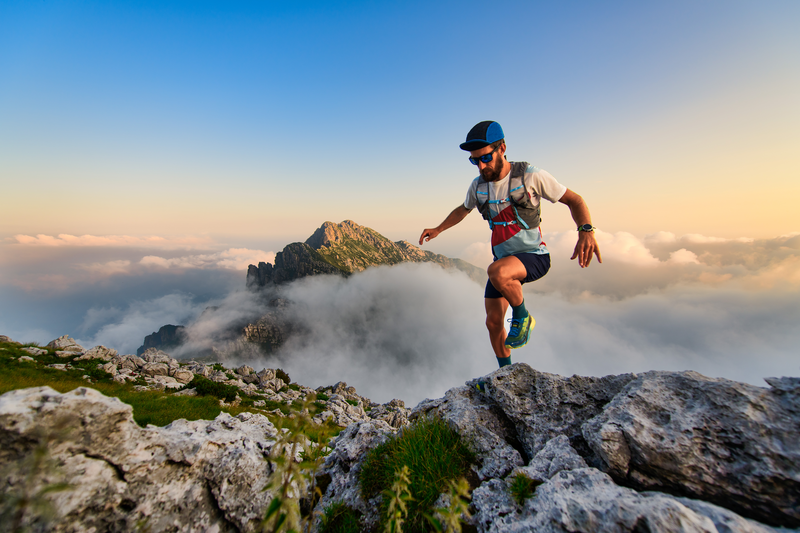
94% of researchers rate our articles as excellent or good
Learn more about the work of our research integrity team to safeguard the quality of each article we publish.
Find out more
ORIGINAL RESEARCH article
Front. Anim. Sci. , 27 July 2023
Sec. Animal Nutrition
Volume 4 - 2023 | https://doi.org/10.3389/fanim.2023.1213890
This article is part of the Research Topic Mitigating the Impact of Animal Production on the Environment: The Ecosystem Integration View all 8 articles
Macroalgae, or seaweeds, have potential for use as feed ingredients and are currently unexploited despite their content of vitamins, minerals, and protein. Brown species can accumulate iodine from seawater and there are strict limits set by the European Food Safety Authority and the FDA regarding iodine content in animal feeds. Iodine can cause health problems for consumers if over or under-consumed and its presence in end food products is strictly regulated. The aim of the present experiment was to gain knowledge on intake, distribution, and excretion of iodine in sheep supplemented with Laminaria hyperborea by-product known to contain iodine. Twelve Norwegian White Sheep male lambs, four months of age, were blocked according to initial live weight (average 37.8 kg) and randomly allocated to two diet groups. Animals were fed gras silage and concentrate, without (CTR) and including the alga by-product at a 6% inclusion rate (HYP). The iodine concentrations were 4.1 and 476 mg/kg dry matter in the CTR and HYP concentrate, respectively. After 26 days of adaptation in a barn, animals were placed in metabolism crates for three consecutive days (Period 1) with collection of rumen fluid (via esophagus), grass silage, feces, urine, and blood for iodine content. After 5 weeks in the barn, animals returned to the metabolism crates for a subsequent three consecutive day sampling and iodine analyzes (Period 2). Data were analyzed via ANOVA using a repeated measure mixed model procedure. Dry matter intake (P = 0.001) and live weight (P = 0.001) increased from Period 1 to Period 2. Lambs fed CTR had higher daily growth rate than those fed HYP (P = 0.001). Iodine intake and excretion in feces and urine increased from Period 1 to Period 2 (P < 0.001, P = 0.010, P = 0.007, respectively). Iodine excreted in feces was 37% and 67% for lambs in fed the CTR and HYP diets, respectively. None of the animals showed signs of iodine poisoning during ten the experiment. We found that most of the iodine excreted from lambs fed the HYP diet was in feces.
There is a growing interest world-wide in the use of macroalgae as livestock feed ingredients for both ruminants and monogastric animals. The composition of macroalgae varies significantly from species to species and is further impacted by the season and geographical location of collection (Roleda et al., 2018). Brown macroalgae are considered to have less nutritional value than red or green macroalgae as they contain less protein and are richer in ash and minerals (Tayyab et al., 2016). However, brown species contain bioactive compounds like phlorotannins, which are polyphenols with known benefits including anthelmintic activity (Belanche et al., 2016). Furthermore, they may impact emission of methane from ruminants (Abbott et al., 2020). All macroalgae can accumulate minerals, heavy metals, and iodine from their growth environment. Feeding macroalgae containing excess iodine or toxic heavy metals may have negative impacts on animal and human health (Makkar et al., 2016). While the toxic heavy metals in general are undesirable, iodine is an essential nutrient and is often added to animal feed (Grabez et al., 2022). The primary function of iodine is the incorporation in the thyroid hormones, triiodothyronine (T3) and tetraiodothyronine/thyroxine (T4). Dietary iodine is easily absorbed (70 to 90%) in the rumen, reticulum, and omasum (NRC, 2007), and continues to be absorbed in the small intestine before it enters the liver via the portal vein. From the liver, it enters the blood and circulates loosely bound to plasma proteins until it reaches the thyroid gland. Most of the iodine in the body is found in the thyroid gland (up to 80%), where it is utilized for the biosynthesis of T3 and T4 (Paulíková et al., 2002). Together, T3 and T4 are increasing both energy production and cellular respiration. These mechanisms will affect energy metabolism, muscle function, growth, circulation, immune defense, and fertility cycles (Huszenicza et al., 2002).
