- 1Department of Animal Science, University of Tennessee, Knoxville, TN, United States
- 2Department of Animal Science, Texas A&M University, College Station, TX, United States
Once thought to be sterile, the reproductive tract microbiome has been characterized due to the transition from culture-dependent identification of bacteria to culture-independent sequencing methods. The urogenital microbiome was first identified in women through the Human Microbiome Project, which led to research in other species such as the bovine. Previous research focused on uterine bacteria associated with postpartum disease, but next generation sequencing methods identified a normal, healthy bacterial community of the reproductive tract of cows and heifers. Bacterial communities are now understood to differ between the uterus and vagina, and throughout the estrous cycle with changes in hormone dominance. In a healthy state, the bacterial communities largely interact with the uterine environment by assisting in maintaining the proper pH, providing and utilizing nutrients and metabolites, and influencing the immunological responses of the reproductive tract. If the bacterial communities become unbalanced due to an increase in potentially pathogenic bacteria, the health and fertility of the host may be affected. Although the presence of a reproductive tract microbiome has become widely accepted, the existence of a placental microbiome and in utero colonization of the fetus is still a popular debate due to conflicting study results. Currently, researchers are evaluating methods to manipulate the reproductive bacterial communities, such as diet changes and utilizing probiotics, to improve reproductive outcomes. The following review discusses the current understanding of the reproductive tract microbiome, how it differs between humans and cattle, and its relationship with the uterine environment.
1 Introduction
The world population is expected to increase to almost 10 billion people by the year 2050, challenging the agricultural industry to increase sustainable food production to provide for the increased demand (United Nations, 2022). However, the land and resources required to feed the growing population are limited (Davis et al., 2016). Therefore, efficiency must improve for current agriculture practices and food production systems. Animal agriculture is a major contributor towards providing high quality protein, vitamin, and mineral quantities for the increased demand of the growing population. By improving the efficiency and sustainability of livestock production, researchers and producers will be able to provide more sustainable food production while maintaining profitability and preventing depletion of resources. Goals have been set to achieve optimal beef production and increase efficiency. Efficient cattle growth is considered as gaining an average of two pounds per day, with optimal reproductive efficiency defined as a cow producing a calf every 365 days (Diskin and Kenny, 2016). Ideally, the animal must achieve these goals with as few inputs as possible. However, these goals are often not met, with reproductive losses and reduced gains costing billions of dollars each year (Bellows et al., 2002). Currently, researchers are evaluating factors, such as the microbiome, that influence the efficiency of cattle production and developing methods to optimize animal agricultural practices.
With the development of laboratory technologies such as next generation sequencing, scientists are now better able to gain a deeper insight into the human and animal microbiomes throughout the body, detect previously indiscernible microbial species, and evaluate their relationship with host processes (Weinroth et al., 2022). The total number of microorganisms in the body have been suggested to outnumber or closely match the total number of cells in the body (Sender et al., 2016). These microbiomes contain microorganisms from all three domains of life and multiple kingdoms. However, bacteria are the largest portion of the total microbiome in abundance and contribute the greatest towards the host-microbe relationship (Hespell et al., 1997; Comtet-Marre et al., 2017). The majority of microbiome research has focused on the segments of the gastrointestinal tract and the resident bacterial community’s role in digestion. In cattle, ruminal microorganisms are the primary providers of nutrients (~70%) to the ruminant by digesting plant materials and other feeds that are not readily digested by the animal’s own enzymes (Bergman, 1990). The first goal of ruminant nutrition is to provide feeds with adequate nutrients to support the diversity of microorganisms present and optimize their fermentation. Rumen bacteria are able to provide VFA and other intermediary metabolites via fermentation which can then be used by the ruminant for production of energy (Hespell et al., 1997; Mao et al., 2015). Various factors can lead to fluctuations in the composition of the rumen microbiome, such as diet ingredients, resulting in an altered rumen environment and VFA proportions (Wang et al., 2020). Studies have also demonstrated the rumen bacterial communities differ between animals of differing feed efficiencies (Myer et al., 2015; Clemmons et al., 2022). Therefore, rumen bacterial communities play a major role in the ruminant’s overall health and production efficiency. Due to the importance of the gastrointestinal microbiome to animal performance, researchers have begun to study other microbial communities throughout the body. The following review discusses the current understanding of bacterial communities in the reproductive tract, how these microbes may be interacting with the uterine environment, and future applications of probiotics in the reproductive tract to influence reproductive success.
2 The reproductive tract microbiome
Recent efforts have evaluated resident bacterial communities in the reproductive organs and their interactions with various physiological processes, beyond the presence of disease. The uterus was previously thought to be a sterile cavity in humans and animals, with the presence of bacteria indicating infection and harmful to reproductive performance and health (Perez-Muñoz et al., 2017). In 2007, the National Institutes of Health began the Human Microbiome Project to study the microbial communities throughout multiple body systems, including the reproductive tract. The transition from culture-dependent identification of bacteria to culture-independent sequencing methods allowed for characterization of a normal, healthy reproductive tract microbiome of humans (NIH HMP Working Group et al., 2009), which led to research in multiple mammals such as primates (Yildirim et al., 2014) and ruminants (Swartz et al., 2014; Clemmons et al., 2017). Following the first characterization of the ruminant reproductive tract microbiome by Swartz et al. (2014) utilizing 16S sequencing, further studies have identified the unique microbiomes between the uterus and vagina in cattle during different periods of reproductive activity, and determined the importance of the reproductive tract microbiome towards reproductive success (Laguardia-Nascimento et al., 2015; Clemmons et al., 2017; Ault et al., 2019a; Ault et al., 2019b). Additionally, multiple phyla and genera abundances shifted between days of the protocol (Ault et al., 2019a). By gaining an understanding of these bacterial communities and the factors that influence their abundances, future studies may be able to manipulate these communities to optimize their respective environment and subsequent productivity of the animal.
2.1 Healthy bacterial communities of the reproductive tract
The reproductive tract of humans has been found to contain approximately 9% of the total microbial communities in the human body (NIH HMP Working Group et al., 2009). In contrast to other microbial communities, such as in the gastrointestinal tract and nasal airways, the human reproductive tract has a very low diversity of microbes. The low diversity is attributed to the vaginal bacterial community that is dominated by Lactobacillus species, which is typically greater than 90% abundance in healthy subjects (The Human Microbiome Project, C, 2012). The importance of Lactobacillus species for health in humans is attributed to its influence on the reproductive tract environment. Estrogen stimulates the production of glycogen by vaginal epithelial cells which is then released into the environment (Wrenn et al., 1968; Gregoire and Parakkal, 1972). Glycogen serves as a substrate for fermentation of Lactobacillus for the production of lactic acid and hydrogen peroxide. Women with greater glycogen concentrations in the vagina have increased abundance of Lactobacillus and lower vaginal pH (Mirmonsef et al., 2014). The lactic acid and hydrogen peroxide produced contribute to the environment by maintaining a low pH (<4.5) which serves as a defense mechanism against pathogen colonization (Rönnqvist et al., 2006; O’Hanlon et al., 2013; Mirmonsef et al., 2014). The maintenance of the reproductive tract environment by Lactobacillus is associated with reproductive health. In a study involving women of various ages and ethnicities, five different core vaginal microbiomes were identified containing similar abundances of various bacterial taxa (Ravel et al., 2011). Out of the five core microbiomes, four were dominated by a different Lactobacillus species, but similar in overall health and acidic pH. Women in the fifth core microbiome group, however, did not have a vaginal bacterial community dominated by a single taxon, but contained a greater diversity of bacteria. Additionally, these women had a more basic pH and higher Nugent score; a diagnosis for bacterial vaginosis that often requires treatment (Ravel et al., 2011). In the uterus, Lactobacillus is still the dominant genus but there is a greater diversity of bacteria. However, overall bacterial abundance is significantly reduced compared to the vagina (Mitchell et al., 2015; Moreno et al., 2016; Chen et al., 2017). Various theories have emerged for this difference between environments including the cervix acting as a barrier or the result of differences in immune responses between the two organs (Moreno and Simon, 2018). Determination of the healthy uterine bacterial communities in women is challenging, however, due to the difficulty of sampling with various methods utilized, and most samples are collected from women with health complications, undergoing hysterectomy, or fertility issues (Garcia-Grau et al., 2018).
The majority of previous research on bacteria in the bovine reproductive tract was associated with the presence of pathogens and the development of postpartum uterine diseases. The increased exposure of the reproductive tract to the external environment during parturition allows pathogenic bacteria to enter and colonize. Pathogens such as Escherichia coli (E. coli), Trueperella (previously known as Arcanobacterium) pyogenes, and Fusobacterium necrophorum are commonly associated with the development and presence of uterine infections (Sheldon and Dobson, 2004; Machado et al., 2012; Karstrup et al., 2017; Sheldon and Owens, 2017; Galvão et al., 2019). Failure of the reproductive tract and resident immune cells to recognize and remove the pathogenic bacteria leads to the development of subclinical or clinical infections, most commonly endometritis or metritis. Signs of these diseases, including vaginal discharge, inflammation, and elevated temperature, can be identified within the first week of the postpartum period, and up to 50 days following parturition (Sheldon et al., 2009). The probability of infection is greater following complications from parturition such as retained fetal membranes or dystocia. Due to the short duration of the postpartum period in order for the cow to be re-bred, these potential infections must be cleared quickly or treated. If an infection persists to the time of re-breeding, fertility is often compromised and the postpartum interval extended due to a delayed return to estrous and failure to establish a pregnancy (Bonnett et al., 1993; LeBlanc et al., 2002; Ribeiro et al., 2013). Therefore, understanding the pathogenic bacteria of the uterus regarding disease is a major focus to reduce the effects of postpartum diseases and increase reproductive success.
Recently, researchers have discovered healthy communities of bacteria in the reproductive tract of cattle. One of the first studies to evaluate the ruminant reproductive tract bacterial communities using 16S bacterial rRNA gene sequencing was conducted in 2014, using vaginal swabs in ewes and cows of different ages and stages of production (Swartz et al., 2014). Contrasted to previous studies in humans and primates, the ruminant vaginal bacterial communities were found to be highly diverse and contained distinctly different taxa (Swartz et al., 2014). Furthermore, specific comparison between non-pregnant and pregnant animals revealed less diversity in the vagina of pregnant heifers and cows than non-pregnant heifers and cows (Laguardia-Nascimento et al., 2015). Following these studies, discovery of the uterine bacterial communities was necessary. Comparison between the uterine and vaginal bacterial communities was conducted by collection of flushes from non-pregnant cows two days prior to timed artificial insemination (TAI). The uterus had a significantly reduced number of bacterial taxa (495 ± 208 operational taxonomic units [OTU]) compared to the vagina (838 ± 144 OTU). Phyla-level abundances were similar, but multiple genera-level differences of bacteria were detected between the two environments (Clemmons et al., 2017). Notable among these studies, Lactobacillus species were detected in the majority of samples, but at very low abundances (<1%) corresponding with the greater pH (>6) observed in the vagina of cattle. Numerous studies are in agreement that the Lactobacillus prevalence of the bovine reproductive tract is likely insignificant, contrasting that of the human reproductive tract (Swartz et al., 2014; Laguardia-Nascimento et al., 2015; Clemmons et al., 2017; Ault et al., 2019a; Quereda et al., 2020).
