- 1School of Animal Sciences, Virginia Polytechnic Institute and State University, Blacksburg, VA, United States
- 2Department of Large Animal Clinical Sciences, Virginia Polytechnic Institute and State University, Blacksburg, VA, United States
Proper production of steroid hormones, such as progesterone (P4), is essential for maintenance of pregnancy. Provision of gluconeogenic substrates from the diet may alter postabsorptive energy supplies and associated hormone signaling in a manner supportive of elevating P4 concentrations. Crossbred Angus heifers (n=27) were balanced for pre-trial bodyweight (BW) and body condition score (BCS), then assigned to isoenergetic diets leveraging starch (ES) or fat (EF) as the primary source of energy (45.7% starch vs 11.5% fat). The heifers were placed on the assigned diets 8 days prior to the initiation of estrous synchronization and continued the diets through the second pregnancy diagnosis, 52 days after the initial insemination. On day 28 post-breeding, pregnancy status was diagnosed via ultrasonography. Measurements of follicle size and number were collected via rectal palpation and ultrasonography on days −10, −8, −4, and day 0 relative to breeding. Blood samples were collected on days −10, 1 through 7, 10, 14, 18, 21, 24, and 28 to analyze plasma for P4 concentrations and placental-associated glycoprotein (PAG) concentrations. Data were analyzed using a linear mixed effects model with a fixed effect for treatment and a random effect for pen. Observations collected over time were analyzed using a repeated measures approach with fixed effects for treatment, time, initial condition, and a random effect for pen. Interactions between treatment and pregnancy status were also tested for PAG and P4 concentrations. No treatment differences were observed in final BCS (P=0.12) and total dry matter intake (DMI; P=0.51), though heifers on the ES treatment tended to reach an increased BW (P=0.10). Although heifers fed ES diets had numerically higher conception rates compared to heifers fed EF diets (71.4% vs. 66.7%), there was no statistically significant difference. Furthermore, there were no significant differences observed among diets in terms of pregnancy outcomes, follicle size, or number. A treatment by pregnancy status interaction was detected for both PAG and P4 concentrations. These results suggest that short-term dietary starch supplementation provided around the time of breeding can result in shifts to reproductive hormone abundance that may be conducive for pregnancy establishment.
Introduction
Research focused on improving the reproductive efficiency in beef females has been ongoing for many years (Diskin and Kenny, 2014). Certain tools such as artificial insemination, embryo transfer, and in vitro fertilization, can be used by producers in the beef industry to improve reproductive efficiency and genetic selection intensity (Rosales-Torres et al., 2017). Despite the advantages these reproductive management tools have to offer, their adoption, particularly in the US, has been slow due to producers concerns related to increased expenses, labor, and risk (USDA, 2017). The addition of nutritional management recommendations to help reduce the risk of investment in assisted reproductive technologies could be beneficial in increasing widespread adoption of these technologies.
Reproductive efficiency is correlated with the nutritional status of the animal, and factors such as a proper body condition score and a positive energy balance are critical (D’Occhio et al., 2019). As a result, the efficiency of reproductive technologies are partially reliant on nutritional status as well (De Medeiros Bastos et al., 2004). Certain feed additives have held promise to improve reproductive success (Juchem et al., 2010). For example, omega-3 fatty acids can improve prostaglandin concentrations (Grant et al., 2005) and plasma P4 concentrations when included in ruminant diets (Burke et al., 1996). Due to hesitation around the adoption of assisted reproduction technologies in the U.S. beef industry, the association between an animal’s nutritional status and reproductive success may be an area of interest because dietary refinement presents a low-cost, low-input strategy to possibly mitigate risk associated with technology adoption.
Propionate, a volatile fatty acid produced in the rumen, alters reproductive hormone profiles (Dicostanzo et al., 1999), and serves as the major gluconeogenetic substrate in ruminants (Huntington, 1990). These functions are likely linked because glucose production is associated with elevated IGF-1 concentrations, which can enhance embryo development (Thatcher et al., 2003) and P4 production (Spicer and Chamberlain, 1998). Indeed, when jugular propionate was infused daily during the first 5 days after ovulation, plasma P4 concentrations were increased by d 14 post-ovulation compared to control animals receiving infusion of saline solution (Bedford et al., 2018). Increased P4 concentrations are associated with improved pregnancy rates (Johnson et al., 1958); therefore, these findings indicate that propionate is a strong candidate metabolite for nutritional modulation of reproduction. However, the energy availability of animals in the study from Bedford et al. (2018) differed, and isoenergetic assessment is necessary to better pinpoint the importance of energy substrate versus energy supply.
High starch diets, especially those containing rapidly rumen fermentable starches (Hatew et al., 2015), are widely acknowledged to increase propionate production and plasma propionate concentrations (Bauman et al., 1971). High starch diets have led to elevated P4 half-life in beef cows, compared to those fed high fiber diets (Lemley et al., 2009). However, the comparison of high starch and high fiber feeds in the previous literature is also confounded with energy supply. Similarly, incorporating long chain fatty acids into diets at a moderate amount has led to enhanced follicle parameters and increased P4 concentrations (Santos et al., 2008). As such, more targeted investigation of how feed supplements with similar energy contents influence reproductive outcomes is warranted.
Our objective was to evaluate how dietary supplementation of isoenergetic feeds leveraging greater starch or greater fat as the primary energy source influenced growth performance, pregnancy rates, follicle parameters, and plasma P4 and PAG concentration in grazed heifers. The hypothesis was that heifers supplemented with the diet leveraging starch would have a similar BW and BCS compared with heifers supplemented with a diet leveraging fat, but would have increased reproductive hormone concentrations and pregnancy outcomes.
Materials and methods
All animals sourced in this study belonged to Virginia Polytechnic and State University. All procedures with animals were performed in accordance with the protocols approved by the Institutional Animal and Care and Use Committee in Virginia Polytechnic and State University (IACUC #18-108).
