- 1Department of Animal Science, University of Tennessee, Knoxville, TN, United States
- 2Department of Animal Science, South Dakota State University, Brookings, SD, United States
- 3College of Agriculture, Arkansas State University, Jonesboro, AR, United States
- 4Department of Animal Science, Texas A&M University, College Station, College Station, TX, United States
- 5Texas A&M AgriLife Research and Extension Center, Overton, TX, United States
- 6Division of Animal Sciences, University of Missouri, Columbia, MO, United States
- 7Department of Animals and Dairy Science, University of Wisconsin, Madison, WI, United States
- 8Roman L. Hruska US Meat Animal Research Center, United States Department of Agriculture -Agriculture Research Services (USDA-ARS), Clay Center, NE, United States†
Introduction: Sperm interacts with the female reproductive tract and oocyte through proteins, and these cell-to-cell interactions may play a role in sperm fertility. For consideration of a protein as a potential marker of fertility, there must be variability expressed among animals. The proteins dystroglycan (DAG1) and plasma serine protease inhibitor (SERPINA5) have been reported to play a role in cell-to-cell interactions. Thus, the objectives of this study were to characterize the localization and abundance variability of DAG1 and SERPINA5 in bovine sperm, and to investigate the relationship of DAG1 and SERPINA5 with field fertility (i.e., sire conception rate; SCR), in vitro embryo production (IVP), and sperm parameters.
Material and methods: Dairy bulls (n = 22) were classified as high-SCR (SCR > 1.0) or low-SCR (SCR < –4.0), and good [blastocyst (BL)-by-cleavage (CL) ratio (BL/CL) > 39%] or poor (BL/CL < 38%) BL/CL. Sperm was evaluated for DAG1 and SERPINA5 immunolocalization, and concentration in two separate ejaculates. Variance between bulls compared with within bulls was evaluated using a generalized linear model (GLM) procedure. The relationship of SCR and IVP classification on DAG1 and SERPINA5 concentrations, percentage of tail labeled for SERPINA5, SCR, sperm total and progressive motility, sperm plasma membrane integrity (PMI), CL, BL, and BL/CL were evaluated with the GLIMMIX procedure, and the correlations between these variables were evaluated.
Results: Both proteins were localized on the sperm head; however, SERPINA5 was also localized on the sperm tail. There was greater variance in concentration among bulls than within bulls for DAG1 (P < 0.0001; 69.4 vs. 49.1, respectively) and SERPINA5 (P < 0.0001; 325.8 vs. 285.4, respectively). There was a positive correlation between the concentrations of DAG1 and of SERPINA5 (P = 0.01; r = 0.54). In addition, the percentage of tail labeled for SERPINA5 was correlated with PMI (P = 0.05; r = 0.44). There was no relationship between SCR and IVP classifications and DAG1 (P ≥ 0.55), SERPINA5 (P ≥ 0.54), or the percentage of sperm tail labeled for SERPINA5 (P ≥ 0.22).
Discussion: In conclusion, DAG1 and SERPINA5 were localized to the sperm head, and SERPINA 5 was also localized to the tail. Concentrations of DAG1 and SERPINA5 on the sperm head were correlated with each other. The percentage of tail labeled for SERPINA5 was correlated with sperm PMI; however, neither protein was associated with SCR or IVP. Thus, when evaluated by immunofluorescent microscopy, DAG1 and SERPINA5 concentrations are variable and are not good fertility markers for bull sperm.
1 Introduction
Bulls have a greater impact on the genetic gain of a herd than any single cow because of the large number of cows artificially inseminated (AI) or serviced by an individual bull compared with a single offspring generated by a cow each year. Therefore, it is important to study the impact of male fertility on cattle operations. Thus, the identification of seminal traits that can be predictors of a bull’s fertility is of great importance. After differentiation, sperm lose the ability to grow, divide, repair, and synthesize proteins (Hammerstedt, 1993). Although the metabolic function of sperm may be limited, it is heavily regulated by proteins (Talluri et al., 2022) and its environment (Zoca et al., 2022a). After spermiation, sperm travel through the testis tubules into the epididymis (where further maturation occurs) and are stored in the epididymis tail in a quiescent state until ejaculation (Acott and Carr, 1984; Carr and Acott, 1984; Barth and Oko, 1989). On ejaculation, epididymal sperm are diluted with seminal plasma from accessory sex glands and motility is initiated (Acott and Carr, 1984; Carr and Acott, 1984). Following natural service (Hunter and Wilmut, 1984; Wilmut and Hunter, 1984; Lefebvre et al., 1995) or AI (Mitchell et al., 1985. Suarez et al., 1997), sperm with fertilizing ability reach the oviduct approximately 6–12 h after insemination, populate the isthmus portion of the oviduct, and form the sperm reservoir. Sperm that bind to oviductal cells in vitro have prolonged motility and fertilization ability (≈ 30 h) compared with sperm free in the media (Pollard et al., 1991).
Cell-to-cell interactions (i.e., sperm to oviduct and sperm to oocyte) are mediated through proteins; therefore, these interactions are important for successful fertilization. The sperm’s apical surface binds to the oviductal isthmus and ampullary ciliated cells (Pollard et al., 1991; Lefebvre et al., 1995) and binder of sperm proteins (BSP) has been reported to be involved in sperm reservoir formation (Ignotz et al., 2001; Gwathmey et al., 2003; Gwathmey et al., 2006). There are few proteins known to be required for fertilization, and these include CD9 (Kaji et al., 2000; Le Naour et al., 2000; Miyado et al., 2000) and JUNO (Bianchi et al., 2014) on the egg, and IZUMO1 in the sperm (Inoue et al., 2005). Other proteins have been identified as being associated with mammalian fertility but are not required (reviewed by Sutovsky, 2009).
Both DAG1 and SERPINA5 were present and loosely attached to the ejaculated sperm of bulls, but they were not present on the epididymal sperm, although SERPINA5 was present in both epididymal fluid and seminal plasma (increased abundance in seminal plasma) and DAG1 was present in seminal plasma (Zoca et al., 2022a; Zoca et al., 2022b). Furthermore, the function of both proteins is associated with cell-to-cell interactions. More specifically, the DAG1 gene encodes the dystroglycan precursor that generates two proteins, alpha- and beta-dystroglycan, through posttranslational modification. Alpha-dystroglycan is an extracellular/surface protein and beta-dystroglycan is a transmembrane protein (Ibraghimov-Beskrovnaya et al., 1992). In humans, the presence of DAG1 has been reported in seminal plasma but not on sperm (Jodar et al., 2016). Beta-dystroglycan has been reported to be localized to the tail middle piece of guinea-pig sperm (Hernández-González et al., 2001) and the post-acrosomal region and middle piece of mouse sperm (Hernández-González et al., 2005). The gene SERPINA5 encodes the plasma serine protease inhibitor. This protein is also known as serpin family A member 5, protein C inhibitor, and others. The presence of the SERPINA5 protein has been reported in many body fluids, including plasma (blood), seminal plasma, follicular fluid, amniotic fluid, and milk (Laurell et al., 1992). In knockout mice for SERPINA5, females were fertile and males were infertile in both in vitro (0.5% pregnancy) and in vivo (0% pregnancy) experiments. Also, sperm motility (12.5% motility) and the percentage of morphologically normal sperm (5% normal morphology) were decreased in knockout mice (Uhrin et al., 2000). Similarly, SERPINA5 concentrations were decreased in normozoospermic infertile men compared with normozoospermic fertile men (Panner Selvam et al., 2019). Nevertheless, in men, SERPINA5 has been localized to the sperm head (Zheng et al., 1994; Elisen et al., 1998). In bovines, the localization of SERPINA5 and DAG1 is still uncertain, and the function of DAG1 on sperm is not well understood. Furthermore, the impact of the abundance of these proteins on bull fertility has not been evaluated. It was hypothesized that both proteins would be associated with bull fertility and that bulls with greater concentrations would have both greater field and in vitro fertility. Thus, the first objective of this study was to characterize DAG1 and SERPINA5 immunolocalization on bovine sperm and their potential as fertility markers by evaluating variability within and among bulls. The second objective was to investigate the relationship of DAG1 and SERPINA5 with field fertility [i.e., sire conception rate (SCR)], in vitro fertility [in vitro embryo production (IVP)], and sperm parameters.
2 Materials and methods
2.1 Experimental design
Dairy bulls (n = 22) with different SCR values, ranging from –7.7 to 4.45, were classified as high (high-SCR < 1.0; n = 11) or low (low-SCR < –4.0; n = 11) field fertility (Table 1). Semen from two ejaculates (140 ± 278 days between ejaculates) was used to assess presence, localization, and sperm-relative concentrations (fluorescence intensity) of DAG1 and SERPINA5. Also, total motility (TMOT) and progressive motility (PROG) were assessed with a computer-assisted sperm analysis system (CASA; IVOS II; Hamilton Thorne, Beverly, MA, USA) and plasma membrane integrity (PMI; n = 20; semen of two bulls had already been processed before PMI could be assessed) was assessed with a dual-fluorescence stain using a Nikon fluorescence microscope. Semen was used for IVP (n = 19; one high-SCR and two low-SCR bulls’ semen was not available for IVP). Based on the IVP results of cleavage rate (CL) and blastocyst rate (BL) bulls were further classified according to the BL/CL ratio as good (BL/CL < 39%) or poor (BL/CL < 38%) embryo producers (Table 1).
