- 1College of Fisheries, Guangdong Ocean University, Zhanjiang, China
- 2Guangdong Provincial Shrimp Breeding and Culture Laboratory, Guangdong Ocean University, Zhanjiang, China
To identify optimal mating combinations for Litopenaeus vannamei, a linear mixed model was used to estimate the general combining ability (GCA) and specific combining ability (SCA) for growth and multiple stress tolerance [high salt (35‰), low pH (6 ± 0.1), and high ammonia nitrogen (70 mg/L) co-stress] traits in 47 combinations of L. vannamei. The results showed that the SCA in the parents played a dominant role in the offspring traits. The highest GCAs were observed for females of strain O and males of strain B (0.602 and 8.889, respectively), indicating that the dams of strain O and sires of strain B could be used as maternal and paternal lines to increase multiple stress resistance in the next generation. The growth traits of the hybrid combination strain G♂ × strain H♀ exhibited the highest degree of heterosis (9.838%–46.518%) and a generally high SCA (0.643–8.596) among all mating combinations. The SCA was the highest for the strain N♂ × strain O♀ multiple stress tolerance (30.131), while the heterosis for that combination strain was the third-highest. The combinations of strain G♂ × strain H♀ and strain N♂ × strain O♀ can be used as candidate combinations for rapid growth and multiple stress tolerance, respectively.
Introduction
The Pacific white shrimp, Litopenaeus vannamei, is the most commonly farmed shrimp worldwide (Huang et al., 2022). This species was introduced to China in 1988. In 2020, the seawater production of L. vannamei in China reached 119.774 × 104 t, constituting more than 80.50% of the total national production of marine shrimp aquaculture. L. vannamei has thus become the marine shrimp species with the highest level of production in China (Bureau of Fisheries, 2021). Because L. vannamei is not a species native to China, most farmed populations are produced using parents imported from abroad or cultured over multiple generations (Lu et al., 2016). The small effective population sizes after multiple generations of culture may pose a risk of decline in important economic traits because of inbreeding (De Donato et al., 2005; Lu et al., 2017a).
Hybridization is one of the effective methods to solve the above problems (Lu et al., 2016), and it is an important way to create variation. Crossbreeding can produce super-parent vigor (obtain heterosis) that the parents never showed (Maluwa and Gjerde, 2006), thereby significantly improving the viability of the progeny. At present, there are many reports of genetic improvement using selective breeding programs in shrimp (Lu et al., 2016; Sui et al., 2016; Lu et al., 2017b; Zhang et al., 2017b; Yuan et al., 2018). This approach has yielded considerable increases in worldwide shrimp production, from 13% in 1993 to 45% in 2008 (Gjedrem, 2012). After only one generation of selection, the selected strain showed 12% greater growth, compared with the control strain (Argue et al., 2002). Nine improved strains of L. vannamei have been bred in China, including Kehai No. 1, Zhongxing No. 1, and Xinghai No. 1 (Zhang et al., 2017b; Kong et al., 2020). However, the research and application of cross-breeding in prawns are less (Lu et al., 2016; Kong et al., 2020), and there are more reports on cross-breeding of other aquatic animals. Examples: in abalone (Deng et al., 2008; Deng et al., 2010), clam (Dai et al., 2014; Huo et al., 2015), and oyster (Yao et al., 2015; Yan et al., 2017). Thus, heterosis has not been extensively explored in shrimp, resulting in insufficient utilization of heterosis. Combining ability analysis is important in studies of aquatic animal hybrid breeding, which is an important genetic improvement approach; combining ability analysis is also a prerequisite for the utilization of heterosis (Hedgecock and Davis, 2007). There have been many reports regarding combining abilities in aquatic animals (Deng et al., 2010; Bosworth and Waldbieser, 2014; Costa et al., 2019; Chaivichoo et al., 2020). Chaivichoo et al. (2020) reported that the total combining ability was higher in male catfish than in female catfish 0.25 to 362.64 vs. 0.23 to 190.32, suggesting that the growth performance of a hybrid is largely dependent on additive genetic variation from its male parent, followed by variation from its female parent.
Combining ability analysis is one of the important tasks in the research of aquatic animal hybrid breeding, an important means of genetic improvement, and a prerequisite for the utilization of heterosis (Hedgecock and Davis, 2007). However, there have been few reports regarding the combining ability of L. vannamei; published reports have mainly focused on growth and resistance to a single environmental stress factor (Wang et al., 2013; Hu et al., 2016; Wang et al., 2022), Wang et al. (2022) found P♂ × XH♀ combination had obvious heterosis in growth and high salt tolerance traits. Therefore, the application of the combination in breeding and production shall be promoted in the future. However, in real-world aquaculture production, shrimp usually experience stress from multiple environmental factors. For example, intensive high-density shrimp farming has become increasingly common; however, this approach increases ammonia nitrogen content and reduces pH in the aquaculture water environment (Zhou et al., 2009; Yuan et al., 2018). Ammonia nitrogen concentrations as high as 46.11 mg/L have been reported in the late stage of high-density shrimp farming (Chen et al., 1988). In addition, high temperatures in summer lead to increased salinity in aquaculture water (Colombani et al., 2017). The above changes in water quality indicators are likely to simultaneously expose shrimp to multiple stresses of high ammonia nitrogen, low pH, and high salt concentration. In water with a high ammonia nitrogen content, low pH, or high salinity, shrimp survival should be could lead to reduced because of slow growth, decreased immunity, and increased pathogen susceptibility (Pillai and Diwan, 2002; Ye et al., 2009; Cui et al., 2017; Joseph and Philip, 2020; Yu et al., 2020). Compared with a single stressor, exposure to multiple stresses may be more harmful to shrimp. The genetic parameters of shrimp resistance to multiple stresses have not yet been identified. Thus, there is a need to investigate the combining ability for multiple stress tolerance in L. vannamei.
This study was performed to analyze the combining ability and heterosis of growth and multiple stress tolerance traits in L. vannamei. The findings will provide insights regarding crossbreeding and stress resistance breeding of L. vannamei; they will also help to improve growth and stress resistance traits in this species.