Animals, diet, and experimental design
Twenty-seven crossbred Angus heifers (9 to 11 months old, 310 ± 79.8 kg of BW) were balanced into two groups based on BW and BCS and randomly assigned to one of two isoenergetic supplements formulated to leverage either starch (ES) (n=15) or fat (EF) (n=14) as the primary energy source. Both dietary treatments had ad libitum access to hay (10.3% CP, 71% NDF; Table 1), and the EF group was allowed to consume a supplement containing 10.5% fat and 11.6% starch, while the ES group was provided a supplement containing 0.27% fat and 64.7% starch (Table 1). These supplements were delivered to the animal via using a SmartFeed Pro Trailer (C-Lock, Rapid City, SD) at a maximum rate of 4.54 kg/d. To maintain appropriate stocking densities for the automated feeders, heifers were split into two groups balanced by supplement type and body weight and placed in two different pens. Supplement treatment was nested within pen such that equal numbers of animals in each pen were exposed to each supplement type. These two pens were adjacent dry lots approximately 3 acres in size, and therefore were not expected to differ in factors affecting production conditions. The diets were formulated to meet or exceed the nutrient requirements for heifers (National Academies of Sciences and Medicine, 2016), as well as to maintain similar formulated allowable gains based on metabolizable energy (ME) and protein (MP) (Table 1). Hay, water, and trace mineral salt were offered ad libitum, separately from treatment supplemental feed, while the intake of the provided supplements was limited by utilization of the SmartFeed Pro trailer. Heifers were trained to use the feeders over a 4-day period prior to the start of treatment diets, using cracked corn as a training feed. Heifers were then transitioned to their treatment supplementation strategy 18 d prior to the first artificial insemination (AI).
Estrus synchronization and breeding
On d −10 relative to first AI, ovulation synchronization began using the 7 d CO-Synch + CIDR protocol (Patterson et al., 2010). On d −10, each heifer had a controlled internal P4 drug release (CIDR) (Pfizer Animal Health, New York, NY) inserted, and was administered 100 μg of gonadotropin releasing hormone (GnRH) (Factrel; Pfizer Animal Health, New York, NY) via intramuscular injection. CIDRs were removed and an intramuscular injection of prostaglandin F2alpha (PGF2α) (PG; Lutalyse; dinoprost tromethamine; Pfizer Animal Health, New York, NY) was given on d −3. Heifers were then time-AI bred (TAI) with frozen semen, 72 hours post CIDR removal and PGF2α administration. At the time of breeding, an additional GnRH injection was administered (d 0, Figure 1). After the initial TAI, heifers were closely inspected every 12 hours for the display of heat behaviors, utilizing heat detection patches (Estrotect, Wisconsin, USA) to aid in estrus detection and insemination timing. All heifers displaying standing estrus at any point following the initial TAI breeding were re-bred 12 hours after behavioral estrus signs were observed.
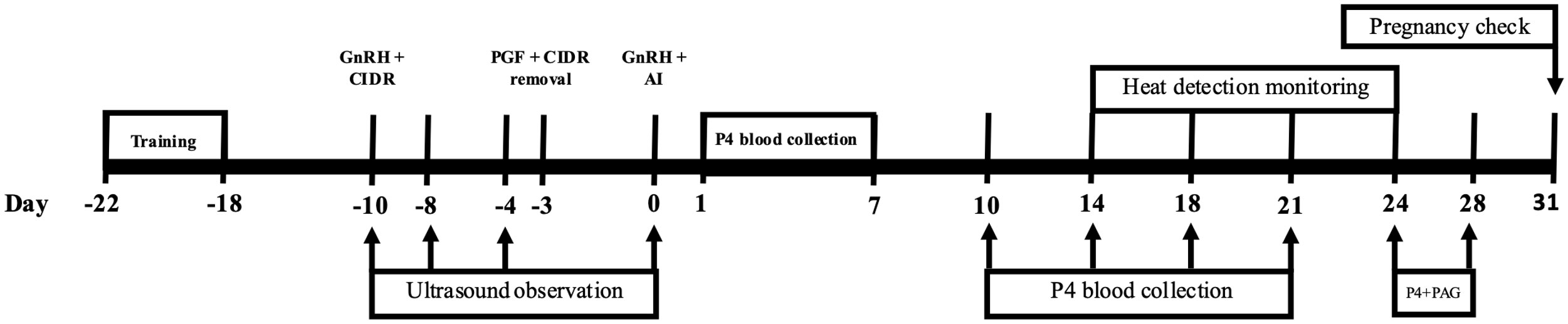
Figure 1 Timeline of timed artificial insemination (TAI) with estrus synchronization. As well as timeline of days that ovarian ultrasonography was performed and progesterone (P4) and placental associated glycoprotein (PAG) blood samples were performed.
Blood collection and hormonal assays
Blood samples were collected and analyzed on all heifers on days −10, 1, 2, 3, 4, 5, 6, 7, 10, 14, 18, 21, 24, and 28 relative to the animal’s most recent breeding date. Blood samples were collected via coccygeal venipuncture, and immediately placed on ice prior to being centrifuged at 2,500 × g for 15 minutes. All blood plasma was frozen at −20°C until further analysis. Progesterone concentration analysis was performed on all days collected, and pregnancy associated glycoprotein (PAG) concentration analysis was performed on d 24 and d 28. Analysis of serum P4 was conducted using Immulite P4 chemiluminescence assay kits (DPC, Los Angeles, CA, USA). Blood samples collected on d 24 and d 28 were assayed for PAG concentrations following a similar protocol to (Reese et al., 2018). In short, PAG was assessed using an in-house ELISA established by (Green et al., 2005) and modified using a polyclonal antibody (Ab 63), with duplicates run for each PAG sample. The inter-assay coefficient of variation for the PAG ELISA was 4.89%, while the intra-assay coefficient of variation was 8.84%.
Ultrasonography and ovarian measurements
Both ovaries were examined using ultrasonography by an experienced technician on days −10, −8, −4, and 0 relative to the first breeding. Ultrasonography was accomplished using an Ibex Evo (E.I. Medical Imaging, Loveland, CO) with a 7.5 Mhz probe. Both vertical and lateral measurements were taken of all follicles present. Additionally, ultrasonography was used on day 31 post-breeding for pregnancy diagnosis in heifers that did not exhibit heat after first breeding. Heifers displaying heat following the initial TAI were re-bred and diagnosed for pregnancy via ultrasonography on d 28, 31, 34, 36, relative to their most recent breeding date (Figure 1).
