- 1Research and Education, CR Eco-efficient Agriculture Consultancy (CREAC®), Bushland Beach, QLD, Australia
- 2Tropical Pastures Program, Formerly International Center for Tropical Agriculture (CIAT), Cali, Colombia
- 3Agriculture, Formerly Commonwealth Scientific and Industrial Research Organisation, (CSIRO), Australian Tropical Sciences and Innovation Precinct, James Cook University, Townsville, QLD, Australia
- 4Agriculture, R. R. Vera Infanzón Private Consultant Services, Viña del Mar, Chile
- 5International Center for Tropical Agriculture (CIAT), Cali, Colombia
Introduction: Previous research has shown increased productivity amongst sown grass pastures compared to native savanna pastures by year-round grazing for fattening of adult and young Brahman (Bos indicus)-bred cattle in the well-drained native savanna ecosystem of the Colombian Orinoquía. But there is limited information on the carbon footprint (CF) of commercial young-Brahman heifers and steers reared throughout life on well-managed Brachiaria decumbens Stapf pastures.
Methods: The present study characterized growth, lifetime enteric methane (CH4) emissions, carcass carbon dioxide equivalent (CO2-eq) CH4 efficiency intensities (i.e., emissions per kg of product), and estimated the overall CF of young cattle grazing B. decumbens pastures subject to a range of daily liveweight gains (DLWGs; 0.428 – 0.516 kg) and fattening framework (405 – 574 kg). Weaning data from seven consecutive calving seasons in a commercial Brahman breeding herd continuously grazed on B. decumbens were integrated with a Microsoft Excel® dynamic greenhouse gas emission (GHGE) simulation of stockers-yearlings, and seven fattening, and processing scenarios.
Results: The model predicted that heifers subject to low and high DLWGs (0.428 vs 0.516 kg) and steers (0.516 kg) may be successfully fattened without supplementation assuming that animals had access to a well-managed grass pasture. Depending on the fattening strategy, kg CO2-eq CH4/kg edible protein values ranged from 66.843 to 87.488 ± 0.497 for heifers and from 69.689 to 91.291 ± 0.446 for steers.
Discussion: Assuming that forage on offer is at least 1,500-2,000 kg of dry matter/ha during the rainy season, all the simulated systems showed potential for C neutrality and net-zero C emission when considering GHGEs from the soil, pasture, and animal components vs the estimated soil C capture over seven seasons. However, under a more optimistic scenario, these beef systems could accomplish substantial net gains of soil C, over the period for which field data are available. Overall, this study projects the positive impact of the design of plausible fattening strategies on grasslands for improving cattle productivity and reducing emission intensities with concomitant increases in technical efficiency.
Introduction
Emerging agri-food production systems aim to achieve food security and environmental sustainability while reducing poverty through technological innovations, alternative food sources, safe supply chains, affordable diets, food preferences, and lifestyle shifting, among other factors (Food and Agriculture Organization (FAO), 2022). However, it is still unclear how the new agri-food systems will meet regulatory pathways, consumer perspectives, planetary health expectations, and multidimensional livelihoods (Stephens et al., 2018; FAO, 2022). For example, when available, lab-cellular meat could have a larger detrimental carbon footprint (CF) impact than the environmental imprint from conventional beef farming systems (BFS) (Lynch and Pierrehumbert, 2019; Chriki and Hocquette, 2020).
In Latin America and the Caribbean, livestock systems operated by 60 million people generate 41.5% of the agricultural gross domestic product in the region and supply 11% and 23% of the milk and beef global market, respectively (FAO, 2021). In the specific case of cattle farming systems, their sustainability scales depend on several factors. These include (i) animal metabolism and animal farm exchanges (Vera and Ramírez-Restrepo, 2017; De Rosa et al., 2021); (ii) generation of ecosystem services (Rao et al., 2015); (iii) animal nutrition based on the adequacy of concentrates, crop-based biomass, industrial-scale by-products, and forage resources (Ramírez-Restrepo et al., 2017; Cumbe et al., 2021; Marín-López et al., 2022); (iv) participatory adaptation to climate variability (Marshall and Smajgl, 2013; Sen et al., 2022); and (v) socio-cultural and livelihoods functions (Marshall et al., 2014; Sen et al., 2021).
The Orinoco River Basin extends over 1.1 million km2 encompassing the Pre-Cambrian Guyana Shield, Colombia, and Venezuela (Frappart et al., 2015). In this context, from a resource-use perspective (Greenwood, 2021), the 170,000 km2 of grassland savannas (i.e., Llanos; Romero-Ruiz et al., 2011) within the biodiversity and natural transitions of 205 ecosystems of the Colombian Orinoquía (347,208 km2; Instituto de Investigación de Recursos Biológicos Alexander von Humboldt and Deutsche Gesellschaft für Internationale Zusammenarbeit GmbH, 2020) constitute a good example of some of the challenges faced by the BFS. This grassland savanna is a fragile and complex riverine ecosystem encompassing Amerindian groups, cultural history, socio-political dynamics, traditional ecological knowledge, and biodiversity (Navas Ríos, 1999; Gassón, 2002).
Biodiversity dynamics in the Colombian Orinoquía encompass 191 amphibians, 1,043 birds, 663 fish, 254 mammals, 155 reptiles, 5,411 plants, and 680 insect species (Instituto de Investigación de Recursos Biológicos Alexander von Humboldt and Deutsche Gesellschaft für Internationale Zusammenarbeit GmbH, 2020). To the authors’ knowledge, there are no inventories that describe the details of insect fauna, distribution, biological and ecological differences, habitats, host plants, and plant-herbivore interactions in the Llanos dealing with the effects of grassland savanna conversion to improved grass monocultures or grass-legume pastures.
Previous work indicated that there are opportunities for the intensification of beef farming through the careful use of natural resources including the well-drained native savanna ecosystem (WNSE) landscape, limited external inputs, newer technical knowledge, and enhanced management expertise (Ramírez-Restrepo et al., 2019a; Vera-Infanzón et al., 2023). Lavelle et al. (2014) evaluated soil macroinvertebrate communities and soil-based ecosystem services (climate regulation, hydrologic functions, soil stability provided by macro aggregation, and nutrient provision potential) in four major production systems of the Orinoco River Basin of Colombia that includes improved pastures, annual crops, oil palm, and rubber plantations, and compared them to the original savanna. They found that indicators for macroinvertebrate biodiversity and activity and soil macro aggregation were highest within improved pastures. According to Decaëns et al. (2001), termites and earthworms are the dominant components of macrofauna communities in the WNSE while the abundance and diversity of macroinvertebrate communities are determined by the structure and the nature of the vegetation. Therefore, the conversion of the native savanna into an improved pasture, either grass alone or mixed with legumes, maintains the macrofauna taxonomy richness and increases up to almost 13 times the macrofauna biomass (Decaëns et al., 2001). This is mainly due to the use of the Brachiaria decumbens Stapf cultivar Basilisk in association with the herbaceous Pueraria phaseoloides (accession CIAT 9900 - kudzú) legume and the increase of organic matter from leaf litter and cattle manure (Jiménez et al., 1998).
Recent studies on cow-calf Brahman (Bos indicus) herds grazing on WNSE (Ramírez-Restrepo et al., 2019b) and B. decumbens (syn. Urochloa decumbens; Ramírez-Restrepo et al., 2020) pastures, and cull cow-fattening systems on B. decumbens pastures (Ramírez-Restrepo et al., 2023) showed that BFS on the WNSE not only reduce environmental impacts by operating from near equilibrium in overall CF balance to net increases in soil organic C (SOC) accumulation but may also contribute toward the United Nation’s Sustainable Development Goals (UN, 2015). In parallel, increased productivity of grass-legume pastures in the WNS ecosystem has been demonstrated by the fattening of adult (Ramírez-Restrepo and Vera, 2019) and young Brahman-influenced cattle (Rincón et al., 2018; Rincón and Villalobos, 2021). Nevertheless, to the authors’ knowledge, no study has assessed the CF of suckler Brahman heifers and steers and their interconnected postweaning growth and finishing systems at pasture level in the WNSE of the Colombian Orinoquía.
In this view, the present study aims to characterize growth, lifetime CH4 emissions, the environmental efficiency of carcass characteristics, and the overall CF of young cattle subject to lifespan-grazing on B decumbens pastures. It is focused on testing the underlying relationships through scenario (SCE) analysis of forage management and consumption, greenhouse gas emissions (GHGE), SOC stocks, and potential C-holding capacity as the components of CF in a single-issue life cycle assessment of a functional farming system (Weiler et al., 2014; Ramírez-Restrepo et al., 2020; González-Quintero et al., 2022; Ramírez-Restrepo et al., 2023). Possible outcomes from SCE analyses are likely to assist the investigation of sustainable circularity of BFS (Mehrabi et al., 2020; Broom, 2021), land-based GHGE removal projects and climate finance research (Costa et al., 2022), and law and policy recommendations (Bowman et al., 2012; Ayarza et al., 2022).
Thus, the present study integrated WNSE weaning data from seven continued calving-weaning seasons in a commercial Brahman breeding herd continuously grazed on B. decumbens using a dynamic Microsoft Excel® GHGE simulation of stockers-yearlings, fattening, and processing phases, and GHG dynamics. We tested the hypothesis that in a WNSE, suckling growth paths would affect lifetime production and the sustainable efficiency of carcass yields in commercial young-Brahman heifers and steers reared throughout life on well-managed B. decumbens pastures. This hypothesis was tested to better understand the GHGE environmental impact of WNSE beef-herd farming systems equated through the life cycle as an integral part of current and future agri-food systems.
Methods
Database
The breeding herd dataset (Vera et al., 2002) extended by Ramírez-Restrepo et al. (2023) was integrated with the herd’s unpublished seventh reproductive cycle (RC; i.e., conception-weaning) at Carimagua Research Centre (latitude 4°36’44.6” N, longitude 74°08’42.2” W) in the Colombian WNSE. Data from 30 cows provided individual liveweight (LW) and age fluctuations across male (n = 55) and heifer (n = 75) calves from birth [28.6 ± 0.50 vs 27.8 ± 0.42 kg (least-squares means ± SEM)] to weaning (183.9 ± 4.80 vs 162.5 ± 4.10 kg and 8.5 ± 0.18 vs 8.4 ± 0.15 months) up to 10.3 ± 0.49 years. Details of the breeding herd on year-round grazing of B. decumbens cultivar Basilisk pastures outlining long-term reproductive efficiency and the allocation of GHG costs to cows and cow-calf pairs were described by Ramírez-Restrepo et al. (2020).
Animal management
Calving events were daily recorded on the paddock while at approximately 4 month intervals, cow’s rectal palpation, and visual body condition score [on a scale of 1 (poor) to 5 (fat)], LWs, branding, and castration of males (steers) at weaning were performed in the yard. Briefly, animals were adequately restrained in cattle crushes to minimize the risk to the welfare of cattle, particularly stress while also ensuring the field staff’s well-being. Branding irons were well maintained and heated correctly to perform a 2-3 seconds blue-hot-iron branding [CIAT’s symbol, and two and three numbers to identify the year of birth and stock sequence, respectively] on a dry hide (near the left side rump) of the animal. Surgical removal of testicles involving enough twist of the spermatic cord and the severed scraping of it to allow quick blood clotting was performed through the implementation of good hygiene practices related to the use of an antiseptic solution in a bucket for hands, scrotum disinfection handling, and instruments (scalpel handles-No 3 and sterile disposable scalpel blades-No 10 changed regularly). Post-surgical healing was also facilitated by the use of antibacterial fly powder and antiseptic spray on the incision before releasing weaners from the yard, monitoring on a clean paddock for 14 days, the use of anti-inflammatory drugs, and previous tetanus (Clostridium tetani) vaccination. In both, branding and castration practices pain minimization strategies were followed.
Weaners from different provenance at Carimagua became stockers and yearlings (i.e., backgrounding) on native savanna (Ramírez-Restrepo and Vera-Infanzón, 2019) and experimental pastures (Lascano and Estrada, 1989; Lascano, 1991) whereas surplus heifers and yearling steers were sold to regional farms. Among the livestock categories, pooled stockers-yearlings extended the efficient utilization of low-input pastures based on the relevance of replacement heifers (Vera-Infanzón and Ramírez-Restrepo, 2020; Vera-Infanzón and Ramírez-Restrepo, 2022) and the association of mixed ages and stock classes (Ramírez-Restrepo and Vera, 2019) of farming systems.
In all cases, cattle had always free access to fresh water plus a commercial mineral supplement containing as-kg fed 137 g Ca, 269 g Cl, 0.01 g Co, 1.038 Cu, 0.076 g I, 175 g Na, 80 g P, 20 g S, and 3.5 g Zn. Supplement intake was recorded on a fortnightly basis. Trial design and implementation were done by accredited Colombian Doctors of Veterinary Medicine according to Colombian husbandry and animal welfare standards and guidelines (MINEDUCATION, 1985).