Materials and methods
All shrimps used in this study were from the experimental base of the Zhanjiang Guoxing Aquatic Technology Co., Ltd. (Zhanjiang City, Guangdong Province, China). All animal experimental procedures for the current study were approved by Animal Ethical and Welfare Committee of Guangdong Ocean University, China.
Origin of the base population and rearing
In 2019, five populations (four imported and one cultured) were collected and shipped to Zhanjiang Guoxing Aquatic Technology Co., Ltd. The four imported populations were from Thailand (strains W and L) and the USA (strains K and M); the farmed population was L. vannamei Xinghai No. 1, cultivated in our laboratory for six consecutive generations. The core group of new varieties (GX: including six different strains) reached 10 generations by 2021; the above 10 strains had 24 parents, which were used to construct 47 combinations (Table 1).
All populations were kept separately at a stocking density of eight individuals per square meter in a 25-m2 concrete pond. Two months before the spawning season, using body weight as the criterion, the top 30% of the females (100 individuals) and top 15% of the males (50 individuals) were selected as broodstock from each population. The selected shrimp were individually tagged by numbered rings placed on one ocular peduncle and were raised in concrete tanks, with males and females placed in separate ponds for condition. During the acclimation period, male and female broodstock were fed a condition diet of frozen squid, oysters, artemia, and bloodworms to accelerate the gonadal maturation process (Zhang et al., 2017a). Families were produced by an incomplete diallel cross, mature females of each population were selected and transferred into the pond where male broodstock of a single population was placed for mating, a total of 60 families (47 combinations) were obtained. Mated females were place in individual 500-L tanks for spawning. After hatched, approximately 3500 were randomly selected and cultivated in nursery buckets to postlarval stage 15 (PL15); they were then moved to an independent cement pond for culture and were fed a mixed diet of Chaetoceros and Aremia. When the shrimp reached 3cm length, in total, 383 shrimp were randomly selected from each combination for fluorescent labeling (two visible implant elastomer VIE tags were employed either on the left or right-hand side of the first and sixth segments); all labeled shrimp were placed in a pond measuring 13 m × 15 m × 1 m in length, width, and height, respectively, in the common environment for 60 days, all individuals in the pond were reared under standard commercial conditions during the growth-out phase and were feed with commercial prawn pellet containing 40% crude protein. The pond had a water exchange rate of 15–30% of the total water volume per day (Zhang et al., 2017a; Zhang et al., 2017b; Yuan et al., 2018).
Multiple-stress experiment
In 2019, Zhanjiang Guoxing Aquatic Products Technology Co., Ltd. monitored the bottom water quality indicators of the pond used for L. vannamei aquaculture.
The upper limit of salinity was 35‰, and the nadir pH was 6. In a previous study (Yuan et al., 2018), we determined that the median lethal concentration (LC50) of ammonia nitrogen for L. vannamei (at PL15) was 120 mg/L for 48 h. We set the high salinity and low pH conditions to 35‰ and pH 6 ± 0.1, respectively. Reduce the LC50 (120 mg/L) for 48 h under high ammonia nitrogen stress by half (60 mg/L), and then decrease in the direction of 1 mg/L or increase in the direction of 120 mg/L at intervals of 1 mg/L, and can be divided into 120 gradients. A group of experiments is difficult to complete, divided into 40 gradients as a group (total, 3 groups). Group 1: salinity and pH constant at 35‰ and 6 ± 0.1, ammonia nitrogen concentration decreased from 60mg/L by 20 (60, 59, 58, 57, 56, 55, 54, 53, 52, 51, 50, 49, 48, 47, 46, 45, 44, 43, 42, 41mg/L); ammonia nitrogen concentration increased by 20 from 60mg/L (61, 62, 63, 64, 65, 66, 67, 68, 69, 70, 71, 72, 73, 74, 75, 76, 77, 78, 79, 80 mg/L). Groups 2 and 3 pre-experiments will continue to be added or subtracted in the future.
The materials used for high salt, low pH, and high ammonia nitrogen measurements were coarse salt (Salinity Meter Refractometer, HT211ATC), 1 mg/L HCl, 1 mg/L NaOH (Duan et al., 2019) (High-Precision pH Test Pen, ATC), and NH4Cl analytical pure crystals (water quality analyzer). In a preliminary experiment, three replicates (30 shrimp per replicate) per gradient were placed in the adjusted experimental seawater. Because low pH in a water column slowly returns to normal, the experimental seawater pH was adjusted at 2-h intervals and deaths were counted. The dissolved oxygen content was maintained at > 6.0 mg/L. At a salinity of 35‰, pH of 6 ± 0.1, and ammonia nitrogen level of 70 mg/L (because the concentration of multiple-stress was found in group 1 of pre-experiments, we didn’t need to proceed to group 2 and group 3 of pre-experiments), the median lethal time (LT50) was 47.31 h (close to 48 h). Therefore, we used a salt concentration of 35‰, pH of 6 ± 0.1, and ammonia nitrogen concentration of 70 mg/L in the multiple stress experiment.
The multiple stress [simultaneous high salt (35‰), low pH (6 ± 0.1), and high ammonia nitrogen (70 mg/L) stress] experiment was designed based on findings in the preliminary experiment. The seawater concentration was adjusted to the multiple stress concentration. Sixty fishing nets were placed in cement ponds (width, length, and height of 5 m × 8 m × 1 m, respectively); 30 experimental shrimp were randomly selected from each family (Zhang et al., 2017a; Dong, 2018; Liu et al., 2019; Li et al., 2021) and placed into fishing nets (pool water level, 30 cm). The experimental seawater pH was adjusted at 2-h intervals. The number of deaths was counted, the survival time (ST) was recorded, and growth-related traits were measured. An analytical balance (0.01-g accuracy) connected to a computer was used to automatically determine body weight (BW). Shrimp were photographed using a digital camera; total length (TL), body length (BL), carapace length (CL), carapace width (CW), and abdomen length (AL) measurements were performed using ImageJ (NIH). The experiment ended when all shrimp had died.
