- 1Mazingira Centre, International Livestock Research Institute (ILRI), Nairobi, Kenya
- 2Faculty of Agriculture and Veterinary Sciences, University of Melbourne, Melbourne VIC, Australia
- 3Agroscope, Research Division Agroecology and Environment, Integrative Agroecology Group, Zurich, Switzerland
In Africa, cattle are often fed low quality tropical roughages resulting in low-yielding animals with high methane (CH4) emission intensity (EI, g CH4/per unit of product). Supplementation with protein is known to improve the nutritive value of the otherwise low-quality diets. However, animal nutrition studies in East Africa that are accompanied by CH4 emission measurements are lacking. Thus, an animal experiment was conducted to quantify the effect of supplementing cattle fed mainly on low-quality Urochloa brizantha hay (control diet; CON; crude protein (CP) = 7.4%) or supplemented with either a tannin-rich leguminous fodder, Desmodium intortum hay (DES) or a commercial dairy concentrate (CUBES) on voluntary dry matter intake (DMI), nutrient apparent total tract digestibility, nitrogen (N) retention, enteric CH4 production and animal performance (milk and average daily gain). Twelve mid-lactating crossbred (Friesian × Boran) cows (initial liveweight = 335 kg) were used in a 3×3 (Period × Diet) Latin square design with each period running for four weeks. Compared to CON, DES decreased nutrient (DM, OM, CP) intake, apparent total tract digestibility and daily milk yield. In contrast, CUBES increased nutrient intake and animal performance compared to CON, while nutrients’ apparent total tract digestibility was not different, except for CP digestibility that increased. Compared to CON, DES and CUBES improved overall N retention by the animals as a proportion of N intake. The DES diet compared with CON and CUBES, shifted the proportion of N excretion via urine to the fecal route, likely because of its tannin content. Both DES and CUBES, compared to CON, reduced methane yield (MY, g CH4/kg DMI) by 15% and 9%, respectively. The DES diet reduced absolute enteric CH4 emissions by 26% while CUBES increased emissions by 11% compared to CON. Based on the present findings, high supplementation levels (>50%) of Desmodium intortum hay is not recommended especially when the basal diet is low in CP content. Supplementation with lower levels of better managed Desmodium intortum forage however, need to be investigated to establish optimal inclusion levels that will improve animal productivity and reduce environmental impact of livestock in smallholder tropical contexts.
Introduction
One of the main limiting factors to ruminant production in smallholder systems in developing countries is feeding of low-quality forages (Kebreab et al., 2005; Castro-Montoya et al., 2018). In Sub-Saharan Africa, tropical grasses and crop residues form the main diet component for cattle with low supplementation, if any. These high roughage diets are often found to be low in crude protein (CP; <7.5%) and high in fiber (>70%) (Leng, 1990) which compromises their utilization by animals resulting in low animal productivity. Also, such diets are thought to lead to higher enteric methane (CH4) yield (MY; CH4 emitted per unit feed intake (Goopy et al., 2020) and higher CH4 emission intensities (EI; CH4 per unit of product; (Opio et al., 2013; Ericksen and Crane, 2018) compared to animal diets that are of better nutritive value. Earlier studies have, however, shown that supplementing of such low-quality diets with protein concentrates could improve their nutritive value and hence animal production (Koster et al., 1996; Mathis et al., 2000; Shreck et al., 2021).
Protein supplementation is known to increase intake and apparent total tract digestibility of low-quality roughages by cattle (Bohnert et al., 2011) by improving microbial activity in the rumen. The degradation of supplementary protein in the rumen increases the ammonia concentration and this enhances microbial growth and activity because nitrogen is known to be limiting to microbial nourishment with low quality tropical grasses (Detmann et al., 2014). Commercial protein supplements such as soybean meal are commonly used in intensive cattle production, however, these are often inaccessible and/or unaffordable to many smallholder livestock farmers in the Global South (Bakrie et al., 1996; Franzel et al., 2005). Leguminous forage crops and shrubs that are produced on-farm at a lower cost have then over time been promoted as alternative protein sources to ruminants in smallholder systems even though adoption of these feed resources is still quite low (Kebede et al., 2016).
Leguminous fodder crops such as Desmodium spp. and lucerne inherently have higher CP content than most of the tropical grasses (Topps, 1992) and have been used as a supplement in tropical cattle production to improve the feeding value of low quality roughages (Tolera and Sundstol, 2000). Furthermore, most leguminous forages grown in tropical environments are rich in plant secondary compounds (PSC), such as tannins, saponins and essential oils (Topps, 1992). Presence of tannins, both condensed tannins (CT) or hydrolysable tannins (HT) in ruminant diets have been shown to decrease enteric MY and EI (Beauchemin et al., 2007; Williams et al., 2020) and reduced ammonia and nitrous oxide emitted from manure by binding dietary nitrogen (N) and shifting its excretion from the urinary to the less volatile fecal route (Grainger et al., 2009; Korir et al., 2016). With high affinity for protein (Makkar and Singh, 1991), moderate level of tannins in ruminant’s diet has been shown to increase dietary bypass protein which improves protein utilization efficiency (Frutos et al., 2004). The effects of tannins depend on their source and concentration in the diet (Makkar, 2003; Pineiro-Vazquez et al., 2018). Feed intake and nutrient apparent digestibility (DMD) have been observed to increase or remain unaltered at low to moderate concentration of tannins in the diet (1-3% on DM basis; (Kariuki et al., 1999; Tolera and Sundstol, 2000; Mbuthia and Gachuiri, 2003; Pineiro-Vazquez et al., 2018). At high dietary content (>5% on DM basis) however, diet these parameters have often been shown to be reduced and this had been associated with reduced CP availability in the rumen and low palatability (Waghorn, 2008; Ahnert et al., 2015). Studies from East Africa quantifying the potential of locally available tannin-rich leguminous fodder crops on improving the feeding value of low-quality grass-based diets remain scanty and inconclusive, with no research quantifying their enteric CH4 mitigation potential in-vivo. Animal studies with leguminous fodder elsewhere have shown that, in addition to increasing rumen passage rate that minimizes contact time with methanogens and reducing MY (Piñeiro-Vázquez et al., 2018), tannin rich fodder have also been shown to directly exert anti-methanogenic activity in the rumen by reducing methanogen numbers (Beauchemin et al., 2007; Aboagye et al., 2018).
The present experiment was conducted to test whether Desmodium intortum, a locally grown legume, could be a potential alternative supplement to commercial dairy concentrates in smallholder dairy production systems in East Africa. We hypothesized that: i) both Desmodium intortum hay and commercial dairy concentrate supplementation would increase voluntary feed intake, apparent total tract digestibility of nutrients and improve animal production (milk yield and ADG) compared to animals fed a low quality basal diet alone; ii) both Desmodium intortum hay and commercial dairy concentrate supplementation would reduce MY and EI (g CH4/unit of product) compared to feeding a low quality basal diet alone to lactating cows, and iii) Desmodium hay but not dairy concentrate supplementation would reduce absolute enteric CH4 emissions and shift N excretion from the urinary to the fecal route.
Materials and methods
The study was conducted at the Mazingira Centre of the International Livestock Research Institute (ILRI), Nairobi, Kenya. The experimental protocol was reviewed and approved by the Institutional Animal Care and Use Committee (IACUC, Reference number: IACUC 2019-09).
Experimental design and animals used
A 3×3 (Period × Dietary treatment) Latin square design was used. Each experimental period ran for 28 d consisting of 14 d of dietary adaptation, 7 d of separate collection of feces and urine and 7 d of measurements of enteric CH4 emissions.
Twelve crossbred cows (Friesian × Boran) with initial live weights (LW) of 335 ± 14.2 kg (mean ± standard error of mean) in either parity 1 or 2 and in mid-lactation (days in milk; DIM: 119 ± 6.4 d) were included in the experiment. The animals were sourced from ILRI’s Kapiti Research Station situated 60 km South-East of Nairobi, where the animals were maintained extensively on natural pastures in a rangeland ecosystem and were only supplemented with mineral licks. The animals were milked by hand once a day. Selection of the cows was done from a herd of 128 lactating animals. Calves were weaned and the cows moved to ILRI campus 8 d later. On arrival, the animals were quarantined for 21 d in an enclosed animal facility, where they were kept in two group pens (six cows per pen). While in quarantine, the animals were fed on Brachiaria hay alone for the first 3 d. From Day 4, a commercial dairy concentrate was introduced in the animals’ diet to improve their milk production to a level that was considered sensitive to treatments’ effect (> 4 L) before the start of the experiment and thus also minimize chances of the cows drying up midway through the experiment. The dairy concentrate, that was fed during milking that was done once per day in the morning by hand, was gradually introduced to the animals with 2 kg per animal offered in Day 4 and 5 and increased to 3 kg per day from Day 6. The concentrate was formulated from wheat bran, whole maize, maize bran, rice bran, soya bean meal, wheat pollard, sunflower seed cake, stock feed lime and molasses. Two animals that were already at ILRI campus and fitted in the selection criteria were added to this cohort to allow for replacement animals in case an animal would have to be removed from the experiment. The cows were producing on average 1.7 ± 0.48 kg of milk per day at the start of quarantine and gradually increased to a peak of 4.7 ± 0.49 kg by Day 11 of quarantine. From Day 6 of quarantine, a milking machine (Sezer PLS 2, Bursa, Turkey) was introduced and used subsequently instead of hand-milking. We anticipated that the dietary changes introduced during the pre-trial and at the onset of the experiment would have minimal confounding effects on milk production, if any, because the cows used were low yielding animals.
After completion of the 21-d quarantine period, the cows were moved to the experimental animal facilities and housed in individual pens (2 m × 3 m), with each animal fed singly and therefore served as an experimental unit. The pens had the roof covered with shade sails and a concrete floor covered with a rubber mat. The walls were partitioned with aluminum rails. The animals were allocated to one of the three experimental groups (each with four animals), balanced for milk yield, DIM, liveweight and parity. The three dietary treatments were then randomly allocated to the three animal groups for the first period of the experiment and rotated among the animal groups in subsequent periods (Period 2 and 3) such that all animals went through all the dietary treatments. Carryover effect was minimized by assigning two animals from one treatment group to the other treatments in subsequent periods instead of all the four animals being assigned to the same diet, as detailed in Table 1. The average (± SEM) liveweight, milk yield and DIM immediately before the experiment were 325 ± 18, 348 ± 16 and 332 ± 35 kg; 3.6 ± 0.5, 3.6 ± 0.6 and 3.6 ± 1.0 kg; and 118 ± 9, 120 ± 15 and 117 ± 12 d for the three animal groups.
