- 1Division of Food and Animal Science, School of Agriculture, Communities and the Environment, Kentucky State University, Frankfort, KY, United States
- 2Phibro Animal Health Corporation, Teaneck, IL, United States
The aim of our study was to examine the effects of an immunomodulatory feed additive (OmniGen® PRO, Phibro Animal Health, Teaneck, NJ) on ruminal fermentation homeostasis and biomarkers of stress and inflammation in dairy heifers following subacute ruminal acidosis (SARA). Holstein heifers (n = 32, 8.4 ± 0.3 months old) were allocated to receive two treatments based on body weight (BW). Treatments were (1) control (CON; n = 16, no additive) and (2) OmniGen® PRO (OGPRO, n = 16, 10 g/100 kg BW, top-dressed). From d 1 to 69, and d 72 to 77, heifers were fed ad libitum a basal TMR formulated for early lactation. On d 70, the TMR offerings were reduced by 50% based on the intake of the previous 3 days. On d 71, heifers were fed ad libitum a starch challenge diet, which was a 50/50 mix steam-rolled barley and the basal TMR on a DM basis, to induce SARA. Rumen fluid and blood samples were collected on d 66, 71, and 73. Prior to the challenge, DMI, ADG, and feed efficiency were not affected by treatment. Following the challenge, all heifers experienced a 43% decline in DMI. Rumen pH was lower on the challenge day than pre and post challenge but was unaffected by treatment. Ruminal lactate was negligible pre and post challenge but increased on the challenge day; OGPRO reduced ruminal lactate compared to CON. At all sampling points, rumen total VFA were higher in OGPRO than in CON. The challenge caused fluctuations in the acetate to propionate ratio in CON, while OGPRO heifers had less variation. Two days post challenge, OGPRO heifers tended to have lower plasma cortisol, haptoglobin, and non-esterified fatty acids (NEFA) than CON heifers. The OGPRO heifers maintained the mean corpuscular hemoglobin concentration (MCHC) and platelet concentration after the challenge, while their levels declined in CON. In this study, supplementing OGPRO to heifers fed an early lactation diet improved rumen fermentation measures prior to the starch challenge and reduced the negative effects of the challenge on rumen fermentation. In addition, following the challenge, indicators of systemic inflammation tended to be lower in heifers supplemented with OGPRO.
Introduction
Rumen microorganisms metabolize feed substrates and produce volatile fatty acids (VFAs). These major fermentation products supply about 70% of the ruminant’s metabolic energy needs (Bergman, 1990) toward the requirements for maintenance, growth, pregnancy, and milk production. Therefore, it is important to ensure efficient rumen fermentation to achieve high milk efficiency in dairy cows. However, stress can disrupt or alter a cow’s genetic potential for maximum milk production. Many common stressful events, such as transportation, heat stress, and ruminal subacute and acute acidosis, can affect cow health and reduce milk production (Stone, 2004; Hong et al., 2019; Briggs et al., 2021). Subacute ruminal acidosis (SARA) is one of the most common metabolic diseases for lactating dairy cows, affecting about 19% and 26% of early and mid-lactation dairy cows, respectively (Garrett et al., 1997; Oetzel et al., 1999). Plaizier et al. (2008) conducted a thorough review on SARA in dairy cows and suggested that SARA can decrease intake, reduce fiber digestion, and cause milk fat depression, laminitis, diarrhea, liver abscesses, and inflammation in dairy cows. A recent review has postulated that, because SARA induces endotoxemia, as does severe heat stress, the potential for SARA to exacerbate endotoxemia is even higher in heat-stressed cows (Burhans et al., 2022).
An immunomodulatory feed additive (OmniGen® AF, Phibro Animal Health Corporation, Teaneck, NJ) has been previously reported to help support the immune system during periods of stress. For example, heat-stressed lactating cows supplemented with OmniGen AF had lower plasma haptoglobin (Leiva et al., 2017) and lower cortisol (Hall et al., 2018) than non-supplemented controls. OmniGen PRO (OGPRO) is an immunomodulatory feed additive (Phibro Animal Health, Teaneck, NJ) that contains fermentation components for enhancing rumen fermentation in dairy cattle. The purpose of this study was to investigate the effect of OGPRO on rumen fermentation parameters and markers of systemic stress in heifers nutritionally induced with SARA. We hypothesized that OGPRO would improve ruminal fermentation of an early lactation diet and reduce stress and inflammation after a SARA challenge.
Materials and methods
Animals, diet, and experimental design
The animal experiment was conducted in the Corvallis Research Center of Phibro Animal Health Corporation (Corvallis, OR, USA). All animals were cared for in accordance with the Phibro Animal Health Animal Care and Use Policy.
Two groups of 16 Holstein heifers (n = 32) were enrolled in the study 2 days apart. This was done to ensure that there was enough labor available during each of the sample collection periods. Within each group, heifers were blocked to one of two treatments based on BW and started treatment on the same day. Treatments were (1) CON, basal TMR diet with no additives, and (2) OGPRO, basal TMR and OmniGen PRO (10 g/100 kg BW/day, equivalent to 63 g/head/day, top-dressed). OmniGen Pro is a proprietary combination of all-natural silicates and yeast components with functional metabolites and essential precursors. The basal TMR represented an early lactation diet with 45% corn silage, 6.2% alfalfa hay, 3.2% fescue hay, and 45.2% concentrate (Table 1). Animals were housed in free-stall barns equipped with a Calan Broadbent Feeding System (American Calan, Northwood, NH). An early lactation diet was used to determine the effects of OGPRO on ruminal fermentation of the early lactation diet and help induce subsequent SARA challenges. Animals had free access to water. From d 1 to 69, and d 72 to 77, all heifers were fed ad libitum a basal total mixed ration (TMR) formulated for early lactation (16% CP, 31% starch, 28% NDF, 1.62 Mcal/kg NEL) once per day in the morning. On d 70, the TMR offerings were reduced by 50% based on the average intake of the previous 3 days. On d 71, heifers were fed ad libitum a 50/50 mix containing steam-rolled barley and the basal lactation diet on a DM basis to induce SARA. The SARA diet was offered for 1 day during regular morning feeding time and allowed to be consumed throughout the day in the same manner as regular feeding days. From the morning of d 72, the basal diet was fed to all heifers ad libitum until the end of the trial. The SARA diet had a starch content of 41.3% on a DM basis (Table 1). Offered feed and refusal weights were recorded daily. Except for the feed restriction day (d 70), the amounts of feed offered were adjusted daily to ensure that the orts were 5%–10% of the offered amount. Since a well-mixed TMR was offered and the orts amount was controlled throughout the trial, no apparent sorting behavior was observed.
Sample collection and analysis
Body weight was measured weekly. Dry matter content of TMR and refusal was determined daily by drying samples in a forced-air oven at 60°C for at least 48 h for calculation of DMI (AOAC, 1980). The nutrient analysis of the basal TMR is presented in Table 1. The TMR samples were ground to pass through a 1-mm screen on a Wiley mill (Thomas Scientific, Swedesboro, NJ) and composited across days and analyzed for nutrient composition at a commercial laboratory (Dairyland Laboratories Inc, De Pere, WI) using the NIR spectroscopy method.
