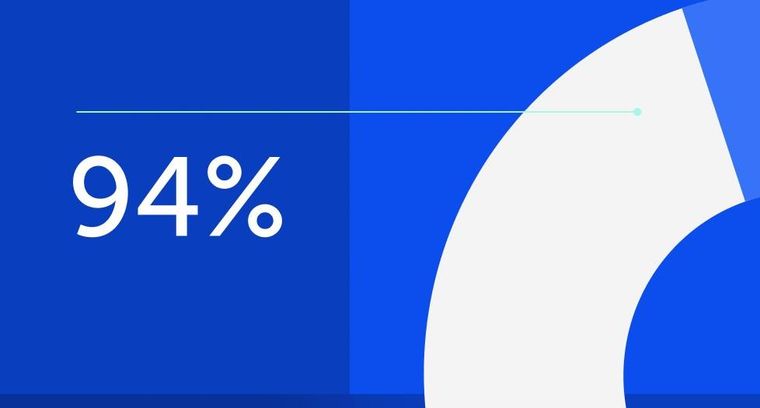
94% of researchers rate our articles as excellent or good
Learn more about the work of our research integrity team to safeguard the quality of each article we publish.
Find out more
ORIGINAL RESEARCH article
Front. Anim. Sci., 25 July 2022
Sec. Animal Nutrition
Volume 3 - 2022 | https://doi.org/10.3389/fanim.2022.951789
In this study, Lippia origanoides Kunth, native to the Alto Patía region in Southwest Colombia, and Pichia guilliermondii LV196, an inactivated yeast from the germplasm bank of Agrosavia (Colombian Agricultural Research Corporation), alone or combined, were tested for their long-term effect on rumen fermentation and methanogenesis whilst also characterising their effect on bacterial and methanogen communities. Whereas essential oils act through selective inhibition of microbial groups, yeasts are thought to work through the selective stimulation of key microbes in the rumen. We hypothesized that yeast supplementation could modulate the antimicrobial effect of a high thymol-containing oregano oil, allowing a more efficient feed utilization whilst decreasing methane production. When added to a rumen simulating fermentor (RUSITEC), L. origanoides Kunth at 132 µL/d had a detrimental effect on rumen fermentation which was accompanied by a reduction in the relative abundance of protozoa and fungi and a profound impact on the bacterial and archaeal communities. P. guilliermondii LV196 at 0.5 g/L, however, had no effect on fermentation parameters or nutrient utilization, and neither changes in microbial abundances or in the structure of bacterial and archaeal communities were observed. P. guilliermondii LV196 did not stimulate microbial numbers nor activity and, consequently we could not test whether it could have counterbalanced the antimicrobial effect of the essential oil. Future studies need to both investigate lower levels of essential oil addition, but also to re-examine the effects of P. guillermondii in the rumen and/or to replace it with other yeast of known biological activity when combined with oils extracted from L. origanoides Kunth.
Cattle production is a major income source in many developing countries but also a major source of greenhouse gas emissions (Arango et al., 2020). We have suggested that an approach focused on natural feed additives such as essential oils and yeast products, might be used to improve efficiency of feed utilization, reduce methane production and enhance overall productivity (Newbold and Ramos-Morales, 2020). However, we have also noted that one of the limitations of the use of plant extracts in ruminant diets is the inconsistent effects due to a variable composition in bioactive compounds (Ramos-Morales et al., 2019). In addition, supply chains and availability may also represent barriers in developing countries. We have thus investigated natural products sourced in-country as possible routes to rumen manipulation in the Colombian cattle sector.
Thymol has been identified as a bioactive compound capable of manipulating rumen fermentation and decreasing methane emissions (Yu et al., 2020). L. origanoides Kunth, endemic in arid tropical lands, is adapted to the dry soil and drought conditions of the Alto Patía region in Southwest Colombia. Its high thymol content and low composition variability give this essential oil great biological value (Betacourt et al., 2019). A preliminary in vitro study with this essential oil (Rodríguez Quiroz, 2014), has already shown its potential as an antimethanogen. Furthermore, as it is produced by marginalized rural communities that inhabit these areas, if a market can be developed for its incorporation in ruminant diets, it would potentially have a significant economic and social impact. However, thymol has also been reported to inhibit rumen fermentation in general, decreasing volatile fatty acid production (Evans and Martin, 2000).
Whilst some natural additives such as essential oils act through selective inhibition of microbial groups, probiotics are thought to work through the selective stimulation of key microbes in the rumen (Newbold and Ramos-Morales, 2020). Saccharomyces cerevisiae is the most frequently used probiotic yeast in ruminant nutrition. It has been shown to stimulate microbial protein synthesis and fibre degradation in the rumen (Newbold et al., 1996), whereas less studied yeast from the genus Pichia have also been shown to stimulate microbial numbers in the rumen enhancing dry matter digestibility (Suntara et al., 2021). P. guilliermondii LV196, from the germplasm bank of Agrosavia (Colombian Agricultural Research Corporation), is a unique, whole-cell, inactivated yeast with potential as a feed additive to enhance productivity in ruminants (Rodríguez et al., 2015). Whilst somewhat limited in number, studies suggest that P. guillemondii can stimulate rumen fibre breakdown (Marrero et al., 2016), and it has also been shown to improve intestinal immune health in pigs, broilers and calves (Shanmugasundaram and Selvaraj, 2012; Bass et al., 2019; Pastén et al., 2021).
As noted above yeast and essential oils act in different ways through the promotion of beneficial microbes and the inhibition of detrimental microbes, respectively. Although it has been suggested that combinations of feed additives with complementary mechanisms of action may synergistically or additively decrease methane production without any adverse effects on feed digestion or fermentation (Patra and Yu, 2014), studies on additive or associative effects of feed additives are surprisingly lacking. In our study we have investigated if yeast supplementation may modulate the potentially negative effects on fermentation of a high thymol-containing oregano oil, allowing a more efficient feed utilization whilst decreasing methane production.
