- Department of Biochemistry, Memorial University of Newfoundland, St. John’s, NL, Canada
Guanidinoacetic acid (GAA) is an amino acid derivative and precursor for creatine which plays a significant role in energy metabolism. However, because of creatine’s instability during the manufacturing process and cost, GAA has been explored as an effective alternative to creatine supplements. GAA has been tested as a potential feed additive to enhance energy utilization and growth performance in the poultry and swine industries. Moreover, GAA has been combined with methionine to improve growth outcomes and may also act as an arginine-sparing agent in birds. The safety of the GAA supplements for animals, consumers, and the environment and its efficacy in numerous livestock species have been proven. This narrative review discusses the scientific evidence regarding the metabolism and effects of GAA supplementation in swine and poultry, identifying the knowledge gaps and future directions for further research on GAA supplementation. A systematic search of the literature identified published research findings related to GAA supplementation in swine and poultry and their findings are summarized in this narrative review to confirm the impacts of GAA supplementation on growth performance, reproductive performance, and meat quality in swine and poultry. Amongst its many demonstrated benefits, GAA is effective at improving body creatine concentration, growth parameters, feed conversion ratio, and performance of animals. Although GAA exerts many non-creatine roles, including the stimulation of insulin secretion, neuromodulation, and vasodilation, further research may require in-depth elaboration.
Introduction
The aim of this narrative review is to offer a broad overview of the effects of guanidinoacetic acid (GAA) supplementation in livestock, mainly focussing on swine and poultry. Guanidinoacetic acid, also known as glycocyamine or guanidinoacetate, is an amino acid derivative and endogenous substance in body tissues (Mori et al., 1996). Guanidinoacetate is an immediate precursor for creatine, which plays a major role in energy metabolism in cells (Ostojic et al., 2013). Creatine can be phosphorylated and acts as an energy buffer in high energy-demanding tissues such as skeletal muscle, heart, retina, and spermatozoa (Wyss and Kaddurah-Daouk, 2000). Creatine and phosphocreatine are converted to creatinine and excreted in urine. Therefore, endogenous creatine synthesis and dietary creatine intake are essential to replenish daily creatine losses (Wyss and Kaddurah-Daouk, 2000). Creatine has been extensively studied as an ergogenic supplement due to its role in energy metabolism (Wyss and Kaddurah-Daouk, 2000). However, because of creatine’s relative instability in foodstuffs (Baker, 2009), GAA has been explored as a potential alternative to creatine supplements. This narrative review about GAA supplementation is divided into three sections. First, an overview of GAA biosynthesis and excretion, mechanism of action, transport, and distribution is provided to introduce the background of GAA metabolism. Next, the importance and effects of GAA on growth performance, meat quality, reproduction, and brain functions in swine and poultry are discussed based on literature review. Finally, the link between GAA, arginine, and methionine metabolism is further elaborated based on the findings from swine and poultry research.
Methods
In June 2022, we searched the databases PubMed, Scopus, and Google Scholar for papers on GAA metabolism and the effects of GAA supplementation in swine and poultry species. Synonyms of the following terms (linked by ‘AND,’ ‘OR’) were used to search titles and/or abstracts of indexed references: GAA supplementation; swine; poultry; GAA metabolism (See Table 1). Only records published in English were considered, and additional papers were discovered by examining the manuscript’s references, which were discovered through literature database searches.
Study characteristics
A total of 1247 non-unique entries were found in PubMed, Scopus, and Google Scholar searches. We found 71 publications to examine further, after publications were eliminated because they were not related to our research issue or did not report on novel research findings. Fifteen research articles focused on swine, 52 on poultry, and six on the metabolism of GAA supplements. The effects of GAA as a supplement or feed additive for swine and poultry appear to be well-documented in peer-reviewed research. Reviewed papers suggest widespread evidence for the importance and effects of GAA as a supplement in swine and poultry. The summary of the research concerning the effects of GAA supplementation on growth performance in poultry and swine is presented in Table 2.
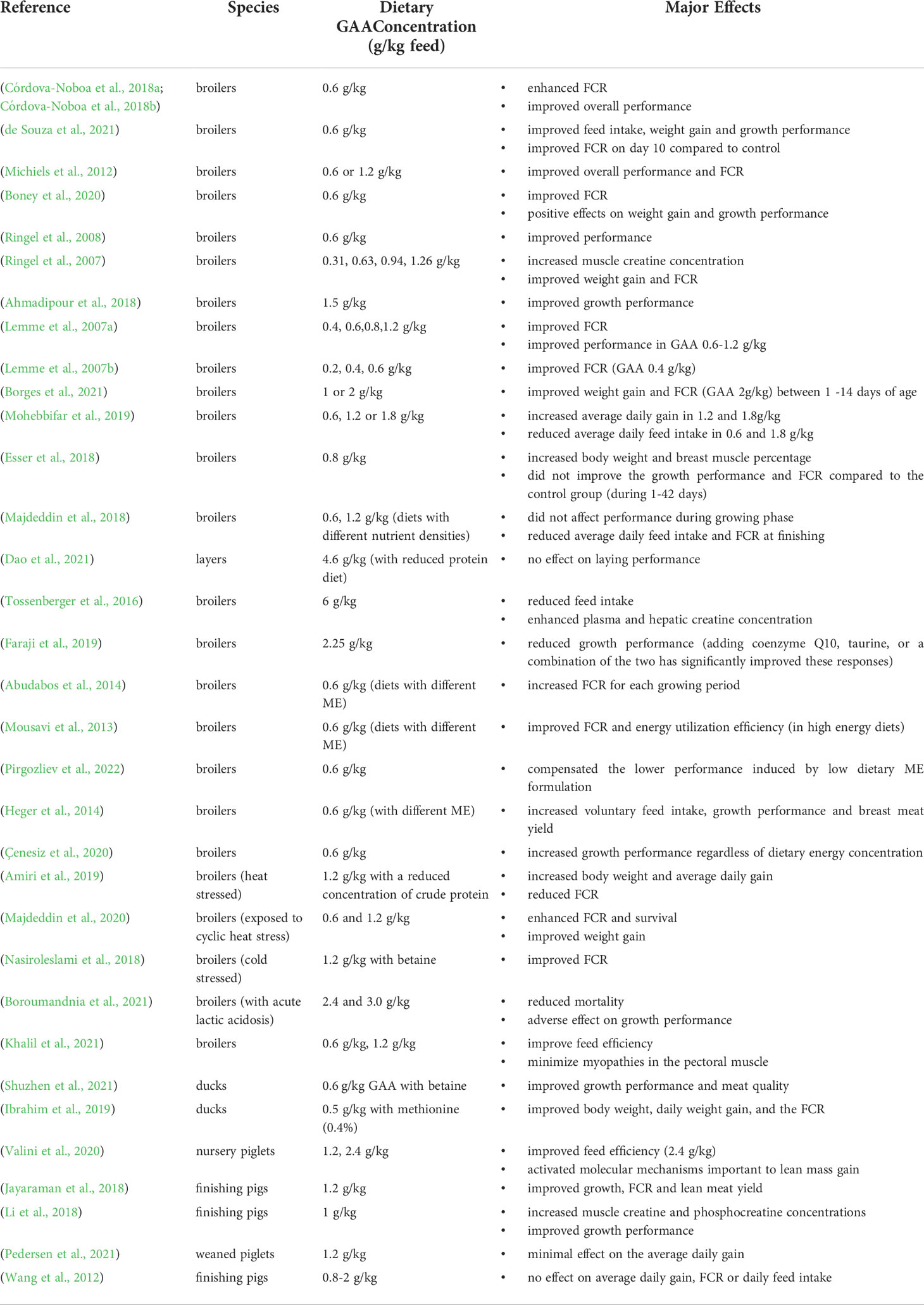
Table 2 An overview of the research concerning the effects of GAA supplementation on growth performance in poultry and swine.
GAA biosynthesis and excretion
Guanidinoacetate synthesis requires two amino acids, arginine, and glycine. Arginine transfers its amidino group to the amino group of glycine to produce ornithine and GAA, catalyzed by L-arginine: glycine amidinotransferase (AGAT). Guanidinoacetate is then methylated using a methyl group from S-adenosylmethionine (SAM), which is synthesized from methionine. This reaction produces creatine and S-adenosylhomocysteine (SAH) and is catalyzed by guanidinoacetate N-methyltransferase (GAMT) (Wyss and Kaddurah-Daouk, 2000). Creatine synthesis is an inter-organ metabolic process with GAA synthesis occurring primarily in the kidney and GAA methylation occurring mainly in the liver (Wyss and Kaddurah-Daouk, 2000).
However, GAA production seems to have variations across species. For example, GAA synthesis occurs in kidneys of both rats and humans, but the capacity of GAA production varies (Edison et al., 2007). The kidney is considered the primary site for GAA production in rats and piglets, but in humans, the kidney only contributes 20% of GAA production (Edison et al., 2007). Regarding tissue distribution of enzymes, AGAT activity is highest in the kidney of rats (Da Silva et al., 2009) and piglets (Brosnan et al., 2009), but piglets also have considerable AGAT activity in the pancreas (Brosnan et al., 2009). Nevertheless, recent findings from our lab group showed that this greater level of pancreatic AGAT activity does not translate into a significant source of GAA for the rest of the body (Dinesh et al., 2020). Indeed, more than 80% of total GAA released in the whole body comes from the kidney, suggesting the kidney is quantitatively the most important source of GAA for creatine synthesis in young piglets (Dinesh et al., 2020). Moreover, Dinesh et al. (2020) found a net release of GAA from the piglet gut, suggesting intestinal cells also have a significant capacity for GAA synthesis, despite a modest specific activity of AGAT. Comparable data in other species have not been reported.
Similar to GAA synthesis, GAA methylation also exhibits different rates among various tissues. GAA methylation is highest in the liver, which is reflected by the highest GAMT specific activity among tissues in rats (Da Silva et al., 2009), piglets (Brosnan et al., 2009), and many other mammalian species (Wyss and Kaddurah-Daouk, 2000). Lesser but notable levels of GAMT activity have also been measured in the pancreas, kidney, intestine, brain, and muscle in piglets (Brosnan et al., 2009). However, a scarce amount of research is done on poultry to identify the enzymatic activities in GAA and creatine synthesis pathways.
Feedback regulation of creatine synthesis mainly occurs at AGAT (Wyss and Kaddurah-Daouk, 2000). Moreover, GAA synthesis is regulated by negative feedback repression of AGAT by creatine and L-ornithine concentrations (Wyss and Kaddurah-Daouk, 2000). For example, creatine supplements can downregulate GAA synthesis by inhibiting AGAT activity and mRNA expression in rat kidneys (Edison et al., 2007). In piglets, AGAT activity is reduced in both kidneys and pancreas with creatine supplementation, but not with GAA supplements (Dinesh et al., 2021). Although there is no evidence of feedback regulation at GAMT, its activity seems to be induced when precursors GAA and methionine are supplemented to the diet (Dinesh et al., 2021). Availability of precursors for these enzymes may also regulate flux through these enzymes as a key creatine transporter (SLC6A8) has been shown to be regulated by extracellular creatine concentration (Loike et al., 1988; Wyss and Kaddurah-Daouk, 2000).
In contrast to other organs, the expressions of AGAT, GAMT, and creatine transporters in the central nervous system (CNS) suggest that the CNS can synthesize its own creatine from GAA (Braissant and Henry, 2008). However, the expressions of AGAT, GAMT, and creatine transporters vary among different brain regions. Most types of brain cells express any of the above enzymes or transporters, while most brain regions do not have AGAT and GAMT together, suggesting the inability of creatine synthesis within the same brain region (Hanna-El-Daher and Braissant, 2016). However, inadequate information is available on the brain GAA metabolism in poultry and swine. If there are any brain effects related to GAA supplements, it may affect behavior and the production of livestock species. Therefore, future researchers should address the questions related to GAA transport and metabolism in the brain to fully understand dietary GAA’s fate in livestock.
GAA excretion mainly occurs through conversion to creatine. However, GAA can also be directly excreted via the kidney, in addition to its major losses via creatine and creatinine synthesis. Tossenberger et al. (2016) demonstrated that broilers have an effective method to offset GAA oversupply by increasing GAA transformation to creatine and creatinine, and then excreting these metabolites and excess GAA through the urine. These findings suggest GAA excretion can increase rapidly with increasing dietary GAA. However, there is little other information on the GAA excretion in broilers and swine available in the literature. More studies are needed on elaborating the effects of GAA supplementation on GAA excretion pathways.
Mechanism of action, transport, and distribution
Once GAA is synthesized, it needs to be transported to the target cell for further metabolism. GAA transport depends on several types of transporters, and their distribution varies throughout the body. Although GAA transport mechanisms are not fully described, some plausible candidates have been suggested. GAA transport has been suggested to be mediated by creatine transporters (CRT/Solute carrier SLC6A8), taurine transporters (TauT/SLC6A6), γ-aminobutyric acid (GABA) transporter (SLC6 A13), and via passive diffusion through plasmalemma (Tachikawa et al., 2009). For example, hepatic GAA uptake in rat liver seems to occur mainly through GABA transporter (Tachikawa et al., 2012). Although GAA can be transported via creatine transporters, GAA appears to have a lesser affinity than creatine (Tachikawa et al., 2009). Therefore, supplementing GAA with creatine may lead to creatine transporter saturation and greater GAA transport via other transporters, with a net synergistic result of enhanced cellular GAA and creatine concentrations (Ostojic, 2017). However, more studies are needed on GAA/creatine interactions during transport in various tissues in swine and poultry.
GAA supplementation
Guanidinoacetate as a therapeutic agent has been studied since the early 1950s (Borsook and Borsook, 1951). However, GAA supplementation gained more attention in the poultry industry after the ban on meat and bone meal in Europe in 2001. Prohibition of animal by-products, which provided creatine, led to reduced performance in poultry (Córdova-Noboa et al., 2018a). In addition, most growing-finishing pig diets in Europe are plant-based and do not contain sufficient amounts of creatine (He et al., 2018). Therefore, GAA supplementation has been explored to enhance growth performance in the poultry and swine industry as an alternate method to giving creatine.
Importance of GAA as a supplement
Creatine supplements have been used as growth performance-enhancing agents in livestock, because of its role in muscle metabolism. However, due to the supplemental creatine’s high cost, GAA has been tested in animal diets, especially during the later growth periods, when feed consumption is the greatest (He et al., 2018). Moreover, creatine supplements have other significant drawbacks, including instability during manufacturing (Baker, 2009) and relatively low bioavailability (Alraddadi et al., 2018). Guanidinoacetate supplementation has proven to be a stable feed additive in animal feed by the European Food Safety Authority (2009; 2022). Moreover, a recent study investigating the stability of GAA in canine food during manufacturing and storing (van der Poel et al., 2019) found that granulated and crystallized GAA have high stability compared to added creatine. GAA supplements appear to have twice the solubility of creatine supplements and 40% lesser cost (Ostojic and Vojvodic-Ostojic, 2015). Therefore, as the only natural precursor for creatine, GAA may be considered a safe and beneficial substitute for creatine.
In animals, GAA has been tested as a potential supplement to enhance growth performance, feed conversion ratio (FCR), meat yield and quality, reproductive performance, and as an arginine sparing agent (Figure 1). Although GAA is thought to exert its effects via creatine, GAA supplementation also acts via several other metabolic pathways. For example, GAA can exert direct effects on endocrine functions, neuromodulation, and oxidant-antioxidant processes, which is beyond the scope of this narrative review. Nevertheless, the main effect of GAA is as a precursor for creatine as it can increase creatine stores effectively. Many studies have demonstrated GAA supplementation’s effectiveness in enhancing creatine concentration in muscles (Liu et al., 2015), liver, kidney, and plasma (McBreairty et al., 2013; Tossenberger et al., 2016), leading to better growth and performance.
Effects of GAA supplementation on growth performance
The swine and poultry (broiler) industry aim to achieve the best growth performance with the lowest possible cost and a minor impact on the environment. Therefore, maximizing feed efficiency is important to avoid unnecessary expenses and to minimize the environmental pollution associated with feeding excess nutrients.
Guanidinoacetate supplementation in broiler diets could be an effective technique for improving growth and gastrointestinal health (Ahmadipour et al., 2018). Recently, many studies have reported the positive effects of GAA supplementation on growth performance in broilers (Zhao et al., 2021). Because of the reduced broiler performance observed after adopting pure plant-based diets in broilers, GAA was proposed as a potential supplement to improve broiler performance (Ringel et al., 2007). Supplemental GAA in all-plant diets during the finishing period enhanced feed to gain ratio in broilers and, consequently, led to better overall performance (Michiels et al., 2012; Córdova-Noboa et al., 2018b). Dietary GAA (0.6 g/kg) improved feed intake, weight gain and growth performance of broiler chickens at day 10 (de Souza et al., 2021). Moreover, dietary GAA inclusion had positive effects on weight gain and improved FCR in broilers (Lemme et al., 2007a; Lemme et al., 2007b; Ringel et al., 2007; Ringel et al., 2008; Mousavi et al., 2013; Córdova-Noboa et al., 2018a; Boney et al., 2020; Borges et al., 2021; de Souza et al., 2021).These studies suggest GAA can be used as a supplement to enhance FCR and energy efficiency in broiler chickens. Improved FCR suggests efficient nutrient and energy utilization, which has been promoted as one of the best benefits of GAA supplements.
The effect of GAA supplementation on growth performance parameters depends on the GAA dosage. Guanidinoacetate supplementation up to 1.5 g/kg of feed improves growth performance in broilers, but beyond that concentration, the growth response decreases (Ahmadipour et al., 2018). In other studies, broiler chickens received a diet that was supplemented with 1.2 or 1.8 g/kg had a increased average daily gain than broilers fed with 0.6 g/kg (Mohebbifar et al., 2019). However, there was a reduced average daily feed intake in birds fed with 0.6 and 1.8 g/kg GAA than the birds fed with 1.2 g/kg GAA, suggesting dietary GAA’s effect on feed efficiency might also depend on the dosage in broilers (Mohebbifar et al., 2019). Optimum GAA supplementation to enhance growth performance and FCR seems to range from 0.6 – 1.2 g/kg in broilers (Lemme et al., 2007a), while 0.6 g/kg GAA is suggested to be the minimum dose for improving performance (He et al., 2019). Moreover, according to the European Food Safety Authority (2009; 2022), 0.6 – 0.8 g/kg of GAA may be considered a safe concentration as a feed additive to enhance growth performance in broilers. However, it should be noted that some studies have reported that GAA is not effective as a supplement on growth performance (Lemme et al., 2007a; Mousavi et al., 2013; Abudabos et al., 2014; Tossenberger et al., 2016). For example, GAA (0.8 g/kg) inclusion in plant-based diets increased the body weight and breast muscle percentage of one-week-old broiler chickens but did not improve the growth performance and FCR compared to the control group during the period from 1 to 42 days of age (Esser et al., 2018). Moreover, when layers were supplemented with GAA (to the reduced protein diet) reduced feed intake, increased FCR and did not affect laying performance (Dao et al., 2021). According to Majdeddin et al. (2018), GAA supplementation in broiler diets with different nutrient densities, did not affect performance during growing phase and reduced average daily feed intake and FCR at finishing period. These differences may be due to GAA dosage, experiment duration, initial body weight, nutrient composition of the diet, or species variation. With respect to feed intake, these concentrations of GAA supplementation generally do not affect feed intake in broilers in any phase, even when live performance is enhanced (Córdova-Noboa et al., 2018a). However, very high GAA supplementation (6 g/kg) leads to reduced feed intake in broilers, suggesting that the effect is akin to an overdose of amino acids, which also reduce feed intake at very high doses (Tossenberger et al., 2016). For example, broiler chickens’ growth response was deteriorated by high amounts of GAA (2.25 g/kg) (Faraji et al., 2019). However, adding coenzyme Q10, taurine, or a combination of the two in broiler diets significantly improved these responses (Faraji et al., 2019). Notably, excess GAA significantly enhances plasma and hepatic creatine concentration in broilers (Tossenberger et al., 2016; Córdova-Noboa et al., 2018a). This newly synthesized creatine can downregulate endogenous GAA synthesis via inhibition of AGAT activity, which may help prevent GAA accumulation (Tossenberger et al., 2016).
There is also an interaction between dietary GAA and energy content on growth performance in poultry. Supplementing GAA to a diet with lower metabolizable energy (ME) (by 50 kcal/kg) improved FCR in broilers (Abudabos et al., 2014). In addition, dietary GAA with greater energy concentrations (95-100% of energy requirement) enhanced energy efficiency and improved FCR throughout the growing periods in broiler chickens (Mousavi et al., 2013). Moreover, the caloric intake per kg of body weight gain and per kg of carcass weight was reduced by GAA supplementation (Mousavi et al., 2013). These studies suggest that broilers’ finishing period requires more energy with GAA to synthesize sufficient creatine to support enhanced muscle growth and maintenance. When broilers were given a basal diet with the standard concentrations of ME or reduced ME, and graded concentrations of GAA supplements (0.6 and 1.2 g/kg), the highest concentration of GAA inclusion had positive effects on growth performance, which was compromised by the lesser ME diet. Hence, high GAA supplementation (1.2 g/kg) to a low energy diet may offset the negative effects of feed energy reduction on growth performance (Fosoul et al., 2018). Additionally, GAA offers many advantages beyond enhancing growth performance, making it beneficial and a cost-effective supplement, even in low-energy diets. These findings are consistent with those in other studies (Metwally et al., 2020; Ceylan et al., 2021). Moreover, Pirgozliev et al. (2022) has shown GAA 0.6 g/kg GAA supplementation may compensate the lower performance induced by low dietary ME formulation. Dietary GAA supplements (0.6 g/kg) reduced voluntary feed intake in broilers during the finishing period, while significantly improving FCR and energy utilization efficiency (Mousavi et al., 2013). However, GAA added to a diet with slightly reduced apparent ME resulted in an increased voluntary feed intake and consequently increased protein and amino acid intakes, which was manifested by improved growth performance and breast meat yield (Heger et al., 2014). In contrast, supplementing GAA to plant-based or poultry by-product meal-containing diets could increase broiler growth performance regardless of dietary energy concentrations (Çenesiz et al., 2020). According to the above findings, GAA supplementation’s effects with a diet containing variable energy concentrations are inconsistent. Further studies are warranted address this as required to determine the significance of GAA as a feed additive to improve the cost of feed per kg of body weight gain and, consequently, net profitability.
Effects of dietary GAA during stress conditions- heat stress, cold stress, and diseases
According to the literature, many studies have been performed under several stress conditions to evaluate GAA’s effectiveness to improve adverse effects. Environmental stress is common in broiler production which often occurs due to various conditions. For example, the changes of ambient temperature may affect bird’s behavior, metabolism and physiology, resulting in low production efficiency (Nawaz et al., 2021). In heat-stressed animals, reducing feed intake is an adaptation method to reduce metabolic heat output, and heat-stressed birds experience a considerable reduction in feed intake, body weight gain, and feed efficiency (Nawaz et al., 2021). Several studies have demonstrated that supplemental GAA improves growth performance in heat-stressed chickens. For example, dietary GAA (1.2 g/kg) with a reduced concentration of crude protein (90% of the normal CP concentration) increased the body weight and average daily gain and reduced FCR in heat-stressed broilers (Amiri et al., 2019). Also, the GAA effect was more profound during the finishing period, as birds at this stage require more energy during the highest muscle growth (Mousavi et al., 2013; Amiri et al., 2019). Furthermore, GAA supplementation (0.6 and 1.2 g/kg) enhanced feed conversion ratio and survival, with the greatest gains occurring during the finishing phase when the birds were exposed to cyclic heat stress (Majdeddin et al., 2020). Hence, GAA may be considered a feed additive to improve broilers’ growth performance under heat stress (Amiri et al., 2019). Cold stress is another physical environmental stress that hugely impacts the poultry industry. During commercial broiler production, cool temperatures are the primary cause of ascites and related deterioration of growth performance (İpek and Sahan, 2006). Dietary inclusion of 1.2 g/kg GAA and betaine improved FCR in broilers under cold stress, suggesting GAA can be used as an efficient supplement to improve the harmful effects of cold stress in broilers (Nasiroleslami et al., 2018). Sudden death syndrome (SDS) is one of the main causes of mortality in broilers and causes severe economic losses (Sosnówka-Czajka and Skomorucha, 2022). Increased blood lactic acid concentrations are likely to play a role in broiler chicken SDS. Dietary GAA at 2.4 and 3.0 g/kg reduced mortality in broiler chickens with acute lactic acidosis but had an adverse effect on growth performance (Boroumandnia et al., 2021). However, there was no clear evidence that extra nutritional GAA significantly influenced the incidence of SDS in chickens. Furthermore, GAA can be utilized as a feed supplement in intensive rearing systems to improve feed efficiency and minimize myopathies in the pectoral muscle (Khalil et al., 2021). Overall, GAA has been shown to be an effective modulator of various stressors in commercial chicken production (Nasiroleslami et al., 2018; Khalil et al., 2021).
Ducks
Ducks supplemented with 0.6 g/kg GAA with betaine experience improved growth performance and meat quality (Shuzhen et al., 2021). Other studies have evaluated GAA’s effectiveness when supplemented with additional methionine to provide both precursors for creatine synthesis. Dietary GAA supplementation (0.5 g/kg) with methionine (0.4%) improved body weight, daily weight gain, and the feed to gain ratio in ducks (Ibrahim et al., 2019). Moreover, overall performance in ducks was more enhanced by GAA with 0.4% methionine supplementation, than by creatine supplementation. European Food Safety Authority (2022) suggests 0.5 g GAA/kg feed is efficacious in improving the performance of ducks for fattening.
Swine
Several studies have evaluated GAA’s effect on growth performance in pigs. In growing-finishing pigs supplemented with GAA, the average daily gain and gain to feed ratio increased with increasing dietary GAA dose, and a GAA dosage of 0.3 g/kg was considered the optimum concentration for maximizing growth performance by increasing muscle creatine and ATP concentrations in pigs (He et al., 2018). Pigs with 1.2 g/kg GAA had better growth and lean meat yield from weaning to finishing ages. Finishing pigs supplemented with 1.2 g/kg GAA for 60 days before slaughter had increased average daily gain, feed efficiency, and lean meat yield than those fed unsupplemented diets (Jayaraman et al., 2018). Dietary GAA supplementation increased creatine and phosphocreatine loading in finishing pigs while improving their growth performance (Li et al., 2018). In piglets, GAA supplementation at 1.2 g/kg had a minimal effect on the average daily gain at weaning (Pedersen et al., 2021). Moreover, nursery piglets supplemented with 2.4 g/kg GAA had improved FCR and activated molecular mechanisms important for lean mass gain compared to the control group (Valini et al., 2020). According to the European Food Safety Authority (2022), 0.3 – 1.2 g/kg of GAA has positive effects on the growing pigs. These studies suggest GAA could be an efficient feed additive as a precursor of creatine for improving growth performance in growing-finishing pigs. In contrast, Wang et al. (2012) found no effect of GAA supplementation (0.8-2 g/kg for 54 days) on average daily gain, feed to gain ratio or daily feed intake in growing-finishing pigs. This difference might be due to the lesser concentrations of protein and methionine in the diet in the study by Wang et al. (2012). These inconsistent findings suggest the necessity of more performance studies to evaluate the effectiveness of GAA in swine. In summary, most studies have demonstrated the effectiveness of GAA supplementation in enhancing FCR, growth performance, and energy utilization in swine and poultry. Moreover, GAA supplementation with methionine can also lead to positive growth outcomes and, as such, should be investigated further given its role in ensuring complete conversion of GAA to creatine.
Effects of GAA supplementation on meat quality
Given its role in muscle metabolism, GAA supplements have been considered as a potential feed additive to improve meat quality in the animal industry. Many studies have evaluated the effects of GAA supplements on meat pH, meat yield, and other carcass traits in poultry and swine. Dietary GAA of 1.8 g/kg increased meat pH in broiler chickens, improving meat quality (Mohebbifar et al., 2019). Furthermore, dietary supplementation with GAA enhanced creatine concentrations in breast meat in broilers, which led to greater energy provision for muscle performance and growth (Michiels et al., 2012). For example, GAA supplementation (0.31 – 1.26 g/kg) linearly increased breast muscle creatine concentration in broiler males (Lemme et al., 2007b; Ringel et al., 2007), while muscle GAA concentration decreased with increasing GAA intake (Lemme et al., 2007b; Tossenberger et al., 2016). Overall, based on available studies and according to the European Food Safety Authority, GAA’s dietary inclusion can lead to increased creatine and ATP concentration in chicken breast muscle (Lemme et al., 2007b; European Food Safety Authority, 2009; Tossenberger et al., 2016).
However, whether these GAA supplement-induced increased concentrations of tissue creatine and ATP translate to greater meat yield is still unclear. Although some studies have found that breast muscle yield has been enhanced by the addition of dietary GAA in broilers (Lemme et al., 2007a; Michiels et al., 2012; Esser et al., 2017; Córdova-Noboa et al., 2018a), several researchers found that GAA supplementation did not affect breast muscle yield in broiler chickens (Ringel et al., 2007; Mousavi et al., 2013; Tossenberger et al., 2016). Moreover, there are inconsistent findings on the effects of GAA and meat quality. For example, some studies found that GAA supplementation did not have any significant effect on meat quality (Esser et al., 2017), carcass yield percentage (Abudabos et al., 2014) and carcass traits (Majdeddin et al., 2020) in broilers, such as post-mortem pH and shear force (Michiels et al., 2012; Tossenberger et al., 2016; Esser et al., 2018). On the other hand, GAA’s effectiveness on meat quality may be enhanced with supplemental amino acid sources. More specifically, supplemental GAA with greater concentration of methionine (4 g/kg feed) enhanced carcass and breast muscle yield, meat pH, and plasma and muscle creatine concentrations in ducks to a greater extent than with creatine supplementation (Ibrahim et al., 2019). Taken together, the evidence suggests that supplemental GAA may be an effective alternative for creatine supplements to enhance creatine concentrations, but evidence showing the benefits of GAA on meat quality in poultry is equivocal.
Researchers suggest that GAA provision shortly before slaughter positively affects meat quality. Many studies have evaluated the proper timing for GAA administration to improve carcass characteristics in broilers. Dietary GAA supplementation before slaughtering was found to increase the content of accessible energy sources such as phosphocreatine (PCr) and ATP, which helps to delay glycogen conversion to lactic acid and, as a result, maintains a post-mortem pH value. Moreover, dietary treatment with 1.2 g/kg GAA improved meat quality in broilers subjected to a 3-hour pre-slaughter transit by reducing muscular energy expenditure and delaying anaerobic glycolysis. Compared to broilers subjected to 3-hour transit stress, those fed 0.6 g/kg GAA and 1.2 g/kg GAA for two weeks before slaughtering exhibited reduced drip loss and L*values and a greater pH 24-h value (Zhang et al., 2021). In contrast, growth performance, carcass characteristics, and chemical composition of the muscles were unaffected by dietary supplementation with GAA at 0.6 and 1.2 g/kg for 14 days before slaughter (Zhang et al., 2019). These inconsistent findings should direct future researchers to explore in depth how dietary GAA affects meat quality.
Few studies have evaluated the impact of dietary GAA on carcass traits of swine. Dietary GAA enhances hot carcass weight, carcass length, and lean percentage in pigs, suggesting GAA can be a potential feed additive to enhance carcass quality in pigs (He et al., 2018). When growing-finishing pigs were fed with increasing concentrations of supplemental GAA, there was a linear increase in pH value and improvement of pork quality (Wang et al., 2012). Similarly, Liu et al. (2015) found that supplemental GAA led to reduced lactic acid, increased muscle pH, reduced drip loss, cooking loss, and shear force. These data demonstrate the beneficial effects of dietary GAA on pork quality. Many researchers have proposed the connection between supplemental GAA and better-quality meat, and a detailed rationale can be found in Liu et al. (2015). When animals get slaughtered, anaerobic glycolysis starts as the tissue oxygen concentration depletes slowly. With lactic acid production, muscle pH rapidly declines, consequently leading to protein denaturation. Providing GAA supplements during the pre-slaughter process can minimize the pH drop as GAA enhances the availability of immediate energy sources and delays lactic acid production (Liu et al., 2015).
Moreover, by modifying muscle fibre properties and lowering mandibular fat index in finishing gilts, GAA can improve carcass characteristics and meat quality in growing-finishing pigs (Zhu et al., 2020). Furthermore, GAA improved meat quality by increasing protein solubility and calpain 1 (CAPN1) mRNA expression and enhancing the pH value, water-holding capacity, and tenderness (Li et al., 2018). By altering myogenic gene expression and myofibre characteristics, dietary GAA supplementation (0.6 g/kg) enhanced skeletal muscle development (Lu et al., 2020). Therefore, supplemental GAA can be suggested as a beneficial supplement to improve meat quality in the swine industry (Liu et al., 2015).
GAA supplementation and reproduction
As a precursor for creatine, GAA also improves energy metabolism in the reproductive system. Several studies have suggested that dietary GAA can enhance reproductive performance in poultry and swine. Supplemental GAA can be used to enhance the fertility rate and sperm penetration in aged broiler breeder hens, possibly by increasing ATP availability in sperm mitochondria, thereby increasing sperm motility and fertility rate (Sharideh et al., 2016). By providing different concentrations of dietary GAA, Tapeh et al. (2017) similarly found that GAA increases semen quality and fertility rate in broiler breeder roosters (Tapeh et al., 2017). Furthermore, in meat-type quail breeders, dietary GAA positively affected creatine levels in eggs and muscle tissue of progeny (Murakami et al., 2014). Supplemental GAA (1.71 and 1.14 g/kg, respectively) addition to a corn-soybean meal based diet reduced egg weight and increased luteinising hormone (LH) and follicle stimulating hormone (FSH) concentrations, compared to control groups. Adding GAA to the diet does not seem to be an effective technique for boosting laying hen performance (Khakran et al., 2018). When laying quails were fed varied doses of dietary GAA supplementation (0, 0.6, 1.2, and 1.8 g/kg), the greatest laying rate was attained with 1.8 g/kg of GAA, while the optimum egg weight, egg mass, shell, and yolk weight was recorded with 1.2 g/kg of GAA (Raei et al., 2020). When laying hens were fed a reduced protein diet with supplemental GAA, egg production and feed intake decreased compared to the control diet (Dao et al., 2021). Supplementing GAA (1.5 g/kg) in broiler breeder feed improved its absorption and deposition into hatching eggs, altering GAA and creatine absorption and synthesis within broiler progeny (Reicher et al., 2020). Moreover, adding 0.6 g/kg GAA to the feed of hens during the post-hatch period or injecting eggs with a 1% arginine solution could increase growth rates in low-temperature post-hatch environments Miri et al., 2022). In laying hens, dietary GAA supplementation at 1.0 or 1.5 g/kg may increase laying performance, antioxidant activity, and cellular energy metabolism status (Salah et al., 2020). In male birds, dietary GAA supplementation (1.30-1.45 g/kg GAA) influences testicular histology and the expression of several spermatogonia mitotic and survival markers. Increased spermatogenesis and improved seminal quality and reproductive features in aged broiler breeder roosters could result in these GAA-driven changes (Nasirikhah et al., 2019). Therefore, according to these overall consistent findings, GAA can be used as a potential supplement to avoid age-related reproductive deficits and improve progeny and reproductive performance in poultry.
In swine, feeding GAA during gestation and lactation improves multiparity and gilt performance, milk quality, and production, as well as litter growth performance (Panisson et al., 2019). In contrast, Mendonca et al. (2019) showed that supplementing sows and their offspring with 1 g/kg GAA had no impact on the growth performance during the nursery phase. However, more studies need to be carried out to confirm the effectiveness of GAA as a feed additive to improve reproductive parameters in swine.
GAA supplementation and brain functions
Given creatine’s well-described role in brain function, the role of GAA in brain metabolism has generated interest. Dietary GAA inclusion may have significant effects on creatine and GAA concentrations in brain tissue. If supplemental GAA increases brain creatine concentration, then this newly synthesized creatine may be utilized to enhance energy metabolism in the brain. Whether supplementation of GAA translates to cognitive function has been studied in swine. For example, daily GAA supplementation (157 mg/kg body weight) for two weeks did not improve memory performance, as no relationship was found between GAA and spatial memory in Yucatan miniature pigs (Robinson et al., 2020). Nevertheless, supplemental GAA increased the cerebellum’s GAA concentration and led to increased creatine and decreased GAA concentration in the prefrontal cortex (PFC) in these pigs. Furthermore, GAA supplementation enhanced the creatine kinase activity in the PFC, suggesting the PFC and the cerebellum are significant sites for creatine metabolism in pigs. Although there was a positive association between GAA concentration in the PFC and creatine concentration in the cerebellum with memory performance (Robinson et al., 2020), these concentrations were unrelated to supplementation. Given the positive effects of tissue GAA and creatine on memory, further studies are required to establish a supplementation regimen that can enhance these concentrations. More work is needed in this area, given these exciting recent data.
GAA metabolism
GAA and arginine metabolism
Dietary GAA supplementation may exert its effects by sparing arginine for health and growth-promoting functions, independent of its role in synthesizing creatine. Arginine is an essential amino acid in poultry and conditionally essential in neonatal pigs. Arginine has been shown to improve functional health and growth performance in many animals (Wu et al., 2009; Wu et al., 2016; Yu et al., 2018). Moreover, creatine synthesis consumes a significant amount of arginine; indeed, the equivalent of ~20% of dietary arginine intake is used for GAA synthesis in suckling piglets, in spite of significant creatine in milk (Brosnan et al., 2009). In the poultry industry, arginine has been used as a supplement to enhance live performance (Yu et al., 2018). Several studies have shown that GAA can be used as an arginine-sparing agent in animals. Broilers fed an arginine-deficient diet with supplemental GAA (1.2 g/kg) had improved growth, feed efficiency, and growth performance, demonstrating that GAA supplementation can be used as a potential arginine replacing agent in young chicks (Dilger et al., 2013). Moreover, another study in broiler chickens showed that dietary GAA can act as an arginine-sparing agent while decreasing mortality related to ascites (Mohebbifar et al., 2019). Increased dietary GAA concentration (0.6 and 1.2 g/kg) in broilers subjected to heat stress resulted with greater plasma arginine (Majdeddin et al., 2018). This shows that arginine is more readily available for metabolic processes other than de novo GAA production (Majdeddin et al., 2018). Dietary GAA can be utilized to substitute 150% of arginine in broiler diets with a moderately low crude protein to achieve comparable growth, meat quality, and creatine production (Sharma et al., 2022). Greater replacement rates may be considered for improved feed efficiency and increased muscle creatine deposition (Sharma et al., 2022). When broiler chicks were fed either arginine-deficient or arginine-adequate diets, GAA is capable of sparing dietary arginine (DeGroot et al., 2018). These findings show that supplementing with 1.2 g/kg GAA is the most effective way to boost growth performance (DeGroot et al., 2018; DeGroot et al., 2019). In broiler chickens subjected to hypobaric hypoxia, arginine promotes growth and avoids right ventricular hypertrophy (RVH). Supplementing with GAA at a 1.5 g/kg dose demonstrated the enhanced growth and RVH response (Ahmadipour et al., 2018). Meanwhile, increasing the amount of GAA in the diet beyond 1.5 g/kg may reduce these effects (Ahmadipour et al., 2018).
In piglets, GAA did not spare arginine for whole body protein synthesis, although these diets were not limiting in arginine (Dinesh et al., 2021). Otherwise, no other studies (to our knowledge) have been conducted in pigs on the sparing effect of GAA on arginine, although the concept is feasible given arginine’s positive effects on protein synthesis, nitric oxide (NO) production, and growth hormone release (Ostojic, 2015). To better evaluate the arginine-sparing effects of GAA, future research should focus on dose-dependent changes in L-arginine:glycine aminotransferase enzyme activity (DeGroot et al., 2019).
GAA and methionine metabolism
The conversion of GAA to creatine requires ~70% of the labile methyl groups in humans, which is the highest requirement among transmethylation reactions (Stead et al., 2006). Moreover, because GAMT has no feedback regulation (Da Silva et al., 2009; McBreairty et al., 2013), supplemental GAA will proportionately increase the consumption of methyl groups from SAM to produce more creatine. Consequently, with GAA supplementation, a low availability of methyl groups may interfere with other important methylation reactions competing for SAM, such as DNA methylation and conversion of phosphatidylethanolamine to phosphatidylcholine (PC). Moreover, increased utilization of methyl groups may reduce available methionine for protein synthesis as well. For example, in both suckling and weaned Yucatan miniature pigs, intraportal infusion (McBreairty et al., 2013) or daily feeding (McBreairty et al., 2015) of GAA significantly increased creatine synthesis, while reducing methionine incorporation into hepatic protein and methyl incorporation into PC.
Methylated DNA is another transmethylated product that is quantitatively minor but critical with respect to epigenetic regulation of genes (Randunu and Bertolo, 2020). Few researchers have evaluated the relationship between GAA supplementation and DNA methylation. In young pigs, McBreairty et al. (2015) showed that supplementation of GAA for 18-19 days did not change methyl incorporation rate into DNA, in spite of ~4-fold greater use of methyl groups to convert supplemented GAA to creatine, diverting methyl groups from PC synthesis, which was ~80% lesser. These data suggest that methyl groups were not significantly diverted from DNA methylation by the increased conversion of GAA to creatine.
Supplemental GAA also has significant effects on other metabolites and enzymes in the methionine cycle. For example, because GAA is rapidly transmethylated to creatine, GAA supplementation in rats (5 and 10 g/kg) significantly reduces hepatic SAM concentration and increases SAH concentration, which increases cystathionine-β-synthase (CBS) activity to remove homocysteine (Fukada et al., 2006). CBS is likely induced to remove greater homocysteine concentrations. Interestingly, although GAA supplementation increases methionine synthase activity in rats (Stead et al., 2001), remethylation via the alternate pathway, hepatic betaine-homocysteine methyltransferase (BHMT), is likely reduced with GAA supplementation due to lesser enzyme activity (Liu et al., 2011). According to Liu et al. (2011), dietary supplementation of GAA decreases hepatic betaine in rats by increasing betaine consumption due to the accelerated methionine cycle. Moreover, a low concentration of SAM may reduce PC synthesis via the PEMT pathway, or a greater concentration of SAH may inhibit transmethylation reactions (Liu et al., 2011). Although enhanced GAA methylation to creatine generates excess homocysteine, it is possible that insulin is involved in the various changes to the methionine cycle. For example, GAA supplementation can lead to increased insulin concentration along with decreased homocysteine concentration (Ostojic, 2014). Guanidinoacetate may stimulate insulin secretion directly, which may positively affect glycine N-methyltransferase (GNMT) activity, which is known as one of the main regulatory enzymes in methyl group metabolism (Ostojic, 2014). Nevertheless, more studies are required to understand the exact mechanisms behind how GAA affects methionine cycle flux, which in turn affects metabolite concentrations.
Although methionine metabolism is tightly connected to GAA metabolism, only a few studies have examined the effects of dietary methionine and GAA in poultry or swine. For example, in finishing broilers, the effects of dietary GAA supplementation were influenced by the dietary methionine concentration, indicating the importance of adequate sulfur amino acid composition in broiler diets when feeding GAA (Majdeddin et al., 2019). Furthermore, dietary total sulfur amino acid concentrations influenced the effects of dietary GAA supplementation in growing broilers, demonstrating that dietary sulfur amino acid composition determines the effectiveness of GAA supplementation (Zarghi et al., 2020). However, more studies are needed to evaluate the amount of methionine needed to enhance the effectiveness of GAA supplementation in poultry and swine.
Conclusion
As highlighted in this review, research on GAA supplementation has widely expanded in recent years. GAA has been demonstrated to be a safe feed additive or dietary supplement in the commercial animal industry, especially in swine and poultry. Amongst its many demonstrated benefits, GAA, likely via conversion to creatine, promotes growth, physical performance, reproductive parameters, and meat quality, while some non-creatine roles are also evident, but require further research. Although several studies have addressed GAA transport mechanisms in the brain, GAA absorption and transport across the gut are not fully understood and need to be elucidated to fully understand the fate of GAA supplementation. Moreover, more information is needed on the interaction between GAA supplements and dietary methionine and creatine, which can both enhance overall performance. Taking all together, GAA appears to be an effective and safe supplement in animals, and future studies addressing the above issues will further promote GAA use and more clearly target specific functional benefits.
Author contributions
Conceptualization, MA, RB; writing—original draft preparation, MA; writing—review and editing, RB; supervision, RB; funding acquisition, RB. All authors have read and agreed to the published version of the manuscript.
Funding
This research was funded by Natural Sciences and Engineering Research Council of Canada (RB).
Conflict of interest
The authors declare that the research was conducted in the absence of any commercial or financial relationships that could be construed as a potential conflict of interest.
Publisher’s note
All claims expressed in this article are solely those of the authors and do not necessarily represent those of their affiliated organizations, or those of the publisher, the editors and the reviewers. Any product that may be evaluated in this article, or claim that may be made by its manufacturer, is not guaranteed or endorsed by the publisher.
References
Abudabos A. M., Saleh F., Lemme A., Zakaria H. A. (2014). The relationship between guanidinoacetic acid and metabolisable energy level of diets on performance of broiler chickens. Ital. J. Anim. Sci. 13 (3), 3269. doi: 10.4081/ijas.2014.3269
Ahmadipour B., Khajali F., Sharifi M. R. (2018). Effect of guanidinoacetic acid supplementation on growth performance and gut morphology in broiler chickens. Poult. Sci. J. 6 (1), 19–24. doi: 10.22069/PSJ.2018.13854.1279
Ahmadipour B., Naeini S. Z., Sharifi M., Khajali F. (2018). Growth performance and right ventricular hypertrophy responses of broiler chickens to guanidinoacetic acid supplementation under hypobaric hypoxia. J. Poult. Sci. 55 (1), 60–64. doi: 10.2141/jpsa.0170044
Alraddadi E. A., Lillico R., Vennerstrom J. L., Lakowski T. M., Miller D. W. (2018). Absolute oral bioavailability of creatine monohydrate in rats: debunking a myth. Pharmaceutics 10 (1), 31. doi: 10.3390/pharmaceutics10010031
Amiri M., Ghasemi H. A., Hajkhodadadi I., Farahani A. H. K. (2019). Efficacy of guanidinoacetic acid at different dietary crude protein levels on growth performance, stress indicators, antioxidant status, and intestinal morphology in broiler chickens subjected to cyclic heat stress. Anim. Feed Sci. Technol. 254, 114208. doi: 10.1016/j.anifeedsci.2019.114208
Baker D. H. (2009). Advances in protein-amino acid nutrition of poultry. Amino Acids 37, 29–41. doi: 10.1007/s00726-008-0198-3
Boney J. W., Patterson P. H., Solis F. (2020). The effect of dietary inclusions of guanidinoacetic acid on D1-42 broiler performance and processing yields. J. Appl. Poult. Res. 29 (1), 220–228. doi: 10.1016/j.japr.2019.10.008
Borges K. M., de C Mello H. H., Café M. B., Arnhold E., Xavier H. P., de Oliveira H. F., et al. (2021). Effect of dietary inclusion of guanidinoacetic acid on broiler performance. Rev. Colomb. Cienc. Pecu. 34 (2), 95–104. doi: 10.17533/udea.rccp.v34n2a02
Boroumandnia Z., Khosravinia H., Masouri B., Parizadian Kavan B. (2021). Effects of dietary supplementation of guanidinoacetic acid on physiological response of broiler chicken exposed to repeated lactic acid injection. Ital. J. Anim. Sci. 20 (1), 153–162. doi: 10.1080/1828051X.2021.1873075
Borsook M. E., Borsook H. (1951). Treatment of cardiac decompensation with betaine and glycocyamine. Ann. West. Med. Surg. 5 (10), 830–855.
Braissant O., Henry H. (2008). AGAT, GAMT and SLC6A8 distribution in the central nervous system, in relation to creatine deficiency syndromes: a review. J. Inherit. Metab. Dis. 31 (2), 230–239. doi: 10.1007/s10545-008-0826-9
Brosnan J. T., Wijekoon E. P., Warford-Woolgar L., Trottier N. L., Brosnan M. E., Brunton J. A., et al. (2009). Creatine synthesis is a major metabolic process in neonatal piglets and has important implications for amino acid metabolism and methyl balance. J. Nutr. 139 (7), 1292–1297. doi: 10.3945/jn.109.105411
Çenesiz A. A., Yavaş İ., Çiftci İ., Ceylan N., Taşkesen H. O. (2020). Guanidinoacetic acid supplementation is favourable to broiler diets even containing poultry by-product meal. Br. Poult. Sci. 61 (3), 311–319. doi: 10.1080/00071668.2020.1720909
Ceylan N., Koca S., Adabi S. G., Adabi S. G., Kahraman N., Bhaya M. N., et al. (2021). Effects of dietary energy level and guanidino acetic acid supplementation on growth performance, carcass quality and intestinal architecture of broilers. Czech J. Anim. Sci. 66 (7), 281–291. doi: 10.17221/11/2021-CJAS
Córdova-Noboa H. A., Oviedo-Rondón E. O., Sarsour A. H., Barnes J., Ferzola P., Rademacher-Heilshorn M., et al. (2018b). Performance, meat quality, and pectoral myopathies of broilers fed either corn or sorghum-based diets supplemented with guanidinoacetic acid. Poult. Sci. 97 (7), 2479–2493. doi: 10.3382/ps/pey096
Córdova-Noboa H. A., Oviedo-Rondón E. O., Sarsour A. H., Barnes J., Sapcota D., López D., et al. (2018a). Effect of guanidinoacetic acid supplementation on live performance, meat quality, pectoral myopathies and blood parameters of male broilers fed corn-based diets with or without poultry by-products. Poult. Sci. 97 (7), 2494–2505. doi: 10.3382/ps/pey096
Dao H. T., Sharma N. K., Bradbury E. J., Swick R. A. (2021). Response of laying hens to l-arginine, l-citrulline and guanidinoacetic acid supplementation in reduced protein diet. Anim. Nutr. 7 (2), 460–471. doi: 10.1016/j.aninu.2020.09.004
Da Silva R. P., Nissim I., Brosnan M. E., Brosnan J. T. (2009). Creatine synthesis: hepatic metabolism of guanidinoacetate and creatine in the rat in vitro and in vivo. Am. J. Physiol. Endocrinol. Metab. 296 (2), 256–261. doi: 10.1152/ajpendo.90547.2008
DeGroot A. A., Braun U., Dilger R. N. (2018). Efficacy of guanidinoacetic acid on growth and muscle energy metabolism in broiler chicks receiving arginine-deficient diets. Poult. Sci. 97 (3), 890–900. doi: 10.3382/ps/pex378
DeGroot A. A., Braun U., Dilger R. N. (2019). Guanidinoacetic acid is efficacious in improving growth performance and muscle energy homeostasis in broiler chicks fed arginine-deficient or arginine-adequate diets. Poult. Sci. 98 (7), 2896–2905. doi: 10.3382/ps/pez036
de Souza C., Eyng C., Viott A. M., de Avila A. S., Pacheco W. J., Junior N. R., et al. (2021). Effect of dietary guanidinoacetic acid or nucleotides supplementation on growth performances, carcass traits, meat quality and occurrence of myopathies in broilers. Livest. Sci. 251, 104659. doi: 10.1016/j.livsci.2021.104659
Dilger R. N., Bryant-Angeloni K., Payne R. L., Lemme A., Parsons C. M. (2013). Dietary guanidino acetic acid is an efficacious replacement for arginine for young chicks. Poult. Sci. 92 (1), 171–177. doi: 10.3382/ps.2012-02425
Dinesh O. C., Brunton J. A., Bertolo R. F. (2020). The kidneys are quantitatively more important than pancreas and gut as a source of guanidinoacetic acid for hepatic creatine synthesis in sow-reared Yucatan miniature piglets. J. Nutr. 150 (3), 443–449. doi: 10.1093/jn/nxz266
Dinesh O. C., Kankayaliyan T., Rademacher M., Tomlinson C., Bertolo R. F., Brunton J. A. (2021). Neonatal piglets can synthesize adequate creatine, but only with sufficient dietary arginine and methionine, or with guanidinoacetate and excess methionine. J. Nutr. 151 (3), 531–539. doi: 10.1093/jn/nxaa369
Edison E. E., Brosnan M. E., Meyer C., Brosnan J. T. (2007). Creatine synthesis: production of guanidinoacetate by the rat and human kidney in vivo. Am. J. Physiol. Renal Physiol. 293, 1799–1804. doi: 10.1152/ajprenal.00356.2007
EFSA Panel on Additives and Products or Substances used in Animal Feed (FEEDAP), Bampidis V., Azimonti G., Bastos M. D. L., Christensen H., Dusemund B., et al. (2022). Safety and efficacy of a feed additive consisting of guanidinoacetic acid for all animal species (Alzchem trostberg GmbH). EFSA J. 20 (5), 07269. doi: 10.2903/j.efsa.2022.7269
Esser A. F. G., Gonçalves D. R. M., Rorig A., Cristo A. B., Perini R., Fernandes J. I. M. (2017). Effects of guanidionoacetic acid and arginine supplementation to vegetable diets fed to broiler chickens subjected to heat stress before slaughter. Braz. J. Poultry Sci. 19, 429–436. doi: 10.1590/1806-9061-2016-0392
Esser A. F. G., Taniguti T. L., da Silva A. M., Vanroo E., Kaneko I. N., dos Santos T. C., et al. (2018). Effect of supplementation of guanidinoacetic acid and arginine in vegetable diets for broiler on performance, carcass yield and meat quality. Semina Cienc. Agrar. 39 (3), 1307–1318. doi: 10.5433/1679-0359.2018v39n3p1307
European Food Safety Authority (EFSA) (2009). Safety and efficacy of guanidinoacetic acid as feed additive for chickens for fattening. EFSA J. 7 (3), 988. doi: 10.2903/j.efsa.2009.988
Faraji M., Karimi Dehkordi S., Zamiani Moghadam A. K., Ahmadipour B., Khajali F. (2019). Combined effects of guanidinoacetic acid, coenzyme Q10 and taurine on growth performance, gene expression and ascites mortality in broiler chickens. J. Anim. Physiol. Anim. Nutr. 103 (1), 162–169. doi: 10.1111/jpn.13020
Fosoul S. S. A. S., Azarfar A., Gheisari A., Khosravinia H. (2018). Energy utilisation of broiler chickens in response to guanidinoacetic acid supplementation in diets with various energy contents. Br. J. Nutr. 120 (2), 131–140. doi: 10.1017/S0007114517003701
Fukada S. I., Setoue M., Morita T., Sugiyama K. (2006). Dietary eritadenine suppresses guanidinoacetic acid-induced hyperhomocysteinemia in rats. J. Nutr. 136 (11), 2797–2802. doi: 10.1093/jn/136.11.2797
Hanna-El-Daher L., Braissant O. (2016). Creatine synthesis and exchanges between brain cells: what can be learned from human creatine deficiencies and various experimental models? Amino Acids 48 (8), 1877–1895. doi: 10.1007/s00726-016-2189-0
He D. T., Gai X. R., Yang L. B., Li J. T., Lai W. Q., Sun X. L., et al. (2018). Effects of guanidinoacetic acid on growth performance, creatine and energy metabolism, and carcass characteristics in growing-finishing pigs. J. Anim. Sci. 96 (8), 3264–3273. doi: 10.1093/jas/sky186
Heger J., Zelenka J., Machander V., de la Cruz C., Lešták M., Hampel D. (2014). Effects of guanidinoacetic acid supplementation to broiler diets with varying energy content. Acta Univ. Agric. Silvic. Mendelianae Brun. 62 (3), 477–485. doi: 10.11118/actaun201462030477
He D., Yang L., Li J., Dong B., Lai W., Zhang L. (2019). Effects of guanidinoacetic acid on growth performance, creatine metabolism and plasma amino acid profile in broilers. J. Anim. Physiol. Anim. Nutr. 103 (3), 766–773. doi: 10.1111/jpn.13081
Ibrahim D., El Sayed R., Abdelfattah-Hassan A., Morshedy A. M. (2019). Creatine or guanidinoacetic acid? which is more effective at enhancing growth, tissue creatine stores, quality of meat, and genes controlling growth/myogenesis in mulard ducks. J. Appl. Anim. Res. 47 (1), 159–166. doi: 10.1080/09712119.2019.1590205
İpek A., Sahan U. (2006). Effects of cold stress on broiler performance and ascites susceptibility. Asian-australas. J. Anim. Sci. 19 (5), 734–738. doi: 10.5713/ajas.2006.734
Jayaraman B., La K. V., La H., Doan V., Carpena E. M., Rademacher M., et al. (2018). Supplementation of guanidinoacetic acid to pig diets: effects on performance, carcass characteristics, and meat quality. J. Anim. Sci. 96 (6), 2332–2341. doi: 10.1093/jas/sky137
Khakran G., Chamani M., Foroudi F., Sadeghi A. A., Afshar M. A. (2018). Effect of guanidine acetic acid addition to corn-soybean meal-based diets on productive performance, blood biochemical parameters and reproductive hormones of laying hens. Kafkas. Univ. Vet. Fak. Derg. 24 (1), 99–105. doi: 10.9775/kvfd.2017.18407
Khalil S., Saenbungkhor N., Kesnava K., Sivapirunthep P., Sitthigripong R., Jumanee S., et al. (2021). Effects of guanidinoacetic acid supplementation on productive performance, pectoral myopathies, and meat quality of broiler chickens. Animals 11 (11), 3180. doi: 10.3390/ani11113180
Lemme A., Ringel J., Rostagno H. S., Redshaw M. S. (2007a). “Supplemental guanidino acetic acid improved feed conversion, weight gain, and breast meat yield in male and female broilers,” in Proceedings of the 16th European symposium on poultry nutrition (Beekbergen, Netherlands: World's Poultry Science Association (WPSA)), 335–338.
