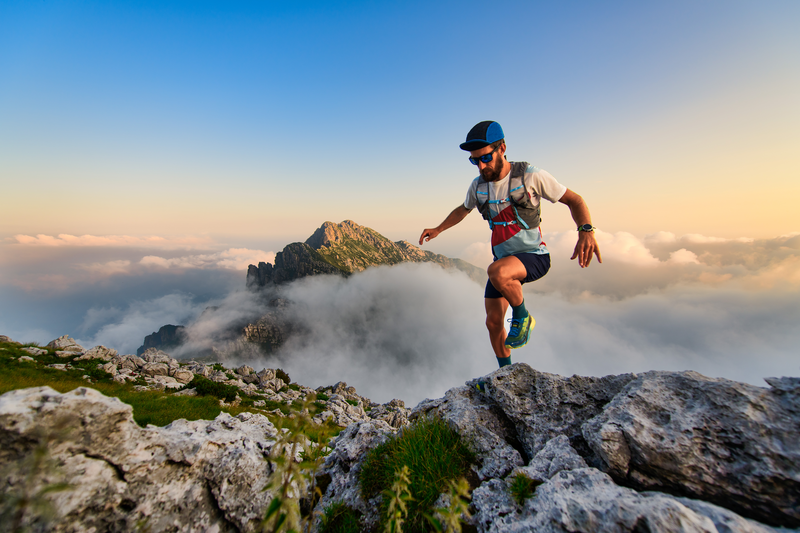
94% of researchers rate our articles as excellent or good
Learn more about the work of our research integrity team to safeguard the quality of each article we publish.
Find out more
REVIEW article
Front. Anim. Sci. , 04 October 2022
Sec. Animal Nutrition
Volume 3 - 2022 | https://doi.org/10.3389/fanim.2022.998042
This article is part of the Research Topic Seaweeds and Algae in Animal Nutrition View all 4 articles
Modern poultry production systems face numerous economic, environmental, and social sustainability challenges that threaten their viability and acceptability as a major source of animal protein. As scientists and producers scramble to find cost-effective and socially acceptable solutions to these challenges, the dietary use of marine macroalgae (seaweeds) could be an ingenious option. Indeed, the incredible array of nutritive and bioactive compounds present in these macroscopic marine organisms can be exploited as part of sustainable poultry production systems of the future. Incorporating seaweeds in poultry diets could enhance feed utilization efficiency, growth performance, bird health, meat stability and quality, and consumer and environmental health. Theoretically, these benefits are mediated through the putative antiviral, antibacterial, antifungal, antioxidant, anticarcinogenic, anti-inflammatory, anti-allergic, antithrombotic, neuroprotective, hypocholesterolemic, and hypoglycemic properties of seaweed bioactive compounds. Despite this huge potential, exploitation of seaweed for poultry production appears to be constrained by a variety of factors such as high fibre, phenolics, and ash content. In addition, conflicting findings are often reported when seaweeds or their extracts are used in poultry feeding trials. Therefore, the purpose of this review paper is to collate information on the production, phytochemical components, and nutritive value of different seaweed species. It provides an overview of in vivo effects of dietary seaweeds as measured by nutrient utilization efficiency, growth performance, and product quality and stability in poultry. The utility of dietary seaweeds in sustainable poultry production systems is explored, while gaps that require further research are highlighted. Finally, opportunities that exist for enhancing the utility of seaweeds as a vehicle for sustainable production of functional poultry products for better global food and nutrition security are presented.
The important role that poultry products play in the food and nutrition security of millions of households globally remains unequivocal. This is supported by poultry production statistics that show rapid, continuous gains worldwide. For example, in 2017, chicken meat contributed 37% of total meat produced globally (FAOSTAT, 2020). Poultry products are especially important for human nutrition in developing nations because of their affordability, higher availability, and limited religious restrictions (FAO, 2013). The race to meet a rapidly increasing demand for poultry products has seen the emergency of large-scale, industrialized poultry farms that are heavily reliant on chemotherapeutics (Lee et al., 2012) while paying little attention to bird welfare (Karcher and Mench, 2018), product quality or environmental stewardship (Amato et al., 2020). There is a general tendency for poultry producers to focus on maximizing production at any cost, an approach that is environmentally, socially, and economically unsustainable, given that most resources on earth are finite. The net effect of this approach has been the widespread erosion of societal confidence in the ability and desire of the industry to meet its obligations to society (Hafez and Attia, 2020). Recent changes towards alternative organic poultry production systems (Jeni et al., 2021) are an attempt to restore this confidence and secure the social license needed to continue operations. To compound matters, most of the land-based feed resources used in the formulation of poultry diets, such as maize grain and soybeans, are also good enough for direct human consumption thus inflating their demand and market prices on the world market (Masenya et al., 2021). In addition, cultivation of these feed resources directly generates greenhouse gas emissions (Theurl et al., 2020) while the expansion of arable lands often leads to deforestation that decimates vital carbon sinks (Theurl et al., 2020). This makes current poultry production systems environmentally unsustainable. Yet another challenge relates to the use of prophylactic antibiotics in modern intensive poultry production systems to prevent infectious and stress-induced diseases and enhance feed utilization efficiency (Engberg et al., 2000). This practice has been shown to promote antibiotic resistance and accumulation of antibiotic residues in poultry products, outcomes that have negative implications on human health (Cervantes, 2015). Attempts to mitigate the sustainability challenges in poultry production have taken many forms, including the dietary incorporation of seaweeds (Nhlane et al., 2020; Matshogo et al., 2021; Stokvis et al., 2022). These marine macroalgae can be produced sustainably in oceans with no requirement for fresh water, fertilizers, or land (Duarte et al., 2017). They play a role in climate change adaptation and mitigation through carbon sequestration as part of the blue carbon economy (Yong et al., 2022). These marine organisms can be used as safe and environmentally friendly feed ingredients. Their nutrients and bioactive compounds have been reported to modulate the immune system, improve nutrient utilization efficiency and growth performance, enhance product quality and stability, and prevent disease outbreaks in poultry (Matshogo et al., 2021; Stokvis et al., 2022). These desirable effects are thought to be mediated through antibacterial, antifungal, antiviral, vermifuges, neuroprotective, antitumoral, anti-inflammatory, anti-allergic, antithrombotic, hypocholesterolemic, and hypoglycemic properties of seaweed bioactive compounds (Matshogo et al., 2021). Seaweeds are also rich in n-3 long chain polyunsaturated fatty acids (LC-PUFA), thus products from birds reared on seaweed-containing diets are likely to supply physiologically effective doses of beneficial LC-PUFA to consumers. Despite these potential benefits, the use of seaweeds in poultry diets is not as prevalent as expected due to several challenges, ranging from low availability, poor accessibility, high cost, low digestibility (Matshogo et al., 2021; Costa et al., 2022), lack of regulatory framework specific to their use as nutraceuticals for poultry, and lack of information on how best to incorporate them in practical poultry diets. This review provides an overview of in vivo effects of dietary seaweeds as measured by nutrient utilization efficiency, growth performance, and product quality and stability in poultry. The paper also explores the consensus and contradictions that exist in literature regarding the utility of dietary seaweeds in sustainable poultry production systems while highlighting the gaps that require further research.
Like it has been for millennia, poultry products continue to serve as affordable sources of animal protein for people, especially in low-income countries. It is, therefore, not surprising that the poultry industry is currently the largest and fastest growing animal agriculture sub-sector in developing countries. Globally, poultry meat and eggs are one of the most consumed animal products and their demand is expected to continue to rise owing to the ever-growing human population, urbanization, and increasing income (Øverland et al., 2019). Indeed, the Food and Agriculture Organisation reported a global population of 23 billion poultry birds in 2016, translating to at least three birds per person (FAOSTAT, 2016). About 73 million tons of eggs and nearly 100 million tons of poultry meat are produced globally (Mottet and Tempio, 2017). Approximately 92% of poultry meat comes from high-input large-scale broiler systems with layers only contributing 6% of the total (Mottet and Tempio, 2017). The backyard systems are said to contribute around 2% of poultry meat and 8% of eggs worldwide (Mottet and Tempio, 2017). However, it should be noted that there is a huge variation in the consumption of poultry meat and eggs among countries, with much lower figures likely in poorer countries. This suggests that sustained efforts are required to ensure that households in low-income countries have access to affordable and high-quality poultry products. This requires the development of sustainable and efficient production systems that would ensure that the poultry industry continues to contribute towards food and nutrition security across diverse cultures, religions, and ethnic groups. The high nutrient density of poultry products together with the birds’ fast growth rates, high feed conversion efficiency, and short production cycles should be exploited as favorable attributes to alleviate protein-energy malnutrition and eradicate food insecurity in hunger-stricken countries.
Even though the poultry industry is the fastest-growing agricultural subsector, production is projected to increase at a slower rate than in previous decades owing to high production costs driven by exorbitant feeding costs. Nkukwana (2018) alluded that feed costs, which constitutes about 75% of total production costs, hamper profit margins in animal agro businesses. This is commonly attributed to the volatile changes in maize and soybean prices. These two ingredients have direct food value for humans and their demand continues to increase in the food, feed, and biofuel sectors, which consequently leads to higher market prices. Accordingly, many scholarly reports have indicated that the over-reliance on these ingredients for poultry feed formulation is economically, socially, and environmentally unsustainable for large-scale poultry intensification (Marareni and Mnisi, 2020). Moreover, cultivation of maize and soybeans requires large hectares of arable land, large volumes of water, fertilizers, pesticides, fuel, and machinery, among others (Marareni and Mnisi, 2020). From an environmental standpoint, the cultivation of maize and soybeans results in deforestation, soil compaction and erosion, eutrophication, pollution, and high atmospheric carbon footprint (Castanheira and Freire, 2013), which is undesirable in the face of climate change. In addition, to prevent stress-induced disease outbreaks and enhance feed utilization efficiency in modern intensive poultry production systems, in-feed prophylactic antibiotics have been the strategy of choice (Engberg et al., 2000). However, reliance on in-feed antibiotics also increases production costs because they are relatively expensive (Thema et al., 2019). Moreover, there is on-going public concern that using antibiotics in this manner contributes to antibiotic resistance with negative implications on human health (Cervantes, 2015). Antibiotic residues in poultry products are also a major concern as they are reported to cause negative health outcomes in consumers (Thema et al., 2019).
In response to climate change and population growth, there has been increasing global interest in the use of seaweeds to promote sustainable animal production systems. Seaweeds are a valuable natural resource that serves as a remarkable source of functional constituents with promising applications in food and feed for humans and animals, respectively. They are categorized into three colour pigment groups: red including about 7000 species of Rhodophyta; brown including about 2000 species of Phaeophycea; and green with about 1000 species of Chlorophyta (Ganesan et al., 2019). Various seaweed species have attracted extensive research attention from biomedicine to agri-food industries as putative sources of multiple biologically active compounds such as proteins, glycoprotein, minerals, vitamins, essential fatty acids, fucoidan, carotenoids, peptides, dietary fibers, oxylipins, violaxanthin, steroids, zeaxanthin, phlorotannins, lutein, fucoxanthin, and laminarin (Venkatesan et al., 2019). Due to the presence of these bioactive compounds, there has been growing interest from researchers to use seaweeds for various applications in medicine, cosmetics, biofuel, and fertilizer-manufacturing industries (Poza et al., 2022). Indeed, these bioactive compounds have functional benefits with antioxidant, antimicrobial, hypoglycemic, antiradiation, immunomodulatory, anti-inflammatory, anticancer, growth-stimulating, meat-boosting, and health-promoting properties (Wang et al., 2019). Apart from their nutraceutical properties, seaweeds are sustainable natural bioresources that do not compete with terrestrial plants for arable land, fresh water, pesticides, fertilizers, and insecticides to grow, and yet they have high productivity (Balina et al., 2017). Seaweeds acquire nutrients directly from the sea. All these desirable characteristics suggests that utilizing seaweeds as a functional feed ingredient in poultry nutrition could reduce feed-food competitions and thus deliver efficient, sustainable, and profitable poultry production systems. The use of seaweeds as poultry feed ingredients will also ensure that consumers have indirect access to bioactive compounds through the consumption of high-quality, functional poultry products. This strategy requires the optimization of seaweed production, harvesting, processing, and distribution systems to ensure that the product is readily available at low cost.
Seaweeds are botanically classified as macroalgae and can grow in the shallow waters at the edge of the ocean. Globally, there are several types of seaweeds that can be found floating and submerged in the intertidal zone and ocean tropical waters (Hayashi et al., 2010). In their natural environment, seaweeds grow on rocks, stones, pebbles, and dead corals while some exist as epiphytes (Bhagyaraj and Kunchithapatham, 2016). Given the rapidly increasing demand for seaweeds that is driven by the search for natural bioactive products (Harb and Chow, 2022) for use in pharmaceutical and feed/food industries, it is important to sustainably intensify the production of seaweeds. In 2015, an estimated 196 570 tons of seaweeds were produced worldwide, however, there was a 43% reduction in production between 2015 and 2018 due to climate-induced outbreaks of pests and diseases (Sánchez-Velásquez et al., 2021). In 2019, total algae production was recorded at about 36 million t (FAO, 2021), of which 50% was produced from seaweed aquaculture systems.
