- 1Department of Preventive Veterinary Medicine and Animal Health, School of Veterinary Medicine and Animal Science, University of São Paulo, Pirassununga, SP, Brazil
- 2Graduation Program in One Health, University Santo Amaro, Sâo Paulo, SP, Brazil
- 3Facoltà di Medicina Veterinaria, Università degli Studi di Teramo, Teramo, Italy
- 4Department of Internal Medicine, School of Veterinary Medicine and Animal Science, University of Sao Paulo, Sao Paulo, SP, Brazil
- 5Department of Basic Sciences, School Animal Science and Food Engineering, University of São Paulo, Pirassununga, SP, Brazil
- 6Federal Institute of Education, Science, and Technology of Rio Grande do Sul, Sertão, RS, Brazil
Introduction: Boars are often housed in stressful environments on commercial farms, experiencing poor welfare. These conditions may cause epigenetic changes in the boars' gametes, which could potentially be transmitted to their offspring. We aimed to investigate the effect of three different boars housing environments on the survival, aggression, and nociceptive responses of their offspring.
Methods: For four weeks, 18 boars were housed in three different systems: crates (C;n=6), pens (P;n=6), and enriched pens (E;n=6). The environmental enrichment was provided twice daily (brushing, shower, and hay). Thirteen gilts were housed in outdoor paddocks and inseminated with pooled semen from the boars kept in the three treatments. We evaluated the number of live-born, stillborn, and weaned piglets, sex, and mortality rate. Weaning was performed at 29 days of age. For each piglet, six body photographs were taken for five days postweaning to measure skin lesions (n=138). On Day 34, the nociceptive pressure threshold was assessed using an analgesimeter (n=138). DNA paternity tests were carried out at the end of the study (n=181). A generalized linear model with a negative binomial distribution was used to compare the number of live-born/weaned piglets and skin lesions among the treatment groups. We used a Kruskal‒Wallis test to analyze nociceptive data.
Results: More live-born and weaned piglets were fathered from boars kept in the E group than the P group (p=0.002;p=0.001, respectively). A trend was observed in the number of skin lesions on the left side of piglets (P<C;p=0.053). For nociceptive assessments, offspring from P boars showed less right leg withdrawal than piglets from E and C boars (p=0.008); the P group had a higher average nociceptive value than the C group (p=0.002). All treatments differed in the region adjacent to the tail for nociceptive pressure threshold (P>E>C;p<0.001).
Discussion and conclusion: Our results suggest that providing an enriched environment for boars can increase the number of live-born and weaned piglets. Moreover, the boars housing conditions can influence nociceptive threshold in their offspring. Further research must be performed to understand the underlying mechanism associated with these changes using epigenetics protocols and measuring physiological indicators and other molecular markers in semen and/or sperm cell samples.
1 Introduction
Intergenerational effects have generally been investigated in sows (Bernardino et al., 2016; Tatemoto et al., 2019b; Nery da Silva et al., 2021; Sarmiento et al., 2021). Housing of sows in unstable social environment during pregnancy induced long-term alterations in the nociceptive responses of their offspring (Rutherford et al., 2009; Sandercock et al., 2011). Moreover, piglets born from sows with lameness during pregnancy showed altered reactions to noxious mechanical stimuli (Parada et al., 2019). Furthermore, environmental conditions that improved the welfare of gestating sows resulted in modulation of the developmental outcomes of their offspring by altering the aggressive behavioral profile and the number of skin lesions (Bernardino et al., 2016; Sarmiento et al., 2021), changing the hypothalamic-pituitary-adrenal axis responsiveness (Tatemoto et al., 2019a), and impacting the weaning weight of offspring (Sarmiento et al., 2021). Conversely, few scientific studies evaluating the welfare of boars and the consequences on their offspring have been carried out. The scientific literature showed that when boars were exposed to positive environmental conditions, they produced better quality semen (Bernardino et al., 2022). Other studies have shown that piglets born from boars from different genetic lines that received or did not receive herbal diets have different levels of aggressiveness (Aikins-Wilson et al., 2022). Such effects may be related to epigenetic factors. Evidence of these molecular changes was found in semen from boars with high fertility. This study demonstrated that there were approximately three times more differentially methylated sperm DNA regions in boars with high fertility than boars with low fertility (Khezri et al., 2019; Pértille et al., 2021), and the modulation of gene expression and DNA methylation impacted the carcass yields of the offspring of boars fed a methyl-enriched diet (Braunschweig et al., 2012). Therefore, based on the scientific literature, changes in the environment of breeding boars may alter their offspring’s behavior, performance, and physiology.
Most adopted pig commercial systems are intensive and have a high animal density, which may result in social challenges, limited options for environmental control, and a lack of stimulation (Pedersen, 2018). Studies have reported that many management procedures in swine production can cause stress and acute or chronic pain (Ison et al., 2016). The weaning period, a routine for all swine production farms, is a crucial moment to evaluate an animal; in the face of this challenge, piglets need to overcome several stressful challenges, such as maternal deprivation, being in a novel environment, mixing with unknown piglets, and even diet changes (Campbell et al., 2013). These management and housing conditions are not fully in accordance with modern good production practices and are likely to impair pig welfare (Broom et al., 1995), potentially modulating features of future generations through underlying germ-cell-based mechanisms.
Understanding the underlying mechanisms of the interaction between genes and the environment and their effects on an individual’s phenotype and progeny (Nilsson et al., 2018) requires an approach to systematically assess the neurophysiological and cognitive responses of animals with contrasting experiences (Murphy et al., 2021). The inherited transmission and environmental modulation of an individual’s phenotype can provide the opportunity to alter behavior and physiology (Skinner and Guerrero-Bosagna, 2009; Ferraz, 2011; Bale, 2015; Otten et al., 2015; Donkin and Barrès, 2018; Siddeek et al., 2018; Guerrero-Bosagna et al., 2020), with possible consequences for pig welfare. Stressful conditions experienced by progenitors can also increase offspring vulnerability to several pathological conditions, including those that are mainly related to interruptions in stress response mechanisms (Lacal and Ventura, 2018). The intergenerational relationship is important, as piglet survival strategies result from complex interactions among sows, piglets and the environment (Edwards, 2002), and possibly from boar influences.
Poor welfare and stressful challenges can lead to epigenetic alterations in gametes that result in modulated gene expression, altering offspring behavior and phenotypes (Rodgers et al., 2013; Bale, 2015). Understanding the effects of the environment to which progenitors are exposed can contribute to assessing and understanding progeny resilience. Therefore, in the present study, our objective was to investigate whether the housing conditions of boars could alter the mortality, aggressive behavior, and nociception responses of their offspring.
2 Materials and methods
This study was conducted at the University of São Paulo Campus Fernando Costa, Pirassununga - São Paulo, Brazil, after receiving approval from the Ethics Committee on Animal Use from the School of Veterinary Medicine and Animal Science (FMVZ) of the University of São Paulo (USP) under the number 6555081018. In addition, the study was conducted according to the ARRIVE guidelines (https://arriveguidelines.org/).
2.1 Experimental design
A semen pool from all boars, which had similar genetic backgrounds and were housed under different conditions, further described in the next section, was used for the artificial insemination of gilts housed outdoors (see Figure 1). Piglets born from these gilts were evaluated during the farrowing and nursery periods. Paternity testing using DNA from hair samples was carried out for all piglets at the end of the experiment.
