- 1COISPA Tecnologia & Ricerca, Experimental Station for the Study of Sea Resources, Bari, Italy
- 2National Reference Laboratory for Fish, Mollusc and Crustacean Diseases, Istituto Zooprofilattico Sperimentale delle Venezie, Legnaro, Italy
- 3OIE Reference Laboratory for Viral Encephalo-retinopathy, National Reference Laboratory for Fish Diseases, Istituto Zooprofilattico Sperimentale delle Venezie, Legnaro, Italy
- 4SCS3 - Special Diagnostics, Histopathology and Parasitology, Istituto Zooprofilattico Sperimentale delle Venezie, Legnaro, Italy
- 5Department of Earth and Marine Science, University of Palermo, Palermo, Italy
Stress coping styles (SCSs) are defined as coherent sets of individual physiological and behavioral differences in stress response consistent across time and context and are described in a wide range of taxa, including fishes. These differences in behavior and physiology are of great interest because they may have direct implications on animal health, welfare, and performance in farming systems, including aquaculture. In this study, the physiological responses of sea bream (Sparus aurata) from different SCSs following Vibrio anguillarum vaccination were monitored. Fish were first screened either bold or shy (proxy of proactive and reactive SCSs, respectively) using group risk-taking tests and were then injected with a vaccine against V. anguillarum. Following vaccination, the fish were implanted with an accelerometer tag to monitor their swimming activity (proxy of energy expenditure), and blood sampling was carried out to measure health and welfare parameters (e.g., cortisol, glucose, hemoglobin) and aspecific immunity (e.g., protease, total proteins). In addition, blood was also collected at three different sampling times to screen antibody levels and, thus, to evaluate the efficiency of the vaccine. Following vaccination, bold fish displayed lower swimming activity values, indicative of lower energy expenditure, and also displayed higher levels of hematocrit, total proteins, and lysozyme in the plasma than the shy ones, which could be indicative of better health/welfare status and greater aspecific immunity. Finally, the V. anguillarum vaccination appeared to be more efficient in bold fish since the number of total antibodies was found higher than in shy fish 1 month after vaccination. Such results could help improve both health/welfare and productivity of farmed sea breams by selecting more robust fish, better adapted to farming conditions.
Introduction
Stress coping style (SCS) has been defined as “a coherent set of individual physiological and behavioral differences in stress responses consistent across time and context” (Koolhaas et al., 1999). SCSs have been described in various animal species, including fishes, as a continuum between two extreme phenotypes, called proactive and reactive (Øverli et al., 2007; Castanheira et al., 2017). In terms of behavior, proactive fish are overall bolder and more aggressive, explore their environment faster, and display less flexible behavior than reactive fish (Castanheira et al., 2017; Geffroy et al., 2020). Moreover, the correlations between these behavioral responses across time and context are now established in several fish species, as well as between behavior and physiology (Castanheira et al., 2017), generally used for defining SCS.
Differences in behavioral and physiological responses to stress may have direct implications on the growth, reproduction, and fitness of animals, including in the context of aquaculture (Réale et al., 2010; Castanheira et al., 2017; Geffroy et al., 2020). On one hand, SCS may help to improve the welfare and management of the fish, by adapting housing conditions to fish with different SCSs (Castanheira et al., 2017). On the other hand, proactive fish sometimes showed better feeding motivation and feed efficiency than reactive ones (Castanheira et al., 2017), suggesting that selecting for proactivity could help to improve production. In addition, some recent studies have also pointed out that immunity features are different between the two SCSs, suggesting that proactive individuals have better immune response than reactive ones (Kittilsen et al., 2012; Vargas et al., 2018; Balasch et al., 2019; Sadoul et al., 2022). Altogether, a better understanding of SCS features could be very interesting for the aquaculture sector, but more work is needed including in the major farmed species, such as gilthead sea bream (Sparus aurata), an important species of the European aquaculture (FAO, 2020).
In this study, the physiological responses of sea bream with different SCSs following Vibrio anguillarum vaccination were monitored. Fish were first screened either as bold or shy (proxy of proactive and reactive SCSs, respectively) and were then vaccinated with a vaccine against V. anguillarum, which is one of the most important bacterial diseases affecting marine species (Austin and Austin, 2013; Zrncic, 2020). Following vaccination, the fish were implanted with an accelerometer tag to monitor their swimming activity, indicative of energy expenditure (Alfonso et al., 2021), and blood samples were carried out at different sampling times to measure health and welfare parameters and aspecific immunity. In addition, antibody screening was carried out to evaluate vaccine efficiency.
Materials and methods
All experiments were performed in accordance with EU recommendations (Directive 2010/63/EU) and the Italian national legislation (D. lgs. 26/2014) on fish welfare, with the authorization of the Italian Health Ministry (number code 665/2016-PR).
Fish rearing
A total of 159 fish (total length: 30.96 ± 0.92 cm; mass: 423.75 ± 46.16 g) have been randomly selected from a group of about 500 sea bream specimens (tagged under the skin with RFID transponders, Trovan Dorset Identification BV, Aalten, The Netherlands) reared in a 16-m3 fiberglass tank and divided into three different 1.2-m3 fiberglass tanks (see Carbonara et al., 2019). The average stocking density in the tanks was around ~19 kg/m3 (tank 1: 18.5 kg/m3, tank 2: 19.1 kg/m3, tank 3: 18.6 kg/m3). Fish were kept undisturbed for 2 weeks before running the SCS screening (see below). Over the trial duration, the fish were reared with a constant photoperiod of 12:12 h (dark/light) in salt water (35‰) at a constant temperature of 18°C. The experimental conditions were maintained for the entire duration of the experiment. The fish were fed once a day, with 1% of their total body weight with commercial pellets Marine 3P (Skretting, Vignetto, Italy) using automatic feeders. The level of dissolved oxygen in the tanks was monitored continuously and remotely through an automatic system scheduled to maintain the concentration on levels above the threshold of 5.0 ± 1.0 ppm with the release of pure oxygen.