Statistical analyses
We used the following analytical model for combining growth ability and integrated stress tolerance traits (Bosworth and Waldbieser, 2014; Tran et al., 2021):
Where yijk is the trait value of the k-th hybrid offspring, μ is the population mean, gi is the general combining ability (GCA) of the i-th parent, gj is the GCA of the j-th maternal line, sij is the specific combining ability (SCA) of the i-th sire crossed with the j-th dam, Agek is the age covariate, Sexkis the sex fixed effect, and eijk is the random error effect. Variance component division and combining ability estimation were performed using ASReml4 software (Butler et al., 2017).
We used the following heterosis formula for growth and combined stress tolerance traits (Lu et al., 2016):
Where F1, P1, and P2 represent the first generation of reciprocal offspring of parent 1 and parent 2, the mean value of inbred offspring of parent 1, and the mean value of the representative type of inbred offspring of parent 2, respectively; H (%) is the reciprocal offspring heterosis rate.
In this study, it was difficult to distinguish between males and females at the time of the experiment. Furthermore, the model did not converge after the addition of the fixed effect of sex. Therefore, we removed the sex-fixed effect from the model in our analysis.
Results
GCA of L. vannamei parents
The GCAs for each trait of L. vannamei are shown in Table 2. The GCAs for six growth traits (BW, TL, BL, CL, CW, and AL) and multiple stress tolerance traits in female parents ranged from −2.088 × 10−6 to 3.210 × 10−6 and from −0.531 to 0.602, respectively; those values in male parents ranged from −9.061 × 10−6 to 1.298 × 10−5 and from −8.755 to 8.889, respectively. Thus, the GCAs for the six growth traits in male and female parents were close to 0. The highest GCAs were observed for females of strain O and males of strain B (0.602 and 8.889, respectively), indicating that they were resistant to multiple stressors.
SCAs of the mating combinations of L. vannamei
The SCAs for growth and integrated stress tolerance traits in the offspring of 47 combinations of L. vannamei are shown in Table 3. The highest SCA-ranked combinations for six growth traits (BW, TL, BL, CL, CW, and AL) of 47 combinations were: R♂ × P♀ (3.023), R♂ × P♀ (9.725), R♂ × P♀ (8.685), F♂ × F♀ (1.707), F♂ × F♀ (0.791), and R♂ × P♀ (7.216), respectively. The combinations with the second-highest SCA values were G♂ × H♀ (2.572), G♂ × H♀ (8.596), G♂ × H♀ (7.648), R♂ × P♀ (1.466), R♂ × P♀ (0.716), and G♂ × H♀ (6.344), respectively. The ratios of GCA variance to phenotypic variance for parental growth and multiple stress tolerance traits ranged from 1.006 × 10−6% to 4.536 × 10−5% and from 0.253% to 6.502%, respectively. The ratios of variance of the SCA to the phenotypic variance of the growth and multiple stress tolerance traits of the parents were 10.125% to 11.351% and 6.502%, respectively. In addition, the ratio of GCA variance to the phenotypic variance was less for the female parent (0.253%) than for the male parent (Table 4). And the parental P-value ranged from 2.69×10-63 to 2.19×10-6(Table 4).
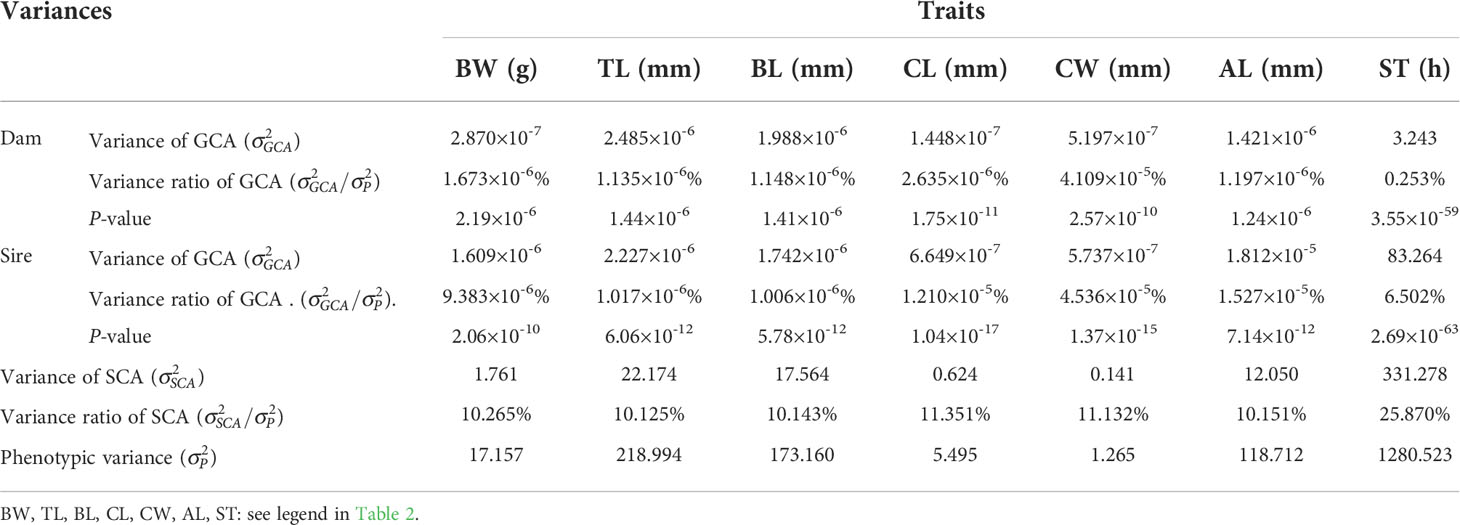
Table 4 Variance components of combining ability for growth and multiple stress tolerance traits in L. vannamei..
Heterosis of growth and integrated stress tolerance traits in L. vannamei hybrid combinations
Figure 1 shows growth and multiple stress tolerance heterosis in L. vannamei. The heterosis ranges of seven traits (BW, TL, BL, CL, CW, AL, and multiple stress tolerance) in 24 hybrid combinations were −18.977% to 46.518%, −7.508% to 15.288%, −7.483% to 15.214%, −4.815% to 9.838%, −5.504% to 10.964%, −8.408% to 17.116%, and −50.42517% to 68.462%, respectively. In terms of BW, TL, BL, CL, CW, and AL, heterosis was highest in the combination G♂ × H♀: 46.518%, 15.288%, 15.214%, 9.838%, 10.964%, and 17.116%, respectively. The three best combinations of heterosis were R♂ × P♀ (68.462%), P♂ × R♀ (43.077%), and N♂ × O♀ (24.500%). In addition, the heterosis of seven traits in 24 hybrid combinations had both positive and negative values, indicating that heterosis could be obtained by crossing and that harmful alleles can be exposed by hybridization.
