- 1College of Animal Science and Technology, Hunan Agricultural University, Changsha, China
- 2Ministry of Education Engineering Research Center of Feed Safety and Efficient Use, Changsha, China
- 3Hunan Engineering Research Center of Poultry Production Safety, Changsha, China
- 4Hunan Co-Innovation Center of Animal Production Safety, Changsha, China
- 5Jiurui Biology & Chemistry Co., Ltd., Zhangjiajie, China
The purpose of this experiment was to explore the effects of Galla chinensis extract (GCE) supplementation in broiler diets on growth performance, carcass traits, serum antioxidation, immune function, and gut microbiota. In this study, broiler chicken diets were supplemented with a product of GCE at 0 mg/kg (control), 250 mg/kg (GCE250), 500 mg/kg (GCE500), 1,000 mg/kg (GCE1000), or 2,000 mg/kg (GCE2000). In total, 360 birds were randomly distributed into five treatments with six replicate pens and fed from 1 to 60 days. The birds in the GCE250 group had higher final body weights (p < 0.05) compared with the control group. Low-dose supplementation (250 mg/kg) of GCE significantly improved the broiler chicken's antioxidative and anti-inflammatory abilities by decreasing serum levels of malondialdehyde, interleukin 6, and interferon-γ at day 60 (p < 0.05). Additionally, principal coordinate analysis clustering showed that GCE supplementation shifted the cecal microbiota of broiler chickens compared with control at day 60, increasing the relative abundance of beneficial bacterial genera such as Faecalibacterium, Parabacteroides, and Alistipes, and decreasing the potentially pathogenic bacteria Streptococcus. Taken together, the results of this study showed that dietary supplementation of low concentrations of GCE (250 mg/kg) produced a positive influence on growth performance, gut microbiota, serum antioxidation, and anti-inflammatory markers in broiler chickens.
Introduction
Galla chinensis is a Chinese traditional medicine that is obtained by cooking and drying galls that form on Rhus chinensis leaves by the parasitic Chinese sumac aphid larvae. The main bioactive components in Galla chinensis extract (GCE) are polyphenolic compounds, which contain gallotannins and gallic acid (Xie et al., 2005). Gallotannins represent one class of hydrolyzable tannins, which can be either directly absorbed by the small intestine, or first hydrolyzed into gallic acid and then absorbed by animals (Kamiloglu et al., 2016). Gallic acid is a secondary metabolite of tannin and has been reported to have great potential to inhibit tumor growth (Raina et al., 2008; Wang et al., 2020), relieve oxidative stress (Romero-Montero et al., 2020; Moradi et al., 2021), ameliorate inflammation (BenSaad et al., 2017), and control the propagation of pathogenic bacteria (Choinska et al., 2021). Additionally, dietary supplementation of 100 mg/kg of gallic acid was found to decrease the feed conversion ratio and increase the breast muscle percentage for broilers (Samuel et al., 2017). Specifically, gallic acid increased the unsaturated fatty acid content and meat quality (water holding capacity) of the broiler breast muscle, possibly due to its antioxidative effects (Jung et al., 2010; Starcevic et al., 2015).
The intensive production and excessive growth of modern commercial broilers have led to many unexpected novel problems related to animal welfare and health, such as woody breast (Sihvo et al., 2014; Brambila et al., 2017), foot pad dermatitis (Swiatkiewicz et al., 2017), and bone abnormalities (Fleming, 2008). A possible reason for these problems is that high energy uptake from the broiler feed causes an increased metabolic burden and considerable oxidative stress, which impairs the intestinal, core digestive, and absorptive organs in the digestive tract (Mishra and Jha, 2019; Xing et al., 2021). Cellular oxidative stress is caused by the overload of free radicals, including reactive oxygen species (ROS) and reactive nitrogen species, which further result in lipid peroxidation, protein nitration, DNA breakage, and even cell death (Estevez, 2015). The intestinal epithelium is a barrier firmly formed by single-layer columnar cells aligned with tight junctions. Oxidative stress attacks the intestinal epithelial cells and reduces the number of cellular junctions by suppressing the expression of junction proteins (Osselaere et al., 2013; Zhang et al., 2017). The intestinal commensal microorganisms serve an indispensable role in intestinal homeostasis, but also interact with the host animal to utilize gastrointestinal nutrients to generate ROS or endogenous, triggering oxidation stress (Ulluwishewa et al., 2011). Plant-derived extracts are abundant with bioactive polyphenol compounds, which possess strong antioxidative and prebiotic abilities (Veskoukis et al., 2012). However, little is known about the effects of dietary GCE supplementation on broiler chickens. In this study, we assumed that dietary supplementation of GCE could improve the growth performance of broiler chickens by ameliorating oxidative stress and inflammatory reactions or balancing the gut microbiome. Thus, we aimed to investigate the growth performance, carcass traits, profiles of the serum antioxidative and immune factors, and intestinal microbiota of the birds fed with different concentrations of GCE (250, 500, 1,000, and 2,000 mg/kg) in the diet.
Materials and Methods
Product, Animals, and Housing Conditions
The Galla chinesis extract (GCE) with approximately 97% hydrolyzable tannins was bought from Jiurui Biology & Chemistry Co., Ltd. (Zhangjiajie Economic Development Zone, Hunan Province, China). The products were newly formulated and presented with a fresh, light-yellow color. A total of 432 one-day-old yellow-feathered broiler chicks (37.36 ± 0.68 g) were purchased from Hunan Jitai Animal Husbandry Company and reared at Hunan Agricultural University Broiler Standard Experiment Base. All animal experiments and procedures were approved by the Institutional Animal Care and Use Committee of Hunan Agricultural University. All of the birds were randomly distributed into six treatment groups with six replicates per treatment and 12 birds per replicate in a completely randomized design. The experiment was performed according to a two-phase feeding program with a starter phase from day 1 to 28, and a finisher phase from day 29 to 60. Ingredients and nutrient compositions of the basal diet were based on nutrient requirements of poultry revised by NRC (National Research Council, US), 1994 recommendations and are listed in Table 1. A total of five dietary treatments were fed from days 0 to 60, including a control, and negative control supplemented with 250, 500, 1,000, and 2,000 mg/kg CGE. Housing temperature was maintained at 32°C during the first 3 days and then reduced gradually to 26°C on day 21. The lighting schedule was continuous dim white light for 3 days after hatch, followed by a schedule of 23 h of lighting and 1 h of darkness throughout the experiment. All broilers had ad libitum access to feed and freshwater.
Growth Performance
All of the birds in each replicate were weighed together on day 1, day 28, and day 60. The growth performance was calculated as body weight (BW), average daily gain (ADG), average daily feed intake (ADFI), and feed conversion rate (FCR). When a bird unexpectedly died during the feeding period, the total feed intake of this replicate was corrected using the following equation:
Corrected total feed intake (g) = Whole period feed intake (g) –
FCR at day of death = Total feed intake at day of death (g)/Total BW at day of death (g)
The FCR on day of death was calculated using the data from all six replicates.
Carcass Traits and Immune Organ Index
At the end of the experiment, one bird per replicate (six per treatment) was sampled randomly for carcass evaluations and was weighed and slaughtered. The weights of the abdominal fat, pectoral muscle, thigh, spleen, cloacal bursa, thymus, and liver were recorded, respectively. All the carcass traits (%) were expressed as indexes calculated as follows:
Dressing yield = (carcass weight/live weight) × 100%
Semi-evisceration rate = ((carcass weight – weight of trachea, esophagus, crop, intestine, spleen, pancreas, gall bladder, and reproductive organs)/carcass weight) × 100%
Evisceration rate = ((semi-evisceration weight – weight of heart, liver, gizzard, stomach, and abdominal fat) /carcass weight) × 100%
Abdominal fat yield = (abdominal fat weight/carcass weight) × 100%
Breast yield = (breast muscle weight/carcass weight) × 100%
Leg yield = (leg muscle weight/carcass weight) × 100%
All of the immune organs (spleen, cloacal bursa, thymus, and liver) were clearly removed from the bird and weighed, and the corresponding immune organ index (g/kg) was calculated as follows:
Organ index (g/kg) = organ weight (g)/live BW (kg).
Meat Quality
On day 60, one broiler per replicate was randomly slaughtered for the determination of the meat quality. The pH value was measured on the breast muscle at approximately the same location for each bird after 1 and 24 h of storage at 4°C post-slaughter using a pH meter (Mettler Toledo, Zurich, Switzerland). The pressing loss, drip loss, lightness (L*), redness (a*), yellowness (b*), and shear force were measured within 45 min immediately after euthanasia. The meat colors were determined by using a colorimeter (Minolta, Tokyo, Japan). A digital tenderness meter (C-LM3B, Tenovo, Beijing, China) was used to test the shear force of breast muscle samples. Drip loss was measured using a pressure gravimetric method described by Cheng et al. (2019).
Serum Antioxidation and Biochemistry
On day 28 and day 60, wing vein blood was drawn from one bird per replicate (six per treatment) and collected into 4-ml lithium-heparin anticoagulant tubes, followed by centrifuging at 3,000 rpm for 10 min. Serum was transferred into a 1.5-ml labeled sterile tube and frozen at −20°C for further analysis. The concentrations of total antioxidant capacity (T-AOC) and malondialdehyde (MDA) and the activities of superoxide dismutase (SOD) and catalase (CAT) in the serum were measured using commercial kits (Nanjing Jiancheng Institute of Bioengineering, Nanjing, China). The serum concentrations of immunoglobulin A (IgA), immunoglobulin G (IgG), immunoglobulin M (IgM), interleukin 2 (IL-2), interleukin 6 (IL-6), and interferon-γ (IFN-γ) were determined by ELISA kits (Abcam, Shanghai, China).
16S Sequencing and Cecal Microbiota Analysis
On day 28 and day 60, the cecal contents of the slaughtered birds from each treatment (one bird per replicate) were collected and placed in 10-ml sterile centrifuge tubes and stored at −80°C for determining the diversity index of the bacteria in the cecum. Total DNA of the microorganism genomes was extracted from cecal stools using a Stool DNA Extraction Kit (Omega Biotek, Norcross, GA, USA). The bacterial V3–V4 hypervariable regions of the 16S rRNA gene were amplified with forward primer F338 (50-ACTCCTACGGGAGGCAGCAG-30) and reverse primer R806 (50-GGACTACHVGGG TWTCTAAT-30). The PCR procedures typically included pre-denaturation at 95°C for 3 min; 27 cycles of denaturation at 95°C for 30 s, annealing at 55°C for 30 s, and elongation at 72°C for 45 s; and a final extension at 72°C for 10 min. Amplicons were extracted from 2% agarose gels using an AxyPrep DNA Gel Extraction Kit (Axygen Biosciences, Union City, CA, USA). The quantitated amplicons were pooled in equimolar concentrations and paired-end sequenced (2 × 300) on an Illumina MiSeq platform (Illumina, San Diego, CA, USA) according to the manufacturer's protocols. Raw FASTQ files were demultiplexed and quality-filtered using Quantitative Insights into Microbial Ecology (QIIME;Version 1.17; GitHub, San Francisco, CA, USA). Operational taxonomic units were clustered with a 97% similarity cutoff using UPARSE, and chimeric sequences were identified and removed using UCHIME. The taxonomy of each 16S rRNA gene sequence was analyzed using the Ribosomal Database Program (RDP) classifier (http://rdp.cme.msu.edu/) against the Silva (SSU128) 16S rRNA database using a confidence threshold of 80%.
Statistical Analysis
One-way analysis of variance (ANOVA) was applied to analyze the GCE dosage fixed effect and residue error random effect via SPSS 25.0 (SPSS Inc., Chicago, IL, USA). The statistical differences between groups were determined by the Duncan test. All data were presented as means ± SD. Values of p that were lower than 0.05 were considered as the significant and that were lower than 0.01 were considered the extremely significant.
Results
Growth Performance
The effects of dietary supplementation with GCE (0–2,000 mg/kg) on broiler growth performance are shown in Table 2. There was no significant difference in ADFI and FCR between different treatment groups (p > 0.05). However, there was a quadratic response on ADG (1–60 days) of birds (p = 0.022). Compared with the control group, the 250 mg/kg GCE treatment significantly increased the 1–60-day ADG (p = 0.037). In addition, the BW of birds at 60 days exhibited a significant quadratic response (p = 0.019), which increased first and then decreased with the increase of dietary GCE level. The results showed that the final BW of the 250 mg/kg GCE treatment (2.02 ± 0.11 kg) significantly increased (p = 0.032) compared with the control group (1.86 ± 0.07 kg).
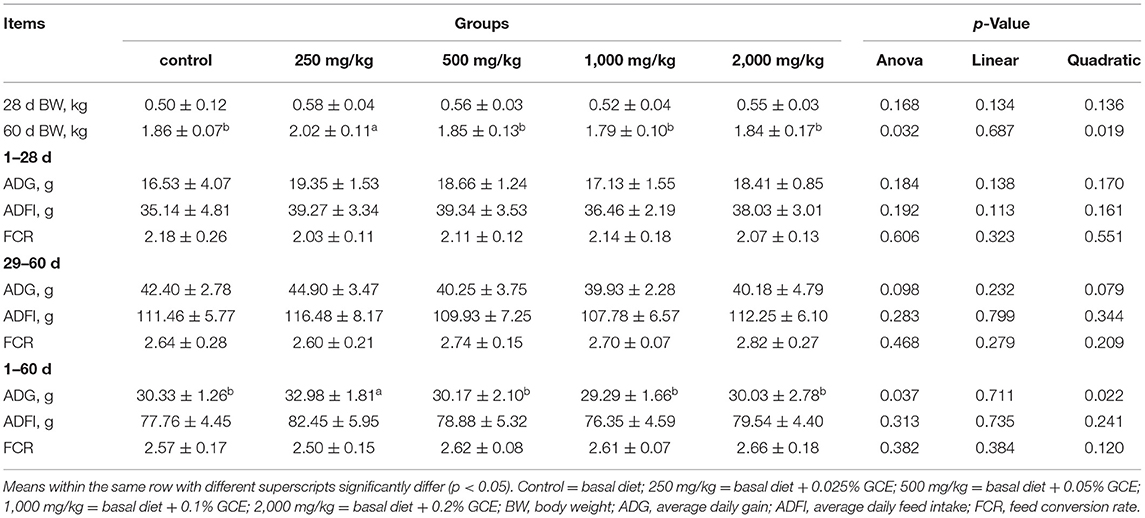
Table 2. Effects of dietary Galla chinensis extract (GCE) supplementation at different levels on growth performance of broilers.
Carcass Traits
Compared with the control group, GCE treatments had no effect on the yield of the full, semi-eviscerated or eviscerated carcass, abdominal fat, or breast or leg muscle (p > 0.05). The immune organ index results showed that dietary supplementation of GCE did not influence the relative weights of the spleen, cloacal bursa, thymus, or liver of the broilers (p > 0.05) (Table 3).
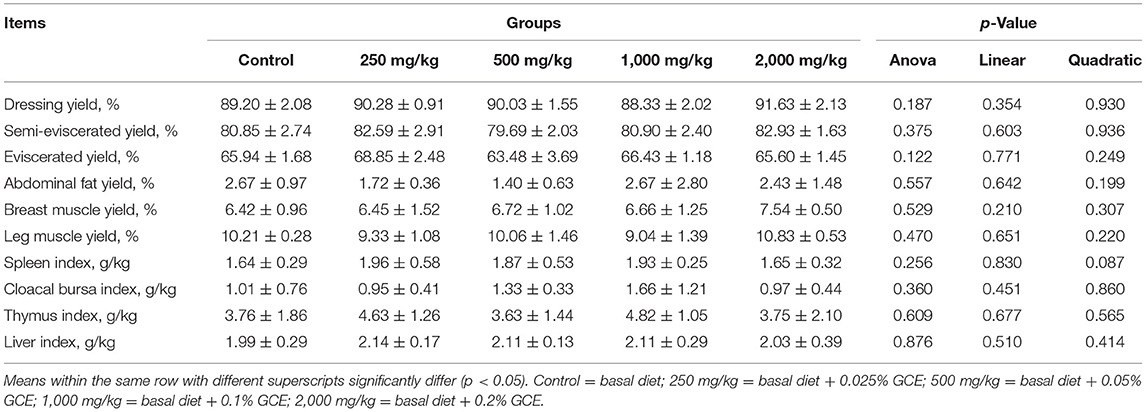
Table 3. Effect of dietary Galla chinensis extract (GCE) supplementation at different levels on carcass traits of broilers.
Meat Quality
The effects of dietary GCE supplementation on the breast meat quality of broilers on day 60 are presented in Table 4. No significant differences between treatments were observed for either the pressing loss, drip loss, or shear force of the meat, or the meat color or pH at 1 and 24 h post-slaughter (p > 0.05).
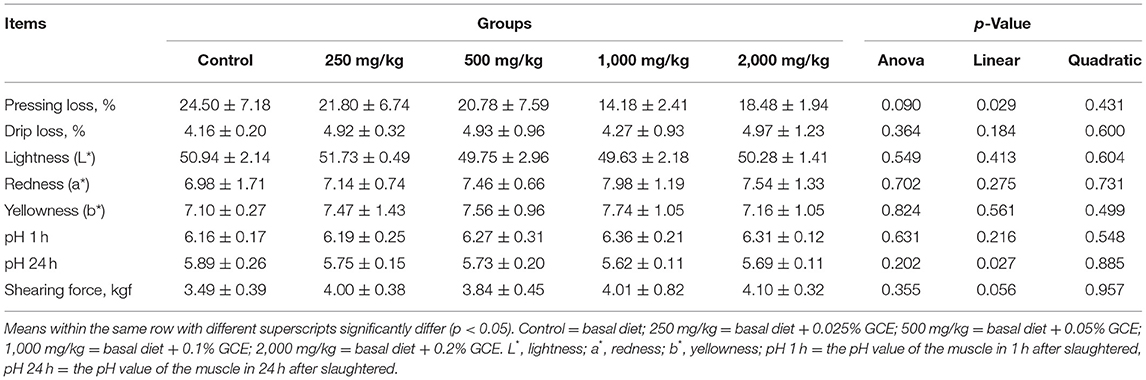
Table 4. Effect of dietary Galla chinensis extract (GCE) supplementation at different levels on meat quality of broilers.
Serum Antioxidative Capacity
The influence of GCE treatment on the serum antioxidative capacity of broilers is shown in Table 5. Compared with the control group, the GCE250 group significantly decreased the concentrations of MDA in the serum of the broilers at 60 days (p = 0.029). The SOD activity in the GCE250 and GCE500 supplementation groups showed an increasing trend with time (p = 0.069). There was no difference in the concentrations of T-AOC or the activity of CAT in the serum of the broilers between different treatment groups (p > 0.05).
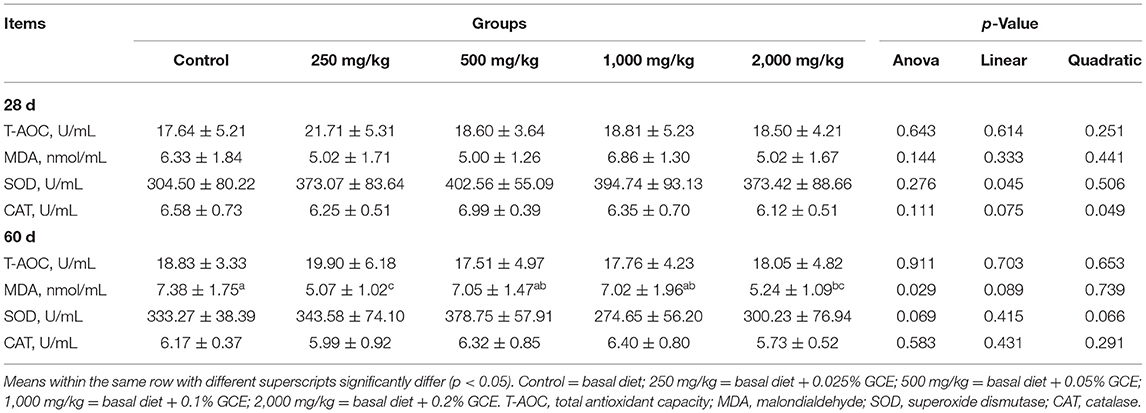
Table 5. Effect of dietary Galla chinensis extract (GCE) supplementation at different levels on serum antioxidation of broilers.
Serum Immune Factors and Immunoglobulins
Broiler serum levels of immune factors and immunoglobulins are shown in Table 6. No effect was found on the concentrations of IgA, IgG, and IgM in the serum among the different experimental groups. However, the serum concentrations of IL-6 and IFN-γ of the birds on day 28 in the GCE250 and GCE500 groups were significantly lower than those in the control group (p < 0.05). Additionally, on day 60, the serum concentration of IL-6 in the GCE250 and GCE500 groups was significantly decreased compared with the control group.
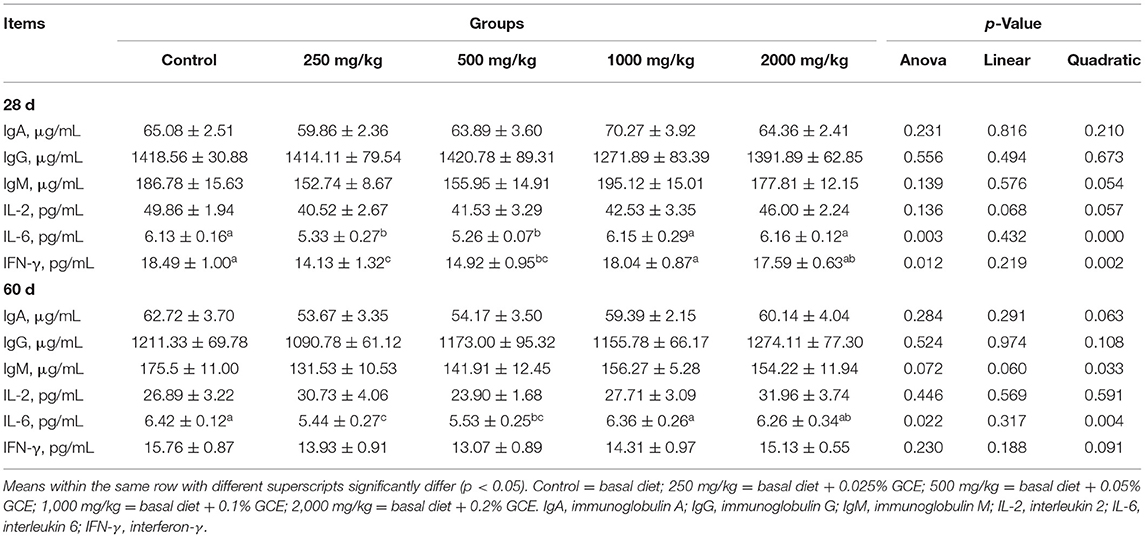
Table 6. Effect of dietary Galla chinensis extract (GCE) supplementation at different levels on serum immune indexes of broilers.
Cecal Microbiota
The relative abundances of cecal microbiota collected from different treatment groups at the phylum level are shown in Figure 1. Firmicutes and Bacteroides were the two dominant phyla in chicken microbiota throughout all groups. On day 28, Bacteroides was the dominant bacterial phylum in the control (60.18%) and GCE250 (53.52%) groups, followed by Firmicutes (control, 37.42%; and GCE250, 40.00%). On day 60, Firmicutes was the dominant bacterial phylum in all groups (control, 86.56%; GCE250, 59.30%; GCE500, 62.08%; and GCE1000, 67.95%; and GCE2000, 57.24%). The taxonomic composition of the cecal bacterial communities at the genus level in different groups by period is shown in Figure 2. On day 28, the dominant genus of the microbiota was Bacteroides (control, 59.87%; GCE250, 52.53%). However, the dominant genus of the microbiota on day 60 was Eisenbergiella (control, 6.86%) and Faecalibacterium (GCE250, 11.33%; GCE500, 19.71%; GCE1000, 30.11%; and GCE2000, 15.63%).
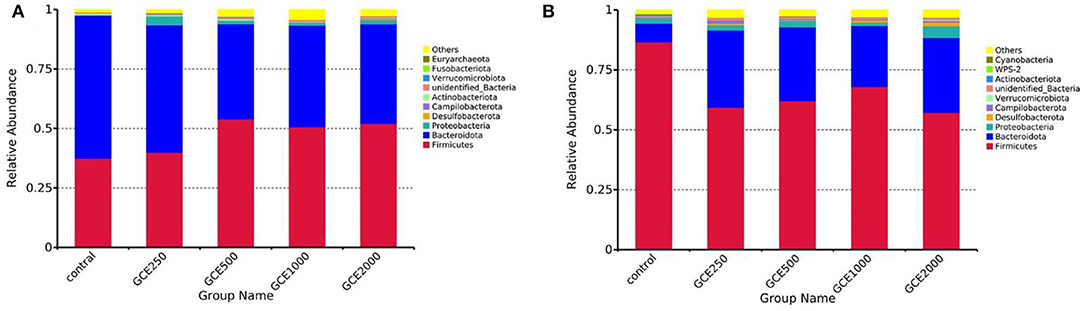
Figure 1. Phylum-level taxonomic composition of the cecal bacterial communities in different groups: (A) 28 days; (B) 60 days.
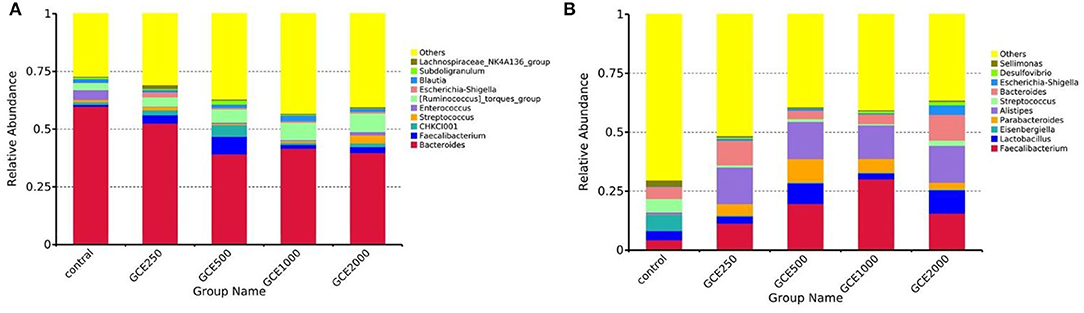
Figure 2. Taxonomic composition of the cecal bacterial communities at genus level in different groups: (A) 28 days; (B) 60 days.
The effects of dietary GCE supplementation on the alpha diversity indexes of cecal microbiota in broilers are presented in Table 7. On day 28, compared with the control, the Shannon and Simpson indexes were significantly increased (p = 0.020) in the GCE500, GCE1000, and GCE2000 groups. However, on day 60, the alpha diversity indexes of the cecal microbiota showed no significant differences between the groups (p > 0.05).
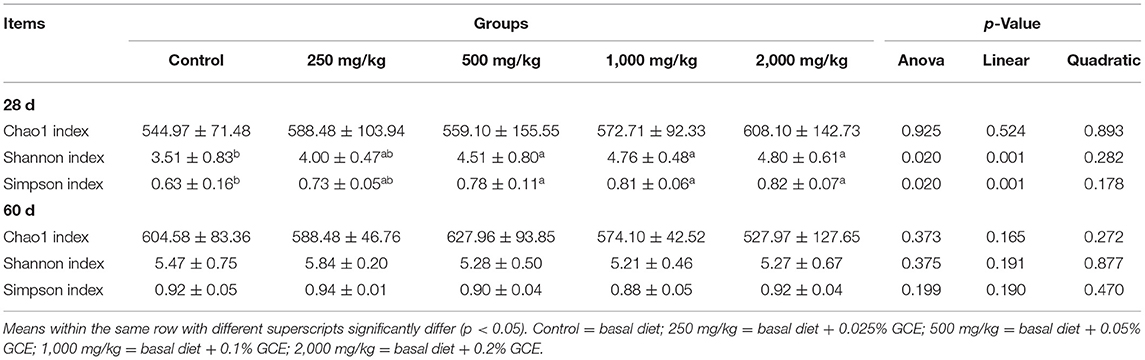
Table 7. Effect of dietary Galla chinensis extract (GCE) supplementation at different levels on alpha diversity indexes of cecal microbiota in broilers.
As shown in Figure 3, the principal coordinate analysis (PCoA) of the cecal microbiota in different groups using the Bray–Curtis similarity method revealed that the first and second principal components explained 31.10% and 11.35% of the variation among samples on day 28, respectively. However, all the samples in the different treatment groups were clustered close to each other on day 28. On day 60, it was clear that all GCE supplementation groups were clustered together and separate from the control group.
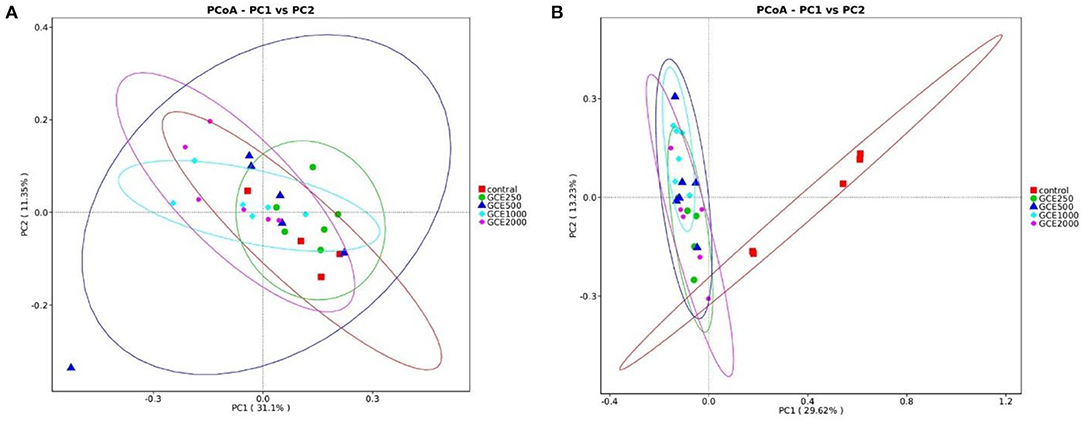
Figure 3. Principle coordinate analysis (PcoA) of broiler cecal bacterial communities at genus level in different groups: (A) 28 days; (B) 60 days.
Discussion
In our study, the dietary supplementation of GCE, which contains approximately 97% hydrolyzable tannin, was investigated for potential effects on growth performance, carcass traits, serum antioxidation, immune function, and gut microbiota of broiler chickens. Our results showed that the feed intake and feed conversion ratio did not change significantly at any concentration of GCE supplementation, although low-dosage supplementation of GCE (250 mg/kg) markedly increased the final BW on day 60. This result was consistent with previous findings that dietary supplementation of chestnut tannin could increase the final BW of broiler birds (Schiavone et al., 2008). It is not surprising that a low concentration of GCE might show a better outcome due to its high concentration of tannins, which may also produce antinutritional or even toxic effects, thus masking any positive consequences. Consistent with our findings, Liu et al. (2020) reported that dietary supplementation of 1,000 mg/kg chestnut wood extract (about 750 mg/kg tannin) showed no significant influence on the overall ADFI, average weight gain, or feed conversion ratio of broilers. In addition, Buyse et al. (2021) found that 500 mg/kg of chestnut tannin had little effect on the average daily weight gain and average feed intake at starter, grower, or finisher stages, while 2,000 mg/kg of chestnut tannin decreased the growth performance for broilers. However, in our research, 2,000 mg/kg of GCE supplementation showed a decreasing trend in chicken growth performance but was not significant.
The carcass traits represent the efficiency by which feed is converted into chicken products, such as breast muscles or leg muscles. However, the weight of immune and metabolic organs such as the spleen, thymus, bursa, and liver contribute to the final BW, but cannot be measured accurately until the birds are slaughtered. GCE supplementation in the chicken diet has been shown to increase the final BW. However, it is still necessary to understand whether the increased BW was from the increase in body meat content or from an increase in visceral organ weight. From our results, the dietary supplementation of GCE showed little effect on the carcass performance and immune organ index of broilers. GCE is abundant with polyphenol compounds such as tannin. Previous studies on tannin-treated birds showed no significant effect on carcass traits, including dressing out, carcass weight, eviscerate weight, and breast and thigh weights (Mannelli et al., 2019). Additionally, a meta-analysis that scanned 22 publications related to tannin or tannic acids in broilers showed that most studies found no significant influence of tannin supplementation on the thigh, abdominal fat, or liver weight, but might result in decreased bursa and spleen weight at higher concentrations (Hidayat et al., 2021).
Although the GCE supplementation decreased the level of MDA in broiler serum, it was not reflected in changes in the meat quality of broilers. In agreement, no changes in quality traits have been observed by Liu et al. (2020) and Buyse et al. (2021), with extracts chestnut extracts. MDA is a product generated from lipid peroxidation in cells and most often serves as a biomarker to measure the status of oxidative stress in an animal (Czerska et al., 2015). The antioxidative function of GCE was found to significantly decrease the level of MDA in broiler serum (Buyse et al., 2021). In our study, dietary supplementation of 250 mg/kg GCE showed a significant decline in the serum concentration of MDA compared with the control group. Gallic acid is the main downstream metabolite of gallotannin and was found to effectively alleviate cellular inflammation and lipid peroxidation due to its polyphenolic structure (Zhang et al., 2020; Nouri et al., 2021). Samuel et al. (2017) also showed that dietary supplementation of 100 mg/kg of gallic acid was able to significantly ameliorate the oxidative status of broiler chickens by decreasing the serum MDA level (Samuel et al., 2017). Additionally, with supplementation of low concentration GCE (250 mg/kg or 500 mg/kg) in chicken diets, the serum levels of pro-inflammatory factors such as IL-6 and IFN-γ were found in our study to significantly decline. Influenced by rapid meat accumulation and metabolic rates, broiler chickens are actually overloaded for meat production and, as a result, are prone to inflammatory and oxidative stress. Therefore, dietary supplementation of antioxidative and anti-inflammatory feed additives increases the ability of broilers to tolerate the metabolic burden caused by high energy uptake and improves their overall growth performance.
Many studies have shown that either tannic acid or its derivatives, such as gallic acid or gallotannins, provide strong antibacterial and anticoccidial effects on broilers (Reyes et al., 2017; Tonda et al., 2018; Mannelli et al., 2019). Thus, we speculated that the dietary supplementation of GCE might change the intestinal microbiota of broiler chickens. Interestingly, the alpha diversity, including the Shannon and Simpson indexes of the broiler microbiota at day 28, was significantly increased with GCE supplemented at more than 500 mg/kg, compared with that of the control group. Additionally, the taxonomic composition and PCoA analysis of cecal microbiota indicated that dietary supplementation of GCE over 250 mg/kg significantly changed the cecal microbial composition, which elevated the proportion of Bacteroidetes and reduced the proportion of Firmicutes; thus, the ratio of Firmicutes to Bacteroidetes was decreased. The high percentage of Firmicutes and high ratio of Firmicutes to Bacteroidetes were associated with excessive fat accumulation and higher inflammatory levels (Koliada et al., 2017; Indiani et al., 2018; Orbe-Orihuela et al., 2018). In our study, 250 mg/kg GCE significantly decreased serum pro-inflammatory factors such as IL-6 and INF-γ. Faecalibacterium is an anaerobic bacteria that can produce butyrate, and potentially exerts an influence on intestinal inflammation (Ferreira-Halder et al., 2017). Parabacteroides influences important anti-obesity and anti-inflammatory effects by modulating metabolic processes (Wang et al., 2019; Lai et al., 2022). Moreover, Alistipes is another member of the Bacteroidetes, which was found to have great benefits for intestinal lipid metabolism (Radka et al., 2020). Our results showed that the relative abundance of Faecalibacterium, Parabacteroides, and Alistipes in the cecum on day 60 was significantly increased when supplemented with 250–2,000 mg/kg GCE. Furthermore, potentially pathogenic bacteria, such as Streptococcus, remarkably decreased with GCE-supplemented diets, which was consistent with the previous findings that the tannin derivative gallic acid was able to suppress the growth of Streptococcus in vitro(Shao et al., 2015).
Conclusion
In this research, we found that a low concentration of GCE supplementation (250 or 500 mg/kg) provided better effects on growth performance, gut microbiota, serum antioxidation, and anti-inflammation in broiler chickens than high-dose GCE (1,000 or 2,000 mg/kg), suggesting that GCE could be a potentially effective growth promoter. Interestingly, all GCE supplementation doses were found to similarly change the cecal microbiota of the broiler chickens by increasing the relative abundance of beneficial bacteria, such as Faecalibacterium, Parabacteroides, and Alistipes, and decreasing the potentially pathogenic bacteria Streptococcus. These findings suggest that the ability of GCE to maintain gut health results from regulating gut microbiota. However, further research is still needed to elucidate the detailed mechanisms and explore the potential of Galla chinensis extract as an antimicrobial alternative.
Data Availability Statement
The datasets presented in this study can be found in online repositories. The names of the repository/repositories and accession number(s) can be found below: NCBI BioProject accession number: PRJNA808775.
Ethics Statement
The animal study was reviewed and approved by Institutional Animal Care and Use Committee of Hunan Agricultural University.
Author Contributions
HZ, XH, and ZS designed the research. XY, PD, JX, and YY worked on the animal handling and collected and processed the samples. YY provided the GCE product and supervised the mixture of GCE in the broiler diets. XY, HZ, and XH analyzed data, prepared the figures, and wrote the manuscript. All other authors reviewed, corrected, and approved the final manuscript.
Funding
This work was funded by the National Key R&D Program of Intergovernmental Key Projects of China (Grant No: 2018YFE0101700), the Support Plan for Scientific and Technological Innovation and Entrepreneurship Team of Enterprises in Hunan Province (2020), and the China Agriculture Research System of MOF and MARA (CARS-41-Z08).
Conflict of Interest
YY is employed by Jiurui Biology & Chemistry Co., Ltd.
The remaining authors declare that the research was conducted in the absence of any commercial or financial relationships that could be construed as a potential conflict of interest.
Publisher's Note
All claims expressed in this article are solely those of the authors and do not necessarily represent those of their affiliated organizations, or those of the publisher, the editors and the reviewers. Any product that may be evaluated in this article, or claim that may be made by its manufacturer, is not guaranteed or endorsed by the publisher.
Acknowledgments
We gratefully acknowledge Hunan Jitai Animal Husbandry Industry Co. Ltd. for providing experimental animals. We would also like to thank LetPub (https://www.letpub.com.cn) for English language editing.
References
BenSaad, L. A., Kim, K. H., Quah, C. C., Kim, W. R., and Shahimi, M. (2017). Anti-inflammatory potential of ellagic acid, gallic acid and punicalagin a&B isolated from Punica granatum. BMC Complement. Altern. Med. 17, 47. doi: 10.1186/s12906-017-1555-0
Brambila, G. S., Chatterjee, D., Bowker, B., and Zhuang, H. (2017). Descriptive texture analyses of cooked patties made of chicken breast with the woody breast condition. Poult Sci. 96, 3489–3494. doi: 10.3382/ps/pex118
Buyse, K., Delezie, E., Goethals, L., Van Noten, N., Ducatelle, R., Janssens, G., et al. (2021). Chestnut tannins in broiler diets: performance, nutrient digestibility, and meat quality. Poult Sci. 100, 101479. doi: 10.1016/j.psj.2021.101479
Cheng, Y., Chen, Y., Li, J., Qu, H., Zhao, Y., Wen, C., et al. (2019). Dietary beta-Sitosterol improves growth performance, meat quality, antioxidant status, and mitochondrial biogenesis of breast muscle in broilers. Animals (Basel) 9, 71. doi: 10.3390/ani9030071
Choinska, R., Dabrowska, K., Swislocka, R., Lewandowski, W., and Swiergiel, A. H. (2021). Antimicrobial properties of mandelic acid, gallic acid and their derivatives. Mini Rev. Med. Chem. 21, 2544–2550. doi: 10.2174/1389557521666210105123834
Czerska, M., Mikolajewska, K., Zielinski, M., Gromadzinska, J., and Wasowicz, W. (2015). Today's oxidative stress markers. Med. Pr. 66, 393–405. doi: 10.13075/mp.5893.00137
Estevez, M.. (2015). Oxidative damage to poultry: from farm to fork. Poult. Sci. 94, 1368–1378. doi: 10.3382/ps/pev094
Ferreira-Halder, C. V., Faria, A., and Andrade, S. S. (2017). Action and function of Faecalibacterium prausnitzii in health and disease. Best Pract. Res. Clin. Gastroenterol. 31, 643–648. doi: 10.1016/j.bpg.2017.09.011
Fleming, R. H.. (2008). Nutritional factors affecting poultry bone health. Proc. Nutr. Soc. 67, 177–183. doi: 10.1017/S0029665108007015
Hidayat, C., Irawan, A., Jayanegara, A., Sholikin, M. M., Prihambodo, T. R., Yanza, Y. R., et al. (2021). Effect of dietary tannins on the performance, lymphoid organ weight, and amino acid ileal digestibility of broiler chickens: a meta-analysis. Vet. World 14, 1405–1411. doi: 10.14202/vetworld.2021.1405-1411
Indiani, C., Rizzardi, K. F., Castelo, P. M., Ferraz, L., Darrieux, M., and Parisotto, T. M. (2018). Childhood obesity and Firmicutes/Bacteroidetes ratio in the gut microbiota: a systematic review. Child Obes. 14, 501–509. doi: 10.1089/chi.2018.0040
Jung, S., Choe, J. H., Kim, B., Yun, H., Kruk, Z. A., and Jo, C. (2010). Effect of dietary mixture of gallic acid and linoleic acid on antioxidative potential and quality of breast meat from broilers. Meat Sci. 86, 520–526. doi: 10.1016/j.meatsci.2010.06.007
Kamiloglu, S., Capanoglu, E., Bilen, F. D., Gonzales, G. B., Grootaert, C., Van de Wiele, T., et al. (2016). Bioaccessibility of polyphenols from plant-processing byproducts of black carrot (Daucus carota L.). J Agric Food Chem. 64, 2450–2458. doi: 10.1021/acs.jafc.5b02640
Koliada, A., Syzenko, G., Moseiko, V., Budovska, L., Puchkov, K., Perederiy, V., et al. (2017). Association between body mass index and Firmicutes/Bacteroidetes ratio in an adult Ukrainian population. BMC Microbiol. 17, 120. doi: 10.1186/s12866-017-1027-1
Lai, H. C., Lin, T. L., Chen, T. W., Kuo, Y. L., Chang, C. J., Wu, T. R., et al. (2022). Gut microbiota modulates COPD pathogenesis: role of anti-inflammatory Parabacteroides goldsteinii lipopolysaccharide. Gut. 71, 309–321. doi: 10.1136/gutjnl-2020-322599
Liu, H. S., Mahfuz, S. U., Wu, D., Shang, Q. H., and Piao, X. S. (2020). Effect of chestnut wood extract on performance, meat quality, antioxidant status, immune function, and cholesterol metabolism in broilers. Poult. Sci. 99, 4488–4495. doi: 10.1016/j.psj.2020.05.053
Mannelli, F., Minieri, S., Tosi, G., Secci, G., Daghio, M., Massi, P., et al. (2019). Effect of chestnut tannins and short chain fatty acids as Anti-Microbials and as feeding supplements in broilers rearing and meat quality. Animals (Basel) 9, 659. doi: 10.3390/ani9090659
Mishra, B., and Jha, R. (2019). Oxidative stress in the poultry gut: potential challenges and interventions. Front. Vet. Sci. 6, 60. doi: 10.3389/fvets.2019.00060
Moradi, A., Abolfathi, M., Javadian, M., Heidarian, E., Roshanmehr, H., Khaledi, M., et al. (2021). Gallic acid exerts nephroprotective, anti-oxidative stress, and anti-inflammatory effects against diclofenac-induced renal injury in malerats. Arch. Med. Res. 52, 380–388. doi: 10.1016/j.arcmed.2020.12.005
Nouri, A., Heibati, F., and Heidarian, E. (2021). Gallic acid exerts anti-inflammatory, anti-oxidative stress, and nephroprotective effects against paraquat-induced renal injury in male rats. Naunyn Schmiedebergs Arch. Pharmacol. 394, 1–9. doi: 10.1007/s00210-020-01931-0
Orbe-Orihuela, Y. C., Lagunas-Martinez, A., Bahena-Roman, M., Madrid-Marina, V., Torres-Poveda, K., Flores-Alfaro, E., et al. (2018). High relative abundance of firmicutes and increased TNF-alpha levels correlate with obesity in children. Salud Publica Mex. 60, 5–11. doi: 10.21149/8133
Osselaere, A., Santos, R., Hautekiet, V., De Backer, P., Chiers, K., Ducatelle, R., et al. (2013). Deoxynivalenol impairs hepatic and intestinal gene expression of selected oxidative stress, tight junction and inflammation proteins in broiler chickens, but addition of an adsorbing agent shifts the effects to the distal parts of the small intestine. PLoS ONE 8, e69014. doi: 10.1371/journal.pone.0069014
Radka, C. D., Frank, M. W., Rock, C. O., and Yao, J. (2020). Fatty acid activation and utilization by Alistipes finegoldii, a representative Bacteroidetes resident of the human gut microbiome. Mol. Microbiol. 113, 807–825. doi: 10.1111/mmi.14445
Raina, K., Rajamanickam, S., Deep, G., Singh, M., Agarwal, R., and Agarwal, C. (2008). Chemopreventive effects of oral gallic acid feeding on tumor growth and progression in TRAMP mice. Mol. Cancer Ther. 7, 1258–1267. doi: 10.1158/1535-7163.MCT-07-2220
Reyes, A., Hong, T. G., Hop, H. T., Arayan, L. T., Huy, T., Min, W., et al. (2017). The in vitro and in vivo protective effects of tannin derivatives against Salmonella enterica serovar Typhimurium infection. Microb Pathog. 109, 86–93. doi: 10.1016/j.micpath.2017.05.034
Romero-Montero, A., Del, V. L., Puiggali, J., Montiel, C., Garcia-Arrazola, R., and Gimeno, M. (2020). Poly(gallic acid)-coated polycaprolactone inhibits oxidative stress in epithelial cells. Mater. Sci. Eng. C. Mater. Biol. Appl. 115, 111154. doi: 10.1016/j.msec.2020.111154
Samuel, K. G., Wang, J., Yue, H. Y., Wu, S. G., Zhang, H. J., Duan, Z. Y., et al. (2017). Effects of dietary gallic acid supplementation on performance, antioxidant status, and jejunum intestinal morphology in broiler chicks. Poult. Sci. 96, 2768–2775. doi: 10.3382/ps/pex091
Schiavone, A., Guo, K., Tassone, S., Gasco, L., Hernandez, E., Denti, R., et al. (2008). Effects of a natural extract of chestnut wood on digestibility, performance traits, and nitrogen balance of broiler chicks. Poult. Sci. 87, 521–527. doi: 10.3382/ps.2007-00113
Shao, D., Li, J., Li, J., Tang, R., Liu, L., Shi, J., et al. (2015). Inhibition of gallic acid on the growth and biofilm formation of Escherichia coli and Streptococcus mutans. J. Food Sci. 80, M1299–M1305. doi: 10.1111/1750-3841.12902
Sihvo, H. K., Immonen, K., and Puolanne, E. (2014). Myodegeneration with fibrosis and regeneration in the pectoralis major muscle of broilers. Vet. Pathol. 51, 619–623. doi: 10.1177/0300985813497488
Starcevic, K., Krstulovic, L., Brozic, D., Mauric, M., Stojevic, Z., Mikulec, Z., et al. (2015). Production performance, meat composition and oxidative susceptibility in broiler chicken fed with different phenolic compounds. J Sci Food Agric. 95, 1172–1178. doi: 10.1002/jsfa.6805
Swiatkiewicz, S., Arczewska-Wlosek, A., and Jozefiak, D. (2017). The nutrition of poultry as a factor affecting litter quality and foot pad dermatitis - an updated review. J. Anim. Physiol. Anim. Nutr. (Berl). 101, e14–e20. doi: 10.1111/jpn.12630
Tonda, R. M., Rubach, J. K., Lumpkins, B. S., Mathis, G. F., and Poss, M. J. (2018). Effects of tannic acid extract on performance and intestinal health of broiler chickens following coccidiosis vaccination and/or a mixed-species Eimeria challenge. Poult. Sci. 97, 3031–3042. doi: 10.3382/ps/pey158
Ulluwishewa, D., Anderson, R. C., McNabb, W. C., Moughan, P. J., Wells, J. M., and Roy, N. C. (2011). Regulation of tight junction permeability by intestinal bacteria and dietary components. J. Nutr. 141, 769–776. doi: 10.3945/jn.110.135657
Veskoukis, A. S., Tsatsakis, A. M., and Kouretas, D. (2012). Dietary oxidative stress and antioxidant defense with an emphasis on plant extract administration. Cell Stress Chaperones 17, 11–21. doi: 10.1007/s12192-011-0293-3
Wang, J., Zhang, M., Gou, Z., Jiang, S., Zhang, Y., Wang, M., et al. (2020). The effect of camellia oleifera cake polysaccharides on growth performance, carcass traits, meat quality, blood profile, and caecum microorganisms in yellow broilers. Animals 10, 266. doi: 10.3390/ani10020266
Wang, K., Liao, M., Zhou, N., Bao, L., Ma, K., Zheng, Z., et al. (2019). Parabacteroides distasonis alleviates obesity and metabolic dysfunctions via production of succinate and secondary bile acids. Cell Rep. 26, 222–235. doi: 10.1016/j.celrep.2018.12.028
Xie, Q., Li, J-y., Zuo, Y-l., and Zhou, X-d. (2005). The effect of galla chinensis on the growth of cariogenic bacteria in vitro. Hua Xi Kou Qiang Yi Xue Za Zhi. 23, 82–84.
Xing, T., Pan, X., Zhang, L., and Gao, F. (2021). Hepatic oxidative stress, apoptosis, and inflammation in broiler chickens with wooden breast myopathy. Front. Physiol. 12, 659777. doi: 10.3389/fphys.2021.659777
Zhang, C., Zhao, X. H., Yang, L., Chen, X. Y., Jiang, R. S., Jin, S. H., et al. (2017). Resveratrol alleviates heat stress-induced impairment of intestinal morphology, microflora, and barrier integrity in broilers. Poult. Sci. 96, 4325–4332. doi: 10.3382/ps/pex266
Keywords: Galla chinesis extract, antioxidation, immune, microbiota, broiler
Citation: Yin X, Ding P, Xiao J, Yang Y, Song Z, He X and Zhang H (2022) Effects of Galla chinensis Extract on Growth Performance, Carcass Traits, Serum Antioxidation, Immune Function, and Gut Microbiota of Broilers. Front. Anim. Sci. 3:880237. doi: 10.3389/fanim.2022.880237
Received: 21 February 2022; Accepted: 31 March 2022;
Published: 20 May 2022.
Edited by:
Xixi Chen, Nutribins, LLC, United StatesReviewed by:
Sudhir Yadav, BioResource International, Inc., United StatesArgenis Rodas-Gonzalez, University of Manitoba, Canada
Copyright © 2022 Yin, Ding, Xiao, Yang, Song, He and Zhang. This is an open-access article distributed under the terms of the Creative Commons Attribution License (CC BY). The use, distribution or reproduction in other forums is permitted, provided the original author(s) and the copyright owner(s) are credited and that the original publication in this journal is cited, in accordance with accepted academic practice. No use, distribution or reproduction is permitted which does not comply with these terms.
*Correspondence: Haihan Zhang, emhob3VzJiN4MDAwNDA7MTYzLmNvbQ==; Xi He, aGV4aTExMSYjeDAwMDQwOzEyNi5jb20=