SCS screening, implantation of the accelerometer tag, and analysis
The fish were subjected to group risk-taking tests (RT) to screen bold and shy individuals following a similar method described in Carbonara et al. (2019). The RT has been chosen because it appeared to be the most sensitive and stable over the time test, to screen SCS in sea bream (Castanheira et al., 2013; Alfonso et al., 2020b), mostly in comparison to other screening tests, such as the hypoxia test (Ferrari et al., 2015; Alfonso et al., 2020b). Briefly, the tests were conducted two times with 1-week interval in the rearing tanks to minimize handling stress; the rearing tank was divided into two equal areas (safe and risky area) by a panel with a hole in the middle (30 cm in diameter). After acclimation overnight in the safe area, the hole was opened at 10 a.m. the next day, allowing fish to pass freely from the safe zone to the unknown risky zone. An antenna (Dorset, Netherlands Dorset Identification BV, Aalten, The Netherlands) connected to a computer was placed around the hole to detect the RFID tag of the fish, and every time the fish passed through the hole, the fish ID was recorded. Data were stored for each fish about the time elapsed until the first passage and the number of passages through the hole. The risk-taking test was ended when half of the fish exited the safe area or 4.5 h after the beginning of the experiment (Castanheira et al., 2013). A score of 1 was assigned to the first half of the fish that passed into the risky area at the end of the experiment. Another score of 1 was assigned to the fish with a number of passages throughout the hole greater than the 67th percentile of the tank population (Carbonara et al., 2019). In the other cases, a score of 0 was assigned to the fish. Scores for each fish were summed between the two tests. Fish with scores >3 were considered as bold and fish with scores <1 were considered as shy (Carbonara et al., 2019).
Following the risk-taking tests, the accelerometer tags VEMCO V9AP (AMIRIX Systems Inc., Nova Scotia, Canada; length: 43 mm; weight: 6.1 g in air and 3.3 g in water) were surgically implanted into the body cavity of n = 18 fish (n = 3 bold and 3 shy randomly selected from each tank; i.e., n = 9 for each personality type) following the method described in Alfonso et al. (2020a). Briefly, the fish were fasted 24 h before the surgery and then anesthetized using a 30-mg/L hydroalcoholic clove oil solution (Erbofarmosan, Bari, Italy). The tag was implanted in the body cavity of the sea bream through an ~1.5-cm incision and then carefully sutured. The gills were continuously irrigated with the anesthetic solution (30 mg/L) during the whole surgical procedure. After surgery, an antibiotic injection (sodic ampicillin–cloxacillin; 1 mg/kg) was carried out and fish were kept undisturbed in the recovery tank until the beginning of the trial (t0). In the days following the surgical procedure, feeding and behavior of fish have been monitored to ensure that all fish recovered well from the procedure. Typically, all fish started again to eat 1–2 days following the surgery and displayed normal swimming behavior, ensuring that all tagged fish recovered well and that they can be included in the next steps of the experiment. The tag became active 7 days after the implantation, ensuring data recording with fish that recovered from surgery (Alfonso et al., 2021).
The accelerometer tags were programmed to record, the acceleration over two axes (X and Z), removing the backward/forward acceleration (Y-axis) with a sampling rate of 10 Hz (10 measurements per second). The tag returned 8-bit values that represent the root mean square (RMS) acceleration, here named as “swimming activity.” These values ranged from 0 to 255 arbitrary units (AUs) and can be converted into acceleration (m/s2) using the equation from the manufacturer [acceleration (m/s2) = 0.01955(x), where x is the value returned by the tag], resulting from the contribution of the axes. The swimming activity (AU) has been monitored for 42 days. The tag IDs and coded acceleration values were stored in the memory of the submergible acoustic receivers VEMCO VR2W (AMIRIX Systems Inc., Nova Scotia, Canada) located at the bottom of each tank. At the end of the trial, the data were extracted from the acoustic receiver using the VUE software (AMIRIX Systems Inc.) until further processing.
One week after the risk-taking test (t0), the experiment began by taking the morphometric measurements [total length (mm) and mass (g)] of all the animals involved in the trial under similar anesthesia condition using 30 mg/L of clove oil, as well as a blood sampling (see details in section “Blood sampling and analysis of blood physiological indicators”). The timeline of the experimental protocol is provided in Figure 1.
Inoculation of the vaccine
At the beginning of the experiment (t0), all the 159 fish involved in the trial were inoculated with 0.2 ml of a formalin-killed vaccine against vibriosis caused by V. anguillarum under similar anesthesia conditions described above (Figure 1). The vaccine was produced by Istituto Zooprofilattico Sperimentale delle Venezie (IZSVe) starting with a pure colony of the V. anguillarum reference strain LMG 10861, incubated at 25°C ± 2°C for 48 h in Tryptone Soya Broth (TSB) with 2% of NaCl and then killed by a 4‰ formalin final concentration (Manfrin et al., 2009; EMA/CVMP/IWP/314550/2010 2011; Nguyen et al., 2017; EMA/CMDv/452656/2016 REC-002-01 2017). The sterility of the vaccine was tested by specific growth media (AS, SDA, and RBCA) incubated for 10 days at 25°C and 37°C. After two washes, the final concentration was 107 colony-forming units (CFU)/ml, so each sea bream was injected with 106 CFU/fish.
Blood sampling and analysis of blood physiological indicators
Blood sampling was carried out at the three sampling times t0, t1, and t2 (t0 = before vaccination, t1 = 1 month after vaccination, and t2 = 2 months after vaccination) (Figure 1) following a similar protocol described in Carbonara et al. (2019). Briefly, after ~2–3 min in anesthetic (clove oil, 30 mg/L), blood was sampled from the first branchial arch of the fish using a heparinized syringe in 18 fish (3 bold and 3 shy randomly selected from each tank; i.e., n = 9 for each personality type). Fish were randomly selected at t0, and the selected fish were kept for sampling at t1 and t2 using RFID identification. The levels of the following blood parameters were determined: hemoglobin (Hb), erythrocyte count (RBCC), hematocrit (Hct), glucose, lactate, cortisol, adrenaline, and noradrenaline according to Carbonara et al. (2019). In addition, four parameters of aspecific immunity were used to describe the activation of the non-specific immunological response: protease, serum peroxidase, total proteins, and lysozyme. Protease activity was quantified using the azocasein hydrolysis assay according to the method of Ross et al. (2000). Serum peroxidase activity was measured according to Quade and Roth (1997), while the total protein amount was determined using Folin phenol reagent according to Lowry et al. (1951). Lysozyme concentration was measured using turbidimetric assay modified for the microplate reader (Carbonara et al., 2010).
Antibody screening
In total, the blood of the 18 fish sampled (n = 3 bold and n = 3 shy per tank; i.e., n = 9 for each personality type) was also used for antibody screening (see Figure 1). The blood was stored at −20°C and then tested all together to check the specific immune response to V. anguillarum by IZSVe enzyme-linked immunosorbent assay (ELISA). The protocol was performed according to Scapigliati et al. (2010) and adjusted on sea bream for this trial to research antibodies against V. anguillarum.
Initially, 100 μl of the inactivated V. anguillarum 01 reference strain LMG 10861 at 3 × 107 CFU/ml prepared with 0.1 M of carbonate–bicarbonate buffer pH 9.4 was employed to coat 96-multiwell plates (MaxiSorp™, Thermo Fisher Scientific Rodano, Italy) overnight at 4°C. The wells were then washed three times with PBS solution containing 0.05% Tween. After blocking the remaining sites with 100 μl of bovine serum albumin (BSA 3% in PBS) and incubating for 30 min at 37°C, the plates were washed three times with PBS solution + 0.05% Tween. Then, 100 μl of fish sera diluted 1:100 in 0.01% PBS were added, incubated for 60 min at 22°C, and washed three times with PBS solution + 0.05% Tween. After washing, 100 μl of a secondary MAb anti-sea bream IgM (Aquatic Diagnostic Ltd, Oban, Scotland) was added, which was diluted in PBS 1:100 and 1:250 and incubated for 60 min at 22°C. Afterward, 100 μl of fluorescein-conjugated MAb against mouse IgG (Sigma-Aldrich, Saint Louis, USA) was added, which was diluted 1:2,000 and incubated for 60 min at 22°C. Finally, after PBS washing, 100 μl of chromogenic detector [tetramethylbenzidine (TMB), Thermo Fisher Scientific] and 100 μl of stop solution (sulfuric acid—2N) were added. The ELISA optical densities (O.D. values) were detected by a 450-nm spectrophotometer reader. The Δ of antibody presence during the screening (numerical difference between the two sampling dates) of Δt0 – t1 and Δt1 – t2 was analyzed.
Statistical analysis
Statistical analyses were performed using the R software version 4.0.4 (R Development Core Team, 2021) and carried out at the 95% level of significance. Swimming activity recorded by the tag was analyzed as a function of SCS (bold or shy) and the days of the experiments using a linear regression model. The swimming activity values over the whole experiment duration were compared between bold and shy fish using the generalized linear mixed-effects models (GLMM) with SCS as the fixed effect and tank and fish ID as the random effects using the lme4 package (Bates et al., 2014). Since acceleration is strictly positive and continuous, GLMM with the Gamma distribution family and identity as a link function was used. Visual inspection of the residuals revealed no violation of the statistical assumptions by the model. For all the physiological parameters monitored, a two-factor ANOVA was carried out using SCS and sampling points (t0, t1, and t2) as factors. Concerning vaccine efficiency, for each sampling time variation (t0–t1 or t1–t2), the Wilcoxon test was carried out to compare the Δ between the bold and shy sea breams.
Results
Swimming activity recorded by the accelerometer tag
Swimming activity recorded by the tag increased during the experimental duration (estimate ± SE: 0.15 ± 0.02; t = 7.58; p < 0.001) regardless of the SCS (Figure 2A). On average, shy fish displayed higher swimming activity values than bold fish during the experiment (Figures 2A, B; estimate ± SE: 1.78 ± 0.10; t = 18.23; p < 0.001).
