three pyrimidines (i.e., cytidine, uridine, and uracil) that have been implicated to play a role in body temperature increases (Cradock et al., 1986; Peters et al., 1987b) were detected in preovulatory follicular fluid. One of these metabolites (uracil) was HEAT-related. Notably, the administration of uracil’s precursor, uridine, has been shown to induce hyperthermia in rabbits, mice, and humans (Cradock et al., 1986; Peters et al., 1987b). Because uridine’s effect on body temperature is delayed, it has been postulated that uridine’s catabolites may be responsible for the induction of hyperthermia (Peters et al., 1987a; Peters et al., 1987b). The administration of uracil to rabbits increased their body temperature by 0.3°C (Peters et al., 1987b). The uracil levels in the follicular fluid collected ~ 14 h after estrus onset were negatively related to the vaginal temperature at first mount when expressed as change from the baseline, maximum vaginal temperature change from the baseline (VTMax), and AUC2, representing a combination of temperature increase and duration. These findings, while still unclear, are the first that we are aware of that associate pyrimidine metabolites with HEAT.
In instances of HEAT, it is not surprising for thermoregulatory and other processes to be impacted as the body strives for homeostasis during bouts of hyperthermia (reviewed by Godyń et al., 2019). The change in vaginal temperature at VTMax was also positively related to follicular fluid uric acid levels. Although the significance of these findings in estrus-active females remains unclear, it is interesting to note that increases in exercise intensity in both horses and humans increase plasma uric acid levels (Green and Fraser, 1988; Räsänen et al., 1996). In humans, uric acid may in certain instances act as an antioxidant through its reduction of exercise-induced oxidative stress (Waring et al., 2003).
Regardless of related or contributive factors, the consequences of elevated body temperature are largely systemic in nature (Finch, 1986; Hahn, 1999). Whether HEAT-related differences in metabolite abundance in the follicular fluid of the preovulatory follicle ~14 h after estrus onset are reflective of changes occurring in the circulation or altered function of cells comprising the preovulatory follicle (i.e., granulosa and or cumulus–oocyte complex) remains unclear. Toward having a potential impact or a putative role, granulosa cells, which are contributors to follicular fluid components, have been shown to increase metabolism and amino acid production approximately 6 h after the LH surge (Gilbert et al., 2011).
To our knowledge, this is the first study to investigate the relationship between estrus-induced changes in body temperature and preovulatory follicular fluid metabolites. The significant outcomes related to temperature changes at or around the time of estrus onset are suggestive of a functional relevance of HEAT in driving the preparatory mechanisms involved in thermoregulation, energy metabolism, and oxidative stress management, and, in turn, possibly impacting oocyte competence and other preovulatory follicle components.
Although outside the scope of this study, it is interesting to note that the best-fit models derived to assess the impact of different aspects of HEAT on follicular fluid metabolomes included, in some instances, covariates related to estradiol and follicle size (see Supplementary Tables 4–6). We are only aware of one other study examining follicular fluid metabolomics in estrual cattle (Hessock et al., 2023), but its authors did not relate their findings to hormone levels or follicle size. In non-estrual cows, metabolites in preovulatory follicular fluid have been reported (Bender et al., 2010; Read et al., 2021; Read et al., 2022). Although estradiol concentration and follicle diameter have been found to be related to a number of follicular fluid metabolites (Read et al., 2021; Read et al., 2022), these findings do not overlap with those in this study. This is not entirely unexpected due to the dynamic changes occurring in the follicle around estrus and the inherent impact of those changes on systemic hormones.
Data availability statement
The original contributions presented in the study are included in the article/Supplementary Material. Further inquiries can be directed to the corresponding authors.
Ethics statement
The animal study was approved by Institutional Animal Care and Use Committee at the University of Tennessee, Knoxville. The study was conducted in accordance with the local legislation and institutional requirements.
Author contributions
Conception and design of study: JE, SM, and FS. Investigation/methodology: AP, EH, JE, SM, JK, RP, FS, and SC. Data and statistical analyses: AP, SM, JE, and RP. Writing—original draft, review, and editing: AP, JE, and SM. Review and editing: RP, EH, JK, FS, and SC. All authors contributed to the article and approved the submitted version.
