- Department of Animal & Food Sciences, Lexington, KY, United States
Since 2018, the growth of industrial hemp (Cannabis sativa) for extraction of cannabidiol (CBD) oil has increased in popularity. By-products resulting from the extraction of the oil have become more available but remain largely unutilized due to their novelty and current restrictions on animal feeding. One potential use for the leftover inflorescence may be as a feedstuff, but reports examining its nutrient quality are limited. Therefore, experiments were conducted to evaluate 9 cultivars of non-extracted and 9 separate cultivars of CBD-extracted foliage. Ground alfalfa cubes were included in experiments as a control. All samples were analyzed for ash, ether extract, crude protein, lignin, ash-free neutral detergent fiber, acid detergent insoluble crude protein, and neutral detergent insoluble crude protein. Total digestible nutrients (TDN) were calculated using summative equations. In vitro true digestibility (IVTD) was determined using a filter bag technique and 48-h digestion period. Cumulative gas production was measured in batch culture over a 48-h period, with methane and ammonia production, volatile fatty acid (VFA) formation, and dry matter disappearance (DMD) measured following this period. Substrates for gas production measurements were composed of the hemp sample, rye silage, and ground corn mixed at a 30:35:35 ratio. Grind size (0.5 vs. 2.0 mm) was evaluated using non-extracted hemp and did not influence IVTD. Nutrient composition ranges of non- and CBD-extracted samples were similar, except for a numerically higher mean ether extract (17.66% vs. 9.29%) and TDN (73.01% vs. 54.03%) in non-extracted hemp. None of the measured nutrients for alfalfa cubes fell within the observed concentration ranges of either hemp group, but TDN was numerically similar to that of CBD-extracted hemp. IVTD was numerically similar between both hemp groups and alfalfa cubes. In comparison to alfalfa cubes, VFA (both concentration and molar proportions), ammonia concentrations, pH, DMD, methane, and total gas production were numerically similar with concurrently run non- and CBD-extracted hemp groups. The results of this study serve as a foundation from which a larger database of nutrient component values for hemp may be compiled. Such databases are necessary to fully evaluate the feasibility of a feedstuff for inclusion in animal diets.
Introduction
Since its relegalization by the Agriculture Act of 2014, Kentucky’s industrial hemp (Cannabis sativa) production has grown to include grain, fiber, and oil production (Williams and Mundell, 2018). Despite its utilitarian reputation, processing of industrial hemp plants for specific end products currently generates significant amounts of waste, particularly regarding extraction of cannabidiol (CBD) oil from inflorescent material. Cannabinoid oil extracted from the inflorescent and leaf portions of the plant is a highly sought after end product for many industrial hemp processors, as this oil has been popularized for its alleged effectiveness as an anti-inflammatory and anticonvulsant agent and as a treatment for some psychiatric disorders (Iffland and Grotenhermen, 2017). After extraction, the resulting biomass is often thrown away, as processors have no further need or application for the by-product. The nutrient composition of extracted biomass reported by Kleinhenz et al. (2020) suggests that this by-product may be a suitable feedstuff for inclusion in cattle rations. However, that report examined only a single cultivar, which may not provide an accurate representation of the nutritive value for all hemp cultivars.
A larger database of nutritional composition that includes multiple cultivars of both non-extracted and CBD-extracted inflorescence is needed if industrial hemp and its associated by-products are to be included in dietary rations. The ability of the California Net Energy System (Lofgreen and Garrett, 1968) to formulate diets for growing animals is dependent upon the accuracy of estimated net energy for maintenance (NEm) and net energy for gain (NEg) feed values (Weiss and Tebbe, 2019). Weiss et al. (1992) presented a set of summative equations to estimate the total digestible nutrients (TDN) of a feedstuff from its obtained chemical composition. Using this calculated value, all other feed energy expressions can be estimated (Tedeschi et al., 2005; Weiss and Tebbe, 2019; Da Cruz et al., 2021). However, due to the novelty of industrial hemp as a potential feedstuff, the true digestibility of both non-extracted and CBD-extracted hemp remains unknown, as does the utility of predicting energy availability from chemical composition using current summative equations.
In addition, today’s society is increasingly focused on creating a healthier environment through several proactive measures, with particular emphasis placed on a reduction in the industrial sector’s carbon footprint. As modern agriculture falls under this category, there has been a call for all areas, particularly animal production, to seek out alternative practices that might decrease greenhouse gas emission rates. Livestock production has specifically been singled out as a large contributor to methane pollution, accounting for approximately 14.5% of anthropogenic global greenhouse gas emissions, with methane representing approximately 30% of these gases (FAO, 2017).
Extraction of CBD oil from the industrial hemp foliage results in large volumes of leftover extracted biomass, which may serve as a suitable protein supplement in cattle rations (Kleinhenz et al., 2020). Other essential oils (EO) are also present in the hemp plant and removed during the CBD-extraction process (Moreno et al., 2020). Previous reports demonstrate that EO are potent modifiers to the ruminal environment, with several studies examining the methane and volatile fatty acid (VFA) formation changes associated with the use of these compounds (Busquet et al., 2006; Castillejos et al., 2007; Cobellis et al., 2016), indicating a potential anti-methanogenic effect of feeding industrial hemp to livestock that may be greater in unextracted material.
Therefore, the overall objective of these experiments was to evaluate non-extracted and CBD-extracted hemp inflorescence from multiple hemp cultivars for digestibility and formation of products of digestion. In addition, this study examined how the nutrient composition of this plant may relate to the prediction of digestibility using established summative equations.
Materials and Methods
All hemp samples were obtained from Kentucky Department of Agriculture-licensed hemp processors. All methods associated with animals were approved by the University of Kentucky Institutional Animal Care and Use Committee (IACUC Protocol 2020-3546).
This study included five experiments. The first was a pilot experiment to determine if grind size affects in vitro true digestibility (IVTD) of non-extracted industrial hemp inflorescence. Methodologies for experiments 2, 3, 4, and 5 were similar, the difference being that experiments 2 and 4 included non-extracted hemp and experiments 3 and 5 utilized CBD-extracted hemp samples. This use of separate experiments was warranted, as, with only one exception, different cultivars were available for the non-extracted and CBD-extracted foliage samples. The experiments are described in further detail below.
Animal Management
All experiments utilized 3 ruminally cannulated Holstein steers [body weight (BW) = ~235 ± 9 kg] as ruminal fluid donors. Approximately 1 kg of ruminal contents was collected 1–3 h post-feeding from each of the 3 steers and prepared using the methods of Xu et al. (2010). Briefly, rumen fluid and contents were transported to the laboratory in an insulated container, blended for 30 s under a CO2 headspace, and filtered through 4 layers of cheesecloth. Cattle were housed together in a 2.4 m × 14.6 m dry lot pen during the collection periods and provided ad libitum access to water and either a rye silage-based (Experiments 1, 4, and 5) or corn silage-based (Experiments 2 and 3) diet (Table 1) with a trace mineral salt supplement and Rumensin® (Elanco Animal Health, Indianapolis, IN, USA). Steers were adapted to each diet for a minimum of 32 days before the first collection date.
Experiment 1: Grind Size Effects on In Vitro True Digestibility
Treatments
Experiment 1 was conducted to determine whether the IVTD of non-extracted industrial hemp was affected by particle size using 9 industrial hemp cultivars. The hypothesis was that the smaller grind size samples would have greater measured true digestibility. Approximately 50 g of herbage from each sample was ground to pass through a 2-mm screen (Model 3 Wiley Mill, Thomas Scientific, Swedesboro, NJ, USA). Each ground sample was divided using a sample splitter (Humboldt Manufacturing Company, Chicago, IL, USA), with one-half of the divided sample ground to pass through a 0.5-mm screen (Model 3 Wiley Mill, Thomas Scientific).
In Vitro True Digestibility Analysis
The determination of IVTD was performed using an ANKOM DaisyII Incubator (ANKOM Technology, Macedon, NY, USA) per the manufacturer’s instructions. Briefly, a single run included two jars. Each jar contained 20 bags, with each sample represented at both grind sizes and two blank bags included for calculating correction factors. Filter bags contained approximately 0.25-g sample, apart from the two empty blank bags. All bags were added to prewarmed digestion jars containing 1,600 ml of the combined buffer mixture of Tilley and Terry (1963). To this mixture, 400 ml of the filtered rumen inoculum was added followed by gassing with CO2 for 30 s. Digestion jars were then placed in the incubator for 48 h. All filter bags were removed from digestion jars at the end of 48 h, rinsed with cold water, and placed in an ANKOM200 Fiber Analyzer (ANKOM Technology). Bags from the same digestion jar were processed together following the manufacturer’s instructions [modified methods of Van Soest et al. (1991)], with the addendum that samples were placed in a glass desiccator rather than a desiccator pouch following removal from the drying oven. Dry matter for inclusion in IVTD calculations was determined using the methods of Holder et al. (2013).
Experiments 2 and 3: Nutrient Composition, Total Digestible Nutrients, and In Vitro True Digestibility
Treatments
Nine cultivars of non-extracted (Experiment 2) and CBD-extracted (Experiment 3) industrial hemp foliage, collected from multiple licensed Kentucky hemp processors, were used to establish a range of nutrient concentrations for hemp inflorescence and examine the potential relationship of IVTD with TDN estimated from chemical composition. Inclusion criteria for cultivars were based on availability, with no duplication of cultivars within the experiment to minimize the risk of biases associated with unequal representation. Approximately 125 g of each sample was ground to pass through a 2-mm screen (Model 3 Wiley Mill, Thomas Scientific). Ground alfalfa cubes were included as a control treatment in both experiments. A subset of all samples was sent to Dairy One (Ithaca, NY, USA) for nutrient composition wet chemistry analysis for concentrations of neutral detergent insoluble crude protein [NDICP; methods of Van Soest et al. (1991) modified for use in an ANKOM 200 Fiber Analyzer, ANKOM Technology; Leco TruMac® N Macro Determinator, Leco Corp., St. Joseph, MI, USA], acid detergent insoluble crude protein (ADICP; AOAC method 973.18 modified for use in an ANKOM200 Fiber Analyzer, ANKOM Technology; Leco TruMac® N Macro Determinator, Leco Corp.), crude protein (CP; AOAC method 990.03 modified for use in a CN628 Carbon/Nitrogen Determinator, Leco Corp.), ash (AOAC method 942.05), ether extract (EE; AOCS Standard Procedure AM 5-04 modified for use in ANKOM XT15 Extractor, ANKOM Technology), and lignin (AOAC method 973.18 modified for use in an ANKOM 200 Fiber Analyzer without sodium sulfite; ANKOM Technology Method 9 for use in ANKOM DaisyII Incubator; ANKOM Technology). Ash-free neutral detergent fiber (NDFOM) concentrations were determined in-house using an ANKOM200 Fiber Analyzer (ANKOM Technology) following the manufacturer’s instructions as described for Experiment 1. Using these obtained concentrations, TDN was calculated using the Weiss summative equation system (Weiss et al., 1992; Weiss, 1993):
where all values are expressed as a percentage of dry matter. Due to the high oil content of both non- and CBD-extracted samples, TDN was also calculated on a non-oil basis (TDNOF) to allow for a better comparison with determined non-oil, ash-free IVTD observations. This was accomplished by amending Equation 1 to:
In Vitro True Digestibility Analysis
The methods for evaluating IVTD were as described in Experiment 1. The measurement of IVTD was replicated for 3 days, with 1 jar per day. Twenty-two bags were placed in each jar: 18 hemp samples (2 per sample), 2 alfalfa cube samples, and 2 blank bags (for calculating correction factors). To determine IVTD values on a non-oil and ash-free basis (IVTDAFOF), original sample weights were corrected for fat and ash content (refer to wet chemistry methods described above). Digested samples, corrected for NDFOM content (refer to wet chemistry methods described above), were divided by the corrected original sample weights.
Experiments 4 and 5: Products of Digestion
Treatments
Inflorescence from nine cultivars of non-extracted (Experiment 4) and nine separate cultivars of CBD-extracted (Experiment 5) industrial hemp was evaluated for measures of total gas production, methane production, VFA and ammonia concentrations, and dry matter disappearance (DMD). Substrates included the hemp sample, rye silage, and ground corn mixed at a 30:35:35 ratio. Ground alfalfa cubes were included as a positive control treatment in both experiments. Non-extracted samples represented different cultivars from CBD-extracted samples, necessitating the use of separate experiments. Treatment substrates were duplicated within each run.
Fermentation Preparation, Sample Collection, and Analyses
Approximately 1 kg of ruminal contents was collected from each of 3 steers on a rye silage diet 1 h after the morning feeding, composited, and prepared as described for experiment 1. Weighed substrates (300 mg, dry matter basis) in fermentation vessels were prewetted with 2 ml of deionized water to aid in dispersal by fermentation media. A 2,700-ml mixture of buffer, macromineral, micromineral, and reducing solutions was prepared as described by Goering and Van Soest (1970) and gassed with CO2 using a dispersing stone for 2 h prior to the start of fermentation. To this mixture, 600 ml of filtered rumen inoculum was added. Combined buffer–inoculum mixture (100 ml) was added to each fermentation vessel, and each vessel was gassed with CO2 for 30 s. ANKOMRF Gas Production modules (ANKOM Gas Production System, Macedon, NY, USA) were tightened onto vessels to seal each system, and vessels were placed in a 39°C water bath for 48 h. Gas samples for methane analysis were collected from each vessel into a 20-ml syringe via a Luer-Lok attached to the vent valve of each module. Needles were immediately attached to the syringes, and samples were dispensed into a red top vacutainer tube and stored at -20°C until analyzed. Samples were analyzed for methane composition on an HP 7890A (Agilent Technologies, Santa Clara, CA, USA) gas chromatograph using an HP-5 capillary column (Agilent Technologies, 30 m × 0.32 mm × 0.25 μm) following the methods of Liu et al. (2018). Briefly, column, inlet, and detector temperatures were 150°C, 200°C, and 250°C, respectively, with helium used as the carrier gas (2.0 ml/min). The split ratio of the inlet chamber was 20:1. Samples (30 µl) were injected and compared with a calibrant gas.
The potential rate and extent of gas production were evaluated using the Blümmel and Becker (1997) one-pool exponential model:
where P is cumulative gas production, b is maximum pressure, k is the rate of pressure (h-1), t is time, and l is the discrete time lag prior to initiation of fermentation (h) using Matlab software (The MathWorks, Inc., Natick, MA, USA). This model assumes that no pressure is produced until the discrete time lag has occurred (Pitt et al., 1999). Total methane (ml) was calculated by multiplying total gas production with the percent methane in each sample determined by gas chromatography with flame-ionization detection (GC-FID).
Immediately following the collection of gas samples for the measurement of methane, all fermentation vessels were placed in an ice bath for rapid cessation of fermentation. The methods of Kim et al. (2014) were used to measure pH and evaluate ammonia and VFA formation in each vessel. Briefly, for VFA measurement, 1 ml of fermentation fluid was combined with 0.1 ml of 50% meta-phosphoric acid and 0.1 ml of 85 mM 2-ethyl butyrate (as an internal standard). For ammonia analysis, 1 ml of fermentation fluid was mixed with 0.2 ml of 25% meta-phosphoric acid. Ammonia and VFA samples were frozen at -20°C to promote protein precipitation. All samples were thawed and centrifuged at 13,000 × g for 15 min. Supernatant from VFA samples was transferred to 12 mm × 32 mm injection vials, and 0.1 µl was analyzed using GC-FID. Column, inlet, and detector temperatures were set to 200°C, 260°C, and 260°C, respectively, with helium (as a carrier gas) flow set to 45 ml/min. Hydrogen and compressed air flows were set to 40 and 450 ml/min, respectively, and split ratio was 2:1. The supernatant from ammonia samples was diluted 1:40 with deionized water and analyzed on a Konelab 20XTi clinical analyzer (Thermo Fisher Scientific Inc., Beverly, MA, USA) using an adapted glutamate dehydrogenase procedure (Kun and Kearney, 1974). Dry matter disappearance (DMD) was determined using the methods of Trotta et al. (2018).
Statistics
Experiment 1
Proc Mixed of SAS (9.4, Cary, NC, USA) was used to evaluate the effects of grind size on IVTD of industrial hemp. Fixed effects included grind size and cultivar, and jar was included as a blocking factor. Kenward-Roger 2 was specified as the denominator degrees of freedom method. Statistical significance was set at p ≤ 0.05.
Experiments 2 and 3
Due to the non-extracted and CBD-extracted samples being unrelated to one another, Experiments 2 and 3 were analyzed separately. However, the statistical methodology was the same for both experiments. The MEANS procedure of SAS (9.4) was used to determine the mean, standard deviation, minimum value, and maximum value of the hemp samples for IVTD, IVTDAFOF, TDN, TDNOF, NDFOM, NDICP, ADICP, CP, ash, EE, and lignin concentrations. Cohen’s d (Cohen, 1988) was used as a measure of effect sizes for comparisons among groups, where the pooled SD was calculated as the root mean square of the separate SD (assumes homogeneity of variance between the groups). In addition, the relationship between TDNOF and IVTDAFOF was investigated using either PROC REG or Proc Reg of SAS (9.4).
Experiments 4 and 5
As mentioned for Experiments 2 and 3, non-extracted and CBD-extracted samples were unrelated to one another and therefore analyzed separately by experiment. All data were analyzed using PROC MEANS of SAS (9.4) to obtain the mean, standard deviation, minimum value, and maximum value of total gas, total methane, percent methane, ammonia, and VFA production as well as DMD, and Cohen’s d (Cohen, 1988) was used for determination of effect sizes.
Results and Discussion
Experiment 1
A study conducted by Damiran et al. (2008) found that the digestibility of a forage may be impacted by grind size. However, as information regarding the digestibility of the inflorescent portion of industrial hemp is limited and remains largely unknown, Experiment 1 was designed to test if the grind size “rule” used with IVTD analyses for common forages also applied to samples of industrial hemp. Following the 48-h digestion period, there were no differences in true digestibility between treatments (Table 2). This agrees with observations by Vastolo et al. (2021), who compared chopped non-extracted hemp separated by size (>4 vs. <4 mm) and reported no difference in in vitro organic matter digestibility. This result indicates that particulate size differences arising from inevitable variations in extraction techniques among industrial hemp processing facilities should have minimal influence on in vitro digestibility estimates.
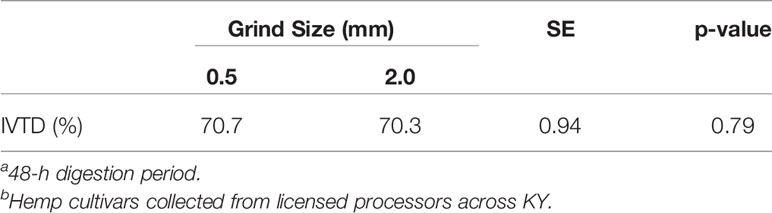
Table 2 Effect of grind size on in vitro true digestibilitya (IVTD) of inflorescence from nine non-extracted industrial hemp cultivarsb.
Experiments 2 and 3
Because different cultivars were available for non-extracted and CBD-extracted samples, direct comparisons between pre- and post-extraction samples could not be made. However, generalized comments regarding differences in IVTD, TDN, and nutrient composition between non-extracted and CBD-extracted foliage are warranted, particularly since the same alfalfa cube and rumen inoculum sources were utilized for both sample types, and results are presented as averages of 9 different cultivars within extraction type (as opposed to individual cultivar means). Thus, these comparisons serve as a suitable starting point for discussing potential differences in nutrient content and digestibility between extracted and non-extracted material.
Non-extracted and CBD-extracted hemp foliage (and alfalfa cubes control) nutrient concentrations and true digestibility descriptive statistics are shown in Tables 3, 4, respectively. Fiber digestibility was not evaluated due to the small sample size per bag (250 mg/bag) and small fiber content of both non- and CBD-extracted foliage (approximately 32%; Tables 3, 4, respectively). The concentrations of most components were similar between non-extracted and CBD-extracted inflorescence (Cohen’s d ≤ 1.5). As expected, CBD-extracted samples had lower concentrations of EE (17.66% vs. 9.29%, Cohen’s d = 2.1) and a higher percentage of ash (Cohen’s d = 2.0) relative to those of non-extracted inflorescence. On average, an 8.37 percentage unit decrease in EE in extracted samples was coupled with an average 8.27 percentage unit increase in ash. This suggests that in addition to the targeted extraction of EE, the extraction process also results in some loss of OM (roughly proportional across the various OM components measured here), with resultant concentration of the ash component. The range of constituent concentrations within extraction types was substantial with coefficients of variation (SD/mean) ranging from 10% to 41%. Given this variation, the range of values for each nutrient was generally consistent with those reported by others (Kleinhenz et al., 2020; Vastolo et al., 2021). The few exceptions to this included a lower NDF for non-extracted samples and a higher EE content for CBD-extracted biomass than were reported by Kleinhenz et al. (2020), and a lower ash content in non-extracted cultivars than the cultivar used by Vastolo et al. (2021). It is important to note that the current study only included 9 cultivars of each extraction type and is not to be construed as a comprehensive assessment of typical ranges for nutrient concentrations. Rather, this study represents a starting point from which a larger database of values may be constructed using future experimental data, which is underscored by the large range among cultivars in constituent concentrations and the discrepancies between the current study and others available in the literature.
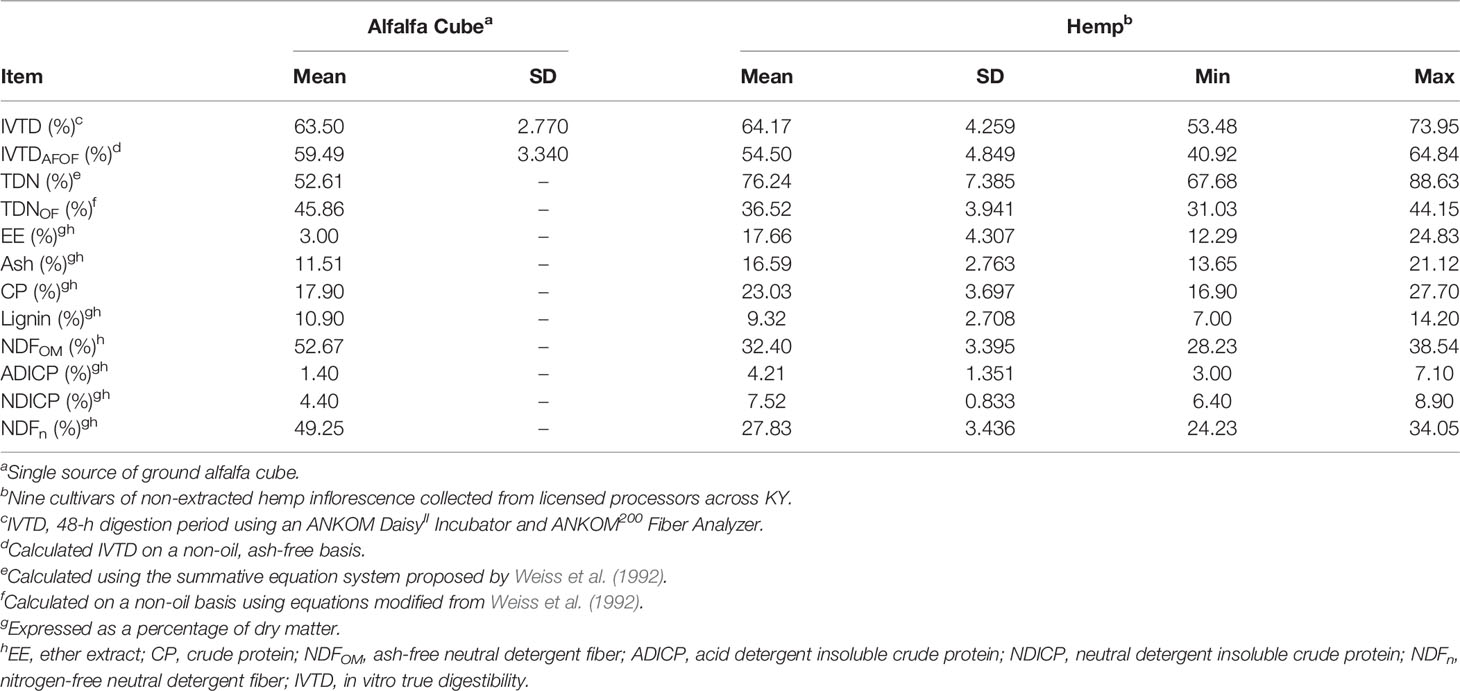
Table 3 Descriptive statistics for digestibility and nutrient concentrations of ground alfalfa cube and inflorescence from 9 non-extracted hemp cultivars.
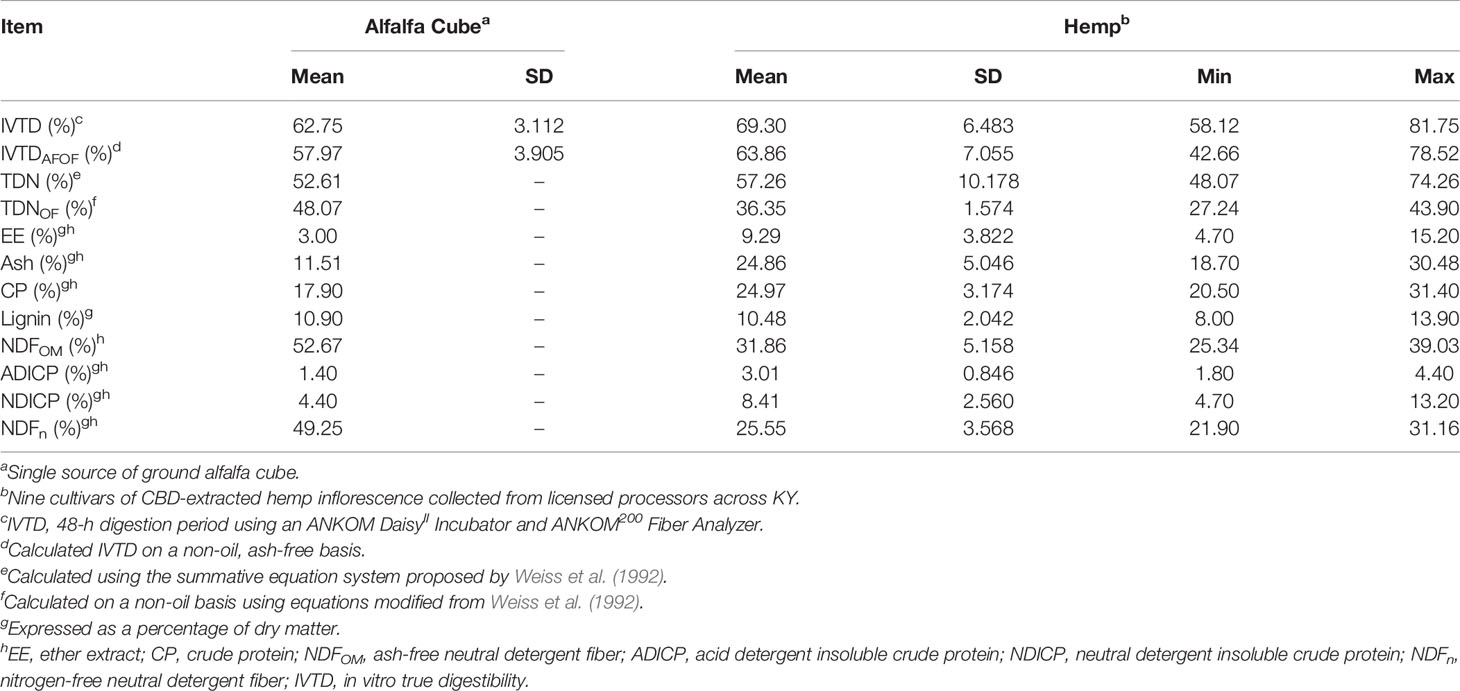
Table 4 Descriptive statistics for digestibility and nutrient concentrations of inflorescence from 9 CBD-extracted hemp cultivars.
There was a slight deviation between the experimental alfalfa cube nutrient concentrations and the reference ranges reported in the latest edition of the beef NRC (National Academies of Sciences, Engineering, And Medicine, 2016). These differences were largely relegated to the fiber fraction, with an approximately 1% and 2% elevated NDF and lignin fractions, respectively, but 8.5% and 2.6% lower ADICP and NDICP contents, respectively, observed in the experimental alfalfa cube. The impact of these differences culminated in a slightly lower TDN for the experimental sample than that obtained in calculating TDN with the Weiss et al. (1992) summative equations for alfalfa cubes using the NRC (National Academies of Sciences, Engineering, And Medicine, 2016) reference ranges ((52.6 vs 58.3), respectively; data not shown). However, these differences illustrate the point by Weiss and St-Pierre (2009) that it is imperative that all feedstuffs for a diet are analyzed for nutrient content if an accurate estimation of energy value is to be obtained, a point which underscores our assertion that a database of multiple cultivars collected from several regions, climates, and years is needed to truly determine the suitability of hemp as a feedstuff and estimate its average nutritional value and ranges.
During the planning stages of Experiments 2 and 3, alfalfa cubes were selected as a comparison feedstuff due to similar nutritional values with those reported for industrial hemp by Kleinhenz et al. (2020), particularly regarding the NDF content. However, in the current study, results indicate these two feedstuffs have greater differences than similarities regarding both digestibility and composition. Except for lignin, differences were observed for every nutrient evaluated, with alfalfa cube composed of lower ash, fat, crude protein, ADICP, and NDICP but higher NDFOM content as compared with both hemp groups. Calculated TDNOF was similar between non- and CBD-extracted hemp samples but comparatively higher for alfalfa cubes than any of the hemp samples, regardless of extraction type. In contrast to TDNOF, the observed IVTDAFOF for alfalfa cube was within the range of CBD-extracted hemp but above that of non-extracted biomass. Of the three treatments, CBD-extracted hemp exhibited a slightly higher mean IVTDAFOF response than alfalfa cubes and non-extracted hemp.
The relationship of TDNOF with the observed IVTDAFOF of foliage from the 9 non-extracted and CBD-extracted hemp cultivars is presented in Figures 1, 2, respectively. For CBD-extracted samples, the prediction equation had a slope of 0.57 with a strong relationship (Adj. R2 = 0.70) observed. In contrast, no relationship (Adj. R2 = -0.10) between these two variables was observed for non-extracted hemp samples, indicating that the calculated TDNOF may be insufficient for predicting the digestibility of the non-extracted hemp. The relationship of alfalfa cube TDNOF and IVTDAFOF was distinct from all non- and CBD-extracted samples (Figures 1, 2), which corresponds to the aforementioned differences in TDNOF for alfalfa cube compared with those of hemp cultivars. The explanation behind the strong relationship between TDNOF and IVTDAFOF for CBD-extracted, but not non-extracted, cultivars is unknown. It is possible that the limited number of samples used in our experiments was insufficient to truly evaluate this relationship. However, it may also relate to EOs other than CBD that have been previously reported as present in the hemp plant and removed during the CBD extraction process (Moreno et al., 2020). Previous reports demonstrate that EOs are potent modifiers of the ruminal environment, with several studies examining the methane and VFA formation changes associated with the use of these compounds (Busquet et al., 2006; Castillejos et al., 2007; Cobellis et al., 2016). However, to our knowledge, how these EOs may affect the metabolism of dietary rations by beef cattle remains unknown. Thus, to fully evaluate potential relationships between TDN and IVTD observed in these experiments, future research using matched pairs of non- and CBD-extracted inflorescence is needed.
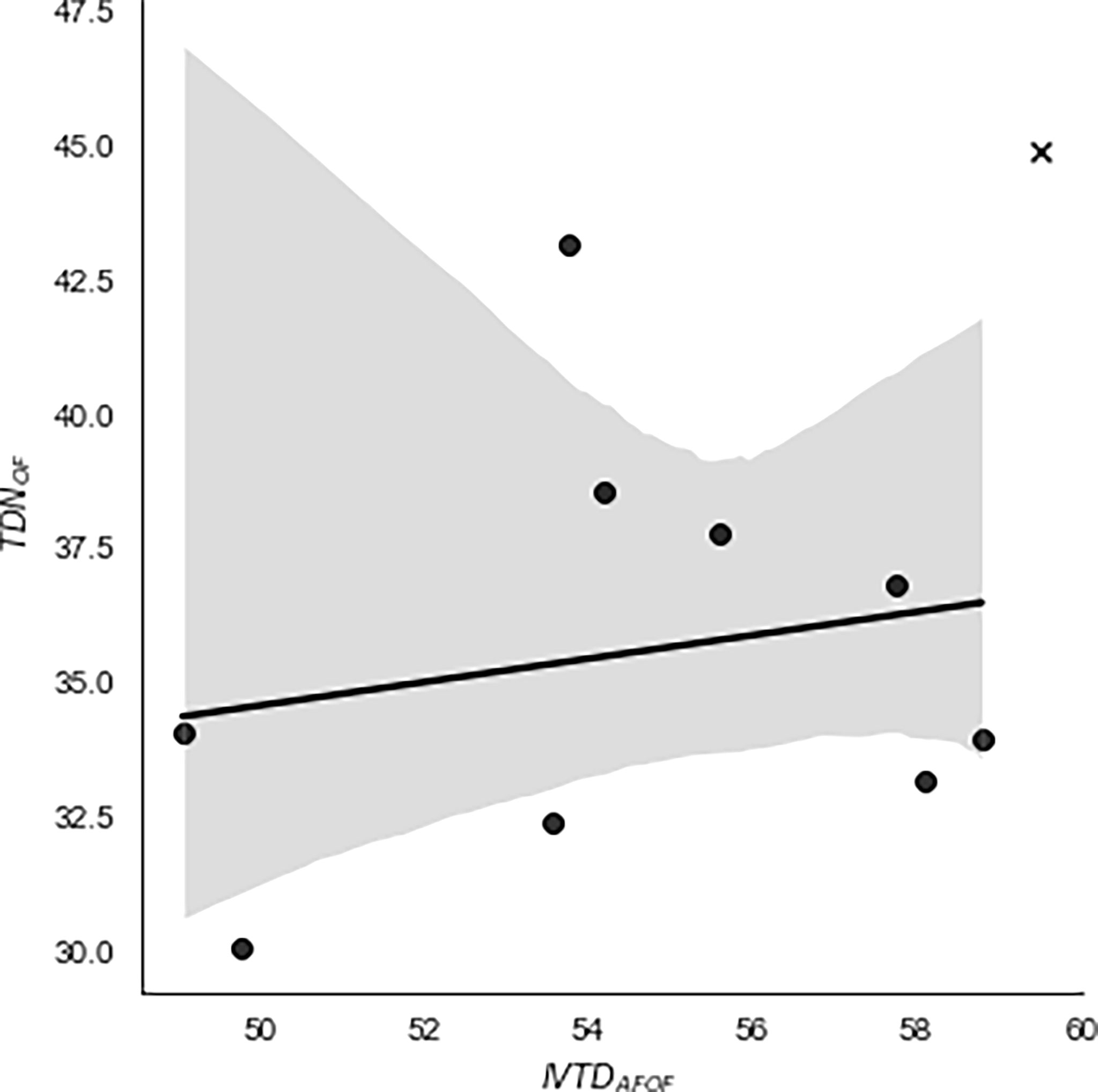
Figure 1 Regression of calculated total digestible nutrients (TDNOF; non-oil basis) against observed in vitro true digestibility (IVTDAFOF; ash-free, non-oil basis) of alfalfa cube and 9 cultivars of non-extracted industrial hemp inflorescence. TDNOF values were calculated using a modified version of the summative equation system proposed by Weiss et al. (1992). To obtain IVTDAFOF measurements, hemp samples were incubated in an ANKOM DaisyII Incubator for 48 h following the manufacturer’s instructions. There was no relationship observed between IVTDAFOF and TDNOF (Adj. R2 = 0.10), and regression of the two variables resulted in the equation TDNOF = 0.2179(IVTDAFOF) + 24.639. Hemp cultivars = •. Alfalfa Cube = X.
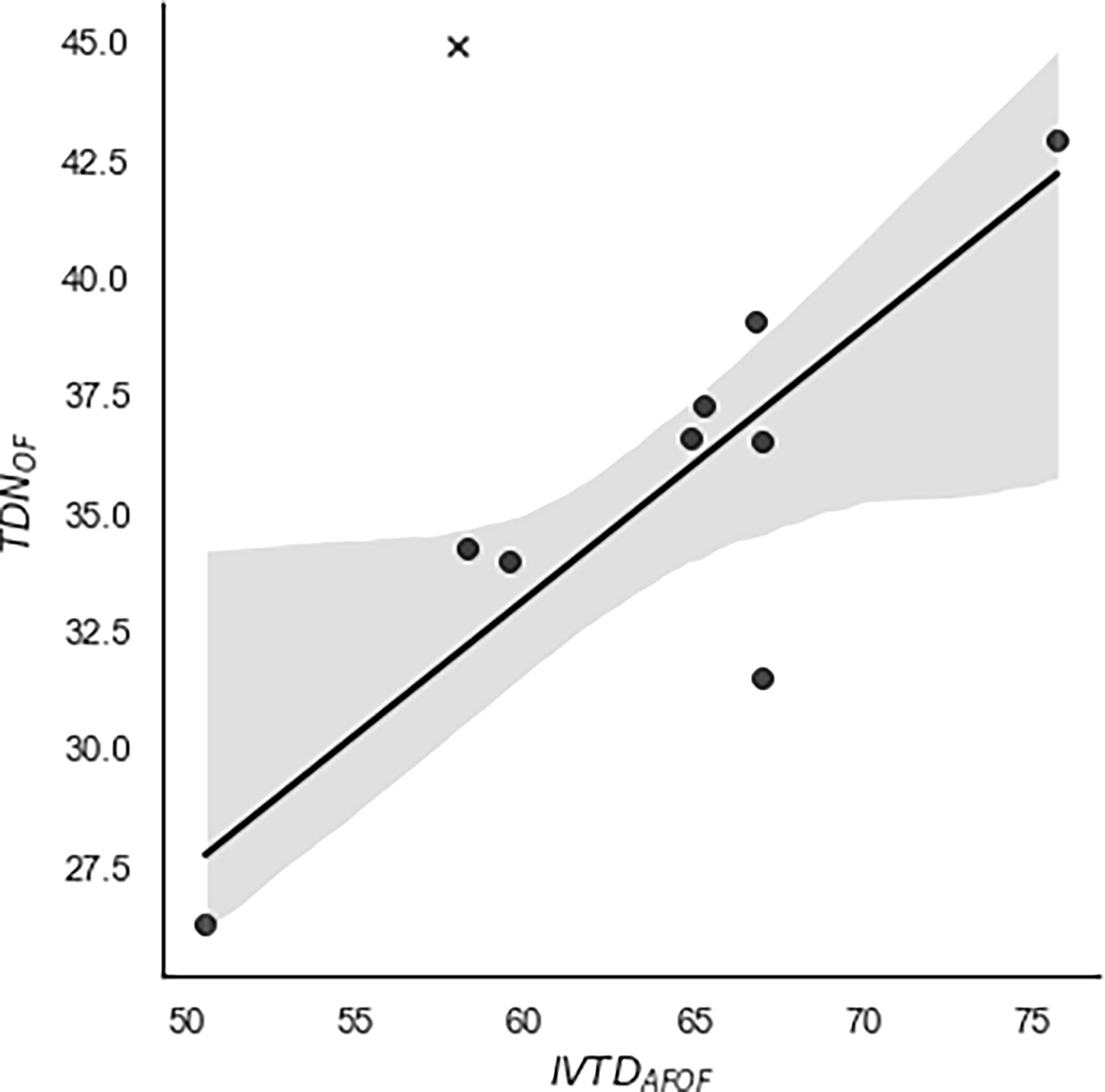
Figure 2 Regression of calculated total digestible nutrients (TDNOF; non-oil basis) against observed in vitro true digestibility (IVTDAFOF; ash-free, non-oil basis) of alfalfa cube and 9 cultivars of CBD-extracted industrial hemp inflorescence. TDNOF values were calculated using a modified version of the summative equation system proposed by Weiss et al. (1992). To obtain IVTDAFOF measurements, hemp samples were incubated in an ANKOM DaisyII Incubator for 48 h following the manufacturer’s instructions. The correlation between IVTDAFOF and TDNOF was moderately strong (Adj. R2 = 0.70) and described using the equation TDNOF = 0.5748(IVTDAFOF) - 0.3502. Hemp cultivars = •. Alfalfa Cube = X.
Experiments 4 and 5
To test the appropriateness of using the Cohen’s d test to compare between Experiments 4 and 5, differences in the products of digestion for the alfalfa cube treatment were compared. As this treatment remained the same across experiments, it would be expected that most, if not all, Cohen’s d values would be equal to or less than 1.5, indicating similarities between experiments. However, most of the products of digestion examined had a Cohen’s d <1.5, suggesting that experimental conditions substantially affected the results and that this type of comparison between experiments may yield results more indicative of differences in experimental conditions (such as inoculum) rather than the extraction status of the hemp. Thus, results between the two experiments were not compared, as any inferences drawn from such a comparison would be confounded by differences in experimental conditions and may not accurately reflect true differences between “average” extracted and non-extracted hemp inflorescence.
Descriptive statistics for non-extracted and CBD-extracted herbage products of digestion resulting from a 48-h incubation period are presented in Tables 5, 6, respectively. Both tables include data from alfalfa cubes digested within the corresponding experiment. Results from both experiments were similar. The average total and individual VFA produced during digestion of the hemp inflorescence did not differ numerically from those of alfalfa cubes digested under the same conditions. Similarly, values for alfalfa cube gas production rate, methane percentage of total gas produced, ammonia, pH, DMD, and total gas production fell within the observed range of the concurrently run hemp cultivars in both experiments. In addition, the standard deviations among hemp samples of the same extraction type were similar to those of replicate alfalfa cube samples within the same experiment, indicating that the observed wide range in hemp nutrient composition had little impact on end products of fermentation. Therefore, it appears that when included at 30% of the diet as part of a mixed ration and evaluated using an in vitro model, foliage from both non- and CBD-extracted hemp are analogous to that of alfalfa cubes regarding the influence on digestibility and formation of products of digestion.
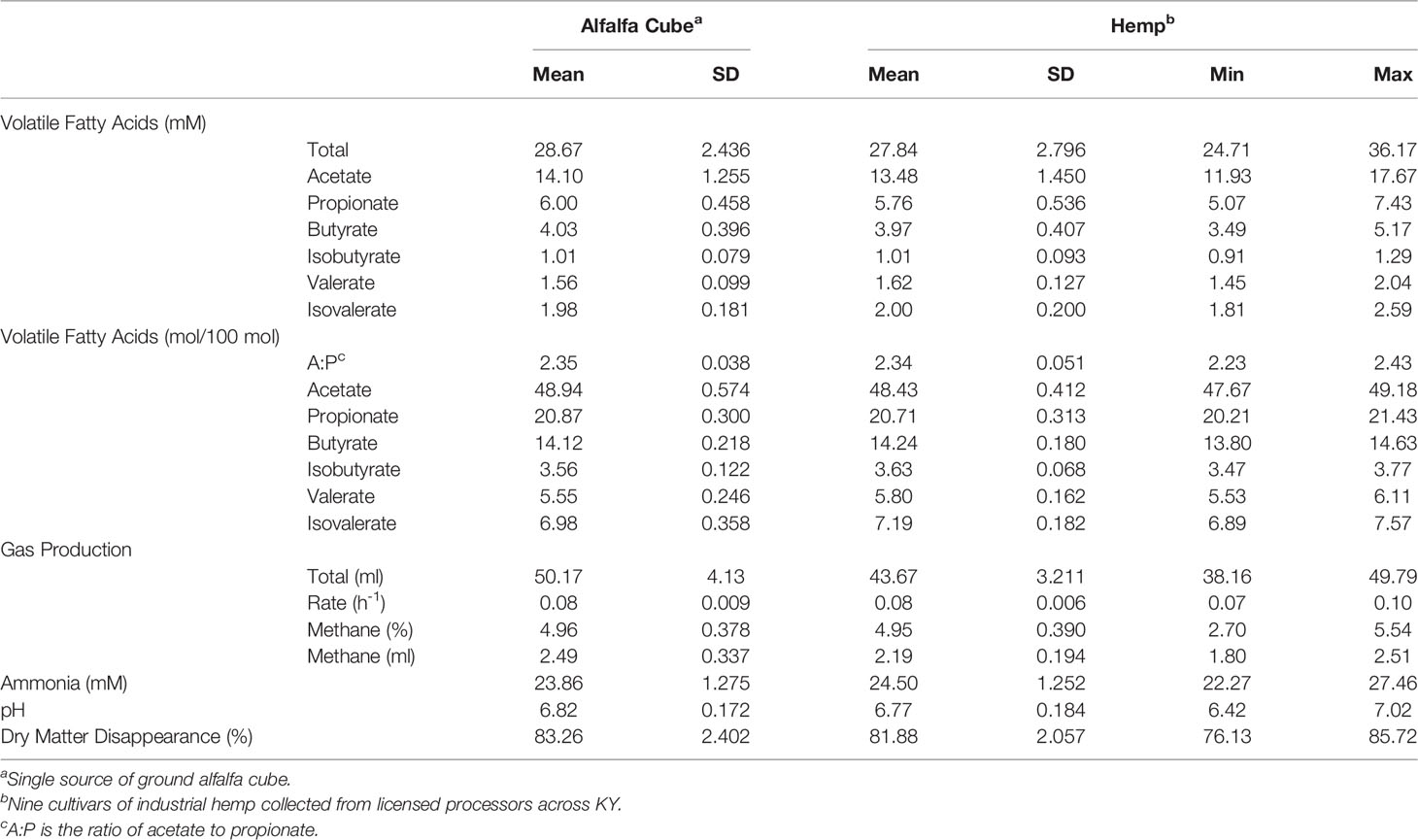
Table 5 Descriptive statistics for in vitro products of digestion of inflorescence from 9 non-extracted hemp cultivars after 48-h digestion period.
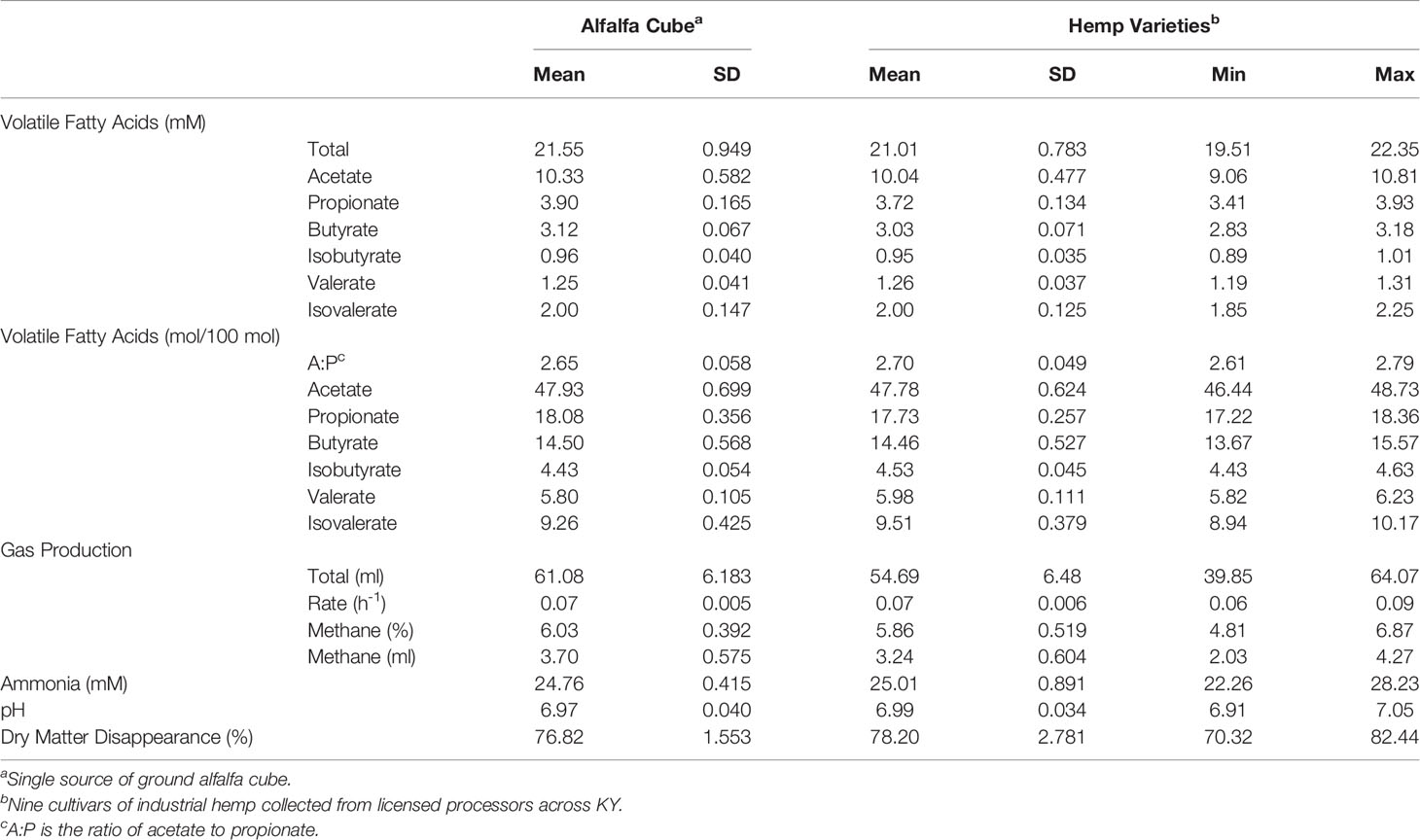
Table 6 Descriptive statistics for in vitro products of digestion of inflorescence from 9 CBD-extracted hemp cultivars after 48-h digestion period.
Conclusion
The results reported in this study serve as a foundation for establishing nutrient reference ranges of industrial hemp foliage, both non- and CBD-extracted forms. Such databases are necessary when establishing feedstuff inclusion during livestock diet formulation. Results here indicate a wide variation of most chemical constituents among cultivars and some systematic differences in composition of extracted and non-extracted inflorescence (in particular for EE and ash components). Despite this variation in composition, there was relatively little variation in in vitro fermentation end products within extraction type when hemp was included at 30% of the in vitro substrate. Furthermore, this is the first report of methane and total gas production measurements when industrial hemp was included as part of a simulated ration. Further analyses using in vivo techniques are required to fully evaluate this unique forage as a potential ruminant feedstuff.
Data Availability Statement
The raw data supporting the conclusions of this article will be made available by the authors, without undue reservation.
Ethics Statement
The animal study was reviewed and approved by Institutional Animal Care and Use Committee.
Author Contributions
AA is post-doc and conducted the research. EV, KM and DH were involved in the concept, data analysis and writing of the manuscript. All authors contributed to the article and approved the submitted version.
Funding
This research was supported by the USDA Agricultural Research Service # 1000100010.The Agronomy of Hemp and Its Uses in Forage-Animal Agriculture.
Conflict of Interest
The authors declare that the research was conducted in the absence of any commercial or financial relationships that could be construed as a potential conflict of interest.
Publisher’s Note
All claims expressed in this article are solely those of the authors and do not necessarily represent those of their affiliated organizations, or those of the publisher, the editors and the reviewers. Any product that may be evaluated in this article, or claim that may be made by its manufacturer, is not guaranteed or endorsed by the publisher.
References
Blümmel M., Becker K. (1997). The Degradability Characteristics of Fifty-Four Roughages and Roughage Neutral-Detergent Fibres as Described by In Vitro Gas Production and Their Relationship to Voluntary Feed Intake. Br. J. Nutr. 77, 757–768. doi: 10.1079/BJN19970073
Busquet M., Calsamiglia S., Ferret A., Kamel C. (2006). Plant Extracts Affect In Vitro Rumen Microbial Fermentation. J. Dairy Sci. 89, 761–771. doi: 10.3168/jds.S0022-0302(06)72137-3
Castillejos L., Calsamiglia S., Ferret A., Losa R. (2007). Effects of Dose and Adaptation Time of a Specific Blend of Essential Oil Compounds on Rumen Fermentation. Anim. Feed Sci. Technol. 132, 186–201. doi: 10.1016/j.anifeedsci.2006.03.023
Cobellis G., Trabalza-Marinucci M., Marcotullio M. C., Yu Z. (2016). Evaluation of Different Essential Oils in Modulating Methane and Ammonia Production, Rumen Fermentation, and Rumen Bacteria In Vitro. Anim. Feed Sci. Technol. 215, 25–36. doi: 10.1016/j.anifeedsci.2016.02.008
Cohen J. (1988). Set Correlation and Contingency Tables. Applied Psychological Measurement. 12, 425–434. doi: 10.1177/014662168801200410
Da Cruz C. H., Santos S. A., De Carvalho G. G. P., Azevedo J., Detmann E., De Campos Valadares Filho S., et al. (2021). Estimating Digestible Nutrients in Diets for Small Ruminants Fed With Tropical Forages. Livest Sci. 249, 104532. doi: 10.1016/j.livsci.2021.104532
Damiran D., Delcurto T., Bohnert D., Findholt S. (2008). Comparison of Techniques and Grinding Size to Estimate Digestibility of Forage Based Ruminant Diets. Anim. Feed Sci. Technol. 141, 15–35. doi: 10.1016/j.anifeedsci.2007.04.007
FAO (2017) Livestock Solutions for Climate Change (Rome, Italy: Food and Agriculture Organization of the United Nations). Available at: http://www.fao.org/3/I8098EN/i8098en.pdf (Accessed May 17, 2021).
Goering H. K., Van Soest P. J. (1970). Forage Fiber Analyses: Apparatus, Reagents, Procedures, and some Applications (Washington, DC:Agricultural Research Service, US Department of Agriculture).
Holder V. B., El-Kadi S. W., Tricarico J. M., Vanzant E. S., Mcleod K. R., Harmon D. L. (2013). The Effects of Crude Protein Concentration and Slow Release Urea on Nitrogen Metabolism in Holstein Steers. Arch. Anim. Nutr. 67, 93–103. doi: 10.1080/1745039X.2013.773647
Iffland K., Grotenhermen F. (2017). An Update on Safety and Side Effects of Cannabidiol: A Review of Clinical Data and Relevant Animal Studies. Cannab Cannab Res. 2, 139–154. doi: 10.1089/can.2016.0034
Kim D., Mizinga K., Kube J., Friesen K., Mcleod K., Harmon D. (2014). Influence of Monensin and Lauric Acid Distillate or Palm Oil on In Vitro Fermentation Kinetics and Metabolites Produced Using Forage and High Concentrate Substrates. Anim. Feed Sci. Technol. 189, 19–29. doi: 10.1016/j.anifeedsci.2013.12.010
Kleinhenz M. D., Magnin G., Ensley S. M., Griffin J. J., Goeser J., Lynch E., et al. (2020). Nutrient Concentrations, Digestibility, and Cannabinoid Concentrations of Industrial Hemp Plant Components. Appl. Anim. Sci. 36, 489–494. doi: 10.15232/aas.2020-02018
Kun E., Kearney E. B. (1974). Ammonia in Methods of Enzymatic Analysis (Cambridge, MA:Elsevier). doi: 10.1016/B978-0-12-091304-6.50031-8
Liu X., Yang J., Ye T., Han Z. (2018). Establishment of Analysis Method for Methane Detection by Gas Chromatography. IOP Conf. Ser: Earth Environ. Sci., 13:012023. doi: 10.1088/1755-1315/113/1/012023
Lofgreen G. P., Garrett W. (1968). A System for Expressing Net Energy Requirements and Feed Values for Growing and Finishing Beef Cattle. J. Anim. Sci. 27, 793–806. doi: 10.2527/jas1968.273793x
Moreno T., Montanes F., Tallon S. J., Fenton T., King J. W. (2020). Extraction of Cannabinoids From Hemp (Cannabis Sativa L.) Using High Pressure Solvents: An Overview of Different Processing Options. J. Supercritic Fluid 161, 104850. doi: 10.1016/j.supflu.2020.104850
National Academies of Sciences, Engineering, And Medicine (2016). Nutrient Requirements of Beef Cattle (Washington, DC: The National Academies).
Pitt R., Cross T., Pell A., Schofield P., Doane P. (1999). Use of In Vitro Gas Production Models in Ruminal Kinetics. Math. Biosci. 159, 145–163. doi: 10.1016/S0025-5564(99)00020-6
Tedeschi L., Fox D., Doane P. (2005). Evaluation of the Tabular Feed Energy and Protein Undegradability Values of the National Research Council Nutrient Requirements of Beef Cattle. Prof. Anim. Sci 21, 403–415. doi: 10.15232/S1080-7446(15)31238-9
Tilley J., Terry D. R. (1963). A Two-Stage Technique for the In Vitro Digestion of Forage Crops. Grass Forage Sci. 18, 104–111. doi: 10.1111/j.1365-2494.1963.tb00335.x
Trotta R., Klotz J., Harmon D. (2018). Effects of Source and Level of Dietary Energy Supplementation on In Vitro Digestibility and Methane Production From Tall Fescue-Based Diets. Anim. Feed Sci. Technol. 242, 41–47. doi: 10.1016/j.anifeedsci.2018.05.010
Van Soest P. V., Robertson J., Lewis B. (1991). Methods for Dietary Fiber, Neutral Detergent Fiber, and Nonstarch Polysaccharides in Relation to Animal Nutrition. J. Dairy Sci. 74, 3583–3597. doi: 10.3168/jds.S0022-0302(91)78551-2
Vastolo A., Calabrò S., Pacifico S., Koura B. I., Cutrignelli M. I. (2021). Chemical and Nutritional Characteristics of Cannabis Sativa L. Co-Products. J. Anim. Physiol. Anim. Nutr. 105, 1–9. doi: 10.1111/jpn.13557
Weiss W. (1993). Symposium: Prevailing Concepts in Energy Utilization by Ruminants. J. Dair Sci. 76, 1802–1811. doi: 10.3168/jds.S0022-0302(93)77512-8
Weiss W., Conrad H., Pierre N. S. (1992). A Theoretically-Based Model for Predicting Total Digestible Nutrient Values of Forages and Concentrates. Anim. Feed Sci. Technol. 39, 95–110. doi: 10.1016/0377-8401(92)90034-4
Weiss W. P., St-Pierre N. R. (2009). “Impact and Management of Variability in Feed and Diet Composition,” in Tri-State Dairy Nutrition Conf. Proc., Fort Wayne, Indiana (East Lansing: Michigan State University), 83–96.
Weiss W. P., Tebbe A. W. (2019). Estimating Digestible Energy Values of Feeds and Diets and Integrating Those Values Into Net Energy Systems. Trans. Anim. Sci. 3, 953–961. doi: 10.1093/tas/txy119
Williams D. W., Mundell R. (2018). An Introduction to Industrial Hemp and Hemp Agronomy (Lexington, Kentucky: University of Kentucky).
Keywords: hemp, cattle, IVTD, CBD, inflorescence, methane
Citation: Altman AW, Vanzant ES, McLeod KR and Harmon DL (2022) In Vitro Measurements of True Digestibility and Products of Digestion Using Multiple Cultivars of Non-Extracted and CBD-Extracted Industrial Hemp Biomass (Cannabis sativa). Front. Anim. Sci. 3:915916. doi: 10.3389/fanim.2022.915916
Received: 08 April 2022; Accepted: 17 June 2022;
Published: 22 July 2022.
Edited by:
Luciana ROSSI, University of Milan, ItalyReviewed by:
Matteo Dell’Anno, University of Milan, ItalyAnusorn Cherdthong, Khon Kaen University, Thailand
Copyright © 2022 Altman, Vanzant, McLeod and Harmon. This is an open-access article distributed under the terms of the Creative Commons Attribution License (CC BY). The use, distribution or reproduction in other forums is permitted, provided the original author(s) and the copyright owner(s) are credited and that the original publication in this journal is cited, in accordance with accepted academic practice. No use, distribution or reproduction is permitted which does not comply with these terms.
*Correspondence: David L. Harmon, ZGF2aWQuaGFybW9uQHVreS5lZHU=