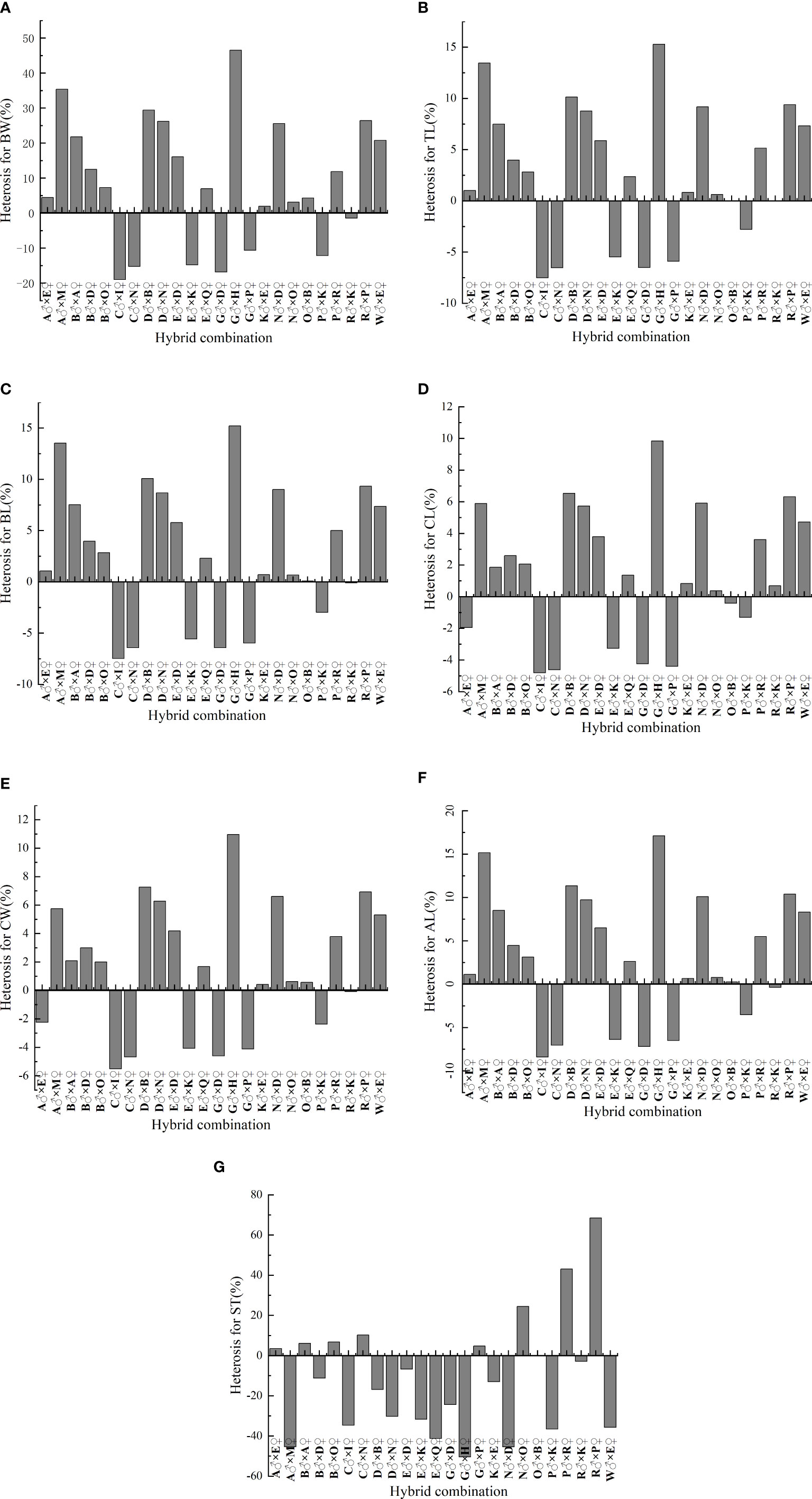
Figure 1 Heterosis in 24 hybrid combinations of L. vannamei.. (A) BW, body weight; (B) TL, total length; (C) BL, body length; (D) CL, carapace length; (E) CW, carapace width; (F) AL, abdomen length; (G) ST, survival time.
Discussions
General combining abilities
Combining ability includes GCA (general combining ability) and SCA(Specific combining ability) (Hayman, 1957; Costa et al., 2019). GCA represents the average performance of parental lines in hybrid combinations, which is mainly improved by pure propagation; it provides information concerning the magnitude of additive genetic effects, which can be used to identify superior parents in breeding programs. High positive GCA values contribute to increased character expression, while negative values tend to have a reducing effect (Hayman, 1957; Eisen et al., 1983). To our knowledge, this is the first attempt to analyze the combining ability for multiple stress tolerance traits in L. vannamei. Our results showed that the GCAs of O strain females and B strain males were highest (0.602 and 8.889, respectively). Thus, females of strain O and males of strain B could be used as the maternal and paternal lines, respectively, to increase multiple stress resistance in the next generation. The GCAs for six growth traits (BW, TL, BL, CL, CW, and AL) in male and female parents ranged from −9.061 × 10−6 to 1.298 × 10−5 and from −2.088 × 10−6 to 3.210 × 10−6, respectively; these values were close to 0. Similar results were previously reported for agricultural papaya (Eisen et al., 1983), maize (Khamphasan et al., 2020), cotton (Hinze et al., 2011), rice, and tilapia (Lin et al., 2016), where the traits had GCAs of 0 or close to 0. The observation of a GCA close to 0 for the growth traits of L. vannamei indicated that there is little potential for continued selection to achieve genetic improvement; crossbreeding should be performed in combination with the results of SCA for strain improvement.