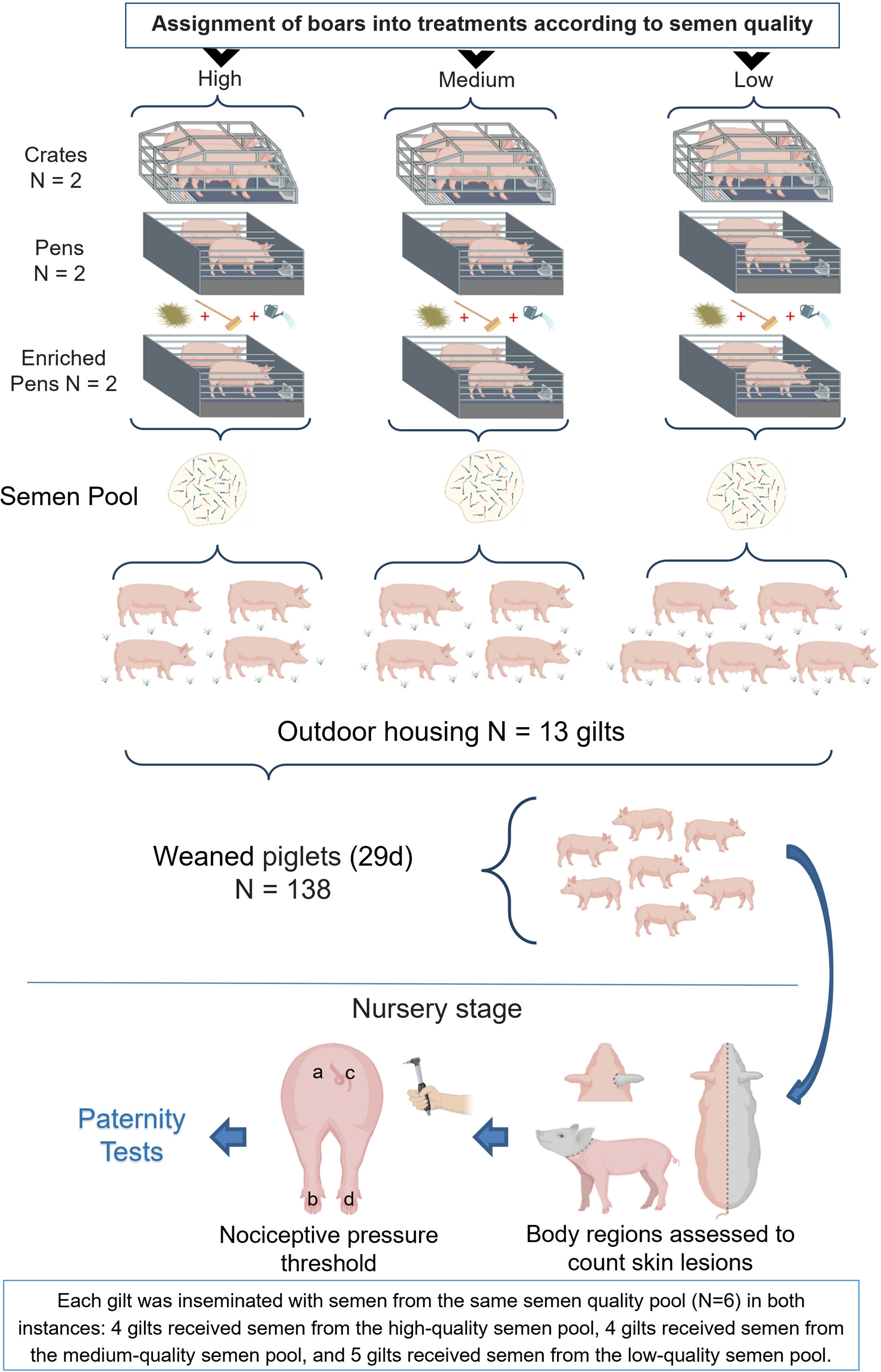
Figure 1 Gilts housed in an outdoor system (n = 13) were inseminated with pooled semen from all boars housed in three different systems: crates (n = 6), pens (n = 6), and enriched pens (n = 6). Offspring (n = 138) were evaluated (skin lesions and nociceptive thresholds) in the nursery stage. Finally, a paternity test was performed for all piglets.
2.1.1 Boar housing conditions
Eighteen 12-month-old hybrid boars (Landrace x Large White) of similar genetic backgrounds were used for the study. Boars were housed under three different conditions for four weeks: crates (C; n = 6; 1.97 x 0.75 m and a height of 0.98 m), individual pens (P; n = 6; 3.76 x 2.41 m) and enriched pens (E; n = 6; 3.76 x 2.41 m). For boars in the enriched pens, environmental enrichment was offered twice daily, one hour after feeding. In a sequence of events per enrichment exposure, boars were brushed for two minutes using a broom, showered with water for 30 seconds, and provided with 500 grams of hay as rooting material for the pen floor. Water was supplied ad libitum by a nipple drinker, and the animals were fed a concentrate diet of approximately 2.8 kg/day/boar in troughs daily, proportioned into two meals at 07 h and 13 h. Further details on housing features can be found in Bernardino et al. (2022).
2.1.2 Semen collection and insemination of gilts
Semen quality was assessed two weeks before boar assignment to treatment groups using computer-assisted sperm analysis (CASA) and sperm morphology. According to the treatment distribution, the animals were classified as having high, medium, or low semen quality and equally distributed among treatment groups. Details about semen quality were previously reported by Bernardino et al. (2022).
After four weeks under the C, P, and E experimental treatments, semen was collected from the 18 boars, diluted with commercial diluent (Androstar® Plus, Minitube, Germany), and stored in an incubator at 17°C. Prior to insemination, the semen from all boars with high semen quality that were housed in crates (n = 2), pens (n = 2), and enriched (n = 2) pens were pooled. The same pooling strategy was adopted for the boars with medium and low semen quality. Immediately preceding insemination, the three quality-based semen pools (high, medium, and low quality) were standardized to 30 million mobile cells/ml for later use in the gilts.
Estrus among all gilts was synchronized using 5 ml of altrenogest (Regumate®, MSD Animal Health) per animal for 18 consecutive days. They were inseminated twice, immediately after estrus detection and 24 h later. Each gilt was inseminated with semen from the same semen quality pool in both instances: four gilts received semen from the high-quality semen pool, four gilts received semen from the medium-quality semen pool, and five gilts received semen from the low-quality semen pool (n = 13 gilts; Figure 1).
2.1.3 Housing and management of gilts and piglets
Thirteen gilts (F1 Large White x Landrace, Afrodite®, Topgen) were housed outdoors and subjected to the same handling conditions throughout the experimental period. All gestating gilts were housed in a single paddock (400 m2 per animal) with electric fences, two entrance gates, one mud pool, and shade from a natural tree (approximately 250 m2). All gilts were floor-fed a conventional gestation concentrate diet of 2.5 kg/day/gilt, portioned into two meals at 07 h and 13 h. Water was supplied ad libitum through nipple drinkers.
Ten days before farrowing, the paddock was divided to allocate gilts (in pairs) access to farrowing shelters built with bamboo and rubber mats as walls (EBV-30 Estrado de Borracha Vedovati®); hay was provided 24 h prior to parturition for nest building. All births were monitored, and manual assistance was provided during parturition when needed. Immediately after birth, all piglets were oriented to the sow´s udder to stimulate colostrum intake, and the umbilical cord was cut and disinfected. Postfarrowing, lactation concentrate was provided ad libitum to all gilts on rubber mats at 07 h and 16 h.
Piglets were ear tagged for identification on the third day after birth. They were not submitted to the standard procedures of commercial farms for piglets, such as iron dextran administration, teeth grinding, tail docking, or surgical castration as a choice for outdoor rearing. Creep feeding was provided for piglets after 14 days of age according to their dietary intake. All 138 piglets were weaned and weighed on day 29 of age and reweighted on day 36 after birth.
2.2 Weaning and nursery
During weaning, at 29 days of age piglets were distributed in three pens (25 m2 each), balanced by body weight within the litter: light (n = 46; 5.0 ± 0.10 kg), medium (n = 44; 6.0 ± 0.12 kg), and heavy (n = 48; 7.0 ± 0.13 kg). Hay was provided in the pens as bedding material. Piglets were fed a grower concentrate diet divided into five meals per day based on their ad libitum intake, and water was provided ad libitum. Saliva samples for cortisol quantification were collected with buccal swabs (3 cotton swabs, Johnson & Johnson®) from piglets in the morning (8 -10h am) on the day of weaning when they were still with the sow and 24 h post-weaning. Afterwards, 50µl saliva samples were analyzed with a cortisol enzyme immunoassay (EIA) (Siegford et al., 2008; Tatemoto et al., 2019a; Bernardino, 2021; de Souza Farias et al., 2021; Sarmiento et al., 2021).