Lemme A., Ringel J., Sterk A., Young J. F. (2007b). “Supplemental guanidino acetic acid affects energy metabolism of broilers,” in Proceedings of the 16th European symposium on poultry nutrition (Beekbergen, Netherlands: World's Poultry Science Association (WPSA)), 26–30.
Liu Y., Li J. L., Li Y. J., Gao T., Zhang L., Gao F., et al. (2015). Effects of dietary supplementation of guanidinoacetic acid and combination of guanidinoacetic acid and betaine on postmortem glycolysis and meat quality of finishing pigs. Anim. Feed Sci. Technol. 205, 82–89. doi: 10.1016/j.anifeedsci.2015.03.010
Liu Y. Q., Liu Y., Morita T., Sugiyama K. (2011). Methionine and serine synergistically suppress hyperhomocysteinemia induced by choline deficiency, but not by guanidinoacetic acid, in rats fed a low casein diet. Biosci. Biotechnol. Biochem. 75 (12), 2333–2339. doi: 10.1271/bbb.110507
Li J., Zhang L., Fu Y., Li Y., Jiang Y., Zhou G., et al. (2018). Creatine monohydrate and guanidinoacetic acid supplementation affects the growth performance, meat quality, and creatine metabolism of finishing pigs. J. Agric. Food. Chem. 66 (38), 9952–9959. doi: 10.1021/acs.jafc.8b02534
Loike J. D., Zalutsky D. L., Kaback E., Miranda A. F., Silverstein S. C. (1988). Extracellular creatine regulates creatine transport in rat and human muscle cells. Proc. Natl. Acad. Sci. 85 (3), 807–811. doi: 10.1073/pnas.85.3.807
Lu Y., Zou T., Wang Z., Yang J., Li L., Guo X., et al. (2020). Dietary guanidinoacetic acid improves the growth performance and skeletal muscle development of finishing pigs through changing myogenic gene expression and myofiber characteristics. J. Anim. Physiol. Anim. Nutr. 104 (6), 1875–1883. doi: 10.1111/jpn.13351
Majdeddin M., Braun U., Lemme A., Golian A., Kermanshahi H., De Smet S., et al. (2020). Guanidinoacetic acid supplementation improves feed conversion in broilers subjected to heat stress associated with muscle creatine loading and arginine sparing. Poult. Sci. 99 (9), 4442–4453. doi: 10.1016/j.psj.2020.05.023
Majdeddin M., Golian A., Kermanshahi H., De Smet S., Michiels J. (2018). Guanidinoacetic acid supplementation in broiler chickens fed on corn-soybean diets affects performance in the finisher period and energy metabolites in breast muscle independent of diet nutrient density. Br. Poult. Sci. 59 (4), 443–451. doi: 10.1080/00071668.2018.1476678
Majdeddin M., Golian A., Kermanshahi H., Michiels J., De Smet S. (2019). Effects of methionine and guanidinoacetic acid supplementation on performance and energy metabolites in breast muscle of male broiler chickens fed corn-soybean diets. Br. Poult. Sci. 60 (5), 554–563. doi: 10.1080/00071668.2019.1631447
McBreairty L. E., McGowan R. A., Brunton J. A., Bertolo R. F. (2013). Partitioning of [methyl-3H] methionine to methylated products and protein is altered during high methyl demand conditions in young Yucatan miniature pigs. J. Nutr. 143 (6), 804–809. doi: 10.3945/jn.112.172593
McBreairty L. E., Robinson J. L., Furlong K. R., Brunton J. A., Bertolo R. F. (2015). Guanidinoacetate is more effective than creatine at enhancing tissue creatine stores while consequently limiting methionine availability in Yucatan miniature pigs. PloS One 10 (6), 0131563. doi: 10.1371/journal.pone.0131563
Mendonça I. B., Watanabe P. H., Silva B. A. N., Boiago M. M., Panisson J. C., Andrade T. S., et al. (2019). Dietary supplementation of guanidinoacetic acid for sows and their progenies: performance, blood parameters and economic viability at nursery phase. Livest. Sci. 227, 105–110. doi: 10.1016/j.livsci.2019.07.011
Metwally A. E., Abdel-Wareth A. A., Saleh A. A., Amer S. A. (2020). Are the energy matrix values of the different feed additives in broiler chicken diets could be summed? BMC Vet. Res. 16 (1), 1–11. doi: 10.1186/s12917-020-02600-3
Michiels J., Maertens L., Buyse J., Lemme A., Rademacher M., Dierick N. A., et al. (2012). Supplementation of guanidinoacetic acid to broiler diets: effects on performance, carcass characteristics, meat quality, and energy metabolism. Poult. Sci. 91 (2), 402–412. doi: 10.3382/ps.2011-01585
Miri B., Ghasemi H. A., Hajkhodadadi I., Farahani A. H. K. (2022). Effects of low eggshell temperatures during incubation, in ovo feeding of l-arginine, and post-hatch dietary guanidinoacetic acid on hatching traits, performance, and physiological responses of broilers reared at low ambient temperature. Poult. Sci. 101 (1), 101548. doi: 10.1016/j.psj.2021.101548
Mohebbifar A., Torki M., Abdolmohammadi A. (2019). Effects of dietary guanidinoacetic acid supplementation on performance, blood parameters and meat quality of male broilers with cold-induced ascites. Iran. J. Appl. Anim. Sci. 9 (1), 125–133. Available at: https://ijas.rasht.iau.ir/article_663577.html.
Mori A., Kohno M., Masumizu T., Noda Y., Packer L. (1996). Guanidino compounds generate reactive oxygen species. IUBMB Life 40 (1), 135–143. doi: 10.1080/15216549600201622
Mousavi S. N., Afsar A., Lotfollahian H. (2013). Effects of guanidinoacetic acid supplementation to broiler diets with varying energy contents. J. Appl. Poult. Res. 22 (1), 47–54. doi: 10.3382/japr.2012-00575
Murakami A. E., Rodrigueiro R. J. B., Santos T. C., Ospina-Rojas I. C., Rademacher M. (2014). Effects of dietary supplementation of meat-type quail breeders with guanidinoacetic acid on their reproductive parameters and progeny performance. Poult. Sci. 93 (9), 2237–2244. doi: 10.3382/ps.2014-03894
Nasirikhah A., Zhandi M., Shakeri M., Sadeghi M., Ansari M., Deldar H., et al. (2019). Dietary guanidinoacetic acid modulates testicular histology and expression of c-kit and STRA8 genes in roosters. Theriogenology 130, 140–145. doi: 10.1016/j.theriogenology.2019.03.006
Nasiroleslami M., Torki M., Saki A. A., Abdolmohammadi A. R. (2018). Effects of dietary guanidinoacetic acid and betaine supplementation on performance, blood biochemical parameters and antioxidant status of broilers subjected to cold stress. J. Appl. Anim. Res. 46 (1), 1016–1022. doi: 10.1080/09712119.2018.1450751
Nawaz A. H., Amoah K., Leng Q. Y., Zheng J. H., Zhang W. L., Zhang L. (2021). Poultry response to heat stress: Its physiological, metabolic, and genetic implications on meat production and quality including strategies to improve broiler production in a warming world. Front. Vet. Sci. 8, 699081. doi: 10.3389/fvets.2021.699081
Ostojic S. M. (2014). An alternative mechanism for guanidinoacetic acid to affect methylation cycle. Med. Hypotheses. 83 (6), 847–848. doi: 10.1016/j.mehy.2014.11.001
Ostojic S. M. (2015). Advanced physiological roles of guanidinoacetic acid. Eur. J. Nutr. 54 (8), 1211–1215. doi: 10.1007/s00394-015-1050-7
Ostojic S. M. (2017). Co-Administration of creatine and guanidinoacetic acid for augmented tissue bioenergetics: A novel approach? Biomed. Pharmacother. 91, 238–240. doi: 10.1016/j.biopha.2017.04.075
Ostojic S. M., Niess B., Stojanovic M., Obrenovic M. (2013). Creatine metabolism and safety profiles after six-week oral guanidinoacetic acid administration in healthy humans. Int. J. Med. Sci. 10 (2), 141–147. doi: 10.7150/ijms.5125
Ostojic S. M., Vojvodic-Ostojic A. (2015). Single-dose oral guanidinoacetic acid exhibits dose-dependent pharmacokinetics in healthy volunteers. Nutr. Res. 35 (3), 198–205. doi: 10.1016/j.nutres.2014.12.010
Panisson J. C., Silva B. A. N., Oliveira S. G., Maiorka A., Azevedo A. M., Mendonça I. B., et al. (2019). Guanidinoacetic acid: how can sows benefit from this novel feed additive. EAAP Sci. Ser. 138, 8–22. Wageningen Academic Publishers. doi: 10.3920/978-90-8686-891-9_31
Pedersen E. K., Dall J., Amdi C., Madsen J. G. (2021). Guanidinoacetic acid as a feed supplement offered to weaned piglets. Acta Agric. Scand. A. Anim. Sci. 70 (3-4), 145–150. doi: 10.1080/09064702.2021.1984560
Pirgozliev V., Rose S. P., Mirza M. W., Whiting I. M., Malins H., Bauer L., et al. (2022). Feeding guanidinoacetic acid to broiler chickens can compensate for low dietary metabolisable energy formulation. Br. Poult. Sci. 63 (3), 368–374. doi: 10.1080/00071668.2021.2014399
Raei A., Karimi A., Sadeghi A. (2020). Performance, antioxidant status, nutrient retention and serum profile responses of laying Japanese quails to increasing addition levels of dietary guanidinoacetic acid. Ital. J. Anim. Sci. 19 (1), 75–85. doi: 10.1080/1828051X.2019.1698325
Randunu R. S., Bertolo R. F. (2020). The effects of maternal and postnatal dietary methyl nutrients on epigenetic changes that lead to non-communicable diseases in adulthood. Int. J. Mol. Sci. 21 (9), 3290. doi: 10.3390/ijms21093290
Reicher N., Epstein T., Gravitz D., Cahaner A., Rademacher M., Braun U., et al. (2020). From broiler breeder hen feed to the egg and embryo: The molecular effects of guanidinoacetate supplementation on creatine transport and synthesis. Poult. Sci. 99 (7), 3574–3582. doi: 10.1016/j.psj.2020.03.052
Ringel J., Lemme A., Araujo L. F. (2008). The effect of supplemental guanidino acetic acid in Brazilian type broiler diets at summer conditions. Poult. Sci. 87 (1), 154 645.
Ringel J., Lemme A., Knox A., McNab J., Redshaw M. S. (2007). “Effects of graded levels of creatine and guanidino acetic acid in vegetable-based diets on performance and biochemical parameters in muscle tissue,” in Proceedings of the 16th European symposium on poultry nutrition (Beekbergen, Netherlands: World's Poultry Science Association (WPSA)), 387–390. In.
Robinson J. L., McBreairty L. E., Ryan R. A., Randunu R., Walsh C. J., Martin G. M., et al. (2020). Effects of supplemental creatine and guanidinoacetic acid on spatial memory and the brain of weaned Yucatan miniature pigs. PloS One 15 (1), 0226806. doi: 10.1371/journal.pone.0226806
Salah A. S., Ahmed-Farid O. A., El-Tarabany M. S. (2020). Effects of guanidinoacetic acid supplements on laying performance, egg quality, liver nitric oxide and energy metabolism in laying hens at the late stage of production. J. Agric. Sci. 158 (3), 241–246. doi: 10.1017/S0021859620000477
Sharideh H., Esmaeile Neia L., Zaghari M., Zhandi M., Akhlaghi A., Lotfi L. (2016). Effect of feeding guanidinoacetic acid and l-arginine on the fertility rate and sperm penetration in the perivitelline layer of aged broiler breeder hens. J. Anim. Physiol. Anim. Nutr. (Berl) 100 (2), 316–322. doi: 10.1111/jpn.12372
Sharma N. K., Cadogan D. J., Chrystal P. V., McGilchrist P., Wilkinson S. J., Inhuber V., et al. (2022). Guanidinoacetic acid as a partial replacement to arginine with or without betaine in broilers offered moderately low crude protein diets. Poult. Sci. 101 (4), 101692. doi: 10.1016/j.psj.2021.101692
Shuzhen L., Yang L., Wenhuan C., Zhimin C., Aijuan Z., Zedong W., et al. (2021). Supplementation of guanidinoacetic acid and betaine improve growth performance and meat quality of ducks by accelerating energy metabolism. Ital. J. Anim. Sci. 20 (1), 1656–1663. doi: 10.1080/1828051X.2021.1978336
Sosnówka-Czajka E., Skomorucha I. (2022). Sudden death syndrome in broiler chickens: a review on the etiology and prevention of the syndrome. Ann. Anim. Sci. 22 (3), 865–871. doi: 10.2478/aoas-2022-0007
Stead L. M., Au K. P., Jacobs R. L., Brosnan M. E., Brosnan J. T. (2001). Methylation demand and homocysteine metabolism: effects of dietary provision of creatine and guanidinoacetate. Am. J. Physiol. Endocrinol. Metab. 281, 1095–1100. doi: 10.1152/ajpendo.2001.281.5.E1095
Stead L. M., Brosnan J. T., Brosnan M. E., Vance D. E., Jacobs R. L. (2006). Is it time to reevaluate methyl balance in humans? Am. J. Clin. Nutr. 83 (1), 5–10. doi: 10.1093/ajcn/83.1.5
Tachikawa M., Ikeda S., Fujinawa J., Hirose S., Akanuma S. I., Hosoya K. I. (2012). γ-aminobutyric acid transporter 2 mediates the hepatic uptake of guanidinoacetate, the creatine biosynthetic precursor, in rats. PloS One 7 (2), 32557. doi: 10.1371/journal.pone.0032557
Tachikawa M., Kasai Y., Yokoyama R., Fujinawa J., Ganapathy V., Terasaki T., et al. (2009). The blood–brain barrier transport and cerebral distribution of guanidinoacetate in rats: involvement of creatine and taurine transporters. J. Neurochem. 111 (2), 499–509. doi: 10.1111/j.1471-4159.2009.06332.x
Tapeh R. S., Zhandi M., Zaghari M., Akhlaghi A. (2017). Effects of guanidinoacetic acid diet supplementation on semen quality and fertility of broiler breeder roosters. Theriogenology 89, 178–182. doi: 10.1016/j.theriogenology.2016.11.012
Tossenberger J., Rademacher M., Németh K., Halas V., Lemme A. J. P. S. (2016). Digestibility and metabolism of dietary guanidino acetic acid fed to broilers. Poult. Sci. 95 (9), 2058–2067. doi: 10.3382/ps/pew083
Valini G. A. D. C., Duarte M. D. S., Rodrigues G. D. A., Veroneze R., Saraiva A., Hausman G., et al. (2020). Guanidinoacetic acid supplementation on growth performance and molecular mechanisms of lean mass gain in nursery pigs. Cienc. Rural 50 (8), 20190948. doi: 10.1590/0103-8478cr20190948
van der Poel A. F., Braun U., Hendriks W. H., Bosch G. (2019). Stability of creatine monohydrate and guanidinoacetic acid during manufacture (retorting and extrusion) and storage of dog foods. J. Anim. Physiol. Anim. Nutr. 103 (4), 1242–1250. doi: 10.1111/jpn.13103
Wang L. S., Shi B. M., Shan A. S., Zhang Y. Y. (2012). Effects of guanidinoacetic acid on growth performance, meat quality and antioxidation in growing-finishing pigs. J. Anim. Vet. Adv. 11 (5), 631–636. doi: 10.3923/javaa.2012.631.636
Wu G., Bazer F. W., Davis T. A., Kim S. W., Li P., Marc Rhoads J., et al. (2009). Arginine metabolism and nutrition in growth, health and disease. Amino Acids 37 (1), 153–168. doi: 10.1007/s00726-008-0210-y
Wu Z., Hou Y., Hu S., Bazer F. W., Meininger C. J., McNeal C. J., et al. (2016). Catabolism and safety of supplemental l-arginine in animals. Amino Acids 48 (7), 1541–1552. doi: 10.1007/s00726-016-2245-9
Wyss M., Kaddurah-Daouk R. (2000). Creatine and creatinine metabolism. Physiol. Rev. 80, 1107–1213. doi: 10.1152/physrev.2000.80.3.1107
Yu J., Yang H., Wang Z., Dai H., Xu L., Ling C. (2018). Effects of arginine on the growth performance, hormones, digestive organ development and intestinal morphology in the early growth stage of layer chickens. Ital. J. Anim. Sci. 17 (4), 1077–1082. doi: 10.1080/1828051X.2018.1434692
Zarghi H., Golian A., Tabatabaei Yazdi F. (2020). Effect of dietary sulphur amino acid levels and guanidinoacetic acid supplementation on performance, carcase yield and energetic molecular metabolites in broiler chickens fed wheat-soy diets. Ital. J. Anim. Sci. 19 (1), 951–959. doi: 10.1080/1828051X.2020.1809537
Zhang B., Liu N., He Z., Song P., Hao M., Xie Y., et al. (2021). Guanidino-acetic acid: a scarce substance in biomass that can regulate postmortem meat glycolysis of broilers subjected to pre-slaughter transportation. Front. Bioeng. Biotechnol. 8, 1572. doi: 10.3389/fbioe.2020.631194
Zhang L., Li J. L., Wang X. F., Zhu X. D., Gao F., Zhou G. H. (2019). Attenuating effects of guanidinoacetic acid on preslaughter transport-induced muscle energy expenditure and rapid glycolysis of broilers. Poult. Sci. 98 (8), 3223–3232. doi: 10.3382/ps/pez052
Zhao W., Li J., Xing T., Zhang L., Gao F. (2021). Effects of guanidinoacetic acid and complex antioxidant supplementation on growth performance, meat quality, and antioxidant function of broiler chickens. J. Sci. Food Agric. 101 (9), 3961–3968. doi: 10.1002/jsfa.11036
Keywords: dietary supplement, animal nutrition, swine, poultry, guanidinoacetate supplementation
Citation: Asiriwardhana M and Bertolo RF (2022) Guanidinoacetic acid supplementation: A narrative review of its metabolism and effects in swine and poultry. Front. Anim. Sci. 3:972868. doi: 10.3389/fanim.2022.972868
Received: 19 June 2022; Accepted: 23 September 2022;
Published: 11 October 2022.
Edited by:
Otávio R. Machado Neto, São Paulo State University, BrazilReviewed by:
John Michael Gonzalez, University of Georgia, United StatesElijah G. Kiarie, University of Guelph, Canada
Cesar Augusto Pospissil Garbossa, University of São Paulo, Brazil
Copyright © 2022 Asiriwardhana and Bertolo. This is an open-access article distributed under the terms of the Creative Commons Attribution License (CC BY). The use, distribution or reproduction in other forums is permitted, provided the original author(s) and the copyright owner(s) are credited and that the original publication in this journal is cited, in accordance with accepted academic practice. No use, distribution or reproduction is permitted which does not comply with these terms.
*Correspondence: Mahesha Asiriwardhana, masiriwardha@mun.ca; Robert F. Bertolo, rbertolo@mun.ca