Specific combining ability
SCA refers to the heterosis between two specific populations that exceed the GCA. Nonadditive effects can be measured using the SCA to determine the presence of epistasis and heterosis. Our results showed that the highest SCA-ranked combinations for six growth traits (BW, TL, BL, CL, CW, and AL) of 47 combinations were: R♂ × P♀ (3.023), R♂ × P♀ (9.725), R♂ × P♀ (8.685), F♂ × F♀ (1.707), F♂ × F♀ (0.791), and R♂ × P♀ (7.216), respectively. The combinations with the second-highest SCA values were G♂ × H♀ (2.572), G♂ × H♀ (8.596), G♂ × H♀ (7.648), R♂ × P♀ (1.466), R♂ × P♀ (0.716), and G♂ × H♀ (6.344), respectively. Among the 47 combinations, the highest SCA for multiple stress tolerance was observed for N♂ × O♀ (30.131). These results indicate that R♂ × P♀, G♂ × H♀, and F♂ × F♀ could be used as candidate mating combinations to obtain offspring with high growth rates. The combination N♂ × O♀ was selected as the candidate mating combination for the offspring with multiple stress tolerance. In addition, the ratios of the GCA variance to phenotypic variance for parental growth and multiple stress tolerance traits ranged from 1.006 × 10−6% to 4.536 × 10−5% and from 0.253% to 6.502%, respectively. The ratios of variance of the SCA to the phenotypic variance of the growth and multiple stress tolerance traits of the parents were from 10.125% to 11.351% and 6.502%, respectively. Moreover, the ratio of the GCA variance to the phenotypic variance was less for the female parent (0.253%) than for the male parent. These observations indicate that the parental SCA played a dominant role in progeny trait performance, and the paternal effect greatly affected the multiple stress tolerance. The dominant role of SCA in the growth and multiple stress tolerance indicates that the L. vannamei population has high heterosis, rich genetic diversity, and unstable genetic variation; thus, this population is suitable for cross-breeding. Studies on aquatic animals, such as catfish (Bosworth and Waldbieser, 2014), salmon (Vandeputte et al., 2002), rainbow trout (Henryon et al., 2002), sea bass (Wang et al., 2006), and Atlantic cod (Tosh et al., 2010), have shown that the breeding traits of the progeny can be markedly influenced by the maternal genetic effects. This is in contrast to that noted in the present results. However, a previous study showed that the paternal effect was greater than the maternal effect in terms of breeding traits in the sea cucumber (Liu, 2015), tilapia (Tang et al., 2015), and Sinonovacula constricta (Li, 2018).
Analysis of heterosis for growth and integrated stress tolerance traits
Heterosis is a phenomenon in which the progeny of a parental cross of two different populations is superior to its parents in terms of reproduction, survival, and growth (Burke and Arnold, 2001; Hua et al., 2003; Hochholdinger and Hoecker, 2007). In commercial production environments, heterosis is analyzed to evaluate the feasibilities of various hybridization schemes. In the present study, the heterosis of 7 traits (BW, TL, BL, CL, CW, AL, and ST) in 24 hybrid combinations had positive and negative values ranging from −18.977% to 46.518%, −7.508% to 15.288%, −7.483% to 15.214%, −4.815% to 9.838%, −5.504% to 10.964%, −8.408% to 17.116%, and −50.42517% to 68.462%, respectively. Therefore, hybridization led to both heterosis and exposure to harmful alleles. This was consistent with the results of a previous study (Lu et al., 2016), in which the heterosis (−13.36% to 13.80%) of the body weight of L. vannamei had both positive and negative values. Similar results have been reported in aquatic animals, such as bighead carp growth and survival (−55.9% to 13.8%) (Duong et al., 2022) and abalone growth and survival (−10.8% to 41.4%) (Li et al., 2017). Lu et al. (2016) suggested that a large amount of heterosis may be caused by the accumulation of favorable dominant alleles or dominant alleles that masking recessive deleterious alleles in hybrids. From the perspective that inbreeding exposes harmful genes, heterosis is only a compensation for the decline caused by inbreeding (i.e., a hybrid progeny does not show any advantages but may show disadvantages) (Tian et al., 2007; Yuan et al., 2015). Dunham (2011) argued that crosses between wild and farmed (domesticated) strains could result in positive heterosis, outbreeding suppression (or negative heterosis), or moderate heterosis. In general, crosses can provide information concerning the frequency of heterozygotes among progenies, produce a degree of heterosis, and can help to alleviate inbreeding decline.
In addition, this study identified a hybrid combination of G♂ × H♀, which generally has high SCAs for BW (2.572), TL (8.596), BL (7.648), and AL (6.344). The combination of six growth traits [BW (46.518%), TL (15.288%), BL (15.214%), CL (9.838%), CW (10.964%), and heterosis of AL (17.116%)] for G♂ × H♀ was the highest among all combinations. The SCA for the hybrid combination N♂ × O♀ (30.131) was the highest, while it’s heterosis (24.500%) was the third-highest. Thus, the combination G♂ × H♀ can be used to achieve rapid growth, while N♂ × O♀ can be used to achieve multiple stress tolerance.
Conclusion
In this study, the parental model was used to analyze the GCA and SCA among six L. vannamei germplasm populations; heterosis was analyzed in each population. The results showed that the growth and multiple stress tolerance performance of the hybrid offspring were mainly affected by the parental SCA, which implies that the effect of genetic improvement through cross-breeding will be better. Strain O females and strain B males can serve as the maternal and paternal parental lines for the next generation to achieve multiple stress resistance. The growth traits of the hybrid combination G♂ × H♀ exhibited the highest heterosis and a generally high SCA, compared with other examined mating combinations examined. The N♂ × O♀ combination had the highest SCA for multiple stress tolerance and the third-highest heterosis. Therefore, in the future, G♂×H♀ can be considered the preferred combination to improve the growth rate of the offspring, and N♂×O♀ as the preferred combination to improve the multi-factor stress tolerance of the offspring.
Data availability statement
The raw data used to support the findings of this study are available from the corresponding author upon request: JL, liujy70@126.com.