Aerial parts of L. origanoides Kunth were collected from wild-growing populations located in the arid and dry ecosystem from Alto Patía, in Colombia. Essential oil was extracted by steam distillation for 3 h and composition was analyzed by GCMS at Universidad Industrial de Santander, as described by Betancourt et al. (2019). The composition of L. origanoides native to the Alto Patía in Colombia has been reported in few studies (Vásquez Carreño, 2012; Rodriguez Quiroz, 2014; Betacourt et al., 2019), showing consistency in terms of major biochemically related groups of compounds with thymol ranging from 70 to 80%, followed by its precursors, p-cymene and γ-terpinene, at much lower amounts (2-7%), and with carvacrol as a trace constituent (0.9%).
P. guilliermondii strain LV196 was obtained from the germplasm bank of Colombian Agricultural Research Corporation (Agrosavia). Yeast cells were produced by discontinuous batch fermentation using a modified medium including a by-product of the purification of glycerine and mineral salts (Chaparro et al., 2017). Starch as a drying protectant for a fluidized bed drying process was used guarantying a greater shelf life than similar commercial products (Chaparro et al., 2017).
The rumen simulation technique (RUSITEC; Czerkawski and Breckenridge, 1977) was used to study the effect of a control diet alone or supplemented with either L. origanoides Kunth at 200 µL/L (132 µL/d), P. guilliermondii at 500 mg/d or a combination of both; these doses were selected based on a dose-response study with oregano oil (unpublished) and previous work of the research group with live strains of S. cerevisae (Newbold et al., 1996). Experimental diets had a 50:50 forage to concentrate ratio and ingredients (hay, barley, soya) were provided chopped to promote microbial attachment and fiber degradability. Ingredients and chemical composition of the experimental diet is shown in Supplemental Table 1.
Rumen digesta was obtained from four rumen-cannulated Holsten–Frisian cows fed a maintenance diet composed of 67% perennial ryegrass hay and 33% concentrate, on a DM basis. Rumen digesta was obtained before the morning feeding, strained through two layers of muslin, transferred to preheated thermo-flasks (39°C) and transported immediately to the laboratory. The trial consisted of a single incubation period using 16 vessels that were considered as experimental units. Each dietary treatment was randomly allocated to the vessels that were inoculated with rumen fluid from four different cows (four replicates). Vessels had an effective volume of 800 mL and were kept at 39°C under permanent vertical agitation.
On day 1, vessels were inoculated with strained rumen fluid mixed with artificial saliva (McDougall, 1948) and demineralised water in a 1:1:1 ratio. Then, artificial saliva was continuously infused at a rate of 660 mL d−1 (dilution rate of 3%/h) using a multichannel peristaltic pump (Watson–Marlow 200 series, Cornwall, UK). For each vessel, squeezed rumen solids (20 g fresh matter) were placed in a nylon bag (110 × 60 mm, pore size 100 μm2) and incubated for 1 d to provide solid-associated bacteria, while experimental feed (20 g fresh matter) was supplied in a second bag. On subsequent days, the feed bag that had remained 2 d in each vessel was squeezed, returning the liquid to the vessel, and discarded; a new bag, containing 20 g fresh matter was then inserted to the vessel.
The trial lasted for 16 d, using the first 12 d for adaptation and the last 4 d for sampling. Dry matter degradation, total gas and methane production and outflow of fermentation products were measured on days 13, 14, 15 and 16. Nylon bags were collected, rinsed with cold water for 20 min, and DM disappearance after 48 h incubation was calculated from the loss in weight. The residue was then analysed for energy, OM, nitrogen (N), NDF and ADF to determine gross energy and nutrient disappearance. Fermentation gases were collected in gas-tight bags (TECOBAG 5L, PETP/AL/PE-12/12/75, Tesseraux container GmbH, Germany) to measure total gas and methane production. Daily production of ammonia and volatile fatty acids (VFA) were measured in the overflow flasks with 10 mL of saturated HgCl2 (diluted 1:5) added to stop the fermentation. For VFA determination, 1.6 mL of sample was diluted with 0.4 mL of deproteinising solution (200 mL L−1 orthophosphoric acid containing 20 mmol L−1 of 2-ethylbutyric acid as an internal standard). For ammonia analysis, 0.8 mL of sample was diluted with 0.2 mL of trichloro-acetate (25% wt:vol). Samples for microbial characterization (15 mL) were collected on days 15 and 16, pooled together and immediately frozen in liquid N2 prior to long- term storage at -80C.
For feed analysis, DM and OM content were determined by drying in an oven at 105°C for 24 h and heating at 550°C for 6 h in a muffle furnace, respectively. Nitrogen concentration was measured by the Dumas combustion method (Elementar analyser, Vario MAX cube, Hanau, Germany). For NDF and ADF determination, the automated fibre analyser (ANKOM 2000, Macedon, USA) was used. Energy was calculated using a bomb calorimeter (6400 Calorimeter, Parr, Moline, IL). Methane concentration was determined by directly injecting 0.5 mL of gas sample into a gas chromatograph (ATI Unicam 610 Series, Cambridge, UK) fitted with a 40 cm Porapak N metal packed column (Agilent, Cheshire, UK) and flame ionisation detector. Ammonia and VFA concentrations in vessels and overflows were determined as described by Weatherburn (1967) using an automated spectrophotometer (ChemWell T,Astoria Pacific, Oregon, USA) and Stewart and Duncan (1985) using gas chromatography, respectively.
Genomic DNA was extracted from pooled samples withdrawn on days 15 and 16 from each vessel. Freeze-dried samples (25 mg DM) were bead beaten in 4% SDS lysis buffer for 45 seconds and DNA was extracted using a CTAB/Chloroform method (adapted from Yu and Morrison, 2004) in that lysis of cells was achieved by incubating with sodium dodecyl sulphate (SDS) buffer for 10 min at 95°C and potassium acetate was substituted for phenol in removing proteins. Concentration and quality of genomic DNA was assessed by spectrophotometry (Nanodrop ND-100, Thermo Scientific, USA). Absolute concentrations of DNA from total bacteria, methanogens, fungi, and protozoa were determined by qPCR and serial dilutions of their respective standards (10−1–10−5) as previously described (Belanche et al., 2012; Belanche et al., 2016). Quantitative PCR (qPCR) was conducted in triplicate using a LightCycler 480 System (Roche, Mannheim, Germany).