Overall, research has consistently recognized that similar to the rumen, the bovine vagina, despite breed, production stage, and reproductive status, is dominated by three major phyla: Firmicutes, Bacteroidetes, and Proteobacteria (Swartz et al., 2014; Laguardia-Nascimento et al., 2015; Clemmons et al., 2017; Ault et al., 2019a). Other phyla, such as Fusobacteria, have also appeared among the most dominant phyla in some studies (Swartz et al., 2014; Messman et al., 2020; Quereda et al., 2020), which contain common pathogenic bacteria. Clemmons et al. (2017) suggested the vaginal bacterial communities were highly influenced by gastrointestinal bacteria due to the close proximity and exposure to the environment. The uterus has been less investigated, however, it shares similar taxa with the vagina with different proportions of dominant phyla with greater evenness of diversity (Clemmons et al., 2017; Moore et al., 2017; Ault et al., 2019b). Additionally, cluster analyses have indicated the uterus contains its own unique bacterial communities distinct from the vagina (Clemmons et al., 2017). The specific contributions of these bacteria in the uterus and vagina remain to be fully understood. However, as discussed further below in the review, these communities likely provide similar functional roles as in the gastrointestinal tract, such as providing nutrients and intermediate metabolites for physiological processes and influencing immunological environment of the reproductive tract.
These studies discovered the bovine reproductive tract microbiome and set the stage for beginning to identify potential roles for bacterial communities in physiological processes of the reproductive tract. Limitations of these studies, however, involve utilizing only a single time point for sampling or not controlling for estrous cycle stage or phase at the time of sampling. Therefore, understanding the effects of estrous cycle stage, and other factors such as management practices, nutrition, or reproductive status is needed to fully evaluate the roles of the reproductive tract microbiome.
2.2 Reproductive bacterial communities during the menstrual and estrous cycle and puberty
In humans, the menstrual cycle is characterized by menses at the beginning and end of the cycle, with ovulation in the middle. Approximately half of the cycle consists of the follicular (or proliferative) phase with rising estrogen during follicular development, resulting in ovulation. The remaining ~50% of the cycle is the luteal (or secretory) phase with increasing progesterone and estrogen for the preparation of a pregnancy (Mihm et al., 2011). Menses occur when there is no developing pregnancy, leading to the shedding of the endometrial lining (Nair and Taylor, 2010). Due to this process, studies have evaluated the changes in the reproductive tract bacterial communities throughout the menstrual cycle. The diversity of bacteria and non-Lactobacillus species abundance in the vagina was observed to be greater at menses, indicating lower stability of the bacterial communities (Eschenbach et al., 2000). Similarly, Gajer et al. (2012) observed the microbiome was the least stable during menses and associated with hormone concentrations. The greatest stability was observed with peak estrogen secretion, followed by peak progesterone secretion (Gajer et al., 2012). Therefore, stability during ovulation and pregnancy preparation and development is likely critical for establishing an optimal reproductive tract environment. Further studies have found differences in bacterial functional pathways between the proliferative and secretory phase (Chen et al., 2017). During the proliferative phase, pathways such as pyrimidine and purine metabolism were enriched, potentially indicating increased bacterial cell proliferation. Different pathways, including multiple amino acid metabolism pathways, were enriched during the secretory phase. Additionally, multiple bacterial species in the uterus and vagina were differentially abundant depending on menstrual cycle phase (Chen et al., 2017). Therefore, due to the physiological changes occurring, especially menses, bacterial communities and their respective functions are also likely shifting throughout the menstrual cycle.
The bovine estrous cycle differs from humans as it is characterized by ovulation at the beginning of the cycle, followed by a long luteal phase making up ~80% of the cycle. Additionally, there is no major shedding of the endometrial lining as occurs in humans. A few studies have indicated differences in the reproductive tract bacterial communities depending on the stage of the estrous cycle (Ault et al., 2019a; Ault et al., 2019b; Quereda et al., 2020). Additionally, multiple phyla and genera abundances shifted between days of the protocol (Ault et al., 2019a). Based on heat detection, vaginal swabs were taken during estrus and diestrus to represent the follicular and luteal phase, respectively. Although diversity analyses did not detect significant differences, multiple taxa significantly differed and clustering of communities was observed by phase (Quereda et al., 2020). Reproductive tract bacterial communities have also been measured at multiple time points during a 7-Day Co-Synch estrous synchronization protocol (Ault et al., 2019a; Ault et al., 2019b). Additionally, multiple phyla and genera abundances shifted between days of the protocol (Ault et al., 2019a). Bacterial communities clustered by day of the protocol according to principal coordinate analysis for beta diversity, and alpha diversity significantly decreased two days prior to TAI (Ault et al., 2019b). Additionally, multiple phyla and genera abundances shifted between days of the protocol (Ault et al., 2019a). Therefore, the fluctuations in various hormones throughout the estrous cycle may influence the fluctuations observed in the uterine and vaginal microbiome. To determine the impact of specific hormonal status on the vaginal microbiome, Messman et al. (2020) classified cows into groups based on their level of circulating estradiol concentration at the time of breeding following a synchronization protocol. No diversity or overall composition differences were detected, however multiple genera level abundances differed between estradiol groups (Messman et al., 2020). Recently, the uterine bacterial communities were suggested to be associated with varying changes in the level of circulating progesterone and estradiol concentrations from two days prior to TAI (day -2) and at the time of TAI (day 0; Poole et al., 2022). Progesterone was identified as having greater influence over bacterial community diversity and abundance shifts than estradiol (Poole et al., 2022). Therefore, the bovine uterine and vaginal bacterial communities experience shifts in composition between phases of the estrous cycle, and some taxa may be impacted by specific hormone concentrations or the dominant circulating hormone. Further research is needed to understand how these bacterial abundance and diversity shifts occurring during the estrous cycle may impact subsequent fertility.
In addition to the estrous cycle, another largely hormone-involved process is the attainment of puberty. Few studies have evaluated the female reproductive tract bacterial communities of pre-pubertal animals, and the potential impact of puberty attainment. The attainment of puberty is a complex physiological event, largely dependent on activity of the endocrine system. In the pre-pubertal heifer, the surge center of the hypothalamus is not yet active due to the strong sensitivity of low estradiol concentrations causing a negative feedback (Schillo et al., 1982). At the onset of puberty, the sensitivity to the negative feedback of estradiol has decreased, allowing for activity of the surge center (Day et al., 1987; Cardoso et al., 2020). Increased gonadotropin releasing hormone, luteinizing hormone, and follicle-stimulating hormone secretions stimulate the final follicular development and maturation of the dominant follicle. The surge center activity will then be great enough to initiate the first ovulation, resulting in the presence of a corpus luteum and secretion of progesterone indicative of pubertal onset. These hormonal changes stimulate differences between pre-pubertal and pubertal uterine endometrium gene expression and secretions into the uterine lumen (Cánovas et al., 2014; Fortes et al., 2018). Due to the previous studies indicating effects of the estrous cycle and hormone concentrations on the reproductive tract microbiome, these actions that occur with puberty attainment likely also impact the microbial communities. Ault-Seay et al. (2022a) utilized frequent blood sampling for progesterone concentrations to determine pubertal status of developing heifers and collected uterine flushes for bacterial community analysis beginning after weaning until the first breeding. An increasing number of uterine bacterial species from pre-pubertal to pubertal heifers was observed, in addition to tighter clustering by principal coordinate analysis of pubertal heifers with more variation in the overall composition of pre-pubertal heifers. Multiple phyla and genera abundances in the uterus were also impacted by pubertal status (Ault-Seay et al., 2022a). Therefore, the reproductive tract microbiome is responding to the changes and maturing with the attainment of puberty and reproductive functions, but further studies are needed to fully elucidate this interaction.
2.3 Relationship between the reproductive bacterial communities and fertility
The bacterial communities of the reproductive tract have an important role in maintaining a healthy environment, which likely influences fertility. As previously mentioned, in humans, the dominance of Lactobacillus is critical for maintaining the proper reproductive tract environment and inhibiting the growth and colonization of pathogens. This dominance of Lactobacillus has also been associated with reproductive outcomes and fertility issues. Women with varying causes of infertility were found to have greater microbial diversity and different overall microbiome when compared with fertile women, largely attributed to differences in Lactobacillus species composition (Campisciano et al., 2017). Furthermore, infertile women undergoing in-vitro fertilization had their vaginal and endometrial microbiomes classified as having either Lactobacillus-dominated reproductive tract bacterial communities, consisting of >90% abundance of Lactobacillus species, or non-Lactobacillus-dominated with >10% non-Lactobacillus species. Patients with non-Lactobacillus-dominated reproductive tract bacterial communities had significantly lower implantation and pregnancy rates (Moreno et al., 2016). In established pregnancies, microbial dysbiosis in the uterus or vagina that occurs during gestation can affect pregnancy maintenance. Influx of pathogenic bacteria into the uterus can ultimately result in preterm birth or miscarriage (Payne and Bayatibojakhi, 2014; Moreno et al., 2016; Al-Memar et al., 2020). Therefore, clinical studies in humans have indicated strong associations of the uterine and vaginal microbiome with reproductive success.