Feed sampling and titanium analysis
Titanium dioxide, an inert marker, was added to each concentrate batch by the feed mill for the purpose of estimating hay intake. Concentrate and hay samples were collected weekly and composited for chemical analysis. Concentrate samples included those obtained from multiple bags used each week, and hay samples were obtained from both pens. All feed samples were stored in a −20°C freezer until chemical analysis. Both concentrate supplements contained 60 g of titanium dioxide per 100 kg dry matter (DM) concentrate. Fecal samples were taken by rectal grab sampling every 6 hours over a 4-day sampling period to retrieve and measure titanium dioxide in the feces. The sampling time was rotated forward 1 hour every new day for 4 days to fully represent a 24 h sampling period. Fecal samples were then dried and ground through a 1 mm screen on a Model 4 Wiley mill (A. H. Thomas Scientific, Swedesboro, NJ). After grinding, samples were pooled by taking 1 g of each sample to create a single composite sample for the individual animal. Pooled samples were analyzed by first ashing the sample in a 500°C muffle furnace for 24 hr. Once ashed, sulfuric acid was added to the samples and boiled for 60 minutes (Gleason et al., 2022). Samples were then suspended in DI water and allowed to pass through a Whatman 541 grade filter (pore size 22 µm), and then DI water was added to bring the samples to 50 ml. Samples were analyzed for titanium using inductively coupled plasma atomic emission spectroscopy by Air, Water & Soil Laboratories, Inc. (Richmond, VA).
Feed analysis
All feedstuffs were dried for 24 hours in a 55°C oven, and then ground through a 1mm screen with a Model 4 Wiley mill (A.H. Thomas Scientific, Swedesboro, NJ). An Ankom 200 fiber analyzer (Ankom Technology, Macedon, NY) was used to determine NDF with addition of a neutral detergent, heat stable amylase, and sodium sulfite. Once NDF analysis was performed, the same sample bags underwent ADF analysis utilizing an acid detergent solution using the same Ankom 200 fiber analyzer (Ankom Technology, Macedon, NY) with the addition of an acidic detergent. Nitrogen content was analyzed utilizing combustion analysis (Vario El Cube CN Analyzer, Elementar Americas Inc., Mount Laurel, NJ). Crude protein was calculated by taking the determined N and multiplying by 6.25. Starch determination utilized the acetate buffer method of Hall (2009), by adding α-amylase from Bacillus licheniformis (FAA, Ankom Technology, Macedon NY) and amyloglucosidase from Aspergillusniger (E-AMGDF, Megaenzyme International, Wicklow, Ireland). Ether extract was determined using and Ankom XT10 extractor (Ankom Technology) with petroleum ether as a solvent (method 920.39; AOAC, 1990). Lastly, ash was determined by combusting ground samples for 8 hours in a muffle furnace at 500°C. Energy values for the supplements and their diets were estimated from the beef NASEM model (National Academies of Sciences and Medicine, 2016).
Statistical analysis
Statistical analyses were performed using the R Software version 4.1.2 (R Core Team, 2021). Data on animal BW, BCS, ADG, feed intake, and PAG concentration, were analyzed using a linear mixed-effect model with a fixed effect for treatment and random effect for pen. Observations collected over time were analyzed using a repeated measures approach with fixed effects for treatment, time, initial condition, the interaction between treatment and time, and a random effect for pen. Interactions between treatment and pregnancy status were also tested for PAG and P4 concentrations given that these outcomes were expected to differ with pregnancy status. First order autoregressive covariance structure was used due to optimal Akaike and Bayesian Information Criteria. Significance levels were set at P< 0.05 and a tendency considered when 0.05 ≤ P < 0.10.
Results and discussion
Animal performance
It was anticipated that animal performance would remain similar over the course of this trial due to the goal of formulating isoenergetic diets to evaluate the effect of energy sources. At the conclusion of the study, both groups of animals had gained weight, but there was no difference observed in final BCS (P=0.12) or average daily gain (ADG) (P=0.12) between treatments (Table 2). However, heifers on the ES treatment tended to approach an increased final BW (P=0.10). The tendency for elevated BW could be due to the higher CP (Table 1) in the ES supplement, despite similar calculate MP intake among diets (Table 2), or due to the potential for high fat intake on the EF diet to depress fiber digestion in the rumen (Oldick and Firkins, 2000). While only a slight difference, this caveat is important to consider within the present study, as an increase in availability of energy use efficiency (driven by improved digestibility) or enhanced protein availability (through higher CP feedstuff provision) could be partially responsible for the reproductive results observed. That said, the prevailing lack of performance difference among animals and the similarities in nutrient and energy intakes (Table 2) suggests the dietary treatments provided similar planes of nutrition, despite marked differences in supplemental feed composition. This similarity was purposeful within the experiment because it was our intention to evaluate how feed supplement energy profile, rather than quantity, influence reproductive outcomes.