Rumen bacterial and methanogenic archaeal communities were studied using high-throughput sequencing (de la Fuente et al., 2014). For bacterial profiling, amplification of the V1–V2 hypervariable regions of the 16S rRNA gene was carried out using bacterial primers (27F and 357R; Spear et al., 2008) followed by Ion Torrent adaptors. For methanogens profiling, amplification of the V2-V3 hypervariable region of the 16S rRNA gene was performed using archaeal primers (86F and 519R; Wright and Pimm, 2003) also followed by Ion Torrent adaptors.
Forward primers were barcoded with 10 nucleotides to allow sample identification. PCR was carried out on a 25 μL reaction containing DNA template (1 μL), 0.2 μL reverse primer, 1 μL forward primer, 5 μL buffer (PCR Biosystems Ltd., London, UK), 0.25 μL bio HiFi polymerase (PCR Biosystems) and 17.6 μL molecular grade water. Amplification conditions for bacteria and methanogens were 95°C for 1 min, and then 22 cycles of 95°C for 15 s, 55°C for 15 s and 72°C for 30 seconds. To assess quality of amplifications, resultant amplicons were visualised on a 1% agarose gel. PCR products were then purified using Agencourt AMpure XP beads (Beckman Coulter Inc., Fullerton, USA), and DNA concentration was determined using an Epoch Microplate Spectrophotometer fitted with a Take 3 Micro-Volume plate (BioTek, Potton, UK) to enable equimolar pooling of samples with unique barcodes.
Libraries were further purified using the EGel system with 2% agarose gel (Life Technologies Ltd., Paisley, UK). Purified libraries were assessed for quality and quantified on an Agilent 2100 Bioanalyzer with High Sensitivity DNA chip (Agilent Technologies Ltd., Stockport, UK). Library preparation for NGS sequencing was carried out using the Ion Chef system (Life Technologies UK Ltd) and the Ion PGM HiQ Chef kit, and sequencing using the Ion Torrent Personal Genome Machine (PGM) system on an Ion PGM Sequencing 316 Chip v2 BC. Due to the lower abundance of methanogens than total bacteria, the methanogens library was sequenced using a smaller chip (Ion PGM Sequencing 314 Chip v2).
Following sequencing, data were processed as previously described (de la Fuente et al., 2014). Briefly, taxonomy was assigned to the OTUs using the mothur software package v. 1.36.0. Data were denoised by removing low-quality sequences, sequencing errors and chimeras (quality parameters: maximum 10 homopolymers, qaverage 13, qwindow 25, for archaea the qwindow was set at 30, and erate = 1; Chimera check, both de novo and database driven using Uchime).
Sequences were clustered into OTUs using the Uparse pipeline at 97% identity. Bacterial taxonomic information on 16S rRNA gene sequences was obtained by comparing against Ribosomal Database Project-II (Wang et al., 2007), while the methanogens were compared with the RIM-DB database (Seedorf et al., 2014). The number of reads per sample was normalised to the sample with the lowest number of sequences. To exclude potential bacterial sequences from the methanogens dataset, methanogens sequences were aligned to the RDP-II with BLAST, and those OTUs identified as bacteria were removed.
Metabolic hydrogen production, incorporation into fermentation products, and recovery, were calculated from the stoichiometry of reducing equivalents released in acetate, propionate, butyrate and methane as described by Moss et al. (2000). Daily production of VFA and ammonia in the RUSITEC system, nutrient and energy disappearance, methane data and diversity indexes, were analysed statistically following a factorial design with EO (essential oil) and Y (yeast) as main factors and, with individual cows as a blocking term. The effect of the factors and their interaction on the relative abundance of different bacterial and archaeal taxa was analysed in a similar way, with P values adjusted for multiple testing using the method proposed by Benjamini and Hochberg (1995) to decrease the false discovery rate. When effects were detected, treatment means were compared by Fisher’s protected LSD test. Findings with P < 0.05, P < 0.10 when applying Benjamini and Hochberg (1995) correction, were regarded statistically significant. Genstat 15th Edition (VSN International, Hemel Hempstead, UK) was used.
Permutation multivariate analysis of variance (PERMANOVA) was used to determine overall significant differences in bacterial and archaeal community structure and was performed in PRIMER 6 and PERMANOVA + (versions 6.1.18 and 1.0.8, respectively; Primer-E, Ivybridge, UK). For both bacterial and archaeal communities, OTUs abundance percentage data were subjected to square root transformation and Bray–Curtis distance matrices calculated. PERMANOVA was carried out using default settings with 9999 unrestricted permutations, and the Monte Carlo P value was calculated. Analysis of Similarity (ANOSIM) was carried out in PRIMER 6 and PERMANOVA + using the Bray–Curtis distance matrix calculated above. This analysis was used to provide a metric of the degree of divergence between communities as given by the R statistic.
To calculate the contribution of environmental data on bacteria and archaea communities, distance-based linear modelling was used to calculate which environmental variables had a significant correlation with the community data. Significant variables were used in distance-based redundancy analysis (dbRDA) (Legendre and Anderson, 1999) as implemented in PRIMER 6 and PERMANOVA+.
Treatments including oregano oil decreased (Table 1) feed and energy disappearance after 48 h of incubation (P<0.001), decreasing DM, OM and N disappearance by 13-16%, NDF and ADF disappearance by 32-40% and energy disappearance by 12-22%. Similarly, the addition of oregano oil had a major effect on fermentation (Table 2), decreasing total VFA and ammonia production (P<0.001) by 29-32% and 59-66%, respectively, as compared with the control. Also, oregano oil promoted a decrease in daily gas (P=0.012; 16-18%) and methane production (P=0.002; 28-32%), both in absolute terms and per unit of DM degraded. Stoichiometry calculations showed that treatments including oregano oil had both lower 2H production and 2H incorporation (around 30% less), compared with the control and yeast only treatments, resulting in a similar recovery (about 100%) for all treatments. The addition of yeast did not affect feed disappearance or the fermentation pattern (P≥0.18). The combination of oregano oil and yeast had a similar effect to that observed when feeding the oil only, with no clear interaction effects observed.
Table 1 Effect of supplementing a control diet (C) with oregano oil (EO), yeast (Y) or a combination of both (EO+Y) on feed and energy disappearance in the RUSITEC system.