Climatic factors and soil properties
The climate recorded during the research period at Carimagua’s weather station was humid-very humid (April-November) and dry (December-March) with annual precipitation of 3,300 mm in 1986, dropping to an average of 2,202 mm between 1979 and 1991. Thirteen-year minimum and maximum ambient temperatures were 25.2°C to 28.1°C, respectively (Vera and Ramírez-Restrepo, 2017).
Soils at the site were well-drained clay loam Oxisols (tropeptic haplustox isohyperthermic) with 4.78 pH and 90.1% Al saturation. The 0-20 cm soil layer contained 2.9% organic matter, 40.2% clay, 19.8% sand, 1.46 µg/g available P (Bray-II), and exchangeable (cmol/kg) Ca (0.17), Mg (0.08), and K (0.06; Ramírez-Restrepo et al., 2020).
Pastures and grazing management
Brachiaria decumbens cultivar Basilisk pastures were established 2-3 years before cow-calf grazing following the recommended application (kg/ha) of Ca (48), P (20), K (20) Mg (14), and S (10) (Ramírez-Restrepo et al., 2020). Every 3 years and for ~ 60 days, cattle were shifted to identical B. decumbens pastures that were used strategically for adult steers fattening to allow pasture renewal practices including two light disking plus the spread of a third of the establishment fertilization (Vera et al., 2002).
The field experiment used a stocking rate of 1.5 head/ha to ensure conservative grazing management that balanced forage growth and use and maintained full soil cover and land conditions to minimize erosion. Pasture performance was monitored six times per year following the BOTANAL procedure (Jones and Tothill, 1985). Forage mass was between 1,500 and 3,500 kg dry matter (DM)/ha with contents (g/100 g DM) of crude protein (CP, 7.1-11.0), neutral detergent fiber (65.0-71.0), ash (8.5-10.0), and fat (1.0) while in vitro DM digestibility (IVDMD; g/100 g) of the whole plant was 63.7 and 55.0, in the wet and dry season, respectively. (Values derived from a Carimagua grazing-forage database assembled by Ramírez-Restrepo and Vera, 2019).
Modeling approach
The basic structure of the modeling framework is one of beef production in which the benefits of plausible growth (Ramírez-Restrepo and Vera-Infanzón, 2019) and fattening (Ramírez-Restrepo et al., 2023) practices were estimated using least-squares regressions concerning LW-derived CH4 emissions (Eq. 1) and DM intake (DMI; Eq. 2) from 56 days of age. These functional relationships (y = a + bx) are derived from the Commonwealth Scientific and Industrial Research Organisation (CSIRO) calibrated (0.99 pure CH4 gas recovery) open-circuit chambers measurements using Brahman (Ramírez-Restrepo et al., 2016a) and Belmont Red Composite [Africander (African Sanga) x Brahman x Hereford-Shorthorn (3/4 B. taurus; Ramírez-Restrepo et al., 2014; Ramírez-Restrepo et al., 2016b) steers fed ad libitum on a daily DM basis (2.1% of total LW; Fisher et al., 1987).
r2 = 0.663, P < 0.0001; CV = 16.78; r.s.d = 30.82; r = 0.814, P < 0.0001
r2 = 0.491, P < 0.01; CV = 18.94; r.s.d = 1.34; r = 0.701, P < 0.01
The second strategy in the model was to link weaning LW differences (P < 0.0001) between heifers and steers with on-farm low (0.428 kg) and high (0.516 kg) post-weaning on-farm daily LW gains (DLWGs) in heifers and steers (0.516 kg) on B. decumbens pastures (Vera and Hoyos, 2019), respectively to reach target slaughter LW SCEs that are representative of those used in the industry (Wiedemann et al., 2015; Ramírez-Restrepo and Vera, 2019). Under this rationale, the model is implicitly ensuring that individual preweaning LW variations within each sex category are maintained up to slaughter at variable ages and grazing times to capture temporal and spatial beef-herd transactions, and existing regional land uses (Vera-Infanzón et al., 2023). The third strategy paired the slaughterhouse LWs with hot (0.4992) and chilled (0.4772) carcass dressing-outs (Velásquez and Ríos, 2010), accumulated CH4 emissions, and DMIs.
Functional scenarios and system boundaries
The post-weaning combination in heifers [confidence limits for weaning 154.4-170.6 kg] of non-feed supplemented low and high DLWGs allowed them to simultaneously reach an early breeding status (272.7 ± 0.01 kg) and the simulation of four target LW SCEs (i.e., 405, 414, 438, and 453 kg representing SCE 1, SCE 2, SCE 3, and SCE 4, respectively (Figure 1). In parallel, assuming a single DLWG, the backgrounding-fattening period of steers (weaning confidence limits 174.4-193.5 kg) to achieve butcher LWs of 435, 474, and 574 kg was represented by SCEs 5, 6, and 7, respectively (Figure 1). Hence, derived carcass carbon dioxide equivalent (CO2-eq) efficiency intensities constituted the specific system’s boundary cut-off criteria (Figure 1). An underlying assumption in using a post-weaning dataset partitioned into male and female sets is that LWGs of young steers are larger than in heifers grazing the same tropical pastures year-round (Ramírez-Restrepo and Vera, 2019). Thus, in the suckling herd, this study considered calves and heifers as experimental units while post-weaning sex-grouped animals represented the experimental units over the simulated fattening SCEs.
Animal GHG footprint, soil C stocks, and C footprint calculation
The scope of this computation considered the interconnected CF of GHG dynamics on B. decumbens portrayed by Ramírez-Restrepo et al. (2020); Ramírez-Restrepo et al. (2023), and to the extent possible, parameters used in this study have been reviewed. Briefly, fecal CH4 assumed the predicted DMI, IVDMD (55 g/100 g), and the 0.112 emission factor (EF; Zhu et al., 2018). Nitrogen excretion assumed DMI, N concentration (1.488 g/100 g), nutrient digestibility (71.62 g/100 g; Vercoe and Frisch, 1970), and the kg N2O-kg N EF of 0.0014 (Lessa et al., 2014) and 0.016 (Chirinda et al., 2019) for feces and urine, respectively. Intakes of DM and CP met the LW-related requirements of female and male B. indicus cattle raised on pasture in Brazil (Rotta et al., 2016). The factor used for a kg of the mineralized supplement was 0.800 kg CO2-eq (C-equivalent; KLIMATMÄRKING FÖR MAT, 2009) while supplement intake assumed the 0.124 g/kg LW factor derived from the expected consumption of 60 g/day by a 500 kg Brahman in the foothills region of the Colombian Llanos (Rincón et al., 2018).
The estimation of the overall C balance was mainly adapted from contemporary studies on soil-plant relations conducted at Carimagua (Fisher et al., 1994; Rondón et al., 2006; Trujillo et al., 2006). Soil organic C values to 1 m depth considered data from Fisher et al. (1994); Rondón et al. (2006), and Fisher et al. (2007). The standing above and belowground DM biomass values used were from published reports (Fisher et al., 1998; Rao, 1998; Kanno et al., 1999; Rao et al., 2001a, b; Grace et al., 2006; Trujillo et al., 2006). Soil CH4 and N2O emissions were estimated from the review studies done by Castaldi et al. (2006) and Rondón et al. (2006). The CO2-eq footprint from pasture establishment and maintenance was assumed on a prorated basis considering 15 years of on-farm productive grazing (Vera and Hoyos, 2019). Fertilizer and tillage imprints were computed from Edwards-Jones et al. (2009); Kim et al. (2011), and the University of Arkansas (2019) while the estimated range of CO2-eq SOC accumulation rate was based on published reports (Fisher et al., 1994; Rao, 1998; Fisher et al., 1998; Rondón et al., 2006; Fisher et al., 2007; Costa et al., 2022; Hyman et al., 2022).
The study used the horizon global warming potential (GWP)100-34 value for CH4 that includes climate-C feedbacks (Gasser et al., 2017; Mueller and Mueller, 2017; Allen et al., 2018) whilst the GWP100-298 value for N2O (Ramírez-Restrepo et al., 2019b). This is consistent with previous studies on GHGE conducted by Vera et al. (2023) that were aimed to assist possible BFS intensification on the WNSE, as well as discussions, and policy-making efforts focusing on environmental trade-offs, planning, natural resources management, rural livelihoods, market chains, and research for development (Latawiec et al., 2014; Cunha Malafaia et al., 2021).
Statistical analyses
The variances associated with age and LW fluctuations, CH4 emissions, dynamics of the relationships between LWs and CH4 emissions, DMI, and carcass measurements were tested using the GLM procedure within the Statistical Analysis System (SAS®, 2021) including the upper and lower confidence limits for each mean observation and the confidence level for the parameter estimation methods. The model considered the fixed effects of breeding-herd RCs (i.e., 1 to 7) and fattening-slaughter SCEs (i.e., 1 to 7), and their interaction. Results are presented as least-squares means ± standard errors of the mean (SEM) while differences between the main factors were reported if significant at P ≤ 0.05.
Results
Heifers’ differences among SCEs in low and high DLWGs from the early breeding status to slaughter were significant (Table 1). The slaughter age increased from SCE 1 to SCE 4 by 12% and 11% for the low and high DLWGs, respectively, while in parallel, a 5% and 4% increase in DMI between the first and fourth fattening SCEs was compensated by the improvements in LWGs. The estimates of CH4 emissions per day, DMI, and during the fattening phase were consistently higher for both DLWGs in SCE 4 than across other SCEs. As expected, CH4 emissions during the fattening period increased up to 45% with increasing slaughter LW.
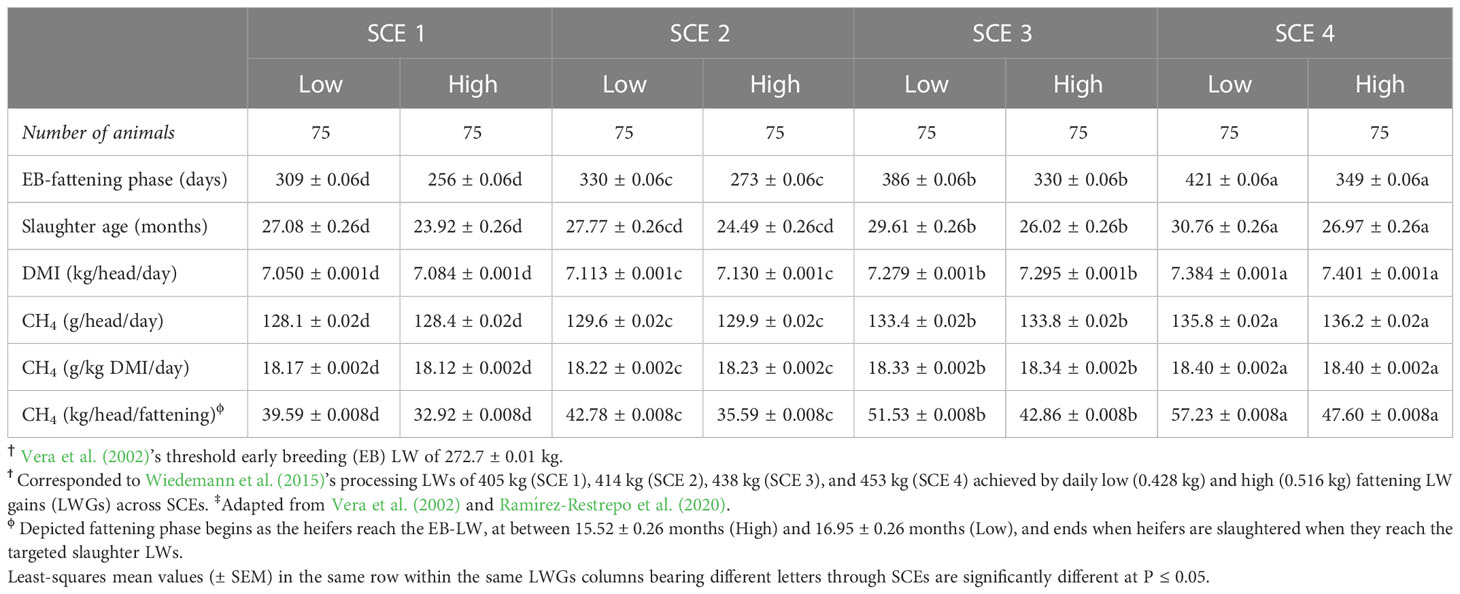
Table 1 Estimated fattening performance, dry matter intake (DMI), and methane (CH4) emissions from †early-breeding (EB) Brahman (Bos indicus) heifers gaining daily either low or high liveweight (LW) on Brachiaria decumbens pastures‡ in relation to slaughterhouse LW scenarios (SCEs)ϯ.