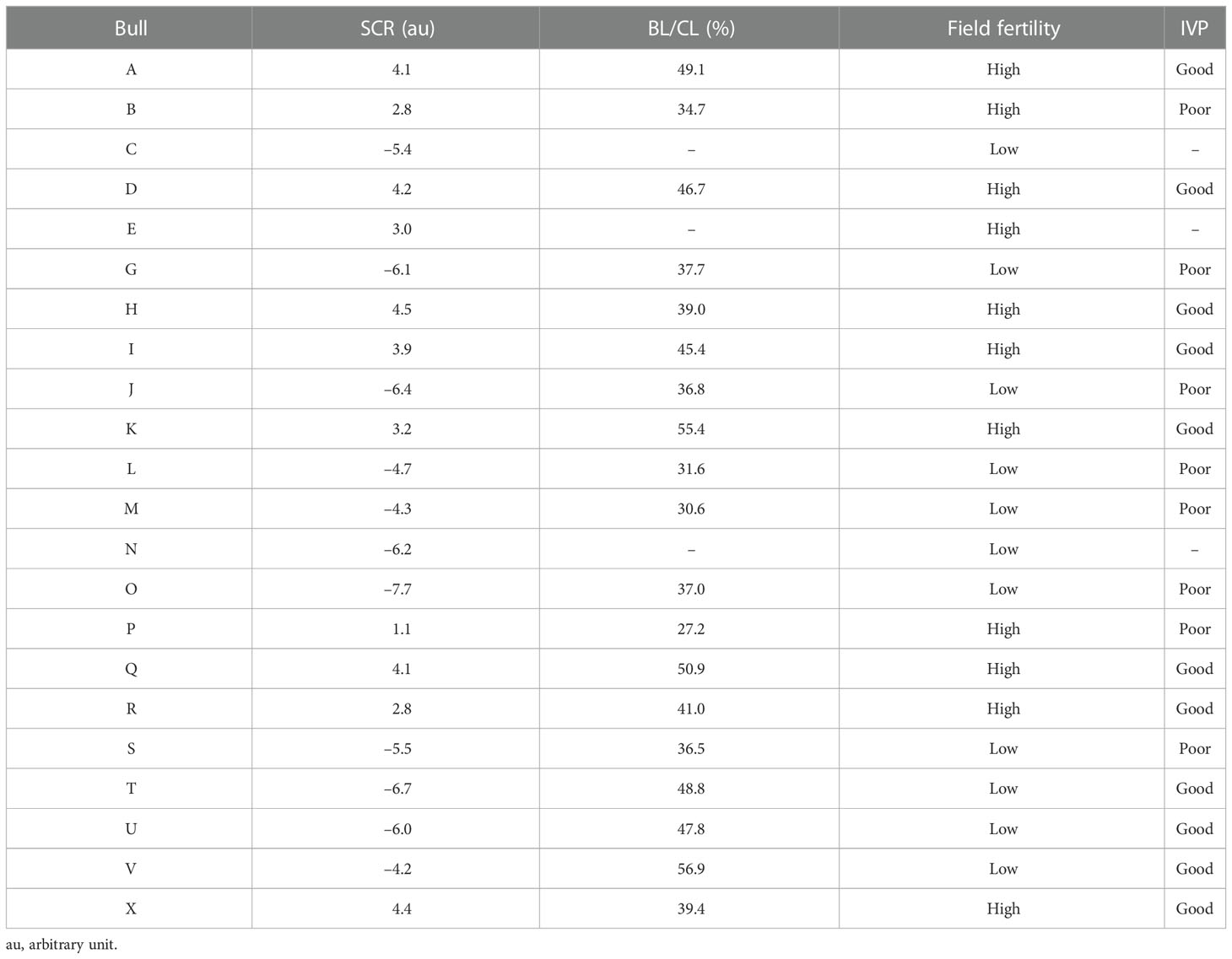
Table 1 Description of sire conception rate (SCR), blastocyst-by-cleavage rate ratio (BL/CL), field fertility classification based on SCR value (high—SCR > 1.0; low—SCR < –4.0), and in vitro embryo production (IVP) classification based on BL/CL ratio (good—BL/CL > 39%; poor—BL/CL < 38%) per bull.
2.2 Sperm motility and plasma membrane integrity analyses
Sperm motility analyses were performed using a CASA. Briefly, an aliquot of frozen-thawed semen was diluted in easy buffer B (IMV Technologies, Brooklyn Park, MN, USA) and incubated with Hoechst 33342 (final concentration 40 µg/mL) at 37°C for 10 min. After incubation, samples were loaded onto a Leja® slide (IMV Technologies) and evaluated for sperm TMOT and PROG. Sperm plasma membrane integrity was performed by the addition of 2 µL of propidium iodide after CASA analysis, and incubated for 5 min. One hundred sperm per sample in a minimum of five fields of view, avoiding the edge of the slide, were evaluated on a Nikon fluorescence microscope (inverted microscope Diaphot, TMD; Nikon Co., Tokyo, Japan) at 200× magnification.
2.3 Sperm protein analyses
2.3.1 Sperm fixation procedure
An aliquot of frozen-thawed semen samples was fixed in a 2% formaldehyde solution [10% formaldehyde (EM grade) diluted with phosphate-buffered saline (PBS)] at room temperature for 40 min (100 µL of 2% formaldehyde solution per ≈ 450 µL of extended semen). Following incubation, samples were washed by centrifugation twice at 500 × g for 5 min, the supernatant was removed with a glass Pasteur pipette, and the sperm pellet was resuspended with PBS. Samples were diluted to 5 million sperm per mL and stored at 4°C until analyzed for DAG1 and SERPINA5.
2.3.2 Sperm DAG1 analysis
An anti-DAG1 antibody (goat anti-human, ab136665, polyclonal; ABCAM, Cambridge, MA, USA) was purified using a 10-kD spin column (ab93349; ABCAM). Briefly, 135 µL of anti-DAG1 was diluted with 300 µL of PBS, added to the 10-kD spin column and centrifuged at 10,000 × g for 10 min. The purified antibody was resuspended in PBS to a final volume of 135 µL. The anti-DAG1 was conjugated to PE/R-Phycoerythrin (ab102918; ABCAM) according to the manufacturer’s instructions and diluted with PBS to a final concentration of 0.05 µg/µL. The anti-DAG1 (5 µL) and the fixed sperm (100 µL at 5 × 106 sperm per mL) were incubated in a 0.5-mL tube for 4 h at room temperature without exposure to light. After incubation, an antibody reaction was stopped by the addition of 100 µL of 2% formaldehyde solution and incubated for 40 min without exposure to light. Samples were centrifuged at 700 × g for 10 min, the supernatant was removed, and the pellet was resuspended with PBS (200 µL) and centrifuged. After the second centrifugation, the supernatant was removed and approximately 20 µL of fluid was remaining and 5 µL of ProLong Diamond Antifade Mountant (P36965; Thermo Fisher Scientific, Waltham, MA, USA) was added. Samples were mounted on a slide with a coverslip and evaluated using a Nikon fluorescence microscope at 400× magnification. A color camera (Nikon DS-Fi3) was used for picture acquisition with an exposure of 700 ms and a gain of 11.4. The NIS-Elements software package was used to outline 100 individual spermatozoa per sample with an elliptical region of interest (ROI) of approximately 65 µm2 and relative concentration was determined. In addition, immunolocalization of DAG1 on the sperm was determined.
2.3.3 Sperm SERPINA5 analysis
An anti-SERPINA5 antibody (rabbit anti-human, -mouse, -rat, PA579976, polyclonal; Invitrogen, Waltham, MA, USA) was conjugated to Dylight 405 Fast (ab201798; ABCAM) according to the manufacturer’s instructions and diluted with PBS to a final concentration of 0.1 µg/µL. The anti-SERPINA5 (5 µL) and the fixed sperm (100 µL at 5 × 106 sperm per mL) were incubated in a 0.5-mL tube for 4 h at room temperature without exposure to light. Samples were evaluated as described for DAG1 for relative concentration and immunolocalization. Exposure was set to 500 ms and gain to 11.4.
2.4 In vitro embryo production
All media and procedures for IVP followed previously published methods (Ortega et al., 2016; Ortega et al., 2018; Tríbulo et al., 2019; Stoecklein et al., 2021). Briefly, cumulus–oocyte complexes (COCs) were retrieved from ovaries collected at a commercial abattoir. Cumulus–oocyte complexes with at least three layers of compact cumulus cells and homogeneous cytoplasm were placed in groups of approximately 50 COCs into 2-mL glass sterile vials containing 1 mL of an oocyte maturation medium equilibrated with air containing 5% (v/v; volume to volume) CO2 covered with mineral oil. Tubes with COCs were shipped overnight in a portable incubator (Minitube USA Inc., Verona, WI, USA) at 38.5°C to the University of Missouri. After approximately 24 h of maturation, groups of 100 COCs were washed three times in HEPES-Tyrode’s albumen lactate pyruvate (HEPES-TALP) medium and placed in a 35-mm dish containing 1.7 mL of fertilization media (IVF-TALP). Each group of COCs was fertilized with sperm from a single bull. Sperm were purified from frozen-thawed straws using a gradient of ISolate® [50% (v/v) and 90% (v/v); Irvine Scientific, Santa Ana, CA, USA], washed two times by centrifugation at 100 × g using HEPES-TALP, and diluted in IVF-TALP to achieve a final concentration of 1 × 106 sperm per mL in the fertilization dish. To improve sperm motility and promote fertilization, 80 µL of a penicillamine–hypotaurine–epinephrine solution was added to each fertilization dish. Fertilization proceeded for approximately 18 h at 38.5°C in a humidified atmosphere of 5% (v/v) CO2. Putative zygotes (oocytes exposed to sperm) were vortexed for 5 min in 400 µL of HEPES-TALP to denude from the surrounding cumulus cells at the end of fertilization. Embryos were then cultured in four-well dishes in groups of up to 50 embryos in 500 µL of culture medium (SOF-BE2) covered with 300 µL of mineral oil per well at 38.5°C in a humidified atmosphere of 5% (v/v) O2 and 5% (v/v) CO2. The percentage of putative zygotes that were cleaved (CL) was determined at day 3 of development (day 0 = day of insemination) and blastocyst formation (BL) at day 8 of development.
2.5 Statistical analysis
The relative concentration of proteins (SERPINA5 and DAG1) was analyzed using the generalized linear model (GLM) procedure in SAS (9.4) with bull as a fixed effect to determine the variance in mean protein abundance between bulls and within bulls. The CORR procedure of SAS was used to evaluate correlations between SCR, TMOT, PROG, PMI, CL, BL, BL/CL, DAG1 and SERPINA5 relative concentrations, and the proportion of sperm tail labeled for SERPINA5. The GLIMMIX procedure of SAS was used to evaluate the relationship of bull field fertility classification (high- and low-SCR) and BL/CL classification (good and poor), and their interactions (n = 19 bulls, except for PMI which n = 17) with bull as a random effect for TMOT, PROG, PMI, CL, BL, BL/CL, DAG1 and SERPINA5 relative concentrations, and also the proportion of sperm tail labeled for SERPINA5. Results are presented as least squares mean ± standard error (SE) unless otherwise stated. The level of significance was α ≤ 0.05 when the P-value was > 0.05, but when the P-value was ≤ 0.10 the results were considered as a tendency.
3 Results
Immunolocalization demonstrated that the SERPINA5 protein was present on both the sperm head (Figure 1) and the tail (Figure 1). On the sperm head, the most consistent pattern of SERPINA5 covered the proximal region over the acrosomal cap (Figure 1). There were 34.2% ± 12.7% [mean ± standard deviation (SD)] of sperm tails that were positive for SERPINA5 (ranging from 6.0% to 57.4%; Figure 2). The relative concentration of SERPINA5 on the sperm head ranged from 38.9 ± 1.1 au (arbitrary unit) to 68.4 ± 1.1 au (Figure 3) with an average of 53.2 ± 6.6 au (mean ± SD). The abundance of SERPINA5 had a greater variance among bulls than within bulls (P < 0.0001; variance 325.8 vs. 285.4, respectively). Furthermore, immunolocalization determined that DAG1 was present on the sperm head in the proximal apical region, over the acrosomal cap (Figure 4). The percentage of sperm with DAG1 present on the sperm head did not differ between treatments (P = 0.74; 32.1% ± 4.1%, 38.1% ± 3.5%, 31.5% ± 4.7%, 34.6% ± 3.4% for high-SCR/good, high-SCR/poor, low-SCR/good, and low-SCR/poor, respectively). Abundance (fluorescence insensitivity) ranged from 29.6 ± 0.5 to 45.7 ± 0.5 au (Figure 5) and averaged 36.0 ± 4.6 au (mean ± SD). There was greater variation in the abundance of DAG1 among bulls than within bulls (P < 0.0001; variance was 69.4 vs. 49.1, respectively).