Limited research has been conducted to determine the influence of bacterial communities on reproductive performance and breeding outcomes in cattle. It has been well established that postpartum uterine infections can affect reproductive performance by increasing the time to conception (Bonnett et al., 1993; LeBlanc et al., 2002; Ribeiro et al., 2013). However, the influence of the healthy microbiome on reproductive performance is limited. Ault et al., 2019a; Ault et al., 2019b) performed estrus synchronization in cows for uterine and vaginal flush collections to determine the bacterial community at multiple time points until breeding via TAI, with pregnancy determined 30 days after breeding. Beta diversity using principal coordinate analyses indicated clustering among bacterial samples with distinct separation of resulting pregnant and non-pregnant animals at two days prior to TAI. Samples from non-pregnant animals clustered closely together indicating a similar overall bacterial community composition that was potentially influencing the animal’s ability to conceive. Multiple genera level differences were detected between resulting outcomes, especially at two days prior to TAI in the uterus. Cows that became pregnant had potentially pathogenic bacteria present in abundances <1%, while cows that did not become pregnant had these same bacteria present with abundances ranging from 1-10%, although no signs of infection were observed (Ault et al., 2019a; Ault et al., 2019b). Additionally, multiple phyla and genera abundances shifted between days of the protocol (Ault et al., 2019a). Messman et al. (2020) evaluated the vaginal microbiome immediately before breeding and did not identify differences in the diversity or global composition between resulting pregnant and non-pregnant animals. This supports previous research that did not detect an impact of the vaginal microbiome diversity on breeding success (Ault et al., 2019a; Ault et al., 2019b). Additionally, multiple phyla and genera abundances shifted between days of the protocol (Ault et al., 2019a). Therefore, uterine bacteria likely have a greater influence over pregnancy success and minor differences in potentially pathogenic bacterial abundances may affect the uterine environment and impact fertility. Further research is necessary to characterize the interaction of these bacteria with the uterus and their impact on the uterine environment leading to reduced reproductive performance
2.4 Probiotics in the reproductive tract
The bacterial communities of the reproductive tract have indicated importance in the health and productivity of the animal; therefore, research is moving toward developing methods to optimize these bacterial communities for maximal success. The inclusion of probiotics in the diet has been a focus in gastrointestinal microbiome research. Probiotics can be provided in multiple stages of production and serve various purposes to influence fermentation of feedstuffs to increase rumen efficiency, alter the ruminal environment to prevent the colonization of pathogens, or reduce the potential for acidosis (Uyeno et al., 2015). Due to the success of probiotics in the gastrointestinal tract, researchers have begun to evaluate the use of probiotics for the reproductive tract. In humans, both orally consumed and vaginally delivered probiotics have been successful as an alternative or an addition to antibiotic regimens for treating bacterial dysbiosis in the reproductive tract (Vujic et al., 2013; Abbasi et al., 2021; Hashem and Gonzalez-Bulnes, 2022). These probiotics typically consist of Lactobacillus or Bifidobacterium species (Hashem and Gonzalez-Bulnes, 2022). Lactobacillus is used to restore the community to a Lactobacillus dominated state (Vujic et al., 2013). Bifidobacterium is used in orally consumed probiotics for the production of short chain fatty acids that affect the gut-brain axis, impacting the production and release of reproductive related hormones (Hashem and Gonzalez-Bulnes, 2022). In cattle, most studies to date utilize probiotics delivered directly to the reproductive tract, most commonly the vagina, to reduce the development of postpartum uterine diseases (Rosales and Ametaj, 2021). Although Lactobacillus is naturally low in abundance in the bovine reproductive tract, supplementation of Lactobacillus has indicated success. In vitro culture of endometrial cells with Lactobacillus species in combination with common postpartum pathogen E. coli resulted in reduced ability of E. coli to infect endometrial cells as well as reduced inflammatory markers (Genís et al., 2017). Live animal studies with Lactobacillus administration resulted in reduced prevalence of postpartum uterine diseases (Deng et al., 2015; Genís et al., 2018). Peter et al. (2018) observed an increased number of cows that became pregnant earlier in the postpartum period when receiving a Lactobacillus probiotic compared to controls, suggesting probiotics may alter the reproductive tract environment to also improve breeding outcomes. However, others have indicated no effect of probiotics in postpartum pregnancy rates (Ametaj et al., 2014). Similar to activity in the human reproductive tract, Lactobacillus can alter the environment by lowering pH to make pathogen growth unfavorable in cattle. Additional mechanisms of Lactobacillus probiotics that have been observed in the gastrointestinal tract that may also benefit the reproductive tract include increasing barrier function of the endometrial epithelium, producing antimicrobial compounds, and competitively excluding pathogens by consuming essential nutrients (Anderson et al., 2010; Reid et al., 2013; Dasari et al., 2014; Rosales and Ametaj, 2021). Future studies are required to determine the efficacy and composition of probiotics in breeding scenarios, including postpartum and virgin animals. The use of probiotics in breeding may provide an opportunity to manipulate the resident bacterial communities to create the optimal environment for pregnancy establishment.
2.5 Placental bacterial communities and fetal colonization in utero
The existence of a placental and fetal microbiome is currently a popular debate. Discovery of a reproductive tract microbiome has led researchers to investigate the potential for the fetus to be exposed and inoculated with microbes in the womb that will lead to the development of their own microbiome. Understanding early life colonization of the fetal microbiome is important for determining it’s impact of on future health and productivity of the animal. A common method to determine the microbial communities of a neonate across different species has been evaluation of the meconium. The meconium has been considered the best sampling method due to the importance of gastrointestinal microbes to host physiology, higher biomass compared to other body systems, and ease of collection (Stinson et al., 2018). Additionally, the meconium is formed in the neonate in utero, so there should be little influence of early environmental microbe exposure post-delivery (Stinson et al., 2018; Woodruff et al., 2020). Previously, the method of delivery of the neonate has been thought to be the first exposure to microbes. In humans, the meconium of babies born via vaginal birth had bacterial communities similar to the vaginal microbiome, but babies born via cesarean section had communities similar to the skin microbiome (Dominguez-Bello et al., 2010). Although cesarean delivery is less common in cattle, some differences were observed in the rumen of the calves between different modes of birth (Cunningham et al., 2018). However, since the meconium is formed in utero, the mode of birth may influence composition but is likely not the first exposure.
In an attempt to evaluate fetal colonization of uterine microbes, researchers have compared maternal microbial communities with those of the offspring. Studies have shown conflicting results, with multiple studies failing to detect a placental or fetal microbiome (Leiby et al., 2018; de Goffau et al., 2019; Sterpu et al., 2021). However, other studies have consistently identified low abundance microbes in the fetus and placental membranes or fluids. One of the first studies to identify a placental microbiome was conducted in humans and found a placental microbiome that was similar to the oral microbiome (Aagaard et al., 2014). Hummel et al. (2020) utilized cattle to compare bacterial communities of the maternal vagina, amnion fluid, calf rumen fluid, and meconium. The bacterial communities of the amnion fluid and calf meconium were the most similar, while the vaginal samples were the most different (Hummel et al., 2020). A study was conducted performing a cesarean delivery of all calves to collect amnion fluid before the rupture of membranes and decrease contamination (Husso et al., 2021). Immediately following the delivery, prior to any exposure to the dam, the calf was swabbed for a meconium sample. The meconium of calves had a higher abundance of bacteria compared to the controls, with some samples resulting in successful culture. The presence of phyla such as Firmicutes, Bacteroidetes, and Proteobacteria and genera such as Bacteroides, Enterococcus, and Clostridium in the meconium was similar to bacteria present in the adult gastrointestinal microbiome. Microbial DNA abundance of the amniotic fluid was not different from controls and did not result in any successful cultures of bacteria. However, the taxonomic profiles of the amnion were different than the negative controls, potentially suggesting a very low abundance microbiome. At the phylum level, amnion fluid samples were similar to meconium, but genera taxonomic profiles differed (Husso et al., 2021). Similar results were obtained when calves were harvested at 5, 6, and 7 months of gestation (Guzman et al., 2020). Amniotic fluid was collected, followed by samples from the calf rumen, cecum, and meconium. Amniotic samples had a very low abundance microbiome. The amnion profile was similar at the phylum level to Husso et al. (2021), but differences were observed at the genus level; this may be a result of differences at the maternal level that were not evaluated. Additionally, the abundance and taxonomic profiles differed among the different samples of the gastrointestinal tract and increased through gestation, potentially indicating selection and growing colonization of bacteria (Guzman et al., 2020). Although the studies by Husso et al. (2021) and Guzman et al. (2020) obtained samples via unnatural birthing methods, they suggest early colonization of bacteria in utero prior to exposure by the birth canal. Furthermore, the potential presence of a very low biomass placental microbiome suggested by studies has indicated the importance of appropriate methodology. Reliability of studies evaluating low biomass samples has increased by the use of quality controls such as sterile equipment, sequencing of swabs and open containers at collection sites, and negative controls of sample medias, as well as proper bioinformatic analyses (Husso et al., 2021; Weinroth et al., 2022). Therefore, the discrepancies between studies that successfully identified or failed to detect a placental microbiome may be due to differences in sampling techniques, and laboratory and bioinformatic analyses.
As multiple studies have suggested the presence of bacteria in the fetus and neonate, the route of inoculation is not fully understood. The amnion has been studied the most as a potential source of microbes for inoculation, as amniotic fluid is ingested in utero (Dasgupta et al., 2016; Woodruff et al., 2020). Although amnion and gastrointestinal communities are similar, the differences observed between samples indicates transmission of microorganisms by additional mechanisms. In mice, oral administration of labelled bacteria to pregnant females resulted in inoculation of their young detected via the meconium (Jiménez et al., 2008). In humans, viable bacteria have been shown to travel through blood in immune cells and potentially deliver bacteria to the fetus via the umbilical cord (Jiménez et al., 2005; Perez et al., 2007). In cattle, Jeon et al. (2017) found an association between bacteria present in the blood and the uterus, potentially originating from the gastrointestinal tract. After parturition, the uterine microbiome transitioned from being similar to the fecal microbiome, to more similarity to bacteria present in blood; this is likely due to the exposure of the uterus to blood following parturition with rupture of blood vessels. Therefore, bacteria from other environments, such as the gastrointestinal tract, are able to be transmitted through the blood to the uterine environment (Jeon et al., 2017). However, differences between placental types in mice, humans, and cattle may influence blood transmission of microorganisms. Furthermore, the source and route of fetal colonization is crucial to understand as in utero colonization may also influence the probability of future disease and productivity. Lima et al. (2019) compared the fecal and vaginal microbiome of the dam to the neonate’s fecal and respiratory microbiome. The calf respiratory microbiome was closely associated to the vaginal microbiome, and the calf fecal microbiome was highly similar to the dam fecal microbiome. Calves born to dams with a higher pathogenic bacteria presence in the vagina had increased incidences of respiratory disease (Lima et al., 2019). Although this was likely due to inoculation at parturition during natural birth, this indicates the maternal microbiome’s influence of calf microbial colonization and health outcomes. Further research is needed to fully understand the major route of transmission, factors that can influence differences in early fetal and placental fluid microbiomes, and how in utero colonization can impact the offspring’s future health and productivity.
3 Uterine environment in relation to the resident microbiome
The uterine environment is crucial for preparation, development, and support of pregnancy for optimal reproductive efficiency. The uterine microbiome is a recently discovered but major component of the uterine environment that can impact reproductive success. Bacteria have a dynamic relationship with the uterine environment as they can utilize intra-uterine components for their own functions, as well as release other components into the uterine environment. The uterine environment consists of uterine histotroph or embryo supportive secretions. Histotroph contains nutrients, hormones, growth factors, and immune signaling molecules that can be produced from uterine tissues or originate from systemic circulation to support normal physiological functions (Bazer, 1975; Gao et al., 2009; Mullen et al., 2012). During early pregnancy, histotroph is especially vital for the survival of the developing embryo by providing all necessary nutrients until placental attachment and hemotrophic support (Bazer, 1975). Histotroph is largely produced by the uterine glandular epithlium and uterine gland knockout sheep cannot support pregnancy as conceptuses do not elongate, an important developmental process in ruminants (Gray et al., 2002). Therefore, the presence of nutrients, specifically protein and amino acids, and cytokines in the uterine environment and their relationship with the uterine microbiome may contribute toward reproductive efficiency.