The effect of SCEs on CO2-eq CH4 emission profiles during the fattening period and lifespan of heifers are presented in Table 2. Compared to SCE 4, fattening emissions per kg LWG and FLW were significantly lower in the low and high DLWGSs of SCE 1 by 6.1% and 29.1%, respectively. However, lifespan emissions in SCE 4 were significantly higher than in SCE 1 for the low and high DLWGs in terms of the LWG (10.9% vs 10.4%), slaughter LW (11.8% vs 11.3%), and birth LW (25.0% vs 24.4%) efficiency indicators.
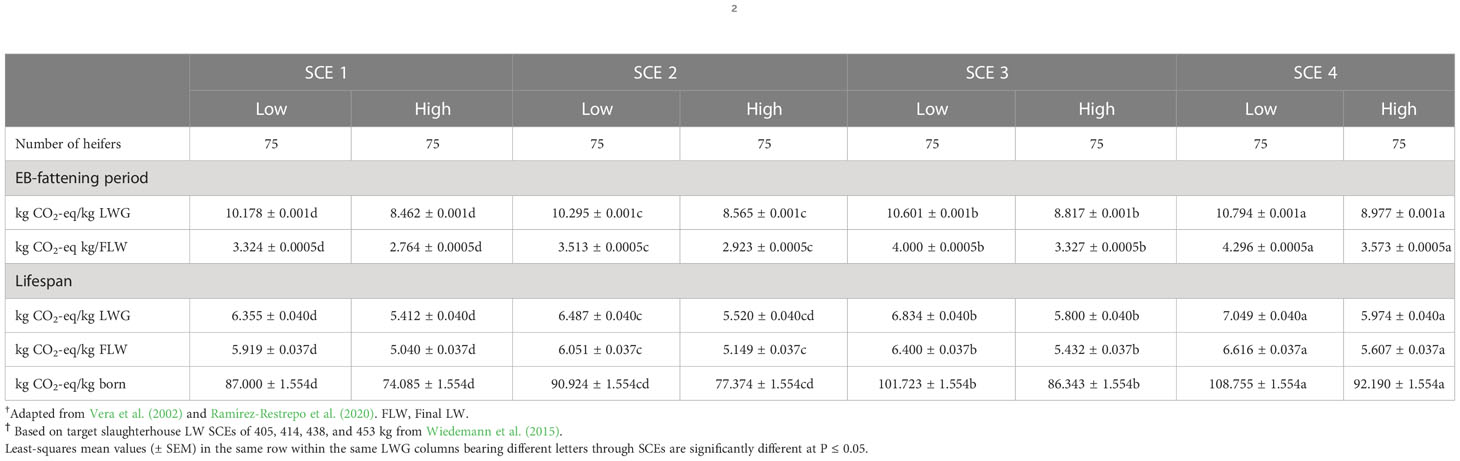
Table 2 Comparison of fattening and lifespan periods in terms of farm-gate carbon dioxide equivalent (CO2-eq) methane emission metrics of surplus early-breeding (EB) Brahman (Bos indicus) heifers born and raised on Brachiaria decumbens pastures† making either low (0.428 kg) or high (0.516 kg) daily gains to achieve commercial slaughterhouse LW scenarios (SCEs)ϯ.
Final age, total LWG, daily DMI, CH4 emissions, and CH4 yield (g/kg/DMI) of steers in the backgrounding phase were significantly lower than for steers in SCE 5 (10%, 16%, 5%, 6%, 1%, and 20%), SCE 6 (20%, 31%, 9%, 12%, 2%, and 41%), and SCE 7 (46%, 70%, 19%, 25%, 5%, 104%; Table 3). Fattening and lifespan CO2-eq CH4 emission metrics from steers are shown in Table 4. Differences in LWG estimations between SCEs were larger during the animals’ lifespan than during the fattening period while the final LW indices were larger over the fattening period than during the lifetime frame. In heifers and steers, CO2-eq CH4 EFs related to carcass characteristics increased linearly and significantly across their respective DLWGs and SCEs (Table 5).
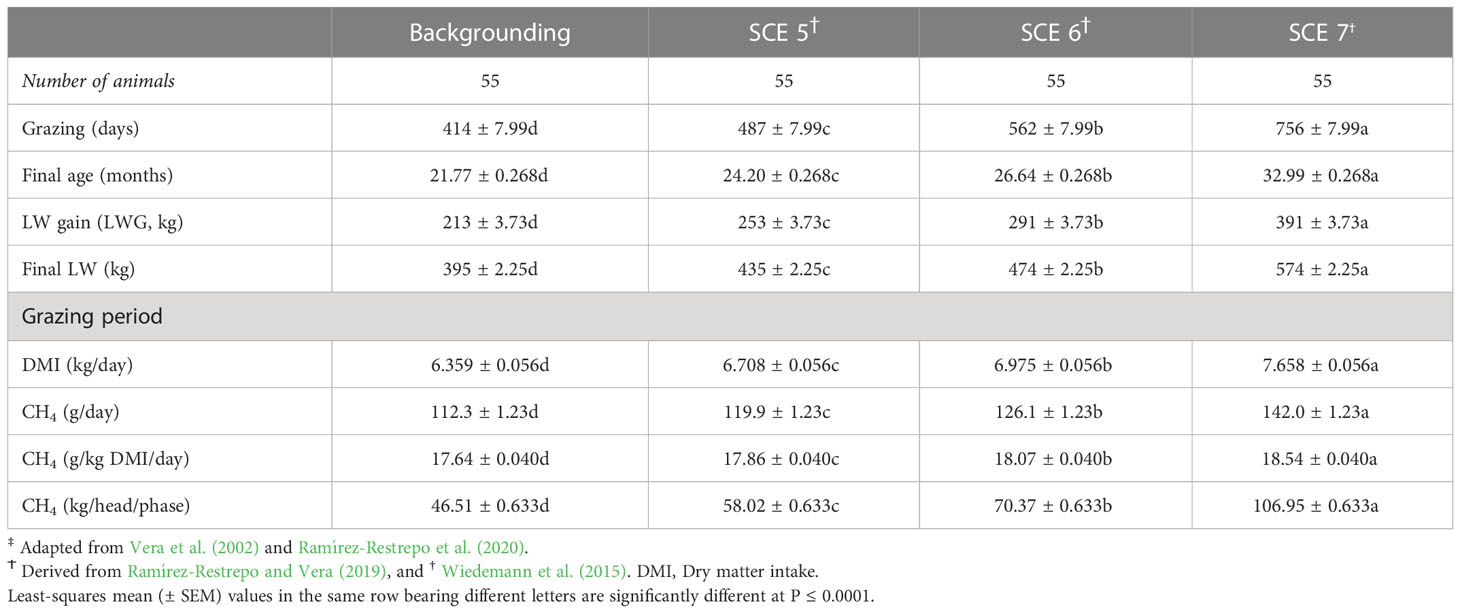
Table 3 Estimated animal performance and methane (CH4) emissions of growing commercial Brahman (Bos indicus) stockers-yearlings‡, and young steers on Brachiaria decumbens pastures associated with a common daily liveweight gain (LWG) of 0.516 kg and three processing target LW scenarios (SCEs).
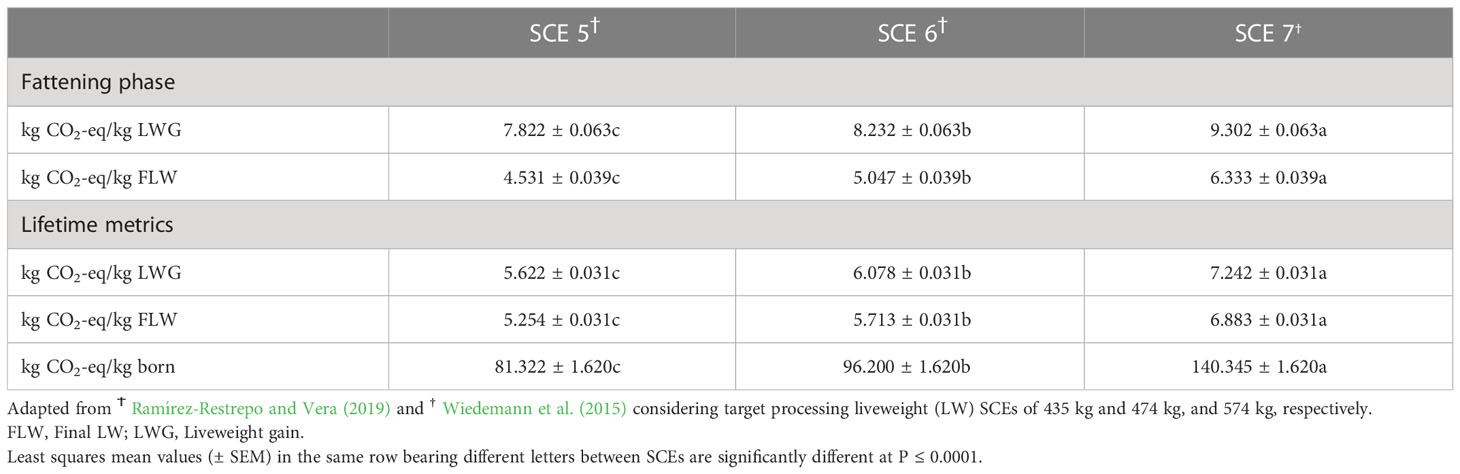
Table 4 Estimated carbon dioxide equivalent (CO2-eq) methane emission impact of commercial Brahman (Bos indicus) steers’ grazing on Brachiaria decumbens pastures before slaughter with three contrasting scenarios (SCEs).
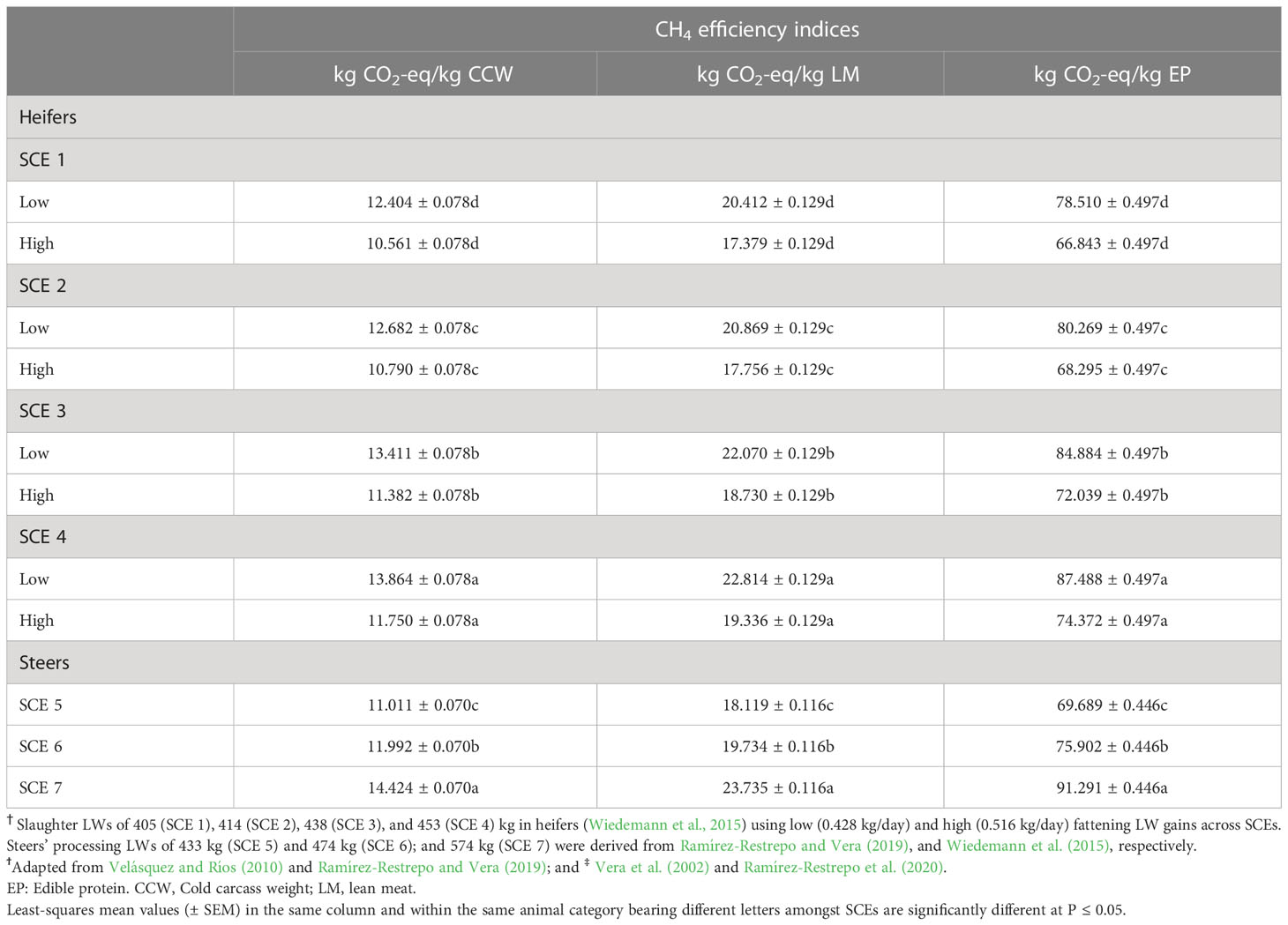
Table 5 Lifespan carbon dioxide equivalent (CO2-eq) methane (CH4) efficiency indices from carcass composition† of young Brahman (Bos indicus) heifers and steers grazing on Brachiaria decumbens pastures‡ with contrasting liveweight (LW) slaughter scenarios (SCEs)ϯ.
