- 1Key Laboratory of Ministry of Education for Genetics and Germplasm Innovation of Tropical Special Trees and Ornamental Plants, Key Laboratory of Germplasm Resources of Tropical Special Ornamental Plants of Hainan Province, College of Forestry, Hainan University, Danzhou, China
- 2Tropical Crops Genetic Resources Institute, Chinese Academy of Tropical Agricultural Sciences, Danzhou, China
- 3Zhanjiang Experimental Station, Chinese Academy of Tropical Agricultural Sciences, Zhanjiang, China
The fermentation quality and microbial diversity of king grass (K), cassava foliage (C), and Broussonetia papyrifera (B) ensiled in the absence of an inoculant (K, C, B) or the presence of Lactobacillus plantarum (KL, CL, BL) for 60 days were investigated. The bacterial community was characterized by using the 16S rDNA sequencing technology. The relative abundance of Lactobacillus in K was very high, and it decreased after adding L. plantarum while Acinetobacter increased to some extent. The relative abundance of Lactobacillus in group C was also very high, and the inoculant L. plantarum enriched it in the CL group. As the second dominant genus of group C, the relative abundance of Pseudomonas decreased significantly in CL. Weissella and Enterobacter were the dominant genera in B and BL, and the relative abundance of Lactobacillus decreased in BL. For K, C, and B, the inoculant L. plantarum decreased the pH value and NH3-N content markedly, inhibited the production of butyric acid, increased the content of lactic acid, and significantly improved the fermentation quality. In conclusion, L. plantarum affected the bacterial community of C and improved the silage quality of K, C, and B to a certain extent.
Introduction
Hainan island has a tropical monsoon climate, which is warm and humid all year round, resulting in difficulties in hay preparation. Silage can preserve the fresh plant feed for a long time, reduce nutrient loss, and facilitate animal digestion and absorption (Kung et al., 2018). Silage of excess forage is of great significance to balance the seasonal supply of livestock feed.
King grass [Pennisetum purpureum Schumacher × P. glaucum (Linnaeus) R. Brown, K], belonging to Pennisetum, is widely used as animal feed in tropical and subtropical regions due to its high biomass yield, nutritional values, and palatability in China (Zi et al., 2021). Cassava (Manihot esculenta Crantz, C) is a major tropical food crop in the Euphorbiaceae family because of its high protein content and gross energy; its foliage becomes the high-quality protein feed resource in the tropical areas in China (Napasirth et al., 2015). Using stems and leaves of Cassava to develop ruminant feed can not only reduce the feed cost but also enrich roughage resources and make full use of resources. However, the growth of K depends on high temperature and a rainy environment, resulting in a surplus in summer and a serious shortage in winter (Li et al., 2014). In addition, C is harvested seasonally and needs to be ensiled for long-term preservation. Hence, it is necessary to prepare silage for feeding ruminants in winter. In the tropical gramineous forage, the water-soluble carbohydrates (WSCs) and dry matter (DM) content are low, so it is difficult to produce high-quality silage (Li et al., 2014). At the same time, the content of crude protein (CP) of K is relatively low while fiber is high, which also limits its application in ruminant production (Zhao et al., 2019). Broussonetia papyrifera L.Vent. (B) is a fast-growing tree species, belonging to the Moraceae family, and it is widely distributed in Southeast Asia (Tang et al., 2021). It has high nutritional value, strong adaptability, high contents of crude protein and crude fat, and low contents of crude fiber and is rich in various amino acids. It is a high-quality unconventional feed resource. The protein content of B is so high that it has been used as a protein source in animal feed, such as chicken (Zheng et al., 2019) and pig (Wüstholz et al., 2017). However, the content of WSC in B is very low. With its high-buffer capacity, direct silage is difficult to succeed. Inoculants are needed to improve fermentation quality. Adding exogenous microbial agents, especially lactic acid bacteria (LAB), is the most convenient and effective way to improve the silage quality of K. Inoculation of exogenous LAB can inhibit the fungi and aerobic bacteria attached to the forage itself, which are conducive to silage fermentation. In addition, for the problem of low WSC content, it is necessary to select homozymic lactic acid bacteria with higher fermentation efficiency (Muck et al., 2018, p. 3980–4000). The homofermentative lactic acid bacteria represented by L. plantarum grow and reproduce rapidly. In the early stage of fermentation, they can quickly produce lactic acid (LA) and reduce pH value, so the fermentation system can quickly form an acidic environment, inhibit the growth and reproduction of harmful microorganisms, and effectively maintain feed nutrition. At present, researchers have inoculated L. plantarum in whole crop corn silage (Wang et al., 2018), sugarcane silage (Rabelo et al., 2019), and Moringa oleifera leaf silage (Wang et al., 2018). Among them, the research on leaves of sugarcane and Moringa oleifera shows that the fermentation effect is better without adding L. plantarum; instead, the effect of L. plantarum as an inoculant of mixed silage of high momentum amaranth and rice straw is ideal. Inoculants increased the relative abundance of Lactobacillus, reduced the relative abundance of Weissella, Pediococcus, Lactococcus, decreased pH, acetic acid (AA), and NH3-N, and increased LA concentration as compared with no addition silage over the ensiling period (Mu et al., 2020).