2.2.1 Assessment of skin lesions
For all piglets, three pictures were taken daily from each side of their body: face, external ear, and body (Figure 2). At the time of weaning and 24 h postweaning for three consecutive days, totaling 30 images per animal (Guy et al., 2009; Bernardino et al., 2016; Sarmiento et al., 2021). Physical restraint was performed gently following the same protocol to obtain the photos. All pictures were taken at a distance of 50 to 70 cm from the piglet with a digital camera. Two observers who were blinded to the treatments carried out the picture evaluation. The total skin lesions counted per day/body region for each piglet were used for statistical analyses using the average data from both observers (Guy et al., 2009; Bernardino et al., 2016; Sarmiento et al., 2021).
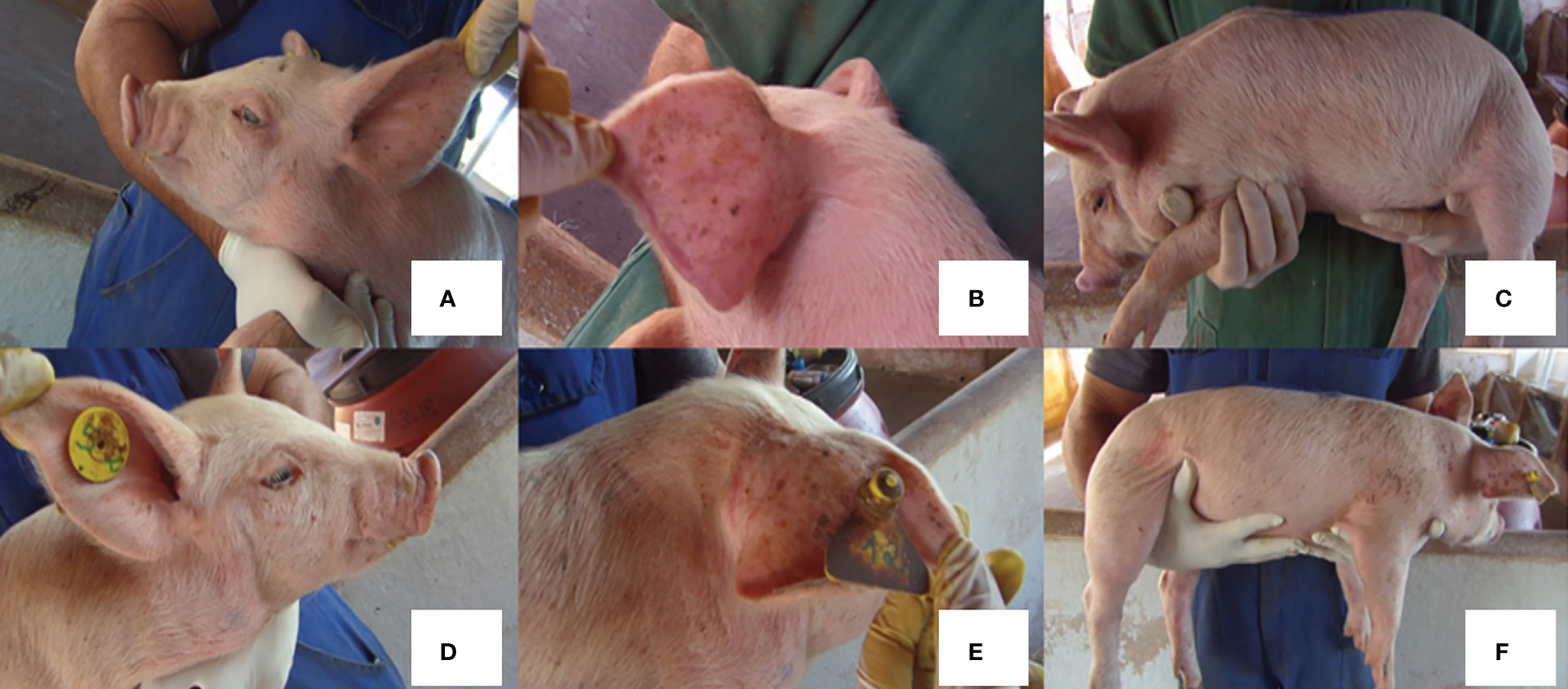
Figure 2 Picture of both body sides of piglets (Right and Left) used to count skin lesions: face (A, D); external ear (B, E); and body (C, F).
2.2.2 Nociceptive pressure threshold
At 34 days of age, all piglets were subjected to a mechanical stimulation test using a portable force transducer equipped with a polypropylene tip to assess the nociceptive threshold (electronic von Frey model EFF 301, Insight max. 10N). The animals were stimulated on the plantar pad and the leg adjacent to the tail on the right and left sides (Figure 1) (Mette and Julie, 2010; Parada et al., 2019). Piglets were individually restrained following the same approach used for skin pictures and placed on the researcher´s arms, leaving their legs to hang freely. Mechanical pressure was released when the animal showed an avoidance behavior response (tail movement and leg withdrawal) to the stimulus. A trained person blinded to the treatment groups performed the nociceptive data collection, including latency to the piglet´s response and pressure measured in grams. Nociceptive threshold pressure values were analyzed in each of the four regions (plantar pad or the leg adjacent to the tail, on the right or left side) and variables were combined to assess the largest regions. The combined variables were the result of averages of the original regions as seen in Figure 1 called the Mean (Mean = a+b+c+d/4), Right side (Right = c+d/2), Left side (Left = a+b/2) Legs (Legs = a+c/2), and Pad sides (Pad = b+d/2).
2.3 Paternity test
For the paternity test, hair samples were collected from the lumbar region of each boar and from all piglets at the end of the experiment. Samples were sent to NEOGEN® (Indaiatuba, São Paulo) for single nucleotide polymorphism (SNP) analysis using the SNP panel developed by NEOGEN® and Illumina beachip technology (Infinium). These results were used to allocate data for all piglets to boar treatment groups for subsequent analysis.
2.4 Statistical analysis
A generalized linear model with a negative binomial distribution was used to compare the numbers of live-born and weaned piglets and their sex, mortality (removed the outlier), and skin lesion counts among the treatment groups. The models included fixed effects for treatment, semen quality, sex, and weight. A Kruskal-Wallis test was applied to analyze the salivary cortisol concentration ratio (the ratio was calculated by dividing the salivary cortisol concentration value before by salivary cortisol concentration after the weaning) and nociceptive threshold data (when significant, Dunn’s test was used to compare treatments). For all statistical analyses performed, a 5% level of significance was adopted, and a trend was considered when a 10% level was found.
3 Results
3.1 Performance and salivary cortisol concentration
Regarding performance results, one outlier boar from the P group was removed. A total of 139 piglets were born alive, with a higher number of piglets sired by boars in the E group than in the P group (E = 12.7 ± 2.1; P = 4.2 ± 1.2; p = 0.002 and C = 9.2 ± 1.3). The total pre-weaning mortality rate was 20.1%, a rate expected for outdoor systems mainly related to crushing (Baxter et al., 2012b; Nicolaisen et al., 2019). A total of 111 piglets were weaned, with more weaned piglets born from boars in the E group than the P group (E = 10.8 ± 1.78; P = 3 ± 0.95; p = 0.001 and C = 6.8 ± 1.3; see Table 1).