Table 6 shows estimated GHGE, observed ranges for important variables, and estimated overall C balance for SCEs 1 and 4 and SCEs 5 and 7. Relative to high DLWGs, the estimated enteric CO2-eq CH4 emissions in heifers were lower in the low DLWG group of SCEs 1 and 4 by 6.6% and 6.8%, respectively, while steers’ emissions were 18.9% higher in SCE 7 than in SCE 5. The estimated CO2-eq CH4 emission from heifers’ feces was 5.4% higher in the low DLWG SCE 4 than in the counterpart SCE 1 while in steers, 268 extra days of grazing between SCE 5 and SCE 7 were associated with 14.1% increase in emissions. In heifers, differences in CO2-eq N2O emission from feces, urine, and mineral supplement intake were small between low and high DLWGs and between SCEs, but emissions from steers were 14.1% lower in SCE 5 than in SCE 7. From comparable days of grazing, total animal CO2-eq GHGE (kg/ha/year) were 7.1% lower in the low DLWG SCE 1 than in the high DLWG SCE 4 whilst averaged GWP GHGE in heifers and steers amounted to 2,531 and 2,877 CO2-eq GHGE (kg/ha/year) from 568 and 622 grazing days, respectively.
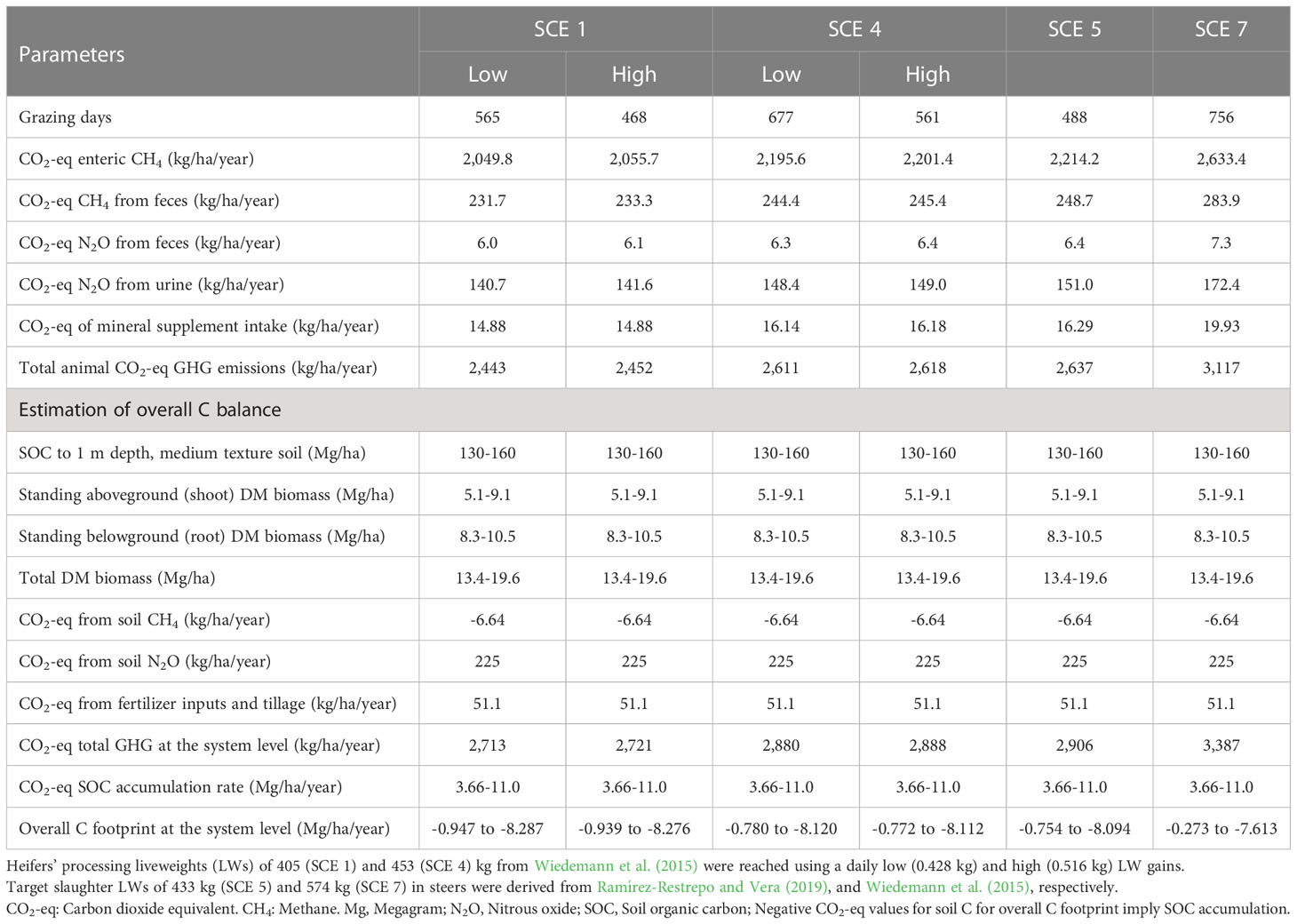
Table 6 Estimated greenhouse gas (GHG) emissions and overall carbon balance in young Brahman (Bos indicus) heifers and steers grazing on Brachiaria decumbens pastures across weaning-fattening systems characterised by contrasting liveweight (LW) processing scenarios (SCEs).
Stocks of SOC to 1 m soil depth for the clay loam soil texture and well-drained Oxisols of SCEs 1, 4, 5, and 7 were estimated to be in the range of 130 to 160 t/ha while the range of standing shoot and root DM biomass values represented the 38% and 46% and the 62% and 54% of the total range of DM biomass, respectively. In parallel, using the CO2-eq CH4 (-6.6; 3.2 x 34 kg/ha/year) and CO2-eq N2O (225; 1.05 x 298 kg/ha/year) emissions (Ramírez-Restrepo et al., 2020) and compared to the total GWP of GHG at the system level in SC1 low DLWG, there was an increase of 6.1% in the counterpart treatment in SCE 4, which in turn, was 17.6% lower than the value from most productive steer SCE. Across groups, heifers and steers subject to the same DLWG (i.e., 0.516 kg) in SCEs 4 and 7 showed the lowest overall level of SOC capture.
Discussion
This study complements our previous efforts that dealt with the estimation of the carbon footprint (CF) of various beef farming systems in the Colombian Orinoquía Llanos. Earlier studies were focused on (i) breeding herds maintained on diverse native and sown tropical grasslands (Ramírez-Restrepo et al., 2019b; Ramírez-Restrepo et al., 2020); (ii) fattening of cull cows from the breeding herd (Ramírez-Restrepo et al., 2023); and (iii) finishing cull cows on contrasting sown pastures, and raising heifers intended as replacement breeders also on sown vs native grasslands (Vera et al., 2023). With this study’s results on CF estimates for fattening young females and males, we complete the production life cycle of cattle raised on grazed pastures.
This research has considered the possible greenhouse gas emission (GHGE) and the CF implications of young fattening BFS, and it particularly focused on the link between individual growth records, B. decumbens pastures, grazing management, and the environmental efficiency of carcass characteristics. In this study, we repurpose a unique long-term Brahman breeding herd data set recorded on the field from 1979 when the breeding herd was born together with enteric CH4 calculations that included the findings from pooled B. indicus and B. indicus x B. taurus breeds open-circuit chamber measurements. By doing this in the absence of recently collected, rigorous field data from the region, we were able to map and share knowledge about common as well as commercial cattle farming systems that are recognized worldwide that may be subjected to human intervention with the use of new technologies and innovation to ensure a significant positive impact on the industry, climate, food systems, and society.
This study’s outcome is considered to be a piece of baseline information that is not readily available in the Colombian cattle industry. The presented model predicted that heifers and steers may be successfully fattened without supplementation assuming that animals had access to a nutritive forage grass as a sole feed after extended periods of conventional farm management. Production issues are known to influence farmers’ acceptance of Brachiaria spp. pastures and these include soil constraints as one of the major factors influencing the decline in tropical forage species’ vigor, density, and productivity over time (Vera and Hoyos, 2019).
Cattle performance improved on grazing lands
The predicted chilled carcass weights (CCWs) in the range of 193-210 kg for heifers and 208-274 kg for steers at 24-31 and 24-33 months of age, respectively, can be compared with the results of León-Llanos et al. (2022) who reported in the Llanos a range of 259-269 kg CCWs at 34-35 months from seven commercial Brahman crosses fattened rotationally for 24 months on B. decumbens and B. humidicola pastures. Although León-Llanos et al. (2022) and the present study used animals from experimental cow-calf systems, differences between the present calculations and León-Llanos et al. (2022) can be explained by the higher initial age (10-11 months), initial LW (246 kg), and stocked paddocks (2.8 head/ha) used by the latter authors which in turn reflected in lower DLWGs (0.442 kg). It is also important to note that León-Llanos et al. (2022) did not assess heifers and used a crop-based supplement during the dry season period.
Rincón and Villalobos (2021) raised Brahman yearlings (18 months of age - 240-280 kg) on rotationally grazed B. decumbens pastures or mixed with the tannin-containing legumes [Lascano et al., 1990; International Center for Tropical Agriculture (CIAT), 2016] including Centrosema molle (accession CIAT 15160), C. macrocarpum (accession CIAT 15799), and Grona heterocarpa subsp. ovalifolia (syn. Desmodium ovalifolium cultivar Maquenque) that yielded average daily liveweight gains (DLWGs) of 0.437, 0.687, 0.396, and 0.440 kg, respectively in Experiment 1 (22 months). A second, shorter experiment (14 months) on the same pastures, resulted in mean DLWGs of 0.545, 0.744, 0.563, and 0.573 kg, respectively. Thus, the final LWs (495-504 kg) recorded by Rincón and Villalobos (2021) fall within the present scenario (SCE) 6 and SCE 7 estimations and these values reflect the model’s mean steers’ target processing LW (494 kg). Similarly, the mean DLWG (0.487 kg), across heifers and steers set by the model, and the overall DLWG (0.486 kg) on B. decumbens after 36 months of grazing (Rincón and Villalobos, 2021) emphasize the predictive capability of the model considering the continuous growth rate from weaning to slaughter approach. However, carcass traits and CO2-eq CH4 concentration in carcass yields were not estimated by Rincón and Villalobos (2021).
The methodology employed in this research provided strong evidence to support the view that LWGs, grazing management, and processing LW frameworks exert an impact on intensification in the beef farming systems (BFS) of the well-drained native savanna ecosystem (WNSE). Slaughtering cattle at a younger age will play a significant role in commodity markets for homogenous products within gender groups or in supply chains where there is much likelihood of heterogeneous quality products from adult cattle. Nevertheless, emphasis on plant characteristics that relate to nutritional and feeding values, and persistence to address increasing climate extremes, shifts, and changes from seasons to years should provide long-term benefits and future opportunities for research and development of the industry. This may require the integration of fodder resources such as trees and shrubs to supply protein, energy, and other nutrients into tropical grazing systems (Vandermeulen et al., 2018a).
Previous studies from WNSE (Ramírez-Restrepo and Vera, 2019) reported that Brahman-influenced cull cows (10-13 years) and steers (3-5 years) can achieve CCWs of 169-193 kg and 202-226 kg, respectively when grazed on Andropogon gayanus plus Stylosanthes capitata pastures after 144-201 days of fattening, while CCWs of 176-194 kg (cull cows) and 187-211 kg (steers) were observed on B. humidicola pastures during 146-181 days. Therefore, as the choice of cattle categories for fattening on improved pastures and/or fodder resources is an important consideration for farmers, further appropriate modeling studies are required to determine the benefits of complementary fattening of young and adult cattle practices to diversify farm outputs while meeting consumers’ expectations and delivering increased economic returns to producers.