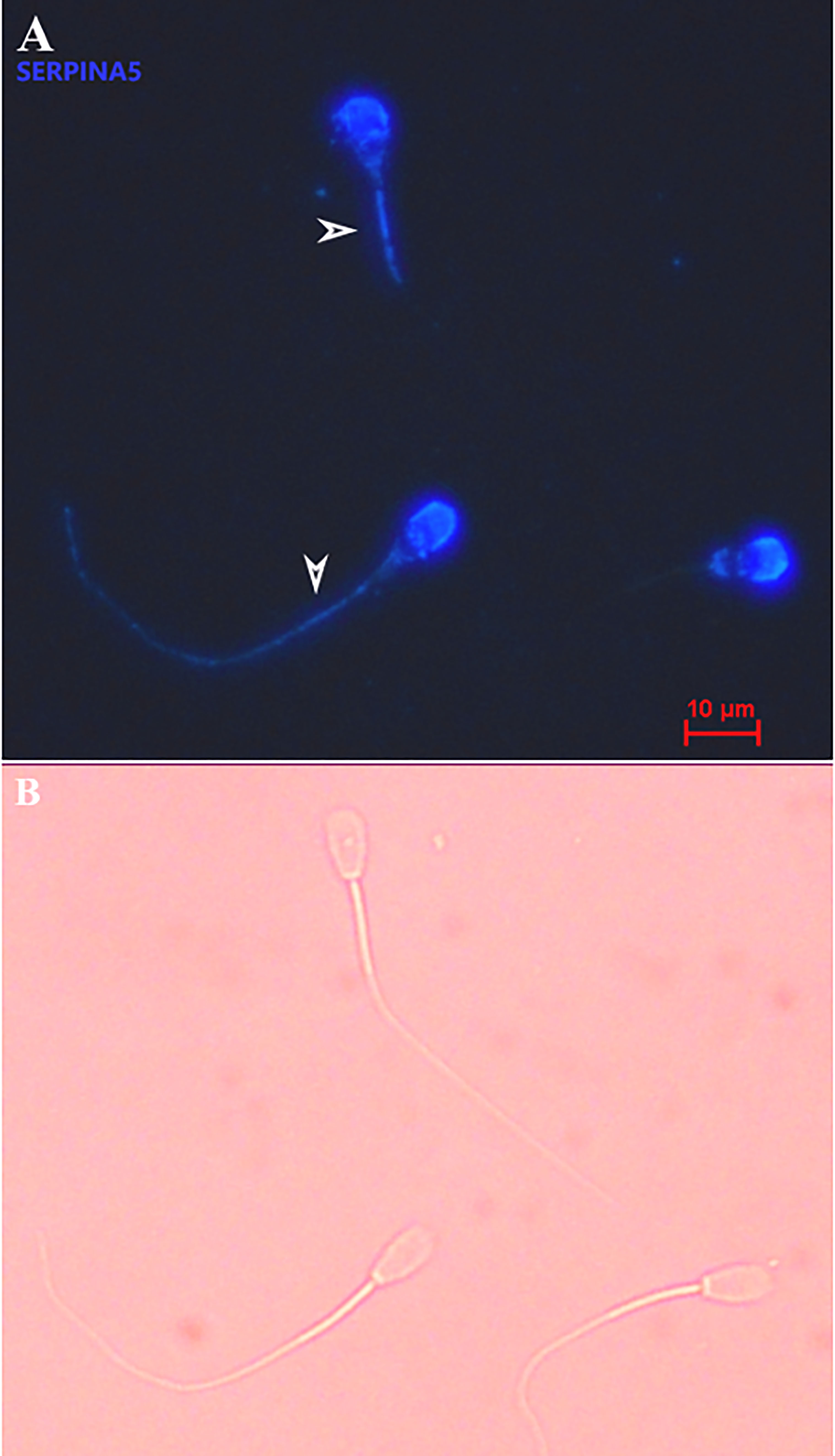
Figure 1 Representative picture of bovine sperm labeled with anti-SERPINA5 [PA579976, Invitrogen; and conjugated to Dylight 405 Fast (ab201798; ABCAM)] on the sperm head and sperm tail (A), and bright field of A (B). White arrows on panel A indicate sperm tail positive for SERPINA5. 400× magnification.
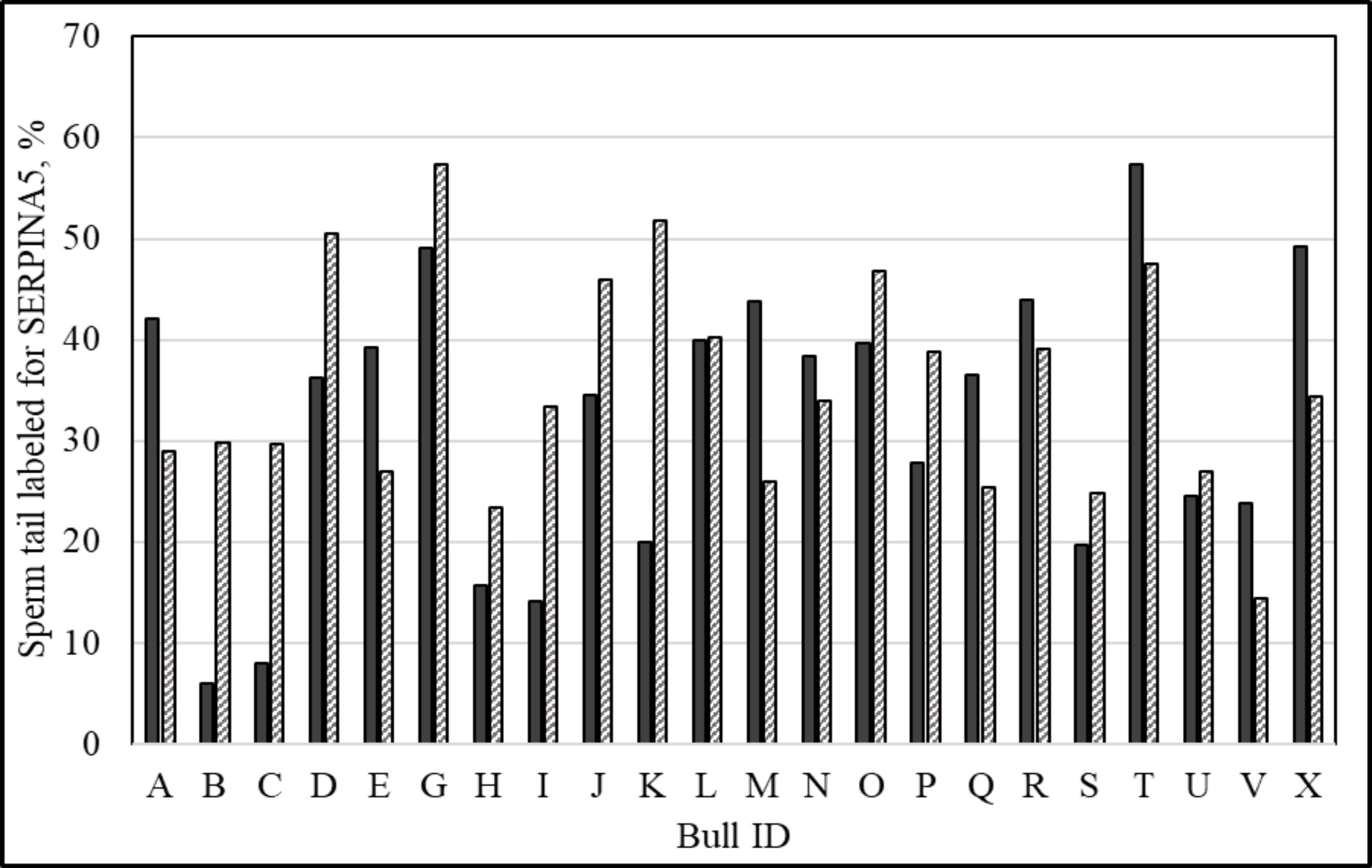
Figure 2 Percentage of sperm tail labeled with anti-SERPINA5 [PA579976, Invitrogen; and conjugated to Dylight 405 Fast (ab201798; ABCAM)] per ejaculate. Solid bars represent ejaculate 1 and dashed bars represent ejaculate 2 of the same animal (140 ± 278 days between ejaculates; mean ± SD).
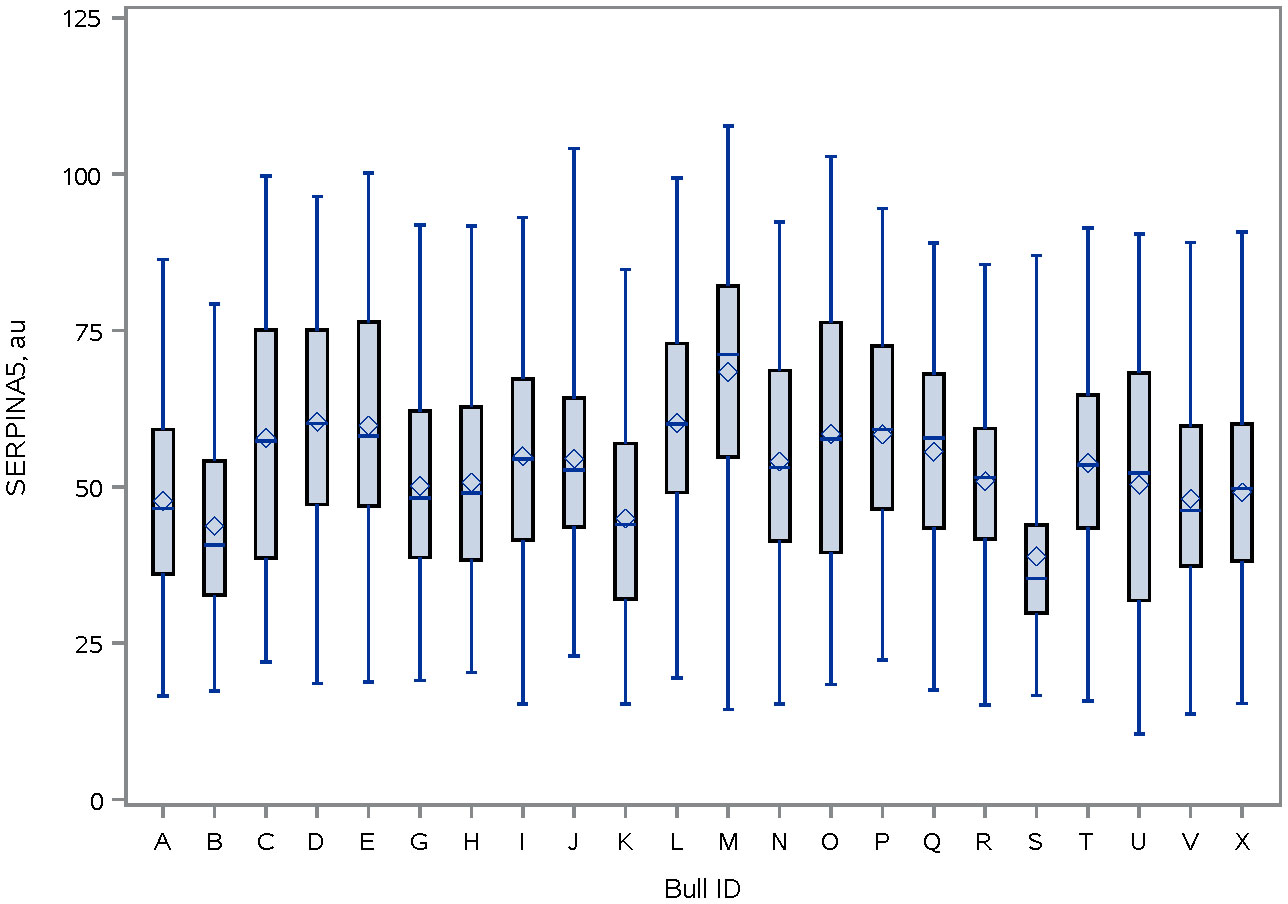
Figure 3 Distribution of SERPINA5 fluorescence intensity (PA579976, Invitrogen; and conjugated to Dylight 405 Fast (ab201798; ABCAM)] on sperm head of bulls. The line within the box represents the median and the diamond shape represents the mean. au, arbitrary unit.
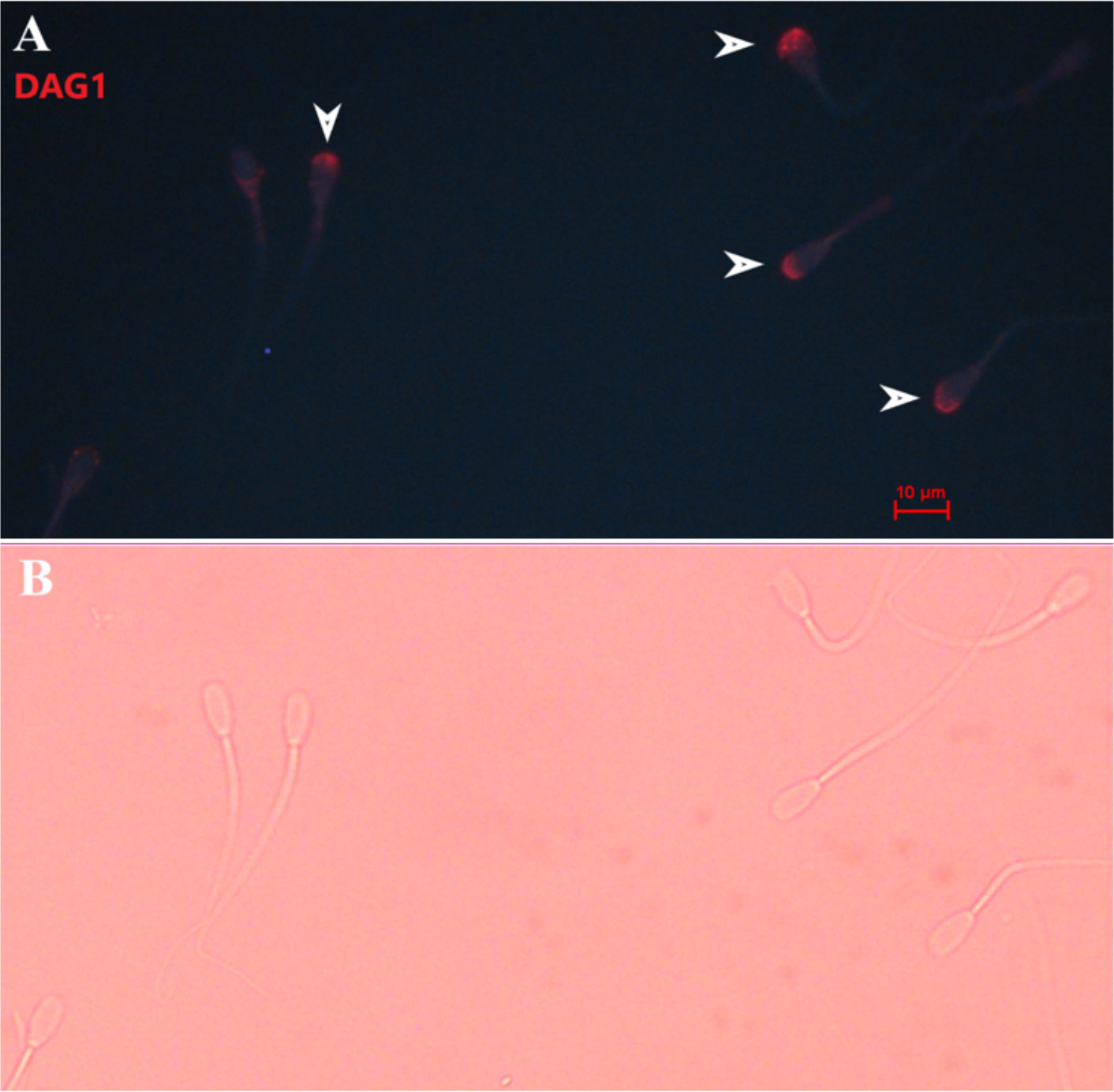
Figure 4 Representative picture of bovine sperm labeled with anti-DAG1 [ab136665, ABCAM; and conjugated to PE/R-Phycoerythrin (ab102918; ABCAM)] on the sperm head (A), and bright field of panel A (B). White arrows indicate sperm positive for DAG1. 400× magnification.
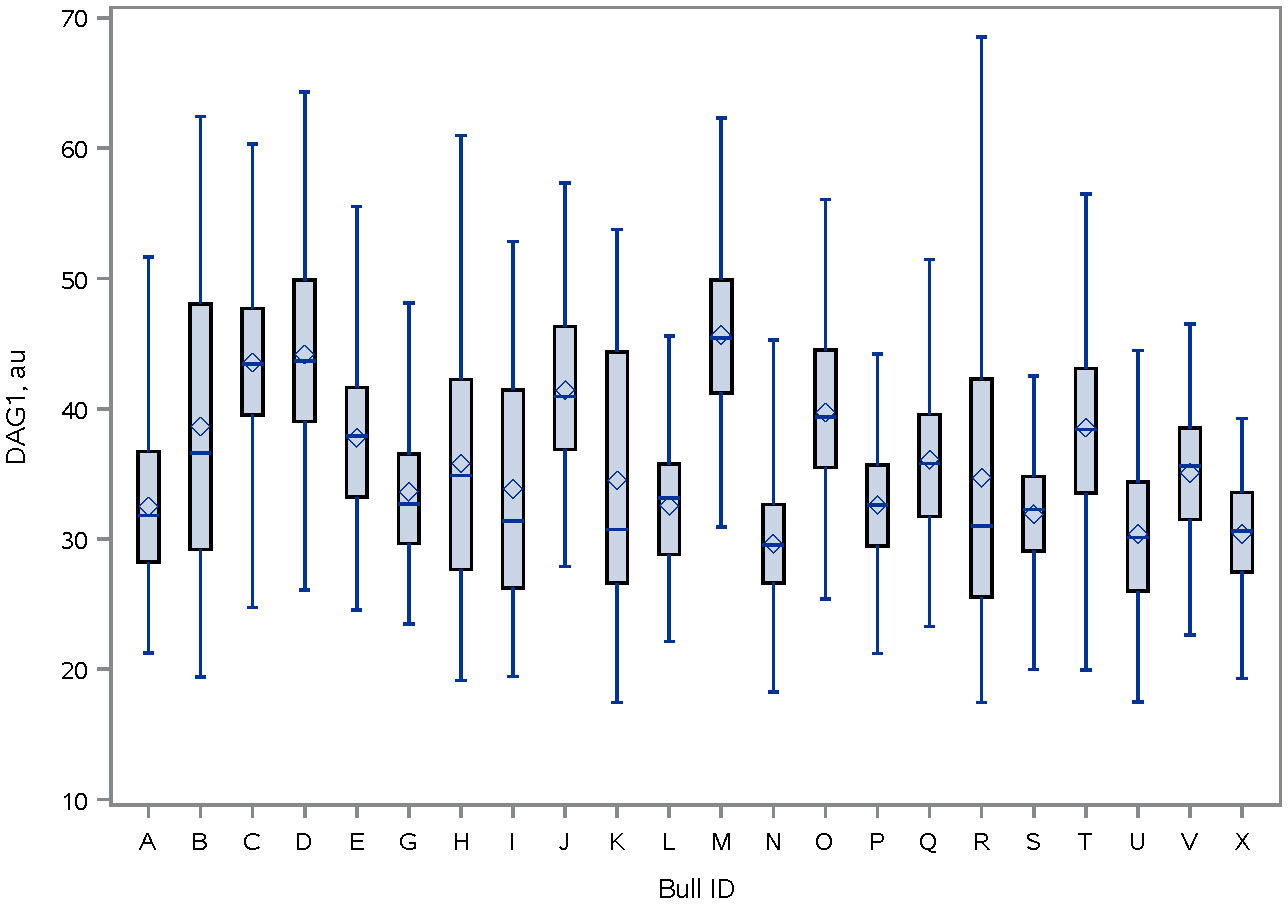
Figure 5 Distribution of DAG1 fluorescence intensity [ab136665, ABCAM; and conjugated to PE/R-Phycoerythrin (ab102918, ABCAM)] on the sperm head of bulls. The line within the box represents the median and the diamond shape represents the mean. au, arbitrary unit.
There were positive correlations between TMOT and PROG (P < 0.01; Table 2), PMI and percentage of sperm tail labeled for SERPINA5 (P = 0.05; Table 2); all other correlations were not significant (P < 0.12; Table 2). There was no SCR classification by IVP classification interaction (P < 0.12) for SCR, PROG, PMI, CL, BL, BL/CL, SERPINA5 and DAG1 concentrations, and the percentage of sperm tail labeled for SERPINA5 (Table 3); nevertheless, the interaction was significant (P = 0.02) for TMOT. Bulls classified as high-SCR and good IVP had greater TMOT than bulls classified as high-SCR and poor IVP and tended (P = 0.08) to have greater TMOT than low-SCR and good IVP; however, they were not different than low-SCR and poor IVP. In addition, low-SCR and poor IVP tended (P = 0.07) to have greater TMOT than high-SCR and poor IVP (Table 3).