Funding
This project was supported by Agriculture and Food Research Initiative (competitive grant no. 2022-67015-36374) (project director: JE) from the USDA National Institute of Food and Agriculture, USDA Multistate project NE2227, and The University of Tennessee AgResearch, and the Department of Animal Science.
Acknowledgments
We would like to thank The University of Tennessee Middle Tennessee AgResearch and Education Center (MTREC) for providing the animals and for its assistance with conducting the study (Kevin Thompson, Wes Gilliam, David Johnson, Nolan Rinks, and other MTREC staff). We are also thankful for the research support provided by Zoetis (Drs. Fernando DiCroce and Richard Wallace). In addition, we greatly appreciate Courtney Christopher, Hector Castro, and Sara Howard for processing the UHPLC-HRMS metabolomics data. Appreciation is also extended to Megan Mills, Jake Watts, Claire Hunkler, Samantha Roberts, Casey Read, Pablo Fioravanti, and Ian Batey for their assistance with sample collection or data analysis. We would also like to thank Michael O’Neil, Julia Zhu, and Josh Price at The University of Tennessee, Knoxville Office of Integrated Technologies-Research Computing Support, for their advice on statistical analysis and figure preparation.
Conflict of interest
The authors declare that the research was conducted in the absence of any commercial or financial relationships that could be construed as a potential conflict of interest.
Publisher’s note
All claims expressed in this article are solely those of the authors and do not necessarily represent those of their affiliated organizations, or those of the publisher, the editors and the reviewers. Any product that may be evaluated in this article, or claim that may be made by its manufacturer, is not guaranteed or endorsed by the publisher.
Supplementary material
The Supplementary Material for this article can be found online at: https://www.frontiersin.org/articles/10.3389/fanim.2023.1241033/full#supplementary-material
References
Abbott C. R., Saxton A. M., Rispoli L. A., Payton R. R., Pohler K. G., Schrick F. N., et al. (2018). An in vivo model to assess the thermoregulatory response of lactating Holsteins to an acute heat stress event occurring after a pharmacologically-induced LH surge. J. Therm. Biol. 78, 247–256. doi: 10.1016/j.jtherbio.2018.10.003
Atkins J. A., Smith M. F., Macneil M. D., Jinks E. M., Abreu F. M., Alexander L. J., et al. (2013). Pregnancy establishment and maintenance in cattle. J. Anim. Sci. 91, 722–733. doi: 10.2527/jas.2012-5368
Bedard K., Szabo E., Michalak M., Opas M. (2005). Cellular functions of endoplasmic reticulum chaperones calreticulin, calnexin, and ERp57. Int. Rev. Cytol. 245, 91–121. doi: 10.1016/s0074-7696(05)45004-4
Bender K., Walsh S., Evans A. C., Fair T., Brennan L. (2010). Metabolite concentrations in follicular fluid may explain differences in fertility between heifers and lactating cows. Reproduction 139 (6), 1047–1055. doi: 10.1530/REP-10-0068
Brad A., Bormann C., Swain J., Durkin R., Johnson A., Clifford A., et al. (2003). Glutathione and adenosine triphosphate content of in vivo and in vitro matured porcine oocytes. Mol. Reprod. Dev. 64, 492–498. doi: 10.1002/mrd.10254
Burdick N., Carroll J., Dailey J., Randel R., Falkenberg S., Schmidt T. (2012). Development of a self-contained, indwelling vaginal temperature probe for use in cattle research. J. Therm. Biol. 37, 339–343. doi: 10.1016/j.jtherbio.2011.10.007