Rumen fluid was collected on d 66, 71, and 73 using an oral tubing method. Rumen fluid (100 mL, after discarding the first 100 mL to avoid saliva contamination) was collected 4 h after morning feeding on d 66 and 73 and 9 h after feeding on d 71. Rumen fluid pH generally starts to decrease after meal and reach a relatively low pH, with only a slight change 4–10 h after feeding (Krause and Oetzel, 2005). When starch challenge diet was provided, ruminal pH continuously dropped rapidly and took 9–10 h to reach ruminal nadir pH (Krause and Oetzel, 2005). Thus, we adopted the different collection times to observe nadir pH after starch challenge while also be able to compare across studies when basal diet is fed, since 3–4 h post-feeding rumen fluid collection is recommended and commonly practiced. Rumen fluid was filtered through four layers of cheesecloth and the pH was measured immediately after collection. Unacidified samples were centrifuged at 3,260 × g, 4°C for 15 min and the supernatant was used to determine NH3-N by distillation method with modification using Kjelsorb reagent (Peters et al., 2003). Another set of rumen fluid was acidified by adding 1% of 50% sulfuric acid and centrifuged at 3,260 × g, 4°C for 15 min. The VFA and lactic acid concentrations in the supernatant were determined by High Performance Liquid Chromatography in accordance with Canale et al. (1984) as modified by Muck.
Blood was collected via the jugular vein into sodium EDTA tubes on d 0, 66, 71, and 73 at the time of rumen fluid collection. Blood in EDTA tubes were aliquoted (1 mL) to measure complete blood count (CBC) using a Heska Element HT5 analyzer (Heska, Loveland, CO), in accordance with the manufacturer’s instructions. The remaining blood samples were centrifuged at 2,500 × g, 4°C for 20 min for plasma harvesting. The resulting plasma was aliquoted and stored at –80°C for further analysis. Plasma concentrations of glucose (Pointe scientific, Canton, MI), NEFA (NEFA-HR kit from Fujifilm Wako Diagnostics; Mountain View, CA), cortisol (Ann Arbor Assays, MI), haptoglobin (Tridelta Development Limited, Maynooth, Ireland), and lipopolysaccharides (LPS) binding protein (LBP, Cell Science, Inc. Canton, MA) were measured following the manufacturer’s recommendations, after testing for appropriate dilutions.
Statistical analysis
Data were analyzed with the GLIMMIX procedure of SAS 9.4, using the model described below.
where Y is the response variable, μ is the overall mean, Ti is the effect of treatment, Dj is the effect of day (or week where applicable), CVk is the effect of covariate, (T × D)ij is the interaction of treatment and day (or week), Gl is the effect of group (random), and εijkl is the residual error.
Dependent variables with repeated observations within an experimental unit were analyzed as repeated measures. The model above was adjusted to include animal as the subject, and AR(1) as the time-series covariance structure, which was selected based on the smallest Akaike information criterion values. The ruminal blood parameters, BW on d 0, and DMI from the week prior to the start of the experiment were used as covariates for the corresponding measures. A slice statement of LSMEANS was conducted to compare treatment differences on individual days. One animal had abnormal CBC values during d 66 to d 71, thus was regarded as an outlier and removed from the statistical analysis of CBC and blood parameters. Two animals from CON showed symptoms of lethargy and no appetite, and therefore were removed from the study on d 74 for subsequent DMI measurement. Complete blood count data were power-transformed prior to the statistical analysis to ensure normal distribution of the residual, and the resulting least squares means and SEM were transformed back to the original scale and reported. Statistical differences were declared significant if P ≤ 0.05. Tendency was declared at 0.05 < P ≤ 0.10.
Results
During the first 69 days of the study, prior to the starch challenge, the CON and OGPRO groups did not differ (P > 0.10, Table 2) in average DMI, DMI as a percentage of BW, weekly BW, and ADG. Treatments did not differ (P > 0.10, Figure 1) in DMI during or after the starch challenge. The day after the starch challenge (d 72), animals were fed ad libitum, but they had a drastic reduction in DMI from d 71 (5.56 vs. 9.61 kg/day, P < 0.01). On day 73, animals on both treatments recovered their intakes to similar levels as those prior to the starch challenge (8.15 kg/day).
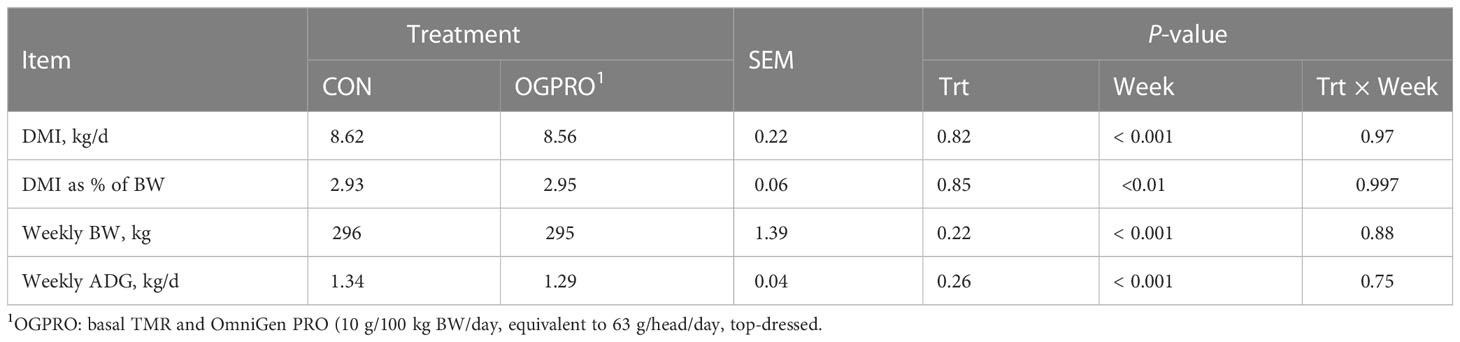
Table 2 Intake and growth performance of Holstein heifers fed the control diet (CON) or OmniGen PRO (OGPRO) for 10 weeks.
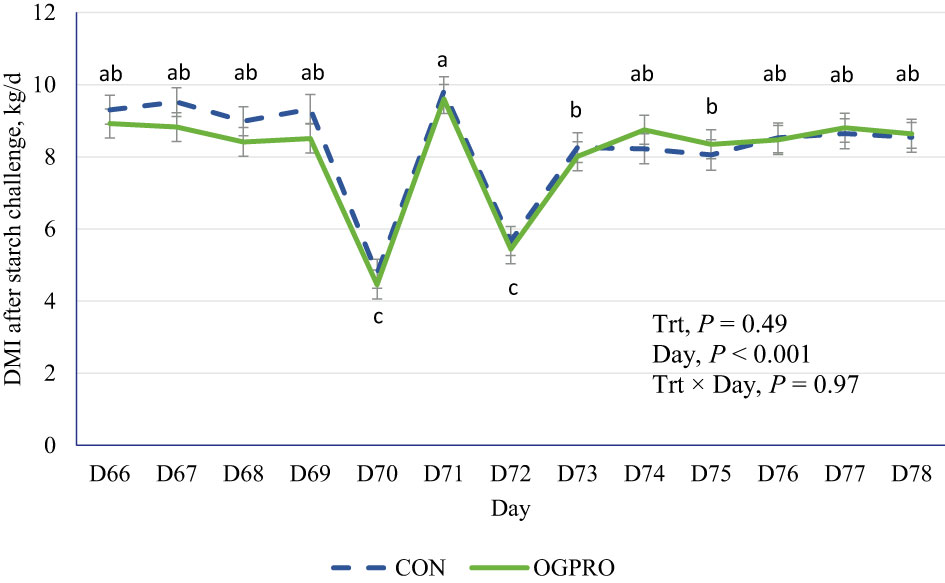
Figure 1 Dry matter intake of Holstein cattle fed the control diet (CON, dotted blue line) or OmniGen PRO (OGPRO, solid green line) before and after the starch challenge. On d 70, the TMR offerings were reduced by 50% based on the average of the previous 3 d intakes. The starch challenge was induced on d 71 by including rolled barley at 50% of dietary DM (mixed with the basal TMR) and offered to animals ad libitum. OGPRO: basal TMR and OmniGen PRO (10 g/100 kg BW/day, equivalent to 63 g/head/day, top-dressed. abc Daily means without common superscript differ, P ≤ 0.05.