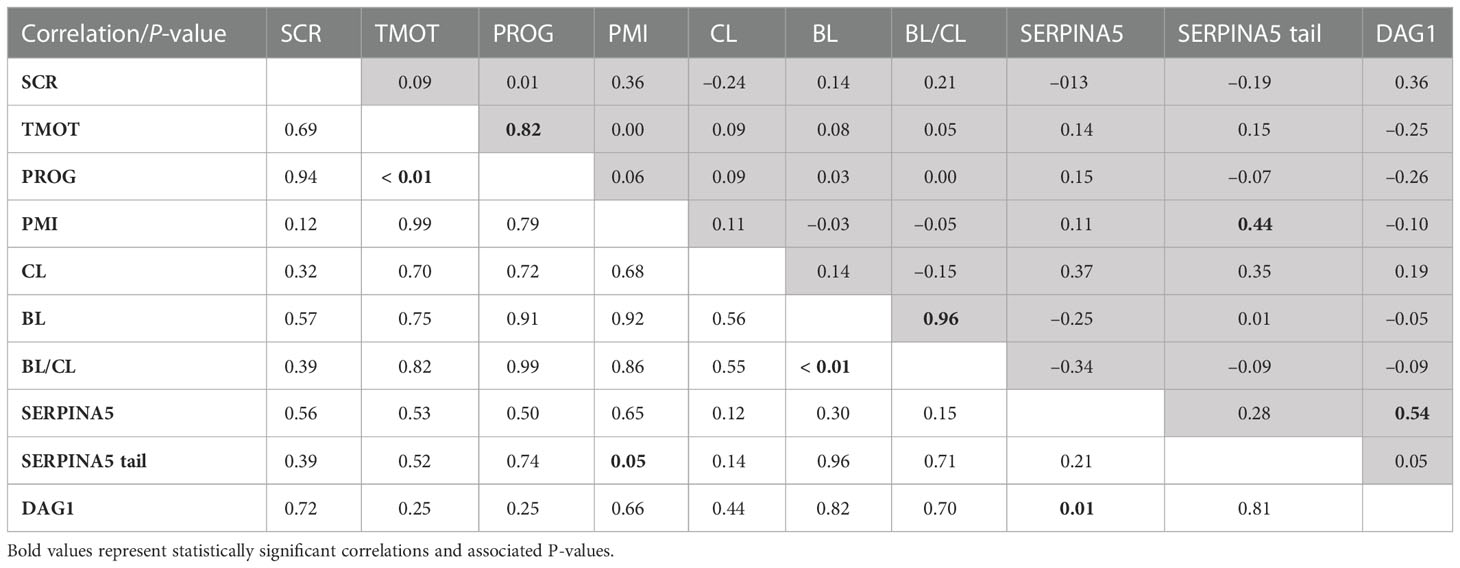
Table 2 Pearson’s correlation coefficient (shaded area above diagonal) and significance level (below diagonal) between sire conception rate (SCR), total motility (TMOT), progressive motility (PROG), sperm plasma membrane integrity (PMI), in vitro produced embryos cleavage (CL) and blastocyst (BL) rate, BL-by-CL ratio (BL/CL), SERPINA5 concentration (SERPINA5), percentage of sperm tail labeled for SERPINA5 (SERPINA5 tail), and DAG1 concentration (DAG1).
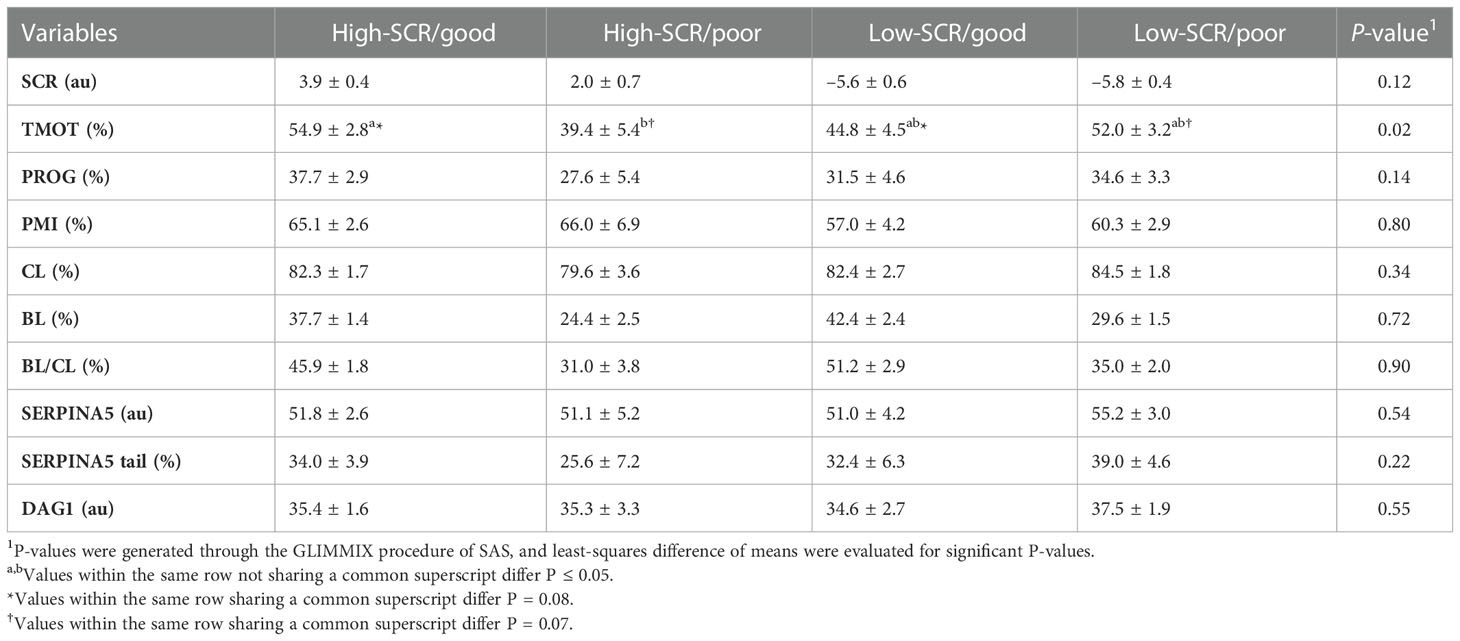
Table 3 Relationship of sire conception rate (SCR) fertility classification and in vitro embryo production fertility classification based on the blastocyst (BL) and cleavage (CL) rates ratio (BL/CL; high-SCR/good, high-SCR/poor, low-SCR/good, low-SCR/poor, respectively) on total motility (TMOT), progressive motility (PROG), sperm plasma membrane integrity (PMI), CL, BL, BL/CL, SERPINA5 concentration (SERPINA5), percentage of sperm tail labeled for SERPINA5 (SERPINA5 tail), and DAG1 concentration (DAG1).
As designed, when evaluating the SCR classification, high-SCR bulls had greater SCRs values than low-SCR bulls (P < 0.0001; Table 4). Interestingly, low-SCR bulls had greater (P = 0.03) BL production and tended (P = 0.10) to have greater BL/CL ratios than high-SCR bulls (Table 4). No other parameters evaluated were associated with SCR classification (P < 0.15; Table 4). Similarly, as designed, bulls classified as having good IVP had greater (P < 0.0001) BL/CL ratios than poor IVP bulls (Table 5). Also, good IVP bulls had greater BL production (P < 0.0001) than poor IVP bulls, but there was no difference (P = 0.96) in CL. Interestingly, good IVP bulls tended (P = 0.07) to have greater SCRs than poor IVP bulls (Table 5). No other parameters evaluated were associated with IVP classification (P < 0.34; Table 5).
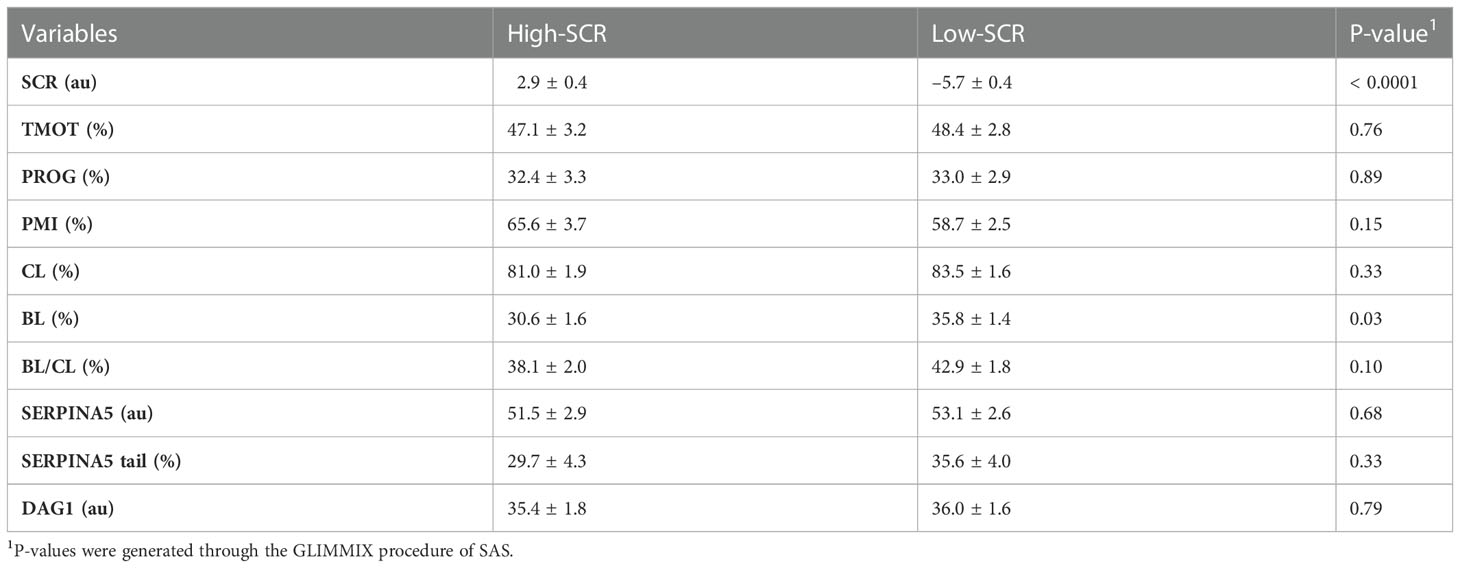
Table 4 Relationship of sire conception rate (SCR) fertility classification (high-SCR and low-SCR) on total motility (TMOT), progressive motility (PROG), sperm plasma membrane integrity (PMI), cleavage rate (CL) and blastocyst (BL) rate, BL by CL ratio (BL/CL), SERPINA5 concentration (SERPINA5), percentage of sperm tail positive for SERPINA5 (SERPINA5 tail), and DAG1 concentration (DAG1).
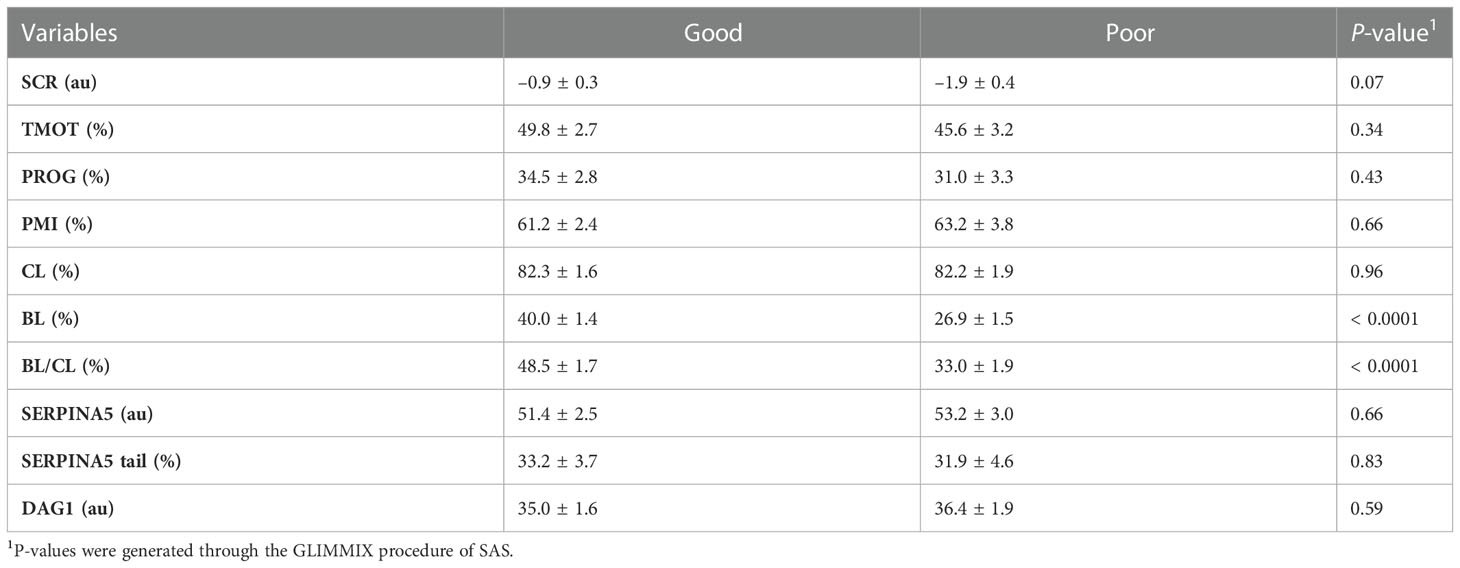
Table 5 Relationship of in vitro embryo production fertility classification based on the blastocyst (BL) and cleavage (CL) rates ratio (BL/CL; good and poor) on total motility (TMOT), progressive motility (PROG), sperm plasma membrane integrity (PMI), CL, BL, BL/CL, SERPINA5 concentration (SERPINA5), percentage of sperm tail positive for SERPINA5 (SERPINA5 Tail), and DAG1 concentration (DAG1).