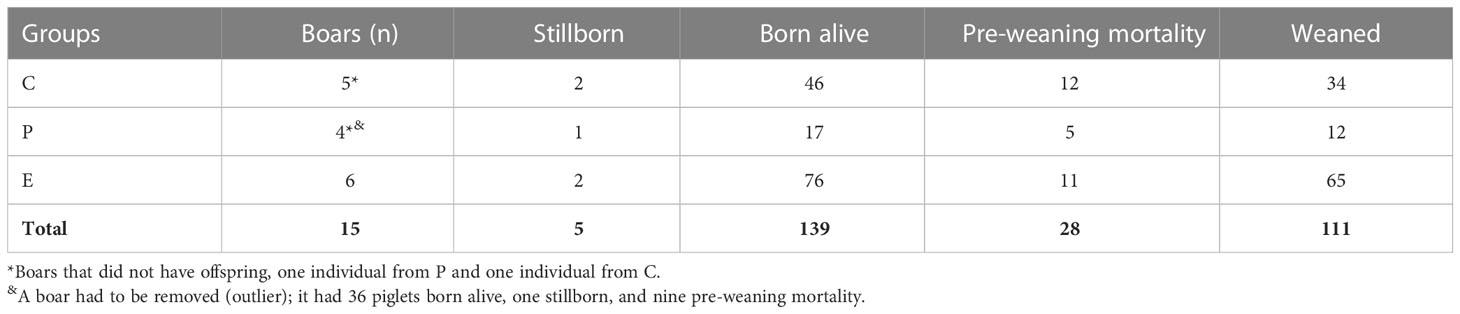
Table 1 Total numbers of stillborn, born alive, pre-weaning mortality, and weaned piglets by boars’ treatment (Crates = C; Pens = P; and Enriched pens = E).
The salivary cortisol concentrations for each group of piglets at pre-weaning were C = 9.35 ± 9.7, P = 7.23 ± 6.2, and E = 18.27 ± 32.4 pg/50µl and at post-weaning were C =10.30 ± 8.6, P = 11.67 ± 18.7, and E = 19.26 ± 23.0 pg/50µl. No differences were found in the salivary cortisol concentration when comparing groups at pre-weaning (p = 0.443), post-weaning (p = 0.285), and the ratio between pre and post-weaning (p = 0.758).
3.2 Skin lesions and nociception pressure threshold
A trend for the number of skin lesions on the left side of the body on the day of weaning was observed between the P and C groups (P < C; p = 0.053). In addition, some effects of semen quality on the skin lesion variables were observed on the first, 2nd, and 4th days after weaning (p < 0.05), as well as effects of piglet weight on the day of weaning (p < 0.05) and on the 3rd day after weaning (p < 0.05; see Supplementary Table 1).
The nociceptive threshold pressure values measured for the right and left legs of the piglets from the P group were higher than those of the piglets from the E and C groups (p ≤ 0.001; see Table 2). Piglets from the P group had fewer right leg withdrawal events than those in the E and C groups (p = 0.008; see Table 3).
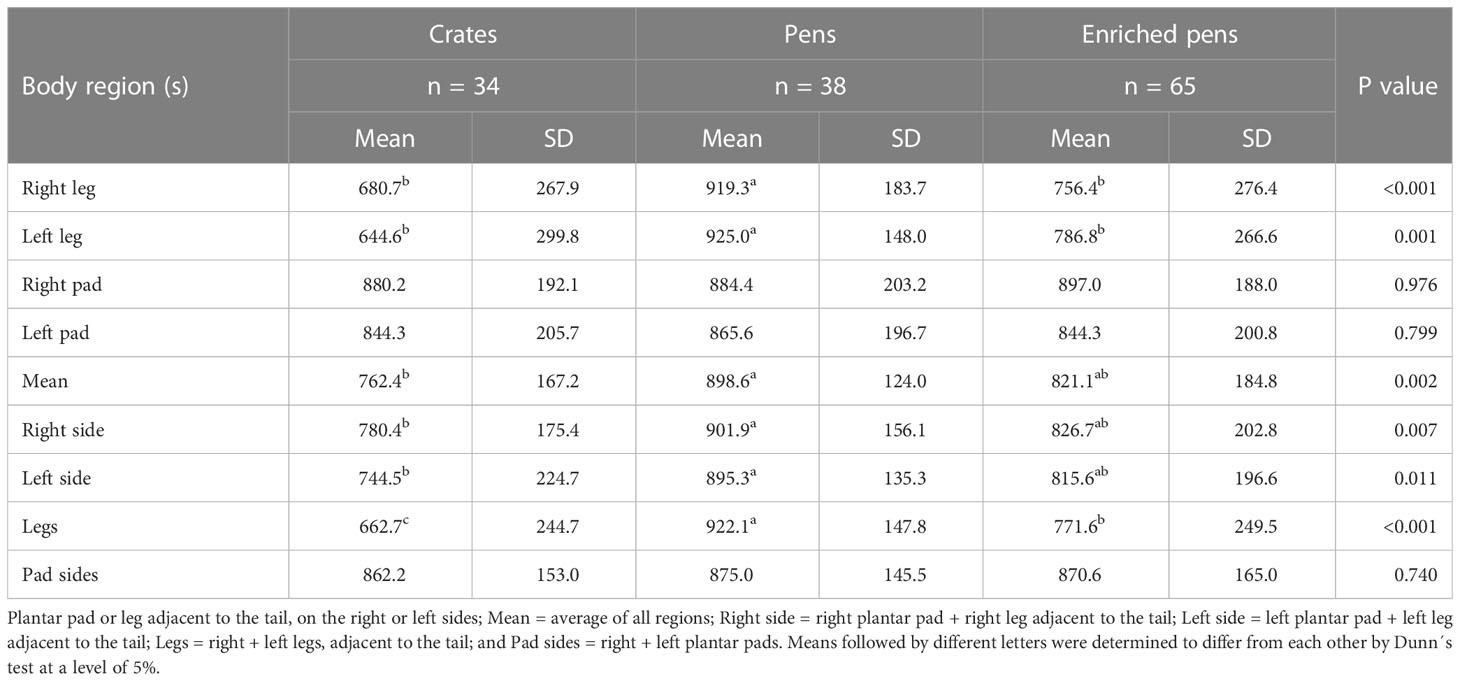
Table 2 Statistics of the piglets’ nociceptive threshold pressure values presented in grams and divided by boar treatment group.
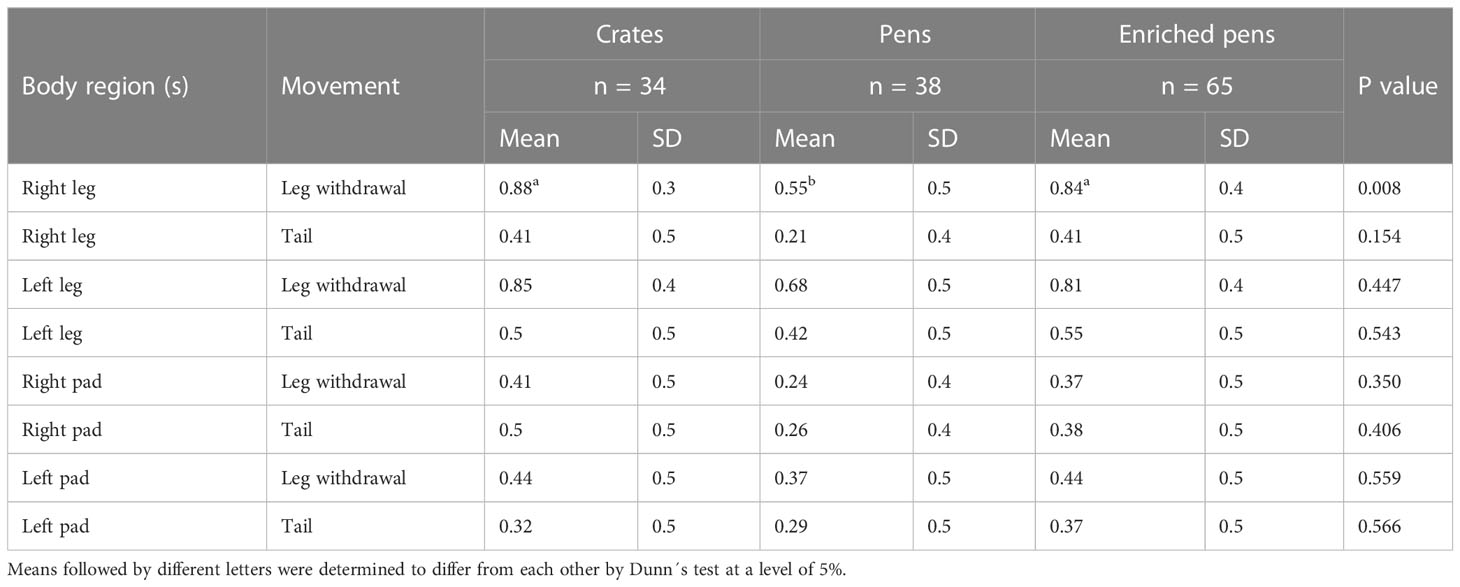
Table 3 Statistics of the piglets’ movement behavior (as events) movement behavior values for the nociceptive threshold by treatment group.