Cultivation of seaweeds is one of the most important global food/feed production activities because it also offers opportunities for mitigation and adaptation to climate change (Duarte et al., 2017), in addition to providing valuable natural bioactive compounds. However, global seaweed production is experiencing relatively low growth rates due to slow growth of tropical seaweeds species (FAO, 2020) and unprecedented pest and disease outbreaks in cultivated seaweed systems (Ward et al., 2020). For example, in the Philippines, outbreaks of ‘ice–ice’ disease and epiphytic pests have reduced seaweeds yields (Kambey et al., 2020). Globally, seaweed diseases and pests have become increasingly common in the past decade, threatening the future of the seaweed industry, especially of the higher value Kappaphycus sp. (Largo et al., 2020). The main method of cultivation is the bottom monoline technique, which is applied in shallow waters in the nearshore/intertidal zone, allowing the lines to be exposed during the spring low tide. Other cultivation methods such as the raft, broadcasting, and net methods, have not been evaluated for economic feasibility to allow their use in commercial farming (Kimathi et al., 2018). Cultivated and naturally occurring seaweeds, including beach-cast ones, are the two major sources of these macroalgae. There is currently limited research on the differences between cultivated and wild seaweeds, however, some studies have reported that their nutritional composition, physical make-up, and subsequent utilization depend on the harvesting site, water temperature or pH, salinity, concentration of heavy metals, light intensity, as well as the employed processing method (Chizhov et al., 1998; Kadam et al., 2015; Nhlane et al., 2020). Nonetheless, frequent collection and utilization of seaweed on beaches also creates an attractive environment for tourism while providing the feed industry with a low-cost source of beneficial bioactive compounds.
The cleaning process of beaches is part of the harvest activities of beach-cast seaweeds, which is done in accordance with the rules and regulations that govern treatment of organic waste in most jurisdictions. However, this waste biomass can be reused to produce agar (Gelidium), as fertilizer for crops (Lorbeer et al., 2013), or as a feed ingredient in various food animal production systems, including poultry. Unfortunately, harvesting beach-cast seaweeds has the unintended consequence of removing between 100 and 400 m3 of sand per harvesting season, thus increasing erosion and changing the topography of beaches (Hyndes et al., 2021). In South Africa, commercially harvested beach-cast seaweed is mainly used for human consumption and in nutraceutical, cosmetic, and fucoidan-processing industries (Poza et al., 2022). For seaweeds that are not beach-cast, harvesting traditionally involves cutting fronds during the low tide using boats. The whole “head” is then pulled aboard, leaving the stipe and holdfast to die. Recovery of the biomass then requires the growth of new sporophytes occur. Ashore, the fronds are then cut off and the primary blade discarded. Another type of harvesting method that is based on experiments reported by Levitt et al. (2002) has been proposed. It involves excising only the distal parts of the secondary blades and leaving at least 20 – 30 cm of their bases (with the basal meristems intact) attached to the primary blade, allowing fronds to be harvested again within a short period of time. This non-lethal harvesting approach by Levitt et al. (2002) is said to allow higher biomass yields over time. However, given that frond regrowth rates decline as the sporophytes age, it may be necessary to carry out periodic lethal harvests to allow new plants to emerge and contribute to biomass yield.
While seaweeds are a rich source of nutrients and bioactive compounds, including carotenoids, proteins, peptides, vitamins, minerals, oxylipins, phlorotannins, steroids, minerals, essential fatty acids, dietary fibers, polysaccharides, and sulphated polysaccharides (Venkatesan et al., 2019), their chemical composition varies greatly. Indeed, the concentration and profile of chemical components of seaweeds is influenced by many factors including species, geographical location, drying process, harvesting, humidity, season, and temperature (Garcia et al., 2016). Species variation in type and concentration of bioactive compounds is so wide that certain species even contain toxic rather than beneficial compounds. This information is crucial as such compounds can limit the utilization of seaweed species as feed for animals. For example, Caulerpa saxifolia contains sesquiterpene caulerpyne that blocks the mitotic cycle of sea urchin embryos and inhibits stimulation of mitogen-activated protein kinase (Mozzachiodi et al., 2001). The likelihood of such compounds exerting their negative effects in food animals such as chickens is high and should be carefully investigated prior to incorporation in poultry diets. Huge within-genus variations in terms of chemical composition have been demonstrated (Lares et al., 2002) as shown in Tables 1, 2. Chemical composition of seaweeds has also been demonstrated to vary with environmental changes as modulated by seasons. Indeed, a study conducted by Marinho-Soriano et al. (2006) reported a positive correlation between carbohydrates content and temperature in the red seaweed, Gracilaria caudate. Similarly, Hu (2004) revealed that high light intensity increases the production of polysaccharides in seaweeds. In a study by Manns et al. (2017) sugar levels were higher in summer compared to winter in the brown seaweeds, Laminaria digitata and Saccharina latissima. The growth stage of seaweed species can also affect the biosynthesis, presence/absence, and potential bioaccumulation of various chemical constituents (Zou et al., 2018). However, limited understanding of the mechanisms through which environmental parameters influence the levels of nutrients and bioactive compounds continue to hinder the full exploitation of seaweeds as animal feed ingredients (Cassani et al., 2020).
The concentration and bioactivity of beneficial compounds in seaweeds can also be affected by processing methods. The most common seaweed processing method is moisture removal through sun-drying (Carrillo et al., 1992), oven-drying (Hamdy and Dawes, 1988), and freeze-drying (Mabeau et al., 1992). These methods can drastically increase the shelf-life of seaweeds. Drying is also a preparatory step in the extraction of important chemical constituents from seaweeds. However, drying can affect the quality, color, aroma, as well as their and nutrient and phytochemical concentration (Chan et al., 1997). The quality of seaweed can be reduced if heat-labile beneficial compounds are lost during the drying process. For example, phenolic compounds (capable of acting as antioxidants) are often depleted upon drying resulting in reduction in antioxidant value of dried products (Badmus et al., 2019). For example, according to Mabeau and Fleurence (1993) drying at high temperatures greatly alters the vitamin C content in brown seaweed. In addition, the bioavailability of molecules such as amino acids, proteins, lipids, fatty acids, and secondary compounds in seaweeds may also be negatively affected by drying process (Silva et al., 2019).
The species-induced variation in proximate composition (on a dry matter basis) of seaweeds is presented in Table 1. For example, within the red seaweed group, neutral detergent fibre (NDF) content can be as high as 43.1% in Porphyra sp. and as low as 27.2% in Asparagopsis armata, while within green seaweeds (Ulva sp.) a much narrower range (22.8 to 26.2%) has been reported (Table 1). In brown seaweeds, NDF content can be as high as 22.0% in Ascophyllum nodosum and as low as 16.6% in Laminaria sp. Wide species-depended variations in ash content are evident in Ulva sp. (7.7 – 23%) and brown seaweeds (22 – 32.9%) but not in red seaweeds (6.5 – 10%). There is limited species variation in lipid (ether extract) content of seaweeds, which is generally below 1% except in A. nodosum where as high as 3.9% ether extracts were recorded (Table 1). Species differences in terms of crude protein content are also evident, especially within the red seaweed group, where it can be as high as 38.1% in Porphyra sp. and as low as 17.8% in A. taxiformis. In brown seaweeds, crude protein content varies widely, ranging from 6 (A. nodosum) – 16.6% (Laminaria sp.). Differences in the crude protein content of the seaweeds can be caused by the drying and/or processing methods used after harvesting. The protein value also differs according to the value of the nitrogen-to-protein conversion factor used. The average value of the nitrogen-to-protein conversion factor is 5.13 for green seaweed, 5.38 for brown seaweed and 4.92 for red seaweed (Lourenço et al., 2002). In addition, seaweed is a significant source of some water-soluble and lipid-soluble vitamins. Water-soluble vitamins in seaweed are represented by vitamin C and vitamins of the B group, especially B1, B2 and B12. In a systematic review of 92 seaweed species, vitamin C content was found to be 0.773 mg/g DM of seaweed with a 90th percentile of 2.06 mg/g DM (Nielsen et al., 2021).
The species-induced variation in mineral composition of seaweeds is represented in Table 2. Within the red seaweed group, Ca content can be high as 4.47% in A. armata and as low as 3.8% in A. taxiformis. However, in brown seaweed, Ca content can be high as 25.3% in Laminaria sp., but as low as 1.0% in A. nodosum. Most seaweed species presented in Table 2 have P levels ranging from 0.1 to 0.27% while Porphyra sp (3.8%) and Macrocystis sp. (2.7%) registered very high P levels. Within red seaweed species, Mg content can be high as 4.9% in Porphyra sp. And as low as 0.8% in A. taxiformis. However, in brown seaweeds, the variation in Mg content is much larger ranging from 0.5% in Ascophyllum nodosum to 39.2% in Macrocystis sp. Species-dependent variation in Na content is much lower in red seaweeds (4.1 – 9.36%) compared to brown seaweeds where it ranges from 2.4% in A. nodosum to as high as 36.5% in Macrocystis sp. In general, brown seaweeds species seem to have higher levels of Fe, Mn, Cu, and Zn compared to red seaweeds (Table 2). Species differences in mineral composition of seaweeds is expected since the processes of absorption, utilization and bioaccumulation of minerals are genetically controlled.
The increasing interest in seaweeds as functional feed ingredients in poultry production is driven by the myriad of primary and secondary plant compounds (Table 3) that have both nutritional and pharmaceutical properties (Ummat et al., 2021). These bioactive chemicals are naturally occurring essential and non-essential compounds that have been demonstrated to alter human and animal health (Michalak and Mahrose, 2020). The listed seaweed bioactive compounds have been demonstrated to possess antioxidant, antimicrobial, immunomodulatory, antiviral, antitumor, and antibacterial properties (Ummat et al., 2021). These makes these compounds essential in optimizing gut environment, inhibiting oxidative stress and inflammation, enhancing immunity, and improving growth performance in animals. Indeed, improvement on feed intake, average daily gain, feed conversion ratio; reduction on meat fat pads; increased egg and shell weights; and reduced egg and serum cholesterol and total lipids have been attributed to these bioactive compounds (Catarino et al., 2020; Michalak et al., 2022).
Carotenoids are pigments that play a key role in light-harvesting through the process of photosynthesis, they make up 8 – 14% of the biomass in red, green, and brown seaweeds (Priyadarshani and Biswajit, 2012; Mulders et al., 2014 ). They can be further classified into primary or secondary carotenoids depending on their metabolism and function. Primary carotenoids are the structural and functional components that aid in photosynthesis while the extra-photosynthetic pigments produced by carotenogenesis under specific environmental circumstances are referred to as secondary carotenoids (Kumar et al., 2021). Primary carotenoids include α-carotene, β-carotene, neoxanthin, violaxanthin, zeaxanthin, fucoxanthin, and lutein, whereas secondary carotenoids include echinenone, canthaxanthin, and astaxanthin (Poojary et al., 2016). Carotene is a key precursor of vitamin A, which prevents night blindness and cataracts. It also aids in glycoprotein formation, mucus secretion from epithelial tissues, cell differentiation, overall bone and body development and reproduction, properties that could be valuable in enhancing poultry productivity (Kumar et al., 2021). Carotenoids impart important functional utility to seaweed meals through their antioxidant and immune-boosting properties, which may reduce cardiovascular disease, inflammation, neurological disorders, obesity, cancer, and age-related muscle diseases (Borowitzka and Borowitzka, 1987; Dutta et al., 2005; Perusek and Maeda, 2013; Miyashita et al., 2020) in a variety of animals and animal models. According to Henriquez et al. (2016), the Chlorophyceae family, which includes Chlamydomonas, Chlorella, Dunaliella, Haematococcus and Muriellopsis spp., is the major source of carotenoids. They have the most pigment diversity and are the only group that overproduces secondary carotenoids in response to stressful growing conditions to provide photoprotective functions (Mulders et al., 2014).
Carrageenan is a naturally occurring anionic sulfated linear polysaccharide found in Chondrus cripus, Euchema, Gigartinastellate, Iridaea, Hypnea, Solieria, Agardhiella, and Sarconema red seaweeds of the Rhodophyceae family (Prajapati et al., 2014). The polysaccharide chains are made up of sulphate half-esters that are linked to sugar units. McHugh (2003) stated that carrageenan comes in various chemical structures and characteristics, depending on the amount and position of the SO3−groups. These compounds are classified into kappa, lambda, and iota forms, which all contain about 22 – 35% of the sulphate groups (Prajapati et al., 2014). Carrageenan is a versatile ingredient that has no nutritional value, but it can be used in both food and non-food industries (Van de Velde et al., 2002). It has recently been used in the pharmaceutical industry as an excipient in pills and tablets, as well as a potent raw material for hydrogels, due to its putative anti-tumour, immunomodulatory, antiviral, anti-inflammatory, anticoagulant, and antithrombotic properties (Wang et al., 2019) that could support immune responses and protect poultry from debilitating pathogenic infections and could also play a role as an alternative for in-feed antibiotics.