Clapper J., Ottobre J., Ottobre A., Zartman D. (1990). Estrual rise in body temperature in the bovine I. Temporal relationships with serum patterns of reproductive hormones. Anim. Reprod. Sci. 23, 89–98. doi: 10.1016/0378-4320(90)90051-G
Cradock J., Vishnuvajjala B., Chin T., Hochstein H., Ackerman S. (1986). Uridine-induced hyperthermia in the rabbit. J. Pharm. Pharmacol. 38, 226–229. doi: 10.1111/j.2042-7158.1986.tb04552.x
De Matos D., Furnus C., Moses D., Martinez A., Matkovic M. (1996). Stimulation of glutathione synthesis of in vitro matured bovine oocytes and its effect on embryo development and freezability. Mol. Reprod. Dev. 45, 451–457. doi: 10.1002/(SICI)1098-2795(199612)45:4<451::AID-MRD7>3.0.CO;2-Q
De Matos D. G., Furnus C. C., Moses D. F. (1997). Glutathione synthesis during in vitro maturation of bovine oocytes: role of cumulus cells. Biol. Reprod. 57, 1420–1425. doi: 10.1095/biolreprod57.6.1420
Diskin M. G., Sreenan J. M. (2000). Expression and detection of oestrus in cattle. Reprod. Nutr. Dev. 40, 481–491. doi: 10.1051/rnd:2000112
Duggavathi R., Murphy B. D. (2009). Ovulation signals. Science 324, 890–891. doi: 10.1126/science.1174130
Dumoulin J., Evers J., Bras M., Pieters M., Geraedts J. (1992). Positive effect of taurine on preimplantation development of mouse embryos. vitro. Reprod. 94, 373–380. doi: 10.1530/jrf.0.0940373
Edwards J., King W., Kawarsky S., Ealy A. (2001). Responsiveness of early embryos to environmental insults: potential protective roles of HSP70 and glutathione. Theriogenology 55, 209–223. doi: 10.1016/s0093-691x(00)00455-6
Edwards J., Saxton A., Lawrence J., Payton R., Dunlap J. (2005). Exposure to a physiologically relevant elevated temperature hastens in vitro maturation in bovine oocytes. J. Dairy Sci. 88, 4326–4333. doi: 10.3168/jds.s0022-0302(05)73119-2
Fallon G. (1962). Body temperature and fertilization in the cow. J. Reprod. Fertil. 3, 116–123. doi: 10.1530/jrf.0.0030116
Finch V. A. (1986). Body temperature in beef cattle: Its control and relevance to production in the tropics. J. Anim. Sci. 62, 531–542. doi: 10.2527/jas1986.622531x
Fisher A., Morton R., Dempsey J., Henshall J., Hill J. (2008). Evaluation of a new approach for the estimation of the time of the LH surge in dairy cows using vaginal temperature and electrodeless conductivity measurements. Theriogenology 70, 1065–1074. doi: 10.1016/j.theriogenology.2008.06.023
Gaalaas R. (1945). Effect of atmospheric temperature on body temperature and respiration rate of Jersey cattle. J. Dairy Sci. 28, 555–563. doi: 10.3168/jds.S0022-0302(45)95208-8
Gilbert I., Robert C., Dieleman S., Blondin P., Sirard M. (2011). Transcriptional effect of the LH surge in bovine granulosa cells during the peri-ovulation period. Reproduction 141, 193–205. doi: 10.1530/rep-10-0381
Ginther O. J., Pinaffi F. L. V., Khan F. A., Duarte L. F., Beg M. A. (2013). Follicular-phase concentrations of progesterone, estradiol-17β, LH, FSH, and a PGF2α metabolite and daily clustering of prolactin pulses, based on hourly blood sampling and hourly detection of ovulation in heifers. Theriogenology 79, 918–928. doi: 10.1016/j.theriogenology.2012.12.015
Gleeson M. (1998). Temperature regulation during exercise. Int. J. Sports Med. 19, S96–S99. doi: 10.1055/s-2007-971967
Godyń D., Herbut P., Angrecka S. (2019). Measurements of peripheral and deep body temperature in cattle – A review. J. Therm. Biol. 79, 42–49. doi: 10.1016/j.jtherbio.2018.11.011