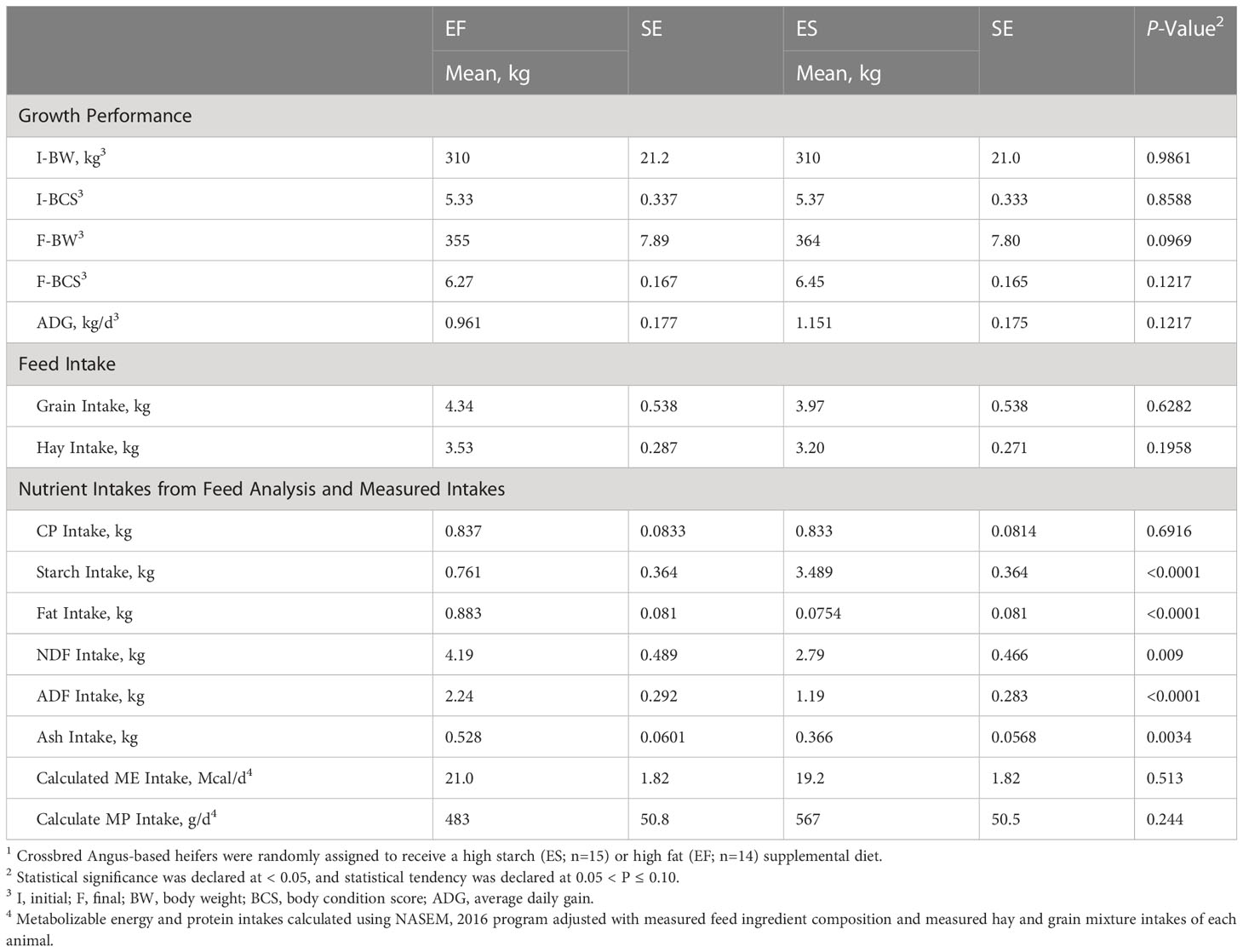
Table 2 Growth performance and intake parameters for beef heifers assigned to two different treatment diets1.
Calculated nutrient and predicted energy intakes
Nutrient intakes were calculated based on the DMI intake of hay and grain mixes, as well as the chemical composition of each diet (Table 2). It was expected that diets would differ in terms of starch, fiber, and fat intakes due to the diet composition necessary to target different energy substrates. Due to differences in diet composition, animals on the ES treatment had an increased intake of starch (P≤0.0001). It is well established that diets containing high starch tend to lead to an increase in propionate production and concentrations within the rumen (Bauman et al., 1971). Presumably, an increased production of propionate could shift post-absorptive metabolism toward gluconeogenesis, for which propionate is a major substrate (Hanson and Owen, 2013). In contrast, animals on the EF treatment had an increased intake of fat (P<0.0001), fiber (P<0.001), and organic matter (P=0.003) (Table 2). Diets high in fiber contribute to biohydrogenation and acetogenesis within the rumen (Ungerfeld, 2020), and are presumed to alter post-absorptive metabolism towards acetate metabolism and beta-oxidation processes (Sabine and Johnson, 1964). While the difference in organic matter stems from difference in composition of the treatment diets, this attribute should be further explored as a potential confounding factor in the study. Despite these differences in chemical composition and energy substrates, diets did not differ in calculated metabolizable energy (ME) and protein (MP). The values for ME and MP consumption were estimated using the NASEM model (National Academies of Sciences and Medicine, 2016), with allowable ME being 0.97 for both diets, and MP being 1.12 for the EF diet and 1.13 for the ES diet (Table 1). These calculations further support the diets’ equivalence in energy and protein, suggesting that downstream physiological differences observed herein should be largely due to energy composition shifts rather than energy availability.
Follicle abundance and size
Follicular dynamics were observed pre-breeding to represent the follicular stage prior to and during the breeding process. Table 3 shows the assessment of average follicle size and number beginning 8 days after the transition to the dietary treatments was completed. It was not expected that the follicular dynamics would differ by 8-day post-diet adaption (Ginther et al., 1989) because the development of those follicles would have been expected to occur before introducing a new metabolic state (as induced by the diet). Neither the abundance of follicles (sizes; small (P=0.40), medium (P=0.13), large (P=0.91)) nor the dimensions of follicles differed between treatment diets (Table 3). The limited influence of diet on follicle dynamics was somewhat unexpected. A diet higher in glucogenic precursors is associated with a higher plasma concentration of IGF-1 (Formigoni et al., 1996). Beneficially, IGF-1 acts on the granulosa cells of the ovary to enhance the ovarian response to gonadotropins and therefore potentiating follicular development (Adashi, 1998). The lack of influence of dietary treatments on follicle dynamics within the present study is likely attributed to the little time available between the start of treatments administration and sample collections for metabolic shifts to be established and influence follicular wave dynamics.