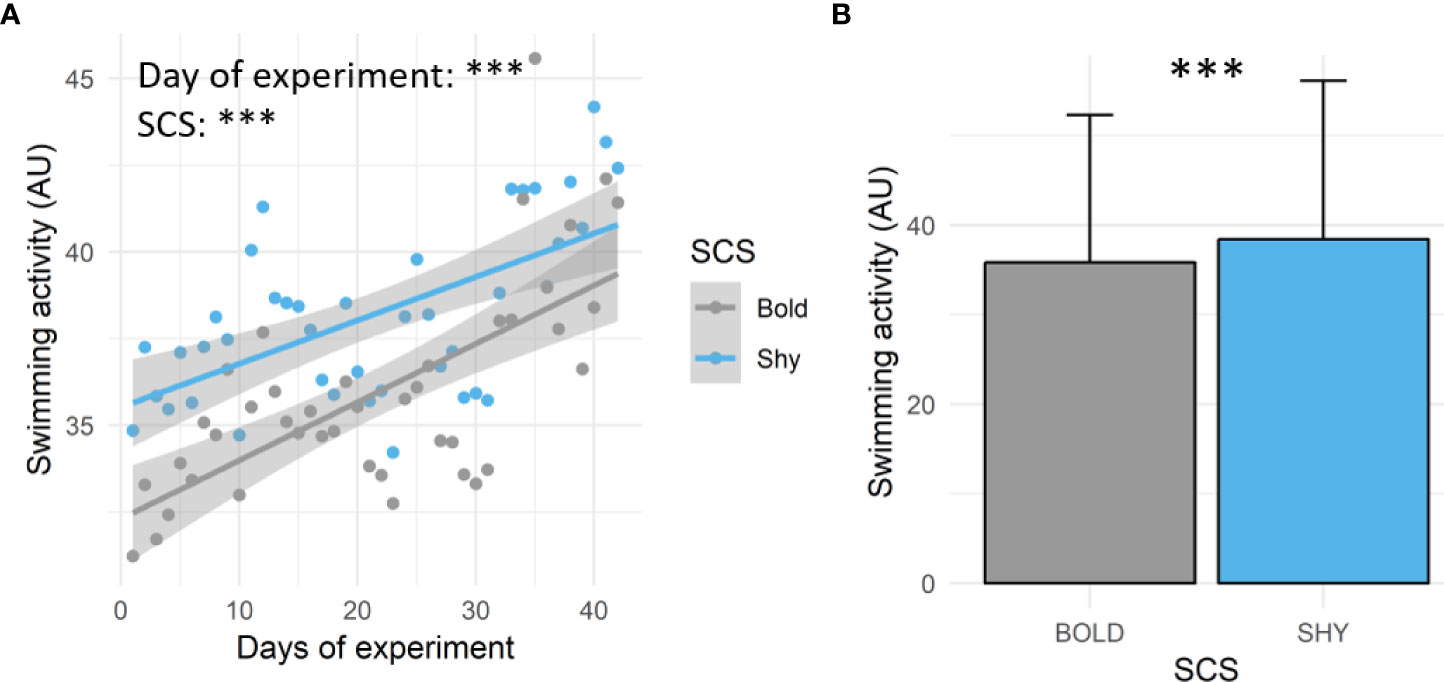
Figure 2 Swimming activity (AU) recorded in bold (gray, n = 9) and shy (blue, n = 9) fish during the experiment. (A) Mean daily values of swimming activity (AU) and linear regressions between swimming activity, days of the experiment, and SCS (bold or shy). (B) Mean ± SD of swimming activity (AU) depending on the SCS (bold or shy). For both panels, significance differences are indicated [the linear model for panel (A) and GLME for panel (B); ***p < 0.001.
Blood physiological parameters
Even if some variations were observed between the different sampling times, the SCS did not influence the concentration of most of the physiological parameters monitored (ANOVA; p > 0.05; Figure 3). Some parameters (hematocrit, lysozyme, and total proteins) were, however, influenced by SCS during the experiment duration (ANOVA: p < 0.05 for all; Figure 3). In more detail, bold fish displayed higher levels of hematocrit, lysozyme, and total proteins, which could be associated with better health status and/or higher aspecific immunity (ANOVA: p = 0.04, p = 0.009, and p < 0.0001 for hematocrit, lysozyme, and total proteins, respectively).
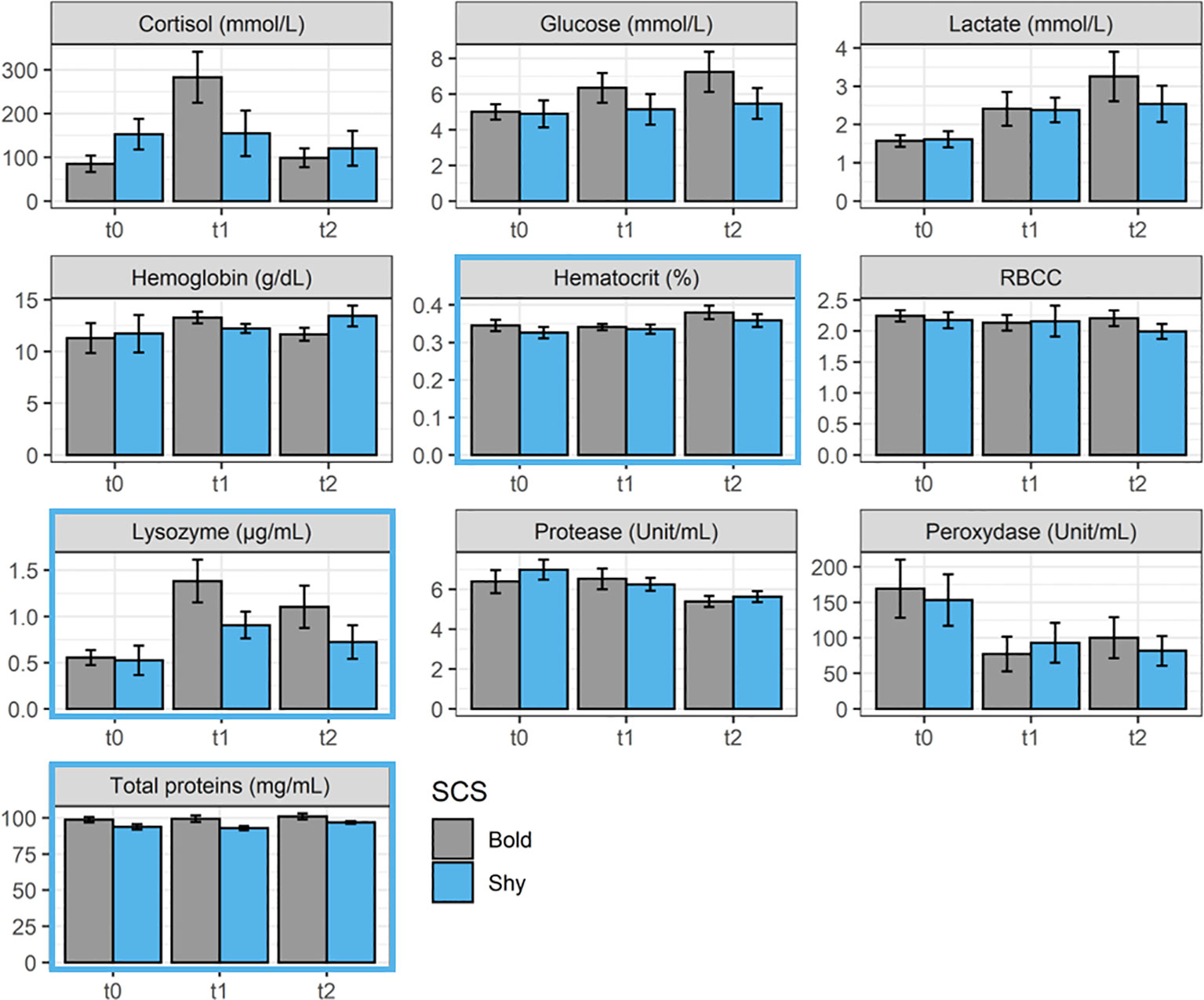
Figure 3 Physiological blood parameter [cortisol, glucose, lactate, hemoglobin, hematocrit, red blood cell count (RBCC), lysozyme, protease, peroxidase, and total proteins] concentrations during the experiment recorded in bold (gray, n = 9 per sampling time) and shy fish (blue, n = 9 per sampling time) at t0, t1, and t2. A blue outline around the figures indicates a significant effect of SCS in the parameter (see statistical details in the text).
Antibody screening
One month after vaccination (t1), 16/18 sea breams (89%) showed increased Δt0−t1 (increased in 9/9 bold fish and only 7/9 shy fish). The Δt0−t1 was increased more than 40% in 7/9 bold fish (representing 78% of fish), whereas it was increased more than 40% only in 2/9 shy fish (~22%). This observation indicated that vaccination worked well regardless of the SCS, and it also showed that a clear difference in antibody concentration in response to vaccination was observed depending on the SCS. Two months after vaccination (t2), the antibody level was stable (i.e., less than 10% of the variation) in the majority of fish (13/17, 76%) regardless of the SCS. The Δt1−t2 increased only in one shy fish and decreased in two bold and one shy fish.
In addition, looking at the average response depending on the SCS, bold fish showed higher Δt0−t1 than the shy ones (Wilcoxon test, p < 0.05), displaying a Δt0–t1 of ~50% vs. 25% for shy fish (Figure 4). However, no difference persists in Δt1−t2 between the divergent SCS (Wilcoxon test, p > 0.05; Figure 4).
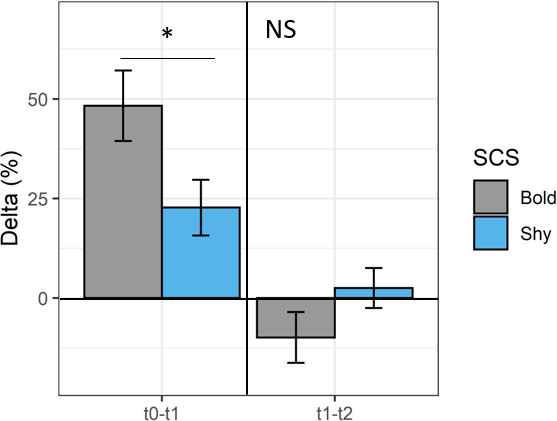
Figure 4 Mean ± SE of delta (Δ, %) between t0−t1 and t1−t2 for bold (gray, n = 9) and shy fish (blue, n = 9). Statistical differences are indicated in the figure (Wilcoxon test; *p < 0.05) or NS (indicating not significant) for each experimental period (t0−t1 or t1−t2).
Discussion
In this study, the physiological response of sea bream from different SCSs following V. anguillarum vaccination was monitored. Following vaccination, the bold fish overall displayed lower swimming activity values recorded by the accelerometer tag, suggesting a lower energy expenditure, but displayed greater levels of lysozyme, hematocrit, and total proteins in the blood. In addition, bold sea breams also responded to vaccination by displaying a greater increase of antibodies 1 month after vaccination, suggesting better immune response than shy sea breams. After 2 months, the level of antibodies reached a physiological plateau, and the difference between the two groups was not yet statistically significant.
Concerning swimming activity recorded by the accelerometer tag, an increase during the experimental duration regardless of SCS following the vaccination procedure was observed. Interestingly, shy sea breams displayed higher swimming activity values than bold ones during the experiment. It is worth noting that bold fish are known to be overall more active than shy fish (Castanheira et al., 2017), but the results of this study are consistent with previous works carried out in sea bream at similar stocking density conditions, where shy fish also displayed higher swimming activity level than the bold ones (Carbonara et al., 2019). Overall, the swimming activity recorded by the tag is recognized as a reliable indicator of oxygen consumption rate and energy expenditure in fishes (Clark et al., 2010; Wilson et al., 2013; Zupa et al., 2015; Zupa et al., 2021; Alfonso et al., 2022), including sea bream (Alfonso et al., 2021). Generally, a higher level of muscle activity implies less availability of energy reserves, reflecting a reduced ability for the fish to compensate for stress events (Korte et al., 2007) and a lower capability to face adverse conditions, such as escape, predation, and competition. Therefore, the results here obtained suggest that, in the experimental condition (moderate stocking density, ~15–20 km/m3), shy fish should cope with competition from bold fish (having a greater amount of energy available) following a vaccination event (Carbonara et al., 2019). By investigating the levels of metabolic-related transcripts, it has been shown that shy sea bream overall display higher metabolic responses than proactive ones following vaccination against V. anguillarum (Vargas et al., 2018). It is worth mentioning that the differences here measured in swimming activity between the two SCSs did reduce progressively during the experimental duration, so they may disappear over time. Interestingly, the better response to vaccination found in bold fish between t0 and t1 also disappeared between t1 and t2. We could hypothesize that bold fish mobilize their energy in immune response following vaccination more than shy fish, which seems consistent when looking at the differences in swimming activity values (shy fish displaying greater values) linked to metabolism. Further work is, however, needed to better understand what factors act to reduce those differences over time (e.g., loss of vaccination-induced effect).
Overall, the SCS did not influence the level of blood physiological parameters for most of the parameters monitored. Some parameters were, however, influenced by SCS during the experiment. Indeed, bold fish displayed higher levels of hematocrit, lysozyme, and total proteins, which could be associated with better health status and/or higher aspecific immunity. Only a few studies investigated the basal level of blood physiological indicators of health and welfare, as well of aspecific immunity depending on fish SCS (Silva et al., 2010; Kittilsen et al., 2012; Ibarra-Zatarain et al., 2016; Alfonso et al., 2020b). Concerning immunity, it is supposed that proactive fish would display greater immunity level than shy ones according to a recent review regarding coping styles in farmed fish species (Castanheira et al., 2017), but it is, however, worth mentioning that our knowledge on the link between SCS and aspecific and specific immunity is limited by very few studies (MacKenzie et al., 2009; Kittilsen et al., 2012; Vargas et al., 2018; Balasch et al., 2019). For instance, Kittilsen et al. (2012) reported higher immunity features of proactive individuals in Atlantic salmon (Salmo salar) when linking lice prevalence, pigmentation, and cortisol level. A recent paper also revealed that bold European sea bass (Dicentrarchus labrax) displayed a greater expression for genes involved in immune system development (e.g., ets1, irf4, klf13) and leukocyte activation (e.g., itgav, notch2, pawr, prkcb) in the head kidney (Sadoul et al., 2022). These examples may support the potential greater immune response in proactive individuals, also highlighted here through the greater level of lysozyme and total proteins measured in the plasma of bold sea bream, even if this was less evident in other studies (Carbonara et al., 2019; Alfonso et al., 2020b). These results are therefore important to better understand the physiology underlying SCS responses. Further studies in this research area are essential since further divergences could be observed between SCSs in terms of growth or reproduction that can ultimately affect the fitness of individuals (Smith and Blumstein, 2008; Castanheira et al., 2017; Geffroy et al., 2020).
In this study, 1 month after vaccination, 89% of sea breams showed an increase of antibody presence in both bold (9/9 fish) and shy fish (7/9 fish). However, the Δt0−t1 was increased more than 40% in 7/9 bold fish (representing 78% of fish), whereas it was increased more than 40% only in 2/9 shy fish (~22%). In addition, the mean Δt0−t1 was ~50% for bold fish, while it was only 25% for shy fish. Altogether, these results indicated that a higher antibody concentration was observed in bold than shy fish in response to vaccination, suggesting a higher efficiency of the vaccine in bold than shy fish. Interestingly, an improved response to V. anguillarum vaccination in bold fish was recently reported in this species (Vargas et al., 2018; Balasch et al., 2019). In more detail, it has been demonstrated that proactive sea bream showed a high immune response and lower metabolism than the shy ones under bath vaccination investigating immune-oxidative stress- and metabolic-related transcripts (e.g., il1β, tnfα, igm, sod, cat, lpl, ghr1, and ghr2) (Vargas et al., 2018). By investigating other endpoints, the results obtained in the present study seem to confirm the few studies available on this topic and highlight the need to further explore immunity features and their functioning mechanisms related to different SCSs. However, in our experiment, 2 months after vaccination (t2), the antibody level was stable between t1 and t2 (i.e., less than 10% of the variation) in the majority of fish (13/17, 76%) regardless of the SCS.
In conclusion, following vaccination against V. anguillarum, shy sea bream displayed higher swimming activity than bold ones, which is overall indicative of greater energy expenditure. Also, following vaccine injection, bold fish displayed higher levels of hematocrit, total proteins, and lysozyme in the plasma, which could be indicative of better health/welfare status and aspecific immunity. Finally, V. anguillarum vaccination appeared to be more efficient in bold than shy ones between t0 and t1, since the number of antibodies was found higher in bold fish. Altogether, these results indicate that proactive sea breams 1) could be more adapted to low stocking density environments (lower swimming activity values and greater levels of lysozyme and total proteins) and 2) seem to be a greater responder to V. anguillarum vaccination. These results are promising but need to be confirmed by further trials including a bigger sample size. Such results could help improve both health/welfare and productivity of farmed sea bream by selecting more robust fish, better adapted to farming conditions.
Data availability statement
The datasets generated during and/or analyzed during the current study are available from the corresponding author on reasonable request. Requests to access the datasets should be directed to SA, c2FsZm9uc29AY29pc3BhLmV1.
Ethics statement
The animal study was reviewed and approved by the Ethics Committee of COISPA Tecnologia & Ricerca (Health Ministry number code 665/2016-PR).
Author contributions
MS, GL, and AM: conceptualization and funding acquisition. PC, WZ, AM, EF, and GL: methodology. PC, SA, WZ, AM, EF, AB, VB, and MC: formal analysis and data curation. AM, MS, and GL: resources. PC and SA: writing—original draft. PC, SA, WZ, AM, EF, MC, MS, and GL: writing—review and editing. AM, MS, GL, and PC: project administration. All authors contributed to the article and approved the submitted version.
Funding
This study was funded by ANIHWA ERA-Net (WIN-FISH) and the Italian Ministry of Health (Department for Public Veterinary Health, Nutrition and Food Safety - ID project - 54 Welfare, Health and Individuality in Farmed FISH). Additional funding that supported this study was provided by Legacoop Agroalimentare (Associazione Nazionale delle Cooperative Agroitticoalimentari per lo Sviluppo Rurale e Costiero).
Acknowledgments
The authors are grateful to Emilie Levavasseur (ZW1pbGllLmxldmF2YXNzZXVyQGxhcG9zdGUubmV0) for drawing the gilthead sea bream in Figure 1.
Conflict of interest
The authors declare that the research was conducted in the absence of any commercial or financial relationships that could be construed as a potential conflict of interest.
Publisher’s note
All claims expressed in this article are solely those of the authors and do not necessarily represent those of their affiliated organizations, or those of the publisher, the editors and the reviewers. Any product that may be evaluated in this article, or claim that may be made by its manufacturer, is not guaranteed or endorsed by the publisher.