4 Discussion
The rate of genetic improvement in a herd is far more efficient through bull selection than female selection owing to the larger number of offspring generated by one single bull compared with one single female. This is especially true in dairy cattle, in which 90% of females are artificially inseminated (Starbuck et al., 2004; Valour et al., 2015; García-Ruiz et al., 2016; Wiggans et al., 2017; USDA, 2018). Bull fertility, as evaluated in the AI industry, has been evaluated heavily through semen quality, which relies predominantly on sperm motility and morphology, and more recently sperm PMI (Barth and Oko, 1989; Koziol and Armstrong, 2018; DeJarnette et al., 2021). Sire conception rate is one of the most common methods of evaluation for bull field fertility. The SCR value given to a bull is generated based on field reports of pregnancies: SCR values represent the bull’s deviation in pregnancy rates at day 70 of gestation compared with the mean pregnancy rates from all other bulls that could have been used (Kuhn et al., 2006; Norman et al., 2011). It was observed that some low-SCR bulls had good BL production; interestingly, overall, low-SCR bulls had greater BL production than high-SCR bulls and tended to have greater BL/CL ratios. Furthermore, in this group of bulls, animals that were classified as good had slightly greater SCRs than those ranked as poor. Ortega et al. (2018) reported similar findings in which one (out of three) low-SCR bull had BL production rates similar to high-SCR bulls; however, overall, in their group of bulls, high-SCR bulls had greater BL production than low-SCR bulls. Sperm must endure far fewer challenges to fertilize an embryo in vitro than in vivo. In vivo, sperm must navigate the female reproductive tract, survive uterine contraction, overcome the utero–tubular junction, form the sperm reservoir, capacitate, and “find” the ovulated oocyte to then fertilize that single oocyte (Suarez, 2015; Suarez, 2016). In addition, AI may happen in different moments during estrus/pro-estrus resulting in the requirement for sperm to survive for prolonged periods of time or capacitate “quicker”, both affecting fertilization rate and embryo quality (Saacke et al., 2000; Dalton et al., 2001; Richardson et al., 2017). In contrast, in vitro, sperm must tolerate manipulation insults (Baldi et al., 2020); however, barriers in the female reproductive tract (except those from the oocyte) are eliminated. Thus, it is possible that bulls with low-SCRs but with good BL production rates have sperm transport problems or are more susceptible to the timing of insemination (i.e., sperm longevity) or the uterine/oviduct environment than low-SCR bulls with lower BL production rates, where the problem may be related to fertilization itself rather than sperm transport. This hypothesis is partially explained by the “compensable” and “uncompensable” characteristics of sperm previously reported (Saacke et al., 1994; Saacke, 2008; Amann et al., 2018). It was also observed that some high-SCR bulls had poor IVP, and a few hypotheses may be drawn from these observations: (1) these bulls could be more sensitive to laboratory manipulation procedures for IVP; (2) the female reproductive tract in vivo may be acting as a “filter” and some of the sperm with uncompensable characteristics may not reach the site of fertilization, whereas in vitro they are not being removed; (3) the ejaculates from these bulls used for IVP were not accurate representations of their field fertility (i.e., high variability between ejaculates); or (4) these bulls (with SCRs of 1.1 and 2.8) were misclassified as high-SCR.
The objective of the bovine AI industry is to provide semen of high quality to cattle producers; semen that passes quality control and is commercially available has met specific thresholds (Harstine et al., 2018; DeJarnette et al., 2021). With that, sperm motility, morphology, and PMI of commercially available semen are not expected to correlate with field fertility, especially in large samples (DeJarnette et al., 2021). The present results corroborated the industry efforts and did not identify any relationship or correlation between field fertility (SCR) or IVP classification and TMOT, PROG, or PMI. Nevertheless, an SCR by IVP classification interaction on TMOT was observed in the present study; however, differences in motility did not conclusively explain fertility differences, especially because high-SCR/good had similar motility to low-SCR/poor.
Sperm interact with the utero–tubular junction (UTJ), oviduct (formation of sperm reservoir and movement through the oviduct), and oocyte through proteins (Lefebvre et al., 1995; Gwathmey et al., 2003; Gwathmey et al., 2006; Ignotz et al., 2007; Sutovsky, 2009; Suarez, 2015; Suarez, 2016). The formation of the sperm reservoir in bovines involves BSP (Ignotz et al., 2001; Gwathmey et al., 2003; Gwathmey et al., 2006). These groups of proteins are attached to the sperm during ejaculation when epididymal sperm come into contact with seminal plasma (Desnoyers and Manjunath, 1992; Müller et al., 1998; Nauc and Manjunath, 2000); similarly, as observed by Zoca et al (2022a; 2022b), DAG1 and SERPINA5 are attached to the sperm during ejaculation, as DAG1 and SERPINA5 were not detected in epididymal sperm samples. Liquid chromatography with tandem mass spectrometry analysis (LCMS/MS) results demonstrated that DAG1 was not very abundant (spectra count 1.1), whereas SERPINA5 was highly abundant (spectra count 37.3) on the sperm (Zoca et al., 2022b). The present results (Figures 1, 3, 4, 5) agree with LCMS/MS findings that SERPINA5 is present in greater abundance on the sperm than DAG1; interestingly, DAG1 and SERPINA5 concentrations were correlated (Table 2). The localization of both DAG1 and SERPINA5 on the sperm head is interesting and consistent with the region of the sperm that attaches to oviductal epithelial cells to form the sperm reservoir (Lefebvre et al., 1995). The function of DAG1 on the sperm is not fully understood, especially alpha-dystroglycan, which is more likely than beta-dystroglycan to have been measured owing to the fact that alpha-dystroglycan is an extracellular/surface protein (Ibraghimov-Beskrovnaya et al., 1992). Beta-dystroglycan, a transmembrane protein (Ibraghimov-Beskrovnaya et al., 1992), was reported on the tail middle piece of guinea-pig sperm (Hernández-González et al., 2001) and the middle piece and acrosomal region of mice sperm (Hernández-González et al., 2005). Hernández-González et al. (2005) demonstrated that mice sperm with beta-dystroglycan deficiencies had increased morphological abnormalities in the sperm tail, and the number of sperm capable of fertilization was lower (≈ 50% less) than in sperm from wild-type mice. In the present study, DAG1 was not associated with field fertility or field and in vitro embryo fertility. Furthermore, DAG1 was not correlated with SCR, CL, or BL. Thus, it may be hypothesized that DAG1 may function to stabilize the acrosomal region as a decapacitating factor, preventing premature acrosomal reaction or formation of the sperm reservoir owing to its localization on the sperm (Figure 4); however, this study failed to identify any relationship between DAG1 concentration and bull fertility, either in vivo or in vitro.
The abundance of SERPINA5 in the seminal plasma and loosely attached to the sperm ranked 13th and 11th based on spectra count, respectively (Zoca et al., 2022b), which agrees with previous reports for SERPINA5 in seminal plasma (Druart et al., 2013; Kasimanickam et al., 2019). Reference for immunolocalization of SERPINA5 in bovine or other livestock species could not be found, but within human sperm, SERPINA5 was localized to the acrosomal region of epididymal and ejaculated sperm (Zheng et al., 1994; Elisen et al., 1998). There was no difference in SERPINA5 localization between capacitated and non-capacitated sperm; however, when an acrosome reaction was induced, SERPINA5 was limited to the equatorial region (Zheng et al., 1994; Elisen et al., 1998). The immunolocalization of SERPINA5 on the bovine sperm head (Figure 1) was similar to that of human sperm (Zheng et al., 1994; Elisen et al., 1998); however, bovine sperm also had SERPINA5 on the sperm tail, differing from human sperm. The protease inhibitory activity of SERPINA5 has been found in multiple body tissues and fluids (España et al., 1989; Ecke et al., 1992; Christensson and Lilja, 1994; Hermans et al., 1994; Zheng et al., 1994; Elisen et al., 1998). The activity of SERPINA5 can be modulated by heparin and other glycosaminoglycans (Kuhn et al., 1990; Pratt and Church, 1992; Ecke et al., 1997). Heparin and glycosaminoglycans are present in the oviduct from oviductal fluid and follicular fluid that have been shown to induce sperm capacitation (Parrish et al., 1985; Parrish et al., 1988; Mahmoud and Parrish, 1996; Bergqvist et al., 2007). When the SERPINA5 gene was disrupted in mice, male mice were infertile both in vitro and in vivo because of morphologically abnormal sperm, lower motility, and lack of sperm–egg binding (Uhrin et al., 2000). In addition, normozoospermic men with an unknown reason for infertility had a lower concentration of SERPINA5 than their fertile counterparts (Panner Selvam et al., 2019). The ability of human sperm to bind to the human zona pellucida was evaluated in the presence of different concentrations of anti-SERPINA5 or SERPINA5 in the media (Elisen et al., 1998). Interestingly, a lower concentration of anti-SERPINA5 increased the ability of sperm to bind to the zona pellucida; however, the greater the concentration of SERPINA5 in the media the lower the ability of sperm to bind to the zona pellucida (Elisen et al., 1998). Another member of the serine protease inhibitor (SERPIN) family, called glia-derived nexin or protease nexin-1 (SERPINE2), has been reported to be a decapacitating factor in mice (Lu et al., 2011). Controversially, there was no association of SERPINA5 concentration or percentage of tail labeled for SERPINA5 with bull fertility (SCR and IVP). The present results indicate that neither SERPINA5 nor DAG1 is a good indicator of in vivo or in vitro bull fertility.
In conclusion, by using immunofluorescent microscopy, DAG1 and SERPINA5 proteins were localized on the bovine sperm head, and SERPINA5 was also localized on the sperm tail. For a protein to be considered a marker of fertility, it is necessary to have animal variation. Also, any new test must not be correlated with current evaluations of semen quality or must provide a simpler method of evaluation over current analyses (DeJarnette, 2005; Harstine et al., 2018; DeJarnette et al., 2021). A greater variation among bulls than within bulls was observed for both DAG1 and SERPINA5, fulfilling the first characteristics for a potential fertility marker. Furthermore, when evaluated by immunofluorescent microscopy, DAG1 and SERPINA5 were not correlated with TMOT, PROG, or PMI, fulfilling the second characteristic of a potential fertility marker; however, the percentage of tails labeled for SERPINA5 was correlated with PMI. In addition, the relative concentrations of both proteins in the sperm were correlated with each other. Although fulfilling the requirements for a new fertility marker, the proteins were not associated with bull fertility. Thus, using immunofluorescent microscopy to determine SERPINA5 and DAG1 may not be a good marker of bull fertility; however, as SERPINA5 and DAG1 do have variation among animals (first criteria), increased sensitivity by evaluating a greater proportion of an ejaculate by immunofluorescent flow cytometry may impact its ability to predict bull fertility.
Data availability statement
The original contributions presented in the study are included in the article. Further inquiries can be directed to the corresponding author.
Author contributions
SZ, RC, and GP: experimental design and conceptualization. SZ, AK, TA, JR, KE, JD, MO, and GP: data collection. SZ: data management. SM, RC, and GP: statistical analyses. JW and GP: funding acquisition. SM wrote the original draft of the manuscript. All authors contributed to the article and approved the submitted version.
Funding
This project was partially funded by Multistate Hatch project 9835.
Conflict of interest
The authors declare that the research was conducted in the absence of any commercial or financial relationships that could be construed as a potential conflict of interest.
Publisher’s note
All claims expressed in this article are solely those of the authors and do not necessarily represent those of their affiliated organizations, or those of the publisher, the editors and the reviewers. Any product that may be evaluated in this article, or claim that may be made by its manufacturer, is not guaranteed or endorsed by the publisher.
References
Acott T. S., Carr D. W. (1984). Inhibition of bovine spermatozoa by caudal epididymal fluid: II. interaction of pH and a quiescence factor. Biol. Reprod. 30 (4), 926–935. doi: 10.1095/biolreprod30.4.926
Amann R. P., Saacke R. G., Barbato G. F., Waberski D. (2018). Measuring male-to-male differences in fertility or effects of semen treatments. Annu. Rev. Anim. Biosci. 6, 255–286. doi: 10.1146/annurev-animal-030117-014829
Baldi E., Tamburrino L., Muratori M., Degl’Innocenti S., Marchiani S. (2020). Adverse effects of in vitro manipulation of spermatozoa. Anim. Reprod. Sci. 220, 106314. doi: 10.1016/j.anireprosci.2020.106314
Barth A. D., Oko R. (1989). Abnormal morphology of bovine spermatozoa (Ames, IA: Iowa State University Press).
Bergqvist A.-S., Ballester J., Johannisson A., Lundeheim N., Rodríguez-Martínez H. (2007). Heparin and dermatan sulphate induced capacitation of frozen-thawed bull spermatozoa measured by merocyanine-540. Zygote 15 (3), 225–232. doi: 10.1017/S0967199407004182
Bianchi E., Doe B., Goulding D., Wright G. J. (2014). Juno Is the egg izumo receptor and is essential for mammalian fertilization. Nature 508 (7497), 483. doi: 10.1038/nature13203
Carr D. W., Acott T. S. (1984). Inhibition of bovine spermatozoa by caudal epididymal fluid: i. studies of a sperm motility quiescence factor. Biol. Reprod. 30 (4), 913–925. doi: 10.1095/biolreprod30.4.913
Christensson A., Lilja H. (1994). Complex formation between protein c inhibitor and prostate-specific antigen in vitro and in human semen. Eur. J. Biochem. 220 (1), 45–53. doi: 10.1111/j.1432-1033.1994.tb18597.x
Dalton J., Nadir S., Bame J., Noftsinger M., Nebel R., Saacke R. (2001). Effect of time of insemination on number of accessory sperm, fertilization rate, and embryo quality in nonlactating dairy cattle. J. Dairy. Sci. 84 (11), 2413–2418. doi: 10.3168/jds.S0022-0302(01)74690-5
DeJarnette J. M. (2005). The effect of semen quality on reproductive efficiency. Vet. Clinics.: Food Anim. Pract. 21 (2), 409–418. doi: 10.1016/j.cvfa.2005.02.011
DeJarnette J., Harstine B., McDonald K., Marshall C. (2021). Commercial application of flow cytometry for evaluating bull sperm. Anim. Reprod. Sci. 246, 106838. doi: 10.1016/j.anireprosci.2021.106838
Desnoyers L., Manjunath P. (1992). Major proteins of bovine seminal plasma exhibit novel interactions with phospholipid. J. Biol. Chem. 267 (14), 10149–10155. doi: 10.1016/S0021-9258(19)50212-5
Druart X., Rickard J., Mactier S., Kohnke P., Kershaw-Young C., Bathgate R., et al. (2013). Proteomic characterization and cross species comparison of mammalian seminal plasma. J. Proteomics 91, 13–22. doi: 10.1016/j.jprot.2013.05.029
Ecke S., Geiger M., Binder B. R. (1997). Heparin binding of protein-c inhibitor analysis of the effect of heparin on the interaction of protein-c inhibitor with tissue kallikrein. Eur. J. Biochem. 248 (2), 475–480. doi: 10.1111/j.1432-1033.1997.00475.x
Ecke S., Geiger M., Resch I., Jerabek I., Sting L., Maier M., et al. (1992). Inhibition of tissue kallikrein by protein c inhibitor. evidence for identity of protein c inhibitor with the kallikrein binding protein. J. Biol. Chem. 267 (10), 7048–7052. doi: 10.1016/S0021-9258(19)50534-8
Elisen M. G., van Kooij R. J., Nolte M. A., Arnoud Marquart J., Lock T. M., Bouma B. N., et al. (1998). Protein c inhibitor may modulate human sperm-oocyte interactions. Biol. Reprod. 58 (3), 670–677. doi: 10.1095/biolreprod58.3.670
España F., Berrettini M., Griffin J. H. (1989). Purification and characterization of plasma protein c inhibitor. Thromb. Res. 55 (3), 369–384. doi: 10.1016/0049-3848(89)90069-8
García-Ruiz A., Cole J. B., VanRaden P. M., Wiggans G. R., Ruiz-López F. J., Van Tassell C. P. (2016). Changes in genetic selection differentials and generation intervals in US Holstein dairy cattle as a result of genomic selection. Proc. Natl. Acad. Sci. 113 (28), E3995–E4004. doi: 10.1073/pnas.1519061113
Gwathmey T. M., Ignotz G. G., Mueller J. L., Manjunath P., Suarez S. S. (2006). Bovine seminal plasma proteins PDC-109, BSP-A3, and BSP-30-kDa share functional roles in storing sperm in the oviduct. Biol. Reprod. 75 (4), 501–507. doi: 10.1095/biolreprod.106.053306
Gwathmey T. M., Ignotz G. G., Suarez S. S. (2003). PDC-109 (BSP-A1/A2) promotes bull sperm binding to oviductal epithelium in vitro and may be involved in forming the oviductal sperm reservoir. Biol. Reprod. 69 (3), 809–815. doi: 10.1095/biolreprod.102.010827
Hammerstedt R. H. (1993). Maintenance of bioenergetic balance in sperm and prevention of lipid peroxidation: a review of the effect on design of storage preservation systems. Reproduction. Fertil. Dev. 5 (6), 675–690. doi: 10.1071/RD9930675
Harstine B., Utt M., DeJarnette J. (2018). Integrating a semen quality control program and sire fertility at a large artificial insemination organization. animal 12 (s1), s63–s74. doi: 10.1017/S1751731118000319
Hermans J. M., Jones R., Stone S. R. (1994). Rapid inhibition of the sperm protease acrosin by protein c inhibitor. Biochemistry 33 (18), 5440–5444. doi: 10.1021/bi00184a012
Hernández-González E. O., Martínez-Rojas D., Mornet D., Rendon A., Mújica A. (2001). Comparative distribution of short dystrophin superfamily products in various guinea pig spermatozoa domains. Eur. J. Cell Biol. 80 (12), 792–798. doi: 10.1078/0171-9335-00202
Hernández-González E. O., Mornet D., Rendon A., Martínez-Rojas D. (2005). Absence of Dp71 in mdx3cv mouse spermatozoa alters flagellar morphology and the distribution of ion channels and nNOS. J. Cell Sci. 118 (1), 137–145. doi: 10.1242/jcs.01584
Hunter R., Wilmut I. (1984). Sperm transport in the cow: peri-ovulatory redistribution of viable cells within the oviduct. Reprod. Nutr. Developpement. 24 (5A), 597–608. doi: 10.1051/rnd:19840508
Ibraghimov-Beskrovnaya O., Ervasti J. M., Leveille C. J., Slaughter C. A., Sernett S. W., Campbell K. P. (1992). Primary structure of dystrophin-associated glycoproteins linking dystrophin to the extracellular matrix. Nature 355 (6362), 696–702. doi: 10.1038/355696a0
Ignotz G. G., Cho M. Y., Suarez S. S. (2007). Annexins are candidate oviductal receptors for bovine sperm surface proteins and thus may serve to hold bovine sperm in the oviductal reservoir. Biol. Reprod. 77 (6), 906–913. doi: 10.1095/biolreprod.107.062505
Ignotz G. G., Lo M. C., Perez C. L., Gwathmey T. M., Suarez S. S. (2001). Characterization of a fucose-binding protein from bull sperm and seminal plasma that may be responsible for formation of the oviductal sperm reservoir. Biol. Reprod. 64 (6), 1806–1811. doi: 10.1095/biolreprod64.6.1806
Inoue N., Ikawa M., Isotani A., Okabe M. (2005). The immunoglobulin superfamily protein izumo is required for sperm to fuse with eggs. Nature 434 (7030), 234. doi: 10.1038/nature03362
Jodar M., Sendler E., Krawetz S. A. (2016). The protein and transcript profiles of human semen. Cell Tissue Res. 363 (1), 85–96. doi: 10.1007/s00441-015-2237-1
Kaji K., Oda S., Shikano T., Ohnuki T., Uematsu Y., Sakagami J., et al. (2000). The gamete fusion process is defective in eggs of Cd9-deficient mice. Nat. Genet. 24 (3), 279. doi: 10.1038/73502
Kasimanickam R., Kasimanickam V., Arangasamy A., Kastelic J. (2019). Sperm and seminal plasma proteomics of high-versus low-fertility Holstein bulls. Theriogenology 126, 41–48. doi: 10.1016/j.theriogenology.2018.11.032
Koziol J. H., Armstrong C. L. (2018). Manual for breeding soundness examination of bulls. Soc. Theriogenol.