The iodine content in macroalgae is species-specific (Roleda et al., 2018). Brown species accumulate iodine to a larger extent than green and red macroalgae species, and of the brown algae, the genera Laminaria and Saccharina (family Laminariaceae) sp. are exceptional, with reported iodine content at 4,000 – 5,000 µg/g dry weight (DW) (Duinker et al., 2020). Ascophyllum nodosum, which is one of the most used and studied macroalgae species in agriculture, has lower levels (700 µg/g DW), but still high compared to red (e.g., Palmaria palmata 160-300 µg/g DW) and green species (e.g., Ulva sp. 90-130 µg/g DW) (Roleda et al., 2018; Duinker et al., 2020). The effect of iodine consumption in lactating, dairy cows fed A. nodosum were studied by Antaya et al. (Antaya et al., 2015; Antaya et al., 2019) and Silva et al. (2022). Antaya et al. (Antaya et al., 2015; Antaya et al., 2019) investigated iodine output in milk while Silva et al. (2022) included iodine output in feces, urine, and blood in their study. Increased levels of A. nodosum in the diet linearly increased the output of iodine in milk, as well as in feces, urine, and blood with the majority ending up in feces.
Several experiments using macroalgae in the diet of sheep have been performed in Norway, with the aim to study the nutritional properties of different species (Özkan-Gülzari et al., 2019; Lind et al., 2020; Lind unpublished). Lind et al. (2020) used the red species Porphyra sp. as a protein source while Özkan-Gülzari et al. (2019) compared protein digestibility of Porphyra sp. and Saccharina latissima. The intake of iodine in the experiment of Özkan-Gülzari et al. (2019) was 18 times higher than the recommended level [180 mg/kg dry matter intake (DMI)] for those animals fed the S. latissima diet. Over a period of eight weeks, none of the animals showed any signs of clinical iodine poisoning.
In Norway, there is commercial cultivation of the brown algae Saccharina latissima and Alaria esculenta, while A. nodosum and L. hyperborea are wild harvested. The largest volume of macroalgae processed in Norway is L. hyperborea for alginate production. After alginate processing, there is a by-product, which to date has no known application and thus is currently unexploited. This by-product has a higher protein concentration and a lower concentration of ash and indigestible polysaccharide (alginate) compared to the unprocessed macroalgae and thus has potential for use as a feed ingredient. However, an analytical characterization showed that the iodine concentration of the by-product was in the same range, or higher, than in the fresh biomass. As this can limit use of the by-products as a feed ingredient, a feeding experiment using growing male lambs was initiated. The aim of the experiment was to obtain knowledge on the intake, distribution, and excretion of iodine in sheep receiving the by-product as feed compared to animals fed a control diet. Our hypotheses were that a high iodine intake will not compromise animal health and that most of the iodine is excreted in the feces.
A sample of two tons of L. hyperborea by-products from industrial alginate production were provided by IFF/DuPont Nutrition Norge AS (Haugesund, Norway). The by-products contained approximately 3% solids DW and were first pressed using a twin-screw press (Fjell Technology, Straume, Norway) to increase the solid content to approximately 8% DW, before drying in a disc dryer (Fjell Technology, Straume, Norway). The drying temperatures were 80 - 90°C for 4 h, followed by 11 h at 60 - 65°C with vacuum, and finally 2 h at 80°C, to achieve a final DW of 93%. The dried biomass was ground in a spice grinder (Multi Grinder, Model RCMZ-1000, Royal Catering, Berlin, Germany), and transported to the commercial factory - Felleskjøpet Agri (Rindsem Mølle, Verdal, Norway). At the factory, two types of concentrate feeds were produced, a control without the alga by-product (CTR) and an experimental concentrate including the alga by-product at a 6% inclusion rate (HYP, Table 1). Ingredients where ground in a hammer mill (Tietjen Verfahrenstechnik GmbH, Hemdingen, The Netherlands) with dimensions 5 and 6 mm and mixed in a Forberg mixer at 77°C (Forberg, Oslo, Norway). To produce the experimental concentrate, alga by-product was weighed and mixed with the same ingredients as used in the control feed (Table 1). The vitamin and mineral mixture contains iodine, and 3.5 mg I/kg was added to both types of concentrate. After mixing, the concentrates were expanded in a Kahl expander (Amandus Kahl GmbH&Co.KG, Reinbek, Germany) at 110 - 115°C before pelleting (Van Aarsen, Heel, Netherland) and cooling. The pellet sizes were on average 4.8 mm in diameter.