However, few studies have focused on the effects of L. plantarum on K, C, and B silages. Therefore, in this study, we evaluated the silage effect of K, C, and B in response to the addition of L. plantarum in terms of chemical composition, fermentation characteristics, and microbial community.
Materials and Methods
Silage Preparation
K (Reyan No. 4), C (South China No. 7), and B were cultivated in the experimental base of the Chinese Academy of Tropical Agricultural Sciences (19°58′E, 19°52′N). The first cut K of the vegetative stage (~1.5–1.8 m in height) was harvested and chopped into about 2 cm pieces by a grass-shredding machine, and then C and B in the growing stage were cut in the same manner (Donghong No. 1, Donghong Mechanical Equipment Co., Ltd., China). The number of L (Snow Brand Seed Co., Ltd., Japan) was 1.0 × 105 CFU/g (Zi et al., 2021).
In total, six different treatments were carried out in our current research as follows: B: Broussonetia papyrifera, BL: Broussonetia papyrifera + L. plantarum, C: cassava foliage, CL: cassava foliage + L. plantarum, K: king grass, and KL: king grass + L. plantarum. According to the manufacturer's guidelines, the inoculants were dissolved in sterile distilled water and then mixed thoroughly with the grass. An equal amount of sterile distilled water was added to the control group. Every treatment was carried out in triplicate. Briefly, 200 g of K, C, and B was blended with inoculants, and the mixture was placed in plastic bags (35 × 12 × 5 cm; Guozhong Packing Co., Ltd., Haikou, China). A total of 18 bags (six treatments × three replicates) were sealed by a vacuum packaging machine (DZQ-380B; Ansheng Machinery Co., Ltd., Quanzhou, China) and stored at normal temperature (25~30°C). The chemical composition, organic acid, and microbial community were determined after 30 days of fermentation.
Chemical and Microbial Analysis
Samples were oven-dried at 65°C for 72 h to determine the DM content, and dried materials were ground for chemical analysis. CP and ether extract (EE) were determined according to the Guidelines of the Association of Official Analytical Chemists (AOAC, 2005). The concentration of neutral detergent fiber (NDF) and acid detergent fiber (ADF) and the content of WSC were determined as described previously (He et al., 2020).
The fermentation quality of silage was determined using distilled water extracts. Briefly, 50 g wet silage was blended with 200 ml distilled water, followed by incubation at 4°C for 24 h and then filtration. The pH (Thunder magnetic phs-3c pH meter) and concentrations of LA, AA, propionic acid (PA), butyric acid (BA), and NH3-N were measured as established previously (Mao et al., 2014).
Microbial counts were analyzed using the plate count method as described previously (Li et al., 2018). Briefly, 20 g silage samples were mixed with 180 ml sterilized saline, and then LAB, coliform, yeasts, and molds were enumerated on Man, Rogosa, and Sharpe (MRS) agar, violet red bile agar, and rose Bengal agar, respectively.