The number of small (P=0.01) and medium (P=0.002) follicles present differed by time relative to breeding, and large (P=0.10) follicles tended to differ over time (Figure 2). On day 0 relative to breeding, there was a greater number of large follicles than at times −8 or −4, and a lower number of small and medium sized follicles (Figure 2). The shift observed in small or medium and large follicles reflects the expected dynamics within a regular follicular wave. The literature on follicle maturation rate suggests that the rate of maturation is dependent on the complicated relationships of the hypothalamic-pituitary and ovarian feedback loop, follicular steroids, and extra ovarian growth factors (BK et al., 1995). Animals with larger pre-ovulatory follicle size at the time of breeding have increased chances of becoming pregnant (Lopes et al., 2007), and the implication of this previous work is that animals with an increased number of large follicles closer to breeding may have improved change of conception.
Reproductive performance
Although heifers in the ES treatment group (n=14) had a greater conception rate (57.14%) after the first service compared to heifers in the EF diet group (n=13) with a conception rate of 50.0%, there was no statistically significant difference between the treatments. After the first service, 11 heifers displayed signs of estrus indicating that they were not pregnant by TAI. After a second service was performed, only 36.4% of these heifers were bred, with the ES treatment group retaining a higher second-service success rate (ES 66.7% vs EF 28.6%). The final pregnancy rate for both treatment groups after two services was 66.7% (Table 4). The differences in reproductive outcomes associated with the diets are biologically sensible and map to the hypothesis of the work. It should also be noted that there was no reproductive tract assessment, or evaluation of the number of cycles individual heifers displayed prior to breeding. This could hold significance in pregnancy outcomes, as it is known that number of cycles prior to breeding can impact pregnancy rates in heifers (Roberts et al., 2019), which also could have influenced pregnancy rates among treatment groups. However, the inability to determine whether these groups differed significantly in reproductive success suggests these results should be advanced with further work in this area with multiple, replicated herds of animals receiving supplementation.
Hormone profiles
Results of plasma P4 analysis suggested diet and pregnancy status interacted significantly to influence P4 concentrations. P4 concentrations were also strongly influenced by initial P4 concentrations (P=<0.0001) and time (P=<0.0001; Table 5). In pregnant animals only, P4 concentrations of animals on the EF treatment were greater than those of animals on the ES treatment. Indeed, animals on the EF and ES treatments differed in the profile of P4 post-breeding, with the ES animals peaking near d 14, while the EF animals had a more sustained elevation to P4 concentration from d 18 to d 24 (Figure 3). Increased P4 circulation immediately after conception corresponds with increased pregnancy retention (Carter et al., 2008), which suggests the EF animals may have had greater hormonal support in pregnancy retention; however, based on the pregnancy data this did not confer to elevated breeding success. On day 24 post-breeding, plasma progesterone (P4) concentrations were greater in the EF treatment group, but there was no statistically significant difference compared to the ES treatment group (Figure 3). Although in opposition to the work previously conducted in our research group (Bedford et al., 2018) the elevated P4 concentrations on the EF diet are sensible, given other studies previously conducted. For example, Burke et al. (1996) found that ewes supplemented with increased Omega-6 fatty acids exhibited higher P4 blood plasma concentrations. The fat source used within the EF treatment was soybean oil, which is high in both Omega-3 and Omega-6 fatty acids (Covington, 2004). The differences in P4 concentrations observed among these treatments may be associated with dietary fatty acid composition rather than gluconeogenic precursors’ availability.
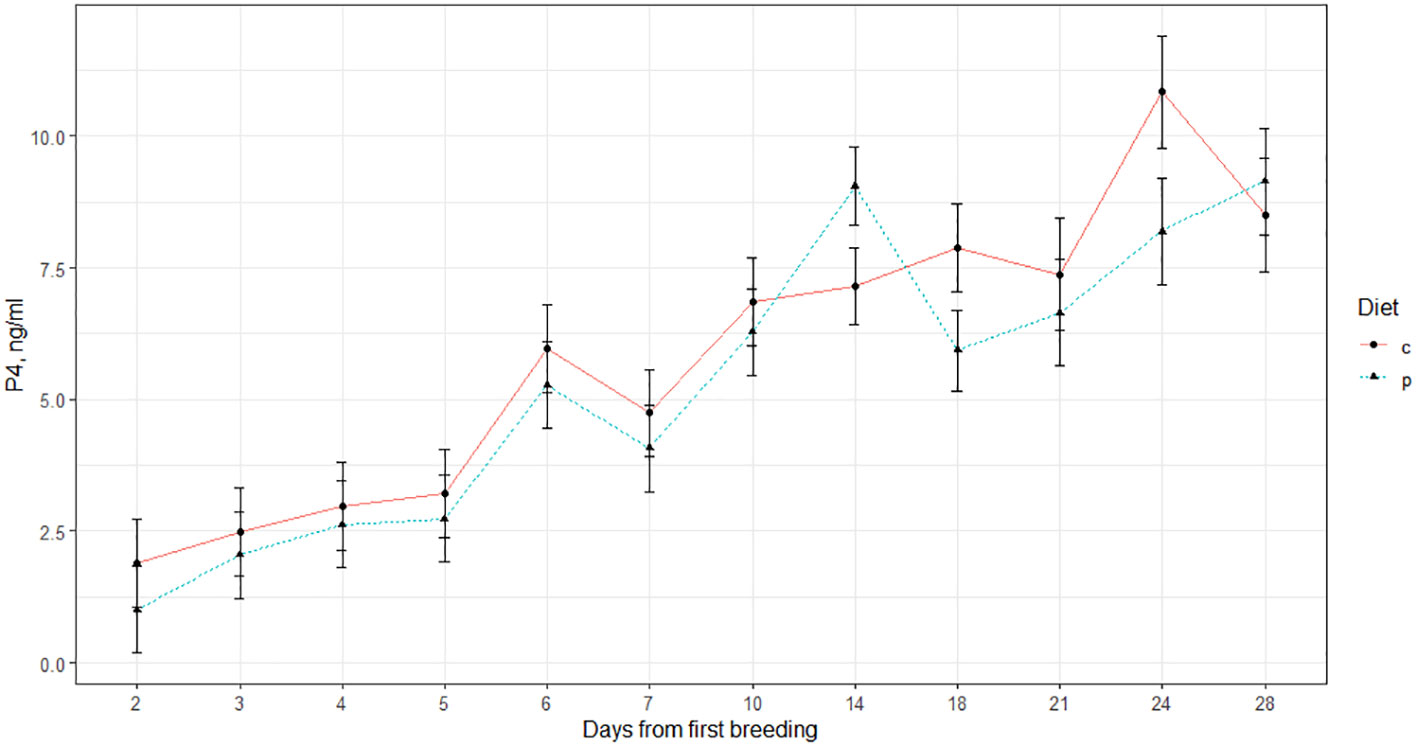
Figure 3 Blood plasma concentrations of progesterone (P4) in beef heifers after breeding. Heifers in the EF1 treatment group received a supplemental diet high in fat while the ES1 treatment group received diets2 high in starch. 1 c=EF treatment, p=ES treatment. 2 Crossbred Angus-based heifers were randomly assigned to receive a high starch (ES; n=15) or high fat (EF; n=14) supplemental diet.