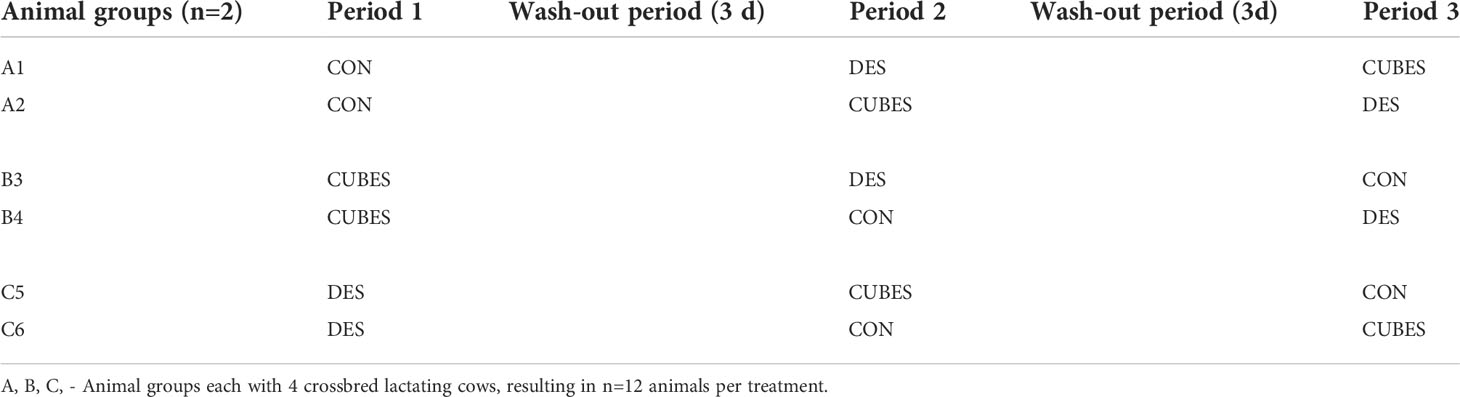
Table 1 Animal groups (A, B, and C) allocation to three the dietary treatments (control: CON; control diet plus Desmodium intortum hay: DES and control diet plus dairy cubes: CUBES) over the three experimental periods.
Experimental diets
Three diets tested in the present study consisted of a low-quality control and two improved rations. The control diet (CON) consisted mainly of grass hay harvested in late bloom (Urochloa brizantha var. Xaraes, further on named Brachiaria hay) while the two improved diets consisted of CON and supplemented either with dairy cubes (commercial protein and energy supplement (Fugo Dairy meal; Unga Feeds Company, Kenya), subsequently named CUBES) or with Desmodium intortum hay (further on named DES). The nutrient density in the two supplemented diets was intended to be isonitrogenous and isocaloric to support the same level of animal production. Due to late harvesting and delay in baling of the Brachiaria hay used, it had only 5.5% CP (% of DM) which was lower than the expected 7-10% (Njarui et al., 2016). Thus, urea was added to the Brachiaria hay to increase the CP content in CON to about 7.5%. The three diets were initially formulated to support a daily milk production of 4 L for the CON and 6 L for the supplemented diets, using an excel-based feed formulation model developed for crossbred animals by Moran (2005). Sugar cane molasses, an energy supplement, was included initially in the formulated diets at 20.0% of the total rations but was reduced to 12.2%, 6.4% and 8.5% for CON, DES and CUBES respectively, after the first 6 d of the experiment. The proportion of molasses included in the ration was reduced to the latter levels because milk yield by the animals peaked at a lower level than anticipated and that the 20% inclusion was already considered marginally high for a ruminant ration and could predispose the cows used to molasses toxicity. Despite these reductions, however, total energy intake by the animals was still sufficient to meet the target level of milk production because the animals’ feed intake was higher than predicted. The proportions of the ingredients used in each experimental diet are shown in Table 2.

Table 2 Proportion (in % DM) of the different feed ingredients used in the formulation of the three experimental diets: control diet consisting mainly of Urochloa brizantha var. Xaraes hay (CON); control diet plus Desmodium intortum hay (DES) and control diet plus commercial dairy supplement (dairy cubes, CUBES).
The molasses, urea and dairy cubes were purchased from local distributors each as a single batch. The grass hay, being the fifth cut since establishment, was harvested from a 2.5 ha Brachiaria plot at ILRI Nairobi campus. Desmodium hay was obtained from eight smallholder farmers in south Nyanza, western Kenya, within a radius of 20 km and similar soil type. Desmodium intortum is a leguminous herb commonly grown in strips in push-pull systems in maize fields in western Kenya to control stem borers and striga weed (Khan et al., 2008). Push-pull is biological strategy for controlling crop pests by intercropping repellant plants; often Desmodium, with a crop (e.g., maize or sorghum) vulnerable to a pest (e.g., stemborer), to keep the pests away from destroying the main crop. Around the perimeter of the production plot, a crop of lower economic value (e.g., grasses) is planted to attract (pull) and trap the pests (Cook et al., 2007). The Desmodium was harvested manually in late vegetative stage, sun dried for 3 d then baled using hand balers and transported to ILRI. Prior to the start of the experiment, all the Desmodium hay was chaffed to a length of about 3-7 cm using a forage chopper, homogenized and stored in plastic sacks. The Brachiaria hay was chaffed on daily basis during the experiment, just before the diets were formulated for the next day.
The diets were prepared daily in the evening by weighing the respective ingredients of each diet according to the proportions in Table 2. The chaffed hay was spread out on a canvas on a concrete floor. Urea was then dissolved in two parts of water and mixed with molasses before the mixture was sprinkled over the hay and thoroughly mixed by hand. The prepared feed was then stored overnight in covered plastic bins separately per treatment until when it was fed to individual animals.
Feeding and milking
The amounts of feed offered to each animal were determined daily as 105% of the previous day’s intake to allow ad libitum feed intake with minimal allowance for selection. The ration for each animal was weighed into plastic bags in the morning and fed out in four tranches spread throughout the day to minimize spillages and soiling of the feed.
Throughout the experimental period, milking was done in the morning starting at 0700 h using a double cow milking machine while the animals were restrained in crushes. Milk output from each animal was then weighed on a digital platform scale (Model JS00-1, Shanghai Puchun Measure Instrument Co., Ltd. Shanghai, China, precision = 1 g) and the milk yields (in kg) were recorded.
Intake and liveweight measurements
Daily feed intake by the animals was determined as the difference between the total feed offered and the refusals collected the following morning over the 28 d in each period. The refusals from each animal were collected every morning, weights taken and pooled per treatment because these were too low for the selection differences from animal to animal to cause any meaningful differences in nutrients intake by the animals. A sub-sample (~300 g) from each treatment pool was then picked, stored in labeled paper bags and kept at -20°C. Every second day, a sample of the feed offered (~300 g as fed) from each dietary treatment was collected and handled as described for the refusals’ samples. At the end of each period, the stored feed and refusal samples were then thawed and pooled within treatment per week (n=4 for rations and refusals separately) from which, subsample were transferred to pre-labeled paper bags and dried in an air-forced oven at 50°C for 96 h.
Liveweight was recorded twice a week before milking and morning feeding, except that during the enteric CH4 and total urine and feces collection periods, animals were weighed only once a week. Liveweight was measured using a digital animal weighing bridge (Gallagher Weigh Scale W210, Australia, precision = 0.5 kg) with the figures obtained corrected for the weight of milk recorded from the respective animals.
Urine and fecal collection for N balance measurements
Total urine and feces were collected separately for seven consecutive d, while the cows were maintained in their individual pens and fed on their respective diets. Urine was collected using purpose-built urine collection devices that were attached to shaved areas around the vulva of the animals using adhesive glue (Ergo 5011, Kisling AG, Wetzikon, Switzerland). Silicon tubing was attached to the base of the devices to direct urine into 10 L barrels prefilled with 300 mL of 20% hydrochloric acid (HCl) to reduce the pH to 2-3. During collection, the animals were restrained in their pens using neck collars to minimize tampering of urine collection devices. The collection barrels were continuously monitored and replaced when about three-quarter full. Each morning, before feeding, the total volume of urine (plus acid) collected from each animal over the 24 h cycle was measured using a measuring cylinder. The collected urine was homogenized by stirring and a 1% aliquot was taken, filtered through a cotton gauze to remove gross dirt, homogenized again by shaking and a subsample of 100 mL dispensed into labelled plastic bottles and stored at -20°C until further processing. Total urine collection was not achieved every day on all animals resulting from either the urine collection bags peeling off from the animal or the tubing breaking. At least three complete collections were, however, achieved for each animal in each period with the incomplete collections discarded.
Feces excreted were collected from the floor every 3 h during the day when the animals were active and defecated more frequently and scooped once the following morning for the night excretions. Total feces collected over 24-h cycles (0900-0900h) were weighed and homogenized by hand mixing. A subsample (500 g) was then picked and oven dried (Genlab Oven, Genlab Ltd, UK.) at 50°C for 96 h. The dried fecal samples were packed in zip-lock polythene bags and stored at room temperature awaiting further processing. The total collection of feces and urine was conducted in weeks 3 and 4 of each experimental period with six animals (two animals per treatment) measured per week. When one cohort of six animals were going through total urine and fecal collections, enteric CH4 emission measurements were carried out on the remaining animals concurrently.
Apparent total tract nutrient (DM, OM, CP, NDF and ADF) digestibility was determined as the difference in total nutrient intake and total faecal excretion. Nitrogen retention was calculated as the net difference between total N intake and N excreted in milk, urine and faeces.
Measurements of enteric methane emissions
Three open-circuit respiratory chambers (No Pollution Industries, Edinburgh, UK) described in detail in Goopy et al. (2020) were used to measure daily enteric CH4 emissions from the experimental animals. The chambers had an internal volume of 8.90 m3, each fitted with a separate environmental control unit. The internal chamber environment was set at 22°C and relative humidity at 50%, while internal air circulation and ventilation rate were set at 220 L/s and 18 L/s, respectively. A cavity ringdown laser absorption spectrometer (Picarro G2508 analyzer, Santa Clara, USA) was used to measure CH4 and CO2 concentration (ppm) both on the incoming and outgoing air streams with the instrument taking a sample from each chamber in rotation, every 12 minutes. Air flow rates (L/s) were measured using the venturi principle and daily enteric CH4 emissions were calculated from the total volume of air flow through the chamber multiplied by the net mean CH4 concentration over each measurement event.
On the measurement days, the total ration for the day was placed in the feed bins in the respective chambers after removal of the refusals from the previous day, and the chamber cleaned. The animals were milked then placed in the individual chambers and the doors closed until the following morning when the animals were taken out and another cohort brought in after cleaning the chambers again. Continuous access to clean water was also provided in the chambers using automatic waterers. The CH4 measurements were conducted for ~22.5 h with an average of the last 3 h of measurements being assumed for the remaining 1.5 h to complete a 24-h data set. Each animal was measured three times in each period on three alternate days, with each measurement event carried out in a different respiration chamber. The three replicates were then averaged for statistical analysis.
The CH4 recovery rate in each chamber system was measured at the start and at the end of each period by injecting pure CH4 at a known mass flow into the respiration chamber using a gas phase titration unit (Environics 4020, Environics Inc., Tolland, USA) and measuring CH4 concentration in the exhaust air at equilibrium. Recovery rates were on average 46 ± 2.6% during the experimental period due to a worn-out rotor seal in the 16-port distribution manifold feeding the analytical instrument and consequently, the enteric CH4 data were corrected mathematically to adjust for the recovery losses. This was done by multiplying the calculated animal emission figure in each measurement event by the inverse of the recovery rates measured for the respective periods. Though the cause of the low recovery rates was detected early in the experiment, this could not be fixed immediately because appropriate spare part was not available locally and we had to source it from the Picarro company in the USA which took up to 3 months to be delivered. The final MY and Ym obtained agreed with those obtained from previous measurements with the same respiration chambers with animals fed almost similar diets.
Laboratory analysis
Dried samples of feed offered, refusals and feces were ground through a 1-mm sieve using a hammer mill (MF 10 basic, IKA, Werke GmbH & CO. KG, Staufen, Germany) and analysed for DM, OM, neutral detergent fiber (NDF), acid detergent fiber (ADF), acid detergent lignin (ADL), ether extract (EE) and total extratable phenols (TEP). True DM was determined by drying the samples at 105°C for 24 h while ash was determined by combustion in a muffle furnace at 550°C for 6 h according to the methods of the Association of Official Analytical Chemists (AOAC, 1990 methods no. 924.05). Feed, refusal and fecal samples were analysed for NDF and ADF by the methods of Van Soest et al. (1991). Total N content in feed, feces, milk and urine was determined by micro-Kjeldhal procedure of AOAC (AOAC, 1990, method no. 988.05) using selenium catalyst. Acid detergent lignin content was analysed in an external laboratory (National Institute of Nutrition, Hyderbad, India) using near infrared spectroscopy. Ether extract in feed and refusal samples was determined in a commercial laboratory (Cropnut Ltd, Kenya), following AOAC procedures (AOAC, 1990, method no. 14.018). Total extratable phenols, total tannins (TT) and CT in feed samples were determined at the University of Hohenheim animal nutrition laboratories using the procedure according to Makkar et al. (1993) with TT, expressed as tannic acid equivalent, separated from TEP using an insoluble matrix of polyvinyl polypyrrolidone as described by Makkar et al. (1995). Metabolizable energy (ME; kJ) in all the three diets was calculated based on equation by AFIA (2014) as follows:
Where %OMD is the organic matter digestibility (%) measured during the experiment. Because of the minimal differences in EE and MY among the three experiemental diets tested in the present study, these were assumed to have a neglible effect on the overall diet-to-diet differences in ME content and hence a similar equation was used for the three diets.
Condensed tannins were determined according to the method by Porter et al. (1986) using butanol-HCl reagent and expressed as leucocyanidin equivalent. Milk was analysed for fat, solid not fat (SNF), lactose, protein and total solids using an ultrasonic milk analyzer (Lactoscan S standard 1040, Milkotronic, Nova Zagora, Bulgaria). Fat and protein corrected milk (FPCM) was calculated according to the equation by Gerber et al. (2011):
Statistical analysis
Data were analyzed using a linear mixed model in R 3.5.3 (R Development Core Team, USA). The dependent variables measured were first tested for normality by plotting normal quantile-quantile plots in R. The model for each variable was then fitted using the “Lme4” package (Bates et al., 2007) with treatment, period and treatment by period interaction included as fixed effects and animal identity as a random effect. Animal group (square) effect was not significant (P > 0.05) and was dropped from the model. ANOVA Type 3 analysis was used to test for significance of the fixed factors and the interaction was dropped in the final model used when P > 0.05. For the model fitted for mean daily milk production, the average milk yield of the three days prior to the start of each experimental period was included in the model fitted as a covariate to account for pre-experimental animal to animal differences in the initial milk yield. Least square means were calculated using the “lsmeans” package (Lenth and Lenth, 2018) and Tukey’s built in “Multicompview” package (Graves et al., 2015) in R was used to separate the means per treatment. Level of significance was determined at P < 0.05.
Results
Composition of diet ingredients and experimental diets
The chemical composition of the feed ingredients and the experimental diets fed to the animals are shown in Table 3. The CON diet had a lower CP content compared to the two supplemented diets, with DES having a slightly lower CP than CUBES (9.7% versus 10.5%, respectively). The CUBES diet had 14% lower NDF, and 17% lower ADF contents compared to CON, while DES had 16% higher ADF and 63% higher ADL content compared to CON (41 ± 2.8 g/kg DM). The CUBES diet had almost double EE content compared to CON and DES that had the same content. The DES diet had almost three times higher TEP, about six times higher TT and more than twenty times higher CT content (1.1%) compared to CON and CUBES (Table 3).
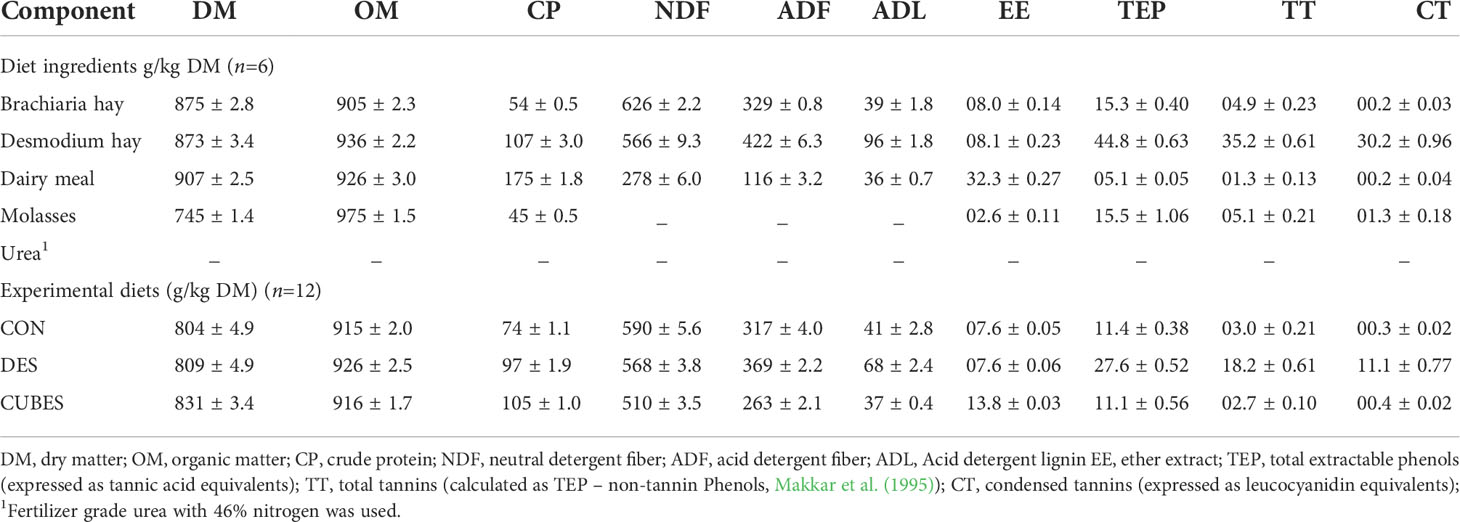
Table 3 Chemical composition (g/kg DM, mean ± SE) of the feed ingredients and the formulated experimental diets consisting mainly of Urochloa brizantha var. Xaraes hay as the basal diet (CON) and supplemented either with Desmodium intortum hay (DES) or commercial dairy supplement (CUBES).
Feed intake, animal performance and milk composition
Dietary treatment influenced (P < 0.05) DM, OM, CP, NDF and ME intake but not ADF intake (P = 0.64, Table 4). The daily DMI and DM, OM and ME intake when expressed per 100 kg LW differed (P < 0.05) among the three treatments with DES having the lowest and CUBES having the highest intakes. Animals fed on CON had 13% lower CP intake compared to DES and 82% compared to animals fed on CUBES. The animals fed on DES had 19% and 29% lower total NDF intake compared to cows fed on CON and CUBES, respectively. The Treatment × Period interaction was significant (P = 0.013) for the ADF intake, with lower values reported in Period 1 for the DES treatment group as compared to the other two experimental periods.
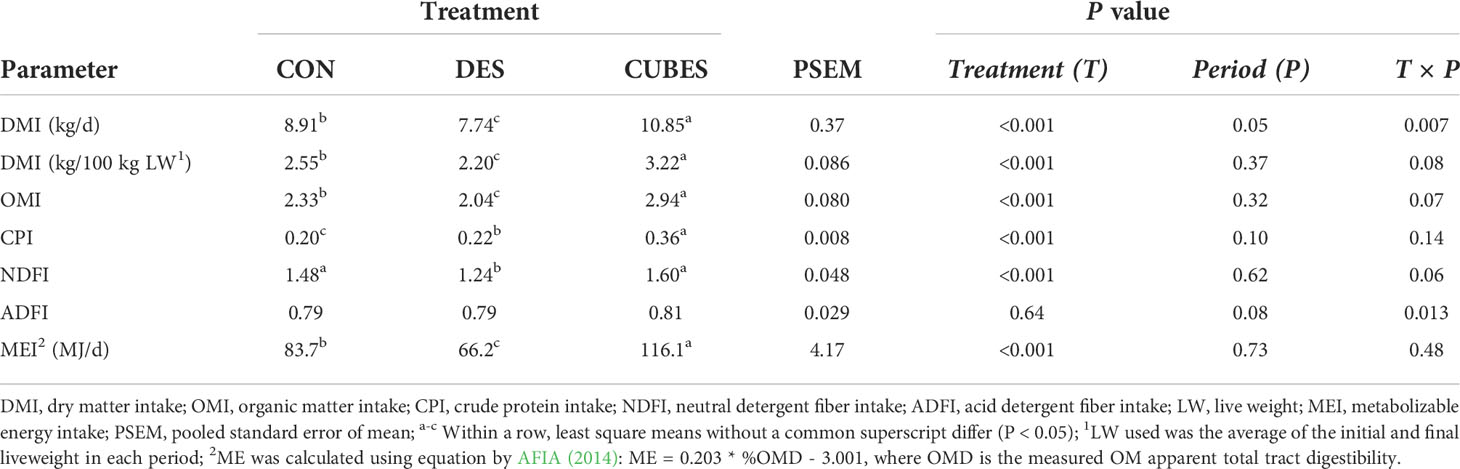
Table 4 Mean daily nutrient (kg/100 kg LW) and metabolizable energy intake (MJ/d) of crossbred cows (n=12) fed either on a control diet (CON) consisting mainly of Urochloa brizantha var. Xaraes hay alone or the control diet supplemented with either Desmodium intortum hay (DES) or a commercial dairy supplement (CUBES) over three periods with each period lasting for 28 d.
Dietary treatment affected milk yield (P < 0.001), milk SNF (P = 0.0069) and lactose (P = 0.02) contents (Table 5). Animals fed on CUBES yielded 54% more milk per day while cows fed on DES produced 24% less milk per d, compared to the CON fed animals, even though milk production across all animal groups was low (<3 L per day). Animals fed on DES had about 6% lower milk lactose content compared to CON (4.34%) and CUBES (4.48%) that did not differ from each other. Feeding CUBES resulted in higher (P < 0.05) milk SNF compared to DES but feeding CON resulted in similar SNF content to the other two diets. Animals fed on CUBES had more than double ADG compared to animals fed on CON and DES that did not differ (P > 0.05) from each other (Table 5).
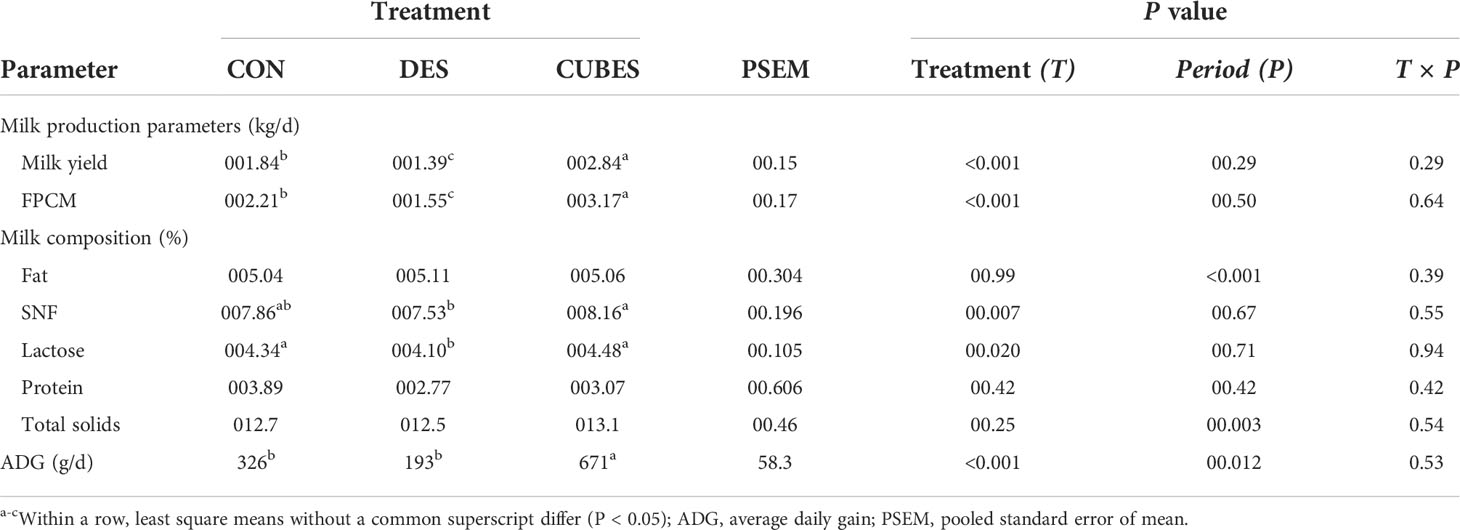
Table 5 Daily milk production, milk composition and average daily gain (ADG) of crossbred cows (n=12) fed either on a control diet (CON) consisting mainly of Urochloa brizantha var. Xaraes hay alone or the control diet supplemented with either Desmodium intortum hay (DES) or a commercial dairy supplement (CUBES) over 3 feeding periods with the measurements lasting for 28 d.
Nutrients apparent total tract digestibility and nitrogen balance
Animals fed on DES had lower (P < 0.05) apparent total tract digestibility of all measured nutrients (DM, OM, NDF and ADF) compared to animals fed on CON and CUBES that did not differ from each other (Table 6). The CP apparent digestibility differed (P < 0.001) among the three diets with DES resulting in the lowest (54.6%) and CUBES in the highest (70.3%) apparent digestibility.
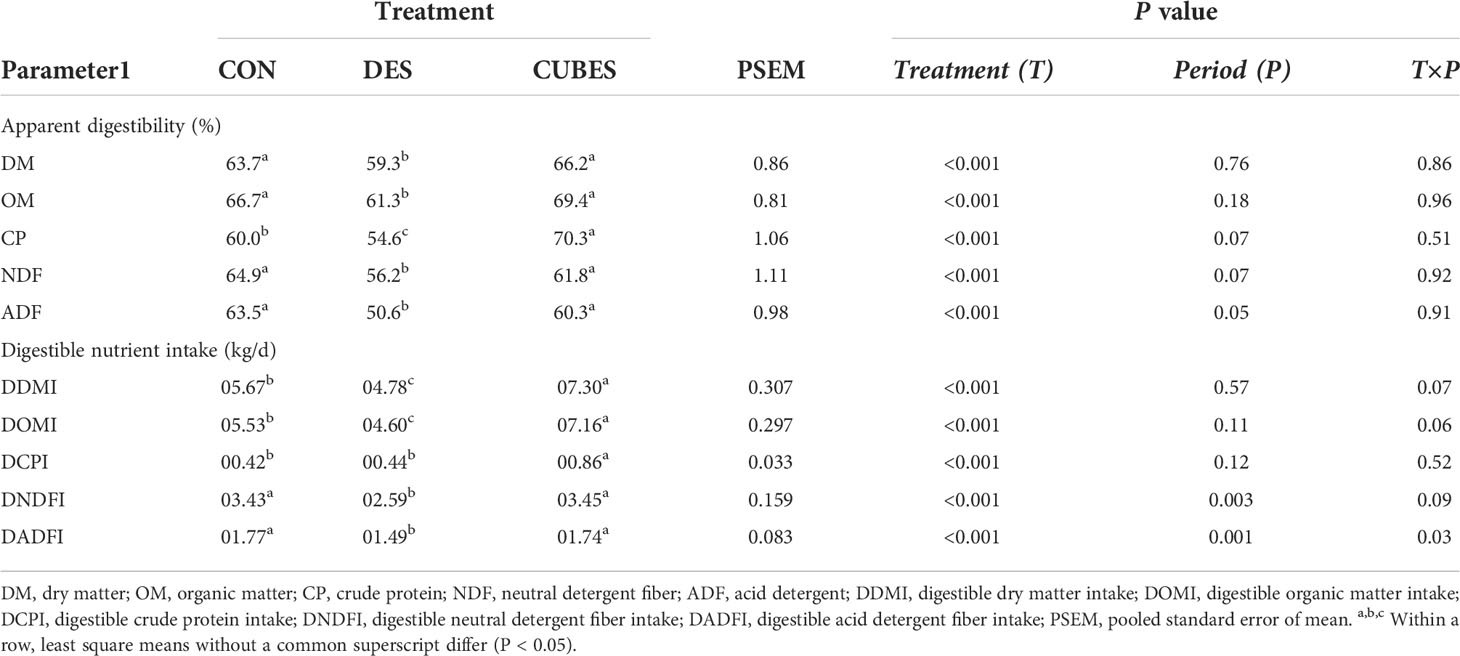
Table 6 Mean apparent total tract digestibility of nutrients and total digestible nutrient intake of crossbred cows (n=12) fed either on a control diet (CON) consisting mainly of Urochloa brizantha var. Xaraes hay alone or supplemented with either Desmodium intortum hay (DES) or a commercial dairy supplement (CUBES) with each measurement period lasting for 7 d.
Like DMI, digestible DM and OM intake differed (P < 0.001) among the dietary treatments (Table 6) with animals fed on DES having 20% lower OM intake compared to CON and 56% lower than CUBES. Animals fed on CUBES had almost double digestible CP intake compared to the other two diets that did not differ from each other. Feeding DES resulted in a lower (P < 0.05) digestible NDF and ADF intake compared to feeding CON and CUBES that did not differ from each other.
Total N intake differed (P < 0.001) among the three dietary treatments (Table 7) with animals fed on CON having the lowest intake while feeding CUBES resulted in the highest N intake. Animals fed on CON excreted 23% lower N in feces compared to DES and CUBES that did not differ (P > 0.05) from each other. However, when fecal N was expressed as a proportion of N intake, CUBES resulted in the lowest fecal N excretion while DES having the highest (P < 0.05). Net urinary and milk N output for animals fed on CUBES were almost twice that excreted by cows fed on CON and DES. When expressed as a percentage of N intake, however, feeding DES resulted in a lower (P < 0.05) urinary N excretion compared to the other two diets that did not differ (P > 0.05) from each other (Table 7). Milk N output as a proportion of N intake was similar across the three dietary treatments (P = 0.10). Total N retained by animals fed on CUBES was almost double that reported with CON and DES that did not differ from each other (P > 0.05). When N retained was expressed as a percentage of N intake, however, the three diets differed from each other (P < 0.001) with CUBES still having the highest N retention and CON having the lowest. Animals fed on CUBES had about eight units higher N use efficiency (retained N + milk N/N intake; %) compared to animals fed on CON and DES that did not differ from each other (P > 0.05). Period had a significant effect (P = 0.02) on N retained as a proportion of N intake with the figures reported in Period 1 being lower compared to the other two feeding periods. Period and Treatment × Period effects were significant (P < 0.05) for net N intake and fecal N excretion with lower values reported in Period 1 for the treatment groups CON and DES compared to the other two periods. There was also a period effect (P < 0.001) on net milk N output as well as when expressed as a proportion of N intake with values reported in Period 1 being lower than Period 3.
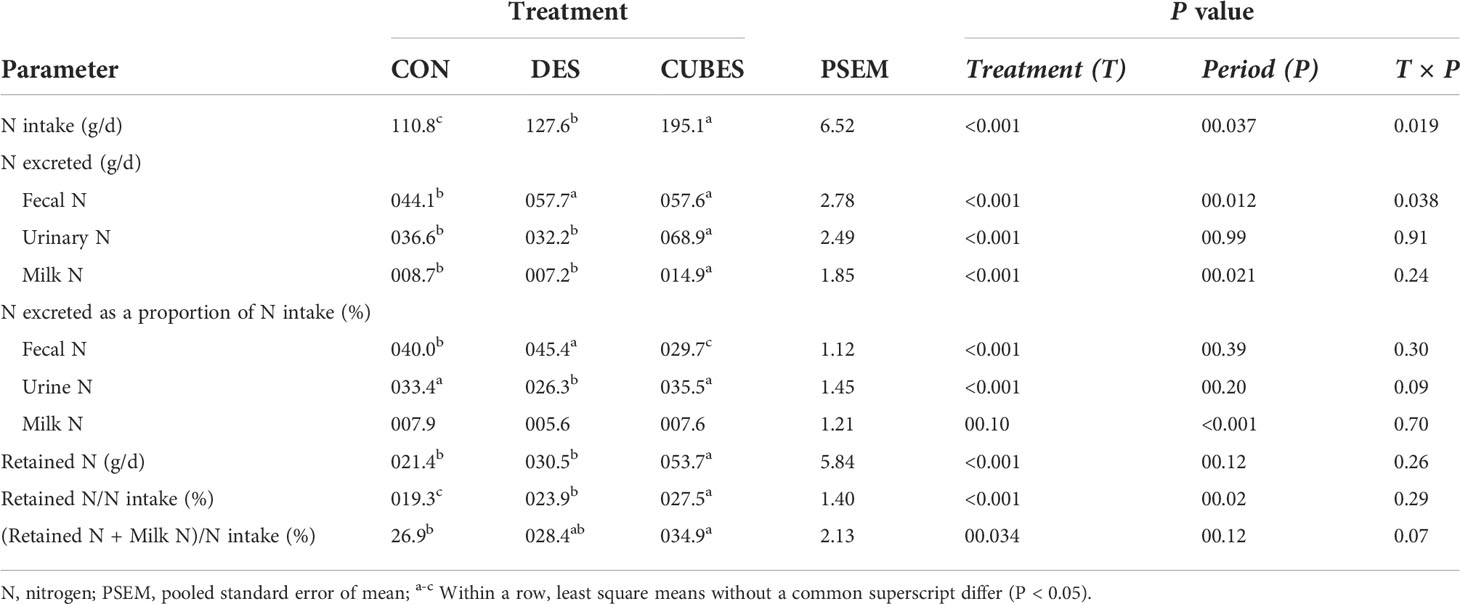
Table 7 Mean daily nitrogen (N) intake, N excretion and N retention of crossbred cows (n=12) fed on a control diet (CON) consisting mainly of Urochloa brizantha hay alone or supplemented with either Desmodium intortum hay (DES) or a commercial dairy supplement (CUBES) over three periods with the measurements lasting for 7 d.
Enteric methane emissions
The daily enteric CH4 emission (g CH4/d) of the animals fed on the three experimental diets differed (P < 0.001) from each other (Table 8) with feeding CUBES resulting in the highest and DES the lowest daily CH4 emissions. When CH4 was expressed either per DMI (MY), OM intake (OMI), digestible OMI (DOMI) or as CH4 conversion factor (Ym: CH4 MJ/GE intake (MJ)), animals fed on CON had 7 - 9% higher CH4 emissions compared with DES and CUBES that did not differ from each other (P > 0.05). The CUBES diet had lower (P < 0.001) EI (g CH4/kg CP out) compared to the other two diets that did not differ from each other but only showed a trend (P = 0.08) when emissions were expressed per unit FPCM (Table 8).
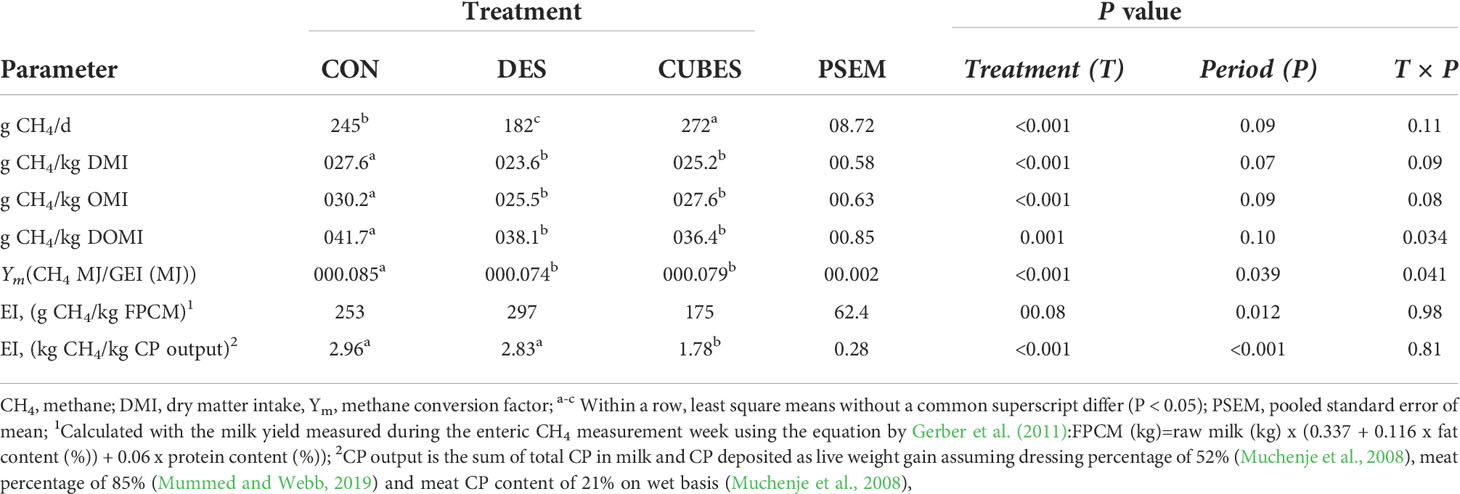
Table 8 Mean daily methane production (DMP), methane yield (MY), methane produced per organic matter intake (OMI) and digested OMI (DOMI), methane conversion factor (Ym) and methane emission intensity (EI; g CH4/kg of fat and protein corrected milk; FPCM) of crossbred cows (n=12) fed on either a control diet (CON) consisting mainly of Urochloa brizantha var Xaraes hay alone or supplemented with either Desmodium intortum hay (DES) or a commercial dairy supplement (CUBES).
Discussion
Chemical composition and apparent total tract digestibility of the experimental diets
The low-quality CON diet (CP < 7.5% and ME = 8 MJ/kg) used in the present study was formulated to represent an average cattle diet in smallholder dairy systems in Sub-Saharan Africa, where high roughage diets form the main ration offered to ruminants. The two supplemented diets (DES and CUBES) were intended to contain a similar but higher CP content (10.5% CP) than CON and therefore support higher level of animal production (4 L vs 3 L of milk yield per d). While DES had marginally lower CP content (97 g CP/kg DM) than CUBES (105 g CP/kg DM), this was probably biologically too small to trigger any differences in animal’s response. The lower CP content (10.7% DM) than expected (13.0-18.3% DM) (Osuji and Odenyo, 1997) in the Desmodium hay used necessitated a higher inclusion rate of about 58%. The low CP in the Desmodium hay was likely the case because the crop was harvested at six months of regrowth instead of the recommended three months (Heuzé et al., 2017) to allow for the primary crop in the push-pull system to mature. The possible loss of some leaf material that is generally higher in CP and tannins content than the stems during hay making and transportation could have also contributed to the rather low CP content (Kumar and Vaithiyanathan, 1990). The other chemical composition parameters such as NDF and ADF of the Desmodium intortum hay, though in line with what is reported elsewhere in literature (Perez-Maldonado and Norton, 1996; Kariuki et al., 1999; Kariuki et al., 2001; Nurfeta, 2010), were on the higher end. The PSC content of the leguminous hay (3.5% DM TT and 3.0% DM CT) agreed with findings by Perez-Maldonado and Norton (1996) who reported 3.2% DM CT and Tolera and Sundstol (2000) who reported 3.5% DM TT for Desmodium intortum hay. Getachew et al. (2000), however, found higher PSC contents in fresh Desmodium intortum forage (TEP: 8.5% and TT: 7.6% DM).
Despite the relatively low tannin content in DES (TT: 1.8% DM), where deleterious effects on feed intake would not be expected to manifest, DMI by animals fed the diet was depressed. The reduction in feed intake was accompanied by a reduction in CP apparent digestibility suggesting a possible suboptimal microbial activity in the rumen resulting in slow digestion playing a part in this. Several previous studies supplementing low quality basal diets offered to ruminants with Desmodium intortum reported no effect of feeding the legume on intake (Kariuki et al., 1999; Nurfeta, 2010), even at higher inclusion level (66%) than used in the present study (Tolera and Sundstol, 2000). Our finding, however, was in agreement with other studies where ruminants fed on diets rich in tannins also decreased feed intake (Grainger et al., 2009: Acacia mearnsii supplementation with dietary CT levels of 0.9 and 1.5% DM; Dschaak et al., 2011: supplementation with Quebracho tannin extract resulting in 3% DM dietary CT). The lower intake with Desmodium hay supplementation could be explained by the depressed DMD compared to the other two diets that could have slowed down rumen passage rate (Jayanegara et al., 2012). When tannins are present in ruminants’ diet, these have been shown to bind to dietary and salivary protein during chewing in the mouth forming Protein-Tannin complexes that are quite stable at normal rumen pH (5.5-7.0) (Makkar et al., 1995). This binding, depending on activity and tannin concentration in the diet can significantly reduce proteolysis in the rumen and therefore influencing microbial growth and activity and hence fibre digestion. It is also thought that presence of tannins in feed reduces its palatability because of the astringent taste it exerts (Mueller-Harvey, 2006). We cannot substantiate this theory in the present study because the tannin content of the total ration offered to the animals was considered low and there was no evidence of animals selecting against the Desmodium hay.
The lower nutrient apparent digestibility observed in animals fed on the tannin-rich DES compared to the animals fed on CON was also reported in other studies where tannin - rich diets were fed to cattle. Pineiro-Vazquez et al. (2018) adding Quebracho tannin extract (91% CT) at an increasing rate of between 1-4% of the total ration to a basal diet consisting of Pennisetum purpureum observed a 2% decrease in DMD for every 1% increase of the extract. Ahnert et al. (2015) also while adding Quebracho tannin extract to the diet of crossbred heifers observed a reduced CP digestibility with dietary TT contents above 1.4% and a reduction in DM, OM and NDF digestibility above 2.7% dietary TT on DM basis. The suppressed nutrient apparent total tract digestibility observed with DES, despite the relatively low concentration of 1.8% dietary TT, could be due to the low CP content in the total diet. Anti-nutritional effects of tannins have been shown to be more pronounced in low protein diets (Hill et al., 1986; Butler, 1989). It is therefore possible that the reduced DMD observed in the present study at rather low TT content, was because of the tannin binding and reducing degradability of already marginal CP content in the diet, compromising on microbial nourishment and hence lower activity.
The increased DMI reported with CUBES agreed with findings elsewhere in the literature where animals fed on low quality basal diets were supplemented with highly digestible protein concentrates (Koster et al., 1996; Mathis et al., 2000). Protein supplementation has been shown to improve overall diet digestibility which in turn increases rumen passage rate and DMI (Koster et al., 1996; Mathis et al., 2000). The dairy supplement used being low in NDF content, could have also reduced overall diet bulkiness and therefore reducing the filling effect in the rumen and hence allowing for higher intake (Ketelaars and Tolkamp, 1992).
The OM digestibility of the control diet in the present study (67%) was higher compared to similar grass-based diets fed to cattle in experiments conducted in the tropics (48-63%, (Korir et al., 2016; Pineiro-Vazquez et al., 2018; Goopy et al., 2020) and this was likely a result of the inclusion of urea and molasses that could have provided readily available carbohydrate and N in the rumen and therefore supporting higher microbial growth and fibrolytic activity (Jetana et al., 2000).
Feeding CUBES resulted in higher animal performance (higher milk and ADG) and this was likely because of the higher digestible nutrient intake compared to animals fed on the other two diets. The lower milk yield by animals fed on DES on the other hand could be explained by the lower digestible nutrient intake, limiting production. With DES having the lowest milk yield yet not the lowest digestible CP intake, this is suggestive that available energy intake was the first limiting nutrient to animal production, and this could be related to the low digestible OM intake in the diet. Supplementation with better managed Desmodium intortum forage therefore need to be investigated to establish optimal inclusion levels that will improve nutrient intake and hence animal production in smallholder tropical context (Kariuki et al., 2001). Overall, the lower milk production (<3 L per day) by all animals used in the present study than what was predicted based on their nutrient intake was probably a result of poor nutrition during their early lactation that could have suppressed their peak and subsequent lactation (Duffield et al., 2009). When ME spent on milk production and weight gain were predicted using a feed formulation model (Moran, 2005), the animals seemed to partition more energy (4 – 17%) towards weight gain than lactation irrespective of dietary treatment. This observation however did not agree with the findings by Jenet et al. (2006) who observed a higher energy partitioning towards milk in cows of similar genotype that were fed on Cynadon dactaylon (CP: 46 g/kg DM; 7.5 MJ) and supplemented with wheat bran (CP: 161 g/kg DM; 10.1 MJ) at 35% of the ration. It should however be noted that the animals in the latter study were at an early stage of lactation (week 0 - 12 post calving) suggesting a more push by the animals towards nourishing their calves at this stage (Jenet et al., 2006) and were consistently in a better plane of nutrition; suggesting a modest loss of body reserves than what possibly occurred in the animals used in the present study. Especially under suboptimal feeding conditions, cows in their later stages of lactation have been shown to preferentially partition energy to replenishing body reserves at the expense of milk production in preparation for the next production cycle (Xue et al., 2011), and this could explain the trend observed in the present study
Effects of supplementation on nitrogen balance
Feeding either CON or CUBES did not affect the proportion of dietary N excreted in urine despite the animals fed on the latter diet having higher N intake. This was likely the case because animals fed on this diet had higher ADG suggesting a higher proportion of dietary N being captured and deposited in body tissues. In the present study, N fraction retained increased with both supplementation options (by 24% and 42% for DES and CUBES, respectively) in comparison to CON and was likely driven by different mechanisms related to the diets’ chemical composition. The increased CP digestibility and higher ME content in the CUBES diet could have improved the N:energy ratio in the rumen, allowing for more dietary N to be captured for microbial protein synthesis and subsequently used by animals for their protein synthesis (Hoover and Stokes, 1991; Colmenero and Broderick, 2006). On the other hand, the presence of tannins in DES, could have improved N retention by reducing CP degradation in the rumen and therefore avoiding high ammonia tension in the rumen that encourages high N losses through the rumen wall into the portal systems and end up being excreted in urine (Stewart et al., 2019). In agreement with other studies involving diets rich in tannins, a shift in N excretion patterns from urine to feces was observed in the animals supplemented with Desmodium hay (Stewart et al., 2019; Herremans et al., 2020). It is not clear whether some tannin- protein complexes formed in the rumen fail to dissociate in the lower digestive tract or they dissociate, and new complexes formed, but what is generally observed is increased faecal N (Frutos et al., 2004). This shift in N excretion towards the fecal route could reduce environmental pollution because N in feces is less volatile compared to that excreted in urine and therefore reduces the proportion of excreted N being converted to ammonia or nitrous oxide (Rotz, 2004).
As the dietary protein was marginal for production in the three diets tested in the present study, the amount of N of dietary origin excreted in faeces may have been overestimated because the proportion of metabolic N in the faecal excretion is known to be higher in low quality diets (Ferrell et al., 2005). Caution therefore should be taken when interpreting CP apparent digestibility and N balance because metabolic N in feces were not measured and thus not accounted for in the calculations.
Effects of supplementation on enteric methane production
Animals fed on DES produced the lowest daily enteric CH4 emission compared to the other two diets tested and this could be explained by the lower DMI and MY, which were likely effects of tannins as described earlier. On the other hand, the higher DMI associated with commercial dairy concentrate supplementation resulted in higher absolute CH4 emissions despite having a lower MY than CON. Both supplementation options (CUBES and DES) resulted in lower MY and Ym compared to the CON diet, which could be attributed to the presence of tannins in DES and possibly a combination of both presence of lipids and higher OM digestibility of the CUBES diet. Tannins and lipids have both been shown to have inhibitory effects on enteric CH4 production when present in ruminants’ diets (Beauchemin et al., 2008). Tannins have been shown to bind to CP and fiber in the diet reducing their digestibility and hence substrate availability for conversion to CH4 by methanogens (Grainger et al., 2009; Williams et al., 2020). The simultaneous reduction in OMD and MY observed for the DES diet supports this explanation. Plant secondary metabolites also have the potential to suppress methanogenic activity by either intoxicating the protozoa or binding to microbial enzymes (McSweeney et al., 2001). The effect of the diets on rumen microbes was, however, not characterized in the present study.
The 9% reduction in MY for CUBES compared to CON was higher than the predicted 2.2 – 3.5% when using equations available in literature (Beauchemin et al., 2008; Moate et al., 2011) based on the EE difference of 6.2 g/kg DM between the two diets. Moate et al. (2011), from a meta-analysis using data from experiments with dairy and beef cattle supplemented with lipid-rich concentrates, observed a reduction of 3.5% in MY for every 10g/kg DM increase in dietary fat, while Beauchemin et al. (2008) in a similar review with cattle and sheep, reported a 5.6% reduction with the same magnitude of increase in dietary fat content. The higher EE content in CUBES than in the other two diets could have triggered several mechanisms suppressing CH4 production per unit feed intake, including reduction in fiber digestion and protozoal numbers in the rumen (McGinn et al., 2004; Beauchemin et al., 2008). A trend towards lower NDF digestibility when feeding CUBES compared to CON supports this hypothesis. The higher response in MY (-9%) than expected when feeding CUBES in addition to the presence of fat, could be explained by higher DMI (than CON) that is also associated with shorter rumen retention time and hence lower MY (Goopy et al., 2014). Though passage rate was not measured in the present study, shorter rumen retention time has been observed to lead to a more propionate fermentation pathway and a reduction in the contact time between the methanogens and the substrate; both leading to a lower MY (Janssen, 2010).
The EI (g CH4/kg FPCM), although not statistically different among the three diets because of huge variability within diets, was 31% lower for animals fed on CUBES compared to CON. This observation was consistent with other findings in the literature where increasing milk production in low yielding animals (< 6 L per d) is associated with a reduction in EI (Gerber et al., 2011). When emissions were, however, expressed per CP output, CUBES had lower EI compared to CON and DES and this is probably because the animals fed on CUBES had the highest ADG as well and therefore depositing more CP compared to the other two diets.
Conclusions and recommendations
The present study shows that supplementing lactating crossbred cows fed on a low-quality basal diet with either a commercial dairy concentrate or a tannin rich Desmodium intortum hay improved N use efficiency and reduced enteric MY and Ym. While dairy concentrate supplementation improved feed intake and animal production, i.e., milk yield and weight gain, supplementation with almost 60% Desmodium intortum hay suppressed total nutrient intake and digestibility, and negatively affected animal production. The low CP and high lignin contents as well as the presence of tannins in the Desmodium hay used might explain these undesired effects on intake and animal performance. Desmodium hay supplementation, however, showed some positive environmental outcomes including reduced enteric CH4 production and a shift in N excretion to the less volatile fecal route. Further animal experiments are needed to evaluate the potential of Desmodium hay as a supplement by using better quality Desmodium intortum hay to determine optimal supplementation levels for realization of the environmental gains reported without compromising animal production. The use of commercial concentrates could be a viable option to improve animal production and reduce enteric CH4 emission intensity but its economic feasibility in smallholder context needs to be investigated.
Data availability statement
The raw data supporting the conclusions of this article will be made available by the authors, without undue reservation, upon request to the corresponding author..
Ethics statement
The animal study was reviewed and approved by the International Livestock Research Institute’s Institutional Animal Care and Use Committee (IACUC, Reference number: IACUC 2019-09).
Author contributions
DK designed, planned and conducted the animal trial, processed, analysed the data and took the lead role in the writing the manuscript. RE contributed to designing and planning of the experiment, and revision of the manuscript to writing the manuscript. JG contributed to the designing of the experiment and revision of the manuscript. CA contributed to data analysis and revision of the manuscript. LM contributed to searching for funds and revision of the manuscript. SM contributed to the designing planning, data processing, writing and revision of the manuscript. All authors have approved the manuscript for publication.
Funding
This project was funded by the German Federal Ministry for Economic Cooperation and Development (BMZ) and the German Technical Cooperation (GIZ) under the project: Programme for Climate Smart Livestock (PCSL, grant no: 2017.0119.2). The authors also acknowledge the CGIAR Fund Council, Australia (ACIAR), Irish Aid, European Union, International Fund for Agricultural Development (IFAD), Netherlands, New Zealand, UK, USAID and Thailand for funding to the CGIAR Research Program on Livestock.
Acknowledgments
The authors are grateful to Jesse Kagai, Erick Kiprotich and Nelson Saya for assisting in conducting the animal trial. We also appreciate the assistance of Dr. Alice Onyango and Rodgers Rogito for carrying out the proximate analyses of the samples collected during the animal experiment. We sincerely thank Dr. Uta Dickhoefer; Institute of Agricultural Sciences in the Tropics, University of Hohenheim for allowing us to send our samples for plant secondary metabolites analyses in their facilities. I also want to acknowledge Dr. Cesar Patino Pinares who provided internal review for this work.
Conflict of interest
The authors declare that the research was conducted in the absence of any commercial or financial relationships that could be construed as a potential conflict of interest.
Publisher’s note
All claims expressed in this article are solely those of the authors and do not necessarily represent those of their affiliated organizations, or those of the publisher, the editors and the reviewers. Any product that may be evaluated in this article, or claim that may be made by its manufacturer, is not guaranteed or endorsed by the publisher.
References
Aboagye I. A., Oba M., Castillo A. R., Koenig K. M., Iwaasa A. D., Beauchemin K. A. (2018). Effects of hydrolyzable tannin with or without condensed tannin on methane emissions, nitrogen use, and performance of beef cattle fed a high-forage diet. J. Anim. Sci. 96, 5276–5286. doi: 10.1093/jas/sky352
AFIA (2014). AFIA - laboratory methods manual. publication no. 03/001 (Melbourne, VIC: Australian Fodder Industry Association Ltd).
Ahnert S., Dickhoefer U., Schulz F., Susenbeth A. (2015). Influence of ruminal quebracho tannin extract infusion on apparent nutrient digestibility, nitrogen balance, and urinary purine derivatives excretion in heifers. Livest. Sci. 177, 63–70. doi: 10.1016/j.livsci.2015.04.004
Bakrie B., Hogan J., Liang J., Tareque A., Upadhyay R. (1996). Ruminant nutrition and production in the tropics and subtropics, Australian centre for international agricultural research, monograph no. 36, 119–29 (Canberra, Australia:Australian Centre for International Agricultural Research).
Beauchemin K. A., Kreuzer M., O'Mara F., McAllister T. A. (2008). Nutritional management for enteric methane abatement: a review. Aust. J. Exp. Agric. 48, 21–27. doi: 10.1071/Ea07199
Beauchemin K. A., McGinn S. M., Martinez T. F., McAllister T. A. (2007). Use of condensed tannin extract from quebracho trees to reduce methane emissions from cattle. J. Anim. Sci. 85, 1990–1996. doi: 10.2527/jas.2006-686
Bohnert D. W., DelCurto T., Clark A. A., Merrill M. L., Falck S. J., Harmon D. L. (2011). Protein supplementation of ruminants consuming low-quality cool- or warm-season forage: differences in intake and digestibility. J. Anim. Sci. 89, 3707–3717. doi: 10.2527/jas.2011-3915
Butler L. G. (1989). Effects of condensed tannin on animal nutrition. In chemistry and significance of condensed tannins, pages 391–402 (Boston, MA: Springer).
Castro-Montoya J. M., Garcia R. A., Ramos R. A., Flores J. M., Alas E. A., Corea E. E. (2018). Dairy cows fed on tropical legume forages: effects on milk yield, nutrients use efficiency and profitability. Trop. Anim. Health Prod. 50, 837–843. doi: 10.1007/s11250-017-1505-3
Colmenero J. J., Broderick G. A. (2006). Effect of dietary crude protein concentration on milk production and nitrogen utilization in lactating dairy cows. J. Dair. Sci. 89, 1704–1712. doi: 10.3168/jds.S0022-0302(06)72238-X
Cook S. M., Khan Z. R., Pickett J. A. (2007). The use of push-pull strategies in integrated pest management. Annu. Rev. Entomol. 52, 375–400. doi: 10.1146/annurev.ento.52.110405.091407
Detmann E., Valente E. E. L., Batista E. D., Huhtanen P. (2014). An evaluation of the performance and efficiency of nitrogen utilization in cattle fed tropical grass pastures with supplementation. Livest. Sci. 162, 141–153. doi: 10.1016/j.livsci.2014.01.029
Dschaak C. M., Williams C. M., Holt M. S., Eun J. S., Young A. J., Min B. R (2011). Effects of supplementing condensed tannin extract on intake, digestion, ruminal fermentation, and milk production of lactating dairy cows. J. Dairy Sci 94, 2508–2519. doi: 10.3168/jds.2010-3818
Duffield T. F., Lissemore K. D., McBride B. W., Leslie K. E. (2009). Impact of hyperketonemia in early lactation dairy cows on health and production. J. Dair. Sci. 92, 571–580. doi: 10.3168/jds.2008-1507
Ericksen P. J., Crane T. A. (2018). The feasibility of low emissions development interventions for the East African livestock sector: Lessons from Kenya and Ethiopia. In.: ILRI. Res. Rep. Nairobi. 46 Int. Livest. Res. Inst. (ILRI) Nairobi:Kenya, pp 1–25. Available at: https://hdl.handle.net/10568/91527.
Ferrell C. L., Freetly H. C., Goetsch A. L. et, Kreikemeier K. K. (2005). The effect of dietary nitrogen and protein on feed intake, nutrient digestibility, and nitrogen flux across the portal-drained viscera and liver of sheep consuming high-concentrate diets ad libitum. J. Anim. Sci. 79, 1322–1328. doi: 10.2527/2001.7951322x
Franzel S., Wambugu C., Stewart J., Sande B. D. (2005). Fodder shrubs for improving incomes of dairy farmers in the east African highlands. Trop. Grassl. 39 (4), 212–212.
Frutos P., Hervas G., Giráldez F. J., Mantecón A. (2004). Tannins and ruminant nutrition. Span. J. Agri. Res. 2, 191–202. doi: 10.5424/sjar/2004022-73
Gerber P., Vellinga T., Opio C., Steinfeld H. (2011). Productivity gains and greenhouse gas emissions intensity in dairy systems. Livest. Sci. 139, 100–108. doi: 10.1016/j.livsci.2011.03.012
Getachew G., Makkar H., Becker K. (2000). Effect of polyethylene glycol on in vitro degradability ofnitrogen and microbial protein synthesis fromtannin-rich browse and herbaceous legumes. Br. J. Nutr. 84, 73–83. doi: 10.1017/S0007114500001252
Goopy J. P., Donaldson A., Hegarty R., Vercoe P. E., Haynes F., Barnett M., et al. (2014). Low-methane yield sheep have smaller rumens and shorter rumen retention time. Br. J. Nutr. 111, 578–585. doi: 10.1017/S0007114513002936
Goopy J. P., Korir D., Pelster D., Ali A. I. M., Wassie S. E., Schlecht E., et al. (2020). Severe below-maintenance feed intake increases methane yield from enteric fermentation in cattle. Br. J. Nutr. 123, 1239–1246. doi: 10.1017/S0007114519003350
Grainger C., Clarke T., Auldist M., Beauchemin K., McGinn S., Waghorn G., et al. (2009). Potential use of acacia mearnsii condensed tannins to reduce methane emissions and nitrogen excretion from grazing dairy cows. Can. J. Anim. Sci. 89, 241–251. doi: 10.4141/CJAS08110
Heuzé V., Tran G., Hassoun P. (2017). Greenleaf desmodium (Desmodium intortum). Feedipedia, a programme by (INRAE, CIRAD, AFZ and FAO). Available at: https://www.feedipedia.org/node/303
Association of Official Analytic Chemists (AOAC) (1990). Association of Official Analytic Chemists (AOAC). Official methods of analysis, Vol 2, 15th edn, Sec. 985, 29 (Arlington:VA).
Herremans S., Vanwindekens F., Decruyenaere V., Beckers Y., Froidmont E. (2020). Effect of dietary tannins on milk yield and composition, nitrogen partitioning and nitrogen use efficiency of lactating dairy cows: A meta-analysis. J. Anim. Physiol. Anim. Nutr. (Berl). 104, 1209–1218. doi: 10.1111/jpn.13341
Hill G. M., Utley P. R., Newton G. L. (1986). Influence of dietary crude protein on peanut skin digestibility and utilization by feedlot steers. J. Anim. Sci. 62, 887–894. doi: 10.2527/jas1986.624887x
Hoover W. H., Stokes S. R. (1991). Balancing carbohydrates and proteins for optimum rumen microbial yield. int. J. Dairy Sci. 74, 3630–3644. doi: 10.3168/jds.S0022-0302(91)78553-6
Janssen P. H. (2010). Influence of hydrogen on rumen methane formation and fermentation balances through microbial growth kinetics and fermentation thermodynamics. Anim. Feed. Sci. Technol. 160, 1–22. doi: 10.1016/j.anifeedsci.2010.07.002
Jayanegara A., Leiber F., Kreuzer M. (2012). Meta-analysis of the relationship between dietary tannin level and methane formation in ruminants from in vivo and in vitro experiments. J. Anim. .Physiol. Anim. Nutr. (Berl). 96, 365–375. doi: 10.1111/j.1439-0396.2011.01172.x
Jenet A., Fernandez-Rivera S., Tegegne A., Wettstein H., Senn M., Saurer M., et al. (2006). Evidence for different nutrient partitioning in boran (Bos indicus) and boran× Holstein cows when re-allocated from low to high or from high to low feeding level. J. Vet. Med. 53, 383–393. doi: 10.1111/j.1439-0442.2006.00853.x
Jetana T., Abdullah N., Halim R. A., Jalaludin S., Ho Y. W. (2000). Effects of energy and protein supplementation on microbial-n synthesis and allantoin excretion in sheep fed guinea grass. Anim. Feed. Sci. Technol. 84, 167–181. doi: 10.1016/S0377-8401(00)00119-X
Kariuki J. N., Gitau G. K., Gachuiri C. K., Tamminga S., Muia J. M. K. (1999). Effect of supplementing napier grass with desmodium and lucerne on DM, CP and NDF intake and weight gains in dairy heifers. Livest. Prod. Sci. 60, 81–88. doi: 10.1016/S0301-6226(99)00035-4
Kariuki J. N., Tamminga S., Gachuiri C. K., Gitau G. K., Muia J. M. K. (2001). Intake and rumen degradation in cattle fed napier grass (Pennisetum purpureum) supplemented with various levels of desmodium intortum and ipomoea batatus vines. S. Afr. J. Anim. Sci. 31, 149–157. doi: 10.4314/sajas.v31i3.3798
Kebede G., Assefa G., Feyissa F., Alemayehu M. (2016). Forage legumes in crop-livestock mixed farming systems: A review. Int. J. @ Livest. Res. 6, 1–18. doi: 10.5455/ijlr.20160317124049
Kebreab E., Smith T., Tanner J., Osuji P. (2005). Review of undernutrition in smallholder ruminant production systems in the tropics. In Coping with feed scarcity in smallholder livestock systems in developing countries, 3–94. Eds. Ayantunde A. A., Fernandez-Rivera S., McCrabb G. (Animal sciences group, wageningen UR, wageningen, the Netherlands, university of reading, reading, UK, ETH (Swiss federal institute of technology), Zurich, Switzerland, and ILRI (International livestock research institute):Nairobi, Kenya. 3–94.
Ketelaars J. J. M. H., Tolkamp B. J. (1992). Toward a new theory of feed-intake regulation in ruminants .1. causes of differences in voluntary feed-intake - critique of current views. Livest. Prod. Sci. 30, 269–296. doi: 10.1016/0301-6226(92)90039-7
Khan Z. R., Midega C. A., Amudavi D. M., Hassanali A., Pickett J. A. (2008). On-farm evaluation of the ‘push–pull’technology for the control of stemborers and striga weed on maize in western Kenya. Field Crops Res. 106, 224–233. doi: 10.1016/j.fcr.2007.12.002
Korir D., Goopy J. P., Gachuiri C., Butterbach-Bahl K. (2016). Supplementation with calliandra calothyrsus improves nitrogen retention in cattle fed low-protein diets. Anim. Prod. Sci. 56, 619–626. doi: 10.1071/An15569
Koster H. H., Cochran R. C., Titgemeyer E. C., Vanzant E. S., Abdelgadir I., StJean G. (1996). Effect of increasing degradable intake protein on intake and digestion of low-quality, tallgrass-prairie forage by beef cows. J. Anim. Sci. 74, 2473–2481. doi: 10.2527/1996.74102473x
Kumar R., Vaithiyanathan S. (1990). Occurrence, nutritional significance and effect on animal productivity of tannins in tree leaves. Anim. Feed. Sci. Technol. 30, 21–38. doi: 10.1016/0377-8401(90)90049-E
Leng R. (1990). Factors affecting the utilization of ‘poor-quality’forages by ruminants particularly under tropical conditions. Nutr. Res. Rev. 3, 277–303. doi: 10.1079/NRR19900016
Lenth R., Lenth M. R. (2018). Package ‘lsmeans’. Am. Statist. 34, 216–221. Available at: http://CRAN.R-project.org/package=emmeans
Makkar H. P. S. (2003). Effects and fate of tannins in ruminant animals, adaptation to tannins, and strategies to overcome detrimental effects of feeding tannin-rich feeds. Small. Rumin. Res. 49, 241–256. doi: 10.1016/S0921-4488(03)00142-1
Makkar H. P., Blümmel M., Borowy N. K., Becker. K. (1993). Gravimetric determination of tannins and their correlations with chemical and protein precipitation methods. J. Sci Food Agric 61, 161–5. doi: 10.1002/jsfa.2740610205
Makkar H. P., Blummel M., Becker K. (1995). Formation of complexes between polyvinyl pyrrolidones or polyethylene glycols and tannins, and their implication in gas production and true digestibility in in vitro techniques. Br. J. Nutr. 73, 897–913. doi: 10.1079/bjn19950095
Makkar H. P. S., Singh B. (1991). Effect of drying conditions on tannin, fiber and lignin levels in mature oak (Quercus-incana) leaves. J. Sci. Food Agric. 54, 323–328. doi: 10.1002/jsfa.2740540302
Mathis C. P., Cochran R. C., Heldt J. S., Woods B. C., Abdelgadir I. E., Olson K. C., et al. (2000). Effects of supplemental degradable intake protein on utilization of medium- to low-quality forages. J. Anim. Sci. 78, 224–232. doi: 10.2527/2000.781224x
Mbuthia E., Gachuiri C. (2003). Effect of inclusion of mucuna pruriens and dolichos lablab forage in Napier grass silage on silage quality and on voluntary intake and digestibility in sheep. Trop. Subtrop. Agroecosyst. 1, 123–128.
McGinn S. M., Beauchemin K. A., Coates T., Colombatto D. (2004). Methane emissions from beef cattle: Effects of monensin, sunflower oil, enzymes, yeast, and fumaric acid. J. Anim. Sci. 82, 3346–3356. doi: 10.2527/2004.82113346x
McSweeney C. S., Palmer B., McNeill D. M., Krause D. O. (2001). Microbial interactions with tannins: nutritional consequences for ruminants. Anim. Feed. Sci. Technol. 91, 83–93. doi: 10.1016/S0377-8401(01)00232-2
Moate P. J., Williams S. R. O., Grainger C., Hannah M. C., Ponnampalam E. N., Eckard R. J. (2011). Influence of cold-pressed canola, brewers grains and hominy meal as dietary supplements suitable for reducing enteric methane emissions from lactating dairy cows. Anim. Feed. Sci. Technol. 166, 254–264. doi: 10.1016/j.anifeedsci.2011.04.069
Moran J. (2005). Tropical dairy farming: feeding management for small holder dairy farmers in the humid tropics (Collingwood, Australia:Csiro publishing).
Muchenje V., Dzama K., Chimonyo M., Raats J., Strydom P. (2008). Meat quality of nguni, bonsmara and Aberdeen Angus steers raised on natural pasture in the Eastern cape, south Africa. Meat. Sci. 79, 20–28. doi: 10.1016/j.meatsci.2007.07.026
Mueller-Harvey I. (2006). Unravelling the conundrum of tannins in animal nutrition and health. J. Sci. Food Agric. 86, 2010–2037. doi: 10.1002/jsfa.2577
Mummed Y. Y., Webb E. C. (2019). Carcass weight, meat yield and meat cuts from arado, boran, barka, raya cattle breeds in Ethiopia. J. Agric. Sci. 11, 45–51. doi: 10.5539/jas.v11n18p45
Njarui D. M., Gichangi E. M., Ghimire S. R., Muinga R. W. (2016). Climate smart brachiaria grasses for improving livestock production in East Africa-Kenya experience. Proc. Proc. Worksh. Held. Naivasha. Kenya., 14–15.
Nurfeta A. (2010). Digestibility and nitrogen utilization in sheep fed enset (Ensete ventricosum) pseudostem or corm and graded levels of desmodium intortum hay to wheat straw-based diets. J. Anim. Physiol. Anim. Nutr. (Berl). 94, 773–779. doi: 10.1111/j.1439-0396.2009.00960.x
Opio C., Gerber P., Mottet A., Falcucci A., Tempio G., MacLeod M., et al. (2013). Greenhouse gas emissions from ruminant supply chains–a global life cycle assessment. Rome:Food Agric. Organ. Unit. Nation. Available at: http://www.fao.org/3/i3461e/i3461e.pdf
Osuji P. O., Odenyo A. A. (1997). The role of legume forages as supplements to low quality roughages–ILRI experience. Anim. Feed. Sci. Technol. 69, 27–38. doi: 10.1016/S0377-8401(97)81620-3
Perez-Maldonado R. A., Norton B. W. (1996). The effects of condensed tannins from desmodium intortum and calliandra calothyrsus on protein and carbohydrate digestion in sheep and goats. Br. J. Nutr. 76, 515–533. doi: 10.1079/bjn19960060
Piñeiro-Vázquez A. T., Canul-Solis J. R., Jiménez-Ferrer G. O., Alayón-Gamboa J. A., Chay-Canul A. J., Ayala-Burgos A. J., et al. (2018). Effect of condensed tannins from leucaena leucocephala on rumen fermentation, methane production and population of rumen protozoa in heifers fed low-quality forage. Asian-australas. J. Anim. Sci. 31, 1738–1746. doi: 10.5713/ajas.17.0192
Pineiro-Vazquez A. T., Jimenez-Ferrer G., Alayon-Gamboa J. A., Chay-Canul A. J., Ayala-Burgos A. J., Aguilar-Perez C. F., et al. (2018). Effects of quebracho tannin extract on intake, digestibility, rumen fermentation, and methane production in crossbred heifers fed low-quality tropical grass. Trop. Anim. Health Prod. 50, 29–36. doi: 10.1007/s11250-017-1396-3
Porter L. J., Hrsrich L. N., Chan B. G. (1986). The conversion of procyanidins and prodelphinidins to cyanidin and delphinidin. Phytochemistry 1, 223–30. doi: 10.1016/S0031-9422(00)94533-3
Rotz C. A. (2004). Management to reduce nitrogen losses in animal production. J. Anim. Sci. 82 E-Suppl (suppl_13), E119–E137. doi: 10.2527/2004.8213_supplE119x
Shreck A. L., Zeltwanger J. M., Bailey E. A., Jennings J. S., Meyer B. E., Cole N. A. (2021). Effects of protein supplementation to steers consuming low-quality forages on greenhouse gas emissions. J. Anim. Sci. 99, skab147. doi: 10.1093/jas/skab147
Stewart E. K., Beauchemin K. A., Dai X., MacAdam J. W., Christensen R. G., Villalba J. J. (2019). Effect of tannin-containing hays on enteric methane emissions and nitrogen partitioning in beef cattle1. J. Anim. Sci. 97, 3286–3299. doi: 10.1093/jas/skz206
Tolera A., Sundstol F. (2000). Supplementation of graded levels of desmodium intortum hay to sheep feeding on maize stover harvested at three stages of maturity - 1 Feed intake, digestibility and body weight change. Anim. Feed Sci. Technol. 85, 239–257 doi: 10.1016/S0377-8401(00)00135-8
Topps J. H. (1992). Potential, composition and use of legume shrubs and trees as fodders for livestock in the tropics. J. Agr. Sci-Cambr. 118, 1–8. doi: 10.1017/S0021859600067940
Van Soest P., Robertson J., Lewis B. (1991). Carbohydrate methodology and nutritional implications in dairy cattle. methods for dietary fiber, neutral detergent fiber and non-starch polysaccharides in relation to animal nutrition. J. Dair. Sci. 74, 3583–3597. doi: 10.3168/jds.S0022-0302(91)78551-2
Waghorn G. (2008). Beneficial and detrimental effects of dietary condensed tannins for sustainable sheep and goat production–progress and challenges. Anim. Feed. Sci. Technol. 147, 116–139. doi: 10.1016/j.anifeedsci.2007.09.013
Williams S. R. O., Hannah M. C., Eckard R. J., Wales W. J., Moate P. J. (2020). Supplementing the diet of dairy cows with fat or tannin reduces methane yield, and additively when fed in combination. Animal 14, s464–s472. doi: 10.1017/S1751731120001032
Keywords: protein supplementation, tannins, milk production, digestibility, low quality diet
Citation: Korir D, Eckard R, Goopy J, Arndt C, Merbold L and Marquardt S (2022) Effects of replacing Brachiaria hay with either Desmodium intortum or dairy concentrate on animal performance and enteric methane emissions of low-yielding dairy cows. Front. Anim. Sci. 3:963323. doi: 10.3389/fanim.2022.963323
Received: 07 June 2022; Accepted: 22 July 2022;
Published: 17 August 2022.
Edited by:
Uchenna Anele, North Carolina Agricultural and Technical State University, United StatesReviewed by:
Ed Charmley, Commonwealth Scientific and Industrial Research Organisation (CSIRO), AustraliaRicardo Andrade Reis, São Paulo State University, Brazil
Copyright © 2022 Korir, Eckard, Goopy, Arndt, Merbold and Marquardt. This is an open-access article distributed under the terms of the Creative Commons Attribution License (CC BY). The use, distribution or reproduction in other forums is permitted, provided the original author(s) and the copyright owner(s) are credited and that the original publication in this journal is cited, in accordance with accepted academic practice. No use, distribution or reproduction is permitted which does not comply with these terms.
*Correspondence: Daniel Korir, ZC5rb3JpckBjZ2lhci5vcmc=; ZGFuaWVsa29yaXI2QGdtYWlsLmNvbQ==
†ORCID: Daniel Korir, orcid.org/0000-0002-1356-8039