- 1Key Laboratory of Ministry of Education for Genetics and Germplasm Innovation of Tropical Special Trees and Ornamental Plants, Key Laboratory of Germplasm Resources of Tropical Special Ornamental Plants of Hainan Province, College of Forestry, Hainan University, Danzhou, China
- 2Tropical Crops Genetic Resources Institute, Chinese Academy of Tropical Agricultural Sciences, Danzhou, China
- 3Zhanjiang Experimental Station, Chinese Academy of Tropical Agricultural Sciences, Zhanjiang, China
In the present study, we aim to investigate the effects of lactic acid bacteria (LAB) and molasses (M) on the microbial community and fermentation performance of mixed silage of king grass (KG) and cassava foliage (CF). A completely randomized design was used for the experiment. Mixed material was ensiled with no additive added (CK) for 60 days. Alternatively, mixed silage was supplemented with M, LAB (L), or M + LAB (ML) and then subjected to fermentation. Compared with the CK group, the contents of lactic acid and propionic acid in the L group were enhanced, whereas the content of acetic acid was reduced. Moreover, the levels of pH, butyric acid, and ammonia-N were not significantly changed. In contrast, the lower contents of pH, acetic acid, propionic acid, and butyric acid, as well as ammonia-N in the M and ML groups were observed, whereas the content of lactic acid was elevated. Additives could change the silage quality of mixed silage to different extents. The effect of the L treatment was not ideal, and the ML group had a better fermentation quality compared with the M group. In terms of microbial community, the relative abundance of desirable Lactobacillus was increased in the M, L, and ML groups. The relative abundance of Pseudomonas was decreased in the M and L groups. Compared with the CK group, the relative abundance of Stenotrophomonas was decreased, especially in the M (0.18%) and ML (0.19%) groups. For Paenibacillus, its relative abundance was increased in the ML group and more significantly increased in the M group. In summary, the combination of LAB and M at an equal ratio had a more positive effect on the fermentation quality and microbial community of mixed silage than LAB and M alone.
Introduction
It is well known that king grass [KG; Pennisetum purpureum Schumacher × P. glaucum (Linnaeus) R. Brown] is characterized by high yield and good palatability. Thus, it becomes a popular feed resource for livestock in tropical and subtropical areas (Li et al., 2014). However, the quality of KG silage alone remains poor due to its low water-soluble carbohydrate (WSC) content and high-fiber content (Zhu et al., 2010), and KG silage possesses low protein content, which cannot meet the protein requirement of livestock. Cassava foliage (CF) (Manihot esculenta Crantz) is rich in protein and contains high gross energy and mineral elements such as calcium and phosphorus (Gómez and Valdivieso, 1985). Therefore, CF is a good source of tropical silage for livestock.
Both KG and CF are harvested in similar seasons. The mixture of these types of two forages can enhance the biomass yield of silage, reduce forage waste, and improve fermentation. Our previous research shows that supplementation of CF can ameliorate fermentation performance, chemical composition, and ruminal degradation of mixed silage, and the optimal ratio of CF to KG in the mixed silage is 50:50 (Li et al., 2019a). However, the raw material characteristics of the mixed silage are still unstable due to growth stage of plants, silage treatment methods, and other reasons, and there are many problems. In view of the above characteristics, we believe that lactic acid bacteria (LAB) and molasses (M) can further improve its fermentation quality. On the one hand, the addition of M can increase the content of crude protein (CP), reduce the content of fiber, and significantly improve the silage quality (Ni et al., 2017). On the other hand, when the abundance of LAB attached to the surface of raw materials is small, LAB inocula can ensure that the initial stage of silage can enter the lactic acid fermentation stage as soon as possible to further inhibit the reproduction of silage harmful microorganisms such as yeasts and aerobic bacteria and reduce the loss of dry matter (DM) and nutrients during ensiling (Wang et al., 2019b). Moreover, a previous study has shown that the mixed silage or LAB additives can enhance the quality of high-moisture Italian ryegrass silage via remodeling the microbiota community (Yan et al., 2019), and the silage quality of soybean is ameliorated when LAB and M are supplemented (Ni et al., 2017).
In the present study, we aim to enhance the fermentation performance of mixed silage of KG and CF and explore the effects of additives on its microorganisms. The mixed silage was supplemented with LAB and M, or their combination. Moreover, silage production is a microorganism-dependent fermentation course (Muck, 2013). However, the effect of LAB and/or M on the fermentation performance of KG and CF remains largely unclear.
Materials and methods
Material processing
The cassava and KG were cultivated in Chinese Academy of Tropical Agricultural Sciences (109°58′E, 19°52′N). The KG of the vegetative stage was collected, and the CF was harvested when the plants reached about 1.5 m in height and right away cut into segments of about 2–3 cm. LAB inocula (Lactobacillus plantarum, L) (Chikuso-1, Snow Brand Seed Co., Ltd., Sapporo, Japan) and M (Bayi Sugarcane Sugar Industry Co., Ltd., Danzhou, China) were used as additives for ensiling. Firstly, KG (100 g) and CF (100 g) were evenly mixed and then packed into a plastic film bag (50 × 20 × 10 cm; Guozhong Packing Co., Ltd., Haikou, China; Menghua Packing Co., Ltd., Guangzhou, China), and additives were supplemented according to four treatments: no additive control (CK), Lactobacillus plantarum was added at a level of 106 CFU/g FM (L), 2 g molasses per 100 FM (M), and M+L (ML). All additives were blended homogeneously with silage and sealed using a vacuum sealer (Jiaren Vacuum Sealer, Jiaren Home Electrical Appliance Co., Ltd., Wuhan, China). A total of 12 bags (four treatments × three replicates) were prepared and kept at ambient temperature (25°C–30°C) for ensiling. After 60 days of ensiling, these bags were opened, followed by assays of fermentation performance and chemical compositions.
Chemical analysis, fermentation, and microbial analysis
The collected samples were dried at 65°C for 72 h for the levels of DM content measurement and then ground to a fine powder, followed by chemical analysis. The assay of CP content was conducted by the Kjeldahl method (AOAC, A, 1990). The WSC content was determined according to the anthrone–sulphuric acid method (AOAC, A, 1990). Neutral detergent fiber (NDF) and acid detergent fiber (ADF) were determined using previously described approaches (He et al., 2020a). The fermentation performance of silage was examined as follows. Briefly, 50 g of wet silage was added to 200 ml of distilled water, and the mixture was stored at 4°C for 24 h and then filtered through four layers of cheesecloth for the determination of pH, organic acid, and ammonia-N. The levels of lactic acid, acetic acid, propionic acid, butyric acid, and ammonia-N were assessed using high-performance liquid chromatography (HPLC) equipped with the column of Shodex RSpak KC-811S-DVB gel C (8.0 mm × 30 cm; Shimadzu, Tokyo, Japan) under the setting conditions: oven temperature, 50°C; mobile phase, 3 mmol/L HClO4; flow rate, 1.0 ml/min; injection volume, 5 μl; detector, SPD-M10AVP (Li et al., 2019b). The concentration of ammonia-N was determined according to the method described by Broderick and Kang (1980). The plate counting method was adopted to determine the microbial population of fresh forage (Wang et al., 2019). The number of LAB, coliform bacteria, yeasts, and molds was counted on de Man–Rogosa–Sharpe agar, violet red bile agar, and Rose Bengal agar, respectively (Land Bridge Technology Co., Ltd., Beijing, China).
Microbial diversity analysis
DNA isolation and 16S rRNA gene sequencing
For the molecular analysis of the microbial communities, the E.Z.N.A.® Soil DNA Kit (Omega Bio-Tek, Norcross, GA, United States) was used to isolate microbial DNA from silage specimens. The concentration and purity of purified DNA were assessed by a NanoDrop 2000 UV-vis spectrophotometer (Thermo Scientific, Wilmington, United States), and the purified DNA was subjected to electrophoresis on 1% agarose gel to confirm the DNA integrity. The DNA specimens were amplified using primers targeting the V3–V4 regions of 16S rRNA genes (forward: 5’ACTCCTACGGGAGGCAGCAG-3’; reverse: 5’GGA CTACHVGGGTWTCTAAT-3’) (GeneAmp 9700, ABI, United States). PCR amplicons were excised, quantified, and subjected to next-generation sequencing on an Illumina MiSeq 2500 platform (Illumina, Inc., San Diego, CA, United States), and paired-end reads of 250 bp were yielded.
Processing and analysis of sequencing data
The original data (FLASH v.1.2.11) were spliced, the quality of the spliced sequence was filtered (Trimmomatic v.0.33), and the chimera was eliminated (UCHIME v.8.1) to yield high-quality reads. The sequences were clustered at the level of 97% similarity (USEARCH v.10.0), and the threshold to filter operational taxonomic unit (OTU) was set at 0.005% of sequenced sequences (Edgar, 2013). Diversity metrics were determined using the core-diversity plug-in within QIIME (Callahan et al., 2016). Moreover, α-diversity indices (MOTHUR v.1.30) were adopted to assess the microbial diversity within an individual sample. First, the structural variation of microbiota across specimens was determined using β-diversity, followed by principal components analysis (PCoA) (Vázquez-Baeza et al., 2013). Among samples and groups, linear discriminant analysis (LDA) effect size (LEfSe) was used to identify the bacteria with different abundances (Segata et al., 2011). Unless specified above, parameters used in the analysis were set as default. The sequencing data were deposited in the Sequence Read Archive (SRA) under the accession number PRJNA556208.
Statistical analysis
The impacts of additives (L and M) and their interactions during ensiling were assessed by two-way analysis of variance using the general linear model (GLM) procedure of SAS 9.3 software (SAS Institute Inc., Cary, NC, United States). Duncan’s multiple range tests were employed to compare the significant differences, and P < 0.05 was considered statistically significant.
Results and discussion
Characteristics of fresh material before ensiling
Table 1 shows the chemical composition and microbial population of fresh material. The DM content (14.79%) in mixed silage was lower, and the CP content (14.12%) was comparable to the previously reported values (Li et al., 2019a; Li et al., 2019c). In addition, the contents of NDF (60.30% DM) and ADF (39.85% DM) in mixed silage of KG and CF were higher compared with other reports (Li et al., 2019a; Dong et al., 2020; Zeng et al., 2020). On the other hand, the WSC content (6.03% DM) in mixed silage was between fresh CF (5.21%) (Li et al., 2020) and KG (7.21%) (Zi et al., 2021). Low DM might affect silage production since high water content often results in effluent loss and broad-scale clostridial fermentation (He et al., 2020b). This may lead to the loss of digestible nutrients of ruminants, and silage is perishable, but the addition of M can solve this problem using the high DM content (Palmonari et al., 2020).
The WSC plays an essential role in silage fermentation, and its content greater than 5% DM is the threshold of acceptable silage quality (Smith, 1962). In addition, the number of LAB for well-preserved silage should be higher than 105CFU/g FM (Cai et al., 1998). In the present study, the number of LAB in freshly mixed silage of KG and CF was 4.11 log10 CFU/g FM. However, the natural coliform population (0.98 log10 CFU/g FM), yeasts (2.32 log10 CFU/g FM), and molds (1.29 log10 CFU/g FM) in fresh materials were relatively high, and it could not ensure desirable silage quality, indicating that silage additives such as LAB were necessary for silage preparation.
Chemical composition and fermentation property during ensiling
Table 2 shows the organic acid contents, pH, and ammonia-N of mixed silage supplemented with L and M. In the L group, there was no apparent change in pH and ammonia-N, the levels of lactic acid and propionic acid were increased, the content of acetic acid was decreased, and there was still a tiny amount of butyric acid. In M and ML groups, the pH value and the contents of acetic acid and ammonia-N were significantly decreased, and the content of lactic acid was increased. In addition, the contents of propionic acid and butyric acid were reduced to 0 g/kg DM.
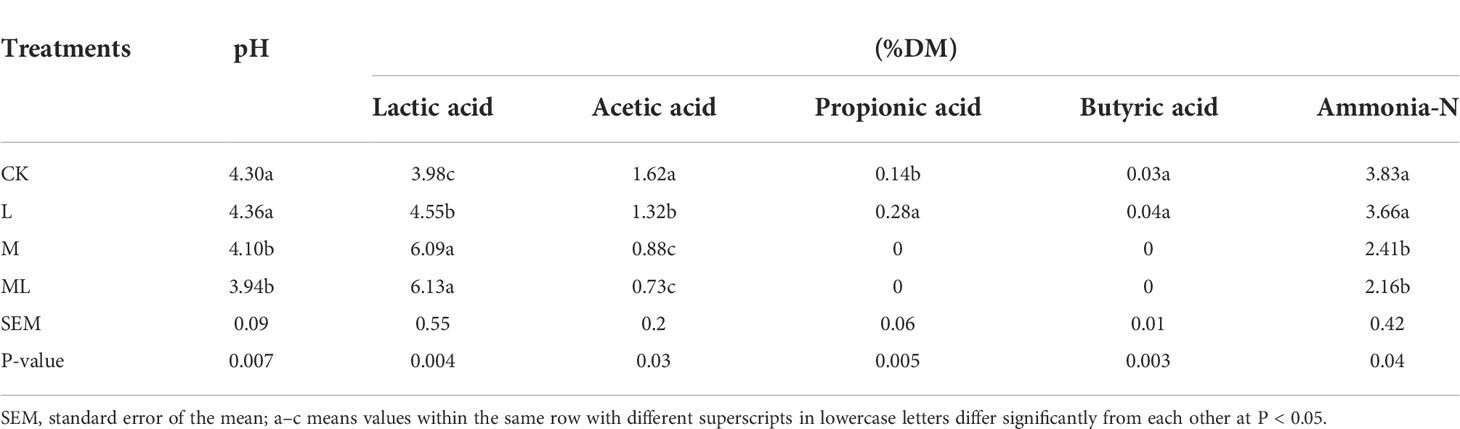
Table 2 Fermentation performance of mixed silage of KG and CF supplemented with L and M. (CK, control; L, lactic acid bacteria; M, molasses; ML, lactic acid bacteria + molasses).
pH plays an essential role in determining the fermentation performance of silage. The growth of harmful bacteria in silage can be inhibited once pH is lower than 4.20 (Wang et al., 2019), and the aerobic stability will be better (McDonald et al., 1991). The pH of the L group was 4.36. Therefore, yeasts, molds, and other aerobic microorganisms would use lactic acid and other nutrients for their reproduction in this group, which might further prevent the pH from decreasing (Woolford, 1990). Moreover, the high CP content (14.12%) of raw materials leads to high buffer capacity. The addition of L alone lacks sufficient WSC, which cannot ensure better fermentation quality (Ni et al., 2018). Our study confirmed this conclusion, showing that the pH of the LM group was significantly decreased compared with the CK group. The level of ammonia-N in silage remains the effective indicator of protein degradation (Pahlow et al., 2003). The lower its content, the less protein is decomposed. The plant protease activity and microbial activity exert a synthetic effect, leading to ammonia-N accumulation in silage. In addition, M and ML also decreased the ammonia-N content of mixed silage, which might be attributed to suppressed growth and proteolytic activity of microorganisms, such as Clostridia. The content of lactic acid was significantly increased in all three treatments, which was a good phenomenon.
L, M, and ML treatments all decreased the acetic acid content. Previous studies have shown that lower acetic acid content can improve energy utilization (Ni et al., 2017a). Propionic acid and butyric acid are undesirable in silage because part of metabolic energy is wasted for their generation. A previous investigation has shown that more than half of DM and some gross energy are lost when lactic acid is converted to butyric acid (Muck, 2010). Furthermore, the feed intake of livestock can be impaired by the overproduction of butyric acid (Dong et al., 2020). In the present study, the contents of propionic acid and butyric acid were decreased to 0 g/kg DM in M and ML treatments. There was also a tiny amount of butyric acid in the L group. Collectively, the fermentation quality of the M and ML groups was better, whereas that in the L group was not very ideal, and the ML group showed the best quality.
The effects of L and M treatments on the microbiota community of mixed silage
A total of 959,587 raw reads and 894,066 raw tags were generated, whereas the average number of clean tags and effective tags was 72,996 and 74,506, respectively, in each silage sample. Figure 1 indicates that 164 OTUs were identified. The L group (162) had the highest number of OTUs, whereas the M group (137) had the lowest number. In addition, the number of OTUs in the CK and ML groups was 159 and 139, respectively. Venn diagram analysis showed that the additive treatment led to 124 common OTUs, and there was one special OTU in the ML group. Additive treatment impaired the bacterial diversity indices, including Ace, Chao 1, Shannon, and Simpson (Figure 2). Ace and Chao 1 represent richness, which can reflect how many types of bacteria there are. Shannon and Simpson represent the degree of uniformity within the community. In terms of community richness, it could be ranked in descending order as follows: CK, L, ML, and M. In terms of community diversity, it could be ranked in descending order as follows: CK, L, M, and ML. The PCoA was used to explore the correlations among the community structures of the silage bacterial community. We found that the CK group was clearly separated from the other three groups (Figure 3). Figure 4A shows the bacterial community at the phylum level. Firmicutes and Proteobacteria were dominant in all groups. The sum of their relative abundance in the L group was not significantly different from the CK group. Bacteroidetes was subdominant in both the CK and L groups. However, the relative abundances of Firmicutes and Proteobacteria reached 99% in the M and ML groups. The relative abundance of Firmicutes in the L, M, and ML groups was elevated, whereas the relative abundances of Bacteroidetes and Proteobacteria were reduced compared with the CK group. Next, we further assessed the bacterial structures of mixed silage at the genus level (Figure 4B). Lactobacillus was found to be predominant in the four groups. The subdominant microbes, in turn, were Stenotrophomonas, Bacillus, and Pseudomonas in the CK group; Stenotrophomonas, Pantoea, and Escherichia-Shigella in the L group; Paenibacillus and Clostridium_sensu_stricto_3 in the M group; and Pseudomonas in the ML group. In addition, the relative abundance of Lactobacillus was enhanced long with the additive treatments, especially for the ML, which accounted for as high as 86% of the total population. However, the relative abundance of Pseudomonas was decreased in the L and M groups, while it was increased in the ML group. Furthermore, compared with the CK group, the relative abundance of Stenotrophomonas was decreased, especially in the M (0.18%) and ML (0.19%) groups. The relative abundance of Pantoea was increased in the L group, whereas it remained unchanged in the M group and was decreased in the ML group. For Paenibacillus, its relative abundance was increased in the ML (1.14%) group and more significantly increased in the M (9.90%) group. The differences in microbial community between four groups were assessed using the LEfSe method, and the specific bacterial strain in each group was explored (LDA score, >4.0). Figure 5 reveals that L significantly affected the microbial community. The most abundant species in the CK and L groups were Uncultured_bacterium_g_Lactobacillus and Lactobacillus_similis, respectively, which could be used as the biomarkers of them.
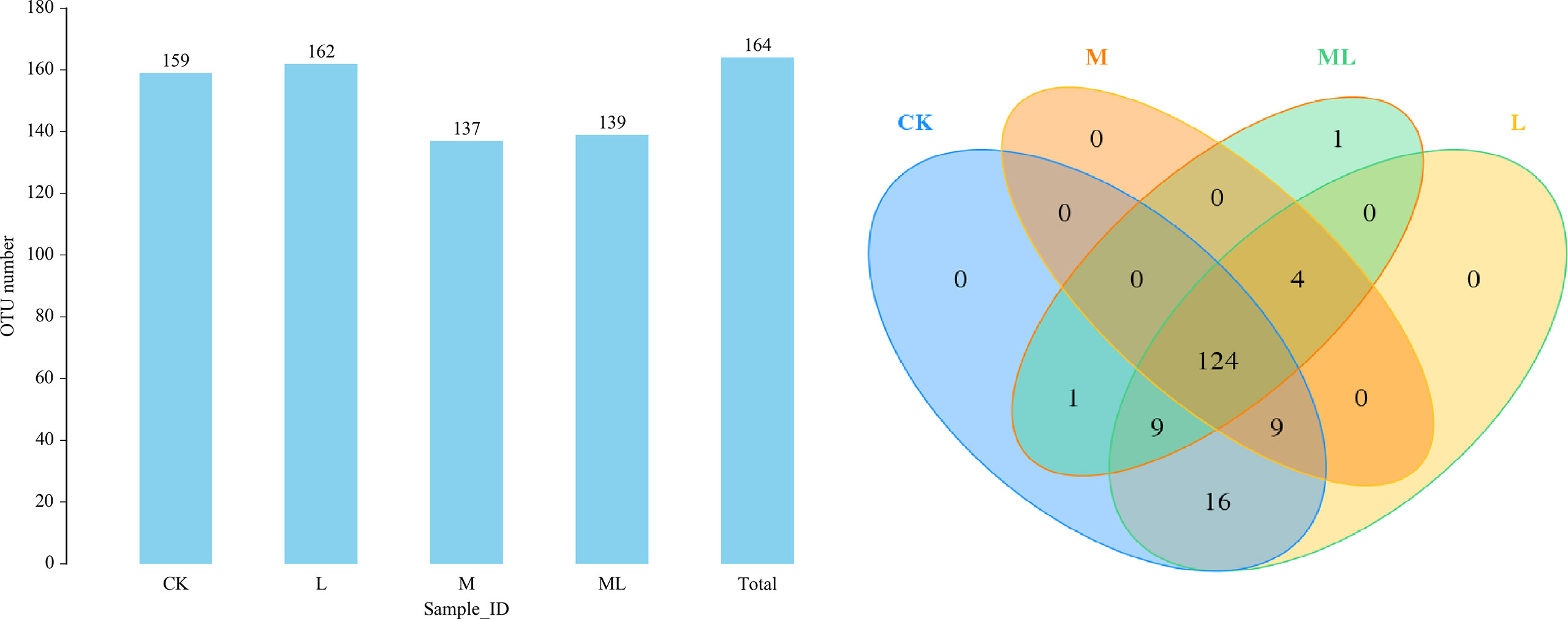
Figure 1 Venn analysis of OTUs for mixed silage of KG and CF supplemented with L and M. (CK, control; L, lactic acid bacteria; M, molasses; ML, lactic acid bacteria + molasses).
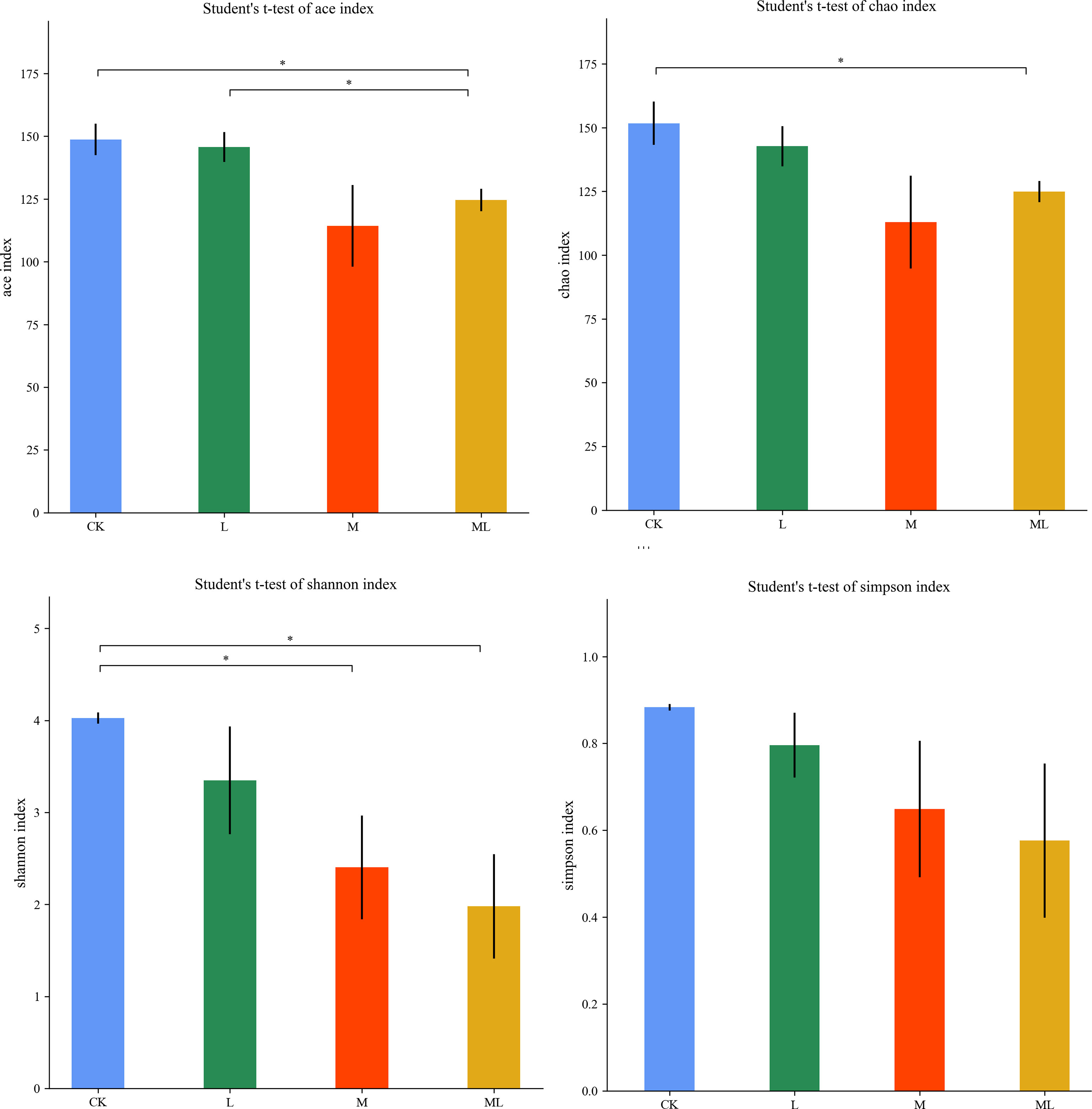
Figure 2 α-Diversity of bacterial diversity of mixed silage of KG and CF supplemented with L and M (CK, control; L, lactic acid bacteria; M, molasses; ML, lactic acid bacteria + molasses). *p < 0.05.
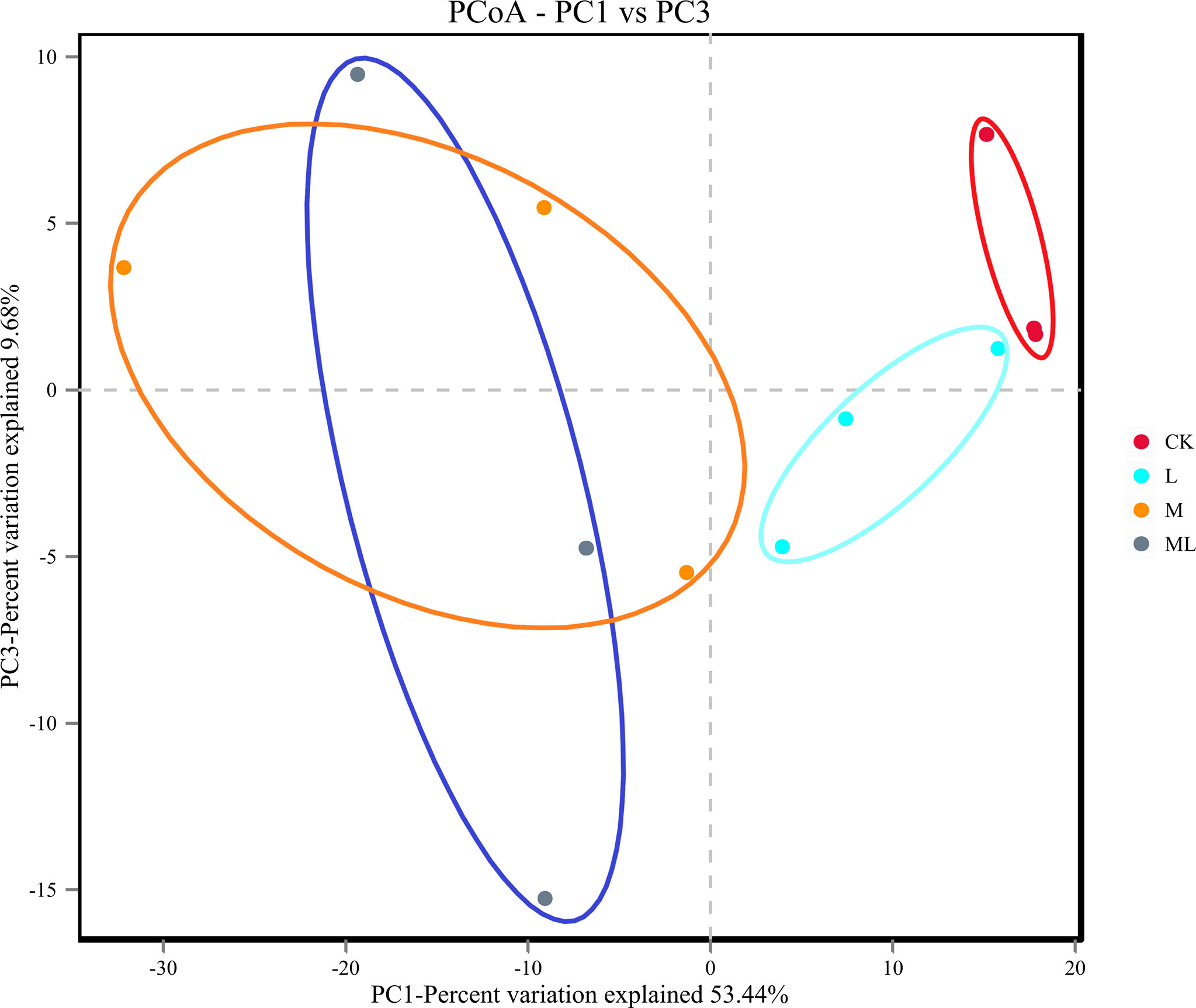
Figure 3 β-Diversity of bacterial diversity of mixed silage of KG and CF supplemented with L and M (CK, control; L, lactic acid bacteria; M, molasses; ML, lactic acid bacteria + molasses).
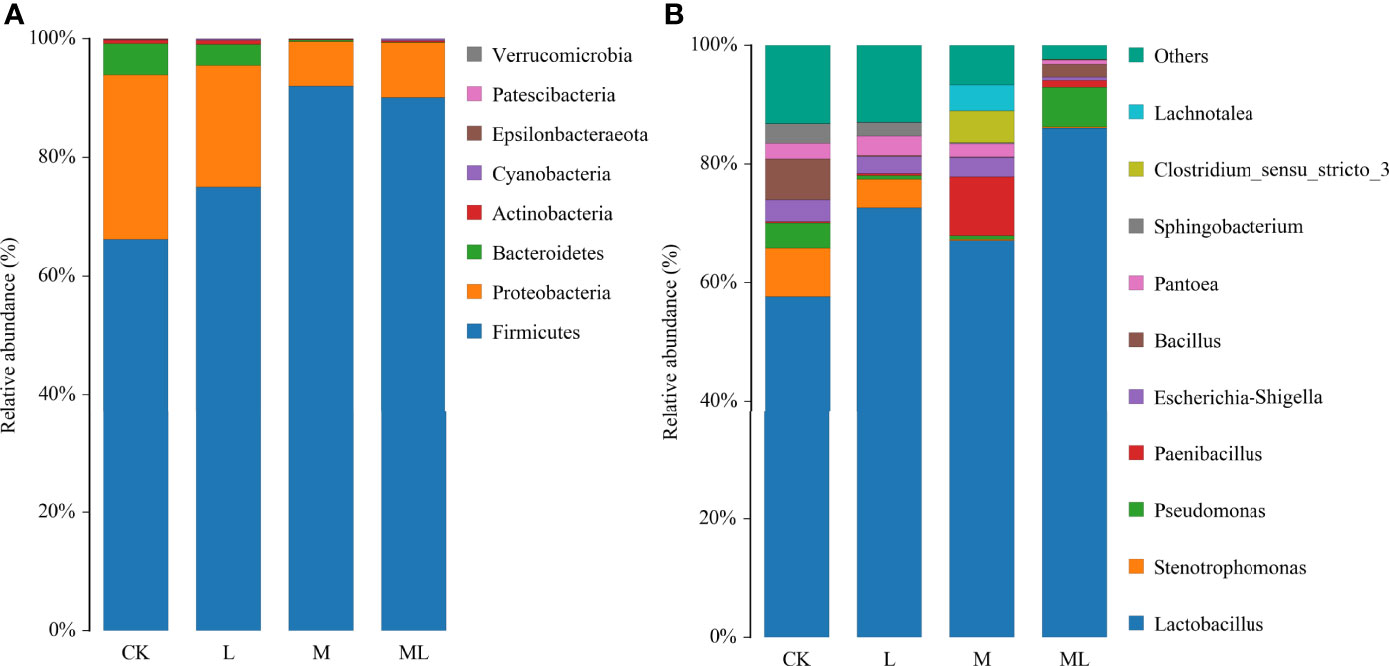
Figure 4 The bacterial relative abundance at the phylum and genus levels in mixed silage of KG and CF supplemented with L and M (CK, control; L, lactic acid bacteria; M, molasses; ML, lactic acid bacteria + molasses). (A) Relative abundance of bacteria at the phylum level. (B) Relative abundance of bacteria at the genus level.
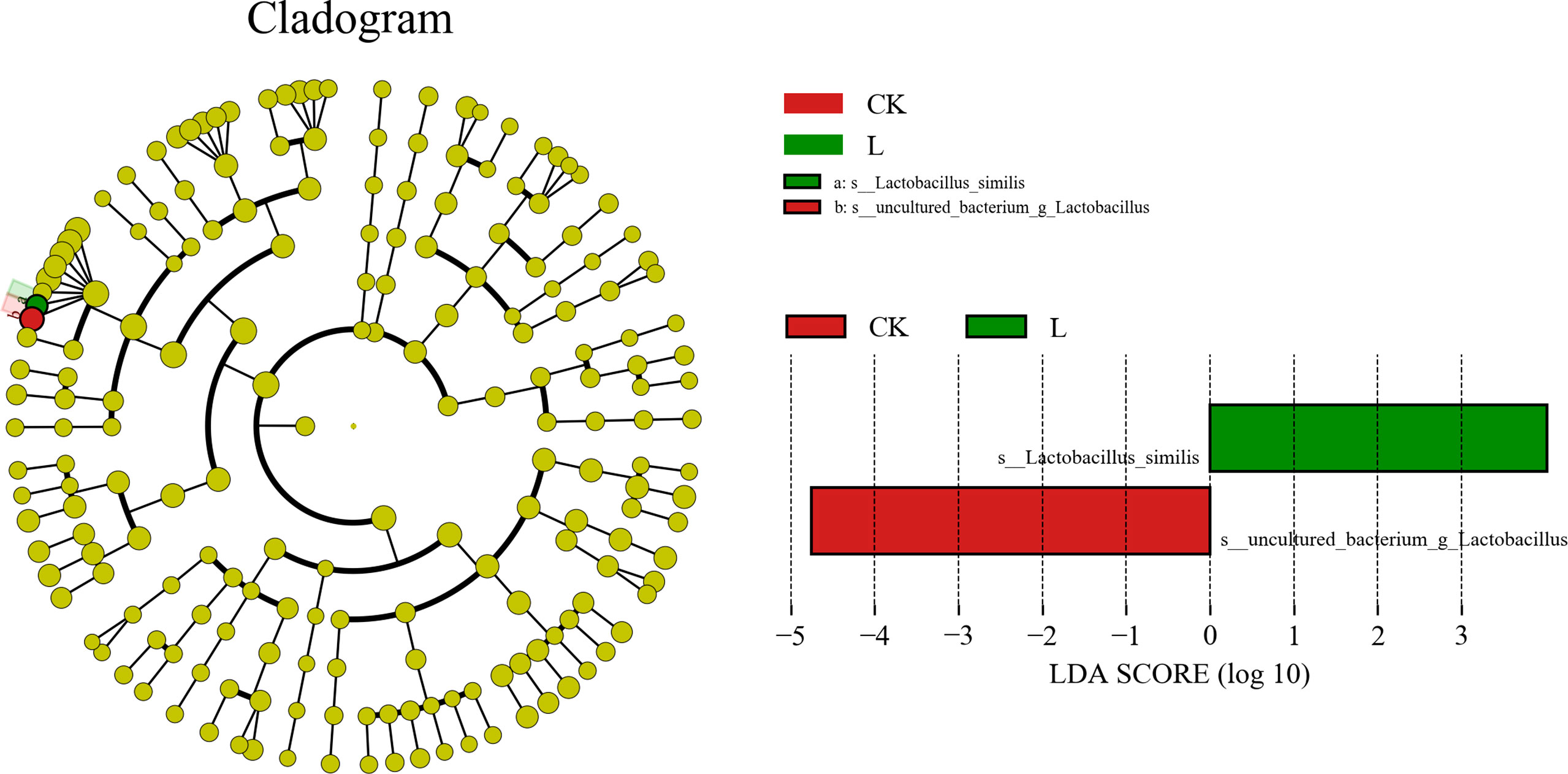
Figure 5 Comparison of microbial variations using the LEfSe online tool for mixed silage of KG and CF supplemented with L and M (CK, control; L, lactic acid bacteria; M, molasses; ML, lactic acid bacteria + molasses).
Moreover, the α-diversity showed that the relative abundance of the M group was the lowest, and the uniformity of the ML group was the lowest. It could be explained by that M provided a substrate for L to rapidly reduce pH in the ML group, inhibiting the growth of detrimental strains and enhancing the growth of LAB species. The bacterial diversity was reduced once LAB became the dominant species. Similar results have been documented in a previous study (Ni et al., 2017b). Meanwhile, PCoA showed that the bacterial composition of the L, M, and ML groups was altered in the ensiling process compared with the CK group. Thus, we could conclude that the bacterial diversity and community structure of mixed silage were impaired in the L, M, and ML groups. In our current work, Firmicutes and Proteobacteria were the top two dominant phyla in mixed silage, which was consistent with previous findings (Yuan et al., 2020). At the genus level, Lactobacillus is normally dominant in well-preserved forage silage because it can drive lactic fermentation during ensiling (Liu et al., 2019). In the present experiment, the relative abundance of Lactobacillus was particularly elevated in the ML group, indicating that M could provide additional substrates to LAB and accelerate its growth, whereas the supplementation of L also enhanced the relative abundance of desirable microorganisms. Paenibacillus, facultatively anaerobic, is a beneficial bacterial strain in silage and can produce lactic acid by using different types of sugars (Ash et al., 1993). Moreover, it also contributed to the significant elevation (P < 0.01) of lactic acid content in the M and ML groups. In contrast, Stenotrophomonas, aerobic or facultative anaerobic non-fermenting Gram-negative bacteria, can use different types of sugars and yield acids (Palleroni and Bradbury, 1993), and it may compete with LAB for substrates and affect their growth and reproduction. In the M and ML groups, the relative abundance of Stenotrophomonas was significantly decreased, which was a good sign, suggesting better fermentation quality. Pseudomonas is an aerobic bacterium and undesirable microorganism for the same reason (Dong et al., 2019). However, a previous study (Ogunade et al., 2018) has examined the bacterial diversity of alfalfa silage and shown that the content of ammonia-N is negatively correlated with the relative abundances of Pantoea, Stenotrophomonas, and Pseudomonas, revealing that the presence of the abovementioned species may be beneficial for protein preservation. The relative abundance of Pseudomonas was elevated after 60 days of ensiling in the ML group, which might partly explain the lower ammonia-N content in inoculated silages. The role of Pantoea in silage fermentation still remains largely unclear (Li et al., 2017), though it was undesirable for the substrate competition with LAB. This conclusion could be supported by the fermentation quality of this experiment.
Conclusions
Compared with the CK group, the addition of L enhanced the contents of lactic acid and propionic acid, whereas the content of acetic acid was reduced, and the levels of pH, butyric acid, and ammonia-N remained barely changed. Moreover, the addition of M and ML reduced the levels of pH, propionic acid, and butyric acid, and the contents of acetic acid and ammonia-N were also decreased, whereas the content of lactic acid was enhanced. Additives could change the silage quality of mixed silage of KG and CF to different extents. The effect of the L group was not good, and the ML group had a better fermentation quality compared with the M group. Besides, the relative abundance of desirable Lactobacillus was increased in the M, L, and ML groups. The relative abundance of Pseudomonas was decreased in the M and L groups. In addition, compared with the CK group, the relative abundance of Stenotrophomonas was decreased, especially in the M (0.18%) and ML (0.19%) groups. For Paenibacillus, its relative abundance was increased in the ML group and more significantly increased in the M group.
Collectively, the combination of M and L could efficiently enhance the silage quality of KG and CF.
Data availability statement
The datasets presented in this study can be found in online repositories. The names of the repository/repositories and accession number(s) can be found below: https://www.ncbi.nlm.nih.gov/bioproject/PRJNA556208.
Author contributions
ML and XZ did the experimental design work. YL, TC, RS, XZ, and ML conducted the experiments. YL, ML, and XZ analyzed the data. YL, ML, and XZ wrote and revised the manuscript. All authors read and approved the manuscript.
Funding
This study was funded by Hainan Province (No. 2019RC145, 322RC774, GHYF2022004), the Ministry of Agriculture and Rural Affairs of the People’s Republic of China (No.16220078) and Integrated Demonstration of Key Techniques for the Industrial Development of Featured Crops in Rocky Desertification Areas of Yunnan-Guangxi -Guizhou Provinces.
Conflict of interest
The authors declare that the research was conducted in the absence of any commercial or financial relationships that could be construed as a potential conflict of interest.
Publisher’s note
All claims expressed in this article are solely those of the authors and do not necessarily represent those of their affiliated organizations, or those of the publisher, the editors and the reviewers. Any product that may be evaluated in this article, or claim that may be made by its manufacturer, is not guaranteed or endorsed by the publisher.
References
AOAC, A (1990). In Helrich K. (Ed.), Official methods of analysis.(15th ed.). (Arlington, VA, United States.).
Ash C., Priest F. G., Collins M. D. (1993). Molecular identification of rRNA group 3 bacilli (Ash, farrow, wallbanks and Collins) using a PCR probe test. Antonie van Leeuwenhoek 64, 253–260. doi: 10.1007/BF00873085
Broderick G., Kang J. (1980). Automated simultaneous determination of ammonia and total amino acids in ruminal fluid and in vitro Media1. J. Dairy Sci. 63 (1), 64–75. doi: 10.3168/jds.S0022-0302(80)82888-8
Cai Y., Benno Y., Ogawa M., Ohmomo S., Kumai S., Nakase T. (1998). Influence of lactobacillus spp. from an inoculant and of weissella and leuconostoc spp. from forage crops on silage fermentation. Appl. Environ. Microbiol. 64 (8), 2982–2987. doi: 10.1128/AEM.64.8.2982-2987.1998
Callahan B., McMurdie P., Rosen M., Han A. W., Johnson J. A., and Holmes S. P. (2016). DADA2: High-resolution sample inference from illumina amplicon data. Nat. Methods 13, 581–583. doi: 10.1038/nmeth.3869
Dong Z., Li J., Chen L., Wang S., Shao T. (2019). Effects of freeze–thaw event on microbial community dynamics during red clover ensiling. Front. Microbiol. 10. doi: 10.3389/fmicb.2019.01559
Dong L., Zhang H., Gao Y., Diao Q. (2020). Dynamic profiles of fermentation characteristics and bacterial community composition of broussonetia papyrifera ensiled with perennial ryegrass. Bioresource Technol. 310, 123396. doi: 10.1016/j.biortech.2020.123396
Edgar R. C. (2013). 2013 UPARSE: highly accurate OTU sequences from microbial amplicon reads. Nat. Methods 10, 996–998. doi: 10.1038/nmeth.2604
Gómez G., Valdivieso M. (1985). Cassava foliage: Chemical composition, cyanide content and effect of drying on cyanide elimination. J. Sci. Food Agric. 36 (6), 433–441. doi: 10.1002/jsfa.2740360602
He L., Lv H., Xing Y., Wang C., You X., Chen X., et al. (2020a). The nutrients in moringa oleifera leaf contribute to the improvement of stylo and alfalfa silage: Fermentation, nutrition and bacterial community. Bioresource Technol. 301, 122733. doi: 10.1016/j.biortech.2020.122733
He L., Zhou W., Xing Y., Pian R., Chen X., Zhang Q. (2020b). Improving the quality of rice straw silage with moringa oleifera leaves and propionic acid: Fermentation, nutrition, aerobic stability and microbial communities. Bioresource Technol. 299, 122579. doi: 10.1016/j.biortech.2019.122579
Liu B., Huan H., Gu H., Xu N., Shen Q., Ding C. (2019). Dynamics of a microbial community during ensiling and upon aerobic exposure in lactic acid bacteria inoculation-treated and untreated barley silages. Bioresource Technol. 273, 212–219. doi: 10.1016/j.biortech.2018.10.041
Li L., Yuan Z., Sun Y., Kong X., Dong P., Zhang J. (2017). A reused method for molasses-processed wastewater: Effect on silage quality and anaerobic digestion performance of pennisetum purpereum. Bioresource Technol. 241, 1003–1011. doi: 10.1016/j.biortech.2017.04.117
Li P., Zhang Y., Gou W., Cheng Q., Bai S., Cai Y. (2019c). Silage fermentation and bacterial community of bur clover, annual ryegrass and their mixtures prepared with microbial inoculant and chemical additive. Anim. Feed. Sci. Technol. 247, 285–293. doi: 10.1016/j.anifeedsci.2018.11.009
Li M., Zhang L., Zhang Q., Zi X., Lv R., Tang J., et al. (2020). Impacts of citric acid and malic acid on fermentation quality and bacterial community of cassava foliage silage. Front. Microbiol. 11. doi: 10.3389/fmicb.2020.595622
Li M., Zi X., Tang J., Zhou H. (2019a). Silage fermentation, chemical composition and ruminal degradation of king grass, cassava foliage and their mixture. Grassl. Sci. 65 (4), 210–215. doi: 10.1111/grs.12235
Li M., Zi X., Zhou H., Hou G., Cai Y. (2014). Effects of sucrose, glucose, molasses and cellulase on fermentation quality and in vitro gas production of king grass silage. Anim. Feed. Sci. Technol. 197, 206–212. doi: 10.1016/j.anifeedsci.2014.06.016
Li M., Zi X., Zhou H., Lv R., Tang J., Cai Y. (2019b). Silage fermentation and ruminal degradation of cassava foliage prepared with microbial additive. AMB. Expr. 9, 180. doi: 10.1186/s13568-019-0906-2
Muck R. (2010). Silage microbiology and its control through additives. Revista Brasileira de Zootecnia [online]39, 183–191. doi: 10.1590/S1516-35982010001300021
Muck R. (2013). Recent advances in silage microbiology. Food Sci. 22 (1), 3–15. doi: 10.23986/afsci.6718
Ni K., Wang F., Zhu B., Yang J., Zhou G., Pan Y., et al. (2017). Effects of lactic acid bacteria and molasses additives on the microbial community and fermentation quality of soybean silage. Bioresource Technol. 238, 706–715. doi: 10.1016/j.biortech.2017.04.055
Ni K., Zhao J., Zhu B., Su R., Pan Y., Ma J., et al. (2018). Assessing the fermentation quality and microbial community of the mixed silage of forage soybean with crop corn or sorghum. Bioresource Technol. 265, 563–567. doi: 10.1016/j.biortech.2018.05.097
Ogunade I., Jiang Y., Cervantes A. P., Kim D., Oliveira A., Vyas D., et al. (2018). Bacterial diversity and composition of alfalfa silage as analyzed by illumina MiSeq sequencing: Effects of escherichia coli O157: H7 and silage additives. J. Dairy Sci. 101 (3), 2048–2059. doi: 10.3168/jds.2017-12876
Pahlow G., Muck R. E., Driehuis F., Elferink S., Spoelstra S. (2003). Microbiology of Ensiling. In Silage Science and Technology (eds D.R. Buxton, R.E. Muck and J.H. Harrison). 42, 31–93. doi: 10.2134/agronmonogr42.c2
Palmonari A., Cavallini D., Sniffen C. J., Fernandes L., Holder P., Fagioli L., et al. (2020). Short communication: Characterization of molasses chemical composition. J. Dairy Sci. 103 (7), 6244–6249. doi: 10.3168/jds.2019-17644
Segata N., Izard J., Waldron L., Gevers D., Miropolsky L., Garrett W. S., et al. (2011). 2011 Metagenomic biomarker discovery and explanation. Genome Biol. 12, R60. doi: 10.1186/gb-2011-12-6-r60
Smith L. H. (1962). Theoretical carbohydrate requirement for alfalfa silage production. Agron. J. 54, 291–293. doi: 10.2134/agronj1962.00021962005400040003x
Vázquez-Baeza Y., Pirrung M., Gonzalez A., Knight R. (2013). EMPeror: a tool for visualizing high-throughput microbial community data. GigaScience 2 (1), 2047-2217X-2042-2016. doi: 10.1186/2047-217X-2-16
Wang Y., He L., Xing Y., Zhou W., Pian R., Yang F., et al. (2019). Bacterial diversity and fermentation quality of moringa oleifera leaves silage prepared with lactic acid bacteria inoculants and stored at different temperatures. Bioresource Technol. 284, 349–358. doi: 10.1016/j.biortech.2019.03.139
Yan Y., Li X., Guan H., Huang L., Ma X., Peng Y., et al. (2019). Microbial community and fermentation characteristic of Italian ryegrass silage prepared with corn stover and lactic acid bacteria. Bioresource Technol. 279, 166–173. doi: 10.1016/j.biortech.2019.01.107
Yuan X., Li J., Dong Z., Shao T. (2020). The reconstitution mechanism of napier grass microiota during the ensiling of alfalfa and their contributions to fermentation quality of silage. Bioresource Technol. 297, 122391. doi: 10.1016/j.biortech.2019.122391
Zeng T., Li X., Guan H., Yang W., Liu W., Liu J., et al. (2020). Dynamic microbial diversity and fermentation quality of the mixed silage of corn and soybean grown in strip intercropping system. Bioresource Technol. 313, 123655. doi: 10.1016/j.biortech.2020.123655
Zhu Y., Nishino N., Xusheng G. (2011). 2010 Chemical changes during ensilage and in sacco degradation of two tropical grasses: Rhodesgrass and guineagrass treated with cell wall-degrading enzymes. Anim. Biosci. 24 (2), 214–221. doi: 10.5713/ajas.2011.10170
Keywords: LAB, molasses, king grass, cassava foliage, mixed silage, bacterial community, fermentation quality
Citation: Liu Y, Chen T, Sun R, Zi X and Li M (2022) The effects of lactic acid bacteria and molasses on microbial community and fermentation performance of mixed silage of king grass and cassava foliage. Front. Anim. Sci. 3:879930. doi: 10.3389/fanim.2022.879930
Received: 20 February 2022; Accepted: 27 June 2022;
Published: 25 July 2022.
Edited by:
Qing Zhang, South China Agricultural University, ChinaReviewed by:
Suban Foiklang, Maejo University, ThailandYimin Cai, Japan International Research Center for Agricultural Sciences (JIRCAS), Japan
Copyright © 2022 Liu, Chen, Sun, Zi and Li. This is an open-access article distributed under the terms of the Creative Commons Attribution License (CC BY). The use, distribution or reproduction in other forums is permitted, provided the original author(s) and the copyright owner(s) are credited and that the original publication in this journal is cited, in accordance with accepted academic practice. No use, distribution or reproduction is permitted which does not comply with these terms.
*Correspondence: Xuejuan Zi, eml4dWVqdWFuQDE2My5jb20=; Mao Li, bGltYW9obkAxNjMuY29t