Table 2 Effect of supplementing a control diet (C) with oregano oil (EO), yeast (Y) or a combination of both (EO+Y) on fermentation products and methanogenesis in the RUSITEC system.
Quantitative PCR (Table 3) revealed decreases in the relative abundance of anaerobic fungi (P=0.005; 8-23%) and protozoa (P<0.001; 24%) with oregano oil. A slight decrease (P=0.032; 4%) in anaerobic fungi was also observed in vessels supplemented with yeast. Again, no interaction between oregano oil and yeast treatments was observed (P>0.05). No effect of treatments on the relative abundance of bacteria (P>0.5) or archaea (P>0.2) was observed.
Table 3 Effect of supplementing a control diet (C) with oregano oil (EO), yeast (Y) or a combination of both (EO+Y) on microbial numbers in the RUSITEC system.
Quality filtering resulted in 1,860,849 high quality sequences that clustered into 2,039 different OTUs with 57,910 reads per sample after normalization.
Permutational analysis of the variance (Table 4) showed a strong effect of treatment on the structure of the bacterial community (P=0.001). Pairwise comparison revealed that the structure of the bacterial community differed between treatments including oregano oil (EO and EO+Y) and control (P=0.001) and yeast only treatments (P=0.001). The dramatic difference in the structure of the bacterial community between these treatments was confirmed by ANOSIM (P=0.001; R=1).
Table 4 Effect of supplementing a control diet (C) with oregano oil (EO), yeast (Y) or a combination of both (EO+Y) on the structure of the bacterial and methanogens communities in the RUSITEC system.
To detect correlations between the structure of the bacterial community and rumen fermentation parameters, dbRDA was performed with Bray-Curtis dissimilarities. The primary axis accounted for 69.2% of total variation, and an obvious separation between treatments including oregano oil and control and only yeast treatments was observed (Figure 1). Total VFA (P=0.001), acetic (P=0.002) and propionic production (P=0.056) were negatively correlated to the structure of the bacterial community of samples from vessels fed with oregano oil (alone or with yeast).
Figure 1 DbRDA illustrating the relationship between the structure of the bacterial community with the rumen fermentation pattern in the RUSITEC system. Arrows show the direction of the gradient. Samples belonging to each treatment are represented by blue and red triangles, essential oil alone or combined with yeast, respectively, green square for samples of the control treatment, and pink rhombus for those of the yeast treatment.
Regarding bacterial diversity (Table 5), treatments including oregano oil decreased both Simpson’s (P=0.011) and Shannon’s (P<0.001) indexes. Bacterial richness also decreased (P<0.001) when feeding oregano oil, either on its own or combined with yeast.
Table 5 Effect of supplementing a control diet (C) with oregano oil (EO), yeast (Y) or a combination of both (EO+Y) on the richness (number of OTUs) and diversity of the bacteria and methanogen communities in the RUSITEC system.
Overall, oregano oil had a great impact on the bacterial community structure. At the phylum level (Table 6), whilst no significant effect on the relative abundance of Bacteroidetes (P=0.991), the most abundant phylum across diets (53%), was observed, the relative abundance of Firmicutes, which made up 28% of the bacterial community, increased (P=0.005) with oregano oil. The relative abundance of other phyla decreased (P<0.005) in vessels supplemented with oregano oil or oregano oil plus yeast, with the greatest effect observed for Fibrobacteres and Elusimicrobia, which were almost completely inhibited, followed by Proteobacteria and Verrucomicrobia.
Table 6 Effect of supplementing a control diet (C) with oregano oil (EO), yeast (Y) or a combination of both (EO+Y) on the relative abundance of bacteria phyla present at an average of more than 0.5% (false discovery rate for Benjamini-Hochberg:0.25).
Within the Bacteroidetes phylum, oregano oil promoted a shift at the family level, with the greatest effect in the relative abundance observed for Prevotellacea and Marinilabiliaceae which increased and decreased, respectively (P=0.001; Supplementary Table 2). In case of Firmicutes, the relative abundance of the families Erysipelotrichaceae, Acidaminococcaceae and Christensenellaceae, increased (P<0.001; Supplementary Table 2). At the genus level (Table 7), the greatest impact on the relative abundance of bacteria was the increase in Sharpea (Erysipelotrichaceae family) and Prevotella (Prevotellacea Family) and the decrease in the unclassified bacteria (P=0.002), followed by that of Fibrobacter (Fibrobacteraceae family).
Table 7 Effect of supplementing a control diet (C) with oregano oil (EO), yeast (Y) or a combination of both (EO+Y) on the relative abundance of bacteria genera present at an average of more than 0.2% (false discovery rate for Benjamini-Hochberg:0.25).
Quality filtering and removal of bacterial sequences resulted in 2,188,976 high-quality methanogen sequences that were clustered into 36 unique OTUs with 50,536 sequences per sample after normalization.
Permutational analysis of the variance revealed a clear effect of oregano oil on the structure of the archaeal community (Table 4), that was similar to that observed on the structure of the bacterial community. The high separation between samples from vessels fed oregano oil, either alone or with yeast, and those vessels belonging to control and yeast treatments, was also shown by ANOSIM (P=0.001, R=1). dbRDA primary axes displayed 71.7% of the total variation and a clear separation between treatments containing oregano oil and those of control and yeast only (Figure 2). Total VFA (P=0.001), acetic (P=0.004), propionic (P=0.024) and ammonia production (P=0.016) were negatively correlated to the structure of the archaeal population in vessels corresponding to the essential oil with or without yeast.
Figure 2 DbRDA illustrating the relationship between the structure of the archaeal community with the rumen fermentation pattern in the RUSITEC system. Arrows show the direction of the gradient. Samples belonging to each treatment are represented by blue and red triangles, essential oil alone or combined with yeast, respectively, green square for samples of the control treatment, and pink rhombus for those of the yeast treatment.
Similar to the effects on the bacterial community structure, methanogen richness and diversity decreased in the presence of oregano oil (Table 5; P< 0.05). Oregano oil had a major effect on the relative abundance of archaeal families in this experiment (Table 8), increasing the abundance of Methanomassiliicoccaceae and Methanobacteriaceae, and decreasing that of Methanomicrobiaceae. Within the family Methanomassiliicoccaceae, oregano oil promoted the substitution of Group G9 and G3b by groups G11 and G10. Oregano oil also increased the relative abundance of Methanobrevibacter and decreased that of Metanomicrobium.
Table 8 Effect of supplementing a control diet (C) with oregano oil (EO), yeast (Y) or a combination of both (EO+Y) on the relative abundance of archaeal genera present at an average of more than 0.2% (false discovery rate for Benjamini-Hochberg:0.25).
Our study was based on three interlinked hypotheses:
1. Oil extracted from L. origanoides Kunth would inhibit methanogenesis but might also inhibit the overall fermentation process.
2. The yeast P. guilliermondii LV196 would stimulate microbial numbers and microbial activity.
3. By combining L. origanoides Kunth oil with P. guilliermondii LV196, the yeast would help to overcome the general inhibition in fermentation caused by the essential oil, leading to lower methane production but no adverse effect on fiber degradation and rumen fermentation.
Hypotheses one was clearly confirmed. Methane production was decreased both in absolute terms and per unit of DM degraded but this was associated with a major shift in the bacterial population and an overall inhibition of fermentation. Essential oils such as oregano oil have been shown to slow down starch and protein degradation, reducing the risk of acidosis when feeding high concentrate diets and increasing the efficiency of N utilization, while reducing methane production (McIntosh et al., 2003; Calsamiglia et al., 2007; Patra, 2011). However, adverse effects on fiber digestion depending on the type and dose of essential oil, as well as basal diet, have also been reported (Calsamiglia et al., 2007; Macheboeuf et al., 2008). Gram-positive bacteria seem to be more susceptible to essential oils than gram-negative, due to their lack of a protective outer membrane surrounding the cell wall (Patra and Yu, 2012). However, essential oils have shown a wide range of antimicrobial activities (Cobellis et al., 2016). Most of the studies with oregano oil have been focused on Origanum spp. with high carvacrol content. The present study evaluated the effect of L. origanoides Kunth, a high thymol- containing species native to the arid and dry ecosystem of Alto Patía in Colombia, as a rumen modulator. To our knowledge, this is the first time that L. origanoides Kunth has been evaluated in a long-term in vitro system with an in depth study of the changes in microbial populations. Both thymol and carvacrol have been reported to have a strong and broad antimicrobial activity due to the presence of a hydroxyl group in their phenolic structure (Calsamiglia et al., 2007; Benchaar and Greathead, 2011). The dose used in our study was based on a preliminary short term in vitro study (data not shown) carried out with increasing doses of L. origanoides Kunth (100, 200 and 400 mg/L), that were selected based on literature available (Calsamiglia et al., 2007). A recent study by Yu et al. (2020), testing those same doses of pure thymol in 24 h in vitro incubations, also suggested that 200 mg/L might improve rumen fermentation.
Several short-term in vitro studies have shown that oregano oil and carvacrol affect N metabolism via the reduction of protein degradation and ammonia production (Benchaar et al., 2008). Reductions in methane emissions have also been reported in the presence of essential oil from Origanum spp. (Benchaar and Greathead, 2011; Cobellis et al., 2016). However, relatively high concentrations of oregano oil can cause an overall reduction in rumen fermentation as compared with other less potent essential oils (Patra and Yu, 2012). Regarding ruminal microorganisms, Zhou et al. (2019) reported that depending on the dose of oregano oil, microbial populations could be affected positively or negatively. In the present study, feeding L. origanoides had a detrimental effect on rumen fermentation, with decreases in nutrient disappearance as well as VFA and ammonia production observed. A decrease in the concentration of fungi and protozoa, which play a significant role in the degradation of plant cellulosic fibres, was also observed in the presence of oregano oil. Although total bacterial and archaeal numbers remained unchanged, oregano oil promoted a much lower bacterial richness and a less diverse bacterial and archaeal community. ANOSIM showed that both, the bacterial and archaeal community structure was strongly affected by treatments containing oregano oil. The dramatic impact of oregano oil on the composition of both the bacterial and archaeal communities further confirmed its profound inhibitory effect on the overall fermentation, at the concentration tested. Yu et al. (2020), when testing thymol in vitro, showed changes in the major phyla, with a decrease in the relative abundance of Bacteroidetes and an increase in that of Firmicutes. In our study we found a slight but not statistically significant decrease in the abundance of Bacteroidetes, and a substantial increase in that of Firmicutes. At the family level, we observed that the bacterial community of vessels fed with oregano oil was enriched in Erysipelotrichaceae, especially Sharpea spp., which has been reported to be characteristic of low methane yield animals (Kamke et al., 2016). This increase, as well as that in the relative abundance of Prevotella, involved in protein degradation (Wallace et al., 1997) and reported to be abundant when methane is inhibited (Tapio et al., 2017), was compensated with a decrease in the abundance of unclassified bacteria. Zhou et al. (2020) also reported an increased relative abundance of Prevotella in the presence of oregano oil, which they attributed to reduced competition from other bacteria that are inhibited by this essential oil. Regarding effects on the composition of the archaeal communities when adding oregano oil, there was a dramatic increase in the relative abundance of members of the Methanomassiliicoccaceae, and to a lesser extent, of those belonging to the Methanobacteriaceae, that was compensated by a decrease in Methanomicrobiaceae. The greatest effect was observed for the genus Methanomicrobium, which in this study and opposite to many others in which Methanobrevibacter strains account for the majority of methanogens in the rumen (Henderson et al., 2015; Friedman et al., 2017), made up more than half of the archaeal population, and almost disappeared with the addition of the oil.
Whilst hypothesis one was confirmed, hypothesis two was not, with no indication of P. guilliermondii LV196 stimulating either microbial numbers or activity. Yeast products, either live or inactivated, are widely used as feed additives in ruminants to improve rumen fermentation and performance. Their effects are variable, depending on yeast strain, viability, yeast culture and media associated with it, and post-fermentation processing (Shurson, 2018). Nevertheless, it has been suggested that supplementation with yeast cell walls could have similar effects (improved fibre digestion, decreased acetate:propionate ratio and reduced methane production) to those observed when feeding live yeast (McCann et al., 2017). The effects observed with inactivated yeast cultures have been attributed to functional metabolites that include uncharacterized yeast growth factors, B vitamins, amino acids, organic acids, and other fermentation products that can stimulate bacterial growth and lead to increased microbial protein production, fiber digestion, or increased utilization of fermentation end products (Miller-Webster et al., 2002; Moallem et al., 2009; Robinson and Erasmus, 2009). Our results showed that the inclusion of P. gillermondii LV196 had no effect on fermentation parameters or nutrient utilization. Neither changes in microbial abundances or in the structure of bacterial and archaeal communities were observed in the presence of P guillermondii. Since inactive yeast may act only by providing stimulatory factors as compared to live yeast, which could either compete for substrates or stimulate specific rumen bacteria, it is reasonable to think that higher doses of inactivated yeast would be needed to offset their lack of biological activity in order to improve their action in rumen.
The failure to support hypothesis two means that hypothesis three is untestable, the yeast did not stimulate microbial numbers nor activity, thus could not be expected to act as to counterbalance the antimicrobial effect of the essential oil. Future studies need to both investigate lower levels of essential oil addition and also re-examine the effects of P. guillermondii in the rumen and/or to replace it with other yeast of known biological activity when combined with oils extracted from L. origanoides kunth.
The datasets presented in this study can be found in online repositories. The names of the repository/repositories and accession number(s) can be found below: European Nucleotide Archive (accession number PRJEB52435).
The animal study was reviewed and approved by Aberystwyth University Ethical Committee.
CJN, OM and CA-N secured the funding for the study. CJN, OM, CA-N, and ER-M conceived and designed the study. ER-M, EB, and LL conducted the trial and collected the samples. EB, LL, DC and EJ performed the laboratory analysis. ER-M conducted data analysis, interpreted the results and drafted the manuscript. CJN reviewed and edited the manuscript. All authors read and approved the final manuscript.
The authors wish to express their appreciation for the financial support provided by British Council, Newton Institutional Links (project: Improving competitiveness, resilience, and sustainability of dairy production in the high tropics of Colombia).
The authors would like to acknowledge the technical support received by Hilary Worgan and Susan Girdwood at Aberystwyth University.
The authors declare that the research was conducted in the absence of any commercial or financial relationships that could be construed as a potential conflict of interest.
All claims expressed in this article are solely those of the authors and do not necessarily represent those of their affiliated organizations, or those of the publisher, the editors and the reviewers. Any product that may be evaluated in this article, or claim that may be made by its manufacturer, is not guaranteed or endorsed by the publisher.
The Supplementary Material for this article can be found online at: https://www.frontiersin.org/articles/10.3389/fanim.2022.951789/full#supplementary-material.
Arango J., Ruden A., Martinez-Baron D., Loboguerrero A. M., Berndt A., Chacón M., et al. (2020). Ambition meets reality: Achieving GHG emission reduction targets in the livestock sector of Latin America. Front. Sustain. Food Syst. 4. doi: 10.3389/fsufs.2020.00065
Bass B. E., Tsai T.-C., Yang H., Perez V., Holzgraefe D., Chewning J., et al. (2019). Influence of a whole yeast product (Pichia guilliermondii) fed throughout gestation and lactation on performance and immune parameters of the sow and litter. J. Anim. Sci. 97, 1671–1678. doi: 10.1093/jas/skz060
Belanche A., Doreau M., Edwards J. E., Moorby J. M., Pinloche E., Newbold C. J. (2012). Shifts in the rumen microbiota due to the type of carbohydrate and level of protein ingested by dairy cattle are associated with changes in rumen fermentation. J. Nutr. 142, 1684–1689. doi: 10.3945/jn.112.159574
Belanche A., Pinloche E., Preskett D., Newbold C. J. (2016). Effects and mode of action of chitosan and ivy fruit saponins on the microbiome, fermentation and methanogenesis in the rumen simulation technique. FEMS Microbiol. Ecol. 92, 1. doi: 10.1093/femsec/fiv160
Benchaar C., Calsamiglia S., Chaves A. V., Fraser G. R., Colombatto D., McAllister T. A., et al. (2008). A review of plant-derived essential oils in ruminant nutrition and production. Anim. Feed Sci. Technol. 145, 209–228. doi: 10.1016/j.anifeedsci.2007.04.014
Benchaar C., Greathead H. (2011). Essential oils and opportunities to mitigate enteric methane emissions from ruminants. Anim. Feed Sci. Technol. 166–167, 338–355. doi: 10.1016/j.anifeedsci.2011.04.024
Benjamini Y., Hochberg Y. (1955). Controlling the false discovery rate— a practical and powerful approach to multiple testing. J. R. Stat. Soc Ser. B Stat. Methodol. 57, 289–300.
Betancourt L., Hume M., Rodríguez F., Nisbet D., Sohail M. U., Afanador-Tellez G. (2019). Effects of Colombian oregano essential oil (Lippia origanoides kunth) and eimeria species on broiler production and cecal microbiota. Poult. Sci. 98, 4777–4786. doi: 10.3382/ps/pez193
Calsamiglia S., Busquet M., Cardozo P. W., Castillejos L., Ferret A. (2007). Invited review: Essential oils as modifiers of rumen microbial fermentation. J. Anim. Sci. 90, 2580–2595. doi: 10.3168/jds.2006-644
Chaparro M. L., Céspedes E., Cruz M., Castillo-Saldarriaga C. R., Gómez- Alvarez M. I. (2017). Fluidized bed drying of a granulated prototype based on a potential probiotic yeast Meyerozyma guilliermondii: Selection of process parameters and drying protectant. Rev. Mex. Ing. Quim. 16, 347–357.