The ES supplemented heifers exhibited a tendency to have different PAG concentrations compared to the EF supplemented heifers on day 28 (P=0.07; Table 6). The higher concentration of PAG could have been driven by the greater availability of glucogenic precursors on the higher starch diet. Theoretically, the improvement of glucogenic function in the placenta would result in an increased concentration of blood plasma PAG in the animal. Research on PAG concentration has shown that this marker may be an excellent predictor of pregnancy in cattle (Thompson et al., 2010). A decrease in PAG concentration has also been associated with increased incidence of embryonic mortality (Pohler et al., 2016). However, the method of action of PAG is still not well understood. Previous literature suggests that an earlier rise in pregnancy specific protein-B concentrations is indicative of earlier implantation(Middleton et al., 2022), potentially reflecting increased activity among binucleate cells. Given the trends identified in this study, further work is warranted to better explore the relationships among PAG, nutrition, and pregnancy outcomes.
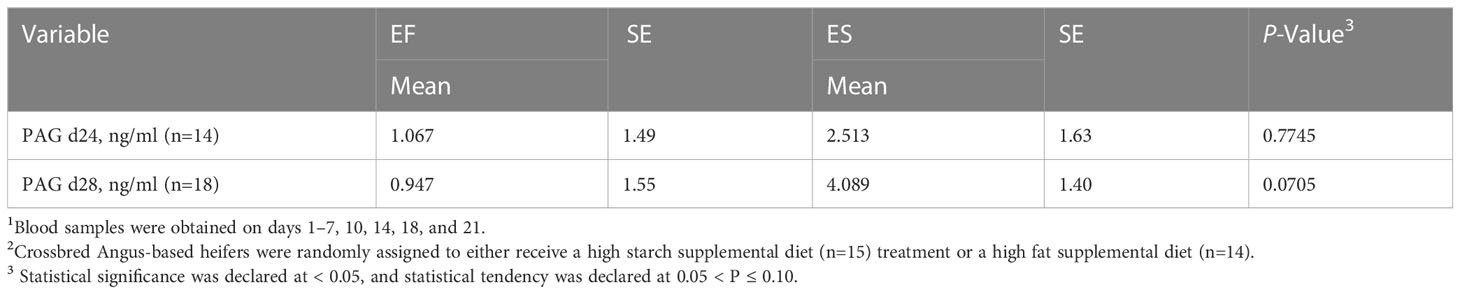
Table 6 Concentration of placental associated glycoprotein (PAG) in successfully pregnant heifers1 assigned to two different experimental diets2.
Conclusion
In conclusion, the comparison of ES and EF diets supports the hypothesis that reproductive system function can be modulated by dietary nutrient supply in the short-term. This rapid physiological response is of particular interest because it may present a low-cost, low-input method to enhance success of assisted reproductive technologies. Providing a 21-d pre-breeding dietary supplement strategy, coupled with a synchronization protocol, may be a more feasible and affordable approach for producers to improve the efficiency of assisted reproductive technologies such as timed-AI, estrus detection, or embryo transfer. That said, it is essential to note that this study’s results are preliminary and should be extended and replicated to refine mechanisms, define responses, and assess consistency. Overall, the comparison of diets suggests that starch-heavy supplements contribute to lower P4 concentrations 24 d post-breeding, and tend to lead to higher PAG concentrations by d 28 post-breeding. These observations suggest that an increase in propionate production may improve reproductive outcomes, but the exact mechanism in which this is accomplished is not known. Further comparison of energy supply and energy substrate within the same experimental context is needed to contextualize the importance of energy supply and energy source in modulating these various aspects of ruminant reproduction.
Data availability statement
The raw data supporting the conclusions of this article will be made available by the authors, without undue reservation.
Ethics statement
The animal study was reviewed and approved by the Virginia Tech Institutional Animal Care and Use Committee.
Author contributions
TD was responsible for animal monitoring, sample collection, data analysis, and manuscript preparation. KA assisted in manuscript preparation. JS, CG, ND, CT, and ZS assisted in animal monitoring and sample collection. AE and VM assisted with study design and contributed to manuscript editing. RW served as principal investigator, determined study design, guided data analysis, and contributed to manuscript editing. All authors contributed to the article and approved the submitted version
Funding
Funding for this work was provided by Agriculture and Food Research Initiative Competitive Grant no. 2017-05943, by Dairy Management Inc, and by state and federal funds appropriated to Virginia Tech.
Conflict of interest
The authors declare that the research was conducted in the absence of any commercial or financial relationships that could be construed as a potential conflict of interest.
Publisher’s note
All claims expressed in this article are solely those of the authors and do not necessarily represent those of their affiliated organizations, or those of the publisher, the editors and the reviewers. Any product that may be evaluated in this article, or claim that may be made by its manufacturer, is not guaranteed or endorsed by the publisher.