References
Øverli Ø., Sørensen C., Pulman K. G. T., Pottinger T. G., Korzan W., Summers C. H., et al. (2007). Evolutionary background for stress-coping styles: Relationships between physiological, behavioral, and cognitive traits in non-mammalian vertebrates. Neurosci. Biobehav. Rev. 31, 396–412. doi: 10.1016/j.neubiorev.2006.10.006
Alfonso S., Zupa W., Manfrin A., Fiocchi E., Dioguardi M., Dara M., et al. (2020a). Surgical implantation of electronic tags does not induce medium − term effect: Insights from growth and stress physiological profile in two marine fish species. Anim. Biotelemetry 8, 21. doi: 10.1186/s40317-020-00208-w
Alfonso S., Zupa W., Manfrin A., Fiocchi E., Spedicato M. T., Lembo G., et al. (2020b). Stress coping styles: Is the basal level of stress physiological indicators linked to behaviour of sea bream? Appl. Anim. Behav. Sci. 231, 105085. doi: 10.1016/j.applanim.2020.105085
Alfonso S., Zupa W., Spedicato M. T., Lembo G., Carbonara P. (2021). Mapping the energetic costs of free-swimming gilthead Sea marine aquaculture. Biol. (Basel). 10, 1357. doi: 10.3390/biology10121357
Alfonso S., Zupa W., Spedicato M. T., Lembo G., Carbonara P. (2022). Using telemetry sensors mapping the energetic costs in European Sea bass (Dicentrarchus labrax), as a tool for welfare remote monitoring in aquaculture. Front. Anim. Sci. 3. doi: 10.3389/fanim.2022.885850
Austin B., Austin D. (2013). Bacterial fish pathogens: Diseases of farmed and wild fish. Fifth Edit (Chichester, U.K: Springer-Praxis Publishing). doi: 10.1007/978-1-4020-6069-4_2
Balasch J. C., Vargas R., Brandts I., Tvarijonaviciute A., Reyes-López F., Tort L., et al. (2019). Divergent personalities influence the myogenic regulatory genes myostatin, myogenin and ghr2 transcript responses to vibrio anguillarum vaccination in fish fingerlings (Sparus aurata). Physiol. Behav. 212, 112697. doi: 10.1016/j.physbeh.2019.112697
Bates D., Mächler M., Bolker B., Walker S. (2014). Fitting linear mixed-effects models using lme4. Journal of Statistical Software 67, 1–48. doi: 10.18637/jss.v067.i01
Carbonara P., Alfonso S., Zupa W., Manfrin A., Fiocchi E., Pretto T., et al. (2019). Behavioral and physiological responses to stocking density in sea bream (Sparus aurata): Do coping styles matter? Physiol. Behav. 212, 112698. doi: 10.1016/j.physbeh.2019.112698
Carbonara P., Corsi I., Focardi S., Lembo G., Rochira S., Scolamacchia M., et al. (2010). The effects of stress induced by cortisol administration on the repeatability of swimming performance tests in the European sea bass (Dicentrarchus labrax l.). Mar. Freshw. Behav. Physiol. 43, 283–296. doi: 10.1080/10236244.2010.504046
Castanheira M. F., Conceição L. E. C., Millot S., Rey S., Bégout M. L., Damsgård B., et al. (2017). Coping styles in farmed fish: Consequences for aquaculture. Rev. Aquac. 9, 23–41. doi: 10.1111/raq.12100
Castanheira M. F., Herrera M., Costas B., Conceição L. E. C., Martins C. I. M. (2013). Can we predict personality in fish? Searching for consistency over time and across contexts. PLoS One 8, 1–9. doi: 10.1371/journal.pone.0062037
Clark T. D., Sandblom E., Hinch S. G., Patterson D. A., Frappell P. B., Farrell A. P. (2010). Simultaneous biologging of heart rate and acceleration, and their relationships with energy expenditure in free-swimming sockeye salmon (Oncorhynchus nerka). J. Comp. Physiol. B Biochem. Syst. Environ. Physiol. 180, 673–684. doi: 10.1007/s00360-009-0442-5
EMA/CVMP/IWP/314550/2010 (2011) Guideline on the design of studies to evaluate the safety and efficacy of fish vaccines. Available at: http://www.ema.europa.eu/docs/en_GB/document_library/Scientific_guideline/2011/11/WC500118226.pdf.
FAO (2020). The state of world fisheries and aquaculture 2020. sustainability in action (Rome). Available at: https://www.fao.org/3/ca9229en/ca9229en.pdf
Ferrari S., Millot S., Leguay D. (2015). Consistency in European seabass coping styles : A life-history approach. Appl. Anim. Behav. Sci. 167, 74–88. doi: 10.1016/j.applanim.2015.03.006
Geffroy B., Alfonso S., Sadoul B., Blumstein D. T. (2020). A world for reactive phenotypes. Front. convervation Sci. 1. doi: 10.3389/fcosc.2020.611919
Ibarra-Zatarain Z., Fatsini E., Rey S., Chereguini O., Martin I., Rasines I., et al. (2016). Characterization of stress coping style in Senegalese sole (Solea senegalensis) juveniles and breeders for aquaculture. R. Soc Open Sci. 3, 160495. doi: 10.1098/rsos.160495
Kittilsen S., Johansen I. B., Braastad B. O., Øverli Ø. (2012). Pigments, parasites and personalitiy: Towards a unifying role for steroid hormones? PLoS One 7, 26–29. doi: 10.1371/journal.pone.0034281
Koolhaas J. M., Korte S. M., De Boer S. F., van der Vegt B. J., Van Reenen C. G., Hopster H., et al. (1999). Coping styles in animals: Current status in behavior and stress- physiology. Neurosci. Biobehav. Rev. 23, 925–935. doi: 10.1016/S0149-7634(99)00026-3
Korte S. M., Olivier B., Koolhaas J. M. (2007). A new animal welfare concept based on allostasis. Physiol. Behav. 92, 422–428. doi: 10.1016/j.physbeh.2006.10.018
Lowry O. H., Rosebrough N. J., Farr A. L., Randall R. J. (1951). Protein measurement with the folin phenol reagent. J. Biol. Chem. 193, 265–275. doi: 10.1016/s0021-9258(19)52451-6
MacKenzie S., Ribas L., Pilarczyk M., Capdevila D. M., Kadri S., Huntingford F. A. (2009). Screening for coping style increases the power of gene expression studies. PLoS One 4, 2–6. doi: 10.1371/journal.pone.0005314
Manfrin A., Bovo G., Selli L., Ceschia G. (2009). The use of vaccines and chemicals in Italy. Options Méditerranéennes Série A. Séminaires Méditerranéens 86, 35–39. Available at: http://om.ciheam.org/om/pdf/a86/00801060.pdf
Nguyen H. T., Thu Nguyen T. T., Tsai M. A., Ya-Zhen E., Wang P. C., Chen S. C. (2017). A formalin-inactivated vaccine provides good protection against vibrio harveyi infection in orange-spotted grouper (Epinephelus coioides). Fish Shellfish Immunol. 65, 118–126. doi: 10.1016/j.fsi.2017.04.008
Quade M. J., Roth J. A. (1997). A rapid, direct assay to measure degranulation of bovine neutrophil primary granules. Vet. Immunol. Immunopathol. 28, (3–4), 239–248. doi: 10.1016/S0165-2427(97)00048-2
R Development Core Team (2021). R software. r a lang. environ. stat. comput (Vienna, Austria: R Found. Stat. Comput). Available at: https://www.r-project.org/.