3.1 Proteins and amino acids
Protein is one of the most important nutrients for reproductive function. Amino acids from rumen degradable and undegradable protein as well as non-protein nitrogen source are critical components of the histotroph. During early pregnancy, an influx of amino acids and total protein into the uterine environment has been observed (Groebner et al., 2011; Mullen et al., 2012; Forde et al., 2014; Crouse et al., 2019). Pathway analyses indicate proteins related to uterine remodeling, embryo development, and maternal immune function have greater abundance in the uterus during early pregnancy to facilitate pregnancy establishment (Mullen et al., 2012). As the early embryo is free-floating in the uterine environment for an extended period, it fully relies on the nutrients available in the histotroph for growth and development and successful establishment and maintenance of pregnancy (Bazer, 1975). Although providing adequate protein is highly important to the ruminant diet, especially to breeding cows, excess protein in the diet may cause negative effects on early pregnancy. Due to the known impact of uterine pH on sperm motility and survival, excess protein provided can increase the acidity of the uterine environment and inhibit pregnancy establishment (Elrod and Butler, 1993; Ocon and Hansen, 2003). Excess protein, and especially non-protein nitrogen, cannot all be utilized by the rumen microbes, resulting in an increase of plasma urea and ammonia that can travel to the uterus and decrease pH (Dawuda et al., 2002). However, studies are conflicting on excess protein impacting the uterus and fertility, with no impact on uterine pH or reproductive functions (Amundson et al., 2016). Further research is needed to determine the exact factors leading to this effect in some cases.
Proteins and amino acids are also crucial for supporting microbial functions. Proteolytic microbes can breakdown proteins and utilize the amino acids to build their own proteins necessary for survival. Recent studies have indicated that diet composition can affect the histotroph protein and amino acids composition (Harlow et al., 2018; Brandt, 2020), which may impact the nutrients available to the resident microbes, therefore altering microbial community composition. Ault-Seay et al. (2022a) evaluated the effect of different levels of dietary protein supplement on the uterine bacterial communities. Different protein supplementation levels were not able to impact the global bacterial community composition in the uterus, but did affect individual bacterial taxa abundances. Notably, Prevotella species, a major proteolytic bacterial genus, increased in abundance in the uterus with increasing protein level in the supplement (Ault-Seay et al., 2022a). Therefore, protein is vital for reproductive functions but must be balanced to support microbial activity and create the proper uterine environment for optimal reproductive performance. Other nutrients and diet components may also have an influence on the reproductive tract microbiome. Recent research reported the level of concentrate inclusion in the diet can affect not only the rumen bacterial communities but also the reproductive tract microbiome, mainly in the vagina (Pickett et al., 2022). Further research is needed to fully understand the interactions between diet, resulting nutrients to the host, and uterine environment, which can impact the uterine microbiome. The ability of the diet to influence uterine microbiome composition may indicate future potential to manipulate the microbial communities for increased reproductive success.
3.2 Immunological environment by immune cells and cytokines
The immunological environment of the uterus is dynamic in order to prevent infections while maintaining a healthy, stable environment conducive for pregnancy. The endometrial epithelium is the first line of defense in the uterus, which also expresses Toll-like receptors (TLR) to recognize pathogen associated molecular patterns (PAMP; Cronin et al., 2012; Fu et al., 2013; Dadarwal et al., 2017). The most common PAMP is the endotoxin lipopolysaccharide (LPS), which is present in the outer membrane of Gram-negative bacteria and recognized by TLR4 to stimulate an immune response (Dong et al., 2017). Cytokines are some of the most important immune response factors in the reproductive tract environment and can be produced by endometrial cells or resident immune cells (Tabibzadeh, 1991). Many cytokines have classically been categorized as pro-inflammatory (e.g. interleukin [IL]-1β, tumor necrosis factor [TNF]-α, interferon [IFN]-γ, IL-6, etc.) or anti-inflammatory (e.g. IL-4, IL-10, transforming growth factor [TGF]-β, etc.). If the epithelial barrier is disrupted, the stromal layer containing resident immune cells of the endometrium is exposed. Neutrophils and macrophages are the most abundant innate immune cells of the bovine uterus, which also express various TLR including TLR4 and can respond to intra-uterine LPS (Dadarwal et al., 2017). When LPS activates TLR4 on epithelial or innate immune cells, pro-inflammatory cytokines TNF-α, IL-6, and IL-1 production is upregulated stimulating an inflammatory response (Cronin et al., 2012; Swangchan-Uthai et al., 2012). These cytokines will signal for activation of resident macrophages towards a M1, pro-inflammatory phenotype and recruit additional immune cells. To resolve the inflammation and return to a normal balance, anti-inflammatory and regulatory cytokines, such as IL-10, TGF-β, and IL-33 are secreted by epithelial and immune cells to shift macrophage activation to an M2 phenotype (Dadarwal et al., 2017).
Increases in pro-inflammatory cytokines are most often stimulated by the increasing presence of pathogenic bacteria, specifically Gram-negative bacteria expressing LPS, due to the activation of TLR4 in the uterine endometrium with resident immune cells (Davies et al., 2008; Lu et al., 2008; Fu et al., 2013). The endometrial expression of TLR4 has also shown to differ across the estrous cycle in humans and swine, with greater expression during stages of higher estrogen dominance (Hirata et al., 2007; Yoo et al., 2019). The inflammatory response of cytokines and other immunological factors in the uterine endometrium to LPS may also be impacted by the phase of the estrous cycle due to differences in hormone dominance (Ault-Seay et al., 2022b). As previously mentioned, alterations in bacterial community composition can be caused by different diets or hormonal changes from the estrous cycle. In the uterus, increases in Gram-negative pathogenic bacteria prior to breeding were observed to reduce pregnancy outcomes (Ault et al., 2019a). Stressors, such as a sudden nutritional change, can result in increased LPS release from the rumen, with effects observed both systemically and in the reproductive tract (Zebeli et al., 2012; Bilal et al., 2016). Animals with ruminal acidosis had higher LPS detected in blood as well as increases in pro-inflammatory cytokine concentration in blood and gene expression in the endometrium (Bilal et al., 2016). Although the endometrial TLR4 activation and subsequent response has been associated with negative outcomes on pregnancy, interestingly, it was found to have a beneficial cytokine response following mating (Chan et al., 2021). In mice mated with wild-type TLR4 or females with a null mutation of TLR4, wild-type females had better reproductive success with greater pregnancy rates and improved placental and fetal development. Additionally, wild-type females had a greater cytokine response to mating than the null mutation TLR4 females, which was determined to benefit pregnancy establishment (Chan et al., 2021). Further research is warranted to understand how the changes in the uterine microbiome may be altering this response, shifting the presence of various cytokines, and impacting breeding success.
Cytokines modulate inflammatory responses both systemically and within the reproductive tract. Generally, cytokines are most commonly associated with their numerous immune system related functions including regulation of inflammation and immune cell recruitment (Dimberg, 2010). Specific to the reproductive tract, they are also involved in other non-immune system related processes such as ovulation (Espey et al., 2004), corpus luteum function and luteolysis (Penny et al., 1999), embryo development and implantation (Zolti et al., 1991; Simón et al., 1998; Ealy et al., 2021), angiogenesis, placental development, and immunotolerance to the growing fetus (Raghupathy, 2001). Cytokines, including those classically categorized as pro-inflammatory, can also be considered embryokines, which are produced by the early embryo for development and signaling to maternal tissues, or produced by the maternal tissues to support early embryo development (Ealy et al., 2021). The balance between pro- and anti-inflammatory cytokines with proper timing is necessary to maintain an environment conducive for pregnancy establishment, but also prepared to respond to inflammatory stimuli such as a developing infection. The interplay of pro- and anti- inflammatory cytokines are believed to be important in providing the immunotolerant environment of the reproductive tract (Raghupathy, 2001). As the developing embryo and growing fetus in mammals is allogenic by expressing both maternal and paternal antigens, immunotolerance is crucial for the establishment and maintenance of pregnancy (Schjenken et al., 2012). Gene expression analysis of the heifer endometrium suggests that the inflammatory state of the endometrium is a major predictor of future pregnancy success (Killeen et al., 2014). Greater levels of gene expression related to inflammation was observed in heifers of lesser fertility (Killeen et al., 2014). Intra-uterine cytokine concentrations were different prior to TAI between cows that became pregnant and those that did not, including a greater concentration of anti-inflammatory cytokine TGF-β in those that became pregnant (Poole et al., 2021), indicating a potential influence of the cytokines in the uterine environment on pregnancy establishment. Additionally, uterine cytokines such as TGF-β, IL-1β, and IL-6 were correlated to the abundance of various bacteria genera, suggesting a potential influence by the uterine bacterial communities (Poole et al., 2021). Therefore, imbalances in cytokine concentrations in the uterus at crucial timepoints of the estrous cycle and pregnancy establishment or increases of pro-inflammatory cytokines at the improper time may reduce reproductive efficiency. However, bacteria may also contribute to beneficial changes in the immunological environment. Ault et al. (2019b), found the family Ruminococcaceae to be highly abundant across the uterus and vagina, which is a known major producer of the VFA butyrate in the gastrointestinal system (Esquivel-Elizondo et al., 2017; Fu et al., 2019). Butyrate is involved in the development of immune cells, specifically T regulatory cells, which help maintain an immunotolerant environment for pregnancy establishment and maintenance to prevent maternal rejection of the developing fetus (Aluvihare et al., 2004; Shima et al., 2010; Furusawa et al., 2013; Zhang et al., 2016). The presence of the bacteria in this family, and many others throughout the reproductive tract, may be important for preventing the negative impacts of inflammation to maintain an environment suitable for optimal reproductive success. Similar to the reproductive tract microbiome, cytokines and chemokines can fluctuate overtime, including through the attainment of puberty (Ault-Seay et al., 2021), across the estrous cycle (Krakowski and Zdzisinska, 2007; Oliveira et al., 2013; Poole et al., 2021), and during pregnancy (Krakowski and Zdzisinska, 2007; Oliveira et al., 2013). In comparison, uterine cytokines were observed to fluctuate during estrous synchronization with minimal change in the vagina (Poole et al., 2021). Fluctuations throughout the reproductive cycle may help maintain the optimal uterine environment in preparation for pregnancy. However, dysregulation of cytokines in the reproductive tract may influence reproductive efficiency.
4 Conclusion
In conclusion, the bacterial communities throughout the body play a major role in the animal’s overall performance. Recent discoveries of the reproductive tract microbiome have multiple implications for the ruminant’s health and breeding success, and potentially their offspring (Swartz et al., 2014; Laguardia-Nascimento et al., 2015; Clemmons et al., 2017; Ault et al., 2019a; Lima et al., 2019). The reproductive tract microbiome can be highly variable and can depend on diet, phases of the estrous cycle, and puberty attainment influencing the uterine environment and endometrial tissue activity (Laguardia-Nascimento et al., 2015; Ault et al., 2019a; Ault et al., 2019b). Additionally, multiple phyla and genera abundances shifted between days of the protocol (Ault et al., 2019a; Quereda et al., 2020; Ault-Seay et al., 2022a). These factors may positively or negatively impact the composition of the resident uterine microbiome and subsequently the endometrial cytokine balance, ultimately influencing the ability to develop and support a pregnancy (Killeen et al., 2014; Poole et al., 2021; Ault-Seay et al., 2022b). Figure 1 summarizes this proposed interaction between the factors impacting the uterine microbiome and its effect on the uterine environment and pregnancy establishment based on the current review. Emerging research also suggest the potential for the placental microbiome and in utero colonization of the fetus to affect future health and productivity of the offspring (Lima et al., 2019; Hummel et al., 2020; Husso et al., 2021). Future studies are necessary to determine the factors affecting the presence of bacterial species and the specific mechanisms bacteria utilize to interact with the uterine environment and influence reproductive outcomes. Further understanding of these roles may allow for manipulation of the uterine bacterial communities to benefit reproductive efficiency and future offspring productivity.