Estimated production patterns, methane emissions, and carcass metrics
The model interpolation of LWs and CH4 emissions between weaning, slaughter, and lifespan proportions allowed the differentiation in CF of young BFS. The analysis indicated that weaning LW to slaughter LW ratios of 0.3932, 0.3848, 0.3634, and 0.3517 ± 0.0076 were achieved in heifers’ SCEs 1, 2, 3, and 4, respectively whilst ratios of 0.4194, 0.3850, and 0.3179 ± 0.0089 corresponded to steers’ SCEs 5, 6, and 7, respectively. Likewise, it was shown that significant differences in the overall growth from birth to weaning between heifers (0.533 ± 0.004 kg) and steers (0.620 ± 0.007 kg), their birth LW to weaning LW ratios (0.1799 ± 0.001 vs 0.1612 ± 0.002), and their cumulative CH4 emissions before fattening (7.73 ± 0.300 kg vs 9.20 ± 0.351 kg) can be linked to the differential significant magnitudes of weaning to slaughter and weaning to lifespan CH4 ratios in heifers (0.1301 ± 0.001 vs 0.1443 ± 0.003) and steers (0.1137 ± 0.001 vs 0.1241 ± 0.002). In particular, the pattern of LWGs during the rearing period in a pasture-based system corresponds to the value of LW at 12 months as a proportion of 21 months of age on cumulative production (Handcock et al., 2020) while LWs and DLWGs in real-time can be predicted in B. indicus cattle during growing and finishing phases (Comminotte et al., 2020).
Taken together, these results indicate that the within and between individual animal variability and herd-level variation in the LW recorded database and the predicted DMI and CH4 emissions were also coupled with the natural variation in measured LWs, DMIs, and CH4 outputs from cattle while in accurate respiratory chambers (Ramírez-Restrepo and Vera, 2019; Zhang et al., 2021). It is important to observe that we hypothesized that suckling growth paths would affect lifetime production and the sustainable efficiency of carcass yields. The mathematical output supports this hypothesis, as we provided insights into the implications of measurements obtained at differential LW and age at slaughter that can be caused by the same variation from birth to weaning on the same pasture with a pivotal effect on the overall CF at the system level. Connectedly, Foote et al. (2016) indicated that in ad libitum-fed beef-finishing heifers and steers, their differential appetite, DLWGs, and feed efficiency are consistently regulated by the circadian-blood expression of levels of acyl ghrelin (gut-peptide hormone), total ghrelin (acyl and desacyl), and ghrelin ratio (acyl: total ghrelin). Hence the current model can be used as part of a whole-farm model to predict the effects of pasture intensification strategies on production dynamics and the environmental footprint of beef herds in the Llanos (Vera et al., 2023).
This study also contributes to current knowledge by documenting the range of values on lifetime CH4 emissions from finished heifers (60.03 to 88.15 ± 0.470 kg) and steers (67.30 to 116.23 ± 0.593 kg) and the subsequent carcass metric implications. This is shown from the calculated CH4 yield ranges of 18.1-18.4 (heifers) and 17.9-18.5 (steers) that match the values from open-circuit respiration chambers values with B. indicus influenced heifers (18.07) and steers (18.4-19.0) that were with fed fresh grasses as reported by Ku-Vera et al. (2018) and Meat and Livestock Australia (MLA, 2020), respectively. Although in agreement with Skidmore et al. (2022), the present modeling effort followed a single-issue life cycle assessment approach across a wide range of slaughter regimes, the current predictive values for steers (24.2-32.9 months) are well below the estimated steers’ lifetime CH4 emissions of 151.23 kg (36 months) and 201.83 kg (48 months) from Brazilian pasture-based BFS. Interestingly, while using the Intergovernmental Panel on Climate Change (IPCC) Tier 2 methodology Skidmore et al. (2022) argued that it is practicable to slaughter lifetime grazing heifers with 168.12 kg of CH4 emissions at 36 months of age while the present modeling showed that un-supplemented younger heifers (23.9-30.7 months) can be slaughtered at a reduced time to lower enteric CH4 emissions by ~44%.
The results from this study point out that at different levels of intensification, the lifespan C footprint at the farm gate is in the range of 5.040 to 6.616 kg CO2-eq/kg FLW in heifers and 5.254 to 6.883 kg CO2-eq/kg FLW in steers. On average, these values are 43.4%, 45.4%, 51.0%, 55%, and 77% lower than the Australian methodologically-variable cradle to farm-gate life cycle assessment estimates of 10.5 CO2-eq/kg beef LW (Eady et al., 2013; 2016), 10.9 CO2-eq/kg beef LW (Ridoutt, 2021), 12.2 CO2-eq/kg beef LW (Wiedemann et al., 2016), 13.3 kg CO2-eq/kg beef LW (MLA, 2022), and 25.8 CO2-eq/kg beef LW sold (Eady et al., 2016). Furthermore, present lifetime means of 6.179 kg CO2-eq/kg LWG for heifers and 6.314 kg CO2-eq/kg LWG for steers are 42% and 80% lower than the CO2-eq/kg LWG fattening range indices of 10.9 and 30.9 suggested by the cradle to farm-gate life cycle assessment estimations for the Colombian piedmont plains using 2019 refined IPCC parameters (González-Quintero et al., 2022). Alternatively, if a CH4 GWP100-28 metric (Myhre et al., 2013) is introduced to compare with the assumed CH4 EF of 1.5 t CO2-eq/animal equivalent (AE; 455 kg LW)/year which is used to estimate the C balance of the Queensland beef industry (QLD Government, 2019) or the 1.56 t CO2-eq/AE/year for farm C accounting (Ekonomou et al., 2020), constant heifers’ LWs of 438 and 453 kg, emit 1.365 vs 1.390 t CO2-eq/year while 1.225, 1.289, and 1.451 t CO2-eq/year emissions, respectively equate to stable steers’ LWs of 435, 474, and 574 kg. These disparities provide the foundation to simulate the environmental impacts of alternative finishing LWs by choosing the assessment methodology and production option that makes sense in the context of sustainable productivity at the BFS level for assisting a low-C emission future. It is also relevant to recognize that 33% and 47% of the variation in predicted CO2-eq emissions per kg LW in BFS is explained by weaning percentage and averaged DLWGs, respectively (Wiedemann et al., 2016).
On the other hand, the model predicted a range of values of 10.5-13.8, 17.3-22.8, and 66.8-87.4 kg CO2-eq/kg of CW, lean meat, and edible protein for heifers and 11.0-14.4, 18.1-23.7, and 69.9-91.2 for steers, respectively for steers. Overall, they represent 19.9% for heifers and 20.7% for steers from the total (cumulative) carcass-reference values of CH4 emission that would be expected from multiparous Brahman cull cows (8.7 years) that were continuously bred and fattened for 86 days on B. decumbens pasture (Ramírez-Restrepo et al., 2023). These estimated values are in contrast with Ridoutt’s (2021) estimate of 25.5 kg CO2-eq/kg of edible beef. Nevertheless, such estimations underscore the value of 16.7 CO2-warming-eq (CO2-w.e.)/kg of edible beef (Ridoutt, 2021) which aligns the future warming with the permanent change in the rate of short-lived GHGE like CH4 (Lynch et al., 2020). Hence, results from the model used in this study may support published values by the potential use of the new GWP* CO2-w.e. metrics to further review the CF of the beef industry of the WNSE.
Estimated overall C footprint at the system level and implications for sustainable regional beef farming systems
The present study estimated the enhancement of soil organic carbon (SOC) storage at different stages of the BF system. First, the long periods of grazing by heifers (677 days) and steers (756 days) strike a positive balance between on-farm productivity, CO2-eq SOC accumulation, and climate change mitigation. The notion that conservatively managed grazing of tropical BFS negatively imprints the environment is not tenable. Indeed, positive trade-offs are involved, and farmers may need to make sensible resource management decisions regarding the appropriate allocation of pastures and animal stocks to ensure red meat target-setting and choose the relative contribution of pastoral abatement strategies.
This is consistent with the view that in the Colombian Orinoquía, increased SOC storage at deep soil layers (30-100 cm), reduced N2O emissions from beef urine deposition, and viable land-based agendas of CO2 removal from the atmosphere can be achieved through the ability of biological nitrification inhibition, N uptake from the ammonium source, and the deep rooting of B. humidicola (Costa et al., 2022). Hyman et al. (2022) showed a large potential for SOC storage in Colombia’s Eastern High Plains. They found less SOC storage than Fisher et al. (1994) and indicated that earlier high levels of SOC accumulation in well-managed pastures returned to a lower equilibrium value under inadequate management conditions. Thus, these two recent reports on SOC stocks in the Llanos not only highlight the need for improving the management of native savanna and degraded pastures across the 1 million ha study but also infer that land-use changes in the WNSE can vary widely in the balance between beef outputs and the overall CF at the farming system level (Vera-Infanzón et al., 2023).
Furthermore, results from this study suggest that early weaning-fattening systems in the tropics may also offer the opportunity for climate mitigation by transferring C credits within or outside the BFS involving land transactions and promoting C markets. Brahman-influenced cow-calf herd systems in the WNSE may be not only close to C neutral under estimated conventional and early weaning management practices (583 vs 579 kg CO2-eq/ha/year; Ramírez-Restrepo et al., 2019b) but also may act as C sinks at the system level by removing atmospheric CO2 across six consecutive RCs by grazing on B. decumbens pastures during the cow-calf-bull breeding phase (-1,180 to -8,513 kg CO2-eq/ha/year; Ramírez-Restrepo et al., 2020). These C sinks may also contribute toward SOC storage imbalances caused by land use change, cropland use, or complementary routine animal feed supplementation locally or elsewhere. Therefore, climate stabilization may be nurtured from land use efficiency through pasture management and the adoption of a climate-neutral target strategy with improved production from the meat industry (MLA, 2021).
The current predictions from this study provide dimensional insights to reduce slaughter age while monitoring GHGE at the system level. However, this approach did not assess the effect of B. decumbens pastures mixed with legumes that contain secondary metabolites that may influence even more on sustainable ruminant intensification (Ramírez-Restrepo and Barry, 2005). Therefore, it is suggested that year-round productivity should not underestimate the feeding value of forage resources containing secondary compounds (Ramírez-Restrepo and Charmley, 2015; Durmic et al., 2017; Vandermeulen et al., 2018b) to further reduce the impact on the C cycle by using the present fattening framework. This argument is strengthened by the counter-balancing effect of LWs and age targets on animal GHGE vs the reduction in GHGE from shorter fattening periods, especially considering not only that CP concentration in B. decumbens plus legumes swards can be 33% higher than in B. decumbens alone pastures (Rincón and Villalobos, 2021) but also the effect of condensed tannins-containing legumes shifting N digestion and increasing fecal N concentration (Waghorn et al., 1987), thereby mitigating CH4, CO2, and N2O emission from excreta in the soil (van Cleef et al., 2022), and increasing soil N retention in grazed pastures (Slebodnik, 2020).
The present estimates also suggest that replacement heifers raised on B. decumbens pastures may favor lifetime breeding systems grazing higher-quality pastures or if transferred, could positively impact extensive cow-calf operations that are subject to improved decision-making (Vera-Infanzón and Ramírez-Restrepo, 2020; Vera-Infanzón and Ramírez-Restrepo, 2022). Nevertheless, defining the physiological and genomic limits on selection pressure on beef genotypes that may have a significant environmental effect remains to be an intriguing issue if grazing low CH4 emitters and efficient producers are targeted. Interestingly, our understanding is limited in many basic details related to the physiological systemic effects of blood CH4 concentration in Brahman cattle (Ramírez-Restrepo et al., 2016a).
Likewise, the current early breeding and backgrounding stages may offer a potential window of opportunity for the transition to other BFS practices that may reduce the GWP of the national GHG inventory. This can be achieved through the finishing of beef cattle on feedlots that are potentially driven by the feed supplementation of ecologically relevant compounds (Tedeschi et al., 2021). Nevertheless, as feed-intensive practices are inevitably linked to the provision of high-concentrated diets, plausible BFS should consider that a kg of feed-milled supplement at the farm gate may represent 0.93 kg CO2-eq under a life cycle assessment methodology (Adom et al., 2013). Relatedly, the evaluation of trade-offs, synergies, and challenges between land use, breeding goals, plant by-products, profitability, environmental stability, and consumers’ recognition of WNSE-BFS in a circular planet-friendly food system should be similarly addressed (Oosting et al., 2022). Hence, as the transformation of finishing BFS on the WNSE is not well documented, future advances will depend on research focused on the integration of beef genotypes, grazing systems with an emphasis on deep-rooted pasture grass and legume species, and several fattening-supplemented practices under grazing or feed yard conditions to assist decision-making and to address environmental policy issues.