Microbial Diversity Analyses
DNA Extraction and 16S rRNA Gene Sequencing
The aforementioned extracts were used for the molecular analysis of the microbiota. Using the E.Z.N.A.® Soil DNA Kit (Omega Bio-Tek, Norcross, GA, United States) to isolate microbial DNA from silage specimens according to the instructions of the manufacturer. The V3-V4 hypervariable region of the bacterial 16S rRNA gene was amplified with primers 338F (5'-CCTACGGGAGGCAGCAG-3') and 806R (5'-GGACTACHVGGGTWTCTAAT-3') by thermocycler PCR system (Gene Amp 9700, ABI, United States). PCR products were purified and quantified, next-generation sequencing was carried out on an Illumina MiSeq 2500 platform (Illumina, Inc., San Diego, CA, United States), and 250-bp paired-end reads were generated.
Processing and Analysis of Sequencing Data
The filtered reads were assembled into tags with the principles as follows: an overlap between paired-end reads that should be more than 10 bp and <2% mismatch and redundant tags was removed by software MOTHUR (v.1.30) to obtain unique tags (Schloss et al., 2009). Then, the resultant unique tags were employed to determine the relative abundance. The high-quality sequences were clustered into operational taxonomic units (OTUs) defined at a similarity of 97%. Using the core-diversity plug-in within QIIME21 to determine diversity metrics (Callahan et al., 2016). The microbial diversity within an individual sample was assessed using the α-diversity indices, including observed OTUs, Chao1 richness estimator, Shannon diversity index, and Simpson and ACE indices. β-diversity was analyzed to assess the structural variation of microbiota across specimens, and then principal coordinates analysis (PCoA) was determined (Vázquez-Baeza et al., 2013). Among samples and groups, appropriate methods, such as LefSe, were employed to identify the bacteria with different relative abundance (Segata et al., 2011). Unless specified earlier, parameters used in the analysis were set as default. The sequencing data were deposited in the Sequence Read Archive (SRA) under the accession number PRJNA556216.
Statistical Analysis
The impacts of L. plantarum were investigated using a two-way ANOVA in SAS 9.3 software (SAS Institute Inc., Cary, NC, United States). Duncan's multiple range test was adopted to identify significant differences, and P < 0.05 was considered statistically significant.
Results and Discussion
Chemical and Microbial Compositions of K, C, and B
Table 1 shows the chemical and microbial compositions of C, K, and B. In this study, for C and K, the concentration of NDF and ADF were higher compared with previous reports, while the contents of DM, OM, CP, and ether extract (EE) were lower or comparable to the previous reports (Li et al., 2019). For B, the level of CP was comparable to the previous reports, and the level of NDF was higher compared with previous reports, while the levels of ADF and DM were lower (Si et al., 2018). The content of WSC plays a significant role in assessing fermentation quality. The content of WSC was 5.21, 7.21, and 6.43% in C, K, and B in this study. This can ensure the fermentation quality in theory (Ni et al., 2018). However, in addition to WSC, LAB quantity also needs to reach the threshold. Well-preserved silage needs no less than 105 CFU/g FM of LAB (Cai et al., 1998). The number of LAB, coliform, yeasts, and mold in the fresh C was 3.89, 1.53, 2.06, and 0 Log10 CFU/g FM, respectively. It was 4.22, 1.77, 2.78, and 3.04 Log10 CFU/g FM, respectively, in fresh K and 3.52, 2.08, 2.63, and 0.78 Log10 CFU/g FM, respectively, in fresh B. Compared with harmful bacteria, LAB were not dominant in quantity among the three forages, at the beginning of ensiling, yeasts would grow and compete for energy materials with LAB and could not ensure good silage quality, illustrating that silage inoculants, such as LAB inoculants, were imperative for silage.
Fermentation Quality of K, C, and B
Table 2 provides the fermentation characteristics of three species of tropical forages. For fermentation quality, the pH of ensiling is the most important key index. The pH was remarkably decreased after fermentation in CL, KL, and BL compared with the control groups. However, the pH values of the inoculant-treated groups were ≥4.2, and related research have indicated a similar pH of L. plantarum treatment (Yang et al., 2019; Ren et al., 2020). The reason may be that there was not enough WSC for LAB growth and reproduction, no more LA was produced, and the pH value cannot be reduced to a lower level (Yan et al., 2019). In addition, inoculants reduced the contents of NH3-N compared with the control group. NH3-N is a universal index of protein hydrolysis, and it usually reflects the levels of peptide bond hydrolysis and amino acid or peptide deamination. The smaller the value, the less the protein is decomposed, which means a better storage quality (Wang et al., 2019). The remarkably decreased content of NH3-N in the inoculant-treated silage might be a result of lower pH values, which could inhibit the activities of some harmful bacteria and proteases and effectively conserve nutrients (Wang et al., 2019).