Cobellis G., Trabalza-Marinucci M., Yu Z. (2016). Critical evaluation of essential oils as rumen modifiers in ruminant nutrition: A review. Sci. Total Environ., 545–546, 556–568. doi: 10.1016/j.scitotenv.2015.12.103
Czerkawski J. W., Breckenridge G. (1977). Design and development of a long-term rumen simulation technique (RUSITEC). Br. J. Nutr. 38, 371–384. doi: 10.1079/BJN19770102
de la Fuente G., Belanche A., Girwood S. E., Pinloche E., Wilkinson T., Newbold C. J. (2014). Pros and cons of ion-torrent next generation sequencing versus terminal restriction fragment length polymorphism T-RFLP for studying the rumen bacterial community. PLoS One 9, 1–13. doi: 10.1371/journal.pone.0101435
Evans J. D., Martin S. A. (2000). Effects of thymol on ruminal microorganisms. Curr. Microbiol. 41, 336–340. doi: 10.1007/s002840010145
Friedman N., Jami E., Mizrahi I. (2017). Compositional and functional dynamics of the bovine rumen methanogenic community across different developmental stages. Environ. Microbiol. 19, 3365–3373. doi: 10.1111/1462-2920.13846
Henderson G., Cox F., Ganesh S., Jonker A., Young W., Janssen P. H. (2015). Rumen microbial community composition varies with diet and host, but a core microbiome is found across a wide geographical range. Sci. Rep. 5, 14567. doi: 10.1038/srep14567
Kamke J., Kittelmann S., Soni P., Li Y., Tavendale M., Ganesh S., et al. (2016). Rumen metagenome and metatranscriptome analyses of low methane yield sheep reveals a sharpea-enriched microbiome characterised by lactic acid formation and utilisation. Microbiome 4, 56. doi: 10.1186/s40168-016-0201-2
Legendre P., Anderson M. J. (1999). Distance-based redundancy analysis: testing multispecies responses in multifactorial ecological experiments. Ecol. Monogr. 69, 1–24. doi: 10.1890/0012-9615(1999)069[0001:DBRATM]2.0.CO;2
Macheboeuf D., Morgavi D. P., Papon Y., Mousset J. L., Arturo-Schaanc M. (2008). Dose–response effects of essential oils on in vitro fermentation activity of the rumen microbial population. Anim. Feed Sci. Technol. 145, 335–350. doi: 10.1016/j.anifeedsci.2007.05.044
Marrero Y., Sosa D., Rodríguez R., García Y. (2016). Inclusion of Pichia guilliermondii on different culture media, on in vitro fermentation of cynodon nlemfuensis. Cuban J. Agr. Sci. 50, 403–409.
McCann J. C., Elolimy A. A., Loor J. J. (2017). Rumen microbiome, probiotics, and fermentation additives. Vet. Clin. Food Anim. Pract. 33, 539–553. doi: 10.1016/j.cvfa.2017.06.009
McDougall E. I. (1948). Studies on ruminant saliva. 1. the composition and output of sheep’s saliva. Biochem. J. 43, 99–109. doi: 10.1042/bj0430099
McIntosh F. M., Williams P., Losa R., Wallace R. J., Beever D. A., Newbold C. J. (2003). Effects of essential oils on ruminal microorganisms and their protein metabolism. appl. environ. Microbiol 69, 5011–5014. doi: 10.1128/AEM.69.8.5011-5014.2003
Miller-Webster T., Hoover W. H., Holt M., Nocek J. E. (2002). Influence of yeast culture on ruminal microbial metabolism in continuous culture. J. Dairy Sci. 85, 2009–2014. doi: 10.3168/jds.S0022-0302(02)74277-X
Moallem U., Lehrer H., Livshitz L., Zachut M., Yakoby S. (2009). The effects of live yeast supplementation to dairy cows during the hot season on production, feed efficiency, and digestibility. J. Dairy Sci. 92, 343–351. doi: 10.3168/jds.2007-0839
Moss A. R., Jouany J. P., Newbold C. J. (2000). Methane production by ruminants: Its contribution to global warming. Ann. Zootech. 49, 231–253. doi: 10.1051/animres:2000119
Newbold C. J., Ramos-Morales E. (2020). Review: Ruminal microbiome and microbial metabolome: effects of diet and ruminant host. Animal 14, 78–86. doi: 10.1017/S1751731119003252
Newbold C. J., Wallace R. J., Mcintosh F. M. (1996). Mode of action of the yeast saccharomyces cerevisiae as a feed additive for ruminants. Br. J. Nutr. 76, 249–261. doi: 10.1079/BJN19960029
Pastén M. G., Montenegro N. C., Trejo L. C., Flores S. V., Bunting L. D., Acetoze G. (2021). Effects of supplementation with a Pichia guilliermondii yeast cell product or essential oils on performance and health of dairy calves during an experimental coccidial infection. Appl. Anim. Sci. 37, 519–524. doi: 10.15232/aas.2021-02186
Patra A. K. (2011). Effects of essential oils on rumen fermentation, microbial ecology and ruminant production. Asian J. Anim. Vet. Adv. 6, 416–428. doi: 10.3923/ajava.2011.416.428
Patra A. K., Yu Z. (2012). Effects of essential oils on methane production and fermentation by, and abundance and diversity of, rumen microbial populations. Appl. Environ. Microbiol. 78, 4271–4280. doi: 10.1128/AEM.00309-12
Patra A. K., Yu Z. (2014). Combinations of nitrate, saponin, and sulfate additively reduce methane production by rumen cultures in vitro while not adversely affecting feed digestion, fermentation or microbial communities. Bioresour. Technol. 155, 129–135. doi: 10.1016/j.biortech.2013.12.099
Ramos-Morales E., Tibble-Howlings J., Lyons L., Ogbu M. O., Murphy P. J., Braganca R., et al. (2019). Slight changes in the chemical structure of haemanthamine greatly influence the effect of the derivatives on rumen fermentation in vitro. Sci. Rep. 9, 2440. doi: 10.1038/s41598-019-38977-x
Robinson P. H., Erasmus L. J. (2009). Effects of analyzable diet components on responses of lactating dairy cows to saccharomyces cerevisiae based yeast products: A systematic review of the literature. Anim. Feed Sci. Technol. 149, 185–198. doi: 10.1016/j.anifeedsci.2008.10.003
Rodríguez T., Chaparro M., Gómez M., Castillo C., García A., Ariza C., et al. (2015). A novel yeast strain Meyerozyma guilliermondii isolated from native fruits from Colombia ecosystems as a prospective probiotic to be used in dairy systems. Proceedings of the 2015 Congress on Gastrointestinal Function. Chigago. pg 56,
Rodríguez Quiroz T. A. (2014). Estudio del efecto del aceite esencial de orégano de monte (Lippia origanoides) del alto patía sobre la metanogénesis y la actividad fibrolítica del ecosistema ruminal. [master´s thesis] (Colombia: Universidad Nacional de Colombia). Available at: https://repositorio.unal.edu.co/handle/unal/75189.