Conclusions
The present modeling, complemented with earlier studies, provides benchmark results that were obtained under good, but not exceptional, pasture and animal management conditions against which less detailed datasets from on-farm records may be evaluated in future research. As expected, heavier slaughter LWs increased enteric CO2-eq CH4 emissions, but these increases in heifers were smaller with higher LWGs during the fattening phase and under the lifetime perspective. Based on the same LWG across the steer’s fattening phase, a 32% rise in the slaughter LWs led to an 84% increase in enteric emissions while lifespan emissions when expressed per kg of final LW or per kg born increased by 31% and 72.6%, respectively. Regardless of slaughter LW, larger LWGs during the fattening phase resulted in an average carcass CH4 efficiency index for heifers and steers of 70.4 and 79.0 kg CO2-eq/kg edible protein, respectively. Lastly, assuming conservative and practicable management of the B. decumbens grass pasture examined, all the finishing systems simulated showed potential C neutrality and net-zero C emission profiles when considering total GHGE from animals, pasture, and soil vs the estimated soil C capture over seven seasons at the system level. Thus, these beef grazing systems have the potential to accomplish substantial SOC accumulation through effective land-based removal of atmospheric CO2 while ensuring sustainable nutritional security and mitigating climate change. Sustainable beef farming systems could ensure socioeconomic-climate justice in the Orinoco River Basin of Colombia.
Data availability statement
The datasets presented in this article are not readily available because model development and generated datasets in this study are legally protected by the intellectual property rights of CR Eco-efficient Agriculture Consultancy (CREAC®) and R. R. Vera Infanzón Private Consultant Services. Requests to access the datasets should be directed to CR-R, c.ramirez@creac.com.au.
Ethics statement
Ethical review and approval was not required for the animal study because the source of animal data is based on the preservation, reuse, and repurposing of heritage files generated ethically in the Neotropical savanna environment of Colombia.
Author contributions
CR-R: Oversaw data collection from the animal study, Investigation, Data curation, Conceptualization, Methodology, Writing, Review and Editing. RV-I: Oversaw data collection from the animal study, Investigation, Data curation, Conceptualization, Methodology, Writing, Review and Editing. IR: Methodology, Writing, Review and Editing. All authors contributed to the article and approved the submitted version.
Funding
A core budget from the International Center for Tropical Agriculture (CIAT) financially supported the original pastoral research conducted between 1979 and 1991 at Carimagua Research Station. This manuscript and model development were funded from 2020 to 2022 by CR Eco-efficient Agriculture Consultancy (CREAC®) and R. R. Vera Infanzón Private Consultant Services.
Acknowledgments
The authors thank the many CRC and CIAT technical staff for their contributions to the initial field research. Special thanks are equally extended to the Commonwealth Scientific and Industrial Research Organisation (CSIRO) for allowing the first author to review, collate, repurpose, and analyze long-term beef herds legacy data between 2016 and 2017.
Conflict of interest
Author CR-R is the Founder & CEO of CR Eco-efficient Agriculture Consultancy CREAC®. Author RV- is the Founder & Director of R. R. Vera Infanzón Private Consultant Services.
The remaining authors declare that the research was conducted in the absence of any commercial or financial relationships that could be constructed as a potential conflict of interest.
CREAC® and R. R. Vera Infanzón Private Consultant Services were involved in the study design, the screening, analysis, and interpretation of data, article writing, and the decision to submit it for publication.
Publisher’s note
All claims expressed in this article are solely those of the authors and do not necessarily represent those of their affiliated organizations, or those of the publisher, the editors and the reviewers. Any product that may be evaluated in this article, or claim that may be made by its manufacturer, is not guaranteed or endorsed by the publisher.
References
Adom F., Worman C., Thoma G., Shonnard D. (2013). Carbon footprint analysis of dairy feed from a mill in Michigan, USA. Int. Dairy J. 31, S1–S28. doi: 10.1016/j.idairyj.2012.09.008
Allen M. R., Shile K. P., Fuglestvedt J. S., Millar R. J., Cain M., Frame D. J., et al. (2018). A solution to the misrepresentation of CO2-equivalent emissions of short-lived climate pollutants under ambitious mitigation. Clim. Atmos. Sci. 16, 1–8. doi: 10.1038/s41612-018-0026-8
Ayarza M., Rao I., Vilela L., Lascano C., Vera-Infanzón R. (2022). Chapter Three - Soil carbon accumulation in crop-livestock systems in acid soil savannas of South America: A review. Adv. Agron. 173, 163–226. doi: 10.1016/bs.agron.2022.02.003
Bowman M. S., Soares-Filho B. S., Merry F. D., Nepstad D. C., Rodrigues H., Almeida O. T. (2012). Persistence of cattle ranching in the Brazilian Amazon: A spatial analysis of the rationale for beef production. Land Use Policy. 29 (3), 558–568. doi: 10.1016/j.landusepol.2011.09.009
Broom D. M. (2021). A method for assessing sustainability, with beef production as an example. Biol. Rev. 96, 1836–1856. doi: 10.1111/brv.12726
Castaldi S., Ermice A., Strumia S. (2006). Fluxes of N2O and CH4 from soils of savannas and seasonally-dry ecosystems. J. Biogeogr. 33, 401–415. doi: 10.1111/j.1365-2699.2005.01447.x
Chirinda N., Loaiza S., Arenas L., Ruiz V., Faverín C., Alvarez C., et al. (2019). Adequate vegetative cover decreases nitrous oxide emissions from cattle urine deposited in grazed pastures under seasonal conditions. Sci. Rep. 9, 908. doi: 10.1038/s41598-018-37453-2
Chriki S., Hocquette J.-C. (2020). The myth of cultured meat: A review. Front. Nutr. 7 (7). doi: 10.3389/fnut.2020.00007
CIAT (2016). Genotype x environment interaction in Desmodium ovalifolium. (Cali: International Center for Tropical Agriculture). doi: 10.7910/DVN/COQEK8
Comminotte A., Fernandes A. F. A., Dorea J. R. R., Rosa G. J. M., Ladeira M. M., van Cleef E. H. C. B., et al. (2020). Automated computer vision system to predict body weight and average daily gain in beef cattle during growing and finishing phases. Livest. Sci. 232, 103904. doi: 10.1016/j.livsci.2019.103904
Costa C. Jr., Villegas D. M., Bastidas M., Rubio N. M., Rao I., Arango J. (2022). Soil carbon stocks and nitrous oxide emissions of pasture systems in orinoquía region of Colombia: Potential for developing land-based greenhouse gas removal projects. Front. Clim. 4. doi: 10.3389/fclim.2022.916068
Cumbe T. A., Sessim A. G., López-González F. A., Zago D., Mendes Paizano Alforma A., Jardim Barcellos J. O. (2021). Bioeconomic evaluation of feedings strategies in the yearling beef cattle system in Mozambique. Lives. Sci. 247, 104466. doi: 10.1016/j.livsci.2021.104466
Cunha Malafaia G., de Vargas Mores G., Gomes Casagranda Y., Jardim Barcellos J. O., Paim Costa F. (2021). The Brazilian beef cattle supply chain in the next decades. Lives. Sci. 253, 104704. doi: 10.1016/j.livsci.2021.104704
Decaëns T., Jiménez J. J., Rangel A. F., Cepeda A., Moreno A. G., Lavelle P. (2001). “La macrofauna del suelo en la sabana bien drenada de los llanos orientales,” in Agroecología y biodiversidad de las sabanas en los llanos orientales de Colombia. Eds. Rippstein G., Escobar G., Motta F. (Cali: CIAT – CIRAD), 111–137.
De Rosa M., Di Pasquale J., Adinolfi F. (2021). The root towards more circularized animal production systems: From animal to territorial metabolism. Animals. 11 (6), 1540. doi: 10.3390/ani11061540
Durmic Z., Ramírez-Restrepo C. A., Gardiner C., O’Neill C. J., Hussein E., Vercoe P. E. (2017). Differences in the nutrient concentrations, in vitro methanogenic potential and other fermentative traits of tropical grasses and legumes for beef production systems in northern Australia. J. Sci. Food Agric. 97 (12), 4075–4086. doi: 10.1002/jsfa.8274
Eady S. J., Havard G., Bray S. G., Holmes W., Navarro J. (2016). Down scaling to regional assessment of greenhouse gas emissions to enable consistency in accounting for emissions reduction projects and national inventory accounts for northern beef production in Australia. Rangel. J. 38, 219–228. doi: 10.1071/RJ15061
Eady S. J., Sanguansri P., Bektash R., Ridoutt B., Simons L., Swiergon P. (2013)Carbon footprint for Australian agricultural products and downstream food products in the supermarket (Melbourne). Available at: http://hdl.handle.net/102.100.100/97112?index=1 (Accessed March 30 2022).
Edwards-Jones G., Plassmann K., Harris I. M. (2009). Carbon footprint of lamb and beef production systems: insights from an empirical analysis of farms in Wales, UK. J. Agric. Sci. 147, 707–719. doi: 10.1017/S0021859609990165
Ekonomou A., Dunn J., Wiedemann S., Eckard R. (2020)A greenhouse accounting framework for beef and sheep properties based on the Australian national greenhouse gas inventory methodology. In: Beta version by integrity Ag and environment. Available at: https://piccc.org.au/resources/Tools.html (Accessed October 6 2022).
FAO (2021). Ambición climática en el sector ganadero de américa latina y el caribe – construcción de redes para su revisión e implementación. (Rome: Food and Agriculture Organization of the United Nations (FAO), Climate & Clean Air Coalition (CCAC), New Zealand Agricultural Greenhouse Gas Research Centre (NZAGRC) and Global Research Alliance on Agricultural Greenhouse Gases). doi: 10.4060/cb7332es
FAO (2022). Thinking about the future of food safety – a foresight report. (Rome). doi: 10.4060/cb8667en
Fisher M. J., Braz S. P., dos Santos R. S. M., Urquiaga S., Alves B. J. R., Boddey R. M. (2007). Another dimension to grazing systems. Soil carbon. Trop. Grassl. 41, 65–83. Available at: https://cgspace.cgiar.org/bitstream/handle/10568/43186/journal%20article.pdf?sequence=1&isAllowed=y (Accessed March 14 2022).
Fisher D., Burns J., Pond K. (1987). Modeling ad libitum dry matter intake by ruminants as regulated by distension and chemostatic feedbacks. J. Theor. Biol. 126, 407–418. doi: 10.1016/S0022-5193(87)80148-0
Fisher M. J., Rao I. M., Ayarza M. A., Lascano C. E., Sanz J. I., Thomas R. J., et al. (1994). Carbon storage by introduced deep-rooted grasses in the south American savannas. Nature. 371, 236–238. doi: 10.1038/371236a0
Fisher M. J., Thomas R. J., Rao I. M. (1998). “Management of tropical pastures in acid-soil savannas of south America for carbon sequestration in the soil,” in Management of carbon sequestration in soil (Advances in soil science). Eds. Lal R., Kimble J. M., Follett R. F., Stewart B. A. (Boca Raton: CRC Press), 405–420.
Foote A. P., Hales K. E., Freetly H. C. (2016). Changes in acyl and total ghrelin concentrations and their association with dry matter intake, average daily gain, and feed efficiency of finishing beef steers and heifers. Domest. Anim. Endocrin. 57, 100–107. doi: 10.1016/j.domaniend.2016.05.004
Frappart F., Papa F., Malbeteau Y., León J. G., Ramillien G., Prigent C., et al. (2015). Surface freshwater storage variations in the Orinoco floodplains using multi-satellite observations. Remote Sens. 7 (1), 89–110. doi: 10.3390/rs70100089
Gasser T., Peters G. P., Fuglestvedt J. S., Collins W. J., Shindell D. T., Ciais P. (2017). Accounting for the climate-carbon feedback in emission metrics. Earth Syst. Dynam. 8, 235–253. doi: 10.5194/esd-8-235-2017
Gassón R. A. (2002). Orinoquia: The archaeology of the Orinoco river basin. J. World Prehist. 16 (3), 237–311. doi: 10.1023/A:1020978518142
González-Quintero T., van Wijk M. T., Ruden A., Gómez M., Pantevez H., Castro-Llanos F., et al. (2022). Yield gap analysis to identify attainable milk and meat productivities and the potential for greenhouse gas emissions mitigation in cattle systems of Colombia. Agric. Syst. 195 (83), 103303. doi: 10.1016/j.agsy.2021.103303
Grace J., San José J., Meir P., Miranda H. S. P., Montes R. A. (2006). Productivity and carbon fluxes of tropical savannas. J. Biogeogr. 33, 387–400. doi: 10.1111/j.1365-2699.2005.01448.x
Greenwood P. L. (2021). Review: An overview of beef production from pasture and feedlot globally, as demand for beef and the need for sustainable practices increase. Animal. 15, 100295. doi: 10.1016/j.animal.2021.100295
Handcock R. C., Lopez-Villalobos N., McNaughton L. R., Back P. J., Edwards G. R., Hickson R. E. (2020). Increased yearling weight as a proportion of 21-month weight was associated with increased milk production in dairy heifers. N Z Vet. J. 68 (5), 272–282. doi: 10.1080/00480169.2020.1750498
Hyman G., Castro A., Da Silva M., Arango M. A., Bernal J., Perez O., et al. (2022). Soil carbon storage potential of acid soils of colombia’s Eastern high plains. Front. Sust. Food Systems. 6. doi: 10.3389/fsufs.2022.954017
Instituto de Investigación de Recursos Biológicos Alexander von Humboldt, Deutsche Gesellschaft für Internationale Zusammenarbeit (GIZ) GmbH (2020)El Gran libro de la orinoquia colombiana. In: Instituto de investigación de recursos biológicos Alexander von humbold, ministerio federal de medio ambiente, protección de la naturaleza y seguridad nuclear de la república federal de alemania, ministerio de medio ambiente y desarrollo sostenible de Colombia. (ECOPETROL. Bogotá). Available at: http://repository.humboldt.org.co/bitstream/handle/20.500.11761/35408/GLOC.pdf?sequence=3&isAllowed=y (Accessed November 1 2022).