In CL, the content of LA was remarkably elevated, and there was no significant change in acetic acid (AA), besides the content of PA in the inoculant-treated groups reduced by about a fifth compared with the control group. Furthermore, the content of BA was reduced to 0. In KL, the content of LA, AA, and PA was similar to the control group, and BA was never detected. In BL, the content of LA was remarkably increased while AA was significantly decreased than the control group, and there was no significant change in PA. Furthermore, the content of BA was never detected. Furthermore, the content of BA was reduced to 0 in the treatment of all three treatments, manifesting that K, C, and B treated by L. plantarum were well-preserved, which was consistent with a previous study (Lv et al., 2020).
These results revealed that the addition of L. plantarum in the ensiling process could efficiently promote the fermentation quality.
Microbial Community of K, C, and B Silages
A total of 1,439,466 raw reads were obtained. After splicing and filtering, 1,311,196 clean tags were generated. Each sample produced at least 69,299 clean tags, with an average of 72,844 clean tags.
Table 3 presents the α-diversity of the bacterial diversity of K, C, and B silages. The average Good's coverage for all samples was over 0.99, suggesting that the sequencing process was sufficient to describe the changes in the bacterial community. Shannon and Simpson stand for diversity, and Chao1 and ACE represent richness. Figure 1 is principal coordinates analysis (PCoA), i.e., principal coordinate analysis, which is a non-constrained data dimensionality reduction analysis method, which can be used to study the similarity or dissimilarity of sample community composition. It was used to search the relevance among the community structures of bacteria during ensiling. Next, the changes in the bacterial community during fermentation were evaluated by a high-throughput sequencing technique based on the 16S ribosomal DNA gene. Figure 2 presents the relative abundance of bacterial communities in C, K, and B. The linear discriminant analysis (LDA) effect size (LEfSe) method was used in Figure 3 to examine the discrepancies of microbial communities between groups and probe the specific bacteria in each group (LDA score > 4.0).
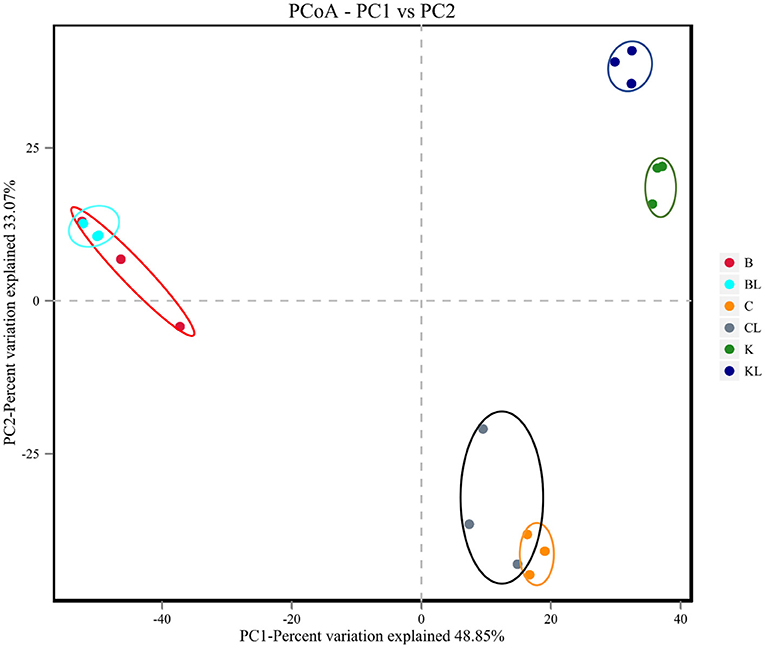
Figure 1. Principal coordinates analysis (PCoA) of the bacterial community of C, K, and B (B, Broussonetia papyrifera; BL, Broussonetia papyrifera+ L. plantarum; C, cassava foliage; CL, cassava foliage+ L. plantarum; K, king grass; KL, king grass+ L. plantarum).