In Table 2, piglets in the P group showed higher nociceptive values for both sides of the body than those in the C group (p = 0.002). The nociceptive pressure values of all treatment groups differed in the both legs adjacent to the tail (P > E > C; p < 0.001; see Table 2).
Regarding time responses, the piglets in the P group took longer to react than those in the E and C groups (p = 0.004; p = 0.015, respectively) when the right leg and legs adjacent to the tail were pressed. Piglets in the P group took longer to respond on the right side than those in the C group (p = 0.033), with no differences from the E group (Table 4). No differences were found in the remaining comparisons.
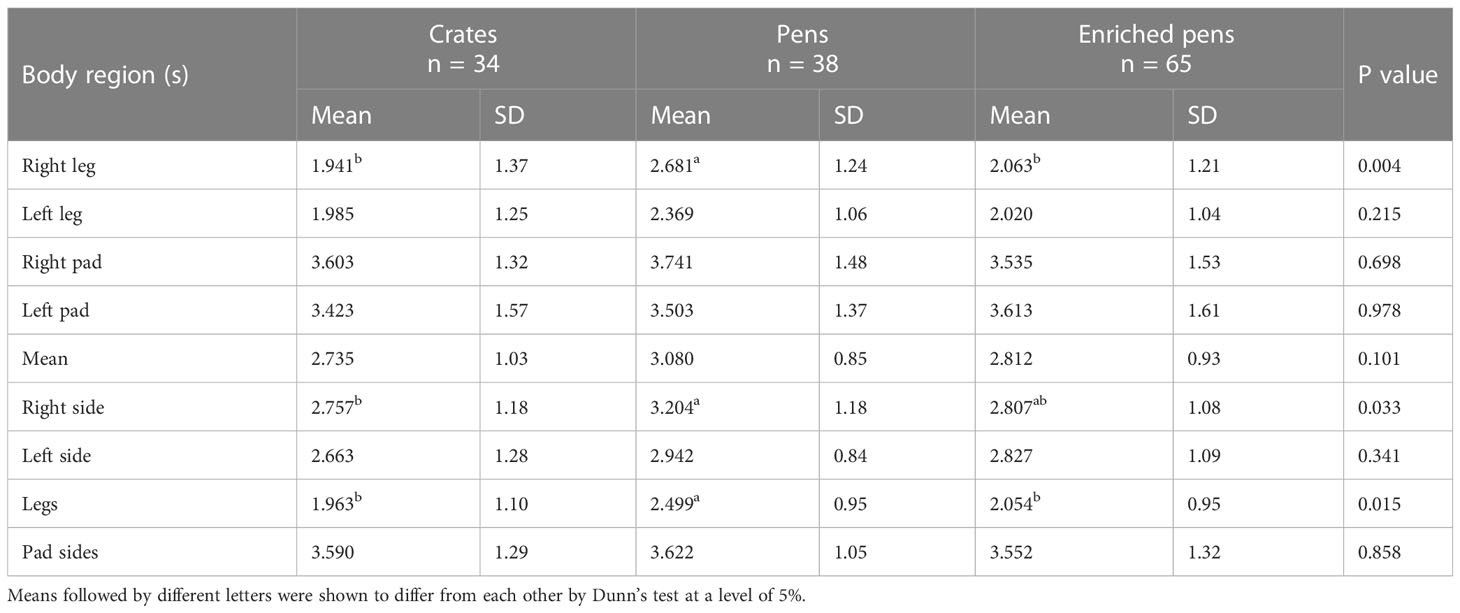
Table 4 Values of reaction time in seconds for nociception threshold perception in the piglets per treatment group.
4 Discussion
In the present study, we found paternal effects on the birth rate, survival, and responsiveness to cope with challenges in offspring, which were evident based on the skin lesions and the differences in nociceptive thresholds. The test results are likely affected by the difference in the early mortality patterns, which may have eliminated some extreme phenotypes. The present results indicated that boars housed under an enriched environment sired more piglets, and their offspring showed intermediary responses to potentially noxious stimuli. These traits are important pillars to define animals’ resilience in adapting to environmental factors throughout their lives, especially those in commercial farm production (Edwards, 2002). Despite not having found differences between sexes in piglet mortality, we observed the effect of fathers’ environmental exposure on the number of live-born and weaning piglets, suggesting a potential impact linked to the boars’ environment and management. To the best of our knowledge, no previous study has evaluated the effects of boar housing conditions on piglet birth rates.
As reported by Bernardino et al. (2022), boars housed in crates had greater perfusion of the testicular parenchyma and higher velocity linear curve values in sperm, which can contribute to reduced fertility. The review by Nätt et al (Nätt and Öst, 2020). on male metabolic and reproductive health affecting progeny discusses mechanisms such as microRNAs that can alter embryo development and, consequently, individual metabolism. In humans, there is a transgenerational effect related to sex, where food supply increases the risk of male offspring mortality (Pembrey et al., 2006). In addition, restrictions on the mating preferences of male rats showed that they produce smaller offspring with lower viability and worse performance when mated with females they do not prefer (Gowaty et al., 2003). Studies with sows indicate that the mortality of male piglets is higher despite the more significant maternal investment, and they are more susceptible to mortality factors such as crushing (Baxter et al., 2012a).
In the present study, we found paternal effects on offspring responsiveness to cope with challenges, as evidenced by skin lesion results and differences in nociceptive thresholds. Offspring sired by boars in the P group showed fewer skin lesions on the left side (p=0.053), indicating that the environment of the boar during the precopulation period could have effects on reducing engagement in agonistic interactions. It has been reported that agonistic behavior negatively impacts physiology, performance and animal welfare (Broom, 1986). Thus, piglets born from the boars in the P group had better chances of experiencing good welfare and coping with social challenges. In addition, piglets born from the boars in the P group engaged in fewer agonistic interactions, evidenced by presenting lower number of skin lesions. It is relevant to mention that due to our experimental design, the piglets from different litters could interact with each other during the lactation period, which could attenuate the number of skin lesions at weaning, creating familiarity between individuals and acting as a positive factor for their welfare (Camerlink et al., 2013).
The measurement of skin lesions in pigs is an indirect and validated method to assess aggressive behavior, indicating that fewer skin lesions in mixed piglets after weaning indicates these piglets’ capacity to avoid aggressive behavior toward pen mates (Turner et al., 2006) We have evidence that the prenatal environment can modulate the aggressive behavior of piglets (Bernardino et al., 2016). The mechanisms are not completely understood. Epigenetic modulation could be a likely mechanism to explain our findings; aggressive phenotypes in pigs have been associated with the expression of many genes (Balaban et al., 1996; Peter et al., 2005), which may be indicative that this is a heritable trait that, when expressed, has effects on other behavioral features (Turner et al., 2008; Turner et al., 2009). Furthermore, the results of previous studies have indicated that factors such as sex (Weller et al., 2019), family group (Camerlink et al., 2013), group size (Samarakone and Gonyou, 2009), lower performance in sows (Tönepöhl et al., 2013), and hunger (Verdon et al., 2015; Bernardino et al., 2016) are associated with the number of skin lesions.