Green H. J., Fraser I. (1988). Differential effects of exercise intensity on serum uric acid concentration. Med. Sci. Sports Exerc. 20, 55–59. doi: 10.1249/00005768-198802000-00008
Greene E., Cauble R., Dhamad A. E., Kidd M. T., Kong B., Howard S. M., et al. (2020). Muscle metabolome profiles in woody breast-(un) affected broilers: effects of quantum blue phytase-enriched diet. Front. Vet. Sci. 7. doi: 10.3389/fvets.2020.00458
Hahn G. L. (1999). Dynamic responses of cattle to thermal heat loads. J. Anim. Sci. 77, 10–20. doi: 10.2527/1997.77suppl_210x
Harris C., Juchau M. R., Mirkes P. E. (1991). Role of glutathione and Hsp 70 in the acquisition of thermotolerance in postimplantation rat embryos. Teratology 43, 229–239. doi: 10.1002/tera.1420430307
Harris D., Shrode R., Rupel I., Leighton R. (1960). A study of solar radiation as related to physiological and production responses of lactating Holstein and Jersey cows. J. Dairy Sci. 43, 1255–1262. doi: 10.3168/jds.S0022-0302(60)90312-X
Hellberg P., Larson L., Olofsson J., Hedin L., Brännström M. (1991). Stimulatory effects of bradykinin on the ovulatory process in the in vitro-perfused rat ovary. Biol. Reprod. 44, 269–274. doi: 10.1095/biolreprod44.2.269
Hessock E. A., Edwards J. L., Schrick F. N., Payton R. R., Campagna S. R., Pollock A. B., et al. (2023). Metabolite abundance in bovine preovulatory follicular fluid is influenced by follicle developmental progression post estrous onset in cattle. Front. Cell Dev. Biol. 11. doi: 10.3389/fcell.2023.1156060
Holtzclaw B. J. (2001). Circadian rhythmicity and homeostatic stability in thermoregulation. Biol. Res. Nurs. 2, 221–235. doi: 10.1177/109980040100200402
Hooper L. M., Payton R. R., Rispoli L. A., Saxton A. M., Edwards J. L. (2015). Impact of heat stress on germinal vesicle breakdown and lipolytic changes during in vitro maturation of bovine oocytes. J. Repod. Dev. 61, 459–464. doi: 10.1262/jrd.2014-168
Horn E. J., Read C. C., Edwards J. L., Schrick F. N., Rhinehart J. D., Payton R. R., et al. (2022). Preovulatory follicular fluid and serum metabolome profiles in lactating beef cows with thin, moderate, and obese body condition. J. Anim. Sci. 100, skac152. doi: 10.1093/jas/skac152
Hoshino Y. (2018). Updating the markers for oocyte quality evaluation: intracellular temperature as a new index. Reprod. Med. Biol. 17, 434–441. doi: 10.1002/rmb2.12245
Jinks E. M., Smith M. F., Atkins J. A., Pohler K. G., Perry G. A., Macneil M. D., et al. (2013). Preovulatory estradiol and the establishment and maintenance of pregnancy in suckled beef cows. J. Anim. Sci. 91, 1176–1185. doi: 10.2527/jas.2012-5611
Kalhan S. C., Hanson R. W. (2012). Resurgence of serine: an often neglected but indispensable amino Acid. J. Biol. Chem. 287, 19786–19791. doi: 10.1074/jbc.R112.357194
Kerbrat S., Disenhaus C. (2004). A proposition for an updated behavioural characterisation of the oestrus period in dairy cows. Appl. Anim. Behav. Sci. 87, 223–238. doi: 10.1016/j.applanim.2003.12.001
Kiddy C. A. (1977). Variation in physical activity as an indication of estrus in dairy cows. J. Dairy Sci. 60, 235–243. doi: 10.3168/jds.s0022-0302(77)83859-9
Kirby C. J., Smith M. F., Keisler D. H., Lucy M. C. (1997). Follicular function in lactating dairy cows treated with sustained-release bovine somatotropin. J. Dairy Sci. 80, 273–285. doi: 10.3168/jds.s0022-0302(97)75935-6
Klabnik J. L., Christenson L. K., Gunewardena S. S., Pohler K. G., Rispoli L. A., Payton R. R., et al. (2022). Heat-induced increases in body temperature in lactating dairy cows: impact on the cumulus and granulosa cell transcriptome of the periovulatory follicle. J. Anim. Sci. 100, skac121. doi: 10.1093/jas/skac121