As a result of the starch challenge, ruminal pH decreased (P < 0.01, Figure 2A) to below 6.0 on the day of the challenge, while the pH was above 6.5 prior to and post challenge. Ruminal pH was not affected by treatments. The low ruminal pH on the day of challenge for the CON group (pH 5.85) suggests a successful induction of SARA in this study. The ruminal pH of animals fed OGPRO was 5.93 on d 71. Ruminal lactate concentration was negligible on d 66 and d 73 for both treatments (Figure 2B). However, as a result of the starch challenge on d 71, lactate production spiked in the CON group while OGPRO minimized the lactate accumulation (2.93 vs. 14.8 mM, P ≤ 0.05 for d 71).
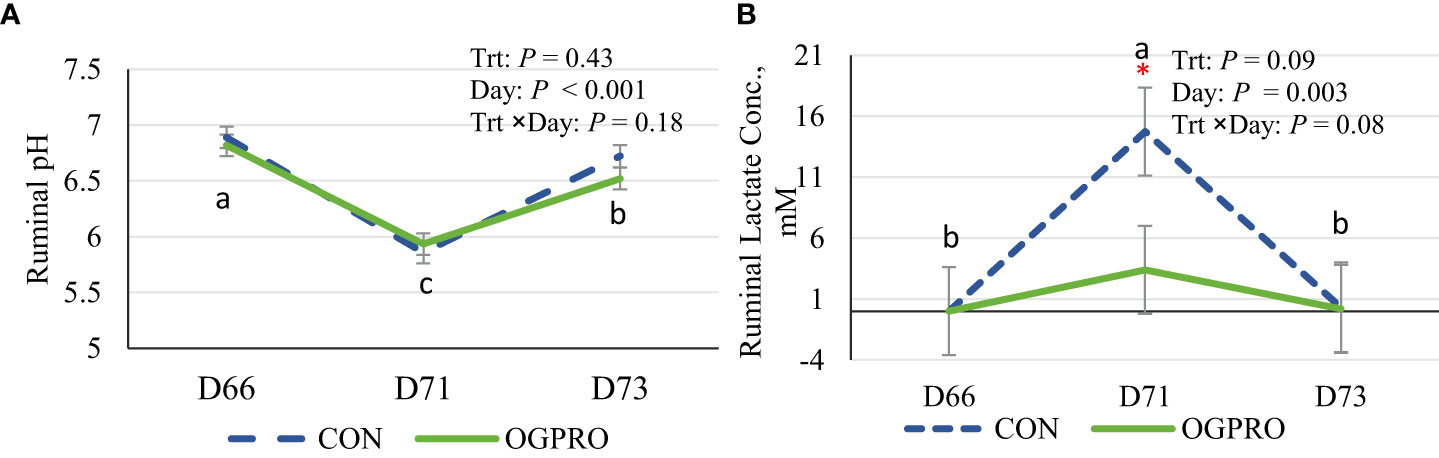
Figure 2 Ruminal pH (A) and lactic acid concentration (B) of Holstein heifers fed the control diet (CON, dotted blue line) or OmniGen PRO (OGPRO, solid green line) before (d 66), during (d 71), and after (d 73) the starch challenge. The starch challenge was induced on d 71 by including rolled barley at 50% of dietary DM (mixed with the basal TMR) and offered to animals ad libitum. OGPRO: basal TMR and OmniGen PRO (10 g/100 kg BW/day, equivalent to 63 g/head/day, top-dressed. *Treatment differed on the given day, P ≤ 0.05. a,b,c: Daily means without common superscript differ, P ≤ 0.05.
Feeding OGPRO consistently increased (P = 0.03) total VFA concentrations compared to CON (Figure 3A). Treatments had similar molar proportions of acetate, propionate, and butyrate on d 66 and 73 (Figures 3B–D). However, on the starch challenge day, those in the OGPRO group had lower (P ≤ 0.05 for d 71) acetate proportions and higher (P ≤ 0.05 for d 71) butyrate proportions than those in the CON group. The proportions of isobutyrate (Figure 3E) and isovalerate (Figure 3F) were reduced after the starch challenge, stayed at a low level on day 73, and were unaffected by treatment. Valerate proportion was slightly lower on d 71 than on d 66 and increased drastically on d 73 but was unaffected by treatment (Figure 3G). The acetate to propionate ratio drastically increased in the CON group on d 71 compared with d 66 (P = 0.05, Figure 3H) and returned to the baseline level on d 73. Unlike in the CON group, in the OGPRO group, the acetate to propionate ratio was relatively unaffected by the starch challenge and stayed at a lower level than in the CON group on d 71 (4.03 vs. 6.03).
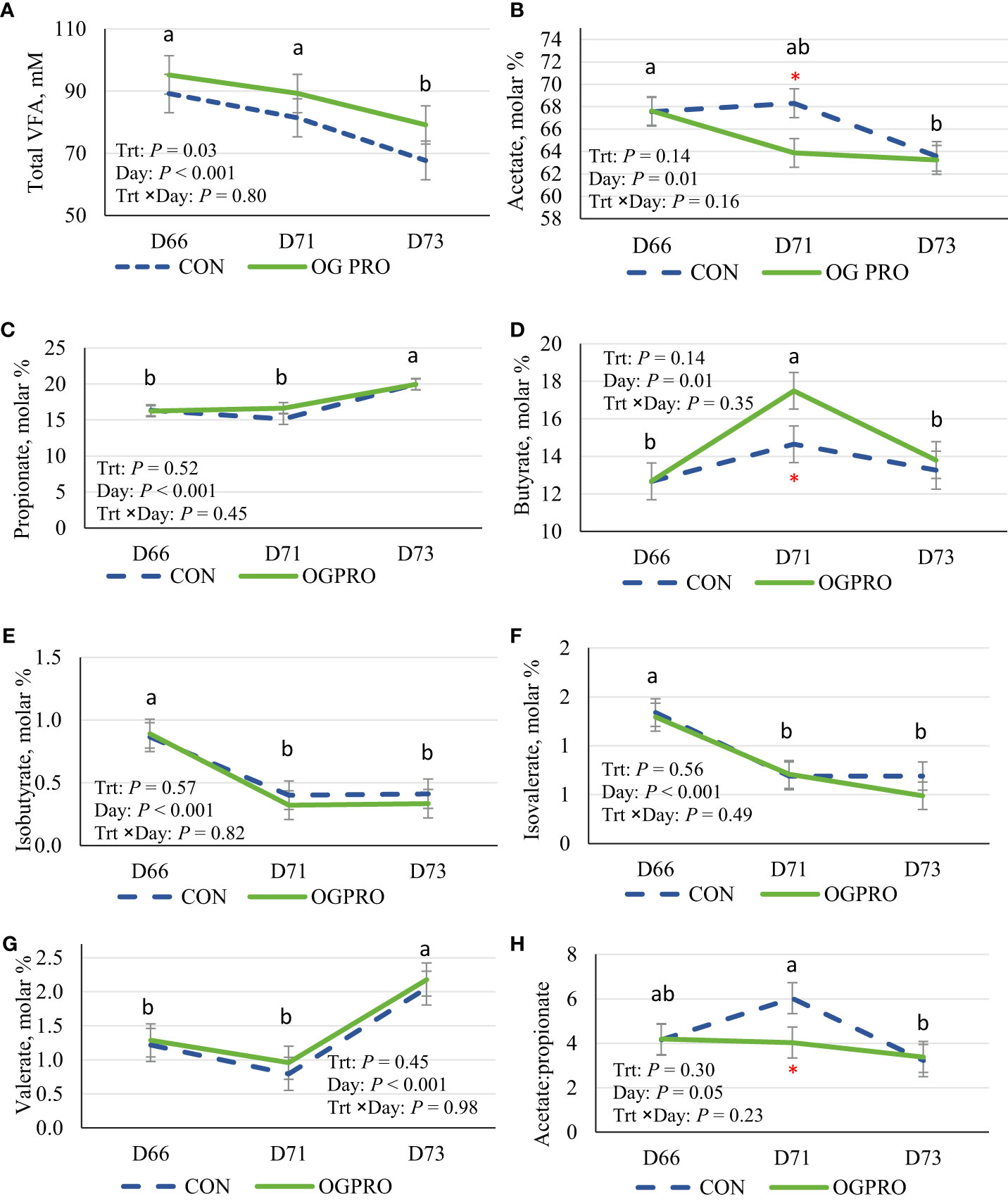
Figure 3 Ruminal total VFA concentration (A), and molar proportions of acetate (B), propionate (C), butyrate (D), isobutyrate (E), isovalerate (F), valerate (G), and acetate to propionate ratio (H) of Holstein heifers fed the control diet (CON, dotted blue line) or OmniGen PRO (OGPRO, solid green line) before (d 66), during (d 71), and after (d 73) the starch challenge. *Treatment differed on the given day, P ≤ 0.05. ab Daily means without common superscript differ, P ≤ 0.05.
