Erratum: Exposing Atlantic Salmon post-smolts to fluctuating sublethal nitrite concentrations in a commercial recirculating aquaculture system (RAS) may have negative consequences
- 1Fiskaaling, Við Áir 11, Hvalvík, Faroe Islands
- 2The Norwegian College of Fishery Science, Faculty of Biosciences, Fisheries and Economics, UiT, The Arctic University of Norway, Tromsø, Norway
Salmon farmers are interested in extending the time post-smolts are reared in recirculating aquaculture systems (RAS). However, there is a lack of knowledge regarding optimal water quality for post-smolts in RAS, and regarding potential consequences of long term exposure to different toxic compounds, such as nitrite, in the RAS water. To address this issue, we conducted a case study at a Faroese Atlantic salmon farm, that rears large post-smolts in fresh water RAS for 22 months before sea transfer, with no additional chloride salt, a known treatment for nitrite toxicity. The aim was to document the potential effects of long-term exposure of fluctuating sub-lethal nitrite concentrations in fresh water RAS on blood physiology of large post-smolts. The study was conducted over six weeks, at the end of the RAS production cycle. Our case study shows that after ~22 months in RAS with no additional chloride, the fish had accumulated a plasma nitrite concentration 8 to 16 times higher than the ambient water. Our results indicate that the accumulation may have resulted in extracellular hyperkaliemia, since there was a positive correlation between plasma nitrite and potassium levels (p=0.00095), with potassium levels almost twice as high than previously reported for Atlantic salmon. This could indicate that Atlantic salmon health is challenged due to prolonged sub-lethal nitrite exposure in fresh water RAS. Further research related to long-term nitrite exposure in RAS is needed to asses the potential negative impact, in order to optimize welfare and growth performance during production of Atlantic salmon post-smolts.
Introduction
Rearing farmed Atlantic salmon (Salmo salar) on-land for an extended time in recirculating aquaculture systems (RAS) is an increasing global trend. This strategy enables intensified fish production by reducing the need for new water, thus allowing for increased production on a given water source. In addition, RAS production increases biosecurity by limiting the exposure time to different pathogens and parasites encountered during the grow-out period at sea (Martins et al., 2010; Dalsgaard et al., 2013).
RAS is therefore viewed as a sustainable solution, and has, over the last 10 years, led to prolonging of the land-based production from 10 to approximately 20 months in the Faroe Islands. The average weight of individuals produced in RAS in the Faroe Islands is currently between 500-1000 g prior to stocking in sea cages for the final grow-out phase (personal communication with Rúni Dam, Avrik A/S).
However, keeping fish in a RAS for an extended period has its own challenges. The most pressing issue is the constant adjustment required to maintain the water quality within existing recommendations in order to provide the fish with an optimal environment.
RAS are better at providing a stable and controlled environment compared to flow through systems (FT) (Summerfelt et al., 2009). RAS is designed to prevent accumulation of potentially toxic waste products. For example, the use of biofilters should prevent acute toxic build-ups of nitrogen waste products, such as nitrite () (Blancheton et al., 2013). However, it is very difficult to maintain stable levels during commercial production of Atlantic salmon in RAS, instead, the levels typically fluctuate on a daily basis at sub-lethal levels (personal communication with commercial salmon producers). This is because the microbial community in the biofilter is easily affected by a variety of parameters. For example increase in biomass, changes in feeding regimes, organic content in the water, changes in pH and oxygen levels, and changes in temperature and salinity have all been associated with fluctuation in (Chen et al., 2006; Fossmark et al., 2021; Dahle et al., 2022).
If the fish are exposed to an unstable and fluctuating environment, they use energy to maintain internal homeostasis and can experience an allostatic overload (Segner et al., 2012). This can compromise fish growth and potentially the robustness needed to withstand the challenges faced after transfer to sea. In addition, if the fish are unable to maintain homeostasis and start to accumulate in the blood plasma from the RAS water it can alter and disrupt multiple physiological functions. For example, accumulation may cause Cl- depletion since and Cl- compete for the same uptake pathway through the gills via the brachial chloride (Cl-) uptake mechanism (Jensen, 2003). Adding Cl- salts to the water is therefore considered the single most important method for protecting fish against uptake, accumulation and toxicity (Jensen, 2003). Accumulation of in the blood may also have a critical influence on the potassium (K+) balance in fish (Jensen, 2003). Studies on fish have shown that exposure causes a general K+ loss from skeletal muscle and red blood cells causing significantly elevated extracellular [K+] concentration, a condition known as hyperkaliemia (Jensen et al, 1987; Jensen, 1990; Knudsen and Jensen, 1997; Vedel et al., 1998; Grosell and Jensen, 1999; Huertas et al., 2002). Furthermore, entry into red blood cells precipitates hemoglobin oxidation to methemoglobin which is structurally incapable of binding and transporting oxygen. Known as methemoglobinemia or “brown blood disease” (Jensen, 2003), this condition results in compromised blood oxygen transport which can have a myriad of deleterious effects on fish health (Lewis and Morris, 1986; Kroupova et al., 2005).
Although there is extensive evidences of the negative effects that and other water parameters can have on fish health, there is surprisingly little literature regarding truly optimal water condition for rearing fish in RAS (Brauner and Richards, 2020). In particular, the potential effects of prolonged exposure to sublethal concentrations in large post-smolts in fresh water RAS is not well documented. In the Faroe Islands, for example, there are no authoritative guidelines regarding water quality in RAS. Instead, the Norwegian guidelines (FDIR, 2004) are often used which state that levels should not exceed 0.1 mg/L in fresh water. However, no reference regarding addition of Cl- to RAS water in order to prevent accumulation and toxicity is provided.
Information about addition of Cl- is particularly relevant since the Faroe Islands uses fresh water in the RAS with no additional Cl- in order to prevent hydrogen sulfide (H2S) build-up, which has caused mass fish mortality in RAS (Letelier-Gordo et al., 2020). Increased salinity is also known to have a negative impact on the nitrification efficiency of the biofilter, making it difficult to maintain stable levels (Fossmark et al., 2021).
We therefore conducted a case study at a Faroese RAS farm over six-weeks at the end of the land-based production. The commercial farm rears large post-smolt Atlantic salmon in freshwater RAS for 22 months without any additional Cl- salt. The aim of our study was to document what effect chronic exposure to fluctuating sublethal concentrations may have on the blood physiology of these large post-smolts. We chose to focus on the blood ions, , Cl- and K+ as they are considered potential welfare indicators of accumulation in farmed fish (Jensen, 2003; Gutiérrez et al., 2019).
Method
Experimental Conditions and Origin of Fish
Atlantic salmon were sourced from a smolt production farm in the Faroe Islands which raises large post-smolts (~400-500 g) in land-based freshwater RAS for 22 months. The study began eight weeks prior to the scheduled transfer of the salmon to sea cages.
All individuals included in the study originated from the Stofnfiskur strain and were reared in the same tank and RAS. The smolt station utilized raw spring make-up water without the addition of supplemental Cl-. No deviations from standard operating procedures or incidence of unexpected stress, disease or mortality occurred during the study period.
Sampling and Analysis
This study was conducted over a six-week period which ended two weeks prior to sea transfer. Once a week 15 large post-smolt Atlantic salmon (90 fish in total) were carefully captured by net and immediately killed by a blow to the head. This was done one fish at a time to prevent struggling since strenuous exercise can cause an outward leak of K+ from cells to plasma (McDonald and Milligan, 1992). All sampling was done at the same time of day.
Blood was drawn from the caudal vein of each salmon using a 21G needle attached to a syringe. Following collection in the syringe, approx. 1.5-2.0 ml of whole blood was immediately transferred to lithium heparin coated vacutainer collection tubes to avoid coagulation of the blood. This two-step collection method was used to avoid hemolysis that can occur due to the vacuum effect of direct vacutainer collection and thus result in an overestimation of the K+ level in the plasma.
Plasma was immediately separated (10 min 6000 rpm, MC 6 Sarstedt centrifuge) and stored at -80°C for later Cl-, and K+ analysis.
Cl- and K+ ions were analyzed on an Architect C8000 (Abbot) by the clinical chemistry laboratory of the National Hospital of the Faroe Islands. The concentration was measured as mmol/L, but for consistency it was converted to mg/L by multiplying it with its molar mass, which is 35.453 and 39.0983 g/mol for Cl- and K+ respectively.
The NO2- concentration in the plasma was analyzed with a Nitrate/Nitrite Colorimetric Assay kit based on the Griess-reaction, but without the nitrate reduction step (Cayman Chemical, 780001). Prior to analysis, the sample was ultrafiltrated through a 10 kDA molecular weight cut-off filter (Merck, UFC501096) to reduce background absorbance due to the presence of hemoglobin and to improve color formation using the Griess Reagents. The plasma samples were diluted 1:10, and run in duplicate. The absorbance was measured on a plate reader at 550 nm (CLARIOstar Plus). The NO2- concentration was calculated from a standard curve from duplicate assays with eight known concentrations (0, 5, 10, 15, 20, 25, 30 and 35 µM) of NO2- (r2 > 0.985 for all curves), and corrected for dilution. The NO2- concentration was then converted to by dividing it by 3.3, the ratio of their molecular weights, assuming atomic weights N: 14.007 and O:15.999.
The concentration in the RAS was provided by the smolt farm operations team. They measure the concentration daily, however, weekend measurements were sometimes not performed. The NO2- concentration was converted to by dividing it by 3.3, as described above.
Since the smolt farm does not measure the Cl- concentration in the water, a water sample was taken on each sampling day from the tank and the Cl- concentration was determined in duplicate by simple precipitation titration with silver nitrate and potassium chromate as indicator (Mohr´s method).
Statistical analysis (simple Person´s linear regression) of the data and figures were done using the statistical software program R (R Core Team, 2021) using the packages tidyverse and ggpubr.
Results
During the on-land production the fish were exposed to fluctuating levels ranging from a minimum of 0.003 mg/L to a maximum of 9.3 mg/L (Figure 1).
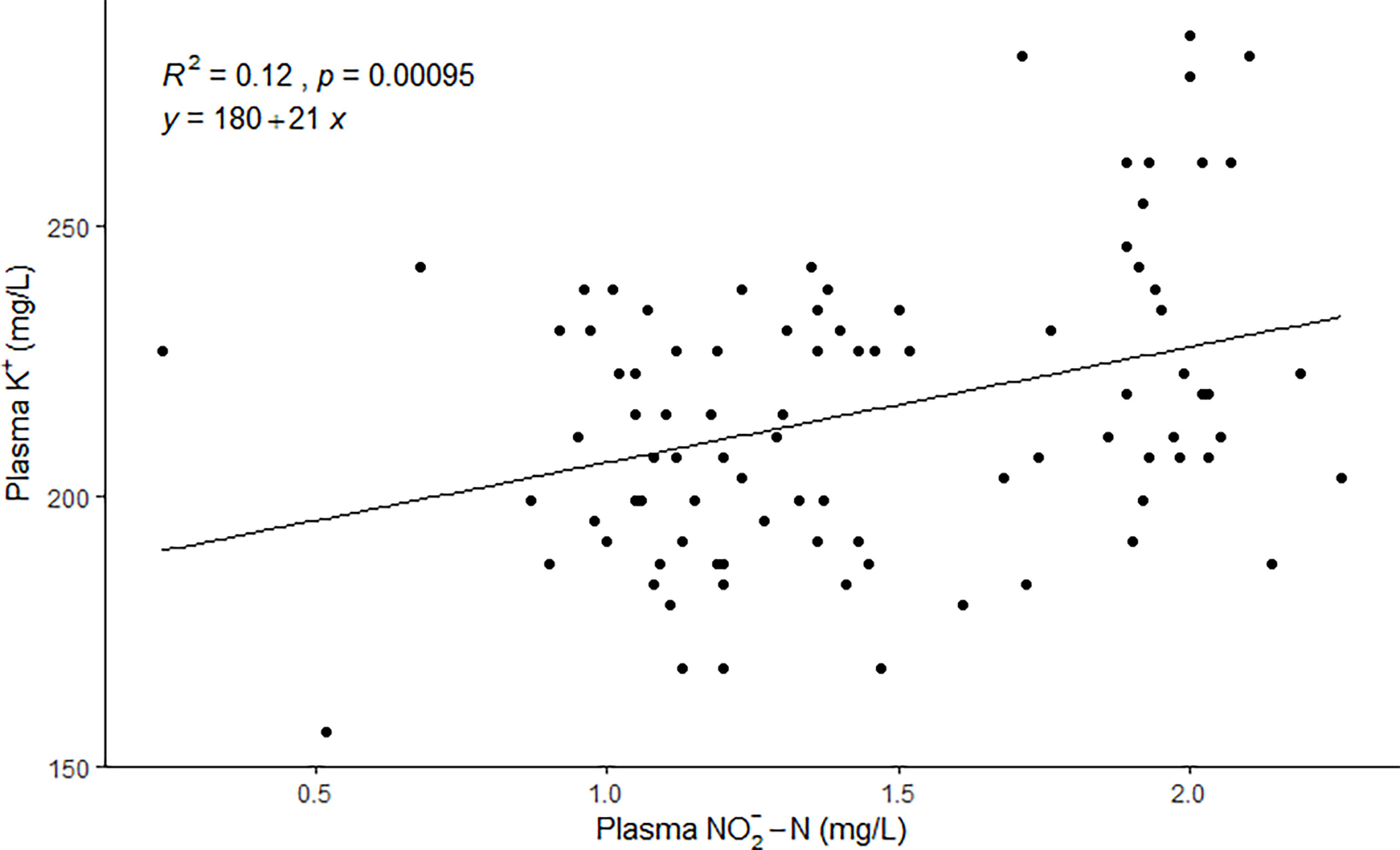
Figure 1 The daily fluctuating concentration (log scale) in the RAS water over the 22-month production period. Brackets and black dots illustrate the six weeks of sampling. The dashed horizontal line shows the FDIR (2004) recommended nitrite limit of 0.1 mg/L .
On the six sampling days the concentration in the tank water ranged between a minimum of 0.08 mg/L to a maximum of 0.22 mg/L . The Cl- concentration in the tank water ranged from a minimum of 10.51 ± 0.71 mg/L to a maximum of 15.01 mg/L, giving a Cl-: ratio between 61:1 and 163:1 on the actual days of sampling (Table 1).
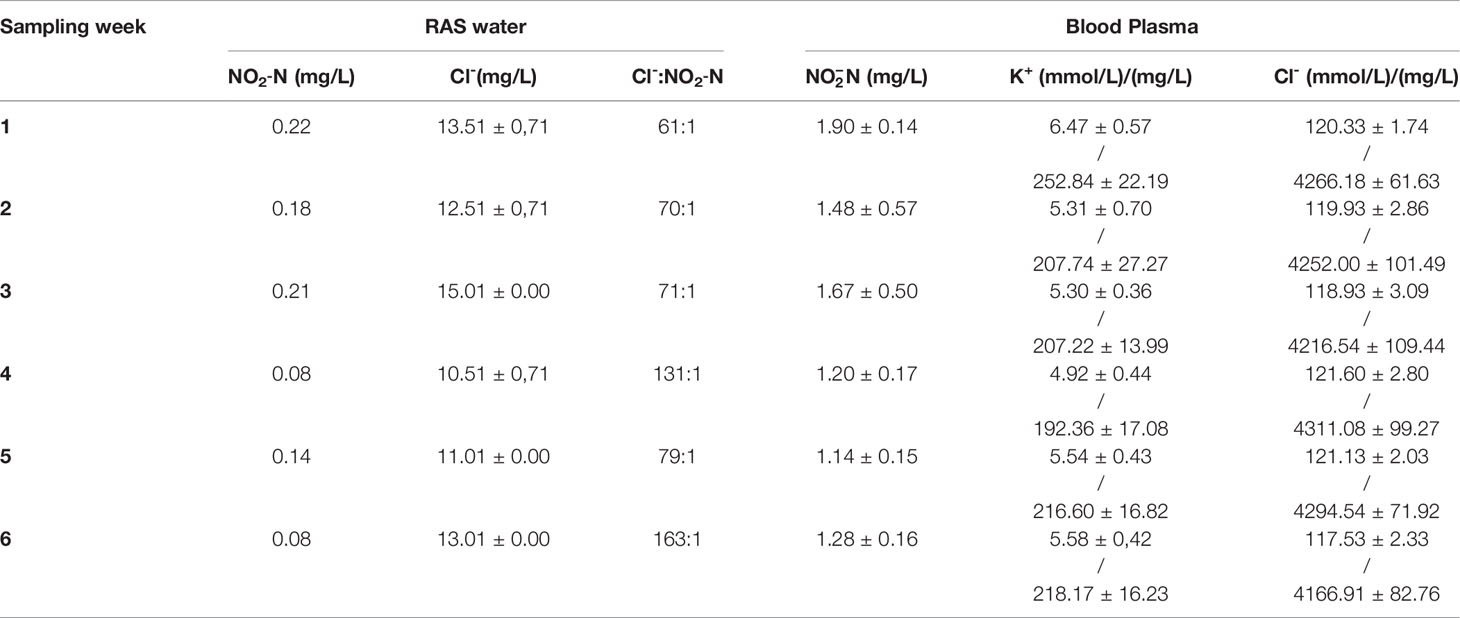
Table 1 To the left the and the mean Cl- (± SD, N = 2) concentration in the RAS water, and the Cl-: ratio on each sampling day. To the right the mean (± SD, N = 15) blood plasma concentration of , K+ and Cl-.
The mean plasma levels ranged between 1.14 ± 0.15 and 1.90 ± 0.14 mg/L (Table 1). The mean plasma K+ levels, ranged between 192.36 ± 17.08 and 252.84 ± 22.19 mg/L (4.92 ± 0.44 and 6.47 ± 0.57 mmol/L) on each sampling day (Table 1), and there was a significant positive relationship (p=0.00095) between the concentration in plasma and the K+ concentration in plasma (Figure 2). The fish had normal plasma Cl- levels for fresh water, ranging from 4166.91 ± 82.76 mg/L (117.53 ± 2.33 mmol/L) to 4311.08 ± 99.27 mg/L (121.60 ± 2.80 mmol/L) (Table 1).
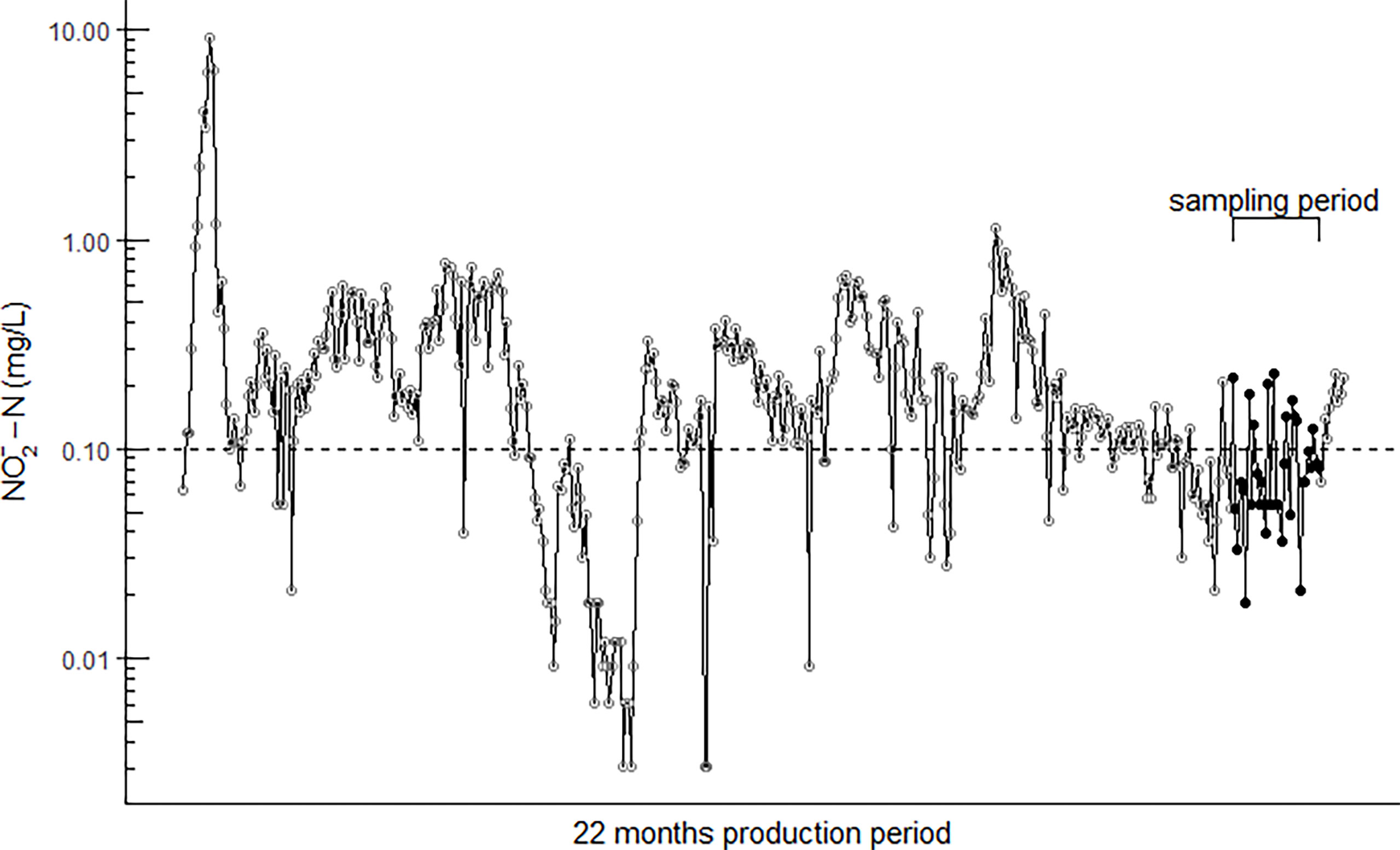
Figure 2 A significant positive correlation (simple Person´s linear regression) is between the and K+ concentration in plasma (N=90 fish).
The fish had a condition factor between 1.14 ± 0.08 and 1.25 ± 0.12 (Table 2).
Discussion
Documentation of prolonged post-smolt production and the effects of water quality on fish welfare and growth performance in commercial RAS are scarce (Brauner and Richards, 2020).
Our case study showed that after ~20 months of fluctuating (0.003-9.27 mg/L) sublethal exposure in a RAS that did not add additional Cl-, fish blood plasma – concentration was 8 to 16 times higher than that of the surrounding RAS water. These results demonstrate that prolonged production of post-smolt Atlantic salmon on-land in freshwater RAS can cause accumulation of potentially toxic - in blood.
One of the reasons for the observed accumulation of in post-smolt plasma may be the low Cl- concentration in process water relative to the concentration. A recent study indicated that maintaining a constant Cl-: ratio of 104:1 is sufficient to protect parr from nitrite accumulation in the blood (Gutiérrez et al., 2019). During our study, this ratio varied from 61:1 and 163:1 due to oscillating concentrations of and Cl- in RAS water. Since no Cl- was added to the water, its concentration depended solely on the natural concentration in the raw water used in the RAS. The Cl- concentration in raw water in the Faroe Islands can vary greatly (~10-40 mg/l) depending on the raw water source which can be either spring water or surface water. The spring water used in this smolt station is more stable and often contains a lower concentration of Cl- (~15-10 mg/L) compared to surface water (Larsen, 2000). In addition to oscillations in water Cl- concentrations, daily changes in are common in commercial RAS. According to the commercial facility, where this case study was conducted, the oscillation of can be explained by washing of the biofilters (done every two to five weeks), overfeeding, increase in biomass, sorting, treatment for fungi and oscillation in water pH.
Since the smolt station does not measure the Cl- concentration in the water, we do not know what the daily Cl-: ratio has been during the 22 months of on-land production prior to the sampling period. Therefore, it is difficult to say what the optimal Cl-: ratio is and what duration is necessary to prevent accumulation of in the blood of Atlantic salmon post-smolt under commercial conditions. More research is needed to answer to this question.
The constant physiological compensation needed to maintain homeostasis in an unstable environment comes at a high energy cost and can result in an allostatic overload (Segner et al., 2012). In this case, having to adapt to the fluctuating levels may have compromised or exhausted the salmon’s ability to regulate . This disequilibrium may then lead to high accumulation of plasma , even at sub-lethal water concentrations. Future controlled studies are needed to corroborate this hypothesis.
The high accumulation of plasma could also be explained by the fact that 28% of all measurements taken in the water over the 22 month on-land production period were ≥ the FDIR recommended limit of 0.1 mg/L .
Despite the high accumulation in plasma, the fish had normal documented plasma Cl- levels for fresh water, if it is assumed that the normal Cl- levels of large post-smolts are the same as for parr in fresh water (Folmar and Dickhoff, 1980; Noble et al., 2018). This result supports the finding of Gutiérrez et al. (2019) and other previous studies (Lewis and Morris, 1986) that demonstrate fish are capable of maintaining a fixed Cl- concentration even when concentrations are high in the surrounding water. One explanation for this could be that the presence of NO2- in fresh water causes Cl- cell proliferation (Gaino et al., 1984), possibly as a compensatory response that counteracts Cl- depletion. Whatever the reason, this finding could indicate that the plasma Cl- concentration is not a reliable biomarker for estimating toxicity in fish after long-term exposure to sublethal NO2- conditions.
The results of our study indicate that the salmon post-smolts suffered from extracellular hyperkaliemia, which could be partly attributed to the high accumulation of in plasma given the significant positive correlation between plasma and K+ concentration (p = 0.00095).
The mean plasma K+ levels in this study ranged between 4.92 and 6.47 mmol/L. For comparison, all previously published reports of K+ plasma levels for fresh water Atlantic salmons ranged from 0.9 to 4.5 mmol/L, making our case study the highest K+ plasma levels reported to date (Carey and McCormick, 1998; Brauner et al., 2000; Quigley et al., 2006; Fridell et al., 2007; Nomura et al., 2009; Davidson et al., 2014; Davidson et al., 2017; Noble et al., 2018). Extracellular hyperkaliemia is problematic for heart and other excitable tissues because it causes excess depolarization that can potentially lead to heart failure and nerve malfunction (Jensen, 2003). A study with rainbow trout (Oncorhynchus mykiss) showed that plasma K+ levels of 5.0 mmol/L significantly decreased the maximum cardiac performance, and a noticeable arrhythmia developed at 7.5 mmo/l K+ (Hanson et al., 2006). In this study, 77% of the sampled fish had plasma K+ levels of 5.0 mmol/l or higher, with the highest concentration measured at 7.3 mmol/L.
Presence of hyperkaliemia and its potential effect on heart health is particularly relevant for the Faroe Islands where salmon is increasingly being produced at exposed locations during the grow-out sea water phase. At exposed sites the fish must adapt to higher speed currents and waves. This is concerning since sudden strenuous exercise can also cause an additional outward leak of K+ from cells to plasma (McDonald and Milligan, 1992), possibly worsening the hyperkalemic situation, and leading to impaired health and even mortalities. In this case study, no abnormal high mortalities were reported during the entire land-based production nor after sea transfer to a site that can be classified as a non-exposed site. Thus, it seems that Atlantic salmon are capable of withstanding high accumulation of plasma and extracellular hyperkaliemia, without resulting in high mortalities. Similarly, a recent study that followed long-term post-smolt exposure to CO2 did not cause mortalities, but did impair the growth in RAS. Moreover, the negative growth effect continued in the seawater phase of production (Mota et al., 2019). In this study we did not focus on the post-smolt growth, but the possibility that prolonged exposure can have a negative effect on growth, potentially during both RAS and at-sea production, should be investigated.
In conclusion, our case study indicates that the blood ions, and K+, can be considered as potential welfare indicators of exposure in post-smolt Atlantic salmon. The results of this case study also indicate that Atlantic salmon health and welfare can potentially be challenged during prolonged production on land in freshwater RAS. However, since we concentrated our sampling only during the last few weeks of the land-based production, we only get a snap-shot of the situation prior to sea transfer. The mitigating effects of Cl- on toxicity in post-smolts in RAS are still unknown and factors such as the optimal Cl-: ratio has to be further investigated. Further controlled studies are therefore needed to validate our findings and to fully evaluate the effect that fluctuating water NO2-N has on salmon in RAS during prolonged land-based production and potentially after transfer to sea.
Data Availability Statement
The original contributions presented in the study are included in the article/Supplementary Material. Further inquiries can be directed to the corresponding author.
Ethics Statement
The animal study was reviewed and approved by Fiskaaling´s (Aquaculture Research Station of the Faroes) internal ethical review board (approval nr. 004).
Author Contributions
HM designed the study, did the sampling and analysis of the samples, as well as all the statistical analysis. HM also wrote the manuscript. EJ conducted sampling and analysis of the samples in the laboratory. EJ also read and approved the submitted version. JK and AV contributed with discussion of the results, drafting and revising the manuscript. All authors contributed to the article and approved the submitted version.
Funding
This study was internally funded by P/F Fiskaaling.
Conflict of Interest
The authors declare that the research was conducted in the absence of any commercial or financial relationships that could be construed as a potential conflict of interest.
The handling Editor CL declared a past co-authorship with the author JK.
Publisher’s Note
All claims expressed in this article are solely those of the authors and do not necessarily represent those of their affiliated organizations, or those of the publisher, the editors and the reviewers. Any product that may be evaluated in this article, or claim that may be made by its manufacturer, is not guaranteed or endorsed by the publisher.
Acknowledgments
We would like to extend our gratitude and appreciation to the clinical chemistry laboratory at National Hospital of the Faroe Islands, especially Marita Simonsen and Ann E. Østerø, for processing of chemical analysis and guidance regarding blood sampling. We would also like to thank the farming company for giving us access to their smolt station and for providing us with data.
Supplementary Material
The Supplementary Material for this article can be found online at: https://www.frontiersin.org/articles/10.3389/fanim.2022.886071/full#supplementary-material
References
Blancheton J. P., Attramadal K. J. K., Michaud L., Roque D’Orbcastel E., Vadstein O. (2013). Insight Into Bacterial Population in Aquaculture Systems and its Implication. Aquacult Eng. 53, 30–39. doi: 10.1016/J.AQUAENG.2012.11.009
Brauner C. J., Richards J. G. (2020). Physiological Performance in Aquaculture: Using Physiology to Help Define Optimal Conditions for Growth and Environmental Tolerance. Fish Physiol. 38, 83–121. doi: 10.1016/BS.FP.2020.10.001
Brauner C. J., Seidelin M., Madsen S. S., Jensen F. B. (2000). Effects of Freshwater Hyperoxia and Hypercapnia and Their Influences on Subsequent Seawater Transfer in Atlantic Salmon (Salmo Salar) Smolts. Can. J. Fish Aquat. Sci. 57 (10), 2054–2064. doi: 10.1139/f00-161
Carey J. B., McCormick S. D. (1998). Atlantic Salmon Smolts are More Responsive to an Acute Handling and Confinement Stress Than Parr. Aquaculture 168 (1–4), 237–253. doi: 10.1016/S0044-8486(98)00352-4
Chen S., Ling J., Blancheton J. P. (2006). Nitrification Kinetics of Biofilm as Affected by Water Quality Factors. Aquacult Eng. 34 (3), 179–975. doi: 10.1016/J.AQUAENG.2005.09.004
Dahle S. W., Attramadal K. J. K., Vadstein O., Hestdahl H. I., Bakke I. (2022). Microbial Community Dynamics in a Commercial RAS for Production of Atlantic Salmon Fry (Salmo Salar). Aquaculture 546, 737382. doi: 10.1016/j.aquaculture.2021.737382
Dalsgaard J., Lund I., Thorarinsdottir R., Drengstig A., Arvonen K., Pedersen P. B. (2013). Farming Different Species in RAS in Nordic Countries: Current Status and Future Perspectives. Aquacult Eng. 53, 2–13. doi: 10.1016/j.aquaeng.2012.11.008
Davidson J., Good C., Welsh C., Summerfelt S. T. (2014). Aquacultural Engineering Comparing the Effects of High vs. Low Nitrate on the Health, Performance, and Welfare of Juvenile Rainbow Trout Oncorhynchus Mykiss Within Water Recirculating Aquaculture Systems. Aquacult Eng. 59 (3), 30–40. doi: 10.1016/j.aquaeng.2014.01.003
Davidson J., Good C., Williams C., Summerfelt S. T. (2017). Aquacultural Engineering Evaluating the Chronic E Ff Ects of Nitrate on the Health and Performance of Post-Smolt Atlantic Salmon Salmo Salar in Freshwater Recirculation Aquaculture Systems. Aquacult Eng. 79 (May), 1–8. doi: 10.1016/j.aquaeng.2017.08.003
FDIR (2004). Directorate of Fisheries. Notes to regulations of 22. December 2004, no. 1785, concerning management of aquaculture facilities (in Norwegian, akvakulturdrifts-forskriften. Available at: https://lovdata.no/dokument/SF/forskrift/2008-06-17-822
Folmar F., Dickhoff W. W. (1980). The Parr—Smolt Transformation (Smoltification) and Seawater Adaptation in Salmonids: A Review of Selected Literature. Aquaculture 21 (1), 1–37. doi: 10.1016/0044-8486(80)90123-4
Fossmark R. O., Attramadal K. J. K., Nordøy K., Østerhus S. W., Vadstein O. (2021). A Comparison of Two Seawater Adaptation Strategies for Atlantic Salmon Post-Smolt (Salmo Salar) Grown in Recirculating Aquaculture Systems (RAS): Nitrification, Water and Gut Microbiota, and Performance of Fish. Aquaculture 532, 735973. doi: 10.1016/j.aquaculture.2020.735973
Fridell F., Gadan K., Sundh H., Taranger G. L., Glette J., Olsen R. E., et al. (2007). Effect of Hyperoxygenation and Low Water Flow on the Primary Stress Response and Susceptibility of Atlantic Salmon Salmo Salar L. @ to Experimental Challenge With IPN Virus. Aquaculture 270 (1–4), 23–35. doi: 10.1016/j.aquaculture.2007.04.081
Gaino E., Arillo A., Mensi P. (1984). Involvement of the Gill Chloride Cells of Trout Under Acute Nitrite Intoxication. Comp. Biochem. Physiol. Part A: Physiol. 77 (4), 611–617. doi: 10.1016/0300-9629(84)90171-3
Grosell M., Jensen F. B. (1999). NO2- Uptake and HCO3- Excretion in the Intestine of the European Flounder (Platichthys Flesus). J. Exp. Biol. 202 (15), 2103–2110. doi: 10.1242/jeb.202.15.2103
Gutiérrez X. A., Kolarevic J., Takle H., Baeverfjord G., Ytteborg E., Fyhn Terjesen B. (2019). Effects of Chronic Sub-Lethal Nitrite Exposure at High Water Chloride Concentration on Atlantic Salmon (Salmo Salar, Linnaeus 1758) Parr. Aquacult Res. 50 (9), 2687–2697. doi: 10.1111/are.14226
Hanson L. M., Obradovich S., Mouniargi J., Farrell A. P. (2006). The Role of Adrenergic Stimulation in Maintaining Maximum Cardiac Performance in Rainbow Trout (Oncorhynchus Mykiss) During Hypoxia, Hyperkalemia and Acidosis at 10°C. J. Exp. Biol. 209 (13), 2442–2451. doi: 10.1242/jeb.02237
Huertas M., Gisbert E., Rodríguez A., Cardona L., Williot P., Castelló-Orvay F. (2002). Acute Exposure of Siberian Sturgeon (Acipenser Baeri, Brandt) Yearlings to Nitrite: Median-Lethal Concentration (LC50) Determination, Haematological Changes and Nitrite Accumulation in Selected Tissues. Aquat. Toxicol. 57 (4), 257–266. doi: 10.1016/S0166-445X(01)00207-7
Jensen F. B. (1990). Nitrite and Red Cell Function in Carp: Control Factors for Nitrite Entry, Membrane Potassium Ion Permeation, Oxygen Affinity and Methaemoglobin Formation. J. Exp. Biol. 152, 149–166. doi: 10.1242/jeb.152.1.149
Jensen F. B. (2003). Nitrite Disrupts Multiple Physiological Functions in Aquatic Animals. Comp. Biochem. Physiol. Part A: Mol. Integr. Physiol. 135 (1), 9–24. doi: 10.1016/S1095-6433(02)00323-9
Jensen F. B., Andersen N. A., Heisler N. (1987). Effects of Nitrite Exposure on Blood Respiratory Properties, Acid-Base and Electrolyte Regulation in the Carp (Cyprinus Carpio). J. Comp. Physiol. B: Biochem Syst Environ. Physiol. 157 (5), 533–541. doi: 10.1007/BF00700972
Knudsen P. K., Jensen F. B. (1997). Recovery From Nitrite-Induced Methaemoglobinaemia and Potassium Balance Disturbances in Carp. Fish Physiol. Biochem. 16 (1), 1–10. doi: 10.1007/BF00004535
Kroupova H., Machova J., Svobodova Z. (2005). Nitrite Influence on Fish: A Review. Veterinar Med 50 (11), 461–471. doi: 10.17221/5650-VETMED
Larsen R. B. (2000). Vandkvalitet I Den Færøske Fiskeindustry. Heilsufrøðiliga Starvsstovan 2000, Vol. 4. Available at: https://hfs.fo/webcenter/ShowProperty?nodeId=%2Fhfs2-cs%2FHFS017905%2F%2FidcPrimaryFile&revision=latestreleased
Letelier-Gordo C. O., Aalto S. L., Suurnäkki S., Pedersen P. B. (2020). Increased Sulfate Availability in Saline Water Promotes Hydrogen Sulfide Production in Fish Organic Waste. Aquacult Eng. 89, 102062. doi: 10.1016/j.aquaeng.2020.102062
Lewis W. M., Morris D. P. (1986). Toxicity of Nitrite to Fish: A Review. Trans. Am. Fish Soc. 115, 183–195. doi: 10.1577/1548-8659(1986)115<183:TONTF>2.0.CO;2
Martins C. I. M., Eding E. H., Verdegem M. C. J., Heinsbroek L. T. N., Schneider O., Blancheton J. P., et al. (2010). New Developments in Recirculating Aquaculture Systems in Europe: A Perspective on Environmental Sustainability. Aquacult Eng. 43 (3), 83–93. doi: 10.1016/j.aquaeng.2010.09.002
McDonald D. G., Milligan C. L. (1992). Chemical Properties of the Blood. Fish Physiol. (New York) Volume XIIB, 55–133. doi: 10.1016/S1546-5098(08)60009-6
Mota V. C., Nilsen T. O., Gerwins J., Gallo M., Ytteborg E., Baeverfjord G., et al. (2019). The Effects of Carbon Dioxide on Growth Performance, Welfare, and Health of Atlantic Salmon Post-Smolt (Salmo Salar) in Recirculating Aquaculture Systems. Aquaculture 498, 578–586. doi: 10.1016/j.aquaculture.2018.08.075
Noble C., Nilsson J., Stien L. H., Iversen M. H., Kolarevic J., Gismervik K. (2018) Velferdsindikatorer for Oppdrettslaks: Hvordan Vurdere Og Dokumentere Fiskevelferd. Available at: https://nofima-326d.kxcdn.com/wp-content/uploads/2016/06/Velferdsindikatorer-for-oppdrettslaks-2018.pdf.
Nomura M., Sloman K. A., von Keyserlingk M. A. G., Farrell A. P. (2009). Physiology and Behaviour of Atlantic Salmon (Salmo Salar) Smolts During Commercial Land and Sea Transport. Physiol. Behav. 96 (2), 233–243. doi: 10.1016/j.physbeh.2008.10.006
Quigley D. T. G., Harvey M. J., Hayden T. J., Dowling C., O’Keane M. P. (2006). A Comparative Study of Smoltification in Sea Trout (Salmo Trutta L.) and Atlantic Salmon (Salmo Salar L.): Seawater Tolerance and Thyroid Hormone Titres. Biol. Environ. 106 (1), 35–47. doi: 10.3318/BIOE.2006.106.1.35
Segner H., Sundh H., Buchmann K., Douxfils J., Sundell K. S., Mathieu C., et al. (2012). Health of Farmed Fish: Its Relation to Fish Welfare and its Utility as Welfare Indicator. Fish Physiol. Biochem. 38 (1), 85–105. doi: 10.1007/s10695-011-9517-9
Summerfelt S. T., Sharrer M., Gearheart M., Gillette K., Vinci B. J. (2009). Evaluation of Partial Water Reuse Systems Used for Atlantic Salmon Smolt Production at the White River National Fish Hatchery. Aquacult Eng. 41 (2), 78–84. doi: 10.1016/j.aquaeng.2009.06.003
Team, R. C. (2021). R: A Language and Environment for Statistical Computing (Vienna, Austria: R Foundation for Statistical Computing). Available at: https://www.r-project.org/.
Vedel N. E., Korsgaard B., Jensen F. B. (1998). Isolated and Combined Exposure to Ammonia and Nitrite in Rainbow Trout (Oncorhynchus Mykiss): Effects on Electrolyte Status, Blood Respiratory Properties and Brain Glutamine/Glutamate Concentrations. Aquat. Toxicol. 41 (4), 325–342. doi: 10.1016/S0166-445X(97)00071-4
Keywords: post-smolt, Atlantic Salmon, fresh water RAS, water quality, fluctuating nitrite concentration, hyperkalaemia, chloride
Citation: Mortensen HS, Jacobsen E, Kolarevic J and Vang A (2022) Exposing Atlantic Salmon Post-Smolts to Fluctuating Sublethal Nitrite Concentrations in a Commercial Recirculating Aquaculture System (RAS) May Have Negative Consequences. Front. Anim. Sci. 3:886071. doi: 10.3389/fanim.2022.886071
Received: 28 February 2022; Accepted: 15 June 2022;
Published: 11 July 2022.
Edited by:
Carlo C. Lazado, Fisheries and Aquaculture Research (Nofima), NorwayReviewed by:
John Phillip Bowman, University of Tasmania, AustraliaRafael Freire, Charles Sturt University, Australia
Copyright © 2022 Mortensen, Jacobsen, Kolarevic and Vang. This is an open-access article distributed under the terms of the Creative Commons Attribution License (CC BY). The use, distribution or reproduction in other forums is permitted, provided the original author(s) and the copyright owner(s) are credited and that the original publication in this journal is cited, in accordance with accepted academic practice. No use, distribution or reproduction is permitted which does not comply with these terms.
*Correspondence: Heidi S. Mortensen, heidi@fiskaaling.fo; Elin Jacobsen, elinja@fiskaaling.fo; Jelena Kolarevic, jelena.kolarevic@uit.no; Amanda Vang, amanda@fiskaaling.fo