Abbreviations
ADG, Average daily gain; AI, Artificial insemination; BCS, Body condition score; BW, Bodyweight; CIDR, Controlled internal progesterone drug release; CL, Corpus luteum; DM, Dry matter; FTAI, Fixed-time artificial insemination; GnRH, Gonadotropin-releasing hormone; IGF-1, Insulin-like growth factor 1; ME, Metabolizable energy; MP, Metabolizable protein; N, Nitrogen; P4, Progesterone; PAG, Placental associated glycoprotein; PGF2α, Prostaglandin F2alpha; TAI, Timed artificial insemination; U.S., United States.
References
Adashi E. Y. (1998). The IGF family and folliculogenesis. J. Reprod. Immunol. 39, 13–19. doi: 10.1016/S0165-0378(98)00026-6
AOAC (1990). “Method 920.39,” in Official methods of analysis, 15th Edition. Ed. Helrich K. (Arlington, VA: The Association of Official Analytical Chemists, Inc.), 79.
Bauman D. E., Davis. C. L., Bucholtz H. F. (1971). Proprionate production in the rumen of cows fed either a control or high-grain, low-fiber diet. J. Dairy Sci. 54, 1282–1287. doi: 10.3168/jds.S0022-0302(71)86021-6
Bedford A., Beckett L., Hardin K., Dias N. W., Davis T., Mercadante V. R. G., et al. (2018). Propionate affects insulin signaling and progesterone profiles in dairy heifers. Sci. Rep. 8, 17629. doi: 10.1038/s41598-018-35977-1
BK C., Scaramuzzi R., Webb R. (1995). Control of antral follicle development and selection in sheep and cattle. J. Reprod. Fertil. Suppl. 49, 335–350.
Burke J. M., Carroll D. J., Rowe K. E., Thatcher W. W., Stormshak F. (1996). Intravascular infusion of lipid into ewes stimulates production of progesterone and Prostaglandin1. Biol. Reprod. 55, 169–175. doi: 10.1095/biolreprod55.1.169
Carter F., Forde N., Duffy P., Wade M., Fair T., Crowe M. A., et al. (2008). Effect of increasing progesterone concentration from Day 3 of pregnancy on subsequent embryo survival and development in beef heifers. Reprod. Fertil. Dev. 20, 368–375. doi: 10.1071/RD07204
D'Occhio M. J., Baruselli P. S., Campanile G. (2019). Influence of nutrition, body condition, and metabolic status on reproduction in female beef cattle: A review. Theriogenology 125, 277–284. doi: 10.1016/j.theriogenology.2018.11.010
De Medeiros Bastos G., Brenner R. H., Willke F. C. W., Neves J. P., De Oliveira J. F. C., Bragança J. F. M., et al. (2004). Hormonal induction of ovulation and artificial insemination in suckled beef cows under nutritional stress. Theriogenology 62, 847–853. doi: 10.1016/j.theriogenology.2003.12.005
Dicostanzo A., Williams J., Keisler D. (1999). Effects of short-or long-term infusions of acetate or propionate on luteinizing hormone, insulin, and metabolite concentrations in beef heifers. J. Anim. Sci. 77, 3050–3056. doi: 10.2527/1999.77113050x
Diskin M., Kenny D. (2014). Optimising reproductive performance of beef cows and replacement heifers. Animal 8, 27–39. doi: 10.1017/S175173111400086X
Formigoni A., Cornil M.-C., Prandi A., Mordenti A., Rossi A., Portetelle D., et al. (1996). Effect of propylene glycol supplementation around parturition on milk yield, reproduction performance and some hormonal and metabolic characteristics in dairy cows. J. Dairy Res. 63, 11–24. doi: 10.1017/S0022029900031502
Ginther O. J., Kastelic J. P., Knopf L. (1989). Composition and characteristics of follicular waves during the bovine estrous cycle. Anim. Reprod. Sci. 20, 187–200. doi: 10.1016/0378-4320(89)90084-5
Gleason C., Wilson T., Mercadante V., White R. (2022). Evaluation of a rumen modifier to limit pellet intake in beef brood cows. Front. Anim. Sci. 3. doi: 10.3389/fanim.2022.1080115
Grant M. H., Alexander B. M., Hess B. W., Bottger J. D., Hixon D. L., Van Kirk E. A., et al. (2005). Dietary supplementation with safflower seeds differing in fatty acid composition differentially influences serum concentrations of prostaglandin F metabolite in postpartum beef cows. Reprod. Nutr. Dev. 45, 721–727. doi: 10.1051/rnd:2005056
Green J. A., Parks T. E., Avalle M. P., Telugu B. P., Mclain A. L., Peterson A. J., et al. (2005). The establishment of an ELISA for the detection of pregnancy-associated glycoproteins (PAGs) in the serum of pregnant cows and heifers. Theriogenology 63, 1481–1503. doi: 10.1016/j.theriogenology.2004.07.011
Hall M. B. (2009). Determination of starch, including maltooligosaccharides, in animal feeds: Comparison of methods and a method recommended for AOAC collaborative study. J. AOAC Int. 92 (1), 42–49. doi: 10.1093/jaoac/92.1.42
Hanson R. W., Owen O. E. (2013). “Gluconeogenesis,” in Encyclopedia of Biological Chemistry, 2nd ed. Eds. Lennarz W. J., Lane M. D. (Waltham: Academic Press).
Hatew B., Podesta S. C., Van Laar H., Pellikaan W. F., Ellis J. L., Dijkstra J., et al. (2015). Effects of dietary starch content and rate of fermentation on methane production in lactating dairy cows. J. Dairy Sci. 98, 486–499. doi: 10.3168/jds.2014-8427
Huntington G. (1990). Energy metabolism in the digestive tract and liver of cattle: influence of physiological state and nutrition. Reprod. Nutr. Dev. 30, 35–47. doi: 10.1051/rnd:19900103
Johnson K., Ross R., Fourt D. (1958). Effect of progesterone administration on reproductive efficiency. J. Anim. Sci. 17, 386–390. doi: 10.2527/jas1958.172386x
Juchem S., Cerri R., Villaseñor M., Galvão K., Bruno R., Rutigliano H. M., et al. (2010). Supplementation with calcium salts of linoleic and trans-octadecenoic acids improves fertility of lactating dairy cows. Reprod. Domest. Anim. 45, 55–62. doi: 10.1111/j.1439-0531.2008.01237.x
Lemley C. O., Wilmoth T. A., Tager L. R., Krause M., Wilson M. E. (2009). Effect of a High Corn Starch Diet on Insulin Concentrations, Cytochrome P450 2C and 3A Activity, and Progesterone Clearance in Dairy Cows (Oxford University Press).