Ethics statement
This study was carried out in accordance with the requirements of Care and Use of Laboratory Animals in China, Animal Ethical and Welfare Committee of China Experimental Animal Society. The protocol was reviewed and approved by the Animal Ethical and Welfare Committee of Guangdong Ocean University, China.
Author contributions
JL designed the study and provided relative experiment material. LW analyzed data, carried out the experiment and wrote this manuscript. All authors contributed to the article and approved the submitted version.
Funding
This study was financially supported by the National Key R&D Plan “Blue Granary Science and Technology Innovation” key special project in 2020 (2020YFD0900205) and 2019 Guangdong Provincial Science and Technology Special Fund (“Special Project + Task List”) Competitive Distribution Project (2019A04008).
Acknowledgments
The authors thank Hongbiao Zhuo, Shuo Fu, Dongshui Luo, Rongye Yang, Haixin Ou, Jiahao Liang, and Jing Wang for their valuable technical assistance in both the laboratory and field trials.
Conflict of interest
The authors declare that the research was conducted in the absence of any commercial or financial relationships that could be construed as a potential conflict of interest.
Publisher’s note
All claims expressed in this article are solely those of the authors and do not necessarily represent those of their affiliated organizations, or those of the publisher, the editors and the reviewers. Any product that may be evaluated in this article, or claim that may be made by its manufacturer, is not guaranteed or endorsed by the publisher.
References
Argue B. J., Arce S. M., Lotz J. M., Moss S. M. (2002). Selective breeding of pacific white shrimp (litopenaeus vannamei) for growth and resistance to taura syndrome virus. Aquaculture 204, 447–460. doi: 10.1016/s0044-8486(01)00830-4
Bosworth B., Waldbieser G. (2014). Reprint of: General and specific combining ability of male blue catfish (ictalurus furcatus) and female channel catfish (ictalurus punctatus) for growth and carcass yield of their f-1 hybrid progeny. Aquaculture 420, S29–S35. doi: 10.1016/j.aquaculture.2014.01.017
Bureau of Fisheries (2021). “National fisheries technology extension station, China fisheries association,” in China Fishery statistical yearbook (Beijing: China Agriculture Press). M. o. A. a. R. A.
Burke J. M., Arnold M. L. (2001). Genetics and the fitness of hybrids. Annu. Rev. Genet. 35, 31–52. doi: 10.1146/annurev.genet.35.102401.085719
Butler D. G., Cullis B. R., Gilmour A. R., Gogel B. G., Thompson R. (2017). Asreml-r reference manual version 4 (Hemel Hempstead, HP1 1ES, UK: VSN International Ltd).
Chaivichoo P., Koonawootrittriron S., Chatchaiphan S., Srimai W., Na-Nakorn U. (2020). Genetic components of growth traits of the hybrid between male north african catfish (clarias gariepinus burchell 1822) and female bighead catfish (c. macrocephalus gunther 1864). Aquaculture 521, 735082. doi: 10.1016/j.aquaculture.2020.735082
Chen J. C., Liu P. C., Lin Y. T. (1988). Super intensive culture of red-tailed shrimp penaeus penicillatus. J World Aquaculture Soc. 19, 127–131. doi: 10.1111/j.1749-7345.1988.tb00940.x
Colombani N., Giambastiani B. M. S., Mastrocicco M. (2017). Impact of climate variability on the salinization of the coastal wetland-aquifer system of the po delta, italy. J. Water Supply Res. Technology-Aqua 7, 430–441. doi: 10.2166/aqua.2017.115
Costa A. C., Botelho H. A., da Silva Gomes R. C., de Sousa Campos S. A., Reis Neto R. V., Balestre M., et al. (2019). General and specific combining ability in serrasalmidae. Aquaculture Res. 50, 717–724. doi: 10.1111/are.13913
Cui Y. T., Ren X. Y., Li J., Zhai Q. Q., Feng Y. Y., Xu Y., et al. (2017). Effects of ammonia-n stress on metabolic and immune function via the neuroendocrine system in litopenaeus vannamei. Fish Shellfish Immunol. 64, 270–275. doi: 10.1016/j.fsi.2017.03.028
Dai P., Wang H. X., Xiao G. Q., Liu B. Z. (2014). Combining ability and heterosis analysis over two environments in a diallel cross of three families of the clam meretrix meretrix. Acta Oceanologica Sin. 33, 37–42. doi: 10.1007/s13131-014-0539-x
De Donato M., Manrique R., Ramirez R., Mayer L., Howell C. (2005). Mass selection and inbreeding effects on a cultivated strain of penaeus (litopenaeus) vannamei in venezuela. Aquaculture 247, 159–167. doi: 10.1016/j.aquaculture.2005.02.005
Deng Y. W., Liu X., Wu F. C., Zhang G. F. (2008). Experimental evaluation of heterobeltiosis and heterosis between two populations of pacific abalone haliotis discus hannai ino. Acta Oceanologica Sin. 27, 112–119.
Deng Y. W., Liu X., Zhang G. F., Wu F. C. (2010). Heterosis and combining ability a diallel cross of three geographically isolated populations of pacific abalone haliotis discus hannai ino. Chin. J. Oceanology Limnology 28, 1195–1199. doi: 10.1007/s00343-010-9903-7
Dong L. J. (2018). Evaluation of genetic parameters and screening related genes of cold tolerance traits in shrimp (Shanghai: Shanghai Ocean University).