Kyle B. L., Kennedy A. D., Small J. A. (1998). Measurement of vaginal temperature by radiotelemetry for the prediction of estrus in beef cows. Theriogenology 49, 1437–1449. doi: 10.1016/S0093-691X(98)00090-9
Laitano O., Kalsi K. K., Pook M., Oliveira A. R., González-Alonso J. (2010). Separate and combined effects of heat stress and exercise on circulatory markers of oxidative stress in euhydrated humans. Eur. J. Appl. Physiol. 110, 953–960. doi: 10.1007/s00421-010-1577-5
Lamb G. C., Stevenson J. S., Kesler D. J., Garverick H. A., Brown D. R., Salfen B. E. (2001). Inclusion of an intravaginal progesterone insert plus GnRH and prostaglandin F2α for ovulation control in postpartum suckled beef cows. J. Anim. Sci. 79, 2253–2259. doi: 10.2527/2001.7992253x
Leroy J., Rizos D., Sturmey R., Bossaert P., Gutierrez-Adan A., Van Hoeck V., et al. (2011). Intrafollicular conditions as a major link between maternal metabolism and oocyte quality: a focus on dairy cow fertility. Reprod. Fertil. Dev. 24, 1–12. doi: 10.1071/rd11901
Lewis G. S., Newman S. K. (1984). Changes throughout estrous cycles of variables that might indicate estrus in dairy cows. J. Dairy Sci. 67, 146–152. doi: 10.3168/jds.S0022-0302(84)81278-3
Liao Y., Hu R., Wang Z., Peng Q., Dong X., Zhang X., et al. (2018). Metabolomics profiling of serum and urine in three beef cattle breeds revealed different levels of tolerance to heat stress. J. Agric. Food Chem. 66, 6926–6935. doi: 10.1021/acs.jafc.8b01794
Liles H. L., Schneider L. G., Pohler K. G., Oliveira Filho R. V., Schrick F. N., Payton R. R., et al. (2022). Positive relationship of rectal temperature at fixed timed artificial insemination on pregnancy outcomes in beef cattle. J. Anim. Sci. 100, skac100. doi: 10.1093/jas/skac100
Lim D. H., Kim T. I., Park S. M., Ki K. S., Kim Y. (2021). Evaluation of heat stress responses in Holstein and Jersey cows by analyzing physiological characteristics and milk production in Korea. J. Anim. Sci. Technol. 63, 872. doi: 10.5187/jast.2021.e62
López-Gatius F., Santolaria P., Mundet I., Yániz J. (2005). Walking activity at estrus and subsequent fertility in dairy cows. Theriogenology 63, 1419–1429. doi: 10.1016/j.theriogenology.2004.07.007
Lyimo Z., Nielen M., Ouweltjes W., Kruip T., Van Eerdenburg F. (2000). Relationship among estradiol, cortisol and intensity of estrous behavior in dairy cattle. Theriogenology 53, 1783–1795. doi: 10.1016/s0093-691x(00)00314-9
Madureira A. M. L., Polsky L. B., Burnett T. A., Silper B. F., Soriano S., Sica A. F., et al. (2019). Intensity of estrus following an estradiol-progesterone-based ovulation synchronization protocol influences fertility outcomes. J. Dairy Sci. 102, 3598–3608. doi: 10.3168/jds.2018-15129
Madureira A. M. L., Silper B. F., Burnett T. A., Polsky L., Cruppe L. H., Veira D. M., et al. (2015). Factors affecting expression of estrus measured by activity monitors and conception risk of lactating dairy cows. J. Dairy Sci. 98, 7003–7014. doi: 10.3168/jds.2015-9672
Malhi P. S., Adams G. P., Singh J. (2005). Bovine model for the study of reproductive aging in women: follicular, luteal, and endocrine characteristics. Biol. Reprod. 73, 45–53. doi: 10.1095/biolreprod.104.038745
Mitchell J. B., Russo A., Kinsella T. J., Glatstein E. (1983). Glutathione elevation during thermotolerance induction and thermosensitization by glutathione depletion. Cancer Res. 43, 987–991.