Fucoidan is a sulfated polysaccharide found in the cell walls of brown seaweeds (Phaeophyceae). The chain is made up of fucose as the primary monosaccharide, together with varying amounts of galactose, mannose, xylose, rhamnose, and glucuronic acid residues (Ponce and Stortz, 2020). It is a polymer made up of 1,2-linked L-fucose-4-sulfate units and, in rare circumstances, 1,3- or 1,4-linked fucan sulphate with side chains of galactose, uronic acid, and xylose residues (Kumar et al., 2021). Lim and Wan Aida (2017) stated that the chemical structures and composition of this sulfated polysaccharide vary substantially based on geographical location, species, seasons, and population age of seaweed. Commercially, fucoidans are extracted from Ascophyllum nodusum, Ecklonia cava, Fucus vesiculosus, Saccharina longicruris, and Undaria pinnatifida brown seaweeds (Athukorala et al., 2006). Fucoidan also exhibits strong and important biological bioactivities, which include antiviral, antitumoral, antioxidant, anticoagulant, immunomodulation, antithrombotic, anti-complement, and anti-proliferative properties (Wang et al., 2019; Saeed et al., 2021) with potential utility as immune-boosters and growth promoters in birds.
Phlorotannins are a class of polyphenolics biosynthesized via the acetate malonate pathway as secondary metabolites in brown seaweeds. They are found either in free form or in complexes with various cell wall components such as alginic acid (Li et al., 2017). Phlorotannins are primarily derived from phloroglucinol (1,3,5-trihydroxy benzene) units with varying degrees of polymerization and a group of heterogeneous polymeric compounds. In general, phlorotannins are divided into four subclasses based on the mode of linkage: ether (fuhalols and phlorethols), phenyl (fucols), ether and phenyl (fucophlorethols), and dibenzodioxin linkages (eckols) (Yotsu-Yamashita et al., 2013). Various phlorotannin compounds, such as bieckol/dieckol, phlorofucofuroeckol, fucophloroethol, fucodiphloroethol, fucotriphloroethol, and 7-phloroeckol, have been found in brown seaweeds such as Ascophyllum nodosum, Gongolaria usneoides, Fucus spiralis, Gongolaria nudicaulis, Pelvetia canaliculata, Ericaria selaginoides, Fucus vesiculosus, and Saccharina longicruris (Ferreres et al., 2012). Seaweed phlorotannins have eight interconnected rings in their structure, making them powerful free radical scavengers (Rajauria et al., 2017). As a result, phlorotannins have antibacterial, anti-inflammatory, antioxidant, antivirus, antidiabetic and antiallergic properties (Tong et al., 2021) that could be useful for poultry health and growth performance.
Ulvan is a cell wall polysaccharide that constitutes about 9 to 36% DM of green seaweeds (Ulva spp.) (Kidgell et al., 2019). It is primarily made up of sulphate (12.8 – 23%), rhamnose (12.7 – 45%), xylose (2 – 12%), and uronic acids (6.5 – 26.0%) (Yaich et al., 2017). Ulva species have three other cell wall polysaccharides (cellulose, glucuronan and xyloglucan), which together account for up to 45% of their dry weight (Lahaye and Kaeffer, 1997). The ulvan backbone is often composed of α- and β-(1,4)-linked monosaccharides (rhamnose, xylose, uronic acid) with repeating disaccharide units. Aldobiuronic acids (types A and B) are the two primary disaccharide repeating units, while ulvanobioses (type U) are the minor disaccharide aldobioses. Type U ulvanobioses are significantly less prevalent than types A and B (Kidgell et al., 2019). In this regard, ulvan could be used to produce biomaterials (for tissue engineering, wound dressings, excipients, and biofilm prevention), nutraceuticals, and functional foods (Alves et al., 2013; Venkatesan et al., 2015; Cunha and Grenha, 2016).
Laminarins are a type of low-molecular-weight storage β-glucan that is made up of 1,3-β-D-glucan linkage (Rioux et al., 2007). They are made up of (1,3)-β-D-glucopyranose residues with some 6-O-branching in the main chain, as well as some β-(1,6)-intrachain linkages (Kadam et al., 2015). Laminaria hyperborea and Laminaria digitata produce two types of laminarans, the soluble and insoluble forms, depending on solubility in cold water. Two chains, M and G, are generated based on the amount of mannitol present in both laminarans, with mannitol residues occupying the reducing terminal area in M-chains and glucose residues occupying the terminal region in G-chains (Kumar et al., 2021). Environmental factors such as nutritive salts and frond age influence the structure and ratio of the two forms of laminarin (Chizhov et al., 1998). In addition, the composition of laminarin is also affected by other environmental parameters such as water temperature, salinity, waves, sea current, and depth of immersion (Kadam et al., 2015). These factors are also thought to affect laminarin’s biofunctional activities (Rioux et al., 2010), which include antitumor, anticoagulant, anti-inflammatory, anti-apoptotic, and antioxidant activity (Kadam et al., 2015).
Over the last two decades, the poultry industry has experimented with seaweeds as novel dietary additives supplying prebiotics, pigments, polyunsaturated fatty acids, and other bioactive compounds that could be beneficial to growth performance and health of birds (Evans and Critchley, 2014). Table 4 provides a summary of some in vivo outcomes when seaweeds have been included in poultry diets. Michalak and Chojnacka (2015) reported that seaweed bioactive components can improve chicken performance and health while also increasing the quantity and quality of eggs and meat produced. Indeed, Kulshreshtha et al. (2020) stated that one of the main goals of dietary supplements for poultry is to improve feed efficacy for cost-effectiveness and improved poultry health. A study by Kumar (2018) reported that broilers fed a diet supplemented with 40 g/kg Sargassum wightii powder had higher final liveweight (2408 g/bird) compared to birds on the control diet (1364 g/bird). The positive effects of S. wightii powder on feed utilization efficiency and weight gain are attributed to its palatability and high nutrient content as well as its ability to improve digestion and absorption of nutrients in the gut. The positive effects on the broilers could be partly due to the presence of essential amino acids, minerals, sterols, vitamins, fucoidan and long chain fatty acids in S. wightii powder (Kumar, 2018). Similarly, the use of macroalgae S. muticum as a functional feed ingredient (0, 50, 100 and 150 g/kg) in broiler chickens resulted in marginal linear increases in ADG (1.54, 1.93, 1.96, and 1.97 g/bird, respectively) and improvements in feed utilization as measured by FCR (2.17, 0.54, 0.69 and 0.72, respectively) (Erum et al., 2017). In contrast, the inclusion of graded levels (up to 35 g/kg) of green seaweed (Ulva sp.) in Cobb 500 broiler chickens was reported to reduce the overall feed conversion efficiency with an FCR of 0.490 in control birds compared to 0.533 in birds consuming diets containing 35 g/kg seaweed (Matshogo et al., 2020). This was attributed to the presence of non-starch polysaccharides like cellulose and hemicellulose in seaweeds, which would have interfered with the digestion and nutrient absorption processes in chickens. Considering that most seaweeds contain significant quantities of these non-starch polysaccharides, strategies such as the use of extracts or pre-digestion with exogenous fibrolytic enzymes should be explored to optimize the use of seaweed bioactive compounds for sustainable poultry production.
In commercial poultry production systems, birds are bred for quick growth, larger breast weight, and enhanced muscle mass and meat quality, which are important factors for consumers when making meat purchasing decisions (Michalak and Mahrose, 2020). In general, seaweeds have a positive impact on meat quality, which includes a reduction in total fat content (Michalak and Mahrose, 2020). However, Bonos et al. (2017) discovered that adding A. nodosum (5, 10, and 20 g/kg) to the diets of broiler chickens did not affect the content of monounsaturated, polyunsaturated, and total saturated fatty acids in the breast and thigh meat. El-Naga and Megahed (2018) reported that adding brown seaweed (A. nodosum) extract in drinking water (1 mL/L) enhanced breast width and length, dressing color, and reduced body fat in Indian River chicks compared to the control. Similarly, Erum et al. (2017) discovered that incremental dietary levels of S. muticum powder reduced the fat pads in broiler birds when 150 g/kg of the powder was added into the diets, a desirable outcome in terms of carcass quality. Simultaneously, meat color changed from yellowish to reddish as the dose of S. muticum increased up to 150 g/kg, which was an undesirable outcome given that consumers prefer pale to pink raw meat (Fletcher, 2002; Wideman et al., 2016). However, changes in poultry meat color could be influenced by various other factors such as pre-slaughter and slaughtering procedures (Fletcher, 2002). Kumar (2018) recorded improved carcass traits (leg, thigh, breast, and dressing weights) as the level of brown seaweed (S. wightii) increased (0, 10, 20, 30, and 40 g/kg) in broiler diets. Sargassum doses of 10 and 20 g/kg had the greatest supplemental benefit, which was linked to the minerals, vitamins, polyunsaturated fatty acids, essential amino acids, sterols, and polysaccharides found in the seaweed. Contrary to these findings, Cañedo-Castro et al. (2019) reported no significant variations in carcass weights and yield of Arbor Acres broilers given increasing dietary levels of air-dried and ground Ulva rigida (0, 20, 40, and 60 g/kg).
Egg production and quality are important criteria for laying hen producers and have important economic implications. When seaweeds are used as feed supplements, they can improve both the biochemical and physical indicators of egg quality, which are important for egg producers and consumers (Michalak and Mahrose, 2020). In a recent study by Zewei et al. (2019), Japanese quail-fed diets supplemented with sun-dried and ground green (Ulva fasciata) and brown (S. cinereum) seaweeds (15 and 30 g/kg, respectively) produced eggs with lower total lipids (364.00 – 386.67 mg/g) and cholesterol (176.33 – 189.33 mg/g) content in the yolk compared to the control eggs (434.67 mg/g total lipids and 211.00 mg/g total cholesterol). In addition, Wang et al. (2013) observed that dietary inclusion of Enteromorpha prolifera (10, 20 and 30 g/kg) improved the shell thickness of Highland brown eggs, which was likely due to an increase in calcium content of the shell (Choi et al., 2018). The abundance of sulfates in ulvan isolated from Ulva sp. can explain the considerable improvement in eggshell strength of Hy–Line Brown hens (Li et al., 2018). Dietary supplementation (0, 2.5, and 5 g/kg) with the brown seaweed (A. nodosum) improved egg weight, shell weight, and yolk color in Lohmann Lite (70 weeks of age) eggs (Stupart, 2019). Hens in the 2.5 g/kg group had considerably larger eggs (63.82 g) and shell weights (6.34 g) compared to the 5 g/kg (62.04 and 6.13 g, respectively) and control groups (63.11 and 6.31 g, respectively), demonstrating that the lower seaweed inclusion level (2.5 g/kg) improved both the productivity and economic efficiency of egg production (Stupart, 2019). However, while dietary inclusion of 5 g/kg of dried and fermented brown seaweeds (Undaria pinnatifida and Hizikia fusiforme) increased egg production in Hy-Line Brown hens, this had no effect on indicators of egg and eggshell quality.
There is evidence that seaweeds contain compounds with antimicrobial and antiviral capabilities, as well as immunomodulatory effects, which could boost the health and productivity of poultry (Michalak and Mahrose, 2020). Indeed, when broiler chicken diets were supplemented at 1, 2, 3, and 4 g/kg levels with a brown seaweed derivate, polymannuronate, the cecal microbiome was altered resulting in increased lactic and acetic acid concentration in the cecum and improved ADG, FCR, antioxidant capacity, and immune status of the birds (Choi et al., 2014). Sobotik et al. (2018) reported that the dietary addition of A. nodosum (0.5 g/kg of feed) ameliorated the effect of prolonged heat stress without compromising growth performance in broiler chickens. This suggests that A. nodosum can be used to improve bird welfare and maintain productivity in heat-stressed birds. Frasiska et al. (2016) studied serum lipid profiles of ducks (22 weeks old) fed a meal comprising of a multi-enzyme pre-treated Gracilaria sp. waste at 100, 125, and 150 g/kg. They reported that ducks consuming 125 g/kg pre-treated Gracilaria sp. had lower serum triglycerides (80.20 vs. 92.54 mg/dL in control ducks), low-density lipoprotein (62.2 vs. 103.32 mg/dl in control ducks), and cholesterol (108.24 vs. 177.02 in control ducks) and higher high-density lipoprotein (52.57 mg/dL compared to 33.92 mmol/L of the control ducks) levels. This outcome was attributed to the biological effect of Gracilaria sp. fiber, which acts as an anticoagulant, antihyperlipidemic, and anti-cholesterol agent (Zhang et al., 2004). Moreover, Cañedo-Castro et al. (2019) reported that the inclusion of U. rigida (up to 60 g/kg) as a prebiotic feed additive in broiler diets lowered the serum total cholesterol (126.7 mg/dL) and triglyceride (78 mg/dL) levels compared to the control (140.1 and 93.3 mg/dL, respectively). With regards to intestinal histological changes induced by dietary seaweeds; villi width, height, and length were enhanced in birds fed 20, 40, and 60 g/kg of U. rigida-containing diets compared to those on the control diet. This shows that both the feed consumption and growth were positively correlated with an increase in villi size. The increase in the width and height of the villi in chicken intestines is associated with an increase in the digestion and absorption function because of the resultant larger absorption area (Awad et al., 2008). However, some of the discordance observed in results reported from feeding trials with seaweeds could be attributed to variations in factors such as the amount of seaweed supplemented (0.5 g/kg being the minimum and 150 g/kg being the maximum amount reported), particle size (ranging between 0.4 and 2 mm), purity of seaweed, drying methods (air, sun or oven drying) and subsequent processing, and seaweed species (Kulshreshtha et al., 2020).
Although seaweeds are a ubiquitous natural biomass of the oceans, their accessibility remains a major challenge for poultry producers who are based many miles away from the shorelines. The rising costs of fuel around the world would also render their bulk transportation very expensive and further increase production costs. Wet seaweeds (70 – 90% moisture) that are washed to the shorelines have a very short life span (Maia et al., 2016), and if not collected, they decay and emit hydrogen sulfide to the environment, posing a risk to human and animal health (Smetacek and Zingone, 2013). Reliance on conventionally farmed seaweeds would also be a challenge for resource-poor farmers and would negate the goal of building profitable and sustainable poultry production systems. Moreover, seaweed farming faces challenges of disease outbreaks with most of the commercially important species like Saccharina, Laminaria, Kappaphycus, Pyropia, and Eucheuma affected by many diseases that are caused by endosymbionts and epibionts, resulting in serious economic losses (Wang et al., 2019). Gachon et al. (2010) observed that the occurrence of diseases is directly linked to intensive large-scale seaweed production, further confirming that reliance on cultivated seaweed would not be sustainable for poultry intensification. Ferdouse et al. (2018) reported that seaweed yields from aquaculture are significantly higher compared to wild harvesting. However, the harvesting of cultivated seaweeds requires proper storage and technology. On the other hand, wild seaweed harvesting can result in several ecological and social consequences if not properly managed (Ferdouse et al., 2018). MacMonagail et al. (2017) reported that over 800 000 t of seaweeds are harvested from natural beds every year with minimal consideration of their sustainability. Thus, over-exploitation associated with increased harvesting pressures to meet commercial demand has led to deterioration of seaweed beds (MacMonagail et al., 2017) and may consequently interfere with its supply chain.
Although several studies have reported positive results with regards to the use of various seaweeds in poultry feeds (Øverland et al., 2019), some reports indicate undesirable outcomes such as reduced feed conversion efficiency in broilers (Matshogo et al., 2020) and a lack of improvement on chicken meat shelf-life indicators (Nhlane et al., 2021). This is because the nutritional composition of seaweeds is highly variable between and within species, with inorganic elements ranging from 11 to 55% on a DM basis (Biancarosa et al., 2017; Øverland et al., 2019). Seaweeds also contain high dietary fibre levels (25 – 75% DM), which are dominated by many non-starch polysaccharides. According to Choudhary et al. (2021) seaweed polysaccharides form an integral part of their cell wall matrix that assist them to survive the harsh environmental sea conditions. However, the rigidity of the cell wall matrix in seaweeds suppresses the release and bioavailability of bioactive substances (Wells et al., 2017) and exhibit a hindering effect that reduces seaweed nutrient digestibility (Tenorio et al., 2018). Ruocco et al. (2016) classified seaweed polysaccharides into structural cell wall, intracellular mucilage, and storage polysaccharides, with the structural polysaccharides reported to be a major challenge for poultry birds. This often requires costly cell wall disruption techniques, thus limiting the usage of seaweed by resource-poor farmers. Indeed, the presence of non-starch polysaccharides (chitosan, fucoidan, cellulose, hemicellulose, and alginate) in the cell wall matrix of seaweed could limit their utility in poultry feeds, especially when included at higher levels in diets. Although the use of seaweed extracts can potentially eliminate the need for pre-treatment methods, Lam (2007) reported that the extraction (except for water extraction) of bioactive compounds from the seaweeds is labor intensive, time consuming, and expensive, and may only be available to resource-rich poultry producers. These extraction processes also require highly skilled personnel to ensure that labile bio-compounds such as carotenoids and polyphenols are not destroyed during the process, especially when thermal treatments are applied (Ummat et al., 2021).
The fishy odor of seaweeds, which is caused by the presence of 1-octene-3-ol, heptanal, hexanal, nonanal, and hexanol, can affect the aroma, taste and, ultimately, the overall acceptance of poultry products from birds reared on seaweed-containing diets (Peinado et al., 2014). This would necessitate raising awareness about the benefits associated with the consumption of poultry products from birds that were previously reared on seaweed-based diets. Gopi et al. (2020) also reported that seaweeds accumulate high concentrations of minerals and heavy metals (Pb, Cd, AS, and Hg) due to the increasing marine pollution, which in turn could affect the utilization of seaweed by poultry birds. Makkar et al. (2016) reported that these heavy metals could be detrimental to animal and human health. Consequently, there has been research attempts to reduce excess minerals and detoxify heavy metals using microorganisms (e.g., Lactobacillus) that transform heavy metals into less toxic forms (Abdel-Megeed, 2021). The accumulation of heavy metals by seaweeds has necessitated strict cultivation practices in compliance with coastal management policy (Froehlich et al., 2019). The use of heavy metal-rich seaweeds for poultry production, especially at higher dietary inclusion levels, would require pre-processing strategies that reduce the concentration of heavy metals and other antinutrients. Lastly, seaweeds are not a familiar bioresource for many poultry producers in Africa, especially those who live far away from coastal areas.
Apart from the logistical problems affecting the accessibility and utilization of seaweeds as functional ingredients in poultry feeds, their complex cellular structure remains a major problem for efficient utilization in poultry diets. To resolve this challenge, bioactive compounds that are bound in the seaweed cell wall matrix can be extracted and used in poultry diets. Serive et al. (2012) proposed a biorefinery approach to ensure sustainable exploitation of seaweeds. Indeed, green solvents (such as water, ionic liquids, deep eutectic solvents, and subcritical and supercritical fluids) and novel extraction technologies have been developed to sustainably produce safe and high-quality seaweed extracts and eliminate the use of unsafe petroleum derived solvents (Gomez et al., 2020). These novel extraction techniques can be categorized as physical, biological, chemical, or combinations. They include techniques that have been widely used to extract a wide range of bioactive agents such as ultrasound-assisted extraction, microwave-assisted extraction, enzyme-assisted extraction, pressurized liquid extraction and supercritical fluid extraction (Kadam et al., 2015). In addition to their extraction efficiency, these technologies also reduce the extraction time, eliminate the use of toxic chemical solvents, and, as a result, enhance the quality and yield of the extract (Kadam et al., 2015). The seaweeds can be dried, milled, extruded, or mechanical pressed prior to the extraction process that may include heating, pressurized liquid extraction, microwave-assisted extraction, ultrasonication, and supercritical fluid extraction. Moreover, chemical extraction methods that involve the use of organic and inorganic solvents can be employed to further extract seaweed bioactive substances, but it should be noted that the efficiency of the extraction would depend on the polarity, viscosity, surface tension, dielectric constant and dipole moment of the solvent used (Belwal et al., 2018). This will in turn affect the yield and biological activity of the extract, thus it is imperative to choose an extraction technique and an appropriate solvent based on the bioactive substance of interest and their envisioned end application. Seaweed cell wall matrix can also be modified using compression puffing, which is a physical pre-treatment technique that simultaneously employs heat and pressure, resulting in the alteration of physicochemical properties. Huang et al. (2016) reported that compression puffing of brown seaweed (S. glaucescens) improved the extraction of sulphated polysaccharide, fucoidan. The disruption of the cell wall occurs through the process of electroporation, where pores are formed in cell membranes leading to increased permeability (Bryant and Wolfe, 1987). Vorobiev and Lebovka (2015) added that high permeability causes diffusion of the solvent into the cell membranes, which enhances the extraction of the target compound. Bead milling techniques have also been shown to improve protein extraction from seaweed (Ulva and Gracilaria spp.) compared to other extraction methods alkaline and ultrasound treatment (Kazir et al., 2019). Optimization of the extraction process is critical, not only to reduce cost of extraction, but also to ensure that the target bioactive compounds are efficiently extracted without significant alterations to the biological activities when eventually incorporated into poultry diets.
Where poultry producers lack the resources and capacity to extract bioactive compounds, the seaweed meal (dried and milled into a powder) is often incorporated into poultry diets. While this is a less costly and more practical approach, the bioavailability of nutrients and bioactive compounds in seaweed meal to birds can be compromised by its other constituents such as fibre and ash. To improve the utilization of seaweed meals in poultry diets, several strategies such as washing, fermentation, ensiling, and pre-treatments with exogenous enzymes can be employed.
Wijesinghe and Jeon (2012) noted that cell wall matrix of seaweed is more difficult to hydrolyze, which suggests a need to employ multi-enzyme polysaccharidases to target the various long-chain polysaccharides present in seaweeds. However, Matshogo et al. (2021) observed a lack of improvement on feed utilization, physiological parameters, and meat quality in broiler chickens reared on diets containing seaweed (Ulva spp.) that was pre-treated with a combination of protease and fibrolytic enzymes. The lack of dietary differences could have been due to many reasons such as limited hydrolysis time, pH of the enzyme-substrate mixture, or low proportion of enzyme in relation to substrate/fibre levels of the diets. Stokvis et al. (2022) reported a reduction in nutrient digestibility in broilers fed with 2.5 and 5% seaweed (Ulva laetevirens) treated with proteolytic enzyme. Contrary to these findings, Trivedi et al. (2015) observed that the treatment of seaweed (Ulva fasciata) with cellulase (10 U/g) derived from the marine fungus, Cladosporium sphaerospermum for 24 h at 40°C and pH 4 successfully hydrolyzed crystalline cellulose microfibrils to produce the maximum yield of sugar (112 ± 10 mg/g dry weight). Nonetheless, there are still unacceptable levels of contradictions with regards to the effects of exogenous enzyme pre-treatments of seaweed on poultry performance, especially considering that this strategy is rather costly.
Fermentation, in a solid or liquid state, is another method that can be used to enhance the aroma, texture, taste, flavour, and the shelf life of seaweeds through microbial enzymatic activities and the resultant secondary metabolites (Admassie, 2018; Reboleira et al., 2021). The fermentation of seaweed (Saccharina japonica) with Aspergillus oryzae and Monascus spp. has been reported to reduce the concentration of iodine and heavy metal content (Bruhn et al., 2019) and increase its antioxidant and anti-inflammatory activities (Lin et al., 2016). This suggests that the use of fermented seaweed in poultry diets could improve growth parameters and the keeping quality or shelf life of the meat. Solid-state fermentation of seaweeds has been reported to increase nutrient utilization (Rafiquzzaman et al., 2015), which could be through the unique mycelial growth pattern of molds that allows them to penetrate the cell wall matrix and release nutrients and bioactive compounds. Other studies have indicated that fermentation can hydrolyze higher molecular weight proteins into lower molecular weight oligopeptides and break down peptide bonds to release amino acids, thereby increasing the digestibility of the protein (Ketnawa and Ogawa, 2019; Mohapatra et al., 2019). Fermentation of seaweeds can also be conducted to reduce the compounds that are responsible for off-flavors and thus enhance compounds that are beneficial for aroma and taste through a series of oxidation, degradation, condensation, and polymerization reactions (Feng et al., 2021). This could consequently improve palatability of the diets leading to higher voluntary feed intake. Fermentation, through microbial hydrolysis reactions, could also liberate phenolic compounds (phlorotannins) that are usually bound to carbohydrates (Hur et al., 2014) and thus increase their antioxidant and antimicrobial activities (Campbell et al., 2020) leading to improved poultry product quality. The presence of phenolic compounds like phlorotannins can also suppress microbial degradation and ultimately hinder fermentation processes creating unfavorable fermentation outcomes. However, it is also important to note that phlorotannins can also be beneficial compounds due to their antioxidant properties, thus any tannin-amelioration strategy to improve substrate fermentation e.g., the use of tannin-binding agents, requires careful consideration.
As already mentioned, fresh seaweeds deteriorate rapidly if not dried. Thus, pre-treatment strategies like ensiling have been commonly used to preserve many forages, especially for ruminants. This could be the reason why there is currently limited knowledge on the characteristics and feed value of seaweed silages (Campbell et al., 2020) in poultry birds. The ensiling of seaweed is a potential strategy to enhance its preservation and consequently promote all-year-round supply of the biomass (Bach et al., 2014). It can also eliminate the need to employ different drying methods and the labor costs associated with drying. Further, it can reduce the challenges that come with sun drying, especially in areas where climatic conditions are unfavorable (Yen et al., 2022). Indeed, Campbell et al. (2020) stated that ensiling is an effective method to preserve seaweeds for animal feed applications. This occurs through anaerobic fermentation where lactate-producing bacteria convert sugars into lactic acid, whose accumulation reduces the pH of ensiled material thereby preserving it (Yen et al., 2022). This has been reported to prevent nutrient losses and microbial (Enterobacteria and Clostridia) spoilage of seaweed (Herrman et al., 2015). However, Herrman et al. (2015) reported fermentation challenges due to high-buffering capacity, high levels of long-chain polysaccharides (resistant to microbial degradation), and poor natural lactic acid-producing microflora in fresh seaweeds. Despite the obvious benefits that ensiling could bestow on the keeping quality and nutritional value of seaweeds, studies where seaweed silage has been evaluated in poultry nutrition are very limited. A recent study by Stokvis et al. (2021) found that ensiling Saccharina latissima, U. lactuca, and L. digitata increased crude protein content, but reduced the ash content and in vitro organic matter digestibility of the fermented substrate. When ensiled seaweeds were included in broiler diets, no improvement in feed utilization efficiency and final body weight was observed, possibly due to poor organic matter digestibility. The ensiling of seaweeds for poultry nutrition requires further experimentation to optimize the process and nutritional value of the product. In addition, there is very limited data on the impact of including ensiled seaweeds in poultry diets on meat quality and bird health. Indeed, Campbell et al. (2020) proposed that additional research should be carried out on microbiome dynamics during ensiling as well as on the effects of inoculants on quality of seaweed silage.
The utility of seaweeds can also be improved using cost-effective treatments such as washing with freshwater and various aqueous solutions to reduce high levels of inorganic elements (15% DM salt and heavy metals) and other impurities that are intrinsically bound to seaweeds (Milledge and Harvey, 2016). However, there are very few studies that have evaluated the efficacy of these methods in improving the feed value of seaweed for poultry (Kawai and Murata, 2016). High levels of ash in seaweeds can also be reduced using aqueous alkali carbonate solution (Tippmer et al., 1978) or by washing using tap water (Milledge et al., 2018). The effectiveness of these simple strategies needs to be evaluated in feeding trials with poultry birds.
This review has described the incredible array of nutritive and bioactive compounds that are present in seaweeds and explored how they can be exploited as part of sustainable poultry production systems of the future. There is amble evidence that including seaweeds in poultry diets enhances feed utilization efficiency, growth performance, and meat stability and quality. However, contradicting results on the effect of dietary seaweeds on growth performance and carcass and meat quality traits were unearthed and these require careful consideration and further investigations. In particular, the optimum level of seaweed meal inclusion in diets tends to vary with seaweed and poultry species but in most cases does not exceed 15% (w/w) due to antinutritional effects of fibre and some phenolics. While there are several challenges that face seaweed exploitation as a dietary component in sustainable poultry production systems, this paper reviewed several possible strategies such as enzyme supplementation, tannin amelioration, ensiling, solid-state fermentation, and use of extracts that can be used to overcome them. These strategies should allow for increased use of seaweed bioactive compounds to create functional poultry diets and thus create a “symphony of good things” in the form of economically, socially, and environmentally sustainable poultry production systems of the future. The use of seaweed extracts, instead of seaweed meal, appears more promising in terms of potency and uniform outcomes despite the associated high cost. Further research, especially to optimize extraction of bioactive compounds, is needed to make seaweed a significant dietary component in commercial poultry production. This approach will also ensure uniform outcomes in terms of bird health, growth performance, and meat quality.
VM and CM conceptualized the review. TM, GM, CM, and VM were equally involved in the write-up, editing, and formatting of the review paper. All authors contributed to the article and approved the submitted version.
Funds for open access publication were received from University of Mpumalanga.
The authors declare that the research was conducted in the absence of any commercial or financial relationships that could be construed as a potential conflict of interest.
All claims expressed in this article are solely those of the authors and do not necessarily represent those of their affiliated organizations, or those of the publisher, the editors and the reviewers. Any product that may be evaluated in this article, or claim that may be made by its manufacturer, is not guaranteed or endorsed by the publisher.
Øverland M., Mydland L. T., Skrede A. (2019). Marine macroalgae as sources of protein and bioactive compounds in feed for monogastric animals. J. Sci. Food Agric. 99, 13–24. doi: 10.1002/jsfa.9143
Abdel-Megeed R. M. (2021). Probiotics: A promising generation of heavy metal detoxification. Biol. Trace elem. Res 199 (6), 2406–2413. doi: 10.1007/s12011-020-02350-1
Abudabos A. M., Okab A. B., Aljumaah R., Samara E., Abdoun K. A., Al-Haidary A. A. (2013). Nutritional value of green seaweed (Ulva lactuca) for broiler chickens. Ital. J. Anim. Sci. 12, 28. doi: 10.4081/ijas.2013.e28
Admassie M. (2018). A review on food fermentation and the biotechnology of lactic acid bacteria. World J. Food Sci. Technol. 2 (1), 19–24. doi: 10.11648/j.wjfst.20180201.13
Alves A., Sousa R. A., Reis R. L. (2013). A practical perspective on ulvan extracted from green algae. J. Appl. Psychol. 25 (2), 407–424. doi: 10.1007/s10811-012-9875-4
Amato H. K., Wong N. M., Pelc C., Taylor K., Price L. B., Altabet M., et al. (2020). Effects of concentrated poultry operations and cropland manure application on antibiotic resistant Escherichia coli and nutrient pollution in Chesapeake bay watersheds. Sci. Total Environ. 735, 139401. doi: 10.1016/j.scitotenv.2020.139401
Aminina N. M., Karaulova E. P., Vishnevskaya T. I., Yakush E. V., Kim Y. K., Name K. H., et al. (2020). Characteristics of polyphenolic content in brown algae of the pacific coast of Russia. Molecules 25, 1e13. doi: 10.3390/molecules25173909
Anderson M. J., Blanton J. R. Jr., Gleghorn J., Kim S. W., Johnson J. W. (2006). Ascophyllum nodosum supplementation strategies that improve overall carcass merit of implanted English crossbred cattle. Asian-aust. J. Anim. Sci. 19 (10), 1514–1518. doi: 10.5713/ajas.2006.1514
Athukorala Y., Jung W. K., Vasanthan T., Jeon Y. J. (2006). An anticoagulative polysaccharide from an enzymatic hydrolysate of Ecklonia cava. Carbohydr. Polym. 66, 184–191. doi: 10.1016/j.carbpol.2006.03.002
Awad W. A., Ghareeb K., Nitch S., Pasteiner S., Raheem S. A., Bohm J. (2008). Effect of dietary inclusion of probiotic, prebiotic and symbiotic on intestinal glucose absorption on broiler chickens. Int. J. Poult. Sci. 7 (7), 688–691. doi: 10.14202/vetworld.2020.2765-2771
Bach Q. V., Sillero M. V., Tran K. Q., Skjermo J. (2014). Fast hydrothermal liquefaction of a Norwegian macro-alga: Screening tests. Algal Res. 6, 271–276. doi: 10.1016/j.algal.2014.05.009
Badmus U. O., Taggart M. A., Boyd K. G. (2019). The effect of different drying methods on certain nutritionally important chemical constituents in edible brown seaweeds. J. Appl. Phycol. 31 (6), 3883–3897. doi: 10.1007/s10811-019-01846-1
Bai J., Wang R., Yan L., Feng J. (2019). Co-Supplementation of dietary seaweed powder and antibacterial peptides improves broiler growth performance and immune function. Braz. J. Poult. Sci. 21, 1–9. doi: 10.1590/1806-9061-2018-0826
Balasubramanian B., Shanmugam S., Park S., Recharla N., Koo J. S., Andretta I., et al. (2019). Supplemental impact of marine red seaweed (Halymenia palmata) on the growth performance, total tract nutrient digestibility, blood profiles, intestine histomorphology, meat quality, fecal gas emission, and microbial counts in broilers. Animals 11, 1244. doi: 10.3390/ani11051244
Balina K., Romagnoli F., Blumberga D. (2017). Seaweed biorefinery concept for sustainable use of marine resources. Energy Proc. 128, 504–511. doi: 10.1016/j.egypro.2017.09.067
Belanche A., Jones E., Parveen I., Newbold C. A. (2016). Cetagenomics approach to evaluate the impact of dietary supplementation with Ascophyllum nodosum or Laminaria digitata on rumen function in rusitec fermenters. Front. Microbiol. 10. doi: 10.3389/fmicb.2016.00299
Belwal T., Ezzat S. M., Rastrelli L., Bhatt I., Daglia M., Baldi A., et al. (2018). A critical analysis of extraction techniques used for botanicals: Trends, priorities, industrial uses and optimization strategies. Trends Anal. Chem. 100, 10082–10102. doi: 10.1016/J.TRAC.2017.12.018
Bhagyaraj I., Kunchithapatham V. R. (2016). Diversity and distribution of seaweeds in the shores and water lagoons of chennai and rameshwaram coastal areas, south-Eastern coast of India. Biodivers. J. 7 (4), 923–934.
Biancarosa I., Espe M., Bruckner C. G., Heesch S., Liland N., Waagbø R. (2017). Amino acid composition, protein content, and nitrogen-to-protein conversion factors of 21 seaweed species from Norwegian waters. J. Appl. Phycol. 29 (2), 1001–1009. doi: 10.1007/s10811-016-0984-3
Bonos E., Kargopoulos A., Nikolakakis I., Florou-Paneri P., Christaki E. (2017). The seaweed Ascophyllum nodosum as a potential functional ingredient in chicken nutrition. J. Oceanogr. Mar. Res. 4 (1), 140. doi: 10.4172/2572-3103.1000140
Borowitzka M. A., Borowitzka L. J. (1987). “Vitamins and fine chemicals from micro-algae,” in Micro-algal biotechnology. Eds. Borowitzka M. A., Borowitzka L.J. (New York: Cambridge University Press).
Bruhn A., Brynning G., Johansen A., Lindegaard M. S., Sveigaard H. H., Aarup B., et al. (2019). ). fermentation of sugar kelp (Saccharina latissima) effects on sensory properties, and content of minerals and metals. J. Appl. Phycol 31 (5), 3175–3187. doi: 10.1007/s10811-019-01827-4
Bryant G., Wolfe J. (1987). Electromechanical stresses produced in the plasma membranes of suspended cells by applied electric fields. J. Membr. Biol. 96 (2), 129–139. doi: 10.1007/BF01869239
Campbell M., Ortuño J., Ford L., Davies D. R., Koidis A., Walsh P. J. (2020). The effect of ensiling on the nutritional composition and fermentation characteristics of brown seaweeds as a ruminant feed ingredient. Animals 10 (6), 1019. doi: 10.3390/ani10061019
Cañedo-Castro B., Piñón-Gimate A., Carrillo S., Ramos D., Casas-Valdez M. (2019). Prebiotic effect of Ulva rigida meal on the intestinal integrity and serum cholesterol and triglyceride content in broilers. J. Appl. Phycol. 31, 3265–3273. doi: 10.1007/s10811-019-01785-x
Carrillo S., Castro M. I., Perez-gil F., Rosales E., Manzano R. E. (1992). The seaweed (Sargassum sinicola setchel & Gardner) as an alternative for animal feeding. Cuban J. Agric. Sci. 26, 177–181.
Cassani L., Gomez-Zavaglia A., Jimenez-Lopez C., Lourenço-Lopes C., Prieto M. A., Simal-Gandara J. (2020). Seaweed-based natural ingredients: Stability of phlorotannins during extraction, storage, passage through the gastrointestinal tract and potential incorporation into functional foods. Food Res. Int. 137, 109676. doi: 10.1016/j.foodres.2020.109676
Castanheira E. G., Freire F. (2013). Greenhouse gas assessment of soybean production: Implications of land use change and different cultivation systems. J. Clean. Prod. 54, 49–60. doi: 10.1016/j.jclepro.2013.05.026
Catarino M. D., Silva A., Cruz M. T., Mateus N., Silva A. M. S., Cardoso S. M. (2020). Phlorotannins from Fucus vesiculosus: Modulation of inflammatory response by blocking NF-κB signaling pathway. Int. J. Mol. Sci. 21, 6897. doi: 10.3390/ijms21186897
Cervantes H. M. (2015). Antibiotic-free poultry production: Is it sustainable? J. Appl. Poult. Res 24, 91–97. doi: 10.3382/japr/pfv006
Chan J. C. C., Cheung P. C. K., Ang P. O. (1997). Comparative studies on the effect of three drying methods on the nutritional composition of seaweed Sargassum hemiphyllum (turn.) c. Ag. J. Agric. Food Chem. 45 (8), 3056–3059. doi: 10.1021/jf9701749
Chizhov A. O., Dell A., Morris H. R., Reason A. J., Haslam S. M., McDowell R. A., et al. (1998). Structural analysis of laminarans by MALDI and FAB mass spectrometry. Carbohydr. Res 310, 203–210. doi: 10.1016/S0008-6215(98)00177-3
Choi Y., Lee E. C., Na Y., Lee S. R. (2018). Effects of dietary supplementation with fermented and non-fermented brown algae by-products on laying performance, egg quality, and blood profile in laying hens. Asian-Australasian J. Anim. Sci. 31, 1654–1659. doi: 10.5713/ajas.17.0921
Choi Y. J., Lee S. R., Oh J. W. (2014). Effects of dietary fermented seaweed and seaweed fusiforme on growth performance, carcass parameters and immunoglobulin concentration in broiler chicks. Asian-Australas. J. Anim. Sci. 27, 862–870. doi: 10.5713/ajas.2014.14015
Choudhary B., Chauhan O. P., Mishra A. (2021). Edible seaweeds: a potential novel source of bioactive metabolites and nutraceuticals with human health benefits. Front. Mar. Sci. 8. doi: 10.3389/fmars.2021.7400
Costa M. M., Pio L. B., Bule P., Cardoso V. A., Duarte M., Alfaia C. M., et al. (2022). Recalcitrant cell wall of Ulva lactuca seaweed is degraded by a single ulvan lyase from family 25 of polysaccharide lyases. Anim. Nutr. 9, 184–192. doi: 10.1016/j.aninu.2022.01.004
Cunha L., Grenha A. (2016). Sulfated seaweed polysaccharides as multifunctional materials in drug delivery applications. Mar. Drugs 4(3), 42. doi: 10.3390/md14030042
Duarte C. M., Wu J., Xiao X., Bruhn A., Krause-Jensen D. (2017). Can seaweed farming play a role in climate change mitigation and adaptation? Front. Mar. Sci. 4. doi: 10.3389/fmars.2017.00100
Dutta D., Chaudhuri U. R., Chakraborty R. (2005). Structure, health benefits, antioxidant property and processing and storage of carotenoids. Afr. J. Food Agric. Nutr. Dev. 4 (13), 1510–1520. doi: 10.4314/ajfand.v4i13.71773
El-Deek A. A., Brikaa M. A. (2009). Nutritional and biological evaluation of marine seaweed as a feedstuff and as a pellet binder in poultry diet. Int. J. Poult. Sci 8, 875–881. doi: 10.3923/ijps.2009.875.881
El-Naga M. A., Megahed M. (2018). Impact of brown algae supplementation in drinking water on growth performance and intestine histological changes of broiler chicks. Egypt. J. Nutr. Feed. 21, 495–507. doi: 10.21608/EJNF.2018.75603
Engberg R. M., Hedemann M. S., Leser T. D., Jensen B. B. (2000). Effect of zinc bacitracin and salinomycin on intestinal microflora and performance of broilers. Poult Sci 79, 1311–1319. doi: 10.1093/ps/79.9.1311
Erum T., Frias G. G., Cocal C. J. (2017). Sargassum muticum as feed substitute for broiler. Asia pacific. J. Educ. Arts Sci. 4, 6–9.
Evans F. D., Critchley A. T. (2014). Seaweeds for animal production use. . J. Appl. Phycol 26, 891–899. doi: 10.1007/s10811-013-0162-9
FAO (2013). Poultry development review. (Rome, Italy: Food and Agriculture Organization of the United Nation).
FAO (2020). The state of world fisheries and aquaculture 2020. Sustainability in action. (Rome, Italy: Food and Agriculture Organization of the United Nations).
FAO (2021). Fishery and aquaculture statistics. global production statistics 1950–2019. (Rome, Italy: Food and Agriculture Organization of the United Nations).
FAOSTAT (2016) FAO statistical database. (Rome, Italy: Food and Agriculture Organization of the United Nations). Available at: http://faostat.fao.org (Accessed July 15, 2016).
FAOSTAT (2020) FAO online statistical database. (Rome, Italy: Food and Agriculture Organization of the United Nations). Available at: http://faostat.fao.org (Accessed March 27, 2022).
Farley R. H. (2012) Chemistry and the aquarium: iodine in marine aquaria. Available at: https://www.austinreefclub.com/FAQ/reef-chemistry/water-chemistry-101/iodine-r31/ (Accessed April 2, 2020).
Feng C., Ye G., Jiang Q., Zheng Y., Chen G., Wu J., et al. (2021). The contribution of ocean-based solutions to carbon reduction in China. Sci. Total Environ. 797, 149168. doi: 10.1016/j.scitotenv.2021.149168
Ferdouse F., Holdt S. L., Smith R., Murúa P., Yang Z. (2018). The global status of seaweed production, trade and utilization Vol. 124 (Rome: Globefish Research Programme), I.
Ferreres F., Lopes G., Gil-Izquierdo A., Andrade P. B., Sousa C., Mouga T., et al. (2012). Phlorotannin extracts from fucales characterized by HPLC-DAD-ESI-MSn: Approaches to hyaluronidase inhibitory capacity and antioxidant properties. Mar. Drugs 10 (12), 2766–2781. doi: 10.3390/md10122766
Fletcher D. L. (2002). Poultry meat quality. Worlds Poult. Sci. J. 58, 131–145. doi: 10.1079/WPS20020013
Frasiska N., Suprijatna E., Susanti S. (2016). Effect of diet containing gracilaria sp. waste and multi-enzyme additives on blood lipid profile of local duck. Anim Prod 18, 22. doi: 10.20884/1.anprod.2016.18.1.534
Froehlich H. E., Afflerbach J. C., Frazier M., Halpern B. S. (2019). Blue growth potential to mitigate climate change through seaweed offsetting. Curr. Biol. 29 (18), 3087–3093. doi: 10.1016/j.cub.2019.07.041
Gachon C. M., Sime-Ngando T., Strittmatter M., Chambouvet A., Kim G. H. (2010). Algal diseases: spotlight on a black box. Trends Plant Sci. 15, 633–640. doi: 10.1016/j.tplants.2010.08.005
Ganesan A. R., Tiwari U., Rajauria G. (2019). Seaweed nutraceuticals and their therapeutic role in disease prevention. Food Sci. Hum. Wellness. 8 (3), 252–263. doi: 10.1016/j.fshw.2019.08.001
Garcia J. S., Palacios V., Roldán A. (2016). Nutritional potential of four seaweed species collected in the barbate estuary (Gulf of cadiz, Spain). J. Nutr. Food Sci. 6 (3), 1–7. doi: 10.4172/2155-9600.1000505
Gomez L. P., Alvarez C., Zhao M., Tiwari U., Curtin J., Garcia-Vaquero M. (2020). Innovative processing strategies and technologies to obtain hydrocolloids from macroalgae for food applications. Carbohydr. Polym. 248, 116784. doi: 10.1016/j.carbpol.2020.116784
Gopi S., Arulkumar A., Ganeshkumar A., Rajaram R., Miranda J. M., Paramasivam S. (2020). Heavy metals accumulation in seagrasses collected from palk bay, south-eastern India. Mar. pollut. Bull. 157, 111305. doi: 10.1016/j.marpolbul.2020.111305
Hafez H. M., Attia Y. A. (2020). Challenges to the poultry industry: Current perspectives and strategic future after the COVID-19 outbreak. Front. Vet. Sci. 7. doi: 10.3389/fvets.2020.00516
Hamdy A. E. A., Dawes C. J. (1988). Proximate constituents and lipid chemistry in two species of Sargassum from the west coast of Florida. Botanica Marina 31, 79–81. doi: 10.1515/botm.1988.31.1.79
Harb T. B., Chow F. (2022). An overview of beach-cast seaweeds: Potential and opportunities for the valorization of underused waste biomass. Algal Res. 62, 102643. doi: 10.1016/j.algal.2022.102643
Hayashi L., Hurtado A. Q., Msuya F. E., Bleicher-Lhonneur G., Critchley A. T. (2010). “A review of kappaphycus farming: Prospects and constraints,” in Seaweeds and their role in globally changing environments, vol. 15 . Eds. Seckbach J., Einav R., Israel A. (Portsmouth Polytechnic, UK: Springer), pp. 251–283. doi: 10.1007/978-90-481-8569-6_15
Henriquez V., Escobar C., Galarza J., Gimpel J. (2016). Carotenoids in microalgae. Subcell. Biochem. 79, 219–237. doi: 10.1007/978-3-319-39126-7_8
Herrmann C., FitzGerald J., O’Shea R., Xia A., O’Kiely P., Murphy J. D. (2015). Ensiling of seaweed for a seaweed biofuel industry. Bioresour. Technol. 196, 301–313. doi: 10.1016/j.biortech.2015.07.098
Hind Z., Rabah A., Christelle B., Hacene B., Yves B. (2014). Chemical and biological evaluation of the nutritive value of Algerian green seaweed Ulva lactuca using in vitro gas production technique for ruminant animals. Int. J. Adv. Res. 2, 916e25.
Holdt S. L., Kraan S. (2011). Bioactive compounds in seaweed: functional food applications and legislation. J. Appl. Phycol. 23, 543e97. doi: 10.1007/s10811-010-9632-5
Hu Q. (2004). “Environmental effects on cell composition,” in Handbook of microalgal culture: biotechnology and applied phycology. Ed. Richmond A. (Oxford: Blackwell Publishing Ltd), 114–122. doi: 10.1002/9781118567166.ch7
Huang C. Y., Wu S. J., Yang W. N., Kuan A. W., Chen C. Y. (2016). Antioxidant activities of crude extracts of fucoidan extracted from Sargassum glaucescens by a compressional-puffing-hydrothermal extraction process. Food Chem. 197, 1121–1129. doi: 10.1016/j.foodchem.2015.11.100
Hur S. J., Lee S. Y., Kim Y. C., Choi I., Kim G. B. (2014). Effect of fermentation on the antioxidant activity in plant-based foods. Food Chem. 160, 346–356. doi: 10.1016/j.foodchem.2014.03.112
Hyndes G. A., Berdan E., Duarte C., Dugan J. E., Emery K. A., Hambäck P. A., et al. (2021). Flotsam and jetsam: a global review of the role of inputs of marine organic matter in sandy beach ecosystems. Ecoevorxiv Preprints. doi: 10.32942/osf.io/68as7 (Submitted: July 05, 2021; Last Editted July 05, 2021).
Jeni R. E., Dittoe D. K., Olson E. G., Lourenco J., Seidel D. S., Ricke S. C., et al. (2021). An overview of health challenges in alternative poultry production systems. Poult. Sci. 100, 101173. doi: 10.1016/j.psj.2021.101173
Kadam S. U., Álvarez C., Tiwari B. K., O’Donnell C. P. (2015). Extraction of biomolecules from seaweeds. In Seaweed sustainability Acad. Press, pp. 243–269. doi: 10.1016/B978-0-12-418697-2.00009-X
Kadam S. U., Tiwari B. K., O'Donnell C. P. (2015). Extraction, structure and biofunctional activities of laminarin from brown algae. Int. J. Food Sci. 50 (1), 24–31. doi: 10.1111/ijfs.1269
Kambey C. S. B., Campbell I., Sondak C. F. A., Nor A. R. M., Lim P. E., Cottier Cook E. J. (2020). An analysis of the current status and future of biosecurity frameworks for the Indonesian seaweed industry. J. Appl. Phycol. 32, 2147–2160. doi: 10.1007/s10811-019-02020-3
Karcher D. M., Mench J. A. (2018). “Overview of commercial poultry production systems and their main welfare challenges,” in Advances in poultry welfare. Ed. Mench J. A. (Cambridge, UK: Woodhead Publishing Series in Food Science, Technology and Nutrition), 3 –25.
Kawai S., Murata K. (2016). Biofuel production based on carbohydrates from both brown and red macroalgae: Recent developments in key biotechnologies. Int. J. Mol. Sci. 17, 145. doi: 10.3390/ijms17020145
Kazir M., Abuhassira Y., Robin A., Nahor O., Luo J., Israel A. (2019). Extraction of proteins from two marine macroalgae, Ulva sp. and Gracilaria sp., for food application, and evaluating digestibility, amino acid composition and antioxidant properties of the protein concentrates. Food Hydrocoll. 87, 194–203. doi: 10.1016/j.foodhyd.2018.07.047
Ketnawa S., Ogawa Y. (2019). Evaluation of protein digestibility of fermented soybeans and changes in biochemical characteristics of digested fractions. J. Funct. Foods 52, 640–647. doi: 10.1016/j.jff.2018.11.046
Kidgell J. T., Magnusson M., de Nys R., Glasson C. R. (2019). Ulvan: A systematic review of extraction, composition and function. Algal Res. 39, 101422. doi: 10.1016/j.algal.2019.101422
Kimathi A. G., Wakibia J. G., Gichua M. K. (2018). Growth rates of Eucheuma denticulatum and Kappaphycus alvarezii (Rhodophyta; gigartinales) cultured using modified off-bottom and floating raft techniques on the Kenyan coast. Western Indian Ocean J. Mar. Sci. 17 (2), 11–24. doi: 10.4314/wiojms.v17i2.2
Kulshreshtha G., Hincke M. T., Prithiviraj B., Critchley A. (2020). A review of the varied uses of macroalgae as dietary supplements in selected poultry with special reference to laying hen and broiler chickens. J. Mar. Sci. Eng. 8 (7), 536. doi: 10.3390/jmse8070536
Kumar A. K. (2018). Effect of Sargassum wightii on growth, carcass and serum qualities of broiler chickens. Vet. Sci. Res. 3, 000156.
Kumar Y., Tarafdar A., Badgujar P. C. (2021). Seaweed as a source of natural antioxidants: Therapeutic activity and food applications. J. Food Quality 5753391, 17. doi: 10.1155/2021/5753391
Lahaye M., Kaeffer B. (1997). Seaweed dietary fibres: structure, physico-chemical and biological properties relevant to intestinal physiology. Sci. Aliment. 17, 563–584.