Réale D., Garant D., Humphries M. M., Bergeron P., Careau V., Montiglio P. O. (2010). Personality and the emergence of the pace-of-life syndrome concept at the population level. Philos. Trans. R. Soc B Biol. Sci. 365, 4051–4063. doi: 10.1098/rstb.2010.0208
Ross N. W., Firth K. J., Wang A., Burka J. F., Johnson S. C. (2000). Changes in hydrolytic enzyme activities of naive Atlantic salmon salmo salar skin mucus due to infection with the salmon louse lepeophtheirus salmonis and cortisol implantation. Dis. Aquat. Organ. 41, 43–51. doi: 10.3354/dao041043
Sadoul B., Alfonso S., Goold C., Pratlong M., Rialle S., Geffroy B., et al. (2022). Transcriptomic profiles of consistent risk-taking behaviour across time and contexts in European sea bass. Proc. R. Soc B. 289, 20220399. doi: 10.1098/rspb.2022.0399
Scapigliati G., Buonocore F., Randelli E., Casani D., Meloni S., Zarletti G., et al. (2010). Cellular and molecular immune responses of the sea bass (Dicentrarchus labrax) experimentally infected with betanodavirus. Fish Shellfish Immunol. 28, 303–311. doi: 10.1016/j.fsi.2009.11.008
Silva P. I. M., Martins C. I. M., Engrola S., Marino G., Øverli Ø., Conceição L. E. C. (2010). Individual differences in cortisol levels and behaviour of Senegalese sole (Solea senegalensis) juveniles: Evidence for coping styles. Appl. Anim. Behav. Sci. 124, 75–81. doi: 10.1016/j.applanim.2010.01.008
Smith B. R., Blumstein D. T. (2008). Fitness consequences of personality: A meta-analysis. Behav. Ecol. 19, 448–455. doi: 10.1093/beheco/arm144
Vargas R., Balasch J. C., Brandts I., Reyes-López F., Tort L., Teles M. (2018). Variations in the immune and metabolic response of proactive and reactive sparus aurata under stimulation with vibrio anguillarum vaccine. Sci. Rep. 8, 17352. doi: 10.1038/s41598-018-35863-w
Wilson S. M., Hinch S. G., Eliason E. J., Farrell A. P., Cooke S. J. (2013). Calibrating acoustic acceleration transmitters for estimating energy use by wild adult pacific salmon. Comp. Biochem. Physiol. - A Mol. Integr. Physiol. 164, 491–498. doi: 10.1016/j.cbpa.2012.12.002
Zrncic S. (2020) Diagnostic manual for the main pathogens in European seabass and gilthead seabream aquaculture. Available at: http://om.ciheam.org/om/pdf/b75/b75.pdf.
Zupa W., Alfonso S., Gai F., Gasco L., Spedicato M. T., Lembo G., et al. (2021). Calibrating accelerometer tags with oxygen consumption rate of rainbow trout (Oncorhynchus mykiss) and their use in aquaculture Facility : A case study. Animals 11, 1496. doi: 10.3390/ani11061496
Keywords: stress coping styles, vaccination, physiology, accelerometer tags, Sparus aurata (L. 1875)
Citation: Carbonara P, Alfonso S, Zupa W, Manfrin A, Fiocchi E, Buratin A, Bertazzo V, Cammarata M, Spedicato MT and Lembo G (2022) Investigating the physiological response and antibody concentration of gilthead sea bream (Sparus aurata) following Vibrio anguillarum vaccination depending on the stress coping style. Front. Anim. Sci. 3:951179. doi: 10.3389/fanim.2022.951179
Received: 23 May 2022; Accepted: 08 August 2022;
Published: 02 September 2022.
Edited by:
Suresh Neethirajan, Wageningen University and Research, NetherlandsReviewed by:
Elisabetta Bonerba, University of Bari Aldo Moro, ItalyCulum Brown, Macquarie University, Australia
Copyright © 2022 Carbonara, Alfonso, Zupa, Manfrin, Fiocchi, Buratin, Bertazzo, Cammarata, Spedicato and Lembo. This is an open-access article distributed under the terms of the Creative Commons Attribution License (CC BY). The use, distribution or reproduction in other forums is permitted, provided the original author(s) and the copyright owner(s) are credited and that the original publication in this journal is cited, in accordance with accepted academic practice. No use, distribution or reproduction is permitted which does not comply with these terms.
*Correspondence: Sebastien Alfonso, c2FsZm9uc29AY29pc3BhLmV1