Miura R., Yoshioka K., Miyamoto T., Nogami H., Okada H., Itoh T. (2017). Estrous detection by monitoring ventral tail base surface temperature using a wearable wireless sensor in cattle. Anim. Reprod. Sci. 180, 50–57. doi: 10.1016/j.anireprosci.2017.03.002
Moorey S. E., Hessock E. A., Edwards J. L. (2022). Preovulatory follicle contributions to oocyte competence in cattle: importance of the ever-evolving intrafollicular environment leading up to the luteinizing hormone surge. J. Anim. Sci. 100, skac153. doi: 10.1093/jas/skac153
Mulla A. A., Fazari A., Elkhouly M., Moghaddam N. (2018). Role of antioxidants in female fertility. Open J. Obstet. Gynecol. 8, 85–91. doi: 10.4236/ojog.2018.82011
Muller C., Botha J. (1993). Effect of summer climatic conditions on different heat tolerance indicators in primiparous Friesian and Jersey cows. S. Afr. J. Anim. Sci. 23, 98–103.
Murray D. M., Yeates N. T. M. (1967). Walking trials with cattle II. A comparison of bulls, steers and heifers. J. Agric. Sci. 69, 71–78. doi: 10.1017/S0021859600016476
Nabenishi H., Ohta H., Nishimoto T., Morita T., Ashizawa K., Tsuzuki Y. (2012). The effects of cysteine addition during in vitro maturation on the developmental competence, ROS, GSH and apoptosis level of bovine oocytes exposed to heat stress. Zygote 20, 249–259. doi: 10.1017/S0967199411000220
Ouellet V., Toledo I. M., Dado-Senn B., Dahl G. E., Laporta J. (2021). Critical temperature-humidity index thresholds for dry cows in a subtropical climate. Front. Anim. Sci. 2. doi: 10.3389/fanim.2021.706636
Page L. K., Jeffries O., Waldron M. (2019). Acute taurine supplementation enhances thermoregulation and endurance cycling performance in the heat. Eur. J. Sport Sci. 19, 1101–1109. doi: 10.1080/17461391.2019.1578417
Perry G. A., Smith M. F., Lucy M. C., Green J. A., Parks T. E., Macneil M. D., et al. (2005). Relationship between follicle size at insemination and pregnancy success. Proc. Natl. Acad. Sci. U.S.A. 102, 5268–5273. doi: 10.1073/pnas.0501700102
Peters G. J., Van Groeningen C. J., Laurensse E., Kraal I., Leyva A., Lankelma J., et al. (1987b). Effect of pyrimidine nucleosides on body temperatures of man and rabbit in relation to pharmacokinetic data. Pharm. Res. 4, 113–119. doi: 10.1023/a:1016410817898
Peters G., Van Groeningen C., Laurensse E., Lankelma J., Leyva A., Pinedo H. (1987a). Uridine-induced hypothermia in mice and rats in relation to plasma and tissue levels of uridine and its metabolites. Cancer Chemother. Pharmacol. 20, 101–108. doi: 10.1007/BF00253962
Piccione G., Caola G., Refinetti R. (2003). Daily and estrous rhythmicity of body temperature in domestic cattle. BMC Physiol. 3, 7. doi: 10.1186/1472-6793-3-7
Pruessner J. C., Kirschbaum C., Meinlschmid G., Hellhammer D. H. (2003). Two formulas for computation of the area under the curve represent measures of total hormone concentration versus time-dependent change. Psychoneuroendocrinology 28, 916–931. doi: 10.1016/s0306-4530(02)00108-7
Randi F., Mcdonald M., Duffy P., Kelly A. K., Lonergan P. (2018). The relationship between external auditory canal temperature and onset of estrus and ovulation in beef heifers. Theriogenology 110, 175–181. doi: 10.1016/j.theriogenology.2018.01.001
Räsänen L. A., Wiitanen P. A., Lilius E. M., Hyyppä S., Pösö A. R. (1996). Accumulation of uric acid in plasma after repeated bouts of exercise in the horse. Comp. Biochem. Physiol. B Biochem. Mol. Biol. 114, 139–144. doi: 10.1016/0305-0491(96)00022-3