Jiménez J. J., Moreno A. G., Decaëns T., Lavelle P., Fisher M. J., Thomas R. J. (1998). Earthworm communities in native savannas and man-made pastures of the Eastern plains of Colombia. Biol. Fert. Soils. 28, 101–110. doi: 10.1007/s003740050469
Jones R. M., Tothill J. C. (1985). “BOTANAL - a field and computing package for assessment of plant biomass and botanical composition,” in Proceedings of the international savanna symposium. Eds. Tothill J. C., Mott J. J. (Canberra: Australian Academy of Science), 318–320.
Kanno T., Macedo M. C., Euclides V. P. B., Bono J. A., Santos J. D. G., Rocha M. C., et al. (1999). Root biomass of tropical grass pastures under continuous grazing in Brazilian savannas. Grassl. Sci. 45, 9–14. doi: 10.14941/grass.45.9
Kim S. C., Kim K. U., Kim D. C. (2011). Prediction of fuel consumption of agricultural tractors. Appl. Eng. Agric. 27 (5), 705–709. doi: 10.13031/2013.39565
KLIMATMÄRKING FÖR MAT (2009) Greenhouse gas emissions in animal feed production. report 2. Available at: https://www.klimatmarkningen.se/underlagsrapporter (Accessed February 14 2022).
Ku-Vera J. C., Valencia-Salazar S. S., Piñeiro-Vázquez A. T., Molina-Botero I. C., Arroyave-Jaramillo J., Montoya-Flores M. D., et al. (2018). Determination of methane yield in cattle fed tropical grasses as measured in open-circuit respiratory chambers. Agric. For. Meteorol. 258, 3–7. doi: 10.1016/j.agrformet.2018.01.008
Lascano A. C. E. (1991). Managing the grazing resource for animal production in savannas of tropical America. Trop. Grasslands. 25, 66–72.
Lascano C., Estrada J. E. (1989). “Long-term productivity of legume-based and pure grass pastures in the Eastern plains of Colombia,” in Association Francaise pour la Production Feurragere Proceedings XVI international grassland congress. nice: Association francaise pour la production feurragere, 1179–1180. Available at: https://publons.com/journal/308194/proceedings-of-the-xvi-international-grassland-con/.
Lascano C. E., Teitzel J. K., Eng P. K. (1990)Nutritive value of centrosema in animal production. In: Centrosema: biology, agronomy, and utilization.International center for tropical agriculture (CIAT), publication no. 92 (Cali). Available at: https://cgspace.cgiar.org/handle/10568/54383 (Accessed February 3 2022).
Latawiec A. E., Strassburg B. B. N., Valentim J. F., Ramos F., Alves-Pinto H. N. (2014). Intensification of cattle ranching production systems: socioeconomic and environmental synergies and risks in Brazil. Animal. 8 (8), 1255–1263. doi: 10.1017/S1751731114001566
Lavelle P., Rodríguez N., Arguello O., Bernal J., Botero C., Chaparro P., et al. (2014). Soil ecosystem services and land use in the rapidly changing Orinoco river basin of Colombia. Agric. Ecosys. Environ. 185, 106–117. doi: 10.1016/j.agee.2013.12.020
León-Llanos L. M., Flórez-Díaz H., Duque-Muñoz L. G., Villarroel M., Miranda-de la Lama G. C. (2022). Influence of temperament on performance and carcass quality of commercial Brahman steers in a Colombian tropical grazing system. Meat Sci. 191, 108867. doi: 10.1016/j.meatsci.2022.108867
Lessa A. C. R., Madari B. E., Paredes D. S., Boddey R. M., Urquiaga S., Jantalia C. P., et al. (2014). Bovine urine and dung deposited on Brazilian savannah pastures contribute differently to direct and indirect soil nitrous oxide emissions. Agric. Ecosys. Environ. 190, 104–111. doi: 10.1016/j.agee.2014.01.010
Lynch J., Cain M., Pierrehumbert R., Allen M. (2020). Demonstrating GWP*: a means of reporting warming-equivalent emissions that captures the contrasting impacts of short- and long-lived climate pollutants. Environ. Res. Lett. 15, 044023. doi: 10.1088/1748-9326/ab6d7e
Lynch J., Pierrehumbert R. (2019). Climate impacts of cultured meat and beef cattle. Front. Sustain. Food Syst. 3 (5). doi: 10.3389/fsufs.2019.00005
Marín-López D., Matamoros-Ochoa I. A., Ramírez-Restrepo C. A. (2022). Production dynamics and greenhouse gas modelled emissions from regional dairy production systems in Honduras. Rev. Med. Vet. Zoot. 69 (1), 46–62. doi: 10.15446/rfmvz.v69n1.101526
Marshall N. A., Smajgl A. (2013). Understanding variability in adaptative capacity on rangelands. Rangeland Ecol. Manage. 66, 84–94. doi: 10.1071/RJ15054
Marshall N. A., Stokes C. J., Webb N. P., Marshall P. A., Lankester A. J. (2014). Social vulnerability to climate change in primary producers: A typology approach. Agric. Ecosys. Environ. 186, 86–93. doi: 10.1016/j.agee.2014.01.004
Mehrabi Z., Gill M., van Wijk M., Herrero M., Ramankutty N. (2020). Livestock policy for sustainable development. Nat. Food. 1, 160–165. doi: 10.1038/s43016-020-0042-9
MINEDUCATION (1985) Ley 0073 de octubre 8 de 1985. ministerio de educación (MINEDUCATION). (Bogotá, Colombia). Available at: https://www.mineducacion.gov.co/1759/w3-article-103974.html?_noredirect=1 (Accessed November 30 2021).
MLA (2020) Desmanthus legume in livestock grazing pastures and its role in methane emissions. (Sydney: CSIRO, Agrimix Pastures, Meat and Livestock Australia (MLA) Limited). Available at: https://www.mla.com.au/globalassets/mla-corporate/research-and-development/final-reports/2021/p.psh.1055-final-report.pdf (Accessed October 12 2022).
MLA (2021) Assessment of climate accounting metrics for the Australian red meat industry. (Sydney: Meat and Livestock Australia (MLA) Limited). Available at: https://www.mla.com.au/globalassets/mla-corporate/research-and-development/final-reports/2021/b.cch.2117-final-report.pdf (Accessed October 9 2022).
MLA (2022) Carbon footprint and reduction options for harvest road group operations. (Sydney: Meat and Livestock Australia (MLA) Limited). Available at: https://www.mla.com.au/globalassets/mla-corporate/news-and-events/documents/p.psh.1259-final-report-public-240322-3.pdf (Accessed October 12 2022).
Mueller R. A., Mueller E. A. (2017). Fugitive methane and the role of atmospheric half-life. Geoinform. Geostat. 5 (3), 1–7. doi: 10.4172/2327-4581.1000162
Myhre G., Shindell D., Br´eon F.-M., Collins W., Fuglestvedt J., Huang J., et al. (2013). “Chapter 8, anthropogenic and natural radiative forcing,” in Climate change 2013: The physical science basis. contribution of working group I to the fifth assessment report of the intergovernmental panel on climate change. Eds. Stocker T. F., Qin D., Plattner G.-K., Tignor M., Allen S. K., Boschung J., Nauels A., Xia Y., Bex V., Midgley P. M. (Cambridge: Cambridge University Press), 659–740.
Navas Ríos C. L. (1999). Caracterización socioeducativa, evaluativa y comparativa de cuatro comunidades en los llanos orientales de Colombia (Master thesis). (Medellín, Colombia: Universidad de Antioquia).
Oosting S., ven der Lee J., Verdegem M., de Vries M., Vermoooij A., Bonilla-Cedrez C., et al. (2022). Farmed animal production in tropical circular food systems. Food Secur. 14, 273–292. doi: 10.1007/s12571-021-01205-4
QLD Government (2019) Net carbon position of the Queensland beef industry. (Brisbane: Department of Agriculture and Fisheries). Available at: https://www.futurebeef.com.au/wp-content/uploads/2011/09/Net-carbon-beef-industry.pdf (Accessed September 15 2022).
Ramírez-Restrepo C. A., Barry T. N. (2005). Alternative temperate forages containing secondary compounds for improving sustainable productivity in grazing ruminants. Anim. Feed Sci. Tech. 120, 179–201. doi: 10.1016/j.anifeedsci.2005.01.015
Ramírez-Restrepo C. A., Charmley E. (2015). “An integrated mitigation potential framework to assist sustainable extensive beef production in the tropics,” in Grasslands: A global research perspective. Eds. Mahanta P. K., Singh J. B., Pathak P. S. (Jhansi: Range Management Society of India), 417–436.
Ramírez-Restrepo C. A., O’Neill C. J., López-Villalobos N., Padmanabha J., McSweeney C. (2014). Tropical cattle methane emissions: the role of natural statins supplementation. Anim. Prod. Sci. 54, 1294–1299. doi: 10.1071/AN14246
Ramírez-Restrepo C. A., O’Neill C. J., López-Villalobos N., Padmanabha J., Wang J. K., McSweeney C. (2016a). Effects of tea seed saponin supplementation on physiological changes associated with blood methane concentration in tropical Brahman cattle. Anim. Prod. Sci. 56, 457–465. doi: 10.1071/AN15582
Ramírez-Restrepo C. A., Tan C., O’Neill C. J., López-Villalobos N., Padmanabha J., Wang J., et al. (2016b). Methane production, fermentation characteristics and microbial profiles in the rumen of tropical cattle fed tea seed saponin supplement. Anim. Feed Sci. Techn. 216, 58–67. doi: 10.1016/j.anifeedsci.2016.03.005
Ramírez-Restrepo C. A., Van Tien D., Le Duc N., Herrero M., Le Dinh P., Dinh Van D., et al. (2017). Estimation of methane emissions from local and crossbreed beef cattle in dak lak province of vietnam. Asian australas. J. Anim. Sci. 30, 1054–1060. doi: 10.5713/ajas.16.0821
Ramírez-Restrepo C. A., Vera R. R. (2019). Bodyweight performance, estimated carcass traits and methane emissions of beef cattle categories grazing Andropogon gayanus, Melinis minutiflora and Stylosanthes capitata mixed swards and Brachiaria humidicola pasture. Anim. Prod. Sci. 59, 729–740. doi: 10.1071/AN17624
Ramírez-Restrepo C. A., Vera-Infanzón R. R. (2019). Methane emissions of extensive grazing breeding herds in relation to the weaning and yearling stages in the Eastern plains of Colombia. Rev. Med. Vet. Zoot. 66 (2), 111–130. doi: 10.15446/rfmvz.v66n2.82429
Ramírez-Restrepo C. A., Vera-Infanzón R. R., Rao I. M. (2020). Predicting methane emissions, animal-environmental metrics and carbon footprint from Brahman (Bos indicus) breeding herd systems based on long-term research on grazing of neotropical savanna and Brachiaria decumbens pastures. Agric. Syst. 184, 102892. doi: 10.1016/j.agsy.2020.102892
Ramírez-Restrepo C. A., Vera-Infanzón R. R., Rao I. M. (2023). The carbon footprint of beef production from cull cows finished on sown pastures in the savannas of the Colombian Orinoquía. Arch. Latinoam. Prod. Anim. 31 (1). doi: 10.53588/alpa.310101
Ramírez-Restrepo C. A., Vera R. R. I., Rao I. M. (2019a). “Environmental performance of grazing beef cattle systems in the well-drained neotropical savannas of Colombia: A review of results from modelling research,” in Nutritional strategies for improving farm profitability and clean animal production. book of abstracts of international conference on animal nutrition. Eds. Das A., Das S., Sarkar S., Patra A. K., Mandal G. P., Soren S. (Kolkata: Animal Society of India), 413.