Regarding the results of the nociceptive threshold, which is a proxy measure for behavioral responses to potentially noxious stimuli, offspring born from the boars in the crate group had significantly lower nociceptive values in the Legs areas than those born from the boars in the two pen treatment groups, indicating that crates were sufficient in creating more reactive phenotypes. The offspring of the boars in the enriched environment group, with more opportunities to carry out natural behavior, had intermediate values when compared to those from boars in the other treatment groups and surprisingly some values are even significantly higher than the P group. Nociception is the detection of potentially injurious stimuli and plays an important role as a self-protective mechanism (Sneddon, 2004). Up or down alterations in the perception of these stimuli can result in hyper- or hypo-analgesic phenotypes, mis-adapting the protection mechanism in the face of potentially harmful challenges. For example, routine painful experiences are presented in commercial swine production (Ison et al., 2016). In Intense World Theory (Markram and Markram, 2010), environmental perception difficulties, such as autism in humans, are theorized to be the cognitive consequences of hyperperception, leading to individuals with less resilience to cope with common challenges. In this way, the poor environment provided to boars in the C group seemed to cause the worst perceptual adjustment of offspring to harmful stimuli.
The results from previous studies indicated that there was a correlation between the prenatal maternal environment and the resulting consequences in nociceptive threshold and behavior responses in pigs (Rutherford et al., 2014). Surprisingly, none of these studies performed an assessment of the effects of the environment in which the boars were housed on their progeny. Several studies have focused on maternal effects on the nociceptive threshold of offspring; piglets sired by lame sows or those produced by socially stressed gilts, where stress was imposed during the second-third of gestation, had larger nociceptive values in response to mechanical noxious stimuli (Sandercock et al., 2011; Parada et al., 2019). These findings are indicative of the possibility that the prenatal maternal environment has effects on nociception in offspring. Therefore, it is possible that the precopulatory environment of boars also contributes to the modulation of responses to noxious stimuli in their offspring. Previous studies showed that parents with stressful lifestyle or exposed to some dietary factors could show altered epigenetics marks on sperm, which could modulate the offspring phenotype (Chan et al., 2020). There are evidences that small RNAs from the extracellular vesicles from epididymis can shape the life trajectory of offspring (Chan et al., 2020). We decided to wait, at least, three complete epididymal transit of sperm (in pigs the epididymal transit is 10 days) to use the ejaculate for insemination. Moreover, other part of the study was carried out to understand the impact of time for the reported housing conditions (Bernardino, 2021). Thus, boars exposed to environments rich in stimuli may transmit information so their progeny can recognize environmental stimuli without responding excessively, based on the findings of our study.
The environmental effects have been studied in broad aspects depending on the quality of life of the parents, assessing issues related to behavioral, neurobiological, and metabolic changes for different species (Siddeek et al., 2018). In the present study, different ways to improve the environmental conditions of the boars were related to alterations in the ability of their offspring to cope with adverse conditions. The opportunity for boars to manipulate hay in the enriched pens and its possible nutritional effect, along with positive human-animal interaction, the possibility of carrying out species-specific behaviors, and the improvement in thermoregulation from showering, likely played a synergistic role in our findings. Although we cannot effectively discriminate the effects from each individual enrichment provided to boars, the main point is to demonstrate that the environmental conditions of parents (males in this case) modulate the phenotype of offspring. In a recent study, it was shown that boars fed chicory herb diet supplements had offspring with fewer skin lesions, suggesting fewer agonist interactions. However, the authors disagree regarding the action of the calming components of the plants or even regarding the action of fiber on prolonging the digestibility of food (Aikins-Wilson et al., 2022). Furthermore, there may be a role of the gut microbiota in modulating aggressiveness, as changes in the diversity of the microbiota were observed between biter, victim, and control pigs (Verbeek et al., 2021).
Similarly, piglets born to sows fed a high fiber diet during gestation had fewer skin lesions at weaning when compared with those born to sows that did not receive additional fiber in their diets (Bernardino et al., 2016). Moreover, in the study conducted by Parada et al. (2019) (Sarmiento et al., 2021), offspring born to sows without lameness during gestation had fewer skin lesions than offspring born to sows with lameness.
The findings from the present study are a biological integrative indicator for understanding adaptive responses of animals in relation to environmental conditions experienced by their progenitors. Integrative mechanisms associated with molecular markers of epigenetic changes in other species have been proposed (Braun and Champagne, 2014). Epigenetic effects in males and females can alter phenotype characteristics related to coping mechanisms that can be transmitted from one generation to the next through DNA-independent routes and mechanisms (Schagdarsurengin and Steger, 2016; Tatemoto and Guerrero-Bosagna, 2018) and/or miRNAs. Epigenetic mechanisms may alter the coping strategies of piglets in response to stimuli with maternal or paternal effects. Individuals who are not stressed or who are not affected by stress are more likely to produce offspring with the same genetic characteristics as their parents (Bateson, 1991). Furthermore, boar housing conditions in commercial systems should receive more scrutiny. This is a relevant concern that must be taken into consideration by the swine industry when promoting sustainability.
5 Conclusions
Boars housed in environments that allow them to perform behaviors close to their biology produce more piglets, lower pre-weaning mortality rates, and intermediate responses to potentially noxious stimuli (nociceptive threshold). These results indicate the necessity to improve boars’ welfare, as their housing conditions can interfere with their offspring’s behavior, physiology, coping styles, and welfare. These findings are from ongoing research investigating different approaches to understand specific molecular mechanisms underlying the paternal effect on progeny. The possibility that the environmental conditions to which males are subjected can lead to modulation of their offspring phenotypes is relevant to all species, including humans.
Data availability statement
The raw data supporting the conclusions of this article will be made available by the authors, without undue reservation.
Ethics statement
The animal study was reviewed and approved by Ethics Committee on Animal Use from the School of Veterinary Medicine and Animal Science (FMVZ) of the University of São Paulo (USP) under the number 6555081018.
Author contributions
LS and TB contributed to the original draft, writing the paper, analyzing and interpreting data, acquiring data, experimental work, designing the work, and preparing the figures. MPS, SF, and BB contributed to the acquisition of data and experimental work. CL and GG contributed to the definition of the statistical approach, analysis, data interpretation, and figure preparation. RP contributed to the interpretation of the data, preparation of the figures, and writing of the paper. AZ contributed to the original draft, grant writing, fund acquisition, acquiring data, experimental work, experimental design, development of the concept, and data interpretation. All the authors contributed to the revision of the final version.
Funding
This project was sponsored with a grant awarded to AZ from the Fundação de Amparo à Pesquisa do Estado de São Paulo (FAPESP), process number 2018/01082-04. LS received a scholarship from the Coordenação de Aperfeiçoamento de Pessoal de Nível Superior - Brazil (CAPES) – (CAPES/PROEX Funding Code 1841/2016). TB received a scholarship from the Fundação de Amparo à Pesquisa do Estado de São Paulo (FAPESP) under process number 2017/05604-2. MPS was granted a PhD scholarship from the University of Teramo in Italy.
Acknowledgments
We are grateful to Certified Humane, which provided partial funds for paying for the paternity tests and purchasing the animals. We are grateful to the Brazilian farm TOPGEN that granted access to the animals used in the experiment. Vedovati Pisos is acknowledged for donating the rubber mats that were used in the experimental work. Professor Dagmar Waberski, University of Veterinary Medicine Hannover, Professor André César Furugen de Andrade, Professor Camila Squarzoni Dale, and Cesar Augusto Pospissil Garbossa from the School of Veterinary and Animal Science, USP, helped with advice, laboratory access and materials for semen dilution and insemination of the gilts. Biorender software was used to create drawings. Finally, we thank all students, professors, and staff involved in this research at the University of São Paulo.
Conflict of interest
The authors declare that the research was conducted without any commercial or financial relationships that could be construed as a potential conflict of interest.
Publisher’s note
All claims expressed in this article are solely those of the authors and do not necessarily represent those of their affiliated organizations, or those of the publisher, the editors and the reviewers. Any product that may be evaluated in this article, or claim that may be made by its manufacturer, is not guaranteed or endorsed by the publisher.