Read C. C., Edwards L., Schrick N., Rhinehart J. D., Payton R. R., Campagna S. R., et al. (2021). Correlation between pre-ovulatory follicle diameter and follicular fluid metabolome profiles in lactating beef cows. Metabolites 11, 623. doi: 10.3390/metabo11090623
Read C. C., Edwards J. L., Schrick F. N., Rhinehart J. D., Payton R. R., Campagna S. R., et al. (2022). Preovulatory serum estradiol concentration is positively associated with oocyte ATP and follicular fluid metabolite abundance in lactating beef cattle. J. Anim. Sci. 100, skac136. doi: 10.1093/jas/skac136
Redden K. D., Kennedy A. D., Ingalls J. R., Gilson T. L. (1993). Detection of estrus by radiotelemetric monitoring of vaginal and ear skin temperature and pedometer measurements of activity. J. Dairy Sci. 76, 713–721. doi: 10.3168/jds.S0022-0302(93)77394-
Rispoli L. A., Edwards J. L., Pohler K. G., Russell S., Somiari R. I., Payton R. R., et al. (2019). Heat-induced hyperthermia impacts the follicular fluid proteome of the periovulatory follicle in lactating dairy cows. PloS One 14, e0227095. doi: 10.1371/journal.pone.0227095
Rowinski J. R., Rispoli L. A., Payton R. R., Schneider L. G., Schrick F. N., Mclean K. J., et al. (2021). Impact of an acute heat shock during in vitro maturation on interleukin 6 and its associated receptor component transcripts in bovine cumulus-oocyte complexes. Anim. Reprod. 17, e20200221. doi: 10.1590/1984-3143-ar2020-0221
Russo A., Mitchell J., Mcpherson S. (1984). The effects of glutathione depletion on thermotolerance and heat stress protein synthesis. Br. J. Cancer 49, 753–758. doi: 10.1038/bjc.1984.118
Sakatani M., Takahashi M., Takenouchi N. (2016). The efficiency of vaginal temperature measurement for detection of estrus in Japanese Black cows. J. Repod. Dev. 62, 201–207. doi: 10.1262/jrd.2015-095
Saumande J., Humblot P. (2005). The variability in the interval between estrus and ovulation in cattle and its determinants. Anim. Reprod. Sci. 85, 171–182. doi: 10.1016/j.anireprosci.2003.09.009
Schaffer S., Kim H. W. (2018). Effects and mechanisms of taurine as a therapeutic agent. Biolmol. Ther. 26, 225. doi: 10.4062/biomolther.2017.251
Seath D., Miller G. (1947). Heat tolerance comparisons between Jersey and Holstein cows. J. Anim. Sci. 6, 24–34. doi: 10.2527/jas1947.6124
Sirard M. A. (2011). Follicle environment and quality of in vitro matured oocytes. J. Assist. Reprod. Genet. 28, 483–488. doi: 10.1007/s10815-011-9554-4
Smith M. F., Mcintush E. W., Smith G. W. (1994). Mechanisms associated with corpus luteum development. J. Anim. Sci. 72, 1857–1872. doi: 10.2527/1994.7271857x
Stumpf T. T., Wolfe M. W., Day M. L., Stotts J. A., Wolfe P. L., Kittok R. J., et al. (1991). Effect of 17β-estradiol on the preovulatory surge of LH in the bovine female. Theriogenology 36, 201–207. doi: 10.1016/0093-691X(91)90379-R
Suthar V., Burfeind O., Patel J., Dhami A., Heuwieser W. (2011). Body temperature around induced estrus in dairy cows. J. Dairy Sci. 94, 2368–2373. doi: 10.3168/jds.2010-3858
Sutovsky P., Schatten G. (1997). Depletion of glutathione during bovine oocyte maturation reversibly blocks the decondensation of the male pronucleus and pronuclear apposition during fertilization. Biol. Reprod. 56, 1503–1512. doi: 10.1095/biolreprod56.6.1503
Sveberg G., Refsdal A., Erhard H., Kommisrud E., Aldrin M., Tvete I., et al. (2013). Sexually active groups in cattle—a novel estrus sign. J. Dairy Sci. 96, 4375–4386. doi: 10.3168/jds.2012-6407
Takahashi Y., Kanagawa H. (1998). Effects of glutamine, glycine and taurine on the development of in vitro fertilized bovine zygotes in a chemically defined medium. J. Vet. Med. 60, 433–437. doi: 10.1292/jvms.60.433