Duan Y. F., Wang Y., Liu Q. S., Zhang J. S., Xiong D. L. (2019). Changes in the intestine barrier function of litopenaeus vannamei in response to ph stress. Fish Shellfish Immunol. 88, 142–149. doi: 10.1016/j.fsi.2019.02.047
Duong T.-Y., Nguyen N.-T. T., Nguyen T. T., Huynh L. T. T., Bui T. M., Pham L. T., et al. (2022). Growth and survival of crossbreeds between wild and domestic strains of mekong delta bighead catfish (clarias macrocephalus gunther 1864) cultured in tanks. Aquaculture 547, 737507. doi: 10.1016/j.aquaculture.2021.737507
Eisen E. J., Horstgen-Schwark G., Saxton A. M., Bandy T. R. (1983). Genetic interpretation and analysis of diallel crosses with animals. TAG. theoretical and applied genetics. Theoretische und angewandte Genetik 65, 17–23. doi: 10.1007/bf00276256
Gjedrem T. (2012). Genetic improvement for the development of efficient global aquaculture: A personal opinion review. Aquaculture 344, 12–22. doi: 10.1016/j.aquaculture.2012.03.003
Hayman B. I. (1957). Interaction, heterosis and diallel crosses. Genetics 42, 336–355. doi: 10.1093/genetics/42.3.336
Hedgecock D., Davis J. P. (2007). Heterosis for yield and crossbreeding of the pacific oyster crassostrea gigas. Aquaculture 272, S17–S29. doi: 10.1016/j.aquaculture.2007.07.226
Henryon M., Jokumsen A., Berg P., Lund I., Pedersen P. B., Olesen N. J., et al. (2002). Genetic variation for growth rate, feed conversion efficiency, and disease resistance exists within a farmed population of rainbow trout. Aquaculture 209, 59–76. doi: 10.1016/s0044-8486(01)00729-3
Hinze L. L., Campbell B. T., Kohel R. J. (2011). Performance and combining ability in cotton (gossypium hirsutum l.) populations with diverse parents. Euphytica 181, 115–125. doi: 10.1007/s10681-011-0442-x
Hochholdinger F., Hoecker N. (2007). Towards the molecular basis of heterosis. Trends Plant Sci. 12, 427–432. doi: 10.1016/j.tplants.2007.08.005
Huang Y., Li Q., Yuan Y., Zhang Z., Jiang B., Yang S., et al. (2022). Silencing of nrf2 in litopenaeus vannamei, decreased the antioxidant capacity, and increased apoptosis and autophagy. Fish Shellfish Immunol. 122, 257–267. doi: 10.1016/j.fsi.2022.02.010
Hua J. P., Xing Y. Z., Wu W. R., Xu C. G., Sun X. L., Yu S. B., et al. (2003). Single-locus heterotic effects and dominance by dominance interactions can adequately explain the genetic basis of heterosis in an elite rice hybrid. Proc. Natl. Acad. Sci. United States America 100, 2574–2579. doi: 10.1073/pnas.0437907100
Hu Z. G., Liu J. Y., Yuan R. P., Zhang J. C. (2016). Combining ability for resistance of litopenaeus vannamei toammonia nitrogen and dissolved oxygen. South China Fisheries Sci. 12, 43–49. doi: 10.3969/j.issn.2095-0780.2016.01.007
Huo Z. M., Yan X. W., Zhao L. Q., Liang J., Yang F., Zhang G. F. (2015). Larval and juvenile growth performance of manila clam hybrids of two full-sib families. J. Ocean Univ. China 14, 564–568. doi: 10.1007/s11802-015-2354-1
Joseph A., Philip R. (2020). Immunocompetence of penaeus monodon under acute salinity stress and pathogenicity of vibrio harveyi with respect to ambient salinity. Fish Shellfish Immunol. 106, 555–562. doi: 10.1016/j.fsi.2020.07.067
Khamphasan P., Lomthaisong K., Harakotr B., Scott M. P., Lertrat K., Suriharn B. (2020). Combining ability and heterosis for agronomic traits, husk and cob pigment concentration of maize. Agriculture-Basel 10, 510. doi: 10.3390/agriculture10110510
Kong J., Luan S., Tan J., Sui J., Luo K., Li X. (2020). Progress of study on penaeid shrim selective breeding. Periodical Ocean Univ. China 50, 81–97. doi: 10.16441/j.cnki.hdxb.20200033
Li M. D., Li J. T., Shi K. P., He Y. Y., Gao B. Q., Liu P., et al. (2021). Estimation of heritability and genetic correlation of saline-alkali tolerance in exopalaemon carinicauda. Prog. Fishery Sci. 42, 117–123. doi: 10.19663/j.issn2095-9869.20200221001
Lin M. X., Yang J., Yue W. C., Kang W., Lei S. Y., Du J. X., et al. (2016). Analysis of combining ability, heterosis and genetic correlation on growth traits of gift tilapia. South China Fisheries Sci. 12, 1–6. doi: 10.3969/j.issn.2095-0780.2016.02.001
Liu S. L. (2015). Selective breeding and performance appraisal in thermotolerant strain of apostichopus japonicus. Qingdao: Ocean University of China.
Liu J. H., Zheng J. J., Liu J. Y. (2019). Genetic parameters for growth-related traits and survival with age in the kuruma shrimp marsupenaeus japonicus. Aquaculture Res. 50, 42–48. doi: 10.1111/are.13862
Li J. Q., Wang M. L., Fang J. G., Liu X., Mao Y. Z., Liu G. M., et al. (2017). Reproductive performance of one-year-old pacific abalone (haliotis discus hannai) and its crossbreeding effect on offspring growth and survival. Aquaculture 473, 110–114. doi: 10.1016/j.aquaculture.2017.01.034
Lu X., Luan S., Cao B. X., Meng X. H., Sui J., Dai P., et al. (2017b). Estimation of genetic parameters and genotype-by-environment interactions related to acute ammonia stress in pacific white shrimp (litopenaeus vannamei) juveniles at two different salinity levels. PloS One 12, e0173835. doi: 10.1371/journal.pone.0173835
Lu X., Luan S., Cao B., Sui J., Dai P., Meng X., et al. (2017a). Heterosis and heritability estimates for the survival of the pacific white shrimp (litopenaeus vannamei) under the commercial scale ponds. Acta Oceanologica Sin. 36, 62–68. doi: 10.1007/s13131-016-0942-6
Lu X., Luan S., Luo K., Meng X. H., Li W. J., Sui J., et al. (2016). Genetic analysis of the pacific white shrimp (litopenaeus vannamei): Heterosis and heritability for harvest body weight. Aquaculture Res. 47, 3365–3375. doi: 10.1111/are.12820
Maluwa A. O., Gjerde B. (2006). Genetic evaluation of four strains of oreochromis shiranus for harvest body weight in a diallel cross. Aquaculture 259, 28–37. doi: 10.1016/j.aquaculture.2006.06.003
Pillai B. R., Diwan A. D. (2002). Effects of acute salinity stress on oxygen consumption and ammonia excretion rates of the marine shrimp metapenaeus monoceros. J. Crustacean Biol. 22, 45–52. doi: 10.1651/0278-0372(2002)022[0045:Eoasso]2.0.Co;2
Sui J., Luan S., Luo K., Meng X. H., Lu X., Cao B. X., et al. (2016). Genetic parameters and response to selection for harvest body weight of pacific white shrimp, litopenaeus vannamei. Aquaculture Res. 47, 2795–2803. doi: 10.1111/are.12729
Tang Z. Y., Chen W. Z., Luo Y. J., Liang J. N., Guo Z. B., Zhong H., et al. (2015). Estimating quantitative trait heritability for tilapia nilotica of different months. Oceanologia Limnologia Sin. 46, 1180–1185.