Supplementary material
The Supplementary Material for this article can be found online at: https://www.frontiersin.org/articles/10.3389/fanim.2023.1142628/full#supplementary-material
References
Aikins-Wilson S., Bohlouli M., Engel P., König S. (2022). Effects of an herbal diet, diet x boar line and diet x genotype interactions on skin lesions and on growth performance in post-weaning pigs using a cross-classified experiment. Livest Sci. 263, 105010 doi: 10.1016/j.livsci.2022.105010
Balaban E., Alper J. S., Kasamon Y. L. (1996). Mean genes and the biology of aggression: A critical review of recent animal and human research. J. Neurogenet 11, 1–43. doi: 10.3109/01677069609107061
Bale T. L. (2015). Epigenetic and transgenerational reprogramming of brain development. Nat. Publ Gr 16, 332–344. doi: 10.1038/nrn3818
Bateson P. (1991). Assessment of pain in animals. Anim. Behav. 49, 1–21. doi: 10.1007/978-1-60761-880-5_1
Baxter E. M., Jarvis S., Palarea-Albaladejo J., Edwuards S. A., Edwards S. A. (2012a). The weaker sex? the propensity for male-biased piglet mortality. PloS One 7, e30318. doi: 10.1371/journal.pone.0030318
Baxter E. M., Lawrence A. B., Edwards S. A. (2012b). Alternative farrowing accommodation: Welfare and economic aspects of existing farrowing and lactation systems for pigs. Animal 6, 96–117. doi: 10.1017/S1751731111001224
Bernardino T. (2021). Boar welfare influence the quality of the gametes. [Ph.D. thesis]. [São Paulo (SP), Brazil]: University of São Paulo. 1–109. doi: 10.11606/T.10.2021.tde-15032022-145834
Bernardino T., Tatemoto P., Morrone B., Mazza Rodrigues P. H., Zanella A. J. (2016). Piglets born from sows fed high fibre diets during pregnancy are less aggressive prior to weaning. PloS One 11, e0167363. doi: 10.1371/journal.pone.0167363
Bernardino T., Teodoro P. C. C., Batissaco L., Celeghini E. C. C., Zanella A. J. (2022). Poor welfare compromises testicle physiology in breeding boars. PLoS One 17, e0268944. doi: 10.1371/journal.pone.0268944
Braun K., Champagne F. A. (2014). Paternal influences on offspring development : behavioural and epigenetic pathways neuroendocrinology. J. Neuroendocrinol 26, 697–706. doi: 10.1111/jne.12174
Braunschweig M., Jagannathan V., Gutzwiller A., Bee G. (2012). Investigations on transgenerational epigenetic response down the Male line in F2 pigs. PloS One 7, e30583. doi: 10.1371/JOURNAL.PONE.0030583
Broom D. D. M. (1986). Indicators of poor welfare. Br. Vet. J. 142, 524–526. doi: 10.1016/0007-1935(86)90109-0
Broom D. M., Mendl M. T., Zanella A. J. (1995). A comparison of the welfare of sows in different housing conditions. Anim. Sci. 61, 369–385. doi: 10.1017/S1357729800013928
Camerlink I., Turner S. P., Bijma P., Bolhuis J. E. (2013). Indirect genetic effects and housing conditions in relation to aggressive behaviour in pigs. PloS One 8, e65136. doi: 10.1371/journal.pone.0065136
Campbell J. M., Crenshaw J. D., Polo J. (2013). The biological stress of early weaned piglets. J. Anim. Sci. Biotechnol. 4, 1–4. doi: 10.1186/2049-1891-4-19/METRICS
Chan J. C., Morgan C. P., Adrian Leu N., Shetty A., Cisse Y. M., Nugent B. M., et al. (2020). Reproductive tract extracellular vesicles are sufficient to transmit intergenerational stress and program neurodevelopment. Nat. Commun. 11, 1499. doi: 10.1038/s41467-020-15305-w
de Souza Farias S., Montechese A. C. D., Bernardino T., Rodrigues P. H. M., de Araujo Oliveira C. A., Zanella A. J. (2021). Two hours of separation prior to milking: Is this strategy stressful for jennies and their foals? Animals 11, 1–17. doi: 10.3390/ani11010178
Donkin I., Barrès R. (2018). Sperm epigenetics and influence of environmental factors. Mol. Metab. 14, 1–11. doi: 10.1016/j.molmet.2018.02.006
Edwards S. A. (2002). Perinatal mortality in the pig : Environmental or physiological solutions? Livest Prod. Sci. 78, 312. doi: 10.1016/S0301-6226(02)00180-X
Gowaty P. A., Drickamer L. C., Schmid-Holmes S. (2003). Male House mice produce fewer offspring with lower viability and poorer performance when mated with females they do not prefer. Anim. Behav. 65, 95–103. doi: 10.1006/anbe.2002.2026
Guerrero-Bosagna C., Pértille F., Gomez Y., Rezaei S., Gebhardt-Henrich S. G., Vögeli S., et al. (2020). DNA Methylation variation in the brain of laying hens in relation to differential behavioral patterns. Comp. Biochem. Physiol. - Part D Genomics Proteomics 35, 100700. doi: 10.1016/j.cbd.2020.100700
Guy J. H., Burns S. E., Barker J. M., Edwuards S. A., Edwards S. A. (2009). Reducing post-mixing aggression and skin lesions in weaned pigs by application of a synthetic maternal pheromone. Anim. Welf 18, 249–255.
Ison S. H., Eddie Clutton R., Di Giminiani P., Rutherford K. M. D. (2016). A review of pain assessment in pigs. Front. Vet. Sci. 3. doi: 10.3389/fvets.2016.00108
Khezri A., Narud B., Stenseth E. B., Johannisson A., Myromslien F. D., Gaustad A. H., et al. (2019). DNA Methylation patterns vary in boar sperm cells with different levels of DNA fragmentation. BMC Genomics 20, 1–15. doi: 10.1186/s12864-019-6307-8
Lacal I., Ventura R. (2018). Epigenetic inheritance: concepts, mechanisms and perspectives. Front. Mol. Neurosci. 11. doi: 10.3389/fnmol.2018.00292
Markram K., Markram H. (2010). The intense world theory - a unifying theory of the neurobiology of autism. Front. Hum. Neurosci. 4. doi: 10.3389/fnhum.2010.00224
Mette S. H., Julie S. R. (2010). Effect of buprenorphine and fentanyl in experimental induced superficial, deep and hyperalgesic pain. Scand. J. Pain 1, 174–175. doi: 10.1016/j.sjpain.2010.05.026
Murphy E., Melotti L., Mendl M. (2021). “Assessing emotions in pigs: determining negative and positive mental states,” in Understanding the behaviour and improving the welfare of pigs. Ed. Edwards S. (Burleigh Dodds Science Publishing), 455496. doi: 10.1201/9781003048220
Nätt D., Öst A. (2020). Male Reproductive health and intergenerational metabolic responses from a small RNA perspective. J. Intern. Med. 288, 305–320. doi: 10.1111/joim.13096
Nery da Silva A., Silva Araujo M., Pértille F., Zanella A. J. (2021). How epigenetics can enhance pig welfare? Animals 12, 32. doi: 10.3390/ani12010032
Nicolaisen T., Lühken E., Volkmann N., Rohn K., Kemper N., Fels M. (2019). The effect of sows’ and piglets’ behaviour on piglet crushing patterns in two different farrowing pen systems. Animals 9, 538. doi: 10.3390/ani9080538
Nilsson E. E., Sadler-Riggleman I., Skinner M. K. (2018). Environmentally induced epigenetic transgenerational inheritance of disease. Environ. Epigenet. 4, 1–13. doi: 10.1093/eep/dvy016
Otten W., Kanitz E., Tuchsherer M. (2015). The impact of pre-natal stress on offspring development in pigs. J. Agric. Sci. 153, 907–919. doi: 10.1017/S0021859614001361
Parada M., Sabei L., Stanigher B., Zanella A. J. (2019). Lameness in sows during pregnancy impacts welfare outcomes in their offspring. Proceedings of the 53a Congress of the ISAE; (Bergen), 78. doi: 10.3920/978-90-8686-889-6
Pedersen L. J. (2018). Overview of commercial pig production systems and their main welfare challenges. Adv. Pig Welf. 2018, 3–25. doi: 10.1016/B978-0-08-101012-9.00001-0
Pembrey M. E., Bygren L. O., Kaati G., Edvinsson S., Northstone K., Sjöström M., et al. (2006). Sex-specific, male-line transgenerational responses in humans. Eur. J. Hum. Genet. 14, 159–166. doi: 10.1038/sj.ejhg.5201538
Pértille F., Alvarez-Rodriguez M., da Silva A. N., Barranco I., Roca J., Guerrero-Bosagna C., et al. (2021). Sperm methylome profiling can discern fertility levels in the porcine biomedical model. Int. J. Mol. Sci. 22, 1–26. doi: 10.3390/ijms22052679
Peter L., Holm L., Lindstr B., Thodberg K., Su G., Rydhmer L. (2005). Aggressive behaviour of sows at mixing and maternal behaviour are heritable and genetically correlated traits. Livest Prod Sci. 93, 73–85. doi: 10.1016/j.livprodsci.2004.11.008
Rodgers A. B., Morgan C. P., Bronson S. L., Revello S., Bale T. L. (2013). Paternal stress exposure alters sperm microRNA content and reprograms offspring HPA stress axis regulation. J. Neurosci. 33, 9003–9012. doi: 10.1523/JNEUROSCI.0914-13.2013
Rutherford K. M. D., Piastowska-ciesielska A., Donald R. D., Robson S. K., Ison S. H., Jarvis S., et al. (2014). Prenatal stress produces anxiety prone female offspring and impaired maternal behaviour in the domestic pig. Physiol. Behav. 129, 255–264. doi: 10.1016/j.physbeh.2014.02.052
Rutherford K. M. D. D., Robson S. K., Donald R. D., Jarvis S., Sandercock D. A., Scott E. M., et al. (2009). Pre-natal stress amplifies the immediate behavioural responses to acute pain in piglets. Biol. Lett. 5, 452–454. doi: 10.1098/rsbl.2009.0175
Samarakone T. S., Gonyou H. W. (2009). Domestic pigs alter their social strategy in response to social group size. Appl. Anim. Behav. Sci. J. 121, 8–15. doi: 10.1016/j.applanim.2009.08.006
Sandercock D. A., Gibson I. F., Rutherford K. M. D. D., Donald R. D., Lawrence A. B., Brash H. M., et al. (2011). The impact of prenatal stress on basal nociception and evoked responses to tail-docking and inflammatory challenge in juvenile pigs. Physiol. Behav. 104, 728–737. doi: 10.1016/j.physbeh.2011.07.018
Sarmiento M. P., Bernardino T., Tatemoto P., Polo G., Zanella A. J. (2021). The in-utero experience of piglets born from sows with lameness shapes their life trajectory. Sci. Rep. 11, 1–11. doi: 10.1038/s41598-021-92507-2
Schagdarsurengin U., Steger K. (2016). Epigenetics in male reproduction : effect of paternal diet on sperm quality and offspring health. Nat. Publ Gr 13, 584–595. doi: 10.1038/nrurol.2016.157
Siddeek B., Mauduit C., Simeoni U., Benahmed M. (2018). Sperm epigenome as a marker of environmental exposure and lifestyle, at the origin of diseases inheritance. Mutat. Res. - Rev. Mutat. Res. 778, 38–44. doi: 10.1016/j.mrrev.2018.09.001
Siegford J. M., Rucker G., Zanella A. J. (2008). Effects of pre-weaning exposure to a maze on stress responses in pigs at weaning and on subsequent performance in spatial and fear-related tests. Appl. Anim. Behav. Sci. 110, 189–202. doi: 10.1016/j.applanim.2007.03.022
Skinner M. K., Guerrero-Bosagna C. (2009). Environmental signals and transgenerational epigenetics. Epigenomics 1, 111–117. doi: 10.2217/epi.09.11
Sneddon L. U. (2004). Evolution of nociception in vertebrates: Comparative analysis of lower vertebrates. Brain Res. Rev. 46, 123–130. doi: 10.1016/j.brainresrev.2004.07.007
Tatemoto P., Bernardino T., Alves L., de Oliveira Souza A. C., José Zanella A. (2019a). Environmental enrichment for pregnant sows modulates HPA-axis and behavior in the offspring. Appl. Anim. Behav. Sci 220. doi: 10.1016/j.applanim.2019.104854
Tatemoto P., Bernardino T., Alves L., Zanella A. J. (2019b). Sham-Chewing in Sows Is Associated With Decreased Fear Responses in Their Offspring. Front. Vet. Sci. 6, 1–9 doi: 10.3389/fvets.2019.00390
Tatemoto P., Guerrero-Bosagna C. (2018). “Biological dogmas in relation to the origin of evolutionary novelties,” in Origin and evolution of biodiversity. (Springer International Publishing), 317–330. doi: 10.1007/978-3-319-95954-2_17/COVER
Tönepöhl B., Appel A. K., Voß B., König U., Borstel V., Gauly M. (2013). Interaction between sows ‘ aggressiveness post mixing and skin lesions recorded several weeks later. Appl. Anim. Behav. Sci. 144, 108–115. doi: 10.1016/j.applanim.2013.01.004
Turner S. P., Farnworth M. J., White I. M. S., Brotherstone S., Mendl M., Knap P., et al. (2006). The accumulation of skin lesions and their use as a predictor of individual aggressiveness in pigs. Appl. Anim. Behav. Sci. 96, 245–259. doi: 10.1016/j.applanim.2005.06.009
Turner S. P., Roehe R., D’Eath R. B., Ison S. H., Farish M., Jack M. C., et al. (2009). Genetic validation of postmixing skin injuries in pigs as an indicator of aggressiveness and the relationship with injuries under more stable social conditions. J. Anim. Sci. 87, 3076–3082. doi: 10.2527/jas.2008-1558
Turner S. P., Roehe R., Mekkawy W., Farnworh M. J., Knap P. W., Lawrencw A. B., et al. (2008). Bayesian Analysis of genetic associations of skin lesions and behavioural traits to identify genetic components of individual aggressiveness in pigs. Behav. Genet. 38, 67–75. doi: 10.1007/s10519-007-9171-2
Verbeek E., Keeling L., Landberg R., Lindberg J. E., Dicksved J. (2021). The gut microbiota and microbial metabolites are associated with tail biting in pigs. Sci. Rep. 11, 1–14. doi: 10.1038/s41598-021-99741-8
Verdon M., Hansen C. F., Rault J. L., Jongman E., Hansen L. U., Plush K., et al. (2015). Effects of group housing on sow welfare: A review. J. Anim. Sci. 93, 1999–2017. doi: 10.2527/jas.2014-8742
Keywords: piglets, fetal programming, paternal stress, stress inheritance, welfare
Citation: Sabei L, Bernardino T, Parada Sarmiento M, Barbosa BS, Farias SS, Ghantous GF, de Lima CG, Poletto R and Zanella AJ (2023) Life experiences of boars can shape the survival, aggression, and nociception responses of their offspring. Front. Anim. Sci. 4:1142628. doi: 10.3389/fanim.2023.1142628
Received: 11 January 2023; Accepted: 28 March 2023;
Published: 11 April 2023.
Edited by:
Kyle McLean, The University of Tennessee, Knoxville, United StatesReviewed by:
Emma Fàbrega Romans, Institute of Agrifood Research and Technology (IRTA), SpainCécile Bienboire-Frosini, Institut de Recherche en Sémiochimie et Ethologie Appliquée (IRSEA), France
Copyright © 2023 Sabei, Bernardino, Parada Sarmiento, Barbosa, Farias, Ghantous, de Lima, Poletto and Zanella. This is an open-access article distributed under the terms of the Creative Commons Attribution License (CC BY). The use, distribution or reproduction in other forums is permitted, provided the original author(s) and the copyright owner(s) are credited and that the original publication in this journal is cited, in accordance with accepted academic practice. No use, distribution or reproduction is permitted which does not comply with these terms.
*Correspondence: Adroaldo José Zanella, adroaldo.zanella@usp.br; Leandro Sabei, sabei_le@alumni.usp.br
†These authors contributed equally to this work and share first authorship