Table 1 Ingredients composition (g/100 g) in the control (CTR) and experimental (HYP) concentrate feeds.
The in vivo experiment was conducted in accordance with the regulation for use of animals in experiments, adopted by the Norwegian Ministry of Agriculture and Food, and approved by the Ethics Commission on Animal Use by the Norwegian Food and Safety Authority, application number FOTS ID 19314. It complies with the EU Directive 2010/63/EU on the use of experimental animals, which was incorporated to the European Economic Area Agreement in May 2015.
Twelve male lambs (Norwegian White Sheep) aged four months with initial weights of on average 37.8 kg +/- 4.1 were used in a feeding experiment where feces, urine, blood, and rumen fluid samples were collected. The experimental period lasted for 10 weeks from September to November 2021. The animals were stalled individually in an uninsulated barn at NIBIO Tjøtta in Nordland County, Norway (65°49’22 N, 12°25’37 E) with free access to water. The lambs were blocked according to initial live weight and randomly, within block (n = 6), assigned to one of two diets, including six animals per diet. The lambs were fed grass silage ad libitum and either the control concentrate (CTR) or the experimental concentrate (HYP). During the first five weeks (Period 1), lambs were offered 400 g concentrate per animal and day, and the next five weeks (Period 2), 600 g per animal and day. The inclusion rate of alga by-product in the total diet was aimed to be 2% throughout the entire experiment. Feed was offered twice daily at 08:30 and 15:00 h. Leftover silage was measured daily to calculate daily feed intake. Concentrate was offered separately from the gras silage, and no leftovers were observed throughout the experiment. Animals were adapted to their respective diets for 26 days before lambs in block 1 - 3 were placed individually in one of six metabolism crates for three consecutive days immediately followed by lambs in block 4 – 6 (Period 1). Management and feeding of the animals were like that in the barn. Lambs returned to the barn after their three days in the metabolism crates. The animals entered the metabolism crates a second time five weeks later, in the same order as during their first visit (Period 2). Feed offered was adjusted relatively to the growth of the lambs. Consequently, the intake of algae, and thus the iodine intake in total diet increased from Period 1 to Period 2.
Grass silage was produced from 1st cut mixed pasture with 25% inclusion of white and red clover. Samples of grass silage offered to the animals were taken twice during each period the animals were fed in the metabolism crates. Samples were dried for 48 h at 60°C to measure dry matter (DM). Feed samples and samples of concentrates were stored at -18°C until analysis.
Feces and urine were collected and measured from each animal on a 24-h basis in the morning before feeding. Buckets were placed under each metabolism crate to collect feces and urine, respectively. One hundred milliliters (ml) of 10% sulphuric acid were added to each urine bucket to avoid loss of ammonia (Özkan-Gülzari et al., 2019). A pH indicator strips non-bleeding pH 0 – 6.0 (Teststrips, pH, MColorpHast™, product number: 1.09531.0001, VWR International, Merck KGaA, Darmstadt, Germany) was used to measure the acidity of urine. Ten percent of the total feces and urine was collected as subsamples and stored at −18°C until further processing. The remaining feces and urine were discarded.
Blood samples were collected from each animal on day 2 within each period. Blood samples were taken from the jugular vein 4 h after morning feeding to Vacuette® EDTA tubes (product number: 454021, Greiner Bio-One, GmbH, Kremsmünster, Austria). Plasma was separated by centrifugation at 4,000 rpm for 15 min at 4°C, after which aliquots of two samples per animal were removed and pooled into a glass vial to give one sample per lamb per period. Plasma was stored at −80°C until further chemical analyses.
Rumen fluid samples were collected from all animals before they were introduced to their respective diets, and within each period the animals were in the metabolism crates. Rumen fluid was collected 4 h post morning-feeding via the esophagus. The samples were collected by inserting a 6 mm flexible PVC tube (Tricoflex) with a stainless-steel sampler with 24 holes of 10 mm diameter into the rumen to a depth of approximately 120 cm. Rumen fluid (a minimum of 50 mL) was obtained using an electric vacuum pump (Edwards RV5, serial no 119464845, West Sussex, UK). The samples were examined visually to ensure they were not contaminated with saliva and strained through a layer of leno bandage (Mesoft, Mölnlycke health care, Göteborg, Sweden). pH values were measured immediately using a pH-meter (VWR Inter-national pHenomenal, PC 5000 H, Serial no 11232089 Leuven, Belgium). The samples were stored at −80°C until further analyses.