Lopes A. S., Butler S. T., Gilbert R. O., Butler W. R. (2007). Relationship of pre-ovulatory follicle size, estradiol concentrations and season to pregnancy outcome in dairy cows. Anim. Reprod. Sci. 99, 34–43. doi: 10.1016/j.anireprosci.2006.04.056
Middleton E. L., Minela T., Ahearne M., Arnold H., Santos A., Pursley J. R. (2022). Dairy heifers have an earlier increase in serum pregnancy-specific protein B compared with lactating dairy cows. Is this an indicator of earlier conceptus attachment? JDS Commun. 3, 291–295. doi: 10.3168/jdsc.2021-0198
National Academies of Sciences, e. & Medicine (2016). Nutrient Requirements of Beef Cattle: Eighth Revised Edition (Washington, DC: The National Academies Press).
Oldick B. S., Firkins J. L. (2000). Effects of degree of fat saturation on fiber digestion and microbial protein synthesis when diets are fed twelve times daily. J. Anim. Sci. 78, 2412–2420. doi: 10.2527/2000.7892412x
Patterson D. J., Mallory D. A., Nash J. M., Smith M. F. (2007). Estrus synchronization protocols for heifers. Proc Applied Reproductive Strategies in Beef Cattle, Billings, MT, 63–97.
Pohler K., Pereira M., Lopes F., Lawrence J., Keisler D., Smith M., et al. (2016). Circulating concentrations of bovine pregnancy-associated glycoproteins and late embryonic mortality in lactating dairy herds. J. Dairy Sci. 99, 1584–1594. doi: 10.3168/jds.2015-10192
Reese S., Pereira M., Edwards J., Vasconcelos J., Pohler K. (2018). Pregnancy diagnosis in cattle using pregnancy associated glycoprotein concentration in circulation at day 24 of gestation. Theriogenology 106, 178–185. doi: 10.1016/j.theriogenology.2017.10.020
R Core Team (2021). R: A language and environment for statistical computing (Vienna, Austria: R Foundation for Statistical Computing). Available at: https://www.R-project.org/.
Roberts A. J., Ketchum J. N., Funston R. N. (2019). Developmental and reproductive characteristics of beef heifers classified by number of estrous cycles experienced by start of first breeding. Transl. Anim. Sci. 3, 541–548. doi: 10.1093/tas/txy118
Rosales-Torres A., López-Cedillo Z., Hernández-Coronado C., Rosete-Fernández J., Mendoza G., Guzmán A. (2017). Short-term dietary concentrate supplementation during estrus synchronization treatment in beef cows increased IGF-I serum concentration but did not affect the reproductive response. Trop. Anim. Health Prod. 49, 221–226. doi: 10.1007/s11250-016-1166-7
Sabine J. R., Johnson B. C. (1964). Acetate metabolism in the ruminant. J. Biol. Chem. 239, 89–93. doi: 10.1016/S0021-9258(18)51751-8
Santos J., Bilby T., Thatcher W., Staples C., Silvestre F. (2008). Long chain fatty acids of diet as factors influencing reproduction in cattle. Reprod. Domest. Anim. 43, 23–30. doi: 10.1111/j.1439-0531.2008.01139.x
Spicer L. J., Chamberlain C. S. (1998). Influence of cortisol on insulin-and insulin-like growth factor 1 (IGF-1)-induced steroid production and on IGF-1 receptors in cultured bovine granulosa cells and thecal cells. Endocrine 9, 153–161. doi: 10.1385/ENDO:9:2:153
Thatcher W., Guzeloglu A., Meikle A., Kamimura S., Bilby T., Kowalski A., et al. (2003). Regulation of embryo survival in cattle. Reproduction-Cambridge-Supplement-, 253–266.
Thompson I., Cerri R., Kim I., Green J., Santos J., Thatcher W. (2010). Effects of resynchronization programs on pregnancy per artificial insemination, progesterone, and pregnancy-associated glycoproteins in plasma of lactating dairy cows. J. Dairy Sci. 93, 4006–4018. doi: 10.3168/jds.2009-2941
Ungerfeld E. M. (2020). Metabolic hydrogen flows in rumen fermentation: principles and possibilities of interventions. Front. Microbiol. 11. doi: 10.3389/fmicb.2020.00589
Keywords: starch diet, fat diet, grazed heifers, reproduction, pregnancy rate, artificial insemination
Citation: Davis TC, Amirault KE, Stewart JW, Gleason CB, Dias NW, Timlin CL, Seekford ZK, Ealy AD, Mercadante VRG and White RR (2023) Effect of dietary energy source on pregnancy rates and reproductive physiology of pastured beef heifers. Front. Anim. Sci. 4:1170377. doi: 10.3389/fanim.2023.1170377
Received: 20 February 2023; Accepted: 07 July 2023;
Published: 01 August 2023.
Edited by:
Todd Riley Callaway, University of Georgia, United StatesReviewed by:
Christi Swaggerty, Southern Plains Agricultural Research Center (USDA), United StatesJon Schoonmaker, Purdue University, United States
Andrew Foote, Oklahoma State University, United States
Copyright © 2023 Davis, Amirault, Stewart, Gleason, Dias, Timlin, Seekford, Ealy, Mercadante and White. This is an open-access article distributed under the terms of the Creative Commons Attribution License (CC BY). The use, distribution or reproduction in other forums is permitted, provided the original author(s) and the copyright owner(s) are credited and that the original publication in this journal is cited, in accordance with accepted academic practice. No use, distribution or reproduction is permitted which does not comply with these terms.
*Correspondence: Robin R. White, cnJ3aGl0ZUB2dC5lZHU=