Plasma glucose concentration was not affected by starch challenge or treatment (P > 0.10 Figure 4A). Plasma concentrations of NEFA were increased (P = 0.01, Figure 4B) by the starch challenge on d 73. Feeding OGPRO tended to reduce (P ≤ 0.10) blood NEFA on d 73 compared to CON (120 vs. 152 µmol/L). In addition, feeding OGPRO tended to reduce (P = 0.06, Figure 4C) plasma cortisol concentration compared to CON group, which was most pronounced on d 73 (P ≤ 0.05). Plasma haptoglobin levels were elevated (P = 0.03, Figure 4D) by the starch challenge on d 73. Feeding OGPRO tended to reduce haptoglobin compared to CON group (481 vs. 787, P = 0.07) on d 73. Plasma LBP concentrations were also increased (P < 0.01, Figure 4E) by the starch challenge on d 73 and unaffected (P > 0.10) by treatment.
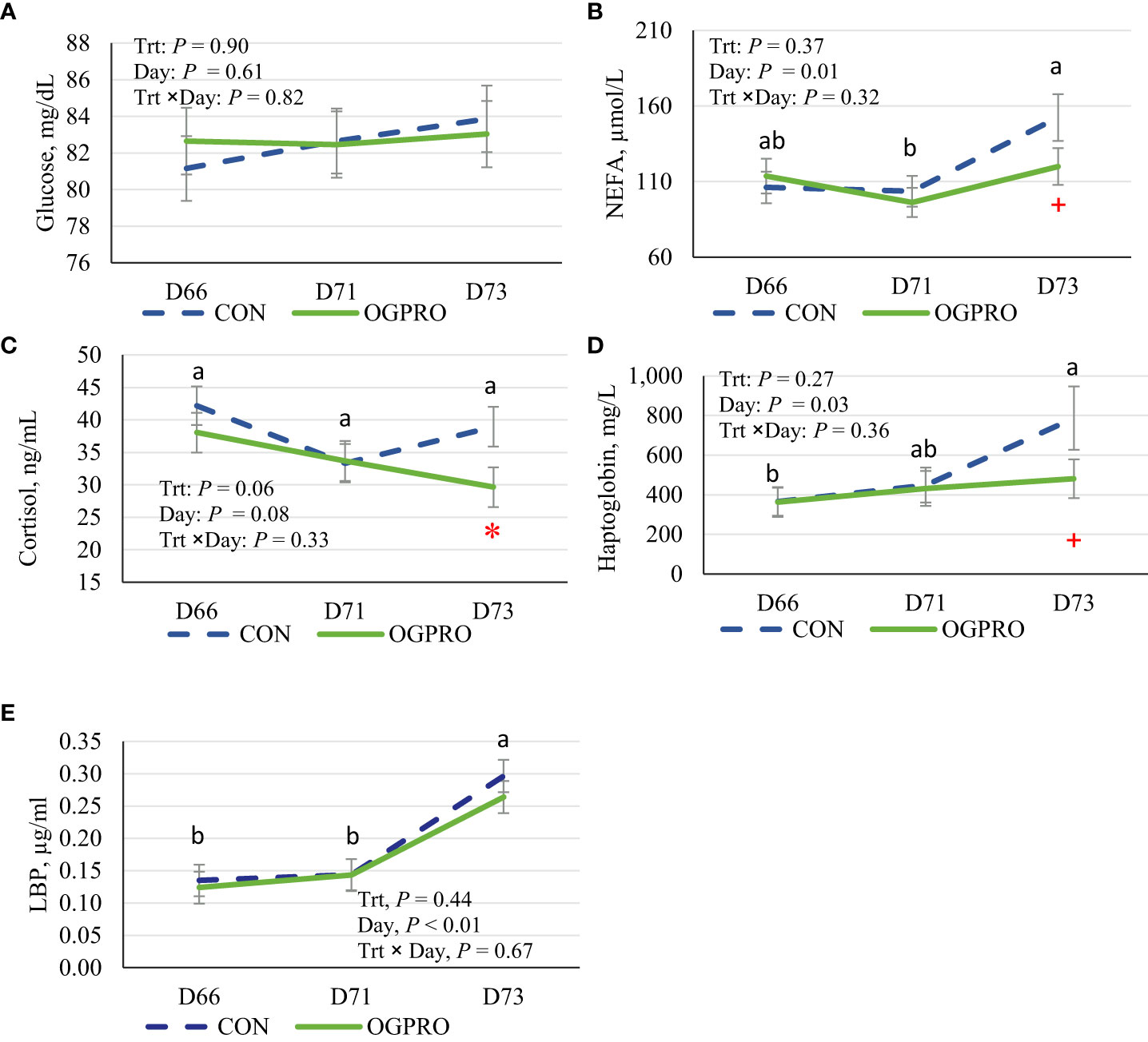
Figure 4 Plasma concentrations of non-esterified fatty acids (NEFA, A), glucose (B), cortisol (C), haptoglobin (D), LPS binding protein (LBP, E) of Holstein heifers fed the control diet (CON, dotted blue line) or OmniGen PRO (OGPRO, solid green line) before (d 66), during (d 71), and after (d 73) the starch challenge. *Treatment differed on the given day, P ≤ 0.05. +Treatment tended to differ on the given day 0.05 < P ≤ 0.10. ab Daily means without common superscript differ, P ≤ 0.05.
The counts of white blood cells, neutrophils, lymphocytes, monocytes, basophils, and MCHC were affected by the starch challenge, and thus differed across days (Table 3 and Figure 5, P ≤ 0.05), while eosinophils, red blood cells, hemoglobin, and hematocrit were not affected (P > 0.10) by the starch challenge. Feeding OGPRO tended to increase (P = 0.06, Figure 5A) monocytes. The starch challenge reduced (P = 0.05) MCHC (Figure 5B). MCHC was lower (P = 0.002) in the CON than OGPRO. In addition, OGPRO tended (P = 0.07, Figure 5C) to have higher circulating platelets in the blood; this is because OGPRO feeding maintained pre-challenge concentrations while CON feeding decreased platelet numbers by 23% from d 66 to d 73.
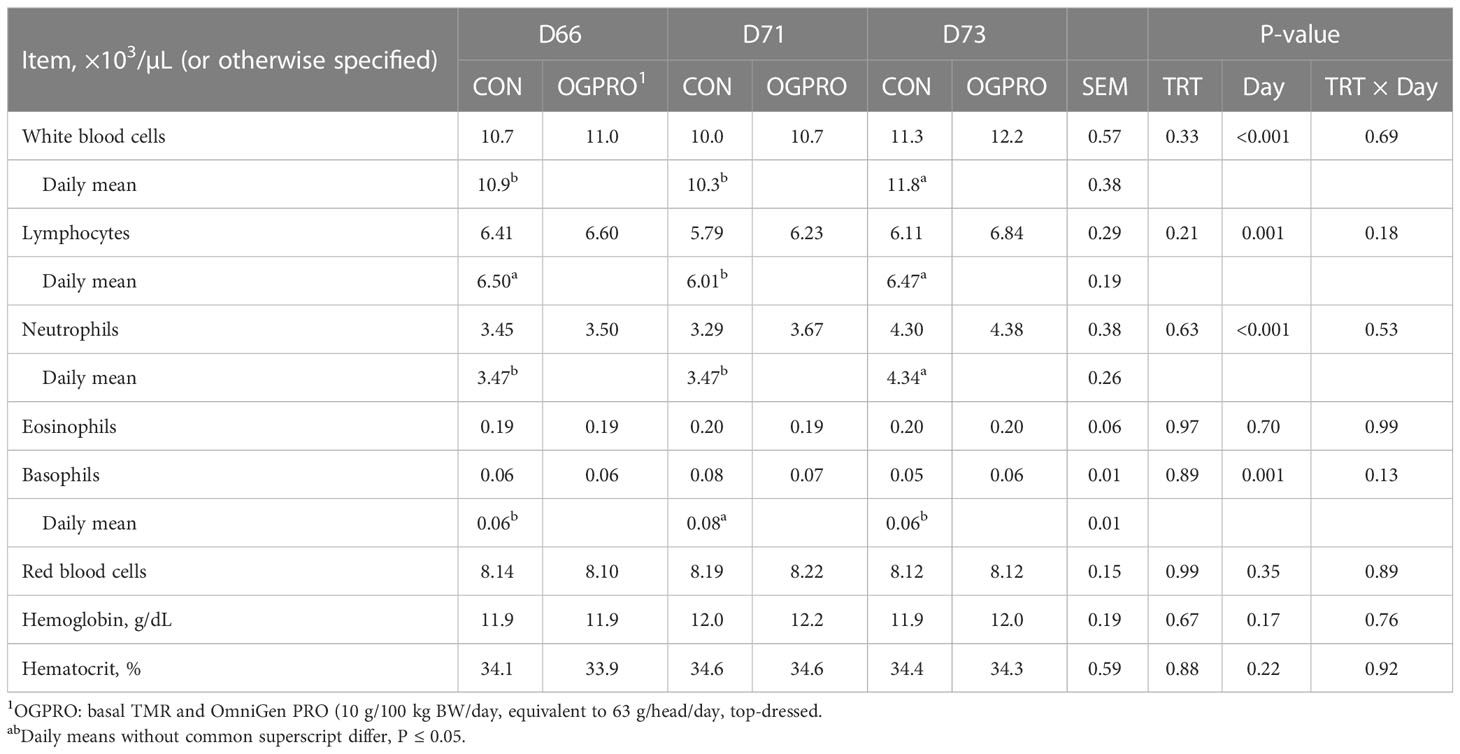
Table 3 Complete blood count of Holstein heifers fed Control (CON) or OmniGen PRO (OGPRO) before (d 66), during (d71) and after (d73) a starch challenge.
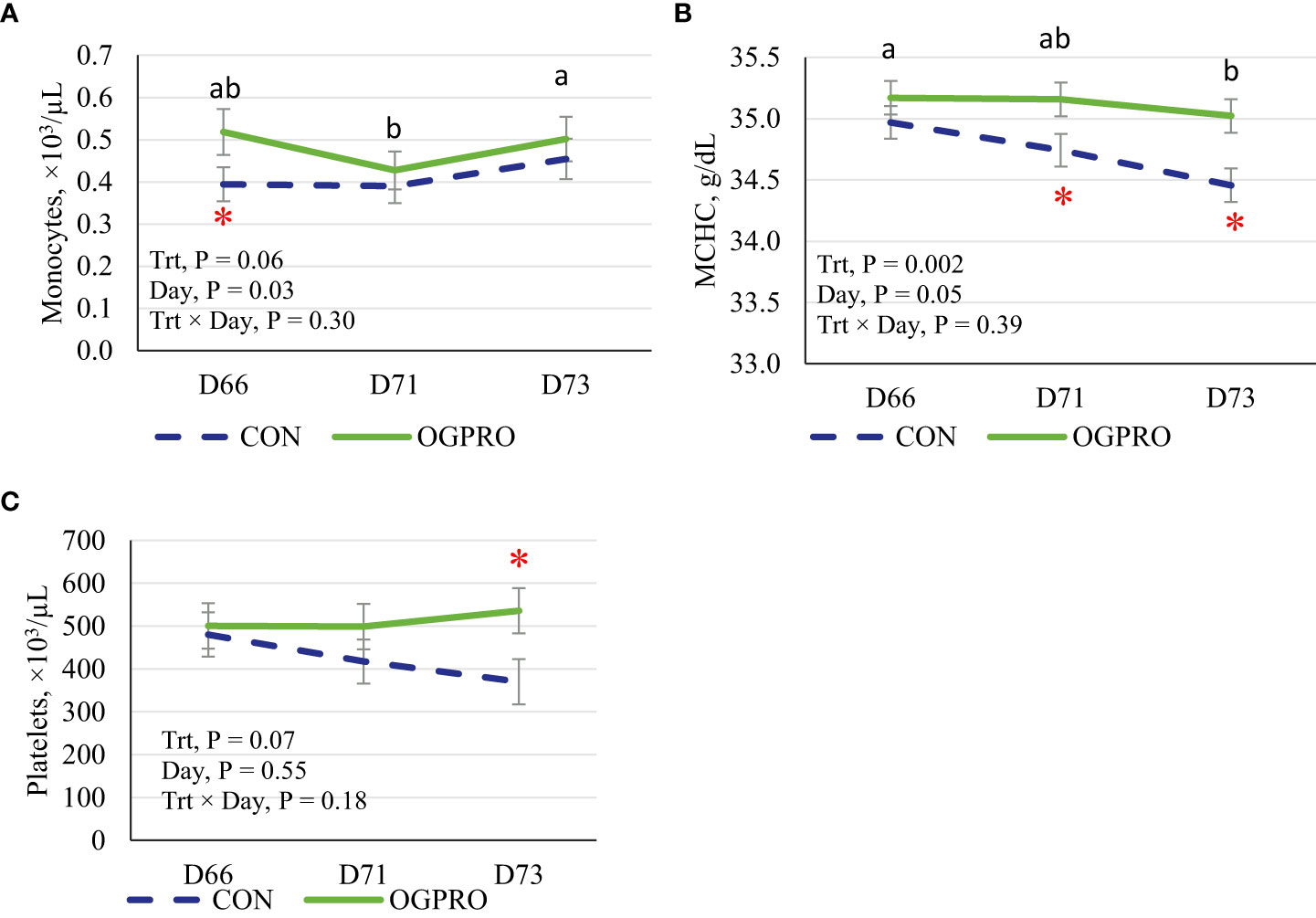
Figure 5 Blood count of monocytes (A), mean corpuscular hemoglobin concentration (MCHC, B), and platelets (C) of Holstein heifers fed the control diet (CON, dotted blue line) or OmniGen PRO (OGPRO, solid green line) before (d 66), during (d 71), and after (d 73) the starch challenge. *Treatment differed on the given day, P ≤ 0.05. ab Daily means without common superscript differ, P ≤ 0.05.
Discussion
The lack of effect of OGPRO on animal growth is not unexpected, given the relatively short duration of the study. Although the total VFA concentration was higher in the OGPRO group on d 66, a longer feeding period may be required to improve growth performance. Treatments did not differ in DMI during or after the starch challenge. On d 71, when the starch challenge was induced, animals had an average DMI of 9.61 kg/day, which was about 1 kg higher than their DMI before the challenge. The slight increase in DMI on d 71 was probably due to the better palatability and faster passage rate of the starch challenge diet. The reduction in DMI on d 72 may be a result of disturbed rumen function, specifically reduced ruminal pH and increased ruminal lactate on the day of the starch challenge. The possible impaired gastrointestinal tract integrity as indicated by increased plasma LBP on d 73 may also partially explain the reduction in DMI on d 72. Starch-induced SARA has been well demonstrated to reduce DMI in cattle (Gozho et al., 2006; Fairfield et al., 2007). Plaizier et al. (2008) suggested that reduction in intake during SARA could be partially attributable to increased inflammation because various studies showed increased levels of acute phase proteins in response to a SARA challenge (Gozho et al., 2006; Gozho et al., 2007).
It was suggested that the thresholds for ruminal pH indicating SARA were 5.8, 5.5, and 5.9 when rumen fluid was collected through a rumen cannula from the ventral sac, using ruminocentesis, and through an oral tubing, respectively (Plaizier et al., 2008). Because rumen fluid was collected via oral tubing, the low ruminal pH on the challenge day for the CON group (5.85) suggests a successful induction of SARA in this study. Feeding OGPRO resulted in higher butyrate production during the SARA challenge, while CON group animals had higher acetate and an accumulation of lactate in the rumen. Heifers fed OGPRO had a more consistent acetate to propionate ratio on d 66 and 73, while drastic fluctuations were observed in the CON group. Krause and Oetzel (2005) induced SARA in lactating dairy cows by supplementing the TMR diet with wheat-barley pellets. Similar to our results, they reported that the SARA challenge reduced mean ruminal pH from 6.31 to 5.85, increased the ruminal acetate to propionate ratio, and spiked mean lactate concentration from 2 to 16 mM. Our results indicate that OGPRO improved ruminal fermentation of an early lactation diet and maintained the fermentation during the starch challenge, as noted in a more stable acetate to propionate ratio and minimum production of lactate.
The elevated plasma NEFA concentration in response to the starch challenge was also reported by Brown et al. (2000), who observed increased plasma NEFA concentrations 3 and 7 days after an acidosis challenge. High blood NEFA was suggested to be an indicator of metabolic disorders and poor nutritional status, associated with immunosuppression and reduced production of lactating cows (Ingvartsen and Moyes, 2013; Benedet et al., 2020). Cortisol is a primary hormone involved in stress responses, is widely used as an indicator of stress (Wernicki et al., 2006), and can mobilize adipose tissue to release more NEFA (Sapolsky et al., 2000; Huzzey et al., 2011). The lower plasma NEFA and cortisol in heifers fed OGPRO indicated a better metabolic status than that of heifers in the CON group. In addition, the improved blood NEFA on d 73 might be partially due to a reduction in DMI on d 72, which caused a temporary negative energy balance. Feeding OGPRO maintained a stable NEFA level while its concentration spiked in the CON, suggesting improved energy balance after the starch challenge when animals were fed OGPRO. This is supported by the higher ruminal VFA concentrations observed in the OGPRO group.
Acute phase proteins are mostly produced by the liver in response to inflammatory signals (elevated cytokines) and are therefore used as biomarkers of infection and inflammation (Eckersall and Bell, 2010). Plasma haptoglobin, an acute-phase protein, is elevated in cows undergoing acute inflammatory diseases (Alsemgeest et al., 1994). The high levels of haptoglobin in CON, 2 days after the starch challenge, suggest the occurrence of inflammation in response to the starch challenge, which was prevented in animals supplemented with OGPRO. OmniGen PRO is a product formulated on the basis of OmniGen AF, and it has been reported to retain the immunomodulatory properties of OmniGen AF (Garcia et al., 2020). Thus, our findings support those of others evaluating the immunomodulatory effect of OmniGen AF in dairy cattle subjected to other stressors. Leiva et al. (2017) found that heat-stressed cows fed OmniGen AF had lower plasma haptoglobin, which was accompanied by lower rectal temperature and lower somatic cells, than heat-stressed cows not supplemented with OmniGen AF. Hall et al., 2018 reported that cows subjected to acute heat stress had elevated plasma cortisol levels but feeding OmniGen AF reduced the acute cortisol response to heat stress.
It was suggested that SARA could stimulate the lysis of ruminal Gram-negative bacteria, which releases LPS, a pro-inflammation molecule, from the cell membrane (Plaizier et al., 2008; Khafipour et al., 2009). Although we did not measure LPS in circulation, ruminal LPS can be absorbed and circulated systemically, causing an elevation of blood LBP (Ceciliani et al., 2012; Cavallini et al., 2022). Thus, the observed elevated plasma LBP in the starch challenge on d 73 was anticipated. However, it is unclear why feeding OGPRO only numerically reduced LBP level while it reduced haptoglobin and cortisol markers for stress and inflammation. A potential mechanism by which OGPRO may reduce translocation of microbes and/or their endotoxins during a SARA challenge could be the maintenance of the gastrointestinal tract integrity. In a previous study, feeding OmniGen AF to leaky gut-induced sheep helped to maintain a better gastrointestinal tract integrity, which was measured with the permeability marker Cr-EDTA (Garcia et al., 2022).
The starch challenge reduced MCHC in the CON group, which was improved by feeding OGPRO. Similar to our results, the decrease in blood MCHC following a SARA challenge was reported by Cavallini et al. (2022). MCHC is the cellular HgB measurement that measures the oxygen-carrying capacity of the red blood cells (Jones and Allison, 2007). It was suggested that animals produce fewer mature red blood cells and more immature forms during stress, and the immature forms of red blood cells, reticulocytes, contain lower hemoglobin content (Hoffman et al., 2017; Cavallini et al., 2022). In addition, inflammatory cytokines can affect the iron metabolism and function of the bone marrow, leading to an increase in the production of immature red blood cells (Jongen-Lavrencic et al., 1995; Yang et al., 2014). Although inflammatory cytokines were not directly measured in this study, we observed an increase in blood haptoglobin, an inflammation biomarker. Taken together, the reduction in MCHC after the starch challenge may be a result of stress and inflammation. The reduction in MCHC in the CON group may have been caused by an impaired capacity of the RBC to carry oxygen, which may have affected animal health and performance. Feeding OGPRO maintained the level of MCHC, which could be due to lowered stress indicated by lower blood cortisol, NEFA, and haptoglobin levels in the OGPRO group than in the CON group.
The reduction in platelets for animals in the CON group after the starch challenge was also reported by others using an LPS challenge as a model for acute inflammation in dairy cows (Horst et al., 2018; Al-Qaisi et al., 2020). In our study, OGPRO prevented the reduction of platelets after the starch challenge. Platelets are essential in driving the inflammatory response, modulating the activity of neutrophils, endothelium, and lymphocytes, and killing infected cells upon pathogen detection (Jenne et al., 2013). The higher circulating platelets in animals fed OGPRO supports an improved immune response during SARA. The tendency of higher monocytes in the OGPRO group may be due to the immunoregulatory role of OGPRO. However, it is unclear why the OGPRO and CON groups had the largest difference in monocyte counts on d 66 since animals were not undergoing any known stresses. Future studies are needed to fully understand the effects of OGPRO on the immunity and metabolism of cattle during SARA.
Conclusion
The starch challenge successfully induced SARA, metabolic, and immunologic stress as measured by ruminal fermentation, and immune and metabolic parameters. The results supported the hypotheses that OmniGen PRO improved ruminal fermentation in cattle fed early lactation diets, and reduced ruminal lactate production and blood biomarkers for stress and inflammation after a subacute acidosis challenge. More studies are needed to investigate the effects of feeding OmniGen on gastrointestinal gut integrity and the productive responses of lactating dairy cows.
Data availability statement
The raw data supporting the conclusions of this article will be made available by the authors, without undue reservation.
Ethics statement
The animal study was reviewed and approved by Animal Health Animal Care and Use Committee, Phibro Animal Health Corporation.
Author contributions
YJ, JC, BH, and MG conceptualized the original idea. YJ and MG designed the study and methodology. YJ conducted the study and data analysis. YJ wrote the initial draft with the support of MG, BH, and JC. All authors critically reviewed, revised, and approved the submitted version.
Funding
The study was funded by Phibro Animal Health Corporation. Additional funding was supported by the United States Department of Agriculture’s National Institute of Food and Agriculture Evans-Allen project KYX-10-17-61P, Accession No. 1014376.
Acknowledgments
The authors would like to thank Matthew Shaw, Elena Hass, Gary Blakeney, and Diana Roldan for their assistance in conducting the animal experiment and providing the analytical services.
Conflict of interest
Authors JC, BH, and MG are employed by Phibro Animal Health Corporation. Author YJ was employed by Phibro Animal Health Corporation.
The authors declare that this study received funding from Phibro Animal Health Corporation. The funder had the following involvement in the study: study design, data collection and analysis, and decision to publish.
Publisher’s note
All claims expressed in this article are solely those of the authors and do not necessarily represent those of their affiliated organizations, or those of the publisher, the editors and the reviewers. Any product that may be evaluated in this article, or claim that may be made by its manufacturer, is not guaranteed or endorsed by the publisher.
References
Al-Qaisi M., Kvidera S. K., Horst E. A., McCarthy C. S., Mayorga E. J., Abeyta M. A., et al. (2020). Effects of an oral supplement containing calcium and live yeast on post-absorptive metabolism, inflammation and production following intravenous lipopolysaccharide infusion in dairy cows. Res. Vet. Sci. 129, 74−81. doi: 10.1016/j.rvsc.2020.01.007
Alsemgeest S. P. M., Kalsbeek H. C., Wensing T., Koeman J. P., Van Ederen A. M., Gruys E. (1994). Concentrations of serum amyloid-a (SAA) and haptoglobin (HP) as parameters of inflammatory diseases in cattle. Vet. Q. 16, 21–23. doi: 10.1080/01652176.1994.9694410
AOAC (1980). Official methods of analysis. 13th ed (Arlington, VA: Assoc. of Official Analytical Chemists).
Benedet A., Costa A., De Marchi M., Penasa M. (2020). Heritability estimates of predicted blood β-hydroxybutyrate and nonesterified fatty acids and relationships with milk traits in early-lactation Holstein cows. J. Dairy Sci. 103, 6354−6363. doi: 10.3168/jds.2019-17916
Bergman E. N. (1990). Energy contributions of volatile fatty acids from the gastrointestinal tract in various species. Physiol. Rev. 70, 567−590. doi: 10.1152/physrev.1990.70.2.567
Briggs N. G., Silva B. C., Godoi L. A., Schoonmaker J. P. (2021). Effect of aspirin to intentionally induce leaky gut on performance, inflammation, and carcass characteristics of feedlot cattle. J. Anim. Sci. 99 (11), skab328. doi: 10.1093/jas/skab328
Brown M. S., Krehbiel C. R., Galyean M. L., Remmenga M. D., Peters J. P., Hibbard B., et al. (2000). Evaluation of models of acute and subacute acidosis on dry matter intake, ruminal fermentation, blood chemistry, and endocrine profiles of beef steers. J. Anim. Sci. 78, 3155−3168. doi: 10.2527/2000.78123155x
Burhans W. S., Burhans C. R., Baumgard L. H. (2022). Invited review: lethal heat stress: the putative pathophysiology of a deadly disorder in dairy cattle. J. Dairy Sci. 105 (5), 3716−3735. doi: 10.3168/jds.2021-21080
Canale A., Valente M. E., Ciotti A. (1984). Determination of volatile carboxylic acids (C1–C5i) and lactic acid in aqueous acid extracts of silage by high performance liquid chromatography. J. Sci. Food Agric. 35 (11), 1178−1182. doi: 10.1002/jsfa.2740351106
Cavallini D., Mammi L. M., Palmonari A., García-González R., Chapman J. D., McLean D. J., et al. (2022). Effect of an immunomodulatory feed additive in mitigating the stress responses in lactating dairy cows to a high concentrate diet challenge. Animals. 12 (16), 2129. doi: 10.3390/ani12162129
Ceciliani F., Ceron J. J., Eckersall P. D., Sauerwein H. (2012). Acute phase proteins in ruminants. J. Proteom 75, 4207−4231. doi: 10.1016/j.jprot.2012.04.004
Eckersall P. D., Bell R. (2010). Acute phase proteins: biomarkers of infection and inflammation in veterinary medicine. Veterinary J. 185, 23−27. doi: 10.1016/j.tvjl.2010.04.009
Fairfield A. M., Plaizier J. C., Duffield T. F., Lindinger M. I., Bagg R., Dick P., et al. (2007). Effects of prepartum administration of a monensin controlled release capsule on rumen pH, feed intake, and milk production of transition dairy cows. J. Dairy Sci. 90, 937−945. doi: 10.3168/jds.S0022-0302(07)71577-1
Garcia M., Jiang Y., Chapman J. D., Humphrey B. D. (2022). Effect of feed restriction and an immunomodulatory feed additive in performance, immune markers, and gut barrier integrity in wethers. J. Dairy Sci. 105 (E-Suppl 1), 305.
Garcia M., Roberts H. A., Armstrong S. A., Chapman J. D., McLean D. J. (2020). OmniGen-AF and OmniGen pro improve immunocompetence of ewes subjected to dexamethasone-induced immunosuppression. J. Dairy Sci. 103 (E-Suppl 1), 176.
Garrett E. F., Nordlund K. V., Goodger W. J., Oetzel G. R. (1997). A cross-sectional field study investigating the effect of periparturient dietary management on ruminal pH in early lactation dairy cows. J. Dairy Sci. 80 (Suppl. 1), 169. doi: 10.3168/jds.s0022-0302(06)72487-0
Gozho G. N., Krause D. O., Plaizier J. C. (2006). Effects of gradual adaptation to concentrate and subsequent induction of subacute ruminal acidosis in steers on ruminal lipopolysaccharide and acute phase proteins. J. Dairy Sci. 89, 4404−4413. doi: 10.3168/jds.s0022-0302(06)72487-0
Gozho G. N., Krause D. O., Plaizier J. C. (2007). Ruminal lipopolysaccharide concentration and inflammatory response during grain-induced subacute ruminal acidosis in dairy cows. J. Dairy Sci. 90, 856–866. doi: 10.3168/jds.S0022-0302(07)71569-2
Hall L. W., Villar F., Chapman J. D., McLean D. J., Long N. M., Xiao Y., et al. (2018). An evaluation of an immunomodulatory feed ingredient in heat-stressed lactating Holstein cows: effects on hormonal, physiological, and production responses. J. Dairy Sci. 101, 7095−7105. doi: 10.3168/jds.2017-14210
Hoffman R., Benz E. J., Silberstein L. E., Heslop H. E., Weitz J. I., Anastasi J., et al. (2017). Hematology: basic principles and practice (Philadelphia: Elsevier Inc). doi: 10.1016/C2013-0-23355-9
Hong H., Lee E., Lee I. H., Lee S. R. (2019). Effects of transport stress on physiological responses and milk production in lactating dairy cows. Asian-australas. J. Anim. Sci. 32, 442. doi: 10.5713/ajas.18.0108
Horst E. A., Kvidera S. K., Mayorga E. J., Shouse C. S., Al-Qaisi M., Dickson M. J., et al. (2018). Effect of chromium on bioenergetics and leukocyte dynamics following immunoactivation in lactating Holstein cows. J. Dairy Sci. 101, 5515−5530. doi: 10.3168/jds.2017-13899
Huzzey J. M., Nydam D. V., Grant R. J., Overton T. R. (2011). Associations of prepartum plasma cortisol, haptoglobin, fecal cortisol metabolites, and nonesterified fatty acids with postpartum health status in Holstein dairy cows. J. Dairy Sci. 94, 5878−5889. doi: 10.3168/jds.2010-3391
Ingvartsen K. L., Moyes K. (2013). Nutrition, immune function and health of dairy cattle. Animal 7 (Suppl. 1), 112−122. doi: 10.1017/s175173111200170x
Jenne C. N., Urrutia R., Kubes P. (2013). Platelets: bridging hemostasis, inflammation, and immunity. Int. J. Lab. Hematol. 35, 254−261. doi: 10.1111/ijlh.12084
Jones M. L., Allison R. W. (2007). Evaluation of the ruminant complete blood cell count. Vet. Clin. North Am. Food Anim. Pract. 23, 377−402. doi: 10.1016/j.cvfa.2007.07.002
Jongen-Lavrencic M., Peeters H. R. M., Vreugdenhil G., Swaak A. J. G. (1995). Interaction of inflammatory cytokines and erythropoeitin in iron metabolism and erythropoiesis in anaemia of chronic disease. Clin. Rheumatol. 14, 519−525. doi: 10.1007/BF02208148
Khafipour E., Krause D. O., Plaizier J. C. (2009). A grain-based subacute ruminal acidosis challenge causes translocation of lipopolysaccharide and triggers inflammation. J. Dairy Sci. 92, 1060–1070. doi: 10.3168/jds.2008-1389
Krause K. M., Oetzel G. R. (2005). Inducing subacute ruminal acidosis in lactating dairy cows. J. Dairy Sci. 88, 3633–3639. doi: 10.3168/jds.S0022-0302(05)73048-4
Leiva T., Cooke R. F., Brandão A. P., Schubach K. M., Batista L. F. D., Miranda M. F., et al. (2017). Supplementing an immunomodulatory feed ingredient to modulate thermoregulation, physiologic, and production responses in lactating dairy cows under heat stress conditions. J. Dairy Sci. 100, 4829–4838. doi: 10.3168/jds.2016-12258
Oetzel G. R., Nordlund K. V., Garrett E. F. (1999). Effect of ruminal pH and stage of lactation on ruminal lactate concentrations in dairy cows. J. Dairy Sci. 82 (Suppl. 1), 38.
Peters J., Combs S., Hoskins B., Jarman J., Kovar J., Watson M., et al. (2003) Recommended methods of manure analysis (Madison, WI: University of Wisconsin Cooperative Extension Publishing). Available at: https://soilsextension.webhosting.cals.wisc.edu/wp-content/uploads/sites/68/2014/02/Manure_Analysis_Methods.pdf (Accessed 18, 2023).
Plaizier J. C., Krause D. O., Gozho G. N., McBride B. W. (2008). Subacute ruminal acidosis in dairy cows: the physiological causes, incidence and consequences. Veterinary J. 176, 21−31. doi: 10.1016/j.tvjl.2007.12.016
Sapolsky R. M., Romero L. M., Munck A. U. (2000). How do glucocorticoids influence stress responses? integrating permissive, suppressive, stimulatory, and preparative actions. Endocr. Rev. 21, 55−89. doi: 10.1210/edrv.21.1.0389
Stone W. C. (2004). Nutritional approaches to minimize subacute ruminal acidosis and laminitis in dairy cattle. J. Dairy Sci. 87, E13−E26. doi: 10.3168/jds.s0022-0302(04)70057-0
Wernicki A., Urban-Chmiel R., Kankofer M., Mikucki P., Puchalski A., Tokarzewski S. (2006). Evaluation of plasma cortisol and TBARS levels in calves after short-term transportation. Rev. Médecine Vétérinaire. 157, 30.
Keywords: dairy cattle, subacute ruminal acidosis, rumen fermentation, inflammation, stress
Citation: Jiang Y, Chapman JD, Humphrey B and Garcia M (2023) Effects of OmniGen® PRO on ruminal fermentation, stress, and inflammation of Holstein cattle induced with a subacute ruminal acidosis. Front. Anim. Sci. 4:1202609. doi: 10.3389/fanim.2023.1202609
Received: 08 April 2023; Accepted: 21 June 2023;
Published: 26 July 2023.
Edited by:
Peter Erickson, University of New Hampshire, United StatesReviewed by:
Vincenzo Lopreiato, University of Messina, ItalySarah Allen, University of New Hampshire, United States
Copyright © 2023 Jiang, Chapman, Humphrey and Garcia. This is an open-access article distributed under the terms of the Creative Commons Attribution License (CC BY). The use, distribution or reproduction in other forums is permitted, provided the original author(s) and the copyright owner(s) are credited and that the original publication in this journal is cited, in accordance with accepted academic practice. No use, distribution or reproduction is permitted which does not comply with these terms.
*Correspondence: Yun Jiang, yun.jiang@kysu.edu; Miriam Garcia, miriam.garcia@pahc.com