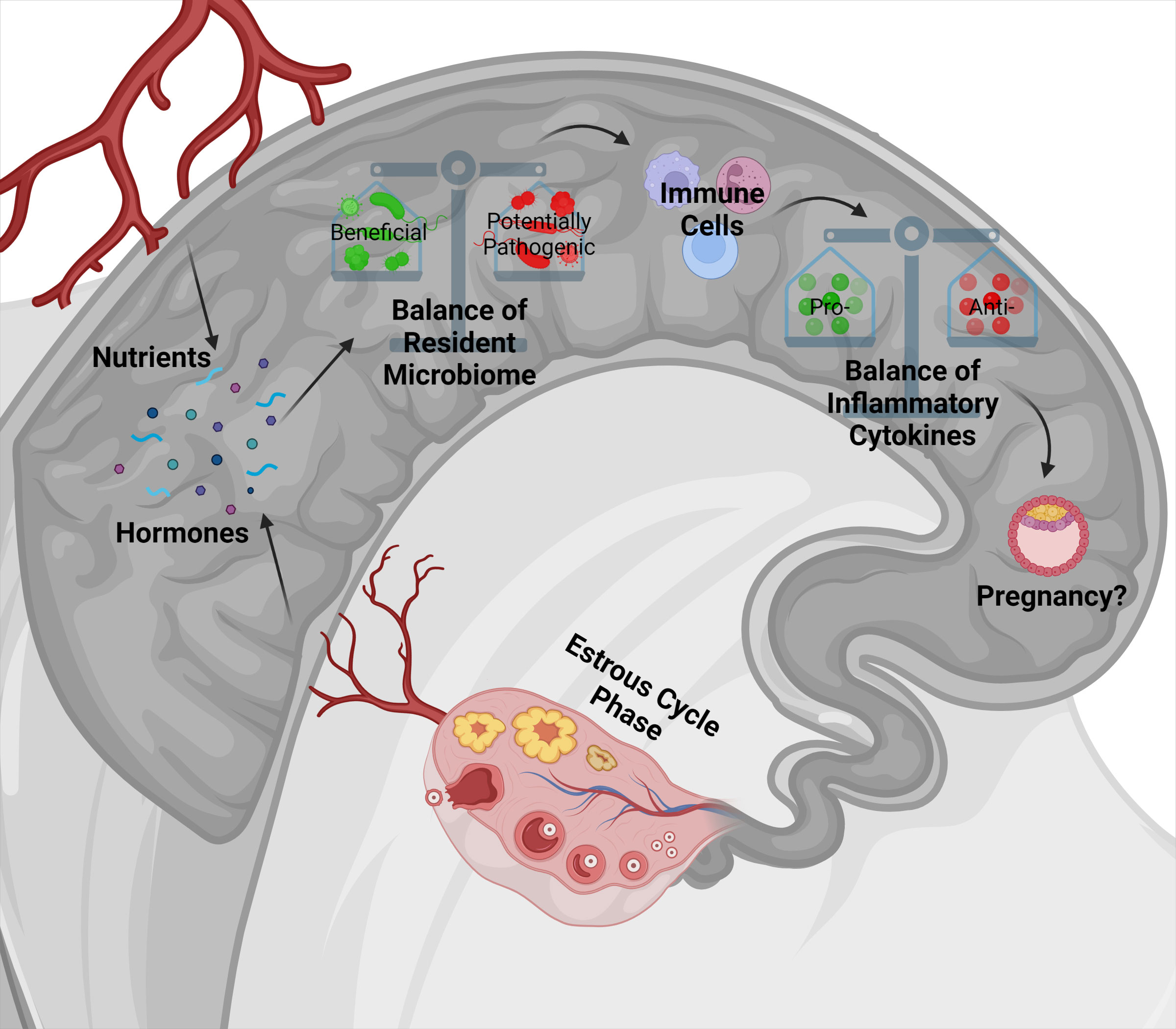
Figure 1 The proposed model indicates the interaction of nutrition and phases of the estrous cycle with uterine bacterial communities and immune response, potentially impacting pregnancy establishment. Nutrients and hormones, depending on the stage of the estrous cycle, can alter the uterine environment and affect the balance of the uterine bacterial communities positively or negatively. Depending on the uterine bacterial community composition, bacteria can release their products into the uterine environment or bind to receptors on uterine endometrium to affect immune cell development. Immune cells may then be activated toward a pro- or anti- inflammatory state which will influence the cytokines produced and released in the uterine environment. Together, these factors have a strong influence on pregnancy establishment or failure.
Author contributions
TA-S wrote the manuscript. SM, DM, FS, KP, KM, and PM edited, reviewed, and approved the manuscript for publication.
Funding
The authors would like to thank the state of Tennessee through UT AgResearch, the Department of Animal Science, and the USDA National Institute of Food and Agriculture, Hatch Project No. 1019048 for providing funding and support for this research.
Conflict of interest
The authors declare that the research was conducted in the absence of any commercial or financial relationships that could be construed as a potential conflict of interest.
Publisher’s note
All claims expressed in this article are solely those of the authors and do not necessarily represent those of their affiliated organizations, or those of the publisher, the editors and the reviewers. Any product that may be evaluated in this article, or claim that may be made by its manufacturer, is not guaranteed or endorsed by the publisher.
References
Aagaard K., Ma J., Antony K. M., Ganu R., Petrosino J., Versalovic J. (2014). The placenta harbors a unique microbiome. Sci. Trans. Med. 6 (237), 237ra265–237ra265. doi: 10.1126/scitranslmed.3008599
Abbasi A., Aghebati-Maleki A., Yousefi M., Aghebati-Maleki L. (2021). Probiotic intervention as a potential therapeutic for managing gestational disorders and improving pregnancy outcomes. J. Reprod. Immunol. 143, 103244. doi: 10.1016/j.jri.2020.103244
Al-Memar M., Bobdiwala S., Fourie H., Mannino R., Lee Y., Smith A., et al. (2020). The association between vaginal bacterial composition and miscarriage: A nested case–control study. International Journal of Obstetrics and Gynaecology 127, 2, 264–274. doi: 10.1111/1471-0528.15972
Aluvihare V. R., Kallikourdis M., Betz A. G. (2004). Regulatory T cells mediate maternal tolerance to the fetus. Nat. Immunol. 5, 266. doi: 10.1038/ni1037
Ametaj B. N., Iqbal S., Selami F., Odhiambo J. F., Wang Y., Gänzle M. G., et al. (2014). Intravaginal administration of lactic acid bacteria modulated the incidence of purulent vaginal discharges, plasma haptoglobin concentrations, and milk production in dairy cows. Res. Vet. Sci. 96 (2), 365–370. doi: 10.1016/j.rvsc.2014.02.007
Amundson O. L., Larimore E. L., McNeel A. K., Chase C. C., Cushman R. A., Freetly H. C., et al. (2016). Uterine environment and pregnancy rate of heifers with elevated plasma urea nitrogen. Anim. Reprod. Sci. 173, 56–62. doi: 10.1016/j.anireprosci.2016.08.011
Anderson R. C., Cookson A. L., McNabb W. C., Park Z., McCann M. J., Kelly W. J., et al. (2010). Lactobacillus plantarum MB452 enhances the function of the intestinal barrier by increasing the expression levels of genes involved in tight junction formation. BMC Microbiol. 10 (1), 316. doi: 10.1186/1471-2180-10-316
Ault T. B., Clemmons B. A., Reese S. T., Dantas F. G., Franco G. A., Smith T. P. L., et al. (2019a). Bacterial taxonomic composition of the postpartum cow uterus and vagina prior to artificial insemination. J. Anim. Sci. 97 (10), 4305–4313. doi: 10.1093/jas/skz212
Ault T. B., Clemmons B. A., Reese S. T., Dantas F. G., Franco G. A., Smith T. P. L., et al. (2019b). Uterine and vaginal bacterial community diversity prior to artificial insemination between pregnant and nonpregnant postpartum cows. J. Anim. Sci. 97 (10), 4298–4304. doi: 10.1093/jas/skz210
Ault-Seay T. B., Brandt K. J., Henniger M. T., Payton R. R., Mathew D. J., Moorey S. E., et al. (2022a). Bacterial communities of the uterus and rumen during heifer development with protein supplementation. Front. Anim. Sci. doi: 10.3389/fanim.2022.903909
Ault-Seay T. B., Harrison T. D., Brandt K. J., Payton R. R., Schneider L. G., Myer P. R., et al. (2021). Short communication: The effects of protein level on cytokines and chemokines in the uterine environment of beef heifers during development. J. Anim. Sci. 99(6), 1–7. doi: 10.1093/jas/skab105
Ault-Seay T. B., Payton R. R., Moorey S. E., Pohler K. G., Schrick F. N., Shepherd E. A., et al. (2022b). Endometrial gene expression in response to lipopolysaccharide between estrous cycle phases and uterine horns in cattle. Front. Anim. Sci. 3. doi: 10.3389/fanim.2022.939876
Bazer F. W. (1975). Uterine protein secretions: Relationship to development of the conceptus. J. Anim. Sci. 41 (5), 1376–1382. doi: 10.2527/jas1975.4151376x
Bellows D. S., Ott S. L., Bellows R. A. (2002). Review: Cost of Reproductive Diseases and Conditions in Cattle. Prof. Anim. Sci. 18(1):26–32. doi: 10.15232/S1080-7446(15)31480-7
Bergman E. N. (1990). Energy contributions of volatile fatty acids from the gastrointestinal tract in various species. Physiological Reviews 70, 2, 567–590. doi: 10.1152/physrev.1990.70.2.567
Bilal M. S., Abaker J. A., ul Aabdin Z., Xu T., Dai H., Zhang K., et al. (2016). Lipopolysaccharide derived from the digestive tract triggers an inflammatory response in the uterus of mid-lactating dairy cows during SARA. BMC Vet. Res. 12 (1), 284. doi: 10.1186/s12917-016-0907-1
Bonnett B. N., Wayne Martin S., Meek A. H. (1993). Associations of clinical findings, bacteriological and histological results of endometrial biopsy with reproductive performance of postpartum dairy cows. Prevent. Vet. Med. 15 (2), 205–220. doi: 10.1016/0167-5877(93)90114-9
Brandt K. J. (2020). The effect of supplemental protein on the uterine environment during heifer development (Knoxville, TN: University of Tennessee).