Seedorf H., Kittelmann S., Henderson G., Hanssen P. H. (2014). RIM-DB: a taxonomic framework for community structure analysis of methanogenic archaea from the rumen and other intestinal environments. Peer J. 2, e494. doi: 10.7717/peerj.494
Shanmugasundaram R., Selvaraj R. K. (2012). Effect of killed whole yeast cell prebiotic supplementation on broiler performance and intestinal immune cell parameters. Poult. Sci. 91, 107–111. doi: 10.3382/ps.2011-01732
Shurson G. C. (2018). Yeast and yeast derivatives in feed additives and ingredients: Sources, characteristics, animal responses, and quantification methods. Anim. Feed Sci. Technol. 235, 60–76. doi: 10.1016/j.anifeedsci.2017.11.010
Spear G. T., Sikaroodi M., Zariffard M. R., Landay A. L., French A. L., Gillevet P. M. (2008). Comparison of the diversity of the vaginal microbiota in HIV-infected and HIV-uninfected women with or without bacterial vaginosis. J. Infect. Dis. 198, 1131–1140. doi: 10.1086/591942
Stewart C. S., Duncan S. H. (1985). The effect of avoparcin on cellulolytic bacteria of the ovine rumen. J. Gen. Microbiol. 131 427–35. doi: 10.1099/00221287-131-3-427
Suntara C., Cherdthong A., Uriyapongson S., Wanapat M., Chanjula P. (2021). Novel crabtree negative yeast from rumen fluids can improve rumen fermentation and milk quality. Sci. Rep. 11, 6236. doi: 10.1038/s41598-021-85643-2
Tapio I., Snelling T. J., Strozzi F., Wallace R. J. (2017). The ruminal microbiome associated with methane emissions from ruminant livestock. J. Anim. Sci. Biotechnol. 8, 7. doi: 10.1186/s40104-017-0141-0
Vásquez Carreño D. R. (2012). El Orégano del monte (Lippia origanoides) del alto patía: efecto del método de obtención de sus extractos sobre la composición y la actividad antioxidante de los mismos. [master´s thesis] (Colombia: Universidad Nacional de Colombia). Available at: https://repositorio.unal.edu.co/handle/unal/11580.
Wallace R. J., McKain N., Broderick G. A., Rode L. M., Walker N. D., Newbold C. J., et al. (1997). Peptidases of the rumen bacterium, prevotella ruminicola. Anaerobe 3, 35–42. doi: 10.1006/anae.1996.0065
Wang Q., Garrity G. M., Tiedje J. M., Cole J. R. (2007). Naive bayesian classifier for rapid assignment of rRNA sequences into the new bacterial taxonomy. Appl. Environ. Microbiol. 73, 5261–5267. doi: 10.1128/AEM.00062-07
Weatherburn M. W. (1967). Phenol-hypochlorite reaction for determination of ammonia. Anal. Chem. 39, 971–974. doi: 10.1021/ac60252a045
Wright A.-D. G., Pimm C. (2003). Improved strategy for presumptive identification of methanogens using 16S riboprinting. J. Microbiol. Methods 55, 337–349. doi: 10.1016/S0167-7012(03)00169-6
Yu J., Cai L., Zhang J., Yang A., Wang Y., Zhang L., et al. (2020). Effects of thymol supplementation on goat rumen fermentation and rumen microbiota in vitro. Microorganisms 8, 1160. doi: 10.3390/microorganisms8081160
Yu Z., Morrison M.. (2004). Improved extraction of PCR-quality community DNA from digesta and fecal samples. BioTechniques 36, 808–812. doi: 10.2144/04365ST04
Zhou R., Wu J., Lang X., Liu L., Casper D. P., Wang C., et al. (2020). Effects of oregano essential oil on in vitro ruminal fermentation, methane production, and ruminal microbial community. J. Dairy Sci. 103, 2303–2314. doi: 10.3168/jds.2019-16611
Keywords: Lippia, methane, microbiome, oregano oil, Pichia, rumen fermentation, yeast, RUSITEC
Citation: Ramos-Morales E, Bolton E, Lyons L, Carreño D, Jones E, Mayorga O, Ariza-Nieto C and Newbold CJ (2022) Evaluation of a Colombian oregano oil (Lippia origanoides Kunth) and a novel yeast product from Pichia guilliermondii, alone or in combination, on rumen fermentation, methanogenesis and the microbiome in the rumen simulation technique. Front. Anim. Sci. 3:951789. doi: 10.3389/fanim.2022.951789
Received: 24 May 2022; Accepted: 05 July 2022;
Published: 25 July 2022.
Edited by:
Todd Riley Callaway, University of Georgia, United StatesReviewed by:
Emilio M. Ungerfeld, Instituto de Investigaciones Agropecuarias, ChileCopyright © 2022 Ramos-Morales, Bolton, Lyons, Carreño, Jones, Mayorga, Ariza-Nieto and Newbold. This is an open-access article distributed under the terms of the Creative Commons Attribution License (CC BY). The use, distribution or reproduction in other forums is permitted, provided the original author(s) and the copyright owner(s) are credited and that the original publication in this journal is cited, in accordance with accepted academic practice. No use, distribution or reproduction is permitted which does not comply with these terms.
*Correspondence: Eva Ramos-Morales, ZXZhLnJhbW9zLW1vcmFsZXNAc3J1Yy5hYy51aw==
†Present address: Eva Ramos-Morales, Estación Experimental del Zaidín (EEZ), Consejo Superior de Investigaciones Científicas (CSIC), Granada, Spain
Disclaimer: All claims expressed in this article are solely those of the authors and do not necessarily represent those of their affiliated organizations, or those of the publisher, the editors and the reviewers. Any product that may be evaluated in this article or claim that may be made by its manufacturer is not guaranteed or endorsed by the publisher.
Research integrity at Frontiers
Learn more about the work of our research integrity team to safeguard the quality of each article we publish.