Tippenhauer C., Plenio J., Heuwieser W., Borchardt S. (2023). Association of activity and subsequent fertility of dairy cows after spontaneous estrus or timed artificial insemination. J. Dairy Sci. 106, 4291–4305. doi: 10.3168/jds.2022-22057
Tokuhiro K., Satouh Y., Nozawa K., Isotani A., Fujihara Y., Hirashima Y., et al. (2015). Calreticulin is required for development of the cumulus oocyte complex and female fertility. Sci. Rep. 5, 1–10. doi: 10.1038/srep14254
Tsafriri A., Bicsak T. A., Cajander S. B., Ny T., Hsueh A. J. W. (1989). Suppression of ovulation rate by antibodies to tissue-type plasminogen activator and α2-antiplasmin. Endocrinology 124, 415–421. doi: 10.1210/endo-124-1-415
Turathum B., Gao E., Chian R. (2021). The function of cumulus cells in oocyte growth and maturation and in subsequent ovulation and fertilization. Cells 10, 2292. doi: 10.3390/cells10092292
Vajrabukka C., Thwaites C. (1984). The relative influences of exercise and coat-type on the thermoregulatory responses of cattle. Int. J. Biometeorol. 28, 9–15. doi: 10.1007/BF02193509
Waring W., Convery A., Mishra V., Shenkin A., Webb D. J., Maxwell S. (2003). Uric acid reduces exercise-induced oxidative stress in healthy adults. Clin. Sci. 105, 425–430. doi: 10.1042/CS20030149
Wu G., Lupton J. R., Turner N. D., Fang Y. Z., Yang S. (2004). Glutathione metabolism and its implications for health. J. Nutr. 134, 489–492. doi: 10.1093/jn/134.3.489
Yoshimura Y., Espey L., Hosoi Y., Adachi T., Atlas S., Ghodgaonkar R., et al. (1988). The effects of bradykinin on ovulation and prostaglandin production by the perfused rabbit ovary. Endocrinology 122, 2540–2546. doi: 10.1210/endo-122-6-2540
Yue S., Ding S., Zhou J., Yang C., Hu X., Zhao X., et al. (2020). Metabolomics approach explore diagnostic biomarkers and metabolic changes in heat-stressed dairy cows. Animals 10, 1741. doi: 10.3390/ani10101741
Zhang H., Mcclatchie T., Baltz J. M. (2020). L-Serine transport in growing and maturing mouse oocytes. J. Cell. Physiol. 235, 8585–8600. doi: 10.1002/jcp.29702
Keywords: estrus, vaginal temperature, HEAT, metabolome, follicular fluid, preovulatory follicle, bovine
Citation: Pollock AB, Moorey SE, Hessock EA, Klabnik JL, Payton RR, Schrick FN, Campagna SR and Edwards JL (2023) Relationship between higher estrus-associated temperatures and the bovine preovulatory follicular fluid metabolome. Front. Anim. Sci. 4:1241033. doi: 10.3389/fanim.2023.1241033
Received: 15 June 2023; Accepted: 19 October 2023;
Published: 08 November 2023.
Edited by:
Elda Dervishi, University of Alberta, CanadaReviewed by:
Mariana Carriquiry, Universidad de la República, UruguayMarcos Colazo, University of Alberta, Canada
Copyright © 2023 Pollock, Moorey, Hessock, Klabnik, Payton, Schrick, Campagna and Edwards. This is an open-access article distributed under the terms of the Creative Commons Attribution License (CC BY). The use, distribution or reproduction in other forums is permitted, provided the original author(s) and the copyright owner(s) are credited and that the original publication in this journal is cited, in accordance with accepted academic practice. No use, distribution or reproduction is permitted which does not comply with these terms.
*Correspondence: J. Lannett Edwards, jedwards@utk.edu; Sarah E. Moorey, smoorey5@utk.edu
†Present address: Jessica L. Klabnik, Department of Clinical Sciences, College of Veterinary Medicine, Auburn University, Auburn, AL, United States