Ramírez-Restrepo C. A., Vera R. R., Rao I. M. (2019b). Dynamics of animal performance, and estimation of carbon footprint of two breeding herds grazing native neotropical savannas in eastern Colombia. Agric. Ecosys. Environ. 281, 35–46. doi: 10.1016/j.agee.2019.05.004
Rao I. M. (1998). “Root distribution and production in native and introduced pastures in the south American savannas,” in Root demographics and their efficiencies in sustainable agriculture, grasslands, and forest ecosystems. Ed. Jr. B. J.E. (Dordrecht: Kluwer Academic Publishers), 19–42.
Rao I. M., Peters M., Castro A., Schultze-Kraft R., White D., Fisher M., et al. (2015). LivestockPlus – the sustainable intensification of forage-based systems to improve livelihoods and ecosystem services in the tropics. Trop. Grassl-Forrajes Trop. 3, 59–82. doi: 10.17138/TGFT(3)59-82
Rao I. M., Plazas C., Ricaurte J. (2001b). “Root turnover and nutrient cycling in native and introduced pastures in tropical savannas,” in Plant nutrition: Food security and sustainability of agro-ecosystems through basic and applied research. Eds. Horst W. J., Schenk M. K., Burkert A., Claassen N., Flessa H., Frommer W. B., Goldbach H., Olfs H.-W., Romheld V., Sattelmacher B., Schmidhalter U., Schubert S., Wiren N. V., Wittenmayer L. (Dordrecht: Kluwer Academic Publishers), 976–977.
Rao I. M., Rippstein G., Escobar G., Ricaurte J. (2001a). “Producción de biomasa vegetal epígea e hipógea en las sabanas nativas,” in Agroecología y biodiversidad de las sabanas en los llanos orientales de Colombia. Eds. Escobar G., Motta F. (Cali: CIAT), 198–222.
Ridoutt B. (2021). Short communication: climate impact of Australian livestock production assessed using the GWP* climate metric. Livest. Sci. 246, 104459. doi: 10.1016/j.livsci.2021.104459
Rincón A., Flórez H., Ballesteros H., León L. M. (2018). Efectos de la fertilización en la productividad de una pastura de Brachiaria humidicola cv. llanero en el piedemonte de los llanos orientales de Colombia. Trop. Grassl-Forrajes Trop. 6 (1), 158–168. doi: 10.17138/TGFT(6)158-168
Rincón A., Villalobos M. (2021). Producción animal en pasturas de tres leguminosas asociadas con Urochloa decumbens en los llanos orientales de Colombia. Trop. Grassl-Forrajes Trop. 9 (2), 192–205. doi: 10.17138/TGFT(9)192-205
Romero-Ruiz M. H., Flantua S. G. A., Tansey K., Berrio J. C. (2011). Landscape transformations in savannas of northern south America: Land use/cover changes since 1987 in the llanos orientales of Colombia. Appl. Geogr. 32, 766–776. doi: 10.1016/j.apgeog.2011.08.010
Rondón M., Acevedo D., Hernández R. M., Rubiano Y., Rivera M., Amézquita E., et al. (2006). “Carbon sequestration potential of the neotropical savannas (Llanos) of Colombia and Venezuela,” in Carbon sequestration in soils of Latin America. Eds. Lal R., Kimble J. (Binghampton: The Haworth Press, Inc.), 213–243.
Rotta P. P., Menezes A. C. B., Costa e Silva F. C., Valadares Filho S., de C., Prados L. F., et al. (2016). “Protein requirements for beef cattle,” in Nutrient requirements of zebu and crossbred cattle BR-CORTE., 3rd. Eds. de Valadares Filho S. C., Costa e Silva L. F., Gionbelli M. P., Rotta P. P., Marscondes M. I., Chizotti M. L., Prados L. F. (Viçosa: Universidade Federal de Viçosa), 185–212.
SAS (2021) Statistical analysis system OnDemand for academics. Available at: https://www.sas.com/en_au/software/on-demand-for-academics.html (Accessed June 17 2022).
Sen L. T. H., Bond J., Dung N. T., Hung H. G., Mai N. T. H., Phuong H. T. A. (2021). Farmers’ barriers to the access and use of climate information in the mountainous regions of Thừa thiên Huế province, Vietnam. Clim. Serv. 24, 100267. doi: 10.1016/j.cliser.2021.100267
Sen L. T. H., Bond J., Hoang H. D. T. (2022). Exploring smallholder farmers’ climate adaptation decisionmaking in mountainous areas of central Vietnam: implications for extension services. J. Agric. Educ. Ext., 1–22. doi: 10.1080/1389224X.2022.2039248
Skidmore M. E., Sims K., Raush L. L., Gibbs H. K. (2022). Sustainable intensification in the Brazilian cattle industry: the role for reduced slaughter age. Environ. Res. Lett. 17, 064026. doi: 10.1088/1748-9326/ac6f70
Slebodnik K. A. (2020) Effect of plant derived tannins on nitrogen and carbon cycling in pasture soils (Master thesis). (Logan, USA: Utah State University). Available at: https://digitalcommons.usu.edu/etd/7803 (Accessed October 20 2022).
Stephens N., Di Silvio L., Ellis M., Glencross A., Sexton A. (2018). Bringing cultured meat to market: Technical, socio-political, and regulatory challenges in cellular agriculture. Trends Food Sci. Technol. 78, 155166. doi: 10.1016/j.tifs.2018.04.010
Tedeschi L. O., Muir J. P., Naumann H., Norris A., Ramírez-Restrepo C. A., Talcott S. (2021). Nutritional and methodological aspects of ecologically relevant phytochemicals in ruminant production. Front. Vet. Sci. 8. doi: 10.3389/fvets.2021.628445
Trujillo W., Fisher M. J., Lal R. (2006). Root dynamics of native savanna and introduced pastures in the Eastern plains of Colombia. Soil Till. Res. 87, 28–38. doi: 10.1016/j.still.2005.02.038
UN (2015) Transforming our world: The 2030 agenda for sustainable development. A/RES/70/. (New York: United Nations, Department of Economic and Social Affairs Sustainable Development). Available at: https://sdgs.un.org/publications/transforming-our-world-2030-agenda-sustainable-development-17981 (Accessed February 20 2022).
University of Arkansas (2019) The field capacity calculator. Available at: https://www.uaex.edu/farm-ranch/economics-marketing/docs/FieldCapacity.xls (Accessed January 15 2022).
van Cleef O. S. F., Dubeux J. C. B. Jr., Ciriaco F. M., Henry D. D., Ruisz-Moreno M., Jaramillo D. M., et al. (2022). Inclusion of a tannin-rich legume in the diet of beef steers reduces greenhouse gas emissions from their excreta. Sci. Rep. 12, 14220. doi: 10.1038/s41598-022-18523-y
Vandermeulen S., Ramírez-Restrepo C. A., Beckers Y., Claessens H., Bindelle J. (2018a). Agroforestry for ruminants: a review of trees and shrubs as fodder in silvopastoral temperate and tropical ruminant production systems. Anim. Prod. Sci. 58 (5), 767–777. doi: 10.1071/AN16434
Vandermeulen S., Singh S., Ramírez-Restrepo C. A., Kinley R. D., Gardiner C. P., Holtum J. A. M., et al. (2018b). In vitro assessment of rumen fermentation, digestibility and methane production of three species of Desmanthus for application in northern Australian grazing systems. Crop Pasture Sci. 69 (8), 797–807. doi: 10.1071/CP17279
Velásquez J. C., Ríos M. (2010). Evaluación de la producción de carne a partir de vacas cebú de descarte. Rev. Ciencia Animal. 3, 23–29.
Vera R. R., Hoyos F. (2019). Long-term beef production from pastures established with and without annual crops compared with native savanna in the high savannas of Eastern Colombia: a compilation and analysis of on-farm results 1979-2016. Trop. Grassl-Forrajes Trop. 7 (1), 1–13. doi: 10.17138/TGFT(7)1-13
Vera-Infanzón R. R., Ramírez-Restrepo C. A. (2020). Long term beef production in extensive cow-calf systems in the tropical savannas of eastern Colombia. Rev. Med. Vet. Zoot. 67 (1), 42–59. doi: 10.15446/rfmvz.v67n1.87678
Vera-Infanzón R. R., Ramírez-Restrepo C. A. (2022). Modeling the reproductive performance of tropical beef herds using long-term experimental grazing data on Urochloa humidicola pastures in the llanos of Colombia. Arch. Latinoam. Prod. Anim. 30 (3), 225–236. doi: 10.53588/alpa.300307
Vera-Infanzón R. R., Rao I. M., Ramírez-Restrepo C. A., Hoyos-Garcés F. (2023). A dynamic simulation model to assess farm-level effects of pasture intensification strategies on beef herd outputs and carbon footprints in acid soil savannas of Eastern colombia. Arch. Latinoam. Prod. Animal. 31 (1). doi: 10.53588/alpa.310102
Vera R. R., Ramírez-Restrepo C. A. (2017). Complementary use of neotropical savanna and grass-legume pastures for early weaning of beef calves, and effects on growth, metabolic status and reproductive performance. Trop. Grassl-Forrajes Trop. 5 (2), 50–65. doi: 10.17138/tgft(5)50-65
Vera R. R., Ramírez C. A., Velásquez N. (2002). Growth patterns and reproductive performance of grazing cows in a tropical environment. Arch. Latinoam. Prod. Anim. 10, 14–19.
Vercoe J. E., Frisch J. E. (1970). “Digestibility and nitrogen metabolism in Brahman, africander and shorthorn x Hereford cattle fed lucerne hay,” in In: Proceedings of the Australian society of animal production; 8th biennial meeting. animal production in Australia., vol. 8. (Armidale: ASAP), 131–135.
Waghorn G. C., Ulyatt M. J., John A., Fisher M. T. (1987). The effect of condensed tannins on the site of digestion of amino acids and other nutrients in sheep fed on Lotus corniculatus. Br. J. Nutr. 57, 115–126. doi: 10.1079/BJN19870015
Weiler V., Udo H. M. J., Viets T., Crane T. A., De Boer I. J. M. (2014). Handling multi-functionality of livestock in a life cycle assessment: the case of smallholder dairying in Kenya. Curr. Opin. Environ. Sustain. 8, 29–38. doi: 10.1016/j.cosust.2014.07.009
Wiedemann S. G., Henry B. K., McGahan E. J., Grant T., Murphy C. M., Niethe G. (2015). Resource use and greenhouse gas intensity of Australian beef production: 1981–2010. Agric. Syst. 133, 109–118. doi: 10.1016/j.agsy.2014.11.002
Wiedemann S. G., McGahan E. J., Murphy C. M., Yan M. (2016). Resource use and environmental impacts from beef production in eastern Australia investigated using life cycle assessment. Anim. Prod. Sci. 56, 882–894. doi: 10.1071/AN14687
Zhang X., Amer P. R., Stachowicz K., Quinton C., Crowley J. (2021). Herd-level versus animal-level variation in methane emission prediction in grazing dairy cattle. Animal. 15, 100325. doi: 10.1016/j.animal.2021.100325
Keywords: beef farming systems, cow-calf operations, improved pastures, methane emission, soil carbon capture
Citation: Ramírez-Restrepo CA, Vera-Infanzón RR and Rao IM (2023) The carbon footprint of young-beef cattle finishing systems in the Eastern Plains of the Orinoco River Basin of Colombia. Front. Anim. Sci. 4:1103826. doi: 10.3389/fanim.2023.1103826
Received: 21 November 2022; Accepted: 06 January 2023;
Published: 03 February 2023.
Edited by:
Christine Janet Nicol, Royal Veterinary College (RVC), United KingdomReviewed by:
Donald M. Broom, University of Cambridge, United KingdomSerkan Ates, Oregon State University, United States
Copyright © 2023 Ramírez-Restrepo, Vera-Infanzón and Rao. This is an open-access article distributed under the terms of the Creative Commons Attribution License (CC BY). The use, distribution or reproduction in other forums is permitted, provided the original author(s) and the copyright owner(s) are credited and that the original publication in this journal is cited, in accordance with accepted academic practice. No use, distribution or reproduction is permitted which does not comply with these terms.
*Correspondence: Carlos A. Ramírez-Restrepo, Yy5yYW1pcmV6QGNyZWFjLmNvbS5hdQ==