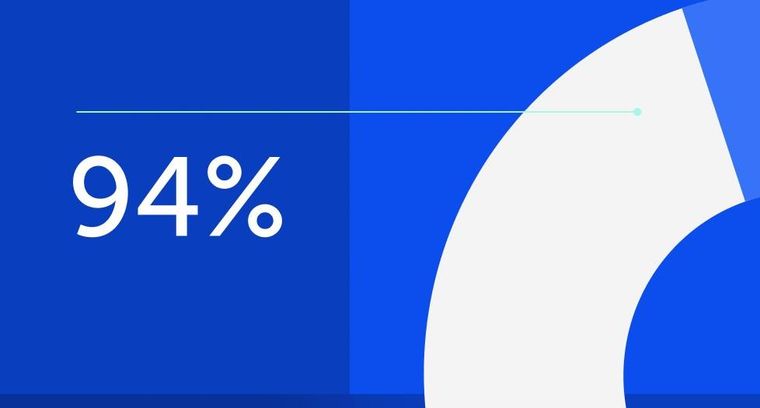
94% of researchers rate our articles as excellent or good
Learn more about the work of our research integrity team to safeguard the quality of each article we publish.
Find out more
ORIGINAL RESEARCH article
Front. Anim. Sci., 07 September 2022
Sec. Animal Nutrition
Volume 3 - 2022 | https://doi.org/10.3389/fanim.2022.919515
We aimed to determine the energy requirements for maintenance and gain of 18 prepubertal dairy heifers of three breed compositions (BC; Holstein, Gyr, and Holstein × Gyr). Diets were formulated for gains of 0, 400, and 800 g/day, corresponding to 1.0×, 1.5×, and 2.0× maintenance, respectively. Each dairy BC had six animals with an initial body weight (iBW) of 219.8 ± 32 kg, 215.8 ± 33 kg, and 228.3 ± 33 kg for Holstein, Gyr, and Holstein × Gyr, respectively. The experiment was designed as a completely randomized design in a factorial scheme 3 × 3 [three BC and three feeding levels (FL)]. Digestibility and metabolism assays were performed to determine energy losses through feces and urine. Heat production was determined using the continuous measurement of oxygen consumption, carbon dioxide production, and methane emissions in respiration chambers. Energy requirements for maintenance (NEm) were calculated based on the relationship between heat production (HP) and metabolizable energy intake (MEI). The efficiency of use of metabolizable energy for maintenance (km) was obtained from the ratio between NEm and metabolizable energy requirements for maintenance. The net energy requirements for growth (NEg) were estimated from the model RE = β0 × EBW0.75 × EBGβ1, where RE is the retained energy (Mcal/day), EBW is empty body weight (kg0.75), and EBG is the empty body gain (kg/day). The efficiency of use of metabolizable energy for gain (kg) was estimated as the slope of the regression between RE and MEI for gain. Gyr heifers presented NEm 15% lower (98 kcal/kg of BW0.75) than HG crossbred animals. Holstein and crossbred heifers had similar NEm, 102 and 112 kcal/kg of BW0.75, respectively. The km was 0.71, 0.74, and 0.75 for HG, Holstein, and Gyr, respectively. Net energy requirement for gain (NEg) did not differ across BC, and a single equation was fit for all BC: RE = 0.069 × BW0.75 × BGW0.852. A single kg of 0.65 was observed for all three BC. Breed composition affected the energy requirements for maintenance and the energy partition, and those differences should be considered when estimating requirements for Gyr, Holstein × Gyr crossbred, and Holstein heifers.
The energy requirements of dairy cows were established from experiments conducted in the 1970s involving comparative slaughters and indirect calorimetry (Moe et al., 1972; NRC, 2001) . The eighth revised version of the Nutrient Requirements of Dairy Cattle (NASEM, 2021) describes nutrient requirement as the daily amount of a certain nutrient necessary to meet a healthy cow’s needs for maintenance, activity, growth, reproduction, and lactation without changing the body reserves or the physiological status. Because of the intensive selection for milk production over the past years, research has suggested that a modern dairy cow has greater metabolic rates than in the past (NASEM 2021). For example, Moraes et al. (2015) reported that the energy requirements for dairy cows had increased over the years, given their increased DMI and milk yield.
To our knowledge, studies measuring requirements for crossbred cattle are scarce, and given the genetic improvements on those animals, newer data are needed. The Zebu cattle (B. taurus indicus) and their crossbreds with European dairy cattle breeds (B. t. taurus) provide the primary basis for the Holstein × Gyr (HG) cattle. This crossbreeding was developed in Brazil back in 1940 and focused on combining the high milk production of Holstein and the adaptability and rusticity of the Gyr breed (Silvaet al., 2017). The Zebu cattle, i.e., Gyr, and HG cattle are the basis of the Brazilian dairy herd since they represent more than 70% of the herd and there is an intense genetic breeding improvement with this breed since the 1980s (Carvalho et al., 2018). Furthermore, based on official data of the Brazilian Trade and Investment Agency, Brazilian bovine semen (mostly Gyr and HG semen) was exported to 21 countries, and the top five markets were Argentina, Angola, Costa Rica, Bolivia, and United Arab Emirates, which represent 2.5 million dollars (ASBIA, 2019).
Therefore, it is clear that Gyr and HG cattle have a high economic value worldwide due to their genetic merit to achieve milk production and adaptability to tropical and sub-tropical climates. Additionally, meeting the dietary needs effectively can reduce the nutrient’s excretion to the environment and greenhouse gas emissions (Knapp et al., 2014). Thus, it is crucial to investigate the nutritional requirements of these breeds to effectively meet production targets because unbalanced diets may affect the proper growth and heifer’s development, resulting in adverse effects on the lactation performance (Hoffman et al., 1996; Daniels et al., 2009; Albino et al., 2015).
A previous study in our laboratory (Silvestre et al., 2021) has shown that the recommendations proposed by NRC for Holstein heifers of 220 kg of BW allowed an average daily gain 50% greater than the formulated, demonstrating an incompatibility of the use of this system for dairy heifers raised in the tropics. However, a new version of INRA (2018) was recently released using a more updated database and more powerful statistical tools, but those new models were never validated in animals raised in tropical countries. Thus, we hypothesized that the NRC (2001) would underestimate the energy requirements of Gyr and HG crossbred heifers and that the requirements of Holstein heifers under tropical environments would differ from those of Gyr and HG crossbred heifers. In addition, we hypothesized that energy requirements estimated using INRA (2018) would be more adequate than those of the NRC (2001). Therefore, the objective of this study was to evaluate the effects of breed composition on the net and metabolizable energy requirements for the maintenance and gain of Holstein, Gyr, and HG prepubertal dairy heifers and compare the estimates with those of NRC (2001) and INRA (2018).
The protocol for the care and manipulation of animals for the accomplishment of this experiment was approved by the Ethics Committee on Animal Use of the University of Sao Paulo Protocol no. 3046. The experiment was performed at the Multi-use Livestock Complex on Livestock Bioefficiency and Sustainability at Embrapa Dairy Cattle, Coronel Pacheco, MG, Brazil and lasted for 90 days.
Eighteen dairy heifers of three breed compositions (BC)—purebred Holstein, purebred Gyr, and Holstein × Gyr crossbred—were used. Each dairy BC had six animals with an initial body weight (iBW) of 219.8 ± 32 kg, 215.8 ± 33 kg, and 228.3 ± 33 kg for Holstein, Gyr, and Holstein × Gyr, respectively. Heifers were housed in a tie-stall facility with individual feed and water bins. All heifers were contemporary and aged 15 ± 3 months. To promote variation in energy intake to estimate energy requirements, we designed the experiment as a completely randomized design in a factorial scheme 3 × 3 [three BC and three feeding levels (FL)]. Diets were formulated using NRC (2001) and fed to supply metabolizable energy intake to allow gains of 0, 400, and 800 g/day, corresponding to 1.0×, 1.5×, and 2.0× maintenance level, respectively. Animals were fed a total mixed ration (TMR) composed of corn silage and concentrate supplement [85:15 w/w based on the dry matter (DM)], and the ingredient and chemical composition are presented in Table 1. The BW of each animal was measured every week and used to adjust the amount of feed offered to each heifer.
The feed was provided once daily in the morning (0800 h). All feeds offered were weighed daily, and samples were dried to determine DM content; dry matter intake was calculated per animal daily.
Heifers were held in a metabolism stall before measurements and nutrient digestibility was measured. The digestibility assay lasted 3 days according to (Rotta et al., 2014), and was used to collect feces and spot urine samples. Two people were on duty in shifts of 24 h during the 72 h. We collected the feces as soon as they were produced, using a scoop shovel. Each heifer had one container, and immediately after defecating, the collected feces were stored inside the container. These procedures prevented feces contamination by urine and the need to keep the heifers in metabolism crates, which would influence animal behavior. At the end of each sampling day, all feces of each animal were weighed. After homogenization, a subsample was taken and frozen for subsequent analysis of gross energy (GE).
Urine samples of 50 mL were collected from all animals over 3 days at four times per day (07:00, 13:00, 19:00, and 01:00 h) by massage on the vulva, filtered in triple-layer gauze, elaborating composite samples of 200 ml per animal. Samples were stored at −20°C for later analysis of GE concentration. Urinary volume was estimated using creatinine concentration as a marker and assuming a daily creatinine excretion of 32.27 mg/day (Chizzotti et al., 2008).
After the digestibility assay, respiration measurements were performed. Live weight was measured daily when the heifers entered and left the respiration chambers. Non-violent training and adaptation to the respirometry chamber were carried out with the heifers, according to Machado et al. (2016).
The open-circuit respiration system used consists of two respiration chambers with a total volume of 21.1 m3. The room temperature and relative humidity were kept at 23 ± 0.5°C and 55 ± 5%, respectively. Each chamber was equipped with its own air treatment unit with a recirculating fan (800 m3/h) and air filters. Each chamber was fitted with an air outlet with a filter box (CSL-851-200HC, Solberg Manufacturing Inc., Itasca, IL, USA), with the air being continuously drawn into the chamber by a sealed rotary pump connected to a mass flow regulator (FlowKit model FK-500, Sable Systems International, Las Vegas, NV, USA). The two chambers shared a common gas analyzer and data acquisition system (Sable Systems International, Las Vegas, NV, USA). Gas samples from the two chambers and the ambient air (baseline) were continuously sampled at 0.5 L/min. A diaphragm sub-sampling pump (SS-4 Sub-Sampler Pump) was used to deliver the subsamples of air to the CH4 analyzer at 200 ml/min. Every 15 min, a subsample was taken over 5 min from ambient baseline air and from each chamber. The samples were delivered to the respiration analyzer (Sable Systems International, Las Vegas, NV, USA), first for analysis of water vapor (RH-300 Water Vapor Analyzer) and then for gas analysis, O2 (FC-10 Oxygen Analyzer), CO2 (CA-10A Carbon Dioxide Analyzer), and CH4 (MA-10, Methane Analyzer) (Sable Systems International, Las Vegas, USA). Calibration of the CO2 and CH4 analyzers (zero and span) is made before the beginning of each measurement.
The O2 and water vapor analyzers are calibrated once a week. For zeroing the CO2, CH4, and O2 analyzers, nitrogen gas (99.999%) is used, and CO2 and CH4 are spanned by using a mixed gas (0.5% CO2 and 0.1% CH4 in N2 as carrier). The O2 analyzer is spanned with dry ambient air, scrubbed from water by using magnesium perchlorate, as it shows an almost constant concentration of 20.95%. A zero value of the water vapor analyzer is achieved with the dry air, and the value for spanning is calculated following the equation: WVP = BP × [(F’iO2 − FiO2)/F’iO2], where WVP is the water vapor pressure in the same units as barometric pressure (kPa), BP is the barometric pressure, and F’iO2 and FiO2 are O2 fractional concentrations of dry and wet ambient air, respectively.
The average recoveries for CO2 and CH4 were (mean ± SD) 99.0 ± 2.1% and 98.0 ± 2.7%, respectively. Recovery in each chamber was estimated using a portable mass flowmeter with a totalizer function (MC-50SLPM-D, Alicat Scientific Inc., Tucson, AZ, USA). Data acquisition and analysis software (ExpeData v.1.7.5, Sable Systems International, Las Vegas, USA) was used to estimate O2, CO2, and CH4 concentrations; flow rate; temperature; barometric pressure; and water vapor pressure during the measurement period. Gas measurements were corrected for differences in water vapor, lag time, and drift, with CH4 emission (L/min) calculated at 15-min intervals for each chamber. Urinary nitrogen was neglected in Brouwer’s equation because of a minimal contribution to HP compared with O2 and CO2 (Verstegen et al., 1987).
Heifers were housed in the chambers for about 22 h when we took gas exchange measurements and heat production (HP) in fed animals. After 22-h, measurements were interrupted, and heifers were removed for 2 h for cleaning the chambers.
The HP was determined from the continuous measurement of oxygen consumption, carbon dioxide production, and CH4 emissions, according to Brouwer (1965) equation. The respiratory quotient was calculated using the ratio of CO2 produced to the O2 consumed. Methane emission was calculated over the 22-h measurement period with correction for the recovery level in each chamber. Digestible energy intake (DEI; Mcal/day) was calculated by subtracting the GE consumed by the energy losses from feces. Metabolizable energy intake (MEI; Mcal/day) was calculated by subtracting the DE intake by the energy losses from urine, CH4, and daily heat production (Blaxter and Clapperton, 1965). The energy loss by CH4 production was calculated considering 9.45 kcal/L CH4 (Brower, 1965). The GE content of the TMR, refusals, and fecal and urine samples were determined in an adiabatic calorimetric pump, IKA WERKE/model C-5000 ADI, Control (AOAC, 2005).
To estimate the energy requirements for maintenance (NEm), a nonlinear exponential model was used to describe the relationship between heat production HP (Mcal/kg of BW0.75) and ME intake (Mcal/kg of BW0.75) according to the following model (Lofgreen and Garret, 1968):
Under this model, β0 represents the NEm (Mcal/kg of BW0.75). By the iterative method, the point where MEI equals HP can be determined as the metabolizable energy requirements for maintenance (MEm, Mcal/kg of BW0.75).
The efficiency of use of MEm (km) was obtained from the ratio between NEm and MEm (NRC, 1996):
Retained energy (RE; Mcal/kg of BW0.75) was calculated as the difference between MEI (Mcal/kg of BW0.75) and HP (Mcal/kg of BW0.75).
To predict the energy requirements for gain (NEg), the following model was used (NASEM (National Academies of Sciences, Enginnering, and Medicine), 2016):
where RE is the retained energy (Mcal/kg of BW0.75), BW0.75 is the body weight, BWG is the body gain (kg/day), and β0 and β1 are regression parameters.
To determine the ME requirements for gain (MEg), it was necessary to estimate the efficiency of use of ME for gain (kg), which was calculated as the slope of the linear regression between RE (Mcal/kg of BW0.75) and MEIg, expressed in Mcal/kg of BW0.75 (Ferrel and Jenkins, 1988):
where RE is retained energy (Mcal/kg of BW0.75) and MEIg is the metabolizable energy intake for gain (Mcal/kg of BW0.75), which was estimated as the difference between total MEI (Mcal/kg of BW0.75) and MEIm (Mcal/kg of BW0.75) calculated as described above, and β0 and β1 are regression parameters. Thus, under this model, β1 represents the kg.
The MEg was then obtained from the relationship between NEg and MEg:
Lastly, the total ME requirements (MEt) were calculated according to the following model: MEt = MEm+MEg, where MEm is the ME requirements for maintenance (Mcal/kg of BW0.75) and MEg is the ME requirements for gain (Mcal/kg of BW0.75).
The models described to estimate energy requirements (RE and HP models) were fit according to linear and nonlinear models using PROC MIXED and PROC NLMIXED, respectively, of SAS University Edition. The data were analyzed as nonlinear models and were adjusted by the Gauss–Newton method. The effect of breed composition was tested in each parameter of all models. Statistical differences were considered at p< 0.10. Data are presented as least squares means.
The current study was designed to estimate the energy requirements of prepubertal heifers using the indirect calorimetry technique and data regarding DMI and animal performance can be found at Silvestre et al. (2021). In addition, the energy partitioning data are presented in Supplementary Table 1. The NEm requirements (Figure 1) were obtained as the intercept of the equation between HP and MEI by Lofgreen and Garrett (1968). Gyr heifers presented NEm 15% lower (p = 0.05; 98 kcal/kg of BW0.75) than HG crossbred animals. As a result, we observed values of 102 kcal/kg of BW0.75 and 112 kcal/kg of BW0.75 of NEm for Holstein and HG crossbred, respectively (p > 0.05).
Figure 1 Prediction equation of the net energy requirements (NEm; Mcal/day) of dairy heifers of three breed compositions (BC) fed at three feeding levels.
As we observed a BC effect on NEm, consequently, we had a BC effect on MEm requirements (β0 – p > 0.043). Using the iterative method, MEm was calculated as 138 kcal/kg of BW0.75, 131 kcal/kg of BW0.75, and 158 kcal/kg of BW0.75 for Holstein, Gyr, and HG crossbred, respectively (Figure 1). As a result, there was a slight BC effect on the efficiency of use of MEm (km), once there were no differences in β1 among BC (p > 0.232). Thus, the results indicated that km was lower in HG crossbred heifers (km = 0.709). Furthermore, the km for HG crossbred cattle was lower than those observed for Holstein and Gyr, which were 0.742 and 0.753, respectively.
To estimate NEg, RE was regressed as a function of metabolic body weight (BW0.75) and ADG (Figure 2), where NEg is measured in Mcal/day; BW and ADG are measured in kg/day. The BC had no effect on both β0 (p = 0.597) or β1 (p = 0.165) of NEg equation. Consequently, a single equation was fit for all BC: RE = 0.069 × BW0.75 × BGW0.852 (MSE = 1.865; R2 = 0.816). As it can be observed, a heavier heifer requires a greater amount of energy within the same ADG.
Figure 2 Representation of retained energy (RE; Mcal/day) equation of dairy heifers of three breed compositions (BC) fed at three feeding levels (FL).
The kg was obtained as a slope of the regression between RE (Mcal/day) and MEIg (Mcal/BW0.75/day). The BC did not affect both β0 (p = 0.861) and β1 (p = 0.869); thus, a single equation was fit (Figure 3): RE = −0.001 + 0.653 × MEIg (MSE = 0.00022; R2 = 0.834). Therefore, the suggested kg value for prepubertal dairy heifers raised under tropical conditions is 0.653 (Figure 3).
Figure 3 Efficiency of use of metabolizable energy for gain (kg) of Holstein, Gyr, and Holstein × Gyr dairy heifers fed at three feeding levels (FL). The blue, green, and red dashed lines represent the regressions for HG, Gyr, and Holstein, respectively. The black dashed line represents the general trend without breed effect.
The ME requirements estimates proposed by the NRC (2001) and INRA (2018) for young cattle as well the total ME requirements (MEt) for dairy heifers raised on tropical conditions are described in Figure 4. Comparing the BC evaluated in this study, we observed that HG crossbred had higher energy requirements (Figures 4A, C, respectively) than those proposed by NRC (2001) and INRA (2018). Interestingly, the requirements of metabolizable energy for maintenance as well the MEt of Holstein heifers were greater than that reported by the NRC (2001).
Figure 4 Total requirements of metabolizable energy of Holstein, Gyr, and F1 Holstein × Gyr dairy heifers estimated using a BW of 260 kg and an ADG of 0.5 kg/day, and by the models proposed by the INRA (2018) and the NRC (2001).
Our hypothesis that the NEm would differ among BC was confirmed as we did observe differences (p< 0.05) on the requirements across the BC evaluated. According to (NRC 2000), B. indicus cattle have 10% lower energy requirements than B. taurus, and this value might be intermediate to crossbred (B. indicus × B. taurus) cattle. Holstein and HG crossbred heifers presented similar (NEm = 102 kcal/kg of BW0.75 and 112 kcal/kg of BW0.75, respectively) requirements in the present study, suggesting that HG crossbred heifers may require the same amount of energy as the pure breeds, i.e., B. taurus cattle. However, our data are greater than that observed by Castro et al. (NRC, 2000; 72 kcal/kg of EBW0.75) for HG crossbred heifers, regardless of the breed we used. Although both studies were conducted during the post-weaning phase, the animals of Castro et al. (2020) were lighter (103 kg) than the ones we used in our study (221 kg). A value of NEm equal to 74.8 kcal/kg of EBW0.75 was reported by Oss et al., (2017); however, this author evaluated growing crossbred bulls. The NRC (2001) dairy cattle model reports a NEm value of 86 kcal of BW0.75, whereas the INRA (2018) estimated it as 101 kcal of BW0.75.
Additionally, the eighth revised edition of (NASEM 2021) supports a higher value for NEm, which is 100 kcal of BW0.75. The present committee recognized that given the intense milk selection over the past 50 years, research has suggested that the modern dairy cow has greater metabolic rates than before (NASEM 2021). This is in line with the current study findings and the latest version of the NASEM (National Academies of Sciences, Enginnering, and Medicine) (2016) also supports a higher value for beef cattle. Recently, Morris and Kononoff (2021) (Rotta et al., 2014) compared the NEm requirements between lactation and dry cows (NEm = 120 vs. 103 kcal/BW0.75, respectively) and reported no differences. In agreement with the current study, the previous authors observed a greater NEm value than those suggested by the NRC (2001), which is similar to our results. This suggests that the NRC (2001) estimates may have been underpredicting dairy cow’s requirements, which seems a limitation of using that system.
Under tropical conditions, Carvalho et al. (2018) reported values of 102 kcal/kg of BW0.75 of NEm for Gyr and Holstein × Gyr lactating cows with no difference between breeds. This value was obtained by a respirometry technique and was similar to that found in the present study. The recommendations by Castro et al. (2020), Oss et al. (2017), and NRC (2001) were estimated using the comparative slaughter technique, while the present study and those of Morris and Kononoff (2021) and Carvalho et al. (2018) were done using respiration chambers. We suspect that the estimation method plays a crucial role in those numbers, and the reason for that difference may be linked to how the support metabolism (HiEv; Willians and Jenkins, 2003) is accounted for in each methodology. The HiEv increases energy demand when the animal changes from a maintenance state to a growing or production state. Whether the HiEv should be accounted for as maintenance or production is still debatable. When the respiration chamber is applied, we usually observe a greater NEm; thus, the HiEv is accounted in the maintenance, and we observe a higher efficiency of use of metabolizable energy for gain (kg), therefore lower requirements of metabolizable energy for gain, whereas when the comparative slaughter is applied, we usually observe a lesser NEm, as well as a lesser kg, and thus, the HiEv is accounted in the requirements for gain. This has been previously discussed by Marcondes et al. (2013), and our relatively high value of kg (0.653, Figure 4) supports this speculation. Furthermore, as the present study applied the respirometry technique and the heifer’s physical activity as time standing and laying down were not recorded, these variables should be considered when interpretating the results of the current study.
Our estimates of MEm requirements for HG crossbred heifers were 15% greater than that found in Holstein and 17% greater than Gyr heifers. Recent studies (Silva et al., 2017; Carvalho et al., 2018) on genetic trends for HG crossbred animals reported an improvement in milk yield of HG when compared to the average of Gyr and Holstein breeds, likely due to the greater heterosis in HG animals. Thus, animals with greater potential for milk production will have an increased maintenance support metabolism, reflecting on the greater MEm observed in our animals (Marcondes et al., 2013). Nevertheless, future studies are still needed to confirm this hypothesis since this study evaluated prepubertal heifers and not lactating cows. However, the current NASEM (2021) suggests that the maintenance requirements may be greater for lactating than nonlactating cows for several reasons, including a greater mass of internal organs and more heat production per unit mass than skeletal muscle.
The estimates of km for prepubertal heifers, determined as the ratio between NEm and Mem, were significantly different among the three evaluated BC. Our calculated km value (0.71) for HG crossbred was 5% lower than the other two BC. The NASEM (2021) reports that the km value has been increased from 0.64 to 0.66. Supporting our findings, Morris and Knonoff (2021) observed a greater km value for dry cows (0.71) compared to lactating (0.69) Jersey cows, suggesting that dry cows might be more efficient utilizing ME for maintenance purposes than for milk production. Evidence indicates that the variation on km between B. indicus and B. taurus can be affected by the plane of nutrition and body composition (Garret, 1980). This is in line with our study, as we did find a BC effect on km. Subepang et al. (2018) reported that km differences between purebred and crossbred cattle might be explained by the level of protein turnover, suggesting that B. indicus cattle are efficient when using MEm due to their lower protein turnover. Solis et al. (1988), comparing energy requirements across five different breeds, found that beef breeds were energetically more efficient in using ME for maintenance than dairy breeds. Contrasting this finding, the Gyr heifers in the current study showed a similar km compared to Holstein heifers.
As the NEg (Mcal/BW0.75) and kg were not affected by BC, a single equation was built for each parameter. The intercept of the equation to estimate NEg was 0.069, which is similar to that observed by Silva et al. (2018) for prepubertal HG crossbred heifers (0.068). Considering heifer’s average BW in this study (221 kg) and an ADG of 0.5 kg/day, the NEg calculated is 2.19 Mcal/day. For the same animal, the NRC (2001) suggests a value of 1.18 Mcal/day, and the INRA (2018) suggests a NEg of 1.89 Mcal/BW0.75/day. Thus, our calculated NEg values are 86% and 16% greater than those reported by NRC (2001) and INRA (2018), respectively. Lastly, although our NEg was 86% higher than that reported by the NRC (2001), we speculate that this is due to the heifers’ body gain composition. Supporting our findings, Marcondes et al. (2021) reported that for animals with the same weight and ADG, HG animals deposit more fat than Holstein animals because of their lower mature BW. The efficiency of use of metabolizable energy for gain (kg = 0.65 on average) found is numerically greater than that reported by the NASEM (2021), which is 0.60 for nonlactating cows. As mentioned above, the BC did not affect the kg; however, it is essential to point out that the kg observed for Holstein heifers was 0.61, very close to those reported by NASEM (2021). Thus, the greater energy efficiency for fat synthesis might indicate that Holstein heifers have a higher efficiency for gain than the other two BC. Silva et al. (2018) suggest a kg of 0.41 for crossbred HG heifers of 251 kg, and comparing this study to ours, this difference may be related to the methodology applied, comparative slaughter vs. respirometry, as described above. Silva et al. (2018) used the comparative slaughter technique to estimate their animals’ requirements, which reflected in a lower kg to account for the support metabolism (Willians and Jenkins, 2003).
Interestingly, when comparing our data with other systems, total ME requirements were greater for HG crossbred (NRC, 2001; INRA, 2018). Looking at Figures 4A,B, we can observe that the difference in MEm requirements for a heifer weighing from 150 to 350 kg of BW with a target ADG varying from 0.2 to 0.8 kg/day was higher from those predicted on both systems, INRA (2018) and NRC (2001). Those differences were mainly associated with the greater requirements for maintenance for HG animals than those suggested by the NRC (2001) and INRA (2018) as already discussed above, thus implying that new research is warranted to confirm these greater maintenance requirements of HG and if that is due to increased support metabolism as suggested.
We hypothesized that the NRC (2001) would underestimate the energy requirements of Gyr and HG crossbred heifers and that the requirements of Holstein heifers under tropical environments would differ from Gyr and HG. Confirming our hypothesis, the net and metabolizable energy requirements for Gyr and crossbred heifers were higher than those described by the NRC (2001). Secondly, the estimates of the INRA (2018) were slightly lower than those observed in this study. Lastly, we observed significant differences in energy requirements among breeds, which should be considered when calculating requirements for prepubertal heifers.
The raw data supporting the conclusions of this article will be made available by the authors, without undue reservation.
The animal study was reviewed and approved by Ethics Committee on Animal Use of the University of Sao Paulo Protocol no. 3046. Written informed consent was obtained from the owners for the participation of their animals in this study.
TS: conducting the experiment, data curation, formal analysis, and writing the original draft. AF, FM, MC, TT, LP, and PR: conceptualization, methodology, and data curation. MM: data curation and validation. All authors contributed to the manuscript and approved the submitted version.
The current experiment was supported by the Minas Gerais State Research Support Foundation (FAPEMIG), the National Council for Scientific and Technological Development (CNPq), Higher Education Personnel Improvement Coordination (CAPES), the National Institute of Science and Technology of Animal Science (INCT-CA), and EMBRAPA.
The authors declare that the research was conducted in the absence of any commercial or financial relationships that could be construed as a potential conflict of interest.
All claims expressed in this article are solely those of the authors and do not necessarily represent those of their affiliated organizations, or those of the publisher, the editors and the reviewers. Any product that may be evaluated in this article, or claim that may be made by its manufacturer, is not guaranteed or endorsed by the publisher.
The Supplementary Material for this article can be found online at: https://www.frontiersin.org/articles/10.3389/fanim.2022.919515/full#supplementary-material
Albino R. L., Marcondes M. I., Akers R. M., Detmann E., Carvalho B. C., Silva T. E. (2015). Mammary gland development of dairy heifers fed diets containing increasing levels of metabolisable protein: metabolisable energy. J. Dairy Res. 82, 113–120. doi: 10.1017/S0022029914000697
AOAC (2005). Official methods of analysis. 18th ed. Ed. Horwitz W. (Gaithersburg, MD: AOAC International).
ASBIA (2019) Index ASBIA mercado. Available at: https://www.lancerural.com.br/vendas-de-semen-bovino-crescem-no-1o-semestre-de-2018/presidente-da-asbia-sergio-saud-anuncia-aumento-nas-vendas-de-semen/.
Blaxter K. L., Clapperton J. L. (1965). Prediction of the amount of methane produced by ruminants. Br. J. Nutr. 19, 511–522.
Brouwer E. (1965). Report of the sub-committee on constants and factors Vol. 3 (Troon, Scotland: EAAP Publisher), Pages 441–443. Proceedings of Energy Metabolism. doi: 10.1017/CBO9780511663161.012
Carvalho P. H. A., Borges ALDCC, Silva R. R. E., Lage H. F., Vivenza P. A. D., Ruas J. R. M., et al. (2018). Energy metabolism and partition of lactating zebu and crossbred zebu cows in different planes of nutrition. PLoS One 13, e0202088. doi: 10.1371/journal.pone.0202088
Castro M. M. D., Albino R. L., Rodrigues J. P. P., Sguizzato A. L. L., Santos M. M. F., Rotta P. P., et al. (2020). Energy and protein requirements of Holstein × gyr crossbred heifers. Animal 14, 1857–1866. doi: 10.1017/S1751731120000622
Chizzotti M. L., de Filho S. C.V., Valadares R. F. D., Chizzotti F. H. M., Tedeschi L. O. (2008). Determination of creatinine excretion and evaluation of spot urine sampling in Holstein cattle. Livest Sci. 113, 218–225. doi: 10.1016/j.livsci.2007.03.013
Daniels K. M., McGilliard M. L., Meyer M. J., Van Amburgh M. E., Capuco A. V., Akers R. M. (2009). Effects of body weight and nutrition on histological mammary development in Holstein heifers. J. Dairy Sci. 92, 499–505. doi: 10.3168/jds.2008-1007
Ferrell C. L., Jenkins T. G. (1998). Body composition and energy utilization by steers of diverse genotypes fed a high-concentrate diet during the finishing period: II. Angus, boran, Brahman, Hereford, and tuli sires. J. Anim Sci. 76, 647. doi: 10.2527/1998.762647x
Garrett W. N. (1980). “Energy utilization by growing cattle as determined in 72 comparative slaughter experiments,” In: Proc 8th Symposium of Energy Metabolism (London, UK: University of Cambridge, Cambridge, UK), pp. 3–7.
Hoffman P. C., Brehm N. M., Price S. G., Prill-Adams A. (1996). Effect of accelerated postpubertal growth and early calving on lactation performance of primiparous Holstein heifers. J. Dairy Sci. 79, 2024–2031. doi: 10.3168/jds.S0022-0302(96)76575-X
Knapp J. R., Laur G. L., Vadas P. A., Weiss W. P., Tricarico J. M. (2014). Invited review: Enteric methane in dairy cattle production: Quantifying the opportunities and impact of reducing emissions. J. Dairy Sci. 97, 3231–3261. doi: 10.3168/jds.2013-7234
Lofgreen G. P., Garrett W. N. (1968). A system for expressing net energy requirements and feed values for growing and finishing beef cattle. J. Anim Sci. 27, 793–806.
Machado F. S., Tomich T. R., Ferreira A. L., Cavalcanti L. F. L., Campos M. M., Paiva C. A. V., et al. (2016). Technical note: A facility for respiration measurements in cattle. J. Dairy Sci. 99, 4899–4906. doi: 10.3168/jds.2015-10298
Marcondes M. I. I., Silva A. L. (2021). Determination of energy and protein requirements of preweaned dairy calves: A multistudy approach. J. Dairy Sci. 104, 11553–11566. doi: 10.3168/jds.2021-20272
Marcondes M. I. I., Tedeschi L. O. O., Valadares Filho S. C. C., Gionbelli M. P. P. (2013). Predicting efficiency of use of metabolizable energy to net energy for gain and maintenance of nellore cattle1. J. Anim Sci. 91, 4887–4898. doi: 10.2527/jas.2011-4051
Moe P. W., Flatt W. P., Tyrrell H. F. (1972). Net energy value of feeds for lactation. J. Dairy Sci. 55, 945–958. doi: 10.3168/jds.S0022-0302(72)85601-7
Moraes L. E., Kebreab E., Strathe A. B., Dijkstra J., France J., Casper D. P., et al. (2015). Multivariate and univariate analysis of energy balance data from lactating dairy cows. J. Dairy Sci. 98, 4012–4029. doi: 10.3168/jds.2014-8995
Morris D. L., Kononoff P. J. (2021). Derivation of the maintenance energy requirements and efficiency of metabolizable energy utilization for dry and lactating Jersey cows. J. Dairy Sci. 104, 9726–9734. doi: 10.3168/jds.2020-20056
NASEM (National Academies of Sciences, Enginnering, and Medicine) (2016). Nutrients requirements of beef cattle. 8th rev. ed (Washington, DC: The National Academic Press).
NASEM (National Academies of Sciences, Enginnering, and Medicine) (2021). Nutrients requirements of dairy cattle. 8th rev. ed (Washington, DC: The National Academic Press).
NRC (1996). Nutrient requirements of beef cattle. updated 7t (Washington, D.C: National Academies Press).
NRC (2001). Nutrient requirements of dairy cattle. 7th ed (Washington, D.C: National Academies Press).
Oss D. B., Machado F. S., Tomich T. R., Pereira L. G. R., Campos M. M., Castro M. M. D., et al. (2017). Energy and protein requirements of crossbred (Holstein × gyr) growing bulls. J. Dairy Sci. 100, 2603–2613. doi: 10.3168/jds.2016-11414
Rotta P. P., Valadares Filho S. C., Detmann E., Costa e Silva L. F., Paulino M. F., Marcondes M. I., et al. (2014). Digesta sampling sites and marker methods for estimation of ruminal outflow in bulls fed different proportions of corn silage or sugar cane. J. Anim Sci. 92, 2996–3006. doi: 10.2527/jas.2013-7364
Silva M. V. G. B., Martins M. F., Cembranelli M. A. R., Paiva L. C., do C. Panetto J. C., Machado M. A., et al. (2017). Programa de melhoramento genético da raça girolando, avaliação genômica de fêmeas jovens (Juiz de Fora - MG: Embrapa Gado de Leite).
Silva F. A. S., Valadares Filho S. C., Rennó L. N., Zanetti D., Costa e Silva L. F., Godoi L. A., et al. (2018). Energy and protein requirements for growth of Holstein × gyr heifers. J. Anim Physiol. Anim Nutr. (Berl) 102, 82–93. doi: 10.1111/jpn.12661
Silvestre T., Lima M. A., dos Santos G. B., Pereira L. G. R., Machado F. S., Tomich T. R., et al. (2021). Effects of feeding level and breed composition on intake, digestibility, and methane emissions of dairy heifers. Animals 11, 586. doi: 10.3390/ani11030586
Solis J. C., Byers F. M., Schelling G. T., Long C. R., Greene L. W. (1988). Maintenance requirements and energetic efficiency of cows of different breed types. J. Anim Sci. 66, 764. doi: 10.2527/jas1988.663764x
Subepang S., Suzuki T., Phonbumrung T., Sommart K. (2018). Enteric methane emissions, energy partitioning, and energetic efficiency of zebu beef cattle fed total mixed ration silage. Asian-Australasian J. Anim Sci. 32, 548–555. doi: 10.5713/ajas.18.0433
Verstegen M. W. A., van der Hel W., Brandsma H. A., Henken A. M., Bransen A. M. (1987). “The wageningen respiration unit for animal production research: A description of the equipment and its possibilities,” in Energy metabolism in farm animals: Effects of housing, stress and disease. Eds. Verstegen M. W. A., Henken A. M. (Dordrecht, the Netherlands: MartinusNijhoff Publishers), Pages 21–48.
Keywords: bioenergetics, breed composition, Girolando, indirect calorimetry, maintenance
Citation: Silvestre T, Ferreira AL, Machado FS, Campos MM, Tomich TR, Pereira LGR, Rodrigues PHM and Marcondes MI (2022) Energy requirements of Holstein, Gyr, and Holstein × Gyr crossbred heifers using the respirometry technique. Front. Anim. Sci. 3:919515. doi: 10.3389/fanim.2022.919515
Received: 13 April 2022; Accepted: 10 August 2022;
Published: 07 September 2022.
Edited by:
Luciano Pinotti, University of Milan, ItalyReviewed by:
Etienne Labussière, INRA Centre Bretagne-Normandie, FranceCopyright © 2022 Silvestre, Ferreira, Machado, Campos, Tomich, Pereira, Rodrigues and Marcondes. This is an open-access article distributed under the terms of the Creative Commons Attribution License (CC BY). The use, distribution or reproduction in other forums is permitted, provided the original author(s) and the copyright owner(s) are credited and that the original publication in this journal is cited, in accordance with accepted academic practice. No use, distribution or reproduction is permitted which does not comply with these terms.
*Correspondence: Marcos Inacio Marcondes, bWFyY29zLm1hcmNvbmRlc0B3c3UuZWR1
Disclaimer: All claims expressed in this article are solely those of the authors and do not necessarily represent those of their affiliated organizations, or those of the publisher, the editors and the reviewers. Any product that may be evaluated in this article or claim that may be made by its manufacturer is not guaranteed or endorsed by the publisher.
Research integrity at Frontiers
Learn more about the work of our research integrity team to safeguard the quality of each article we publish.