Lam K. S. (2007). New aspects of natural products in drug discovery. Trends Microbiol. 15 (6), 279–289. doi: 10.1016/j.tim.2007.04.001
Lares M. L., Flores-Muñoz G., Lara-Lara R. (2002). Temporal variability of bioavailable cd, Hg, zn, Mn and Al in an upwelling regime. Environ. pollut. 120 (3), 595–608. doi: 10.1016/S0269-7491(02)00195-1
Largo D. B., Msuya F. E., Menezes A. (2020). Understanding diseases and control in seaweed farming in Zanzibar. FAO Fisheries Aquaculture Tech. Paper 662), pp.0_1–pp.049. doi: 10.4060/ca9004en
Lee K. W., Ho Hong Y., Lee S. H., Jang S. I., Park M. S., Bautista D. A., et al. (2012). Effects of anticoccidial and antibiotic growth promoter programs on broiler performance and immune status. Res. Vet. Sci. 93, 721–728. doi: 10.1016/j.rvsc.2012.01.001
Levitt G. J., Anderson R. J., Boothroyd C. J. T., Kemp F. A. (2002). The effects of kelp harvesting on its regrowth and the understorey benthic community at danger point, south Africa, and a new method of harvesting kelp fronds. S. Afr. J. Mar. Sci. 24, 71–85. doi: 10.2989/025776102784528501
Li Y., Fu X., Duan D., Liu X., Xu J., Gao X. (2017). Extraction and identification of phlorotannins from the brown alga, Sargassum fusiforme (Harvey) setchell. Mar. Drugs 15 (2), 49. doi: 10.3390/md15020049
Li Q., Luo J., Wang C., Tai W., Wang H., Zhang X., et al. (2018). Ulvan extracted from green seaweeds as new natural additives in diets for laying hens. J. Appl. Phycol 30 (3), 2017–2027. doi: 10.1007/s10811-017-1365-2
Li Y. X., Wijesekara I., Li Y., Kim S. K. (2011). Phlorotannins as bioactive agents from brown algae. Process Biochem. 46 (12), 2219–2224. doi: 10.1016/j.procbio.2011.09.015
Lim S. J., Wan Aida M. W. (2017). “Extraction of sulphated polysaccharides (Fucoidan) from brown seaweed,” in Seaweed polysaccharides: isolation, biological and biomedical applications. Eds. Venkatesan J., Anil S., Kim S. K. (Amsterdam, Netherlands: Elsevier), pp. 27–46. doi: 10.1016/B978-0-12-809816-5.00003-7
Lin H. V., Lu W., Tsai G., Chou C., Hsiao H., Hwang P., et al (2016). Enhanced anti-inflammatory activity of brown seaweed Laminaria japonica by fermentation using Bacillus subtilis. Process Biochem. 51, 1945–1953. doi: 10.1016/j.procbio.2016.08.024
Lorbeer A. J., Tham R., Zhang W. (2013). Potential products from the highly diverse and endemic macroalgae of southern Australia and pathways for their sustainable production. J. Appl. Phycol. 25 (3), 717–732. doi: 10.1007/s10811-013-0003-x
Lourenço S. O., Barbarino E., De-Paula J. C., ´ da Pereira O. L. S., Marquez U. M. L. (2002). Amino acid composition, protein content and calculation of nitrogen-to-protein conversion factors for 19 tropical seaweeds. Phycol. Res. 50, 233–241. doi: 10.1111/j.1440-1835.2002.tb00156.x
Mabeau S., Cavaloc E., Fleurence J., Lahaye M. (1992). New seaweed based ingredients for the food industry. Int. Food Ingred. 3, 38–45.
Mabeau S., Fleurence J. (1993). Seaweed in food products: biochemical and nutritional aspects. Trends. Food Sci. Technol. 4 (4), 103–107. doi: 10.1016/0924-2244(93)90091-N
Machado L., Magnusson M., Paul N. A., Kinley R., de Nys R., Tomkins N. (2016). Identification of bioactives from the red seaweed Asparagopsis taxiformis that promote anti-methanogenic activity in vitro. J. Appl. Phycol. 28, 3117e26. doi: 10.1007/s10811-016-0830-7
MacMonagail M., Cornish L., Morrison L., Araújo R., Critchley A. T. (2017). Sustainable harvesting of wild seaweed resources. Eur. J. Phycol. 52 (4), 371–390. doi: 10.1080/09670262.2017.1365273
Maia M. R. G., Fonseca A. J. M., Oliveira H. M., Mendonça C., Cabrita A. R. J. (2016). The potential role of seaweeds in the natural manipulation of rumen fermentation and methane production. Sci. Rep. 6, 32321. doi: 10.1038/srep32321
Makkar H. P., Tran G., Heuzé V., Giger-Reverdin S., Lessire M., Lebas F. (2016). Seaweeds for livestock diets: A review. Anim. Feed Sci. Technol. 212, 1–17. doi: 10.1016/j.anifeedsci.2015.09.018
Malyarenko O. S., Usoltseva R. V., Zvyagintseva T. N., Ermakova S. P. (2019). Laminaran from brown alga Dictyota dichotoma and its sulfated derivative as radioprotectors and radiosensitizers in melanoma therapy. Carbohydr. Polym. 206, 539–547. doi: 10.1016/j.carbpol.2018.11.008
Manns D., Nielsen M. M., Bruhn A., Saake B., Meyer A. S. (2017). Compositional variations of brown seaweeds Laminaria digitata and Saccharina latissima in Danish waters. J. Appl. Phycol. 29 (3), 149–1506. doi: 10.1007/s10811-017-1056-z
Marareni M., Mnisi C. M. (2020). Growth performance, serum biochemistry and meat quality traits of jumbo quails fed with mopane worm (Imbrasia belina) meal-containing diets. Vet. Anim. Sci. 10, 100141. doi: 10.1016/j.vas.2020.100141
Marinho-Soriano E., Fonseca P. C., Carneiro M. A. A., Moreira W. S. C. (2006). Seasonal variation in the chemical composition of two tropical seaweeds. Bioresour. Technol. 97 (18), 2402–2406. doi: 10.1016/j.biortech.2005.10.014
Marino F., Caro G. D., Gugliandolo C., Spano A., Faggio C. (2016). Preliminary study on the in vitro and in vivo effects of Asparagopsis taxiformis bioactive phyco derivatives on teleosts. Front Physiol 7 (459). doi: 10.3389/fphys.2016.0045
Masenya T. I., Mlambo V., Mnisi C. M. (2021). Complete replacement of maize grain with sorghum and pearl millet grains in jumbo quail diets: Feed intake, physiological parameters, and meat quality traits. PloS One 16 (3), e0249371. doi: 10.1371/journal.pone.0249371
Matshogo T. B., Mlambo V., Mnisi C. M., Manyeula F. (2021). Effect of pre-treating dietary green seaweed with fibrolytic enzymes on growth performance, blood indices, and meat quality parameters of Cobb 500 broiler chickens. Livest Sci 251, 104652. doi: 10.1016/j.livsci.2021.104652
Matshogo T. B., Mnisi C. M., Mlambo V. (2020). Dietary green seaweed compromises overall feed conversion efficiency but not blood parameters and meat quality and stability in broiler chickens. Agriculture 10 (11), 547. doi: 10.3390/agriculture10110547
McHugh D. J. (2003). “FAO fisheries technical paper 441,” in A guide to the seaweed industry, vol. 110. (Rome, Italy: Food and Agriculture Organization of the United Nations).
Michalak I., Chojnacka K. (2015). Algae as production systems of bioactive compounds. Eng Life Sci. 15, 160–176. doi: 10.1002/elsc.201400191
Michalak I., Mahrose K. H. M. (2020). Seaweeds, intact and processed, as a valuable component of poultry feed. J. Mar. Sci. Eng. 8, 620. doi: 10.3390/jmse8080620
Michalak I., Tiwari R., Dhawan M., Alagawany M., Farag M. R., Sharun K., et al. (2022). Antioxidant effects of seaweeds and their active compounds on animal health and production - a review. Vet. Q. 42 (1), 48–67. doi: 10.1080/01652176.2022.2061744
Milledge J. J., Harvey P. J. (2016). Ensilage and anaerobic digestion of Sargassum muticum. J. Appl. Phycol. 28, 3021–3030. doi: 10.1007/s10811-016-0804-9
Milledge J. J., Nielsen B. V., Sadek M. S., Harvey P. J. (2018). Effect of freshwater washing pretreatment on Sargassum muticum as a feedstock for biogas production. Energies 11, e1771. doi: 10.3390/en11071771
Min B. R., Parker D., Brauer D., Waldrip H., Lockard C., Hales K., et al. (2021). The role of seaweed as a potential dietary supplementation for enteric methane mitigation in ruminants: Challenges and opportunities. Anim. Nutr. 7 (4), 1371–1387. doi: 10.1016/j.aninu.2021.10.003
Miyashita K., Beppu F., Hosokawa M., Liu X., Wang S. (2020). Nutraceutical characteristics of the brown seaweed carotenoid fucoxanthin. Arch. Biochem. Biophysics 686, 1083642. doi: 10.1016/j.abb.2020.108364
Mohapatra D., Patel A. S., Kar A., Deshpande S. S., Tripathi M. K. (2019). Effect of different processing conditions on proximate composition, anti-oxidants, anti-nutrients and amino acid profile of grain sorghum. Food Chem. 271, 12–135. doi: 10.1016/j.foodchem.2018.07.196
Mottet A., Tempio G. (2017). Global poultry production: Current state and future outlook and challenges. Worlds Poult. Sci. J. 73 (2), 245–256. doi: 10.1017/S0043933917000071
Mozzachiodi R., Scuri R., Roberto M., Brunelli M. (2001). Caulerpenyne, a toxin from the seaweed Caulerpa taxifolia, depresses afterhyperpolarization in invertebrate neurons. Neuroscience 107 (3), 519–526. doi: 10.1016/s0306-4522(01)00365-7
Mulders K., Lamers P. P., Martens D. E., Wijffels R. H. (2014). Phototrophic pigment production with microalgae: Biological constraints and opportunities. J. Phycol 50, 229–242. doi: 10.1111/jpy.12173
Nhlane L. T., Mnisi C. M., Mlambo V., Madibana M. J. (2020). Nutrient digestibility, growth performance, and blood indices of boschveld chickens fed seaweed-containing diets. Animals 10 (8), 1296. doi: 10.3390/ani10081296
Nhlane L. T., Mnisi C. M., Mlambo V., Madibana M. J. (2021). Effect of seaweed-containing diets on visceral organ sizes, carcass characteristics, and meat quality and stability of boschveld indigenous hens. Poult. Sci. 100 (2), 949–956. doi: 10.1016/j.psj.2020.11.038
Nielsen C. W., Rustad T., Holdt S. L. (2021). Vitamin c from seaweed: A review assessing seaweed as contributor to daily intake. Foods 10 (1), 198. doi: 10.3390/foods10010198
Nkukwana T. T. (2018). Global poultry production: Current impact and future outlook on the south African poultry industry. S. Afr. J. Anim. Sci. 48 (5), 869–884. doi: 10.4314/sajas.v48i5.7
Nunes N., Valente S., Ferraz S., Barreto M. C., Carvalho M. A. A. P. (2018). Nutraceutical potential of Asparagopsis taxiformis (Delile) travisan extracts and assessment of a downstream purification strategy. Heliyon 4 (11), e00957. doi: 10.1016/j.heliyon.2018.e00957
Peinado I., Girón J., Koutsidis G., Ames J. M. (2014). Chemical composition, antioxidant activity and sensory evaluation of five different species of brown edible seaweeds. Food Res. Int. 66, 36–44. doi: 10.1016/j.foodres.2014.08.035
Perusek L., Maeda T. (2013). Vitamin a derivatives as treatment options for retinal degenerative diseases. Nutrients 5 (7), 2646–2666. doi: 10.3390/nu5072646
Ponce N., Stortz C. A. (2020). A comprehensive and comparative analysis of the fucoidan compositional data across the phaeophyceae. Front. Plant Sci. 1844. doi: 10.3389/fpls.2020.556312
Poojary M. M., Barba F. J., Aliakbarian B., Donsì F., Pataro G., Dias D. A., et al. (2016). Innovative alternative technologies to extract carotenoids from microalgae and seaweeds. Mar. Drugs 14(11), 214. doi: 10.3390/md14110214
Poza A. M., Fernández C., Latour E. A., Raffo M. P., Delatorre F. G., Parodi E. R. (2022). Optimization of the rope seeding method and biochemical characterization of the brown seaweed Asperococcus ensiformis. Algal Res. 64, 102668. doi: 10.1016/j.algal.2022.102668
Prajapati V. D., Maheriya P. M., Jani G. K., Solanki H. K. (2014). Carrageenan: a natural seaweed polysaccharide and its applications. Carbohydr. Polym. 105, 97–112. doi: 10.1016/j.carbpol.2014.01.067
Priyadarshani I., Biswajit R. (2012). Commercial and industrial applications of micro algae – a review. J Algal Biomass Util. 3 (4), 89–100.