Kuhn L. A., Griffin J. H., Fisher C. L., Greengard J. S., Bouma B. N., España F., et al. (1990). Elucidating the structural chemistry of glycosaminoglycan recognition by protein c inhibitor. Proc. Natl. Acad. Sci. 87 (21), 8506–8510. doi: 10.1073/pnas.87.21.850
Kuhn M., Hutchison J., Wiggans G. (2006). Characterization of Holstein heifer fertility in the united states. J. Dairy. Sci. 89 (12), 4907–4920. doi: 10.3168/jds.S0022-0302(06)72541-3
Laurell M., Christensson A., Abrahamsson P.-A., Stenflo J., Lilja H. (1992). Protein c inhibitor in human body fluids. seminal plasma is rich in inhibitor antigen deriving from cells throughout the male reproductive system. J. Clin. Invest. 89 (4), 1094–1101. doi: 10.1172/JCI115689
Lefebvre R., Chenoweth P. J., Drost M., LeClear C. T., MacCubbin M., Dutton J. T., et al. (1995). Characterization of the oviductal sperm reservoir in cattle. Biol. Reprod. 53 (5), 1066–1074. doi: 10.1095/biolreprod53.5.1066
Le Naour F., Rubinstein E., Jasmin C., Prenant M., Boucheix C. (2000). Severely reduced female fertility in CD9-deficient mice. Science 287 (5451), 319–321. doi: 10.1126/science.287.5451.319
Lu C.-H., Lee R. K.-K., Hwu Y.-M., Chu S.-L., Chen Y.-J., Chang W.-C., et al. (2011). SERPINE2, a serine protease inhibitor extensively expressed in adult male mouse reproductive tissues, may serve as a murine sperm decapacitation factor. Biol. Reprod. 84 (3), 514–525. doi: 10.1095/biolreprod.110.085100
Mahmoud A. I., Parrish J. J. (1996). Oviduct fluid and heparin induce similar surface changes in bovine sperm during capacitation: a flow cytometric study using lectins. Mol. Reprod. Dev. 43 (4), 554–560. doi: 10.1002/(SICI)1098-2795(199604)43:4<554::AID-MRD19>3.0.CO;2-Z
Mitchell J. R., Senger P. L., Rosenberger J. L. (1985). Distribution and retention of spermatozoa with acrosomal and nuclear abnormalities in the cow genital tract. J. Anim. Sci. 61 (4), 956–967. doi: 10.2527/jas1985.614956x
Miyado K., Yamada G., Yamada S., Hasuwa H., Nakamura Y., Ryu F., et al. (2000). Requirement of CD9 on the egg plasma membrane for fertilization. Science 287 (5451), 321–324. doi: 10.1126/science.287.5451.321
Müller P., Erlemann K.-R., Müller K., Calvete J. J., Töpfer-Petersen E., Marienfeld K., et al. (1998). Biophysical characterization of the interaction of bovine seminal plasma protein PDC-109 with phospholipid vesicles. Eur. Biophys. J. 27 (1), 33–41. doi: 10.1007/s002490050108
Nauc V., Manjunath P. (2000). Radioimmunoassays for bull seminal plasma proteins (BSP-A1/-A2, BSP-A3, and BSP-30-Kilodaltons), and their quantification in seminal plasma and sperm. Biol. Reprod. 63 (4), 1058–1066. doi: 10.1095/biolreprod63.4.1058
Norman H., Hutchison J., VanRaden P. (2011). Evaluations for service-sire conception rate for heifer and cow inseminations with conventional and sexed semen. J. Dairy. Sci. 94 (12), 6135–6142. doi: 10.3168/jds.2010-3875
Ortega M. S., Moraes J. G., Patterson D. J., Smith M. F., Behura S. K., Poock S., et al. (2018). Influences of sire conception rate on pregnancy establishment in dairy cattle. Biol. Reprod. 99 (6), 1244–1254. doi: 10.1093/biolre/ioy141
Ortega M. S., Rocha-Frigoni N. A., Mingoti G. Z., Roth Z., Hansen P. J. (2016). Modification of embryonic resistance to heat shock in cattle by melatonin and genetic variation in HSPA1L. J. Dairy. Sci. 99 (11), 9152–9164. doi: 10.3168/jds.2016-11501
Panner Selvam M. K., Agarwal A., Pushparaj P. N., Baskaran S., Bendou H. (2019). Sperm proteome analysis and identification of fertility-associated biomarkers in unexplained male infertility. Genes 10 (7), 522. doi: 10.3390/genes10070522
Parrish J., Susko-Parrish J., First N. (1985). Effect of heparin and chondroitin sulfate on the acrosome reaction and fertility of bovine sperm in vitro. Theriogenology 24 (5), 537–549. doi: 10.1016/0093-691X(85)90060-3
Parrish J., Susko-Parrish J., Winer M., First N. (1988). Capacitation of bovine sperm by heparin. Biol. Reprod. 38 (5), 1171–1180. doi: 10.1095/biolreprod38.5.1171
Pollard J. W., Plante C., Allan King W., Hansen P. J., Betteridge K. J., Suarez S. S. (1991). Fertilizing capacity of bovine sperm may be maintained by binding to oviductal epithelial cells. Biol. Reprod. 44 (1), 102–107. doi: 10.1095/biolreprod44.1.102
Pratt C., Church F. (1992). Heparin binding to protein c inhibitor. J. Biol. Chem. 267 (13), 8789–8794. doi: 10.1016/S0021-9258(19)50348-9
Richardson B. N., Larimore E. L., Walker J. A., Utt M. D., DeJarnette J. M., Perry G. A. (2017). Comparison of fertility of liquid or frozen semen when varying the interval from CIDR removal to insemination. Anim. Reprod. Sci. 178, 61–66. doi: 10.1016/j.anireprosci.2017.01.010
Saacke R. (2008). Sperm morphology: its relevance to compensable and uncompensable traits in semen. Theriogenology 70 (3), 473–478. doi: 10.1016/j.theriogenology.2008.04.012
Saacke R., Dalton J., Nadir S., Nebel R., Bame J. (2000). Relationship of seminal traits and insemination time to fertilization rate and embryo quality. Anim. Reprod. Sci. 60, 663–677. doi: 10.1016/S0378-4320(00)00137-8
Saacke R., Nadir S., Nebel R. (1994). Relationship of semen quality to sperm transport, fertilization, and embryo quality in ruminants. Theriogenology 41 (1), 45–50. doi: 10.1016/S0093-691X(05)80047-0
Starbuck M. J., Dailey R. A., Inskeep E. K. (2004). Factors affecting retention of early pregnancy in dairy cattle. Anim. Reprod. Sci. 84 (1-2), 27–39. doi: 10.1016/j.anireprosci.2003.12.009
Stoecklein K. S., Ortega M. S., Spate L. D., Murphy C. N., Prather R. S. (2021). Improved cryopreservation of in vitro produced bovine embryos using FGF2, LIF, and IGF1. PloS One 16 (2), e0243727. doi: 10.1371/journal.pone.0243727
Suarez S. S. (2015). “Gamete and zygote transport,” in Knobil and neill's physiology of reproduction, 4th ed. Eds. Plant T. M., Zeleznik A. J. (San Diego: CA: Academic Press), 197–232. doi: 10.1016/B978-0-12-397175-3.00005-3
Suarez S. S. (2016). Mammalian sperm interactions with the female reproductive tract. Cell Tissue Res. 363 (1), 185–194. doi: 10.1007/s00441-015-2244-2
Suarez S. S., Brockman K., Lefebvre R. (1997). Distribution of mucus and sperm in bovine oviducts after artificial insemination: the physical environment of the oviductal sperm reservoir. Biol. Reprod. 56 (2), 447–453. doi: 10.1095/biolreprod56.2.447
Sutovsky P. (2009). Sperm-egg adhesion and fusion in mammals. Expert Rev. Mol. Med. 11, e11. doi: 10.1017/s1462399409001045
Talluri T. R., Kumaresan A., Sinha M. K., Paul N., Ebenezer Samuel King J. P., Datta T. K. (2022). Integrated multi-omics analyses reveals molecules governing sperm metabolism potentially influence bull fertility. Sci. Rep. 12, 10692. doi: 10.1038/s41598-022-14589-w
Tríbulo P., Rivera R. M., Ortega Obando M. S., Jannaman E. A., Hansen P. J. (2019). “Production and culture of the bovine embryo,” in Comparative embryo culture: methods and protocols. Ed. Herrick J. R. (New York, NY: Springer New York), 115–129. doi: 10.1007/978-1-4939-9566-0_8
Uhrin P., Dewerchin M., Hilpert M., Chrenek P., Schöfer C., Zechmeister-Machhart M., et al. (2000). Disruption of the protein c inhibitor gene results in impaired spermatogenesis and male infertility. J. Clin. Invest. 106 (12), 1531–1539. doi: 10.1172/JCI10768
USDA (2018). “Dairy 2014,” in Health and management practices on U.S. dairy operations 2014 (Fort Collins, CO). USDA–APHIS–VS–CEAH–NAHMS. Available at: https://www.aphis.usda.gov/animal_health/nahms/dairy/downloads/dairy14/Dairy14_dr_PartIII.pdf.
Valour D., Michot P., Eozenou C., Lefebvre R., Bonnet A., Capitan A., et al. (2015). Dairy cattle reproduction is a tightly regulated genetic process: highlights on genes, pathways, and biological processes. Anim. Front. 5 (1), 32–41. doi: 10.2527/af.2015-0006
Wiggans G. R., Cole J. B., Hubbard S. M., Sonstegard T. S. (2017). Genomic selection in dairy cattle: the USDA experience. Annu. Rev. Anim. Biosci. 5, 309–327. doi: 10.1146/annurev-animal-021815-111422
Wilmut I., Hunter R. (1984). Sperm transport into the oviducts of heifers mated early in oestrus. Reprod. Nutr. Développement. 24 (4), 461–468. doi: 10.1051/rnd:19840411
Zheng X., Geiger M., Ecke S., Bielek E., Donner P., Eberspacher U., et al. (1994). Inhibition of acrosin by protein c inhibitor and localization of protein c inhibitor to spermatozoa. Am. J. Physiol. 267 (2 Pt 1), C466–C472. doi: 10.1152/ajpcell.1994.267.2.C466
Zoca S. M., Northrop-Albrecht E. J., Walker J. A., Cushman R. A., Perry G. A. (2022a). Proteomic analyses identify differences between bovine epididymal and ejaculated spermatozoa that contribute to longevity. Theriogenology 184, 51–60. doi: 10.1016/j.theriogenology.2022.02.021
Keywords: dystroglycan, fertility marker, in vitro fertilization, plasma serine protease inhibitor, sire conception rate, sperm protein
Citation: Zoca SM, Walker JA, Kline AC, Andrews TN, Rich JJJ, Epperson KM, Drum JN, Ortega MS, Cushman RA and Perry GA (2023) Relationship of field and in vitro fertility of dairy bulls with sperm parameters, including DAG1 and SERPINA5 proteins. Front. Anim. Sci. 4:1180967. doi: 10.3389/fanim.2023.1180967
Received: 06 March 2023; Accepted: 15 May 2023;
Published: 05 June 2023.
Edited by:
Maíra Bianchi Rodrigues Alves, University of São Paulo, BrazilReviewed by:
Renata Lançoni, Federal University of Uberlandia, BrazilCéline Augière, Laval University, Canada
Copyright © 2023 Zoca, Walker, Kline, Andrews, Rich, Epperson, Drum, Ortega, Cushman and Perry. This is an open-access article distributed under the terms of the Creative Commons Attribution License (CC BY). The use, distribution or reproduction in other forums is permitted, provided the original author(s) and the copyright owner(s) are credited and that the original publication in this journal is cited, in accordance with accepted academic practice. No use, distribution or reproduction is permitted which does not comply with these terms.
*Correspondence: George A. Perry, George.perry@ag.tamu.edu
†Mention of trade names or commercial products in this publication is solely for the purpose of providing specific information and does not imply recommendation or endorsement by the US Department of Agriculture. The USDA is an equal opportunity provider and employer