Tian Y., Kong J., Yang C. H., Zhang T. S., Luo K. (2007). Study on hybridization of two different populations of fenneropenaeus chinensis. Haiyang Xuebao 29, 157–161.
Tosh J. J., Garber A. F., Trippel E. A., Robinson J. A. B. (2010). Genetic maternal and environmental variance components for body weight and length of atlantic cod at 2 points in life. J. Anim. Sci. 88, 3513–3521. doi: 10.2527/jas.2009-2676
Tran Q. V., Tran L. T., Nguyen D. T. K., Ta L. H., Nguyen L. V., Nguyen T. T. (2021). Dataset on the agronomic characteristics and combining ability of new parental lines in the two-line hybrid rice systems in vietnam. Data Brief 36, 107069–107069. doi: 10.1016/j.dib.2021.107069
Vandeputte M., Quillet E., Chevassus B. (2002). Early development and survival in brown trout (salmo trutta fario l.): Indirect effects of selection for growth rate and estimation of genetic parameters. Aquaculture 204, 435–445. doi: 10.1016/s0044-8486(01)00829-8
Wang H., Luo K., Luan S., Kong J., Xu S. Y., Chen B. L. (2013). Combining ability of hybrid generation from the introduced populations of litopenaeus vannamei. J. Fisheries China 37, 489–495. doi: 10.3724/SP.J.1231.2013.38339
Wang X. X., Ross K. E., Saillant E., Gatlin D. M. III, Gold J. R. (2006). Quantitative genetics and heritability of growth-related traits in hybrid striped bass (morone chrysops female x morone saxatilis male). Aquaculture 261, 535–545. doi: 10.1016/j.aquaculture.2006.07.032
Wang C. Y., Wang L., Liu J. Y., Fu X. L. (2022). Analysis of combining ability and heterosis on growth trait and salinity tolerance of different litopenaeus vannamei populations. Oceanologia Limnologia Sin. 53, 161–167.
Yan L. L., Su J. Q., Wang Z. Q., Yan X. W., Yu R. H. (2017). Selection of reference genes for expression analysis of kumamoto and portuguese oysters and their hybrid. J. Ocean Univ. China 16, 1139–1147. doi: 10.1007/s11802-017-3339-z
Yao T., Zhang Y. H., Yan X. W., Wang Z. P., Li D. C., Su J. Q., et al. (2015). Interspecific hybridization between crassostrea angulata and c-ariakensis. J. Ocean Univ. China 14, 710–716. doi: 10.1007/s11802-015-2546-8
Ye L., Jiang S. G., Zhu X. M., Yang Q. B., Wen W. G., Wu K. C. (2009). Effects of salinity on growth and energy budget of juvenile penaeus monodon. Aquaculture 290, 140–144. doi: 10.1016/j.aquaculture.2009.01.028
Yuan R., Hu Z., Liu J., Zhang J. (2018). Genetic parameters for growth-related traits and survival in pacific white shrimp, litopenaeus vannamei under conditions of high ammonia-n concentrations. Turkish J. Fisheries Aquat. Sci. 18, 37–47. doi: 10.4194/1303-2712-v18_1_05
Yuan R. P., Liu J. Y., Zhang J. C., Hu Z. G. (2015). A comparative study of hypoxia and high-ammonia resistance between litopenaeus vannamei inbred and hybrid offspring at different stages. J. Fishery Sci. China 22, 410–417. doi: 10.3724/SP.J.1118.2015.14305
Yu Q. R., Xie J., Huang M. X., Chen C. Z., Qian D. W., Qin J. G., et al. (2020). Growth and health responses to a long-term ph stress in pacific white shrimp litopenaeus vannamei. Aquaculture Rep. 16, 100280. doi: 10.1016/j.aqrep.2020.100280
Zhang J., Cao F., Liu J., Yuan R. (2017a). Genetic parameters for growth and survival traits in litopenaeus vannamei at different ages. Aquaculture Int. 25, 1901–1911. doi: 10.1007/s10499-017-0163-9
Zhang J., Cao F., Liu J., Yuan R., Hu Z. (2017b). Genetic parameters for growth and hypoxic tolerance traits in pacific white shrimp litopenaeus vannamei at different ages. North Am. J. Aquaculture 79, 75–83. doi: 10.1080/15222055.2016.1194923
Keywords: Litopenaeus vannamei, growth traits, multiple stress-tolerance, combining ability, heterosis
Citation: Wang L and Liu J (2022) Analysis of hybrid combining ability for growth and multiple stress tolerance traits in the Pacific white shrimp, Litopenaeus vannamei. Front. Anim. Sci. 3:948251. doi: 10.3389/fanim.2022.948251
Received: 19 May 2022; Accepted: 18 July 2022;
Published: 04 August 2022.
Edited by:
Amira Leila Dib, Université Frères Mentouri Constantine 1, AlgeriaReviewed by:
Prashant Kaushik, Yokohama Ueki, JapanSayed Haidar Abbas Raza, Northwest A&F University, China
Copyright © 2022 Wang and Liu. This is an open-access article distributed under the terms of the Creative Commons Attribution License (CC BY). The use, distribution or reproduction in other forums is permitted, provided the original author(s) and the copyright owner(s) are credited and that the original publication in this journal is cited, in accordance with accepted academic practice. No use, distribution or reproduction is permitted which does not comply with these terms.
*Correspondence: Jianyong Liu, liujy70@126.com