Rafiquzzaman S. M., Kong I. S., Kim J. M. (2015). Enhancement of antioxidant activity, total phenolic and flavonoid content of Saccharina japonica by submerged fermentation with Aspergillus oryzae. KSBB J. 30 (1), 7–32. doi: 10.7841/ksbbj.2015.30.1.27
Rajauria G., Foley B., Abu-Ghannam N. (2017). Characterization of dietary fucoxanthin from Himanthalia elongata brown seaweed. Food Res. Int. 99 (3), 995–1001. doi: 10.1016/j.foodres.2016.09.023
Reboleira J., Silva S., Chatzifragkou A., Niranjan K., Lemos M. F. (2021). Seaweed fermentation within the fields of food and natural products. Trends food. Sci. Technol. 116, 1056–1073. doi: 10.1016/j.tifs.2021.08.018
Rioux L. E., Turgeon S. L., Beaulieu M. (2007). Characterization of polysaccharides extracted from brown seaweeds. Carbohydr. Polym. 69, 530–537. doi: 10.1016/j.carbpol.2007.01.009
Rioux L. E., Turgeon S. L., Beaulieu M. (2010). Structural characterization of laminaran and galactofucan extracted from the brown seaweed Saccharina longicruris. Phytochemistry 71, 1586–1595. doi: 10.1016/j.phytochem.2010.05.021
Roque B. M., Salwen J. K., Kinley R., Kebreab E. (2019). Inclusion of Asparagopsis armata in lactating dairy cow's diet reduces enteric methane emission by over 50 percent. J. Clean. Prod. 234, 132e8. doi: 10.1016/j.jclepro.2019.06.193
Ruocco N., Costantini S., Guariniello S., Costantini M. (2016). Polysaccharides from the marine environment with pharmacological, cosmeceutical and nutraceutical potential. Molecules 21 (5), 551. doi: 10.3390/molecules21050551
Saeed M., Arain M. A., Ali Fazlani S., Marghazani I. B., Umar M., Soomro J., et al. (2021). A comprehensive review on the health benefits and nutritional significance of fucoidan polysaccharide derived from brown seaweeds in human, animals and aquatic organisms. Aquac. Nutr. 27 (3), 633–654. doi: 10.1111/anu.13233
Sánchez-Velásquez J. J., Reyes-Flores L. E., Yzásiga-Barrera C., Zelada-Mázmela E. (2021). The introduction of the asian red algae Melanothamnus japonicus (Harvey) díaz-tapia & maggs in Peru as a means to adopt management strategies to reduce invasive non-indigenous species. Diversity 13 (5), 176. doi: 10.3390/d13050176
Serive B., Kaas R., Bérard J. B., Pasquet V., Picot L., Cadoret J. P. (2012). Selection and optimisation of a method for efficient metabolites extraction from microalgae. Bioresour. Technol. 124, 311–320. doi: 10.1016/j.biortech.2012.07.105
Silva J., Alves C., Freitas R., Martins A., Pinteus S., Ribeiro J., et al. (2019). Antioxidant and neuroprotective potential of the brown seaweed Bifurcaria bifurcata in an in vitro Parkinson’s disease model. Mar. Drugs 17 (2), 85. doi: 10.3390/md17020085
Shukla P. S., Mantin E. G., Adil M., Bajpai S., Critchley A. T., Prithiviraj B. (2019). Ascophyllum nodosum-based biostimulants: Sustainable applications in agriculture for the stimulation of plant growth, stress tolerance, and disease management. Front. Plant Sci. 10. doi: 10.3389/fpls.2019.00655
Smetacek V., Zingone A. (2013). Green and golden seaweed tides on the rise. Nature 504 (7478), 84–88. doi: 10.1038/nature12860
Sobotik E., Nelson J., Archer G. (2018). “The effect of feeding a seaweed extract during heat stress on broiler production and stress,” in Proceedings of the poultry science association annual, San Antonio, TX, USA: Poultry Science Association, pp. 23–26.
Stokvis L., Kwakkel R. P., Hendriks W. H., Kals J. (2022). Proteolytic enzyme-treated seaweed co-product (Ulva laetevirens) inclusion in corn-soybean and European broiler diets to improve digestibility, health and performance. Poult. Sci. 101(6), 101830. doi: 10.1016/j.psj.2022.101830
Stokvis L., van Krimpen M. M., Kwakkel R. P., Bikker P. (2021). Evaluation of the nutritional value of seaweed products for broiler chickens’ nutrition. Anim. Feed Sci. Technol. 280, 115061. doi: 10.1016/j.anifeedsci.2021.115061
Stupart C. M. (2019). “Supplementation of red seaweed (Chondrus crispus) and tasco® (Ascophyllum nodosum) in laying hen diets,” Master’s thesis. Dalhousie University, Truro, NS, Canada.
Tenorio A. T., Kyriakopoulou K. E., Suarez-Garcia E., van den Berg C., van der Goot A. J. (2018). Understanding differences in protein fractionation from conventional crops, and herbaceous and aquatic biomass-consequences for industrial use. Trends Food Sci. Technol. 71, 235–245. doi: 10.1016/j.tifs.2017.11.010
Thema K., Mlambo V., Snyman N., Mnisi C. M. (2019). Evaluating alternatives to zinc-bacitracin antibiotic growth promoter in broilers: Physiological and meat quality responses. Animals 9 (12), 1160. doi: 10.3390/ani9121160
Theurl M. C., Lauk C., Kalt G., Mayer A., Kaltenegger K., Morais T. G. (2020). Food systems in a zero-deforestation world: Dietary change is more important than intensification for climate targets in 2050. Sci. Total Environ. 735, 139353. doi: 10.1016/j.scitotenv.2020.139353
Tippmer K., Schmitt H., Vinke H., Abendroth G. (1978) Method of removing ash components from high-ash content coals (United States). Available at: https://www.osti.gov/biblio/6848477 (Accessed May 25, 2022).
Tong T., Liu X., Yu C. (2021). Extraction and nano-sized delivery systems for phlorotannins to improve its bioavailability and bioactivity. Mar. Drugs 19 (11), 625. doi: 10.3390/md19110625
Trivedi N., Reddy C. R. K., Radulovich R., Jha B. (2015). Solid state fermentation (SSF)-derived cellulase for saccharification of the green seaweed Ulva for bioethanol production. Algal Res. 9, 48–54. doi: 10.1016/j.algal.2015.02.025
Ummat V., Sivagnanam S. P., Rajauria G., O'Donnell C., Tiwari B. (2021). Advances in pre-treatment techniques and green extraction technologies for bioactives from seaweeds. K Trends Food Sci. Technol. 110, 90–106. doi: 10.1016/j.tifs.2021.01.018
Van de Velde F., Lourenco N. D., Pinheiro H. M., Bakkerd M. (2002). Carrageenan: A food-grade and biocompatible support for immobilisation techniques. Adv. Synth. Catal. 344, 815–835. doi: 10.1002/1615-4169(200209)344:8<815::AID-ADSC815>3.0.CO;2-H
Venkatesan M., Arumugam V., Pugalendi R., Ramachandran K., Sengodan K., Vijayan S. R. (2019). Antioxidant, anticoagulant and mosquitocidal properties of water soluble polysaccharides (WSPs) from Indian seaweeds. Process Biochem. 84, 196–204. doi: 10.1016/j.procbio.2019.05.029
Venkatesan J., Lowe B., Anil S., Manivasagan P., Kheraif A. A. A., Kang K. H., et al. (2015). Seaweed polysaccharides and their potential biomedical applications. Starch -. Stärke 67, 381–390. doi: 10.1002/star.201400127
Vorobiev E., Lebovka N. (2015). “Selective extraction from food plants and residues by pulsed electric field,” in Green extraction of natural products: Theory and practice. Eds. Chemat F., Strube J. (Hoboken, New Jersey: Wiley), 307 –332. doi: 10.1002/9783527676828.ch9
Wang S., Hui J. Y., Hua W. L., Hua Z. F., Ting L. Y. (2013). Enteromorpha prolifera supplemental level: Effects on laying performance, egg quality, immune function and microflora in feces of laying hens. Chin. J. Anim. Nutr. 25, 1346–1352.
Wang Y., Xing M., Cao Q., Ji A., Liang H., Song S. (2019). Biological activities of fucoidan and the factors mediating its therapeutic effects: a review of recent studies. Mar. Drugs 17, 183. doi: 10.3390/md17030183
Ward G. M., Faisan J. P. Jr., Cottier-Cook E. J., Gachon C., Hurtado A. Q., Lim P. E. (2020). A review of reported seaweed diseases and pests in aquaculture in Asia. J. World Aquac. Soc 51, 815–828. doi: 10.1111/jwas.12649
Wells M. L., Potin P., Craigie J. S., Raven J. A., Merchant S. S., Helliwell K. E. (2017). Algae as nutritional and functional food sources: Revisiting our understanding. J. Appl. Phycol. 29 (2), 949–982. doi: 10.1007/s10811-016-0974-5
Wideman N., O'Bryan C. A., Crandall P. G. (2016). Factors affecting poultry meat colour and consumer preferences-a review. Worlds Poult. Sci. J. 72 (2), 353–366. doi: 10.1017/S0043933916000015
Wijesinghe W., Jeon Y. J. (2012). Enzyme-assistant extraction (EAE) of bioactive components: A useful approach for recovery of industrially important metabolites from seaweeds: A review. Fitoterapia 83 (2012), 6–12. doi: 10.1016/j.fitote.2011.10.016
Yaich H., Amira A. M., Abbes F., Bouaziz M., Besbes S., Richel A. (2017). Effect of extraction procedures on structural, thermal and antioxidant properties of ulvan from Ulva lactuca collected in monastir coast. Int. J. Biol. Macromol. 105, 1430–1439. doi: 10.1016/j.ijbiomac.2017.07.141
Yen Y., Weisbjerg M. R., Rautenberger R., Fečkaninová A., Novoa-Garrido M. (2022). Improving fermentation of Saccharina latissima and Alaria esculenta silages with additives for preserving biomass and antioxidants. J. Appl. Phycol. 34, 625–636. doi: 10.1007/s10811-021-02628-4
Yong W. T. L., Thien V. Y., Rupert R., Rodrigues K. F. (2022). Seaweed: A potential climate change solution. Renew. Sust. Energ. Rev. 159, 112222. doi: 10.1016/j.rser.2022.112222
Yotsu-Yamashita M., Kondo S., Segawa S., Lin Y. C., Toyohara H., Ito H., et al. (2013). Isolation and structural determination of two novel phlorotannins from the brown alga Ecklonia kurome okamura, and their radical scavenging activities. Mar Drugs 11 (1), 165–183. doi: 10.3390/md11010165
Zewei S. H., Abu Hafsa S. H., Zahran S. M., Ahmed M. S., Abdel–Rahman N. (2019). Effects of dietary supplementation with green and brown seaweeds on laying performance, egg quality, and blood lipid profile and antioxidant capacity in laying Japanese quail. Egypt. Poult. Sci. J. 39, 41–59. doi: 10.21608/EPSJ.2019.28828
Zhang Q., Li N., Liu X., Zhao Z., Li Z., Xu Z. (2004). The structure of a sulfated galactan from Porphyra haitanensis and its in vivo antioxidant activity. Carbohydr. Res. 339, 105–111. doi: 10.1016/j.carres.2003.09.015
Zou Y., Xu X., Li L., Yang F., Zhang S. (2018). Enhancing methane production from U. lactuca using combined anaerobically digested sludge (ADS) and rumen fluid pre-treatment and the effect on the solubilization of microbial community structures. Bioresour. Technol. 254, 83–90. doi: 10.1016/j.biortech.2017.12.054
Keywords: antibiotic growth promoters, bioactive compounds, meat quality, poultry nutrition, seaweeds, sustainability
Citation: Mlambo V, Mnisi CM, Matshogo TB and Mhlongo G (2022) Prospects of dietary seaweeds and their bioactive compounds in sustainable poultry production systems: A symphony of good things? Front. Anim. Sci. 3:998042. doi: 10.3389/fanim.2022.998042
Received: 19 July 2022; Accepted: 20 September 2022;
Published: 04 October 2022.
Edited by:
Alexandros Mavrommatis, Agricultural University of Athens, GreeceReviewed by:
Edouard Coudert, Centre Mondial de l’Innovation Roullier, FranceCopyright © 2022 Mlambo, Mnisi, Matshogo and Mhlongo. This is an open-access article distributed under the terms of the Creative Commons Attribution License (CC BY). The use, distribution or reproduction in other forums is permitted, provided the original author(s) and the copyright owner(s) are credited and that the original publication in this journal is cited, in accordance with accepted academic practice. No use, distribution or reproduction is permitted which does not comply with these terms.
*Correspondence: Victor Mlambo, VmljdG9yLk1sYW1ib0B1bXAuYWMuemE=
Disclaimer: All claims expressed in this article are solely those of the authors and do not necessarily represent those of their affiliated organizations, or those of the publisher, the editors and the reviewers. Any product that may be evaluated in this article or claim that may be made by its manufacturer is not guaranteed or endorsed by the publisher.
Research integrity at Frontiers
Learn more about the work of our research integrity team to safeguard the quality of each article we publish.