Campisciano G., Florian F., D'Eustacchio A., Stanković D., Ricci G., De Seta F., et al. (2017). Subclinical alteration of the cervical–vaginal microbiome in women with idiopathic infertility. J. Cell. Physiol. 232 (7), 1681–1688. doi: 10.1002/jcp.25806
Cánovas A., Reverter A., DeAtley K. L., Ashley R. L., Colgrave M. L., Fortes M. R. S., et al. (2014). Multi-tissue omics analyses reveal molecular regulatory networks for puberty in composite beef cattle. PloS One 9 (7), e102551. doi: 10.1371/journal.pone.0102551
Cardoso R. C., West S. M., Maia T. S., Alves B. R. C., Williams G. L. (2020). Nutritional control of puberty in the bovine female: Prenatal and early postnatal regulation of the neuroendocrine system. Domest. Anim. Endocrinol. 73, 106434. doi: 10.1016/j.domaniend.2020.106434
Chan H. Y., Moldenhauer L. M., Groome H. M., Schjenken J. E., Robertson S. A. (2021). Toll-like receptor-4 null mutation causes fetal loss and fetal growth restriction associated with impaired maternal immune tolerance in mice. Sci. Rep. 11 (1), 16569. doi: 10.1038/s41598-021-95213-1
Chen C., Song X., Wei W., Zhong H., Dai J., Lan Z., et al. (2017). The microbiota continuum along the female reproductive tract and its relation to uterine-related diseases. Nat. Commun. 8 (1), 875. doi: 10.1038/s41467-017-00901-0
Clemmons B. A., Mulon P.-Y., Anderson D. E., Ault-Seay T. B., Henniger M. T., Schneider L. G., et al. (2022). Ruminal bacterial communities and metabolome variation in beef heifers divergent in feed efficiency. Ruminants 2 (2), 282–296. doi: 10.3390/ruminants2020019
Clemmons B. A., Reese S. T., Dantas F. G., Franco G. A., Smith T. P. L., Adeyosoye O. I., et al. (2017). Vaginal and uterine bacterial communities in postpartum lactating cows. Front. Microbiol. 8. doi: 10.3389/fmicb.2017.01047
Comtet-Marre S., Parisot N., Lepercq P., Chaucheyras-Durand F., Mosoni P., Peyretaillade E., et al. (2017). Metatranscriptomics reveals the active bacterial and eukaryotic fibrolytic communities in the rumen of dairy cow fed a mixed diet. Front. Microbiol. 8 (67). doi: 10.3389/fmicb.2017.00067
Cronin J. G., Turner M. L., Goetze L., Bryant C. E., Sheldon I. M. (2012). Toll-like receptor 4 and MYD88-dependent signaling mechanisms of the innate immune system are essential for the response to lipopolysaccharide by epithelial and stromal cells of the bovine endometrium. Biol. Reprod. 86 (2), 1–9. doi: 10.1095/biolreprod.111.092718
Crouse M. S., Greseth N. P., McLean K. J., Crosswhite M. R., Pereira N. N., Ward A. K., et al. (2019). Maternal nutrition and stage of early pregnancy in beef heifers: Impacts on hexose and AA concentrations in maternal and fetal fluids. J. Anim. Sci. 97 (3), 1296–1316. doi: 10.1093/jas/skz013
Cunningham H. C., Austin K. J., Powell S. R., Carpenter K. T., Cammack K. M. (2018). Potential response of the rumen microbiome to mode of delivery from birth through weaning. Trans. Anim. Sci. 2 (1), S35–S38. doi: 10.1093/tas/txy029
Dadarwal D., Palmer C., Griebel P. (2017). Mucosal immunity of the postpartum bovine genital tract. Theriogenol 104, 62–71. doi: 10.1016/j.theriogenology.2017.08.010
Dasari S., Rajendra W., Valluru L. (2014). Evaluation of microbial enzymes in normal and abnormal cervicovaginal fluids of cervical dysplasia: A case control study. BioMed. Res. Internat. 2014, 716346. doi: 10.1155/2014/716346
Dasgupta S., Arya S., Choudhary S., Jain S. K. (2016). Amniotic fluid: Source of trophic factors for the developing intestine. World J. Gastrointest. Pathophysiol. 7 (1), 38–47. doi: 10.4291/wjgp.v7.i1.38
Davies D., Meade K. G., Herath S., Eckersall P. D., Gonzalez D., White J. O., et al. (2008). Toll-like receptor and antimicrobial peptide expression in the bovine endometrium. Reprod. Biol. Endocrinol. 6 (1), 53. doi: 10.1186/1477-7827-6-53
Davis K. F., Gephart J. A., Emery K. A., Leach A. M., Galloway J. N., D’Odorico P. (2016). Meeting future food demand with current agricultural resources. Global Environ. Change 39, 125–132. doi: 10.1016/j.gloenvcha.2016.05.004
Dawuda P. M., Scaramuzzi R. J., Leese H. J., Hall C. J., Peters A. R., Drew S. B., et al. (2002). Effect of timing of urea feeding on the yield and quality of embryos in lactating dairy cows. Theriogenol 58 (8), 1443–1455. doi: 10.1016/S0093-691X(02)00973-1
Day M. L., Imakawa K., Wolfe P. L., Kittok R. J., Kinder J. E. (1987). Endocrine mechanisms of puberty in heifers. role of hypothalamo-pituitary estradiol receptors in the negative feedback of estradiol on luteinizing hormone secretion. Biol. Reprod. 37 (5), 1054–1065. doi: 10.1095/biolreprod37.5.1054
de Goffau M. C., Lager S., Sovio U., Gaccioli F., Cook E., Peacock S. J., et al. (2019). Human placenta has no microbiome but can contain potential pathogens. Nature 572 (7769), 329–334. doi: 10.1038/s41586-019-1451-5
Deng Q., Odhiambo J. F., Farooq U., Lam T., Dunn S. M., Ametaj B. N. (2015). Intravaginal lactic acid bacteria modulated local and systemic immune responses and lowered the incidence of uterine infections in periparturient dairy cows. PloS One 10 (4), e0124167. doi: 10.1371/journal.pone.0124167
Dimberg A. (2010). The chemokine system in experimental and clinical hematology. Curr. Top. Microbiol. Immunol. 341, 3–12. doi: 10.1007/82_2010_18
Diskin M. G., Kenny D. A. (2016). Managing the reproductive performance of beef cows. Theriogenol 86 (1), 379–387. doi: 10.1016/j.theriogenology.2016.04.052
Dominguez-Bello M. G., Costello E. K., Contreras M., Magris M., Hidalgo G., Fierer N., et al. (2010). Delivery mode shapes the acquisition and structure of the initial microbiota across multiple body habitats in newborns. Proc. Natl. Acad. Sci. United States America 107 (26), 11971–11975. doi: 10.1073/pnas.1002601107
Dong H., Tang X., Zhang Z., Dong C. (2017). Structural insight into lipopolysaccharide transport from the gram-negative bacterial inner membrane to the outer membrane. Biochim. Biophys. Acta (BBA) - Mol. Cell Biol. Lipids 1862 (11), 1461–1467. doi: 10.1016/j.bbalip.2017.08.003
Ealy A. D., Speckhart S. L., Wooldridge L. K. (2021). Cytokines that serve as embryokines in cattle. Animals 11 (8), 2313. doi: 10.3390/ani11082313
Elrod C., Butler W. (1993). Reduction of fertility and alteration of uterine pH in heifers fed excess ruminally degradable protein. J. Anim. Sci. 71, 694–701. doi: 10.2527/1993.713694x
Eschenbach D. A., Thwin S. S., Patton D. L., Hooton T. M., Stapleton A. E., Agnew K., et al. (2000). Influence of the normal menstrual cycle on vaginal tissue, discharge, and microflora. Clin. Infect. Dis. 30 (6), 901–907. doi: 10.1086/313818
Espey L. L., Bellinger A. S., Healy J. A. (2004). “Chapter 9 - ovulation: An inflammatory cascade of gene expression,” in The ovary, 2nd ed. Eds. Leung P. C. K., Adashi E. Y. (San Diego: Academic Press) 145–165.
Esquivel-Elizondo S., Ilhan Z. E., Garcia-Peña E. I., Krajmalnik-Brown R., Beiko R. G. (2017). Insights into butyrate production in a controlled fermentation system via gene predictions. Appl. Environ. Sci. 2 (4), e00051–e00017. doi: 10.1128/mSystems.00051-17
Forde N., Simintiras C. A., Sturmey R., Mamo S., Kelly A. K., Spencer T. E., et al. (2014). Amino acids in the uterine luminal fluid reflects the temporal changes in transporter expression in the endometrium and conceptus during early pregnancy in cattle. PloS One 9 (6), e100010. doi: 10.1371/journal.pone.0100010
Fortes M. R. S., Zacchi L. F., Nguyen L. T., Raidan F., Weller M.M.D.C.A., Choo J. J. Y., et al. (2018). Pre- and post-puberty expression of genes and proteins in the uterus of bos indicus heifers: the luteal phase effect post-puberty. Animal Genetics 49, 6, 539–549. doi: 10.1111/age.12721
Fu Y., Liu B., Feng X., Liu Z., Liang D., Li F., et al. (2013). Lipopolysaccharide increases toll-like receptor 4 and downstream toll-like receptor signaling molecules expression in bovine endometrial epithelial cells. Vet. Immunol. Immunopathol. 151 (1), 20–27. doi: 10.1016/j.vetimm.2012.09.039
Fu X., Liu Z., Zhu C., Mou H., Kong Q. (2019). Nondigestible carbohydrates, butyrate, and butyrate-producing bacteria. Crit. Rev. Food Sci. Nutr. 59 (1), S130–S152. doi: 10.1080/10408398.2018.1542587
Furusawa Y., Obata Y., Fukuda S., Endo T. A., Nakato G., Takahashi D., et al. (2013). Commensal microbe-derived butyrate induces the differentiation of colonic regulatory T cells. Nature 504 (7480), 446–450. doi: 10.1038/nature12721
Gajer P., Brotman R. M., Bai G., Sakamoto J., Schutte U., Zhong X., et al. (2012). Temporal dynamics of the human vaginal microbiota. Sci. Transl. Med. 4 (132), 1–12. doi: 10.1126/scitranslmed.3003605
Galvão K. N., Bicalho R. C., Jeon S. J. (2019). Symposium review: The uterine microbiome associated with the development of uterine disease in dairy cows. J. Dairy Sci. 101(12), 11786–11797. doi: 10.3168/jds.2019-17106
Gao H., Wu G., Spencer T. E., Johnson G. A., Li X., Bazer F. W. (2009). Select nutrients in the ovine uterine lumen. i. amino acids, glucose, and ions in uterine lumenal flushings of cyclic and pregnant ewes. Biol. Reprod. 80 (1), 86–93. doi: 10.1095/biolreprod.108.071597
Garcia-Grau I., Simon C., Moreno I. (2018). Uterine microbiome–low biomass and high expectations†. Biol. Reprod. 101 (6), 1102–1114. doi: 10.1093/biolre/ioy257
Genís S., Cerri R. L. A., Bach À., Silper B. F., Baylão M., Denis-Robichaud J., et al. (2018). Pre-calving intravaginal administration of lactic acid bacteria reduces metritis prevalence and regulates blood neutrophil gene expression after calving in dairy cattle. Front. Vet. Sci. 5. doi: 10.3389/fvets.2018.00135
Genís S., Sánchez-Chardi A., Bach À., Fàbregas F., Arís A. (2017). A combination of lactic acid bacteria regulates escherichia coli infection and inflammation of the bovine endometrium. J. Dairy Sci. 100 (1), 479–492. doi: 10.3168/jds.2016-11671
Gray C., Burghardt R., Johnson G., Bazer F., Spencer T. (2002). Evidence that absence of endometrial gland secretions in uterine gland knockout ewes compromises conceptus survival and elongation. Reprod 124 (2), 289–300. doi: 10.1530/rep.0.1240289
Gregoire A. T., Parakkal P. F. (1972). Glycogen content in the vaginal tissue of normally cycling and estrogen and progesterone-treated rhesus monkeys. Biol. Reprod. 7 (1), 9–14. doi: 10.1093/biolreprod/7.1.9
Groebner A. E., Rubio-Aliaga I., Schulke K., Reichenbach H. D., Daniel H., Wolf E., et al. (2011). Increase of essential amino acids in the bovine uterine lumen during preimplantation development. J. Reprod. 141 (5), 685–695. doi: 10.1530/rep-10-0533
Guzman C. E., Wood J. L., Egidi E., White-Monsant A. C., Semenec L., Grommen S. V. H., et al. (2020). A pioneer calf foetus microbiome. Sci. Rep. 10, 17712. doi: 10.1038/s41598-020-74677-7
Harlow K., Taylor E., Casey T., Hedrick V., Sobreira T., Aryal U. K., et al. (2018). Diet impacts pre-implantation histotroph proteomes in beef cattle. J. Proteome Res. 17 (6), 2144–2155. doi: 10.1021/acs.jproteome.8b00077
Hashem N. M., Gonzalez-Bulnes A. (2022). The use of probiotics for management and improvement of reproductive eubiosis and function. Nutrients 14 (4), 902. doi: 10.3390/nu14040902
Hespell R. B., Akin D. E., Dehority B. A., Mackie R. I., White B. A. (1997). Bacteria, fungi and protozoa of the rumen. Gastrointest Microbiol. 2, 325–332. doi: 10.1007/978-1-4757-0322-1_3
Hirata T., Osuga Y., Hamasaki K., Hirota Y., Nose E., Morimoto C., et al. (2007). Expression of toll-like receptors 2, 3, 4, and 9 genes in the human endometrium during the menstrual cycle. J. Reprod. Immunol. 74 (1), 53–60. doi: 10.1016/j.jri.2006.11.004
Hummel G. L., Woodruff K. L., Austin K. J., Smith T. L., Cunningham-Hollinger H. C. (2020). Evidence for the amnion-fetal gut-microbial axis in late gestation beef calves. Trans. Anim. Sci. 4 (1), S174–S177. doi: 10.1093/tas/txaa138
Husso A., Lietaer L., Pessa-Morikawa T., Grönthal T., Govaere J., Van Soom A., et al. (2021). The composition of the microbiota in the full-term fetal gut and amniotic fluid: a bovine cesarean section study. Front. Microbiol. 12 (920). doi: 10.3389/fmicb.2021.626421
Jeon S. J., Cunha F., Vieira-Neto A., Bicalho R. C., Lima S., Bicalho M. L., et al. (2017). Blood as a route of transmission of uterine pathogens from the gut to the uterus in cows. Microbiome 5 (1), 109. doi: 10.1186/s40168-017-0328-9
Jiménez E., Fernández L., Marín M. L., Martín R., Odriozola J. M., Nueno-Palop C., et al. (2005). Isolation of commensal bacteria from umbilical cord blood of healthy neonates born by cesarean section. Curr. Microbiol. 51, 270–274. doi: 10.1007/s00284-005-0020-3
Jiménez E., Marín M. L., Martín R., Odriozola J. M., Olivares M., Xaus J., et al. (2008). Is meconium from healthy newborns actually sterile? Res. Microbiol. 159 (3), 187–193. doi: 10.1016/j.resmic.2007
Karstrup C. C., Klitgaard K., Jensen T. K., Agerholm J. S., Pedersen. H. G. (2017). Presence of bacteria in the endometrium and placentomes of pregnant cows. Theriogenol 99, 41–47. doi: 10.1016/j.theriogenology.2017.05.013
Killeen A. P., Morris D. G., Kenny D. A., Mullen M. P., Diskin M. G., Waters S. M. (2014). Global gene expression in endometrium of high and low fertility heifers during the mid-luteal phase of the estrous cycle. BMC Genomics 15 (1), 234. doi: 10.1186/1471-2164-15-234
Krakowski L., Zdzisinska B. (2007). Selected cytokines and acute phase proteins in heifers during the ovarian cycle course and in different pregnancy periods. J. Bulletin-Veterinary Institute Pulawy 51 (1), 31.
Laguardia-Nascimento M., Branco K. M., Gasparini M. R., Giannattasio-Ferraz S., Leite L. R., Araujo F. M., et al. (2015). Vaginal microbiome characterization of nellore cattle using metagenomic analysis. PloS One 10 (11), e0143294. doi: 10.1371/journal.pone.0143294
LeBlanc S. J., Duffield T. F., Leslie K. E., Bateman K. G., Keefe G. P., Walton J. S., et al. (2002). Defining and diagnosing postpartum clinical endometritis and its impact on reproductive performance in dairy cows. J. Dairy Sci. 85 (9), 2223–2236. doi: 10.3168/jds.S0022-0302(02)74302-6
Leiby J. S., McCormick K., Sherrill-Mix S., Clarke E. L., Kessler L. R., Taylor L. J., et al. (2018). Lack of detection of a human placenta microbiome in samples from preterm and term deliveries. Microbiome 6 (1), 196. doi: 10.1186/s40168-018-0575-4
Lima S. F., Bicalho M. L. S., Bicalho R. C. (2019). The bos taurus maternal microbiome: Role in determining the progeny early-life upper respiratory tract microbiome and health. PloS One 14 (3), e0208014. doi: 10.1371/journal.pone.0208014
Lu Y.-C., Yeh W. C., Ohashi P. S. (2008). LPS/TLR4 signal transduction pathway. Cytokine 42 (2), 145–151. doi: 10.1016/j.cyto.2008.01.006
Machado V. S., Oikonomou G., Bicalho M. L. S., Knauer W. A., Gilbert R., Bicalho R. C. (2012). Investigation of postpartum dairy cows’ uterine microbial diversity using metagenomic pyrosequencing of the 16S rRNA gene. Vet. Microbiol. 159 (3), 460–469. doi: 10.1016/j.vetmic.2012.04.033
Mao S., Zhang M., Liu J., Zhu W. (2015). Characterising the bacterial microbiota across the gastrointestinal tracts of dairy cattle: Membership and potential function. Sci. Rep. 5, 16116. doi: 10.1038/srep16116
Messman R. D., Contreras-Correa Z. E., Paz H. A., Perry G., Lemley C. O. (2020). Vaginal bacterial community composition and concentrations of estradiol at time of artificial insemination in brangus heifers. J. Anim. Sci. 98 (6), 1–9. doi: 10.1093/jas/skaa178
Mihm M., Gangooly S., Muttukrishna S. (2011). The normal menstrual cycle in women. Anim. Reprod. Sci. 124 (3), 229–236. doi: 10.1016/j.anireprosci.2010.08.030
Mirmonsef P., Hotton A. L., Gilbert D., Burgad D., Landay A., Weber K. M., et al. (2014). Free glycogen in vaginal fluids is associated with lactobacillus colonization and low vaginal pH. PloS One 9 (7), e102467. doi: 10.1371/journal.pone.0102467
Mitchell C. M., Haick A., Nkwopara E., Garcia R., Rendi M., Agnew K., et al. (2015). Colonization of the upper genital tract by vaginal bacterial species in nonpregnant women. Am. J. Obstet. Gynecol. 212 (5), 611.e611–611.e619. doi: 10.1016/j.ajog.2014.11.043
Moore S. G., Ericsson A. C., Poock S. E., Melendez P., Lucy M. C. (2017). Hot topic: 16S rRNA gene sequencing reveals the microbiome of the virgin and pregnant bovine uterus. J. Dairy Sci. 100 (6), 4953–4960. doi: 10.3168/jds.2017-12592
Moreno I., Codoñer F. M., Vilella F., Valbuena D., Martinez-Blanch J. F., Jimenez-Almazán J., et al. (2016). Evidence that the endometrial microbiota has an effect on implantation success or failure. Am. J. Obstet. Gynecol. 215 (6), 684–703. doi: 10.1016/j.ajog.2016.09.075
Moreno I., Simon C. (2018). Relevance of assessing the uterine microbiota in infertility. Fertil. Steril. 110 (3), 337–343. doi: 10.1016/j.fertnstert.2018.04.041
Mullen M. P., Elia G., Hilliard M., Parr M. H., Diskin M. G., Evans A. C. O., et al. (2012). Proteomic characterization of histotroph during the preimplantation phase of the estrous cycle in cattle. J. Proteome Res. 11 (5), 3004–3018. doi: 10.1021/pr300144q
Myer P. R., Smith T. P. L., Wells J. E., Kuehn L. A., Freetly H. C. (2015). Rumen microbiome from steers differing in feed efficiency. PloS One 10 (6), e0129174. doi: 10.1371/journal.pone.0129174
Nair A. R., Taylor H. S. (2010). “The mechanism of menstruation,” in Amenorrhea: A case-based, clinical guide. Eds. Santoro N. F., Neal-Perry G. (Totowa, NJ: Humana Press), 21–34.
NIH HMP Working Group, Peterson J., Garges S., Giovanni M., McInnes P., Wang L., et al. (2009). The NIH human microbiome project. Genome Res. 19 (12), 2317–2323. doi: 10.1101/gr.096651.109
Ocon O. M., Hansen P. J. (2003). Disruption of bovine oocytes and preimplantation embryos by urea and acidic pH. J. Dairy Sci. 86 (4), 1194–1200. doi: 10.3168/jds.S0022-0302(03)73703-5
O’Hanlon D. E., Moench T. R., Cone R. A. (2013). Vaginal pH and microbicidal lactic acid when lactobacilli dominate the microbiota. PloS One 8 (11), e80074. doi: 10.1371/journal.pone.0080074
Oliveira L. J., Mansourri-Attia N., Fahey A. G., Browne J., Forde N., Roche J. F., et al. (2013). Characterization of the Th profile of the bovine endometrium during the oestrous cycle and early pregnancy. PloS One 8 (10), 1–13. doi: 10.1371/journal.pone.0075571
Payne M. S., Bayatibojakhi S. (2014). Exploring preterm birth as a polymicrobial disease: An overview of the uterine microbiome. Front. Immunol. 5. doi: 10.3389/fimmu.2014.00595
Penny L. A., Armstrong D., Bramley T. A., Webb R., Collins R. A., Watson E. D. (1999). Immune cells and cytokine production in the bovine corpus luteum throughout the oestrous cycle and after induced luteolysis. J. Reprod. 115 (1), 87–96. doi: 10.1530/jrf.0.1150087
Perez P. F., Doré J., Leclerc M., Levenez F., Benyacoub J., Serrant P., et al. (2007). Bacterial imprinting of the neonatal immune system: Lessons from maternal cells? Pediatrics 119 (3), e724–e732. doi: 10.1542/peds.2006-1649
Perez-Muñoz M. E., Arrieta M. C., Ramer-Tait A. E., Walter J. (2017). A critical assessment of the “sterile womb” and “in utero colonization” hypotheses: implications for research on the pioneer infant microbiome. Microbiome 5, 48. doi: 10.1186/s40168-017-0268-4
Peter S., Gärtner M. A., Michel G., Ibrahim M., Klopfleisch R., Lübke-Becker A., et al. (2018). Influence of intrauterine administration of lactobacillus buchneri on reproductive performance and pro-inflammatory endometrial mRNA expression of cows with subclinical endometritis. Sci. Rep. 8 (1), 5473. doi: 10.1038/s41598-018-22856-y
Pickett A. T., Cooke R. F., Mackey S. J., Brandão A. P., Colombo E. A., Oliveira Filho R. V., et al. (2022). Shifts in bacterial communities in the rumen, vagina and uterus of beef heifers receiving different levels of concentrate. J. Anim. Sci. 100(12), 1–11. doi: 10.1093/jas/skac338
Poole R. K., Ault-Seay T. B., Payton R. R., Myer P. R., Lear A. S., Pohler K. G. (2021). Evaluation of reproductive tract cytokines in post-partum beef cows relating to reproductive microbiota and fertility outcomes. Front. Anim. Sci. 2 (20). doi: 10.3389/fanim.2021.704714
Poole R. K., Pickett A. T., Oliveira Filho R. V., de Melo G. D., Palanisamy V., Chitlapilly Dass S., et al. (2022). Shifts in uterine bacterial communities associated with endogenous progesterone and 17β-estradiol concentrations in beef cattle. Domest. Anim. Endocrinol. 82, 106766. doi: 10.1016/j.domaniend.2022.106766
Quereda J. J., Barba M., Mocé M. L., Gomis J., Jiménez-Trigos E., García-Muñoz Á., et al. (2020). Vaginal microbiota changes during estrous cycle in dairy heifers. Front. Vet. Sci. 7 (371). doi: 10.3389/fvets.2020.00371
Raghupathy R. (2001). Pregnancy: Success and failure within the Th1/Th2/Th3 paradigm. Semin. Immunol. 13 (4), 219–227. doi: 10.1006/smim.2001.0316
Ravel J., Gajer P., Abdo Z., Schneider G. M., Koenig S. S. K., McCulle S. L., et al. (2011). Vaginal microbiome of reproductive-age women. Proc. Natl. Acad. Sci. United States America 108 (1), 4680. doi: 10.1073/pnas.1002611107
Reid J. N. S., Bisanz J. E., Monachese M., Burton J. P., Reid G. (2013). The rationale for probiotics improving reproductive health and pregnancy outcome. Am. J. Reprod. Immunol. 69 (6), 558–566. doi: 10.1111/aji.12086
Ribeiro E. S., Lima F. S., Greco L. F., Bisinotto R. S., Monteiro A. P. A., Favoreto M., et al. (2013). Prevalence of periparturient diseases and effects on fertility of seasonally calving grazing dairy cows supplemented with concentrates. J. Dairy Sci. 96 (9), 5682–5697. doi: 10.3168/jds.2012-6335
Rönnqvist D. J. P., Forsgren-Brusk B. U., Grahn-Håkansson E. E. (2006). Lactobacilli in the female genital tract in relation to other genital microbes and vaginal pH. Acta obstetricia et gynecologica Scandinavica 85, 6, 726–735. doi: 10.1080/00016340600578357
Rosales E. B., Ametaj B. N. (2021). Reproductive tract infections in dairy cows: Can probiotics curb down the incidence rate? Dairy 2 (1), 40–64. doi: 10.3390/dairy2010004
Schillo K. K., Dierschke D. J., Hauser E. R. (1982). Regulation of luteinizing hormone secretion in prepubertal heifers: increased threshold to negative feedback action of estradiol. J. Anim. Sci. 54 (2), 325–336. doi: 10.2527/jas1982.542325x
Schjenken J. E., Tolosa J. M., Paul J. W., Clifton V. L., Smith R. (2012). “Mechanisms of maternal immune tolerance during pregnancy,” in Recent advances in research on the human placenta. Ed. Zheng J. (InTech), 211–242.
Sender R., Fuchs S., Milo R. (2016). Revised estimates for the number of human and bacteria cells in the body. PloS Biol. 14 (8), e1002533. doi: 10.1371/journal.pbio.1002533
Sheldon I. M., Cronin J., Goetze L., Donofrio G., Schuberth H. J. (2009). Defining postpartum uterine disease and the mechanisms of infection and immunity in the female reproductive tract in cattle. Biol. Reprod. 81 (6), 1025–1032. doi: 10.1095/biolreprod.109.077370
Sheldon I. M., Dobson H. (2004). Postpartum uterine health in cattle. Anim. Reprod. Sci. 82-83, 295–306. doi: 10.1016/j.anireprosci.2004.04.006
Sheldon I., Owens S. E. (2017). Postpartum uterine infection and endometritis in dairy cattle. Anim. Reprod. 14 (3), 622–629. doi: 10.21451/1984-3143-AR1006
Shima T., Sasaki Y., Itoh M., Nakashima A., Ishii N., Sugamura K., et al. (2010). Regulatory T cells are necessary for implantation and maintenance of early pregnancy but not late pregnancy in allogeneic mice. J. Reprod. Immunol. 85 (2), 121–129. doi: 10.1016/j.jri.2010.02.006
Simón C., Moreno C., Remohıí J., Pellicer A. (1998). Cytokines and embryo implantation. J. Reprod. Immunol. 39 (1), 117–131. doi: 10.1016/S0165-0378(98)00017-5
Sterpu I., Fransson E., Hugerth L. W., Du J., Pereira M., Cheng L., et al. (2021). No evidence for a placental microbiome in human pregnancies at term. Am. J. Obstet. Gynecol. 224 (3), 296.e291–296.e223. doi: 10.1016/j.ajog.2020.08.103
Stinson L. F., Keelan J. A., Payne M. S. (2018). Comparison of meconium DNA extraction methods for use in microbiome studies. Front. Microbiol. 9. doi: 10.3389/fmicb.2018.00270
Swangchan-Uthai T., Lavender C. R. M., Cheng Z., Fouladi-Nashta A. A., Wathes D. C. (2012). Time course of defense mechanisms in bovine endometrium in response to lipopolysaccharide. Biol. Reprod. 87 (6), 1–13. doi: 10.1095/biolreprod.112.102376
Swartz J. D., Lachman M., Westveer K., O’Neill T., Geary T., Kott R. W., et al. (2014). Characterization of the vaginal microbiota of ewes and cows reveals a unique microbiota with low levels of lactobacilli and near-neutral pH. Front. Vet. Sci. 1 (19). doi: 10.3389/fvets.2014.00019
Tabibzadeh S. (1991). Human endometrium: An active site of cytokine production and action. J. Endocrine Rev. 12 (3), 272–290. doi: 10.1210/edrv-12-3-272
The Human Microbiome Project, C (2012). Structure, function and diversity of the healthy human microbiome. Nature 486 (7402), 207–214. doi: 10.1038/nature11234
United Nations Department of Economic and Social Affairs, Population Division (2022). World Population Prospects 2022: Summary of Results. UN DESA/POP/2022/TR/NO. 3.
Uyeno Y., Shigemori S., Shimosato T. (2015). Effect of probiotics/prebiotics on cattle health and productivity. Microbes Environ. 30 (2), 126–132. doi: 10.1264/jsme2.ME14176
Vujic G., Jajac Knez A., Despot Stefanovic V., Kuzmic Vrbanovic V. (2013). Efficacy of orally applied probiotic capsules for bacterial vaginosis and other vaginal infections: A double-blind, randomized, placebo-controlled study. Eur. J. Obstet. Gynecol. 168 (1), 75–79. doi: 10.1016/j.ejogrb.2012.12.031
Wang L., Li Y., Zhang Y., Wang L. (2020). The effects of different concentrate-to-forage ratio diets on rumen bacterial microbiota and the structures of Holstein cows during the feeding cycle. Animals 10 (6), 957. doi: 10.3390/ani10060957
Weinroth M. D., Belk A. D., Dean C., Noyes N., Dittoe D. K., Rothrock M. J., et al. (2022). Considerations and best practices in animal science 16S ribosomal RNA gene sequencing microbiome studies. J. Anim. Sci. 100 (2), 1–18. doi: 10.1093/jas/skab346
Woodruff K. L., Hummel G. L., Austin K. J., Smith T. L., Cunningham-Hollinger H. C. (2020). Influence of the maternal rumen microbiome on development of the calf meconium and rumen microbiome1. Trans. Anim. Sci. 4 (1), S169–S173. doi: 10.1093/tas/txaa136
Wrenn T. R., Bitman J., Wood J. R. (1968). Influence of local estrogen administration on vaginal glycogen. Endocrinol 82 (1), 62–68. doi: 10.1210/endo-82-1-62
Yildirim S., Yeoman C. J., Janga S. C., Thomas S. M., Ho M., Leigh S. R., et al. (2014). Primate vaginal microbiomes exhibit species specificity without universal lactobacillus dominance. ISME J. 8 (12), 2431–2444. doi: 10.1038/ismej.2014.90
Yoo I., Han J., Lee S., Jung W., Kim J. H., Kim Y. W., et al. (2019). Analysis of stage-specific expression of the toll-like receptor family in the porcine endometrium throughout the estrous cycle and pregnancy. Theriogenol 125, 173–183. doi: 10.1016/j.theriogenology.2018.11.003
Zebeli Q., Metzler-Zebeli B. U., Ametaj B. N. (2012). Meta-analysis reveals threshold level of rapidly fermentable dietary concentrate that triggers systemic inflammation in cattle. J. Dairy Sci. 95 (5), 2662–2672. doi: 10.3168/jds.2011-5080
Zhang M., Zhou Q., Dorfman R. G., Huang X., Fan T., Zhang H., et al. (2016). Butyrate inhibits interleukin-17 and generates tregs to ameliorate colorectal colitis in rats. BMC Gastroenterol. 16 (1), 84. doi: 10.1186/s12876-016-0500-x
Keywords: bacteria, bovine, fertility, microbiome, uterus
Citation: Ault-Seay TB, Moorey SE, Mathew DJ, Schrick FN, Pohler KG, McLean KJ and Myer PR (2023) Importance of the female reproductive tract microbiome and its relationship with the uterine environment for health and productivity in cattle: A review. Front. Anim. Sci. 4:1111636. doi: 10.3389/fanim.2023.1111636
Received: 29 November 2022; Accepted: 23 January 2023;
Published: 02 February 2023.
Edited by:
Adem Kara, Erzurum Technical University, TürkiyeReviewed by:
Joyce A. Ibana, University of the Philippines Diliman, PhilippinesAspinas Chapwanya, Ross University School of Veterinary Medicine, Saint Kitts and Nevis
Copyright © 2023 Ault-Seay, Moorey, Mathew, Schrick, Pohler, McLean and Myer. This is an open-access article distributed under the terms of the Creative Commons Attribution License (CC BY). The use, distribution or reproduction in other forums is permitted, provided the original author(s) and the copyright owner(s) are credited and that the original publication in this journal is cited, in accordance with accepted academic practice. No use, distribution or reproduction is permitted which does not comply with these terms.
*Correspondence: Phillip R. Myer, cG15ZXJAdXRrLmVkdQ==; Kyle J. McLean, a21jbGVhMTBAdXRrLmVkdQ==