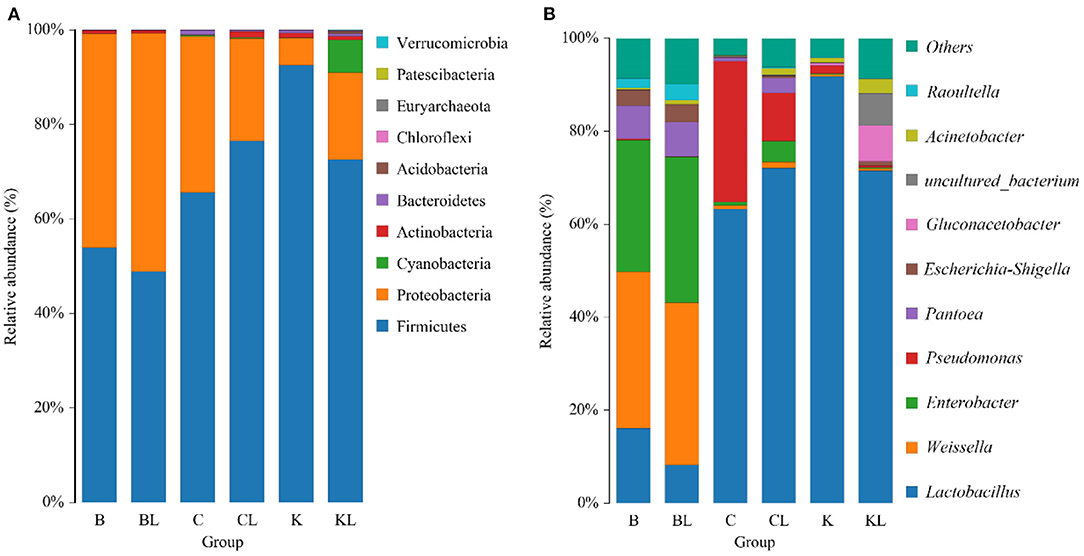
Figure 2. The Silage bacterial community at the phylum (A) and genus (B) levels of C, K, and B (B, Broussonetia papyrifera; BL, Broussonetia papyrifera+ L. plantarum; C, cassava foliage; CL, cassava foliage+ L. plantarum; K, king grass; KL, king grass+ L. plantarum).
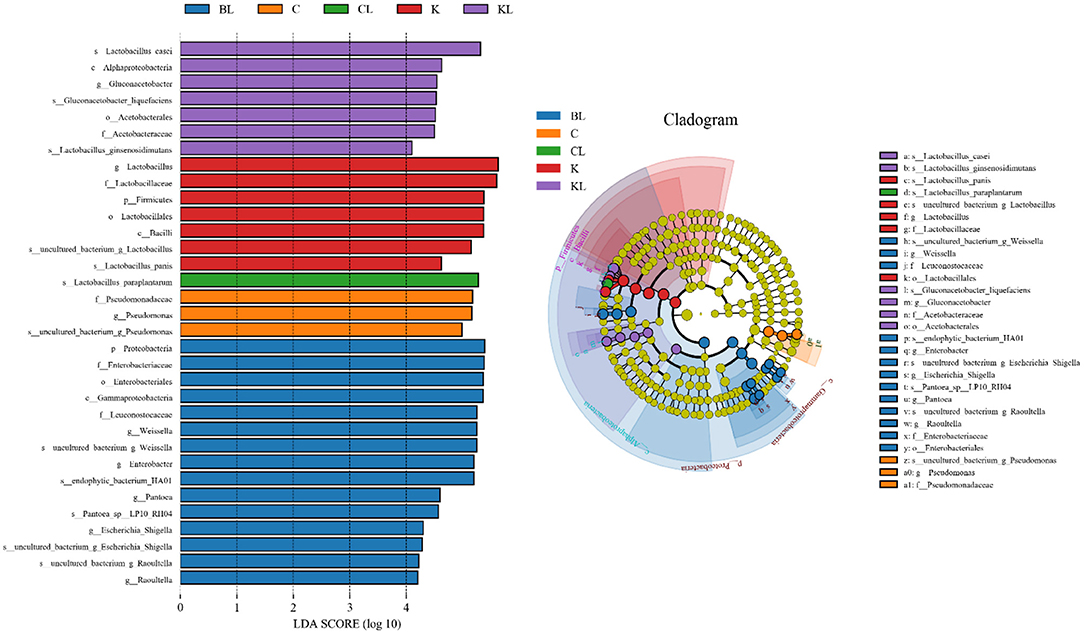
Figure 3. Comparison of microbial variations using the LEfSe online tool for C, K, and B (BL, Broussonetia papyrifera+ L. plantarum; C, cassava foliage; CL, cassava foliage+ L. plantarum; K, king grass; KL, king grass+ L. plantarum).