Carbon and nitrogen in the dried, ground alga by-product was determined using a Vario EL cube Elemental Analyzer (Elementar Analysensysteme GmbH, Langenselbold, Germany). Total amino acids were quantified as described by Forbord et al. (2020) (Supplementary Information for details), and total lipids by the Bligh and Dyer method, using the same protocol as Jakobsen et al. (2008). Mineral content, including iodine, was determined using the ICP-MS as described in 3.3.4.
Feed samples were ground to pass 1-mm screen using a Tecator Cyclotec Sample Mill (Foss Analytical Co., Ldt., Suzhou, China). Dry matter, ash, crude protein (CP) and neutral detergent fiber (NDF) content of the grass silages were analyzed using Near-infrared spectroscopy (NIRs) by Eurofins Norway performed at Eurofins Agro Testing Wageningen (Binnenhaven 5, NL-6709 PD, Wageningen ISO/IEC 17025:2005 RvA L122). The same components of the concentrates were determined by Felleskjøpet Agri using the same NIRs method. Iodine was quantified as described below (3.3.4).
Frozen fecal samples were course ground and freeze-dried for 48 h, using a Labconco FreeZone 4.5 Plus® freeze-drier (Kansas City, Missouri, USA) at a temperature and vacuum ranging between −80°C and −86°C, and 0.52 and 0.97 mbar, respectively. The freeze-dried samples were weighed immediately, and again after 24 h storage at ambient temperature. The samples were then ground to pass through a 1-mm screen using a Tecator Cyclotec Sample Mill® (Foss Analytical Co., Ltd., Suzhou, China). The final weight of the samples was measured, and subsamples were taken and stored in plastic zipped bags at -18°C until further analyses.
Rumen fluid, urine, and blood plasma were analyzed without any further processing.
For halogens (I, Br), freeze-dried samples (200 mg) were extracted with 5 ml 20% (v/v) tetramethylammonium hydroxide (TMAH) in water at 80°C in a bead bath overnight and diluted to 1% (v/v) TMAH upon analysis, according to European standard EN 15111:2007. For analyses of additional elements in the macroalgae by-products (K, Na, Mg, Ca, P, S, Fe, Cu, Se, As, Cd, Pb), 200 mg freeze-dried samples were extracted with 5 ml 50% (v/v) nitric acid (HNO3) and 1.5 ml 30% H2O2 at 220°C for 20 min in an UltraWAVE microwave oven (Milestone, Italy) and diluted to 5% (v/v) nitric acid upon analysis, in accordance with EN 15763:2009.
The prepared samples were analyzed on an 8800 Triple Quadropole ICP-MS (ICPQQQ) with SPS 4 auto sampler (Agilent Technologies, Santa Clara, USA) and a standard sample introduction system (Micro Mist glass concentric nebulizer, quartz double pass spray chamber, quartz torch with 2.5 mm id and standard nickel cones). He and O2 were used for cell reaction. Instrument settings are summarized in Supplementary Information. Samples were quantified by use of standards from Inorganic Ventures (JRC Plankton BCR-414), with 128Te as internal standard for the halogens and 115In for the non-halogens. All extractions were performed once, with analysis using two analytical replicates.
Data were analyzed using the ANOVA repeated measures mixed effects model in Minitab for Windows version 19.2. To determine feed intake, iodine intake, iodine excretion in feces, urine, blood plasma and rumen fluid between diets and periods, block, diets, and periods were considered fixed effects and animals as random effects. Individual animals were nested under the respective diets. When a significant effect of block, diet or period was found, post hoc comparison of means was made using the Tukey test. Differences were considered significant at P < 0.05.
Table 2 shows the chemical composition of L. hyperborea by-product used in the concentrate. The average chemical composition of grass silage harvested from the two periods, and the two types of concentrate is presented in Table 3. The two concentrate types were formulated to be isocaloric and isonitrogenous. Inclusion of L. hyperborea in the total diet of the lambs fed the HYP diet ended on average for both periods at 1.8% on DM. The ratio of the iodine concentrations between the HYP and CTR concentrates was 116.
Table 3 Average chemical composition of grass silage used in Period 1 and Period 2, control (CTR) and experimental (HYP) concentrates. Where no other units are listed in the table, the content is g/kg DM.
Animals were in the growth stage and the live weights increased from an initial average of 37.8 kg to 46 – 48 kg in Period 1 and to an average of between 52 – 56 kg in Period 2 (Table 4). Lambs fed the CTR diet had higher daily weight gain (P = 0.001) compared to lambs fed the HYP diet, resulting in higher live weight for lambs fed the CTR diet (P = 0.001). Dry matter and nutrient intake increased from Period 1 to Period 2 for all animals in accordance with the growth of the animals (P 0.001). There were no significant differences in daily dry matter intake or nutrient intake between diets (P > 0.3; Table 4).
Table 4 Average live weight, live weight gain and daily nutrient intake for male lambs fed control (CTR) and algae (HYP) diets in Period 1 and Period 2.
There were no differences in iodine intake of roughage between diets (P = 0.39) or periods (P = 0.24). The increased total iodine intake was consistent with the increased concentrate intake between diets and periods (P = 0.001).
Total daily iodine excretion in feces and urine was significantly different between diets (P = 0.001) and periods (P = 0.01) (Table 5). One animal fed the CTR diet during Period 1 had high excretion values but deleting the animal from the dataset did not change the statistical results. The iodine excretion was 83% of the intake in Period 1, and 91% in Period 2, 87% in average, for animals fed the HYP diet (Figure 1), while the excretion balanced the intake for the CTR diet. On average, 37% of the iodine excreted from lambs fed the CTR diet was found in the feces. Respectively, for lambs fed the HYP diet, on average 67% of the iodine was excreted in the feces during Period 1 and Period 2.
Table 5 Average iodine (I) intake and excretion (mg/d) for male lambs fed control (CTR) and algae (HYP) diets in Period 1 and Period 2.
Figure 1 Intake of iodine in feed and excretion of iodine in feces and urine for the individual male lambs fed the HYP diet during Period 1 and Period 2.
The measured iodine concentrations in the animal samples taken from animals fed the HYP diet showed increasing levels from Period 1 to Period 2 (Table 6). The concentration of iodine in the feces, urine and rumen fluid samples collected from sheep fed the HYP diet was 20-40% greater than the concentration found in feces, rumen fluid and urine collected from sheep fed the control (CTR) diet. The concentration of iodine found in the blood of animals fed the HYP diet increased by 85% relative to the concentration found in blood collected from animals fed the control diet.
Table 6 Iodine concentrations in feces, urine, blood plasma and rumen fluid samples from male lambs fed control (CTR) and algae (HYP) diets in Period 1 and Period 2.
In the present experiment, we fed male lambs the by-product from industrial production of alginate from L. hyperborea. In this by-product, most of the alginate has been extracted, and water-soluble compounds have been removed in the extensive washing processes. We expected that these washing processes would have removed a significant amount of iodine. It was therefore surprising that the iodine concentration was in the same range as in the fresh biomass. In other, freeze-dried batches of by-products, the content was even higher (10 - 14 g/kg) (unpublished). However, a material balance showed that approximately 70% of the iodine had been removed, but due to the removal of other components, like minerals, soluble carbohydrates and alginate, the concentration in the by-product increased. This implies that some of the iodine must be bound to the insoluble components. If L. hyperborea by-products are used as a feed ingredient, an additional processing may be required to remove more of the iodine. Washing of the residue at high temperature, like the blanching of macroalgae intended for food (Nielsen et al., 2020), may be an option to consider. Regarding other minerals, such as toxic heavy metals, the alginate extraction process reduced arsenic (As) to approximately 30%, and cadmium (Cd) to less than 10% of the concentrations in the fresh biomass. These minerals were therefore not a concern in our study.
Sheep require a diet containing 0.5 mg iodine/kg DM (NRC, 1985; EFSA, 2013). The FEEDAP panel (EFSA Panel on Additives and Products or Substances used in Animal Feed) recommends the maximum iodine concentration in complete feed be 2 mg iodine/kg feed for dairy cows and minor dairy ruminants with consideration of animal and consumer health (EFSA, 2013). No similar recommendations for meat producing ruminants are given, as meat is not a major source of dietary iodine for the consumer. However, for the safety of the animals, EFSA (2013) concludes that iodine intake should be less than 10 mg/animal per day for ewes and lambs. Lambs fed the CTR diet in the present experiment reached their minimum iodine requirement due to the vitamin and mineral mixture included in the concentrate. The concentrates were formulated to ensure all nutritional requirements were met but at a level of I to avoid lambs fed the CTR diet to exceed the threshold of 10 mg/animal per day. However, lambs fed the HYP diet, had an average intake of iodine of 17 and 27 times this threshold for Period 1 and Period 2, respectively. The high iodine level consumed by the HYP lambs seemed to have no negative effect on animal health. None of the lambs showed signs of iodine poisoning such as depression, anorexia, hyperthermia cough, changes in the respiratory system or death. However, those lambs fed the high iodine diet had a lower growth rate than lambs fed the CTR diet, despite that no significant differences in feed and nutrition intake were observed. McCauley et al. (1973) mentioned reduced growth rate and appetite when lambs were fed iodine levels higher than in our experiment, leading to death of some animals. Gravett (2000) found no differences in feed intake and weight gain on castrated rams fed diets containing 0%, 0.25%, 0.50%, 1.0% or 2.0% on DM level of Ascophyllum extract or dried Ascophyllum. Ascophyllum is the macroalgae mostly reported as a dietary supplement for both sheep and dairy cows (Makkar et al., 2016). However, feeding experiments including diets of high iodine content dealing with iodine intake and excretion in feces, urine and blood have been performed with dairy cows (e.g., Antaya et al., 2015; Antaya et al., 2019; Silva et al., 2022), while similar studies including sheep are missing.
Paulíková et al. (2002) suggests that sheep are less sensitive to iodine intoxication than cattle. In their review (Paulíková et al., 2002), the authors report iodine intoxication in adult dairy cows with levels of 0.12 to 1.0 mg iodine per kg body weight. Similar for lambs, clinical disease was reported when fed levels of 13 to 19 mg iodine per kg body weight. The highest iodine intake per kg live weight found in the present experiment was 5.2 mg (HYP Period 2) fed for five weeks.
A major part of the iodine (67%) was daily excreted in the feces for lambs fed the HYP diet (2.0 to 3.1 mg/kg live weight). The amounts that were absorbed and metabolized corresponded to 1.0 mg/day per kg live weight in Period 1 and 1.6 mg/day per kg live weight in Period 2. Silva et al. (2022) supplemented A. nodosum to dairy cows at different inclusion rates. They reported that 63% iodine was excreted in the feces, while the remaining part was equally distributed between urine and milk. The content of iodine in milk increased linearly with the increase of A. nodosum in the diet. Similar increase in milk iodine was reported by Antaya et al. (2019) when dairy cows were supplemented with A. nodosum, compared with a control diet. Increased iodine intake correlated with increase in iodine concentrations in the blood plasma of lambs, which increased by 85% from Period 1 to Period 2. However, in the present experiment, iodine concentrations also increased relatively from Period 1 to Period 2 in feces and urine, but only by 20-40%. The concentrations of iodine in urine and blood found in lambs fed the HYP diet were in average of the two periods, approximately 10 times higher than that found in samples from lambs fed the CTR diet. However, iodine intake was about 100 times higher for lambs fed the HYP diet than those fed the CTR diet. Most of the iodine in the body is found in the thyroid gland, where it is utilized for the biosynthesis of T3, the active form of thyroid hormones, and T4, the inactive transport form (Paulíková et al., 2002). Hunter and Simpson (1914) showed that continuous daily supplementation of sodium iodide raised the iodine content of sheep’s thyroid gland. However, iodine that is not absorbed by the thyroid gland is stored in the extra-thyroideal iodine pool (i.e., muscle and liver) (NRC, 2007). Grabez et al. (2022) investigated the effect of feeding lambs a diet supplemented with S. latissima on micronutrient content in raw and cured lamb meat. The iodine content in the cured meat was 38 times higher (96 µg/100 g cured meat) than meat from lambs fed a control diet (2.6 µg/100 g cured meat). The authors discuss that a consumption of 100 g cured meat would provide 60% of the daily recommended iodine intake of 150 µg I/day (EFSA, 2017) for adults. Swanson et al. (1990) investigated the relationships between iodine intake by lactating Holstein cows and iodine concentrations in milk and meat. They concluded that moderate changes in dietary iodine are quickly reflected in milk iodine, but iodine in meat is relatively stable. For this reason, the iodine inclusion in diets to dairy cows and small dairy ruminants should be considered more carefully than a similar diet fed to meat producing ruminants such as meat sheep and beef cattle.
We found that lambs fed a diet with the inclusion of L. hyperborea by-product, and the corresponding iodine level, showed no sign of clinical iodine intoxication. Most of the iodine excreted was found in feces but not all iodine was excreted when the lambs were fed the L. hyperborea by-product diet which can indicate a cumulation of iodine in the thyroid gland. Further experiments should be performed to examine where excess iodine is stored and to reveal any negative physiological effects of the high iodine levels.
The raw data supporting the conclusions of this article will be made available by the authors, without undue reservation.
The animal study was reviewed and approved by Norwegian Food and safety authority.
VL and IA acquired funding. IA and MO provided the concentrate. VL designed the study. VL and JTS conducted the experiment and collected all samples. IA analysed all samples. All authored wrote the full manuscript. All authors contributed to the article and approved the submitted version.
The project was funded partly by Mabit (UB0085) and is part of the SeaSolutions project, through the ERA-GAS cofound under Horizon 2020 with funding from the Research Council in Norway (308942).
The authors want to thank Trond Helgerud at IFF/DuPont Nutrition Norge AS, who provided the Laminaria hyperborea by-product for the experiment.
Author MO was employed by company Felleskjøpet Fôrutvikling.
The remaining authors declare that the research was conducted in the absence of any commercial or financial relationships that could be construed as a potential conflict of interest
All claims expressed in this article are solely those of the authors and do not necessarily represent those of their affiliated organizations, or those of the publisher, the editors and the reviewers. Any product that may be evaluated in this article, or claim that may be made by its manufacturer, is not guaranteed or endorsed by the publisher.
The Supplementary Material for this article can be found online at: https://www.frontiersin.org/articles/10.3389/fanim.2023.1213890/full#supplementary-material
Abbot D., Aasen I. M., Beauchemin K. A., Grøndahl F., Gruninger R., Haye M., et al (2020). Seaweed and seaweed bioactives for mitigation of enteric methane: Challenges and opportunities. Animals 10, 2432. doi: 10.3390/ani10122432
Antaya N. T., Ghelichkhan M., Pereira A. B. D., Soder K. J., Brito A. F. (2019). Production, milk iodine, and nutrient utilization in Jersey cows supplemented with the brown seaweed Ascophyllum nodosum (kelp meal) during the grazing season. J. Dairy Sci. 102, 8040–8058. doi: 10.3168/jds.2019-16478
Antaya N. T., Soder K. J., Kraft J., Whitehouse N. L., Guidon N. E., Erickson P. S., et al. (2015). Incremental amounts of Ascophyllum nodosum meal do not improve animal performance but do increase milk iodine output in early lactation dairy cows fed high-forage diets. J. Dairy Sci. 98, 1991–2004. doi: 10.3168/jds.2014-8851
Belanche A., Jones E., Parveen I., Newbold C. J. (2016). A metagenomics approach to evaluate the impact of dietary supplementation with Ascophyllum nodosum or Laminaria digitata on rumen function in Rusitec fermenters. Front. Microbiol. 10. doi: 10.3389/fmicb.2016.00299
Duinker A., Kleppe M., Fjære E., Biancarosa I., Heldal H. E., Dahl L., et al. (2020). Knowledge update on macroalgae food and feed safety (Institute of Marine Research Norway), 44. Available at: https://www.hi.no/templates/reporteditor/report-pdf?id=34442&15441571
EFSA (2013). Scientific opinion on the safety and efficacy of Iodine compounds (E2) as feed additives for all species: calcium iodate anhydrous (coated granulated preparation) based on a dossier submitted by Doxal Italia S.p A. EFSA J. 11 (3), 3178. doi: 10.2903/j.efsa.2013.3178
EFSA (2017). Dietary reference values for nutrients. Summary report; Technical report Vol. 14 (Parma, Italy: EFSA Supporting Publications).
Forbord S., Matsson S., Brodahl G. E., Bluhm B. A., Broch O. J., Handå A., et al. (2020). Latitudinal, seasonal and depth-dependent variation in growth, chemical composition and biofouling of cultivated Saccharina latissima (Phaeophyceae) along the Norwegian coast. J. Appl. Phycol. 32, 2215–2232. doi: 10.1007/s10811-020-02038-y
Grabez V., Coll-Brasas. E., Fulladosa E., Hallenstvedt E., Håseth T. T., Øverland M., et al. (2022). Seaweed inclusion in finishing lamb diet promotes changes in micronutrient content and flavour-related compounds of raw meat and dry-cured leg (Fenalår). Foods 11, 1043. doi: 10.3390/foods11071043
Gravett R. B. (2000). The effects of Ascophyllum nodosum on immune function, performance and carcass characteristics of sheep and cattle (Master thesis, animal science, Texas Tech University). Available at: https://ttu-ir.tdl.org/bitstream/handle/2346/16611/31295008017922.pdf?sequence=1&isAllowed=y.
Hunter A., Simpson S. (1914). The influence of a diet of marine algae upon the iodine contenct of sheep’s thyroid. J. Biol. Chem. 20, 119–122. doi: 10.1016/S0021-9258(18)88259-X
Huszenicza G., Kulcsar M., Rudas P. (2002). Clinical endocrinology of thyroid gland function in ruminants. Veterinární medicína 47 (7), 199–210. doi: 10.17221/5824-VETMED
Jakobsen A. N., Aasen I. M., Josefsen K. D., Strøm A. R. (2008). Accumulation of docosahexaenoic acid-rich lipid in thraustochytrid Aurantiochytrium sp. strain T66: effects of N and P starvation and O2 limitation. Appl. Microbiol. Biotechnol. 80, 297–306. doi: 10.1007/s00253-008-1537-8
Lind V., Weisbjerg M. R., Jørgensen G. M., Fernandez-Yepes J. E., Arbesú L., Molina-Alcaide E. (2020). Ruminal fermentation, growth rate and methane production in sheep fed diets including white clover, soybean meal or Porphyra sp. Animals 10, 79. doi: 10.3390/ani10010079
Makkar H. P. S., Tran G., Hauzé V., Giger-Reverdin S., Lessire M., Lebas F., et al. (2016). Seaweeds for livestock diets: A review. Anim. Feed Sci. Technol. 212, 1–17. doi: 10.1016/j.anifeedsci.2015.09.018
McCauley E. H., Linn J. G., Goodrich R. D. (1973). Experimentally induced iodide toxicosis in lambs. Am. J. Vet. Res. 34 (1), 65–70.
Nielsen C. W., Holdt S. L., Sloth J. J., Marinho G. S., Sæther M., Funderud J., et al. (2020). Reducing the high iodine content of Saccharina latissima and improving the profile of other valuable compounds by water blanching. Foods 9, 569. doi: 10.3390/foods9050569
NRC (National Academy of Science) (1985). Nutrient requirements for sheep. 6th rev. edn (Washington DC, National Academy Press). Available at: https:///www.scribd.com/document/517523044/Nutrient-Requirements-of-Sheep-Sixth-Revised-Edition-1985#
NRC (National Academy of Science) (2007). Nutrient Requirements of Small Ruminants: Sheep, Goats, Cervids, and New World Camelids (Washington DC: National Academies Press). doi: 10.17226/11654
Özkan-Gülzari S., Lind V., Aasen I. M., Steinshamn H. (2019). Effect of supplementing sheep diet with macroalgae species in in vivo nutrient digestibility, rumen fermentation and blood amino acid profile. Animal 13, 2792–2801. doi: 10.1017/S1751731119001502
Paulíková I., Kovác G., Bíris J., Paulík S., Seidel H., Nagy O. (2002). Iodine toxicity in ruminants. Vet. Med. Czech. 47, 343–350. doi: 10.17221/5845-VETMED
Roleda M. Y., Skjermo J., Marfaing H., Jónsdottir R., Rebours C., Gietl A., et al. (2018). Iodine content in bulk biomass of wild-harvested and cultivated edible seaweeds: Inherent variations determine species-specific daily allowable consumption. Food Chem. 254, 333–339. doi: 10.1016/j.foodchem.2018.02.024
Silva L. H. P., Reis S. F., Melo A. T. O., Jackson B. P., Brito A. F. (2022). Supplementation of Ascophyllum nodosum meal and monensin: Effects on diversity and relative abundance of ruminal bacterial taxa and the metabolism of iodine and arsenic in lactating dairy cows. J. Dairy Sci. 105, 4083–4098. doi: 10.3168/jds.2021-21107
Swanson E. W., Miller J. K., Mueller F. J., Patton C. S., Bacon J. A., Ramsey N. (1990). Iodine in milk and meat of dairy cows fed different amounts of potassium iodide or ethylenediamine dihydroiodide. J. Dairy Sci. 73, 398–405. doi: 10.3168/jds.S0022-0302(90)78686-9
Keywords: feed intake, lamb growth, feces, urine, blood plasma, rumen fluid
Citation: Lind V, Opheim M, Sandvik JT and Aasen IM (2023) Iodine intake and excretion from sheep supplemented with macroalgae (Laminaria hyperborea) by-product. Front. Anim. Sci. 4:1213890. doi: 10.3389/fanim.2023.1213890
Received: 28 April 2023; Accepted: 11 July 2023;
Published: 27 July 2023.
Edited by:
Melissa Duplessis, Agriculture and Agri-Food Canada (AAFC), CanadaReviewed by:
Daniel Brugger, University of Zurich, SwitzerlandCopyright © 2023 Lind, Opheim, Sandvik and Aasen. This is an open-access article distributed under the terms of the Creative Commons Attribution License (CC BY). The use, distribution or reproduction in other forums is permitted, provided the original author(s) and the copyright owner(s) are credited and that the original publication in this journal is cited, in accordance with accepted academic practice. No use, distribution or reproduction is permitted which does not comply with these terms.
*Correspondence: Vibeke Lind, VmliZWtlLmxpbmRAbmliaW8ubm8=
Disclaimer: All claims expressed in this article are solely those of the authors and do not necessarily represent those of their affiliated organizations, or those of the publisher, the editors and the reviewers. Any product that may be evaluated in this article or claim that may be made by its manufacturer is not guaranteed or endorsed by the publisher.
Research integrity at Frontiers
Learn more about the work of our research integrity team to safeguard the quality of each article we publish.