- 1Department of Animal and Dairy Science, University of Georgia, Athens, GA, United States
- 2Department of Animal Science, Texas A&M University, College Station, TX, United States
- 3ABS Global, DeForest, WI, United States
The use of Doppler ultrasonography to quantify blood vascularization in reproductive organs has increased over the past decade. Doppler technology has predominantly been explored in research settings to evaluate uterine blood flow and to assess follicular and luteal blood perfusion. Recent research has also explored the use of Doppler technology in applied reproductive management for both the beef and dairy industries and has focused on the use of luteal color Doppler ultrasonography to evaluate embryo transfer recipients and perform early pregnancy diagnosis. Although significant progress has been made and current literature indicates a strong potential for the applied use of Doppler ultrasonography to increase reproductive efficiency in the cattle industry, uptake of this technology is still currently limited. This review summarizes the recent developments in the applied use of color Doppler ultrasonography for reproductive management in both beef and dairy cattle herds.
Introduction
Development of ultrasound technology has contributed significantly to bovine reproduction research and veterinary medicine. Conventional B-mode (brightness mode) ultrasonography was initially adopted in bovine reproduction research in the early 1980s and enabled researchers to perform live evaluations of the female reproductive tract by generating two-dimensional greyscale images. This technology was paramount in increasing the understanding of cattle reproductive biology and facilitated the development of certain reproductive biotechnologies. As an example, ultrasonography played an essential role in the characterization of the wave-like pattern of follicular growth (Fortune et al., 1988; Ginther et al., 1989). Such knowledge was fundamental in the development of advanced estrus synchronization programs, which in turn have revolutionized the use of reproductive biotechnologies in both beef and dairy herds (Reviewed by Lamb et al., 2016 and Bisinotto et al., 2014, respectively). In addition to its contributions to research, B-mode ultrasonography is also commonly used in bovine veterinary practice to examine the reproductive tract, assess reproductive pathologies, perform pregnancy diagnoses, evaluate fetal sex and viability, and assess female physiological status.
Doppler ultrasonography is a relatively newer technology used to evaluate reproductive organs and allows for the detection of blood flow and blood perfusion using the Doppler effect. Clinically relevant information on the vascularization of specific organs or structures within these organs is provided and can be used to estimate their functionality. Among the many opportunities for the use of this technology in bovine reproduction, Doppler ultrasonography has been explored as a tool to determine uterine blood flow during gestation (Bollwein et al., 2002) and to assess follicular and luteal blood perfusion (Ginther et al., 2007a, b). More specifically, Color Doppler ultrasonography has been used to identify recipient females with greater probabilities of pregnancy success after embryo transfer (ET; Pugliesi et al., 2019) and as a tool for early pregnancy diagnosis (Siqueira et al., 2013; Pugliesi et al., 2014; Holton et al., 2022a). By allowing non-pregnant females to be diagnosed earlier, color Doppler has also created opportunities for earlier resynchronization with fixed-time artificial insemination (TAI; Pugliesi et al., 2019; Palhão et al., 2020) and fixed-time ET (FTET; Pugliesi et al., 2018). This article aims to review current literature and discuss future opportunities for color doppler ultrasonography in applied bovine reproduction.
Basic Principles of Doppler Ultrasonography
B-mode ultrasonography utilizes sound waves that are emitted from a transducer. These sound waves penetrate tissues and are then reflected back to the transducer at varying frequencies depending on the density of the tissue and are processed by the ultrasound unit into a gray-scale image (Figures 1A–C; Pierson et al., 1988). Doppler ultrasonography allows for the estimation of blood flow and blood perfusion that can be used to indicate the functionality of reproductive structures and organs. These blood parameters are estimated by making use of the principles of the Doppler effect, characterized by the change in frequency of a wave (e.g., sound or light) when the wave observer or wave source moves towards or away from one another. In the context of blood vascularization, Doppler identifies changes in the frequency of sound waves that are sent by the transducer (source) and are reflected by red blood cells (target), which are constantly changing their position in relation to the transducer and also serve as the observer. When red blood cells move towards the transducer, the frequency of reflected ultrasound waves is greater than the frequency of emitted waves, resulting in a positive Doppler shift. When the frequency of the reflected waves is lower than that of the emitted waves, such as when red blood cells move away from the transducer, a negative Doppler shift occurs (Ginther and Utt, 2004).
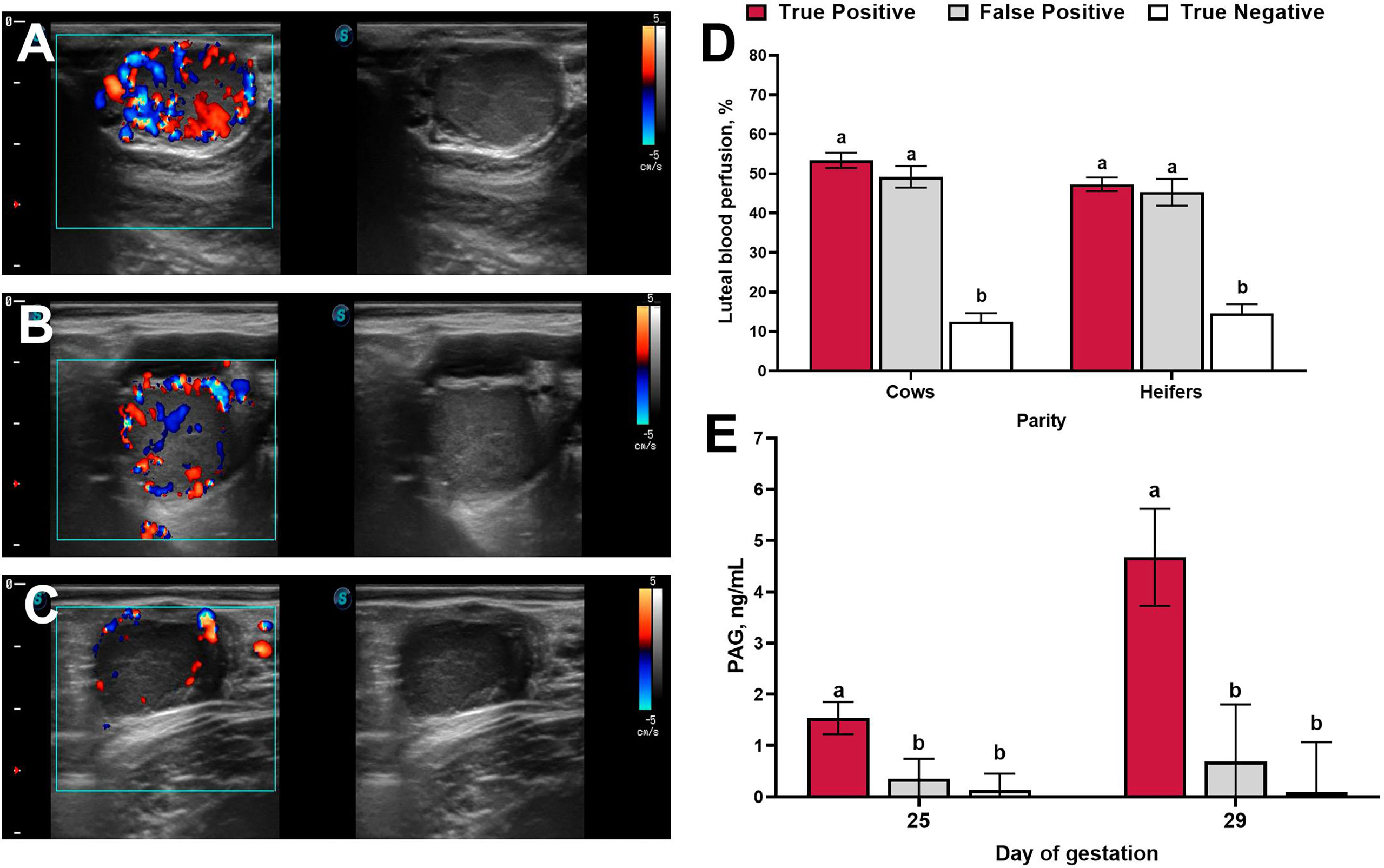
Figure 1 Representative ultrasound images of luteal blood perfusion collected on day 20 of gestation from beef cows classified as true-positive (A), false-positive (B), and true-negative (C). (D): Luteal blood perfusion on day 20 of gestation in cows (n = 208) and heifers (n = 178) with different pregnancy diagnosis results. (E): Circulating concentrations of pregnancy associated glycoproteins (PAG) on days 25 and 29 of gestation according to pregnancy diagnosis results. Uncommon superscripts represent statistical differences in least-square means (P ≤ 0.05). Adapted from Holton et al. (2022a).
Three main modes are used during Doppler ultrasonography — spectral, power, and color Doppler — and each can be used to assess different blood parameters. In spectral Doppler mode, the Doppler shift in frequency of sound waves is represented as a graph where blood flow over a certain period can be visualized. This mode is useful when estimating waveform and blood flow distribution and can be used to calculate blood flow velocity and indices such as pulsatility index and resistance index (Ginther and Utt, 2004). Spectral Doppler mode is commonly used to estimate blood flow in larger blood vessels like the uterine artery, yet due to limitations, for instance the difficulty in establishing the correct angle between the transducer and the flow of blood and the considerable potential for inaccurate results, it is not often used to measure blood flow in smaller vessels such as the ovarian vasculature (reviewed by Viana et al., 2013). Power Doppler mode measures the movement of blood cells through a blood vessel over a certain period, known as the blood flow intensity, and is superior for imaging structures with very little blood flow such as the basement membrane region of dominant follicles (reviewed by Bollwein et al., 2016). The Doppler shift can be visualized by colors that usually range from yellow to red and are superimposed over a B-mode ultrasound image where the intensity of the color represents the intensity of blood flow. These colors indicate the vascularization of a structure but supply no information on the direction of the flow of blood. In color Doppler mode, the Doppler shift can also be visualized as color signals superimposed over a conventional B-mode image (Figure 1A-C). However, higher frequencies, or positive shifts, are indicated by colors ranging from yellow to red, and lower relative frequency changes, or negative shifts, are indicated by colors ranging from green to blue. The color gradients described above are the most conventionally used: however, they can be changed according to ultrasound settings and operator preference. Using color signal information, the percentage of a given area that has blood perfusion can either be objectively estimated using specific software (Ginther and Utt, 2004; Herzog et al., 2010) or subjectively estimated via vascularization scores or ranges (Ginther and Utt, 2004; Pugliesi et al., 2018; Velho et al., 2021).
Blood Flow in the Female Reproductive Tract
Uterine Blood Flow
Doppler ultrasonography has been utilized extensively in research settings to evaluate changes in uterine blood flow (UBF) during the estrous cycle (Bollwein et al., 2000; Honnens et al., 2008; Bollwein et al., 2016), fetal and placental development (Camacho et al., 2014; Brockus et al., 2016; Lemley et al., 2018), and changes in blood flow associated with postpartum uterine involution (Heppelmann et al., 2013). The technique used to estimate UBF is relatively simple and has been well documented and described by others (reviewed by Bollwein et al., 2016). Uterine blood flow fluctuates during the bovine estrous cycle in non-pregnant females and has been correlated to circulating concentrations of estrogen and progesterone, where blood flow velocity is greater during proestrus and estrus and lower during diestrus (Bollwein et al., 2000; Honnens et al., 2008). An increase in uterine blood flow begins shortly after the establishment of pregnancy and can be detected within the first 21 days (Honnens et al., 2008; Silva et al., 2010). Interestingly, the spatial location of the conceptus during early gestation is associated with a local increase in endometrial vascularization that is detectable using Doppler ultrasonography (Silva et al., 2009). However, due to considerable variation among cows, uterine blood flow is not currently a suitable tool for early pregnancy diagnosis in cattle (Honnens et al., 2008).
Substantial increases in blood flow to the reproductive tract are needed to support the exchange of nutrients and waste between the maternal and fetal circulations during pregnancy, where the efficiency of placental nutrient transport is directly related to placental blood flow (Reynolds and Redmer, 1995). Therefore, adequate maternal blood flow to the reproductive tract is crucial for fetal development. Doppler ultrasonography has been used as a non-invasive technique in reproductive research to quantify UBF and to make inferences on how different management strategies may affect offspring development or pregnancy outcomes (Bollwein et al., 2002; McCarty et al., 2018; Gard Schnuelle et al., 2021). For example, differences in UBF were detected by color Doppler between cows exposed to nutrient restriction during early and mid-gestation and control cows (Camacho et al., 2014; Lemley et al., 2018). These differences in UBF were supported by histological differences in post-harvest placentome capillaries and differences in macroscopic vascular density of placentomes (Lemley et al., 2018), indicating that color Doppler can be used to provide insights into uterine and placental vascular adaptation in response to environmental insults (Lemley, 2017). Research using color Doppler to estimate UBF during pregnancy is ongoing and will continue to contribute to our understanding of placental development and prenatal programming of offspring (Bollwein et al., 2002; Panarace et al., 2006; Sharma et al., 2021).
Follicular Blood Perfusion
Changes in follicular blood perfusion have been investigated during follicular growth and development (Acosta et al., 2005). Sufficient blood supply appears to be important for follicles to achieve dominance, as high vascularization is detected in dominant and pre-ovulatory follicles but not in atretic follicles (Acosta et al., 2005; Miyamoto et al., 2006). Follicular blood perfusion has also been positively associated with concentrations of steroid hormones in follicular fluid (Grazul-Bilska et al., 2007; Pancarci et al., 2012; de Tarso et al., 2017). Grazul-Bilska and colleagues harvested ovaries from beef heifers three days after estrus expression at the start of follicle dominance in the subsequent follicular wave. In this study, follicle vascularity, determined through immunolocalization of angiogenic factors, was greater in follicles retrospectively classified as potentially dominant (estrogen-active) when compared with those classified as potentially nondominant (estrogen-inactive) and tended to be positively correlated with the estradiol:progesterone ratio (Grazul-Bilska et al., 2007). Positive relationships between follicle vascularity and the follicular estradiol:progesterone ratio have also been reported in dairy cows examined with color Doppler ultrasonography (Pancarci et al., 2012). In the same study, the percentage of estradiol-active follicles was greater when follicular blood flow was detected, and positive associations were determined between concentrations of estradiol and follicle size. Blood perfusion of the preovulatory follicle is also positively correlated with the diameter and vascularization of the subsequent corpus luteum and its ability to secrete progesterone (de Tarso et al., 2017). These results suggest that follicle vascularization could be used as a tool to indicate functionality. Therefore, because larger dominant follicles have greater vascularization and a greater ability to secrete estradiol (Pancarci et al., 2012; Perry et al., 2014), it could be inferred that they have greater functionality than smaller follicles, particularly since larger follicles have greater ovulation rates and subsequent CL areas, and have resulted in greater pregnancy rates than smaller follicles (Perry et al., 2007; Mesquita et al., 2014; Pugliesi et al., 2016).
High follicular vascularization has also been associated with increased pregnancy rates and follicular blood perfusion measurements have been used to predict pregnancy success after in vitro fertilization and ET in women (Chui et al., 1997; Coulam et al., 1999). In cattle, color Doppler assessment of follicular blood perfusion indicated greater preovulatory blood perfusion in heifers that became pregnant compared with heifers that failed to become pregnant (Siddiqui et al., 2009a). In addition, greater vascular perfusion of the preovulatory follicle was observed in follicles that resulted in cleaved versus non-cleaved oocytes during an in vitro fertilization (Siddiqui et al., 2009b). Pancarci and colleagues also evaluated perifollicular blood flow with color Doppler ultrasonography and found that greater quality cumulus-oocyte complexes were obtained from follicles with detectable blood flow compared with follicles without detectable blood flow (Pancarci et al., 2012). Results from these studies indicate that greater follicular vascularization is positively related to follicular function as well as variables associated with in vitro fertilization and pregnancy outcomes in cattle. Color Doppler analysis of follicular blood flow has contributed to our current understanding of the role of follicular vascularization on fertility; however, there is currently limited use for follicular blood perfusion measurements in reproductive management of cattle.
Luteal Blood Perfusion
Analysis of circulating concentrations of progesterone is the most reliable way to assess CL function, since the primary function of a CL is to secrete progesterone during diestrus and pregnancy. Nevertheless, the time and costs associated with analyzing concentrations of progesterone are major drawbacks to it being regularly used in reproductive management. Due to the speed and reliability of conventional B-mode ultrasonography, this technique is commonly used to evaluate the presence and size of a CL as an estimate of its function, since CL diameter (Spell et al., 2001; Velho et al., 2021) and CL area (Berger et al., 2017; Pugliesi et al., 2019) have been associated with circulating concentrations of progesterone. However, this correlation is reduced during luteolysis as the rates of decrease in circulating concentrations of progesterone are greater than the decrease in CL size (Kastelic et al., 1990; Assey et al., 1993).
The CL is a highly vascularized structure during diestrus yet undergoes an extensive decrease in blood perfusion and luteal tissue volume during luteolysis (Ginther et al., 2007b). Several studies have utilized color Doppler to characterize morphological and functional changes in the CL during this period (Miyamoto et al., 2005; Herzog et al., 2010; Rocha et al., 2019). Interestingly, a decrease in luteal blood perfusion occurs prior to structural regression (Niswender et al., 1994; Herzog et al., 2010) and the correlation between CL blood perfusion and circulating concentrations of progesterone is greater during luteolysis than the correlation between CL area and progesterone (Rocha et al., 2019). For these reasons, the use of color Doppler to evaluate CL functional status during luteolysis has substantially increased in research settings over the past few decades and has provided important insights into temporal changes to CL function.
Luteal Color Doppler Ultrasonography for Early Pregnancy Diagnosis
During early pregnancy in cattle, the free-floating conceptus undergoes remarkable trophectoderm cell proliferation, significantly increasing its surface area prior to adhering to the uterine luminal epithelium (Betteridge and Flechon, 1988; Guillomot, 1995). After trophectoderm attachment the bovine conceptus starts accumulating fluid within the developing chorioallantois (Schlafer et al., 2000; Peter, 2013), which can both be visualized during pregnancy diagnosis with a conventional B-mode ultrasound. While anechoic structures can be visualized within the uterine lumen as early as day 14 of gestation via B-mode ultrasonography (Pierson and Ginther, 1984), it is recommended that veterinarians perform pregnancy diagnosis no earlier than 28 days after breeding. This recommendation is based on studies that show a decrease in accuracy when conventional ultrasound examination is performed earlier than 28 days after breeding (Nation et al., 2003). Color Doppler ultrasonography has been proposed as a method to diagnose pregnancy earlier than conventional ultrasound by recognizing females that are undergoing CL regression (Siqueira et al., 2013; Pugliesi et al., 2014; Scully et al., 2015), as most non-pregnant females undergo luteolysis before pregnancy diagnosis can be performed with conventional ultrasound.
A summary of controlled studies evaluating the estimates of agreement between luteal color Doppler and B-mode ultrasonography is presented in Table 1. When color Doppler was used to diagnose pregnancy in lactating dairy cows on day 20 of gestation, low rates of false-negative results (0.7%) but high rates (24.6%) of false-positive results were reported (Siqueira et al., 2013). Conversely, studies using Bos indicus beef cattle observed no false-negative results and only 9% false-positive results when color Doppler was utilized 20 days after TAI (Pugliesi et al., 2014). A potential contributing factor to the variation in the number of false-positive results in these studies is the percentage of cows that experience early embryonic mortality between days 20 and 30 of gestation, as a greater proportion of dairy cows experience early embryonic mortality compared with beef cows (Wiltbank et al., 2016; Reese et al., 2020). Additionally, the criteria utilized to distinguish pregnant and non-pregnant cows were different in the abovementioned studies and could also potentially explain the differences in the number of false-positive results. Siqueira et al. (2013) considered females that did not have color signals in the central region of the CL as non-pregnant, whereas Pugliesi et al. (2014) considered cows to be non-pregnant when the percentage of their CL area with blood perfusion signals was ≤ 25% or when their CL area was < 2 cm2. Noteworthy, both studies indicated high sensitivity (probability that the test yields a positive result when cows are in fact pregnant) and negative predicted value (probability that a cow is not pregnant when diagnosed as non-pregnant).
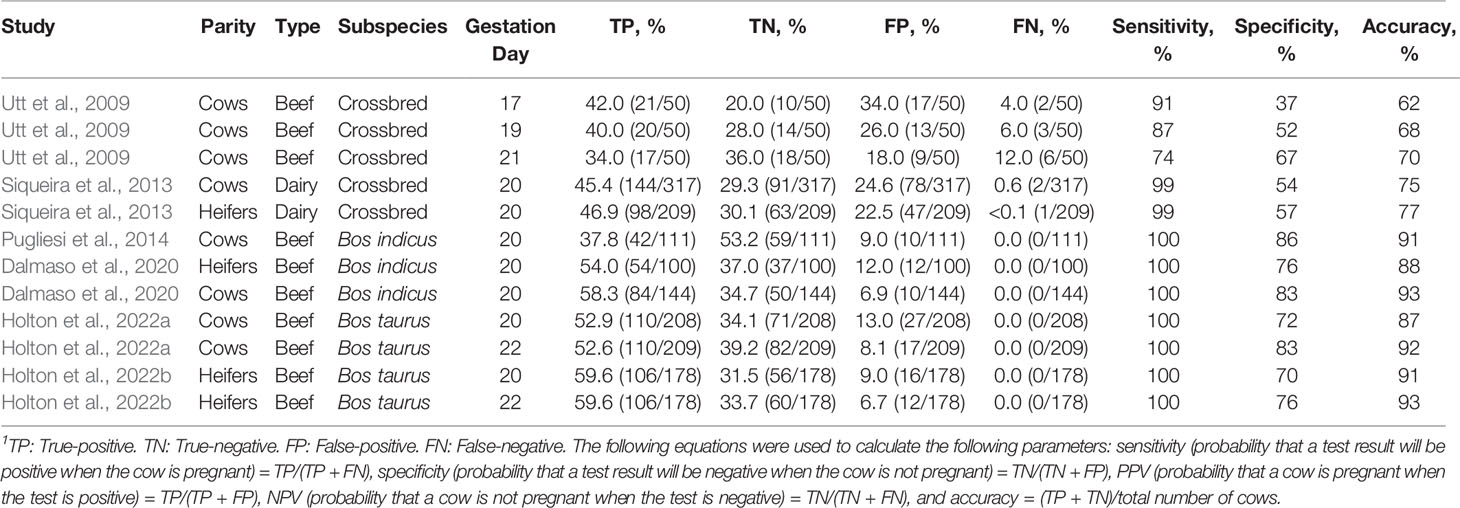
Table 1 Summary of estimates of agreement between luteal color Doppler ultrasonography and conventional ultrasonography to diagnose pregnancy in cattle1.
Most studies evaluating the use of color Doppler for early pregnancy diagnosis in beef cattle were performed in Bos indicus breeds, yet, several physiological differences exist between Bos indicus and Bos taurus subspecies, including differences in reproductive biology that have implications to reproductive management (reviewed by Sartori et al., 2016). Due to differences in CL morphometry (Sartori and Barros, 2011; Sartori et al., 2016) and early embryonic mortality (Reese et al., 2020) between subspecies, it was unclear if the accuracy of color Doppler examinations in Bos taurus and Bos indicus beef cattle populations were comparable. Therefore, Holton and colleagues recently evaluated the use of color Doppler in Bos taurus beef cows and replacement heifers (Holton et al., 2022a; Holton et al., 2022b; Table 1) using similar criteria to Pugliesi et al. (2014) in Bos indicus females. Overall accuracies of color Doppler in postpartum cows were 86 and 91% on days 20 and 22 of gestation, respectively. Comparable results were observed in replacement heifers, where accuracies for days 20 and 22 of gestation were 91 and 93%, respectively. Similar to the studies performed in Bos indicus cattle (Pugliesi et al., 2014; Melo et al., 2020; Table 1), the criteria utilized to identify non-pregnant Bos taurus females yielded no false-negative results in either cows or heifers. These findings are encouraging in the context of resynchronization, as false-negative results would result in pregnancy loss after administration of prostaglandin F2α during the resynchronization protocol. The percentage of overall cows classified as false positives on days 20 and 22 were 13 and 9%, and the percentages of the overall heifers classified as false positives were 9 and 7%, respectively. When evaluated only within non-pregnant females, false-positive results represented 27.5 and 22.2% of the non-pregnant cows and heifers on day 20. On day 22, false-positive results represented 17.1 and 16.7% of the non-pregnant cows and heifers, respectively. These results indicate that luteal color Doppler allows for earlier resynchronization of the majority of non-pregnant females when compared with conventional B-mode ultrasonography.
Representative ultrasound images of luteal blood perfusion collected from cows classified as true positives, false positives, and true negatives are shown in Figures 1A–C. Luteal blood perfusion on days 20 and 22 after TAI were not different in cows or heifers classified as true-positive or false-positive (Holton et al., 2022a; Holton et al., 2022b; Figure 1D). Several factors could be driving the presence of false-positive diagnoses (active luteal blood perfusion in non-pregnant cows) observed when color Doppler ultrasonography is utilized around day 20 of gestation, including delayed ovulation during the estrus synchronization protocol, an intrinsic prolonged luteal phase, or a prolonged luteal phase due to pregnancy loss after conceptus secretion of interferon-τ (Bazer et al., 2008). A recent study investigated differences in the expression of interferon-stimulated genes (ISG15 and OAS1) in peripheral blood leukocytes of cows that had active luteal blood perfusion 20 days after TAI (Melo et al., 2020). Interferon-stimulated genes are upregulated in peripheral blood leukocytes in response to interferon-τ secretion by the conceptus (Gifford et al., 2007; Green et al., 2010) and are commonly utilized as an indirect assessment of conceptus development during early gestation (Fontes et al., 2019). In this study, only 32% of cows considered non-pregnant via conventional ultrasound on day 30 but pregnant via color Doppler on day 20 (false positives) had upregulation of interferon-stimulated genes on day 20 (Melo et al., 2020). Of these cows, 29% (9% of the false-positive cows), had an increase in circulating concentrations of pregnancy associated glycoproteins (PAG) 25 days after TAI (Melo et al., 2020). Similar results were reported by Holton et al., 2022a, where false-positive cows (pregnant via color Doppler on day 20 and non-pregnant via conventional ultrasound on day 30) had similar circulating concentrations of PAG on days 25 and 29 after TAI compared with cows that were considered non-pregnant by both conventional ultrasound on day 30 and color Doppler on day 20 (true negative; Figure 1E). In addition, only 11% of cows classified as false positive by color Doppler had detectable concentrations of PAG on day 25 of gestation. Collectively, these results indicate that only a small proportion of cows classified as false-positive via color Doppler on day 20 appear to experience pregnancy loss, yet some of the false-positive cows could be experiencing pregnancy loss prior to conceptus elongation and placental attachment, resulting in no changes in the expression of interferon-stimulated genes and circulating concentrations of PAG, respectively.
While the prevalence of false-positive results is low, they could represent a limitation for the commercial use of color Doppler ultrasound in the beef industry, particularly when considering the large proportion of pregnancy loss that occurs within the first months of gestation (Reese et al., 2020). Figure 2 provides a summary of the proportion of pregnant cows and heifers based on luteal color Doppler ultrasound on day 20 and B-mode ultrasound on both days 29 to 30 and 70 to110 (final pregnancy diagnosis). Data in Figure 2 were compiled from previous studies that reported changes in the proportion of pregnant cows during early gestation based on sequential ultrasound evaluations (Melo et al., 2020; Holton et al., 2022a,b). Studies utilized in this dataset did not include resynchronization strategies. The proportion of pregnant cows and heifers between the first pregnancy diagnosis performed with color Doppler on day 20 and the second pregnancy diagnosis performed with B-mode ultrasound on days 29 to 30 decreased by 10.5 and 10.1%, respectively. In addition, the proportion of cows and heifers diagnosed as pregnant by B-mode ultrasound between days 29 to 30 and 70 to 110 decreased by 3.7 and 6.5%, respectively. Therefore, there was a 14.2 and 16.6% decrease in the proportion of cows and heifers diagnosed as pregnant between the Doppler ultrasound on day 20 of gestation and the final pregnancy diagnosis. Future research focused on decreasing the proportion of false-positive results when utilizing color Doppler for early pregnancy diagnosis may facilitate its adoption in the cattle industry.
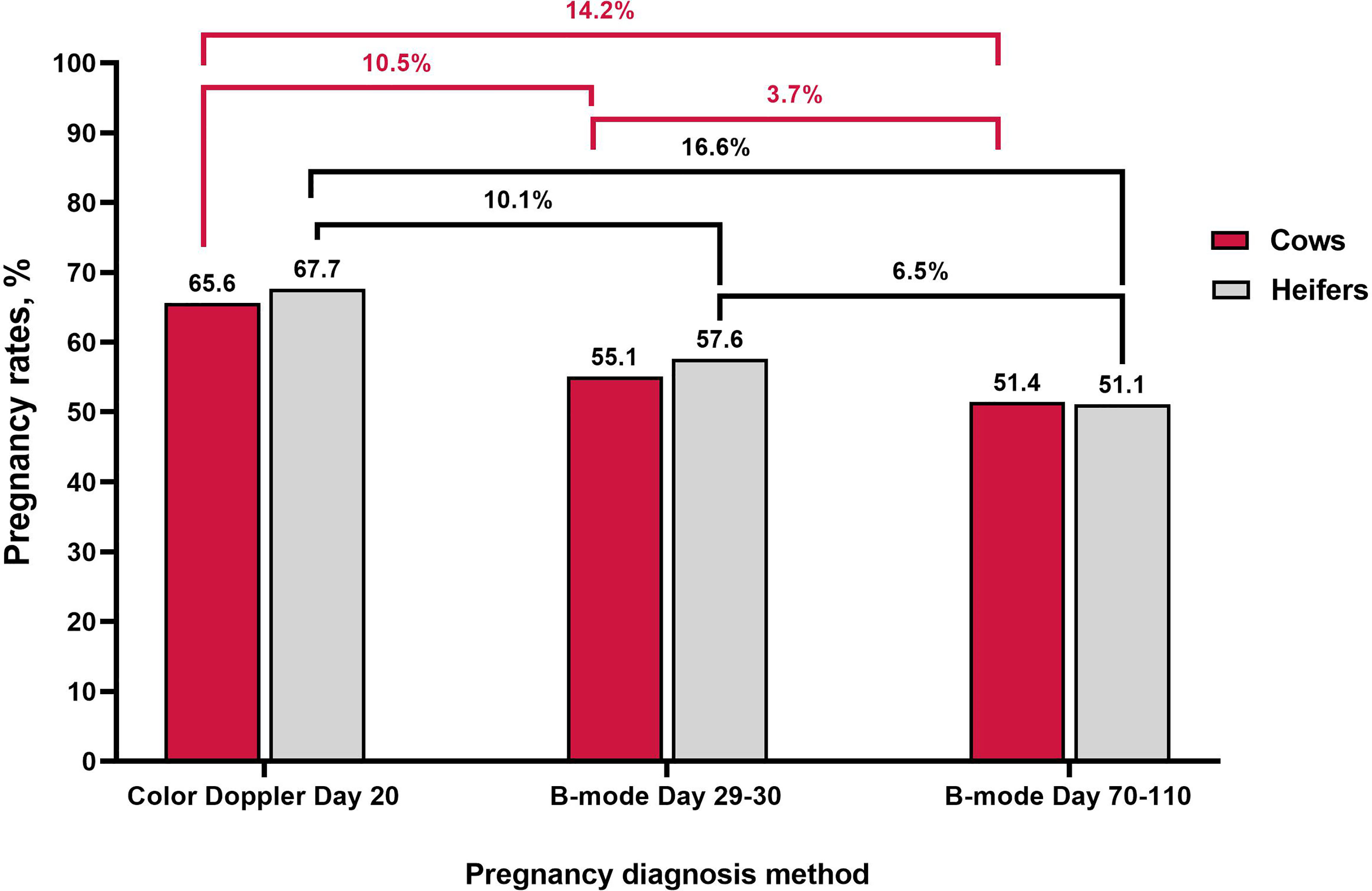
Figure 2 Changes in the percentage of pregnancies during early gestation using luteal color Doppler ultrasonography on day 20 and B-mode ultrasonography on days 29-30 and 70-110. Data adapted from Melo et al., 2020 and Holton et al., 2022a; Holton et al., 2022b using beef cows and heifers.
Luteal Color Doppler for Early Resynchronization
Resynchronization programs have been developed to maximize the proportion of pregnancies generated via reproductive technologies; however, the long interval between breeding events (approximately 30 to 40 days) of currently recommended resynchronization protocols limits their adoption by cattle producers. Particularly in the beef industry. This is largely due to the focus of beef cattle producers on generating pregnancies early in the breeding season. Replacement heifers that calve earlier in the calving season produce more pounds of calves during their productive life (Funston et al., 2012; Cushman et al., 2013) and have increased longevity in the herd (Cushman et al., 2013). Similarly, mature cows that calve earlier in the calving season produce older and heavier calves at weaning (Rodgers et al., 2012) and have increased pregnancy rates in the subsequent breeding season (Stevenson et al., 2015). Considering the importance of generating pregnancies early in the breeding season, novel resynchronization strategies that focus on maximizing the number of genetically superior pregnancies should also pursue a decrease in the interval between breeding events.
Pregnancy diagnosis during resynchronization is typically performed with conventional B-mode ultrasonography no earlier than day 28 of gestation. Because color Doppler can be used to recognize non-pregnant females undergoing CL regression as early as 20 or 22 days of gestation (Pugliesi et al., 2014; Scully et al., 2015; Holton et al., 2022a), there are opportunities to incorporate its use during resynchronization and reduce the interval between TAI or FTET events. In the context of TAI, resynchronization can be initiated approximately a week earlier on days 12 to 15 after the first insemination. Research on early resynchronization for TAI has primarily been performed in South America using estradiol-based synchronization protocols to synchronize the emergence of a new follicular wave (Bo et al., 1995; Palhão et al., 2020; Motta et al., 2020; Andrade et al., 2020). Estradiol-based products (e.g., estradiol benzoate and estradiol cypionate) are used in combination with progesterone to induce follicular atresia, resulting in a synchronized emergence of the subsequent follicular wave. Nevertheless, because estradiol is not approved for use in bovine estrus synchronization protocols in the United States, producers need to rely on GnRH to synchronize follicular waves. Analogues of GnRH are used to synchronize follicular waves by inducing ovulation of dominant follicles (Thatcher et al., 1993); however, because ovulation leads to the formation of a new CL, the use of GnRH analogues during resynchronization may lead to decreased accuracy of pregnancy diagnosis when color Doppler is utilized between days 20 and 22 of gestation. In Bos indicus cows, early resynchronization was successfully initiated between 12 to 14 days after TAI with pregnancy diagnosis performed via color Doppler on days 20 to 22 of gestation (Pugliesi et al., 2019; Palhão et al., 2020; Silva et al., 2022). In these studies, suitable conception rates were achieved for both the first and second TAI, and overall, more females were pregnant to TAI within the breeding season (Palhão et al., 2020). Early estradiol-based resynchronization in Bos indicus beef heifers has also proven successful when initiated 12 (Andrade et al., 2020) or 14 days (Motta et al., 2020) after TAI with pregnancy diagnosis performed with color Doppler on days 21 or 22 of gestation, respectively. Interestingly, estradiol benzoate dosage appears to influence pregnancy establishment to the first TAI in females exposed to early resynchronization in combination with color Doppler ultrasonography. Silva et al. (2022) compared 1 vs. 2 mg of estradiol benzoate 14 days after TAI in Bos indicus beef cows exposed to early resynchronization with color Doppler. Cows receiving 2 mg of estradiol benzoate had decreased pregnancy rates to the first TAI compared with cows receiving 1 mg (Silva et al., 2022). While there were no differences in pregnancy rates to the second TAI, cumulative pregnancy rates to TAI were greater in cows receiving a lower dose of estradiol benzoate.
Although research has been performed on early resynchronization, modified early resynchronization strategies are required in the United States to avoid reduced accuracy of color Doppler pregnancy diagnosis after GnRH administration and new CL formation. The requirement of GnRH at CIDR insertion has been a topic of debate in short-term estrus synchronization protocols, particularly protocols with only 5 days of progesterone exposure (Rabaglino et al., 2010; Kasimanickam et al., 2014; Williams and Stanko, 2020). Omittance of the initial GnRH is particularly relevant in replacement heifers, since their ovulatory response is decreased in comparison to postpartum cows (Cruppe et al., 2014) and pregnancy rates did not differ between heifers that receive the initial GnRH at CIDR insertion and those that did not (Lima et al., 2011; Cruppe et al., 2014). Similar results were observed on Bos indicus-influenced postpartum beef cows synchronized with 5 days of progesterone exposure and without GnRH to synchronize follicular wave emergence (Williams and Stanko, 2020). Therefore, the removal of GnRH at CIDR insertion during early resynchronization may yield acceptable pregnancy rates to AI while allowing for accurate color Doppler pregnancy diagnosis. It is, however, important to acknowledge that when GnRH is omitted a certain small percentage of females may develop persistent follicles (Zimbelman and Smith, 1966; Sirois and Fortune, 1990). Particularly females that have fewer follicular waves and lower circulating concentrations of progesterone during diestrus, such as lactating dairy cows and Bos taurus postpartum beef cows. In contrast with the development of persistent follicles, some females may undergo follicular atresia during the protocol when the initial GnRH is omitted, resulting in a delayed follicular wave emergence and ovulation of a smaller follicle in response to the GnRH administered at TAI.
Research on early resynchronization without estradiol or GnRH has been performed in Bos indicus (Pugliesi et al., 2019; Ataide Junior et al., 2021) and Bos indicus × Bos taurus beef females (Vieira et al., 2021). Pugliesi and colleagues utilized an injectable form of progesterone to resynchronize lactating Nelore cows beginning 12 days after the initial TAI. In this study, all cows received a new intravaginal progesterone releasing device on day 12; however, those which received a concurrent injection of a long-acting progesterone had greater pregnancy rates to the second TAI (Pugliesi et al., 2019). Further research into the use of progesterone for resynchronization reported no differences in the day of follicular wave emergence between females that only received an intravaginal progesterone device compared to those that also received the injection of progesterone (Ataide Junior et al., 2021). However, greater overall pregnancy rates to the second and third TAI were achieved when cows and heifers received an injection of long-acting progesterone in addition to an intravaginal device. Despite these positive findings, when long-acting progesterone was compared with the use of estradiol at intravaginal progesterone device insertion 14 days after TAI, crossbred beef heifers that received estradiol had greater pregnancy rates to resynchronization when compared with those that received the injection of progesterone (Vieira et al., 2021). These studies provide insight into potential early resynchronization strategies for Bos taurus females in the United States without the use of GnRH at CIDR insertion. Nevertheless, further research is required to evaluate the fertility of cows and heifers exposed to early GnRH-based estrus synchronization and resynchronization with or without the use of GnRH at CIDR insertion.
Early pregnancy diagnosis with color Doppler can also be performed successfully two weeks after ET and therefore, the period between FTET events can be reduced (Guimarães et al., 2015). Early estradiol-based resynchronization of FTET has been performed effectively in suckled Bos indicus cows and has achieved suitable pregnancy rates (Pugliesi et al., 2018). In this study, resynchronization was initiated 6 days after the original FTET, on day 13 of gestation, and all cows received a new intravaginal progesterone releasing device and a 100-mg injection of progesterone. Pregnancy diagnosis was performed via color Doppler ultrasonography at progesterone device removal on day 22 of gestation and all non-pregnant females (48.3%) were administered estradiol cypionate and prostaglandin F2α to induce ovulation and luteolysis, respectively. On day 31 of gestation, non-pregnant cows that successfully formed a new CL received a new embryo and thus, the interval between FTET events was reduced from 32 days to 24 days (Pugliesi et al., 2018).
The presence of a persistent follicle is of less concern in ET recipients than in females that will be exposed to TAI, since recipients do not contribute an oocyte to embryo formation. For that reason, resynchronization in combination with early color Doppler pregnancy diagnosis represents a potential alternative to optimize ET recipient utilization even in countries where the use of estradiol products is limited. Nevertheless, research on early resynchronization for FTET without the use of estradiol in Bos taurus females is required to establish recommendations for the commercial application of this technology in these countries.
Color Doppler as a Tool for Embryo Recipient Selection
Establishment of pregnancy after ET depends greatly on the quality of the embryo but also on the uterine environment provided by the recipient female, as successful maternal recognition of pregnancy depends on appropriate maternal progesterone secretion by the CL (Mann and Lamming, 2001). Embryo recipient candidates are normally scanned with a B-mode ultrasound to determine the presence and morphology of the CL before ET; however, these variables are not necessarily suitable indicators of CL function or pregnancy success (Siqueira et al., 2009; Herzog et al., 2010).
Color Doppler ultrasonography has been used to evaluate the blood perfusion of a CL and to make inferences on its functionality prior to ET (Pinaffi et al., 2015; Kanazawa et al., 2016; Pugliesi et al., 2019). Pinaffi et al. (2015) retrospectively categorized suckled Bos indicus cows into low (0 to 40%) or high (41 to 100%) CL blood perfusion groups after color Doppler ultrasonography. Corpus luteum diameter at the time of ET did not differ between groups; however, pregnancy rates to ET were greater in cows classified as having high CL blood perfusion. In lactating dairy cows, CL blood perfusion area was assessed using color Doppler ultrasonography and was 1.4 times greater on the day of ET in cows retrospectively categorized as pregnant than in those categorized as non-pregnant (Kanazawa et al., 2016). In the same study, no differences in luteal area or plasma progesterone concentrations were determined between pregnant and non-pregnant cows. Similar results were observed in FTET settings, where CL area and pixelation evaluated by B-mode ultrasonography were poor predictors of pregnancy outcomes. More recently, crossbred recipient cows were retrospectively categorized into three subgroups based on CL area (small: < 3 cm2; medium: 3 to 4 cm2; large: > 4 cm2) and CL blood perfusion (low: ≤ 40%; medium: 45 to 50%; high: ≥ 55%; Pugliesi et al., 2019). Pregnancy rates were greater in recipients classified as having high blood perfusion when compared with those in the low blood perfusion category (58.4 vs. 45.9%) and a positive linear correlation was determined between luteal blood perfusion and the probability of pregnancy. In the same study, no differences were reported in pregnancy rates or in serum concentrations of progesterone among females in different CL area classifications, yet a quadratic relationship was reported between luteal area and the probability of pregnancy (Pugliesi et al., 2019).
Progesterone during late metestrus and early diestrus plays an important role in pregnancy establishment and elevated circulating concentrations of progesterone during the first week after ovulation is associated with enhanced conceptus development (Forde et al., 2009; O’Hara et al., 2014) and pregnancy rates (Perry et al., 2005; Rodrigues et al., 2018). Nevertheless, the relationship between circulating concentrations of progesterone at the time of ET and pregnancy success remains unclear as previous studies have reported both a positive linear and quadratic relationships between progesterone concentrations and pregnancy rates (Perry et al., 2005; Peres et al., 2009; Rodrigues et al., 2018; Pereira et al., 2016). When the use of circulating concentrations of progesterone for recipient selection was evaluated, studies reported limited value in both beef and dairy cattle (Silva et al., 2002; Siqueira et al., 2009), indicating that luteal blood perfusion is a more suitable indicator of pregnancy maintenance to ET than luteal area or circulating concentrations of progesterone when selecting suitable recipients because of its linear relationship with pregnancy success (Pugliesi et al., 2019). Interestingly, the correlation between luteal area and circulating concentrations of progesterone was reported to be greater during early diestrus than the correlation between circulating concentrations of progesterone and luteal blood perfusion (Rocha et al., 2019). Therefore, luteal blood perfusion during early diestrus might be associated with other unknown factors regulating luteal function and pregnancy establishment beyond simply circulating concentrations of progesterone.
Collectively, currently available data indicates that evaluating luteal blood perfusion at the time of ET allows for better embryo recipient selection. Opportunities for color Doppler luteal evaluation exist to assign more valuable embryos to recipients with greater blood perfusion, as well as to recognize embryo recipients that are less likely to conceive based on decreased luteal blood perfusion. The use of the latter will depend on recipient availability and recipient setup cost. Pugliesi et al. (2019) reported that 30.4% of the recipients enrolled in their study were classified as having low blood perfusion (≤ 40% of the luteal area with blood perfusion signals). Although pregnancy rates are decreased in low compared with high blood perfusion recipients, results were acceptable in this specific group of cows. Hence, in scenarios where recipient availability is limited, practitioners benefit from also transferring embryos to recipients with low luteal blood perfusion. Yet, in scenarios where recipient availability is not limited, embryo practitioners can benefit from avoiding recipients with decreased luteal blood perfusion and consequently decreased pregnancy rates.
Conclusion
Doppler ultrasonography is a non-invasive method to quantify blood perfusion and infer functionality of reproductive organs and structures. By assessing functionality of a CL at ET, more suitable embryo recipients can be selected, and pregnancy rates to ET can be increased. Blood perfusion of the CL can also be used to identify non-pregnant females for very early pregnancy diagnosis on days 20 and 22 of gestation, which can be used to reduce the interval between insemination or ET events during early resynchronization. These applications create opportunities for color Doppler to be used as a tool to improve reproductive efficiency in beef production systems. Expanding opportunities for large animal veterinarian training will also be paramount for the successful adoption of luteal color Doppler examinations for both resynchronization and embryo transfer purposes. Luteal blood perfusion can be underestimated as a result of improper probe positioning, limited ultrasound equipment capabilities or inadequate settings, and excessive pressure against the ovary during probe positioning, which can lead to vasoconstriction. Therefore, adequate veterinarian training and standardization of evaluation procedures should increase the replicability and accuracy of the color Doppler examinations, which may further increase the uptake of this technology in the cattle industry.
Author Contributions
Both authors contributed equally to conceptualization and writing.
Conflict of Interest
The authors declare that the research was conducted in the absence of any commercial or financial relationships that could be construed as a potential conflict of interest.
Publisher’s Note
All claims expressed in this article are solely those of the authors and do not necessarily represent those of their affiliated organizations, or those of the publisher, the editors and the reviewers. Any product that may be evaluated in this article, or claim that may be made by its manufacturer, is not guaranteed or endorsed by the publisher.
References
Acosta T. J., Hayashi K.-G., Matsui M., Miyamoto A. (2005). Changes in Follicular Vascularity During the First Follicular Wave in Lactating Cows. J Reprod Dev 51 (2), 273–280. doi: 10.1262/jrd.16092
Andrade J. P. N., Gomez-León V. E., Andrade F. S., Carvalho B. P., Lacouth K. L., Garcia F. Z., et al. (2020). Development of a Novel 21-Day Reinsemination Program, ReBreed21, in Bos Indicus Heifers. Theriogenology 155, 125–131. doi: 10.1016/j.theriogenology.2020.04.021
Assey R., Purwantara B., Greve T., Hyttell P., Schmidt M. H. (1993). Corpus Luteum Size and Plasma Progesterone Levels in Cattle After Cloprostenol-Induced Luteolysis. Theriogenology 39, 1321–1330. doi: 10.1016/0093-691X(93)90234-V
Ataide Junior G. A., Kloster A., de Moraes É.G., Motta I. G., Claro Junior I., Vasconcelos J. L. M., et al. (2021). Early Resynchronization of Follicular Wave Emergence Among Nelore Cattle Using Injectable and Intravaginal Progesterone for Three Timed Artificial Inseminations. Anim. Reprod. Sci. 229, 106759. doi: 10.1016/j.anireprosci.2021.106759
Bazer F. W., Burghardt R. C., Johnson G. A., Spencer T. E., Wu G. (2008). Interferons and Progesterone for Establishment and Maintenance of Pregnancy: Interactions Among Novel Cell Signaling Pathways. Reprod. Biol. 8, 179–211. doi: 10.1016/S1642-431X(12)60012-6
Berger H., Lietzau M., Tichy A., Herzog K. (2017). Pregnancy Outcome is Influenced by Luteal Area During Diestrus Before Successful Insemination But Not by Milk Production Level. Theriogenology 104, 115–119. doi: 10.1016/j.theriogenology.2017.04.020
Betteridge K. J., Flechon J. E. (1988). The Anatomy and Physiology of Pre-Attachment Bovine Embryos. Theriogenology 29, 155–187. doi: 10.1016/0093-691X(88)90038-6
Bisinotto R. S., Ribeiro E. S., Santos J. E. P. (2014). Synchronisation of Ovulation for Management of Reproduction in Dairy Cows. Animal 8, 151–159. doi: 10.1017/S1751731114000858
Bo G. A., Adams G. P., Caccia M., Martinez M., Pierson R. A., Mapletoft R. J. (1995). Ovarian Follicular Wave Emergence After Treatment With Progestogen and Estradiol in Cattle. Anim. Reprod. Sci. 39, 193–204. doi: 10.1016/0378-4320(95)01389-H
Bollwein H., Baumgartner U., Stolla R. (2002). Transrectal Doppler Sonography of Uterine Blood Flow in Cows During Pregnancy. Theriogenology 57, 2053–2061. doi: 10.1016/s0093-691x(02)00706-9
Bollwein H., Heppelmann M., Lüttgenau J. (2016). Ultrasonographic Doppler Use for Female Reproduction Management. Veterinary Clinics North America Food Anim. Pract. 32, 149–164. doi: 10.1016/j.cvfa.2015.09.005
Bollwein H., Meyer H. H. D., Maierl J., Weber F., Baumgartner U., Stolla R. (2000). Transrectal Doppler Sonography of Uterine Blood Flow in Cows During the Estrous Cycle. Theriogenology 53, 1552–2000. doi: 10.1016/s0093-691x(00)00296-x
Brockus K. E., Hart C. G., Gilfeather C. L., Fleming B. O., Lemley C. O. (2016). Dietary Melatonin Alters Uterine Artery Hemodynamics in Pregnant Holstein Heifers. Domest. Anim. Endocrinol. 55, 1–10. doi: 10.1016/j.domaniend.2015.10.006
Camacho L. E., Lemley C. O., Prezotto L. D., Bauer M. L., Freetly H. C., Swanson K. C., et al. (2014). Effects of Maternal Nutrient Restriction Followed by Realimentation During Midgestation on Uterine Blood Flow in Beef Cows. Theriogenology 81, 1248–1256. doi: 10.1016/j.theriogenology.2014.02.006
Chui D. K. C., Pugh N. D., Walker S. M., Gregory L., Shaw R. W. (1997). Follicular Vascularity-the Predictive Value of Transvaginal Power Doppler Ultrasonography in an in-Vitro Fertilization Programme: A Preliminary Study. Human Reproduction 12 (1), 191–196. doi: 10.1093/humrep/12.1.191
Coulam C. B., Goodman C., Rinehart J. S. (1999). Colour Doppler Indices of Follicular Blood Flow as Predictors of Pregnancy After in-Vitro Fertilization and Embryo Transfer. Human Reproduction 14 (8), 1979–82. doi: 10.1093/humrep/14.8.1979
Cruppe L. H., Day M. L., Abreu F. M., Biehl M. V., Cipriano R. S., Mussard M. L., et al. (2014). The Requirement of GnRH at the Beginning of the Five-Day CO-Synch + Controlled Internal Drug Release Protocol in Beef Heifers. J. Anim. Sci. 92, 4198–4203. doi: 10.2527/jas.2014-7772
Cushman R. A., Kill L. K., Funston R. N., Mousel E. M., Perry G. A. (2013). Heifer Calving Date Positively Influences Calf Weaning Weights Through Six Parturitions. J. Anim. Sci. 91, 4486–4491. doi: 10.2527/jas2013-6465
Dalmaso de Melo G., Mello B. P., Ferreira C. A., Souto Godoy Filho C. A., Rocha C. S., Silva A. G. (2020). Applied use of interferon-tau stimulated genes expression in polymorphonuclear cells to detect pregnancy compared to other early predictors in beef cattle. Theriogenology 152, 94–105. doi: 10.1016/j.theriogenology.2020.04.001
de Tarso S. G. S., Gastal G. D. A., Bashir S. T., Gastal M. O., Apgar G. A., Gastal E. L. (2017). Follicle Vascularity Coordinates Corpus Luteum Blood Flow and Progesterone Production. Reprod. Fertil. Dev. 29, 448–457. doi: 10.1071/RD15223
Fontes P. L. P., Oosthuizen N., Ciriaco F. M., Sanford C. D., Canal L. B., Pohler K. G., et al. (2019). Impact of Fetal vs. Maternal Contributions of Bos Indicus and Bos Taurus Genetics on Embryonic and Fetal Development. J. Anim. Sci. 97, 1645–1655. doi: 10.1093/jas/skz044
Forde N., Carter F., Fair T., Crowe M. A., Evans A. C., Spencer T. E., et al. (2009). Progesterone-Regulated Changes in Endometrial Gene expression Contribute to Advanced Conceptus Development in Cattle. Biol. Reprod. 81, 784–794. doi: 10.1095/BIOLREPROD.108.074336
Fortune J. E., Sirois J., Quirck S. M. (1988). The Growth and Differentiation of Ovarian Follicles During the Bovine Estrous Cycle. Theriogenology 29, 95–109. doi: 10.1016/0093-691X(88)90034-9
Funston R. N., Musgrave J. A., Meyer T. L., Larson D. M. (2012). Effect of Calving Distribution on Beef Cattle Progeny Performance. J. Anim. Sci. 90, 5118–5121. doi: 10.2527/jas2012-5263
Gard Schnuelle J., Blythe E., Cole R., Taylor S., Alfaro G., Muntifering R., et al. (2021). Evaluation of Alterations in Uterine Blood Flow Using Doppler Ultrasonography in Pregnant, Genotyped Beef Cows and Heifers Consuming Endophyte-Infected Tall Fescue Seeds and Supplemented With Rumen-Protected Niacin. J. Veterinary Sci. Res. 6, 1–8. doi: 10.23880/oajvsr-16000207
Gifford C. A., Racicot K., Clark D. S., Austin K. J., Hansen T. R., Lucy M. C., et al. (2007). Regulation of Interferon-Stimulated Genes in Peripheral Blood Leukocytes in Pregnant and Bred, Nonpregnant Dairy Cows. J. Dairy Sci. 90, 274–280. doi: 10.3168/jds.S0022-0302(07)72628-0
Ginther O. J., Gastal E. L., Gastal M. O., Beg M. A. (2007a). Effect of Prostaglandin F2α on Ovarian, Adrenal, and Pituitary Hormones and on Luteal Blood Flow in Mares. Domest. Anim. Endocrinol. 32, 315–328. doi: 10.1016/j.domaniend.2006.04.006
Ginther O. J., Kastelic J. P., Knopf L. (1989). Composition and Characteristics of Follicular Waves During the Bovine Estrous Cycle. Animal Reproduction Science 20 (3), 187–200. doi: 10.1016/0378-4320(89)90084-5
Ginther O. J., Silva L. A., Araujo R. R., Beg M. A. (2007b). Temporal Associations Among Pulses of 13,14-Dihydro-15-Keto-PGF 2alpha, Luteal Blood Flow, and Luteolysis in Cattle. Biol. Reprod. 76, 506–513. doi: 10.1095/biolreprod.106.057653
Ginther O. J., Utt M. D. (2004). Doppler Ultrasound in Equine Reproduction: Principles, Techniques, and Potential. J. Equine Veterinary Sci. 24, 516–526. doi: 10.1016/j.jevs.2004.11.005
Grazul-Bilska A. T., Navanukraw C., Johnson M. L., Vonnahme K. A., Ford S. P., Reynolds L. P., et al. (2007). Vascularity and Expression of Angiogenic Factors in Bovine Dominant Follicles of the First Follicular Wave. J. Anim. Sci. 85, 1914–1922. doi: 10.2527/jas.2007-0044
Green J. C., Okamura C. S., Poock S. E., Lucy M. C. (2010). Measurement of Interferon-Tau (IFN-τ) Stimulated Gene Expression in Blood Leukocytes for Pregnancy Diagnosis Within 18-20d After Insemination in Dairy Cattle. Anim. Reprod. Sci. 121, 24–33. doi: 10.1016/j.anireprosci.2010.05.010
Guillomot M. (1995). Cellular Interactions During Implantation in Domestic Ruminants. J. Reprod. Fertil. Suppl. 49, 39–51.
Guimarães C. R. B., Oliveira M. E., Rossi J. R., Fernandes C. A. C., Viana J. H. M., Palhão M. P. (2015). Corpus Luteum Blood Flow Evaluation on Day 21 to Improve the Management of Embryo Recipient Herds. Theriogenology 84, 237–241. doi: 10.1016/j.theriogenology.2015.03.005
Heppelmann M., Krüger L., Leidl S., Bollwein H. (2013). Transrectal Doppler Sonography of Uterine Blood Flow During the First Two Weeks After Parturition in Simmental Heifers. J. Veterinary Sci. 14, 323–327. doi: 10.4142/jvs.2013.14.3.323
Herzog K., Brockhan-Lüdemann M., Kaske M., Beindorff N., Paul V., Niemann H., et al. (2010). Luteal Blood Flow is a More Appropriate Indicator for Luteal Function During the Bovine Estrous Cycle Than Luteal Size. Theriogenology 73, 691–697. doi: 10.1016/j.theriogenology.2009.11.016
Holton M. P., Dalmaso G., Dias N. W., Pancini S., Lamb G. C., Pohler K. G., et al. (2022b). Early Pregnancy Diagnosis in Bos Taurus Beef Replacement Heifers Using Color Doppler Ultrasonography. J. Anim. Sci. 100, 12–13. doi: 10.1093/jas/skac028.023
Holton M. P., Oosthuizen N., Melo G.D., Davis D. B., Stewart R. L., Pohler K. G., et al. (2022a). Luteal Color Doppler Ultrasonography and Pregnancy-Associated Glycoproteins as Early Pregnancy Diagnostic Tools and Predictors of Pregnancy Loss in Bos Taurus Postpartum Beef Cows. J. Anim. Sci. 100. doi: 10.1093/jas/skac018
Honnens A., Voss C., Herzog K., Niemann H., Rath D., Bollwein H. (2008). Uterine Blood Flow During the First 3 Weeks of Pregnancy in Dairy Cows. Theriogenology 70, 1048–1056. doi: 10.1016/j.theriogenology.2008.06.022
Kanazawa T., Seki M., Ishiyama K., Kubo T., Kaneda Y., Sakaguchi M., et al. (2016). Pregnancy Prediction on the Day of Embryo Transfer (Day 7) and Day 14 by Measuring Luteal Blood Flow in Dairy Cows. Theriogenology 81, 797–804.
Kastelic J. P., Bergfelt D. R., Ginther O. J. (1990). Relationship Between Ultrasonic Assessment of the Corpus Luteum and Plasma Progesterone Concentration in Heifers. Theriogenology 33, 1269–1278. doi: 10.1016/0093-691X(90)90045-U
Kasimanickam R. K., Firth G., Schuenemann B. K., Whitlock J. M., Gay D. A., Moore J. B., et al. (2014). Effect of the First GnRH and Two Doses of PGF2α in a 5-Day Progesteronebased CO-Synch Protocol on Heifer Pregnancy. Theriogenology 81, 797–804.
Lamb G. C., Mercadante V. R. G., Henry D. D., Fontes P. L. P., Dahlen C. R., Larson J. E., et al. (2016). Advantages of Current and Future Reproductive Technologies for Beef Cattle Production. Prof. Anim. Sci. 32, 162–171. doi: 10.15232/pas.2015-01455
Lemley C. O. (2017). Investigating Reproductive Organ Blood Flow and Blood Perfusion to Ensure Healthy Offspring. Anim. Front. 7, 18–24. doi: 10.2527/af.2017-0124
Lemley C. O., Hart C. G., Lemire R. L., King E. H., Hopper R. M., et al. (2018). Maternal Nutrient Restriction Alters Uterine Artery Hemodynamics and Placentome Vascular Density in Bos Indicus and Bos Taurus. J. Anim. Sci. 96 (11), 4823–4834. doi: 10.1093/jas/sky329
Lima F. S., Ayres H., Favoreto M. G., Bisinotto R. S., Greco L. F., Ribeiro E. S., et al. (2011). Effects of Gonadotropin-Releasing Hormone at Initiation of the 5-D Timed Artificial Insemination (AI) Program and Timing of Induction of Ovulation Relative to AI on Ovarian Dynamics and Fertility of Dairy Heifers. J. Dairy Sci. 94, 4997–5004. doi: 10.3168/jds.2011-4240
Mann G., Lamming G. (2001). Relationship Between Maternal Endocrine Environment, Early Embryo Development and Inhibition of the Luteolytic Mechanism in Cows. Reproduction 121, 175–180. doi: 10.1530/rep.0.1210175
McCarty K. J., Owen M. P. T., Hart C. G., Thompson R. C., Burnett D. D., King E. H., et al. (2018). Effect of Chronic Melatonin Supplementation During Mid to Late Gestation on Maternal Uterine Artery Blood Flow and Subsequent Development of Male Offspring in Beef Cattle. J. Anim. Sci. 96, 5100–5111. doi: 10.1093/jas/sky363
Melo G. D., Mello B. P., Ferreira C. A., Souto Godoy Filho C. A., Rocha C. C., Silva A. G., et al. (2020). Applied Use of Interferon-Tau Stimulated Genes Expression in Polymorphonuclear Cells to Detect Pregnancy Compared to Other Early Predictors in Beef Cattle. Theriogenology 152, 94–105. doi: 10.1016/j.theriogenology.2020.04.001
Mesquita F. S., Pugliesi G., Scolari S. C., França M. R., Ramos R. S., Oliveira M., et al. (2014). Manipulation of the Periovulatory Sex Steroidal Milieu Affects Endometrial But Not Luteal Gene Expression in Early Diestrus Nelore Cows. Theriogenology 81, 861–869. doi: 10.1016/j.theriogenology.2013.12.022
Miyamoto A., Shirasuna K., Hayashi K.-G., Kamada D., Kawashima C., Kaneko E., et al. (2006). A Potential Use of Color Ultrasound as a Tool for Reproductive Management: New Observations Using Color Ultrasound Scanning That Were Not Possible With Imaging Only in Black and White. J Reprod Dev. 52 (1), 153–160. doi: 10.1262/jrd.17087
Miyamoto A., Shirasuna K., Wijayagunawardane M. P. B., Watanabe S., Hayashi M., Yamamoto D., et al. (2005). Blood Flow: A Key Regulatory Component of Corpus Luteum Function in the Cow. Domest. Anim. Endocrinol. 29, 329–339. doi: 10.1016/j.domaniend.2005.03.011
Motta I. G., Rocha C. C., Bisinotto D. Z., Melo G. D., Ataide Júnior G. A., Silva A. G., et al. (2020). Increased Pregnancy Rate in Beef Heifers Resynchronized With Estradiol at 14 Days After TAI. Theriogenology 147, 62–70. doi: 10.1016/j.theriogenology.2020.02.009
Nation D. P., Malmo J., Davis G. M., Macmillan K. L. (2003). Accuracy of Bovine Pregnancy Detection Using Transrectal Ultrasonography at 28 to 35 Days After Insemination. Aust. Veterinary J. 81, 63–65. doi: 10.1111/j.1751-0813.2003.tb11435.x
Niswender G. D., Juengel J. L., Mcguire W. J., Belfiore C. J., Wiltbank M. C. (1994). Luteal Function: The Estrous Cycle and Early Pregnancy. Biol. Reprod. 50, 239–247. doi: 10.1095/biolreprod50.2.239
O’Hara L., Forde N., Duffy P., Randi F., Kelly A. K., Valenza A., et al. (2014). Effect of Combined Exogenous Progesterone With Luteotrophic Support via Equine Chorionic Gonadotrophin (eCG) on Corpus Luteum Development, Circulating Progesterone Concentrations and Embryo Development in Cattle. Reproduction, Fertility and Development 28, 269–277. . doi: doi: 10.1071/RD14019
Palhão M. P., Ribeiro A. C., Martins A. B., Guimarães C. R. B., Alvarez R. D., Seber M. F., et al. (2020). Early Resynchronization of non-Pregnant Beef Cows Based in Corpus Luteum Blood Flow Evaluation 21 Days After Timed-AI. Theriogenology 146, 26–30. doi: 10.1016/j.theriogenology.2020.01.064
Panarace M., Garnil C., Marfil M., Jauregui G., Lagioia J., Luther E., et al. (2006). Transrectal Doppler Sonography for Evaluation of Uterine Blood Flow Throughout Pregnancy in 13 Cows. Theriogenology 66, 2113–2119. doi: 10.1016/j.theriogenology.2006.03.040
Pancarci Ş.M., Ari U.Ç., Atakisi O., Güngör Ö., Çiĝremiş Y., Bollwein H. (2012). Nitric Oxide Concentrations, Estradiol-17β Progesterone Ratio in Follicular Fluid, and COC Quality With Respect to Perifollicular Blood Flow in Cows. Anim. Reprod. Sci. 130, 9–15. doi: 10.1016/j.anireprosci.2011.12.013
Pereira M. H. C., Wiltbank M. C., Vasconcelos J. L. M. (2016). Expression of Estrus Improves Fertility and Decreases Pregnancy Losses in Lactating Dairy Cows That Receive Artificial Insemination or Embryo Transfer. J Dairy Sci. 99 (3), 2237–2247. doi: 10.3168/jds.2015-9903
Perry G. A., Smith M. F., Lucy M. C., Green J. A., Parks T. E., MacNeil M. D., et al. (2005). Relationship Between Follicle Size at Insemination and Pregnancy Success. Proc. Natl. Acad. Sci. 102, 5268–5273. doi: 10.1073/pnas.0501700102
Perry G. A., Smith M. F., Roberts A. J., MacNeil M. D., Geary T. W. (2007). Relationship Between Size of the Ovulatory Follicle and Pregnancy Success in Beef Heifers. J. Anim. Sci. 85, 684–689. doi: 10.2527/jas.2006-519
Perry G. A., Swanson O. L., Larimore E. L., Perry B. L., Djira G. D., Cushman R. A. (2014). Relationship of Follicle Size and Concentrations of Estradiol Among Cows Exhibiting or Not Exhibiting Estrus During a Fixed-Time AI Protocol. Domest. Anim. Endocrinol. 48, 15–20. doi: 10.1016/j.domaniend.2014.02.001
Peres R. F., Claro I Jr, Sá Filho O. G., Nogueira G. P., Vasconcelos J. L. (2009). Strategies to Improve Fertility in Bos Indicus Postpubertal Heifers and Nonlactating Cows Submitted to Fixed-Time Artificial Insemination. Theriogenology 72 (5), 681–689. doi: 10.1016/j.theriogenology.2009.04.026
Peter A. T. (2013). Bovine Placenta: A Review on Morphology, Components, and Defects From Terminology and Clinical Perspectives. Theriogenology 80, 693–705. doi: 10.1016/j.theriogenology.2013.06.004
Pierson R. A., Ginther O. J. (1984). Ultrasonography for Detection of Study of Embryonic Development Theriogenology Pregnancy and in Heifers. Theriogenology 22, 225–233. doi: 10.1016/0093-691x(84)90435-7
Pierson R. A., Kastelic J. P., Ginther O. J. (1988). Basic Principals and Techniques for Transrectal Ultrasonography in Cattle and Horses. Theriogenology 29, 3–20. doi: 10.1016/0093-691X(88)90028-3
Pinaffi F. L. V., Santos É. S., da Silva M. G., Filho M. M., Madureira E. H., Silva L. A. (2015). Follicle and Corpus Luteum Size and Vascularity as Predictors of Fertility at the Time of Artificial Insemination and Embryo Transfer in Beef Cattle1. Pesquisa Veterinaria Bras. 35, 470–476. doi: 10.1590/S0100-736X2015000500015
Pugliesi G., Bisinotto D. Z., Mello B. P., Lahr F. C., Ferreira C. A., et al. (2019). A Novel Strategy for Resynchronization of Ovulation in Nelore Cows Using Injectable Progesterone (P4) and P4 Releasing Devices to Perform Two Timed Inseminations Within 22 Days. Reprod. Dom. Anim. 54, 1149–1154. doi: 10.1111/rda.13475
Pugliesi G., Dalmaso de Melo G., Silva J. B., Carvalhêdo A. S., Lopes E., de Siqueira Filho E., et al. (2019). Use of Color-Doppler Ultrasonography for Selection of Recipients in Timed-Embryo Transfer Programs in Beef Cattle. Theriogenology 135, 73–79. doi: 10.1016/j.theriogenology.2019.06.006
Pugliesi G., de Melo G. D., Ataíde G. A., Pellegrino C. A. G., Silva J. B., Rocha C. C., et al. (2018). Use of Doppler Ultrasonography in Embryo Transfer Programs: Feasibility and Field Results. Anim. Reprod. 15, 239–246. doi: 10.21451/1984-3143-AR2018-0059
Pugliesi G., Miagawa B. T., Paiva Y. N., França M. R., Silva L. A., Binelli M. (2014). Conceptus-Induced Changes in the Gene Expression of Blood Immune Cells and the Ultrasound-Accessed Luteal Function in Beef Cattle: How Early can We Detect Pregnancy? Biol. Reprod. 91 (4), 1–12. doi: 10.1095/biolreprod.114.121525
Pugliesi G., Santos F. B., Lopes E., Nogueira É., Maio J. R. G., Binelli M. (2016). Improved Fertility in Suckled Beef Cows Ovulating Large Follicles or Supplemented With Long-Acting Progesterone After Timed-AI. Theriogenology 85, 1239–1248. doi: 10.1016/j.theriogenology.2015.12.006
Rabaglino M. B., Risco C. A., Thatcher M.-J., Kim I. H., Santos J. E. P., Thatcher W. W. (2010). Application of One Injection of Prostaglandin F(2alpha) in the Five-Day Co-Synch+CIDR Protocol for Estrous Synchronization and Resynchronization of Dairy Heifers. J. Dairy Sci. 93, 1050–1058. doi: 10.3168/jds.2009-2675
Reese S. T., Franco G. A., Poole R. K., Hood R., Fernadez Montero L., Oliveira Filho R. V., et al. (2020). Pregnancy Loss in Beef Cattle: A Meta-Analysis. Anim. Reprod. Sci. 212, 106251. doi: 10.1016/j.anireprosci.2019.106251
Reynolds L. P., Redmer D. A. (1995). Utero-Placental Vascular Development and Placental Function. J. Anim. Sci. 73, 1839–1851. doi: 10.2527/1995.7361839x
Rocha C. C., Martins T., Cardoso B. O., Silva L. A., Binelli M., Pugliesi G. (2019). Ultrasonography-Accessed Luteal Size Endpoint That Most Closely Associates With Circulating Progesterone During the Estrous Cycle and Early Pregnancy in Beef Cows. Anim. Reprod. Sci. 201, 12–21. doi: 10.1016/j.anireprosci.2018.12.003
Rodgers J. C., Bird S. L., Larson J. E., Dilorenzo N., Dahlen C. R., Dicostanzo A., et al. (2012). An Economic Evaluation of Estrous Synchronization and Timed Artificial Insemination in Suckled Beef Cows. J. Anim. Sci. 90, 4055–4062. doi: 10.2527/jas.2011-4836
Rodrigues A. D., Cooke R. F., Cipriano R. S., Silva L. G. T., Cerri R. L. A., Cruppe L. H., et al. (2018). Impacts of Estrus Expression and Intensity During a Timed-AI Protocol on Variables Associated With Fertility and Pregnancy Success in Bos Indicus-Influenced Beef Cows. J. Anim. Sci. 96, 236–249. doi: 10.1093/jas/skx043
Sartori R., Barros C. M. (2011). Reproductive Cycles in Bos Indicus Cattle. Anim. Reprod. Sci. 124, 244–250. doi: 10.1016/j.anireprosci.2011.02.006
Sartori R., Gimenes L. U., Monteiro P. L. J., Melo L. F., Baruselli P. S., Bastos M. R. (2016). Metabolic and Endocrine Differences Between Bos Taurus and Bos Indicus Females That Impact the Interaction of Nutrition With Reproduction. Theriogenology 86, 32–40. doi: 10.1016/j.theriogenology.2016.04.016
Schlafer D. H., Fisher P. J., Davies C. J. (2000). The Bovine Placenta Before and After Birth: Placental Development and Function in Health and Disease. Anim. Reprod. Sci. 60–61, 145–160. doi: 10.1016/s0378-4320(00)00132-9
Scully S., Evans A. C. O., Carter F., Duffy P., Lonergan P., Crowe M. A. (2015). Ultrasound Monitoring of Blood Flow and Echotexture of the Corpus Luteum and Uterus During Early Pregnancy of Beef Heifers. Theriogenology 83, 449–458. doi: 10.1016/j.theriogenology.2014.10.009
Sharma I., Singh M., Sharma A., Kumar P., Dogra P. K. (2021). Doppler Sonography for Evaluation of Haemodynamic Changes of Uterine Arteries and Umbilicus During Different Months of Gestation in Dairy Cows. Veterinarija Zootech. 79, 52–58.
Siddiqui M. A. R., Almamun M., Ginther O. J. (2009a). Blood Flow in the Wall of the Preovulatory Follicle and its Relationship to Pregnancy Establishment in Heifers. Anim. Reprod. Sci. 113, 287–292. doi: 10.1016/j.anireprosci.2008.07.008
Siddiqui M. A. R., Gastal E. L., Gastal M. O., Almamun M., Beg M. A., Ginther O. J. (2009b). Relationship of Vascular Perfusion of the Wall of the Preovulatory Follicle to In Vitro Fertilisation and Embryo Development in Heifers. Reproduction 137, 689–697. doi: 10.1530/REP-08-0403
Silva J., Costa L. L., Robalo S. J. (2002). Plasma Progesterone Profiles and Factors Affecting Embryo-Fetal Mortality Following Embryo Transfer in Dairy Cattle. Theriogenology 58, 51–59. doi: 10.1016/S0093-691X(02)00906-8
Silva A. D. G., Nishimura TK, Rocha CC, Motta IG, Neto AL, Ferraz PA, et al. (2022). Comparison of Estradiol Benzoate Doses for Resynchronization of Ovulation at 14 Days After Timed-AI in Suckled Beef Cows. Theriogenology 184, 41–50. doi: 10.1016/j.theriogenology.2022.02.025
Silva L. A., Ginther O. J. (2010). Local Effect of the Conceptus on Uterine Vascular Perfusion During Early Pregnancy in Heifers. Reproduction 139 (2), 453–463. doi: 10.1530/REP-09-0363
Siqueira L. G. B., Areas V. S., Ghetti A. M., Fonseca J. F., Palhão M. P., Fernandes C. A. C., et al. (2013). Color Doppler Flow Imaging for the Early Detection of Nonpregnant Cattle at 20 Days After Timed Artificial Insemination. J. Dairy Sci. 96, 6461–6472. doi: 10.3168/jds.2013-6814
Siqueira L. G. B., Torres C. A. A., Souza E. D., Monteiro P. L. J., Arashiro E. K. N., Camargo L. S. A., et al. (2009). Pregnancy Rates and Corpus Luteum-Related Factors Affecting Pregnancy Establishment in Bovine Recipients Synchronized for Fixed-Time Embryo Transfer. Theriogenology 72, 949–958. doi: 10.1016/j.theriogenology.2009.06.013
Sirois J., Fortune J. E. (1990). Lengthening the Bovine Estrous-Cycle With Low-Levels of Exogenous Progesterone - a Model for Studying Ovarian Follicular Dominance. Endocrinology 127, 916–925. doi: 10.1210/endo-127-2-916
Spell A. R., Beal W. E., Corah L. R., Lamb G. C. (2001). Evaluating Recipient and Embryo Factors That Affect Pregnancy Rates of Embryo Transfer in Beef Cattle. Theriogenology 56, 287–297. doi: 10.1016/s0093-691x(01)00563-5
Stevenson J., Hill S., Bridges G., Larson J., Lamb G. (2015). Progesterone Status, Parity, Body Condition, and Days Postpartum Before Estrus or Ovulation Synchronization in Suckled Beef Cattle Influence Artificial Insemination Pregnancy Outcomes. J. Anim. Sci. 93, 2111–2123. doi: 10.2527/jas.2014-8391
Thatcher W. W., Drost M., Savio J. D., Macmillan K. L., Entwistle K. W., Schmitt E. J., et al. (1993). New Clinical Uses of GnRH and its Analogues in Cattle. Anim. Reprod. Sci. 33, 27–49. doi: 10.1016/0378-4320(93)90105-Z
Utt M. D., Johnson G. L., Beal W. E.. (2009). The evaluation of corpus luteum blood flow using color-flow Doppler ultrasound for early pregnancy diagnosis in bovine embryo recipients. Theriogenology 71, 707–715. doi: 10.1016/j.theriogenology.2008.09.032
Velho G., dos S., Rovani M. T., Ferreira R., Gasperin B. G., Dalto A. G. C. (2021). Blood Perfusion and Diameter of Bovine Corpus Luteum as Predictors of Luteal Function in Early Pregnancy. Reprod. Domest. Anim. 00, 1–7. doi: 10.1111/rda.14046
Viana J. H. M., Arashiro E. K. N., Siqueira L. G. B., Ghetti A. M., Areas V. S., Guimarães C. R. B., et al. (2013). Doppler Ultrasonography as a Tool for Ovarian Management. Anim. Reprod. 10, 215–222.
Vieira C. C., Pinto H. F., Buss V., Gonzalez de Freitas B., Guerreiro B. M., Leivas F. G., et al. (2021). Resynchronization of Follicular Wave Using Long-Acting Injectable Progesterone or Estradiol Benzoate at 14 Days Post-Timed AI in Bos Taurus X Bos Indicus Beef Heifers. Theriogenology 176, 194–199. doi: 10.1016/j.theriogenology.2021.09.017
Williams G. L., Stanko R. L. (2020). Pregnancy Rates to Fixed-Time AI in Bos Indicus-Influenced Beef Cows Using PGF2α With (Bee Synch I) or Without (Bee Synch II) GnRH at the Onset of the 5-Day CO-Synch + CIDR Protocol. Theriogenology 142, 229–235. doi: 10.1016/j.theriogenology.2019.09.047
Wiltbank M. C., Baez G. M., Garcia-Guerra A., Toledo M. Z., Monteiro P. L. J., Melo L. F., et al. (2016). Pivotal Periods for Pregnancy Loss During the First Trimester of Gestation in Lactating Dairy Cows. Theriogenology 86, 239–253. doi: 10.1016/j.theriogenology.2016.04.037
Keywords: beef cattle, doppler ultrasonography, embryo transfer, pregnancy diagnosis, resynchronization
Citation: Fontes PLP and Oosthuizen N (2022) Applied Use of Doppler Ultrasonography in Bovine Reproduction. Front. Anim. Sci. 3:912854. doi: 10.3389/fanim.2022.912854
Received: 04 April 2022; Accepted: 10 June 2022;
Published: 07 July 2022.
Edited by:
Alan D Ealy, Virginia Tech, United StatesReviewed by:
Guilherme Pugliesi, University of São Paulo, BrazilM. Sofia Ortega, University of Missouri, United States
Copyright © 2022 Fontes and Oosthuizen. This is an open-access article distributed under the terms of the Creative Commons Attribution License (CC BY). The use, distribution or reproduction in other forums is permitted, provided the original author(s) and the copyright owner(s) are credited and that the original publication in this journal is cited, in accordance with accepted academic practice. No use, distribution or reproduction is permitted which does not comply with these terms.
*Correspondence: Pedro L. P. Fontes, pedrofontes@uga.edu