In BL, there was no significant change in diversity, while the richness was decreased compared with the control group. PCoA analysis showed the control group and silages treated with L. plantarum were not separated, indicating that the microbial community was not changed remarkably during the ensiling process. The combination of α-diversity and β-diversity showed that the effect of bacterial diversity and community structure of L. plantarum on B was limited. At the phylum level, the total contents of Firmicutes and Proteobacteria were more than 90% in both B and BL. Similar results have been found in Eucalyptus nutans of four different altitudes over the Tibetan Plateau (Ding et al., 2020). The same phenomenon has been observed in cassava foliage (Li et al., 2020). With inoculant L. plantarum, the content of Firmicutes was decreased, while the relative abundance of Proteobacteria was increased. At the genus level, the dominating bacteria in the B and BL groups were Weissella and Enterobacter. In addition, Lactobacillus, Pantoea, Escherichia-Shigella, and Raoultella were also included. Previous research has shown that Weissella initiates the silage fermentation, and then the predominant bacteria gradually turn into Lactobacilli, which are more tolerant to low pH (Cai et al., 1998). However, Lactobacillus was less (16.1–8.3%), and Enterobacter was more (28.4–31.4%) compared with the control group. As the core microorganism in the silage process, Lactobacillus, the beneficial bacteria, can produce lactic acid and determine the quality of silage (Cai et al., 1998). Enterobacteriaceae can grow under anaerobic conditions and trigger protective mechanisms under adverse conditions, including low pH. In this study, Enterobacter was the subdominant bacteria after ensiling, existing in silages that are non-pathogenic (Santos et al., 2016). However, their increase is undesirable because they compete with LAB for WSC at the initial stage of ensiling. Other studies also confirmed that Enterobacter is harmful to silage because it can compete with LAB for nutrients through fermenting lactic acid into acetic acid and producing ammonia, leading to nutrient loss (Silva et al., 2016). Our finding showed that L. plantarum had a weak competitive effect in this treatment, and the high content of oxygen or the high content of Escherichia coli attached to the raw materials might lead to the stable period dominated by Enterobacter (Chen et al., 2021), which might also explain why there was no significant change in bacterial community composition with or without inoculants. In our study, LAB was not the dominant bacteria in B. It is possible that the competitiveness of LAB already existing or the inoculant L. plantarum was weaker than that of the dominant bacteria but the reduction of relative abundance did not affect the fermentation quality of BL. It can be seen from the content of LA and AA. The LDA showed that Proteobacteria was the most abundant phylum in the BL group. Gammaproteobacteria was the most abundant class, Enterobacteriaceae and Leuconostocaceae were the most abundant family, Enterobacteriales was the most abundant order, and Weisella, Enterobacter, Pantoea, Escherichia_Shigella, and Raoultella were the most abundant genera. Moreover, uncultured_bacterium_g_Weisella,endophytic_bacterium_HA01, Pantoea_ sp_LI0_RH04, uncultured_ bacterium_ g_ Escherichia_ Shigella, and uncultured_ bacterium_ g_ Rautella were the most abundant species.
In CL, the diversity and richness were increased compared with the C group. For β-diversity, Figure 1 showed that C and CL were separated. The aforementioned diversity analysis showed that L could affect the bacterial diversity and community structure of C silage. At the phylum level, Firmicutes and Proteobacteria were the dominating bacteria. With inoculant L. plantarum, the content of Proteobacteria was decreased, while Firmicutes was increased. At the genus level, Lactobacillus accounts for a very high proportion in C and CL groups. In particular, C and CL groups contained Pseudomonas. When L. plantarum was added, its relative abundance became less, while the relative abundance of Lactobacillus and Enterobacter was increased, and Acinetobacter appeared. Pseudomonas is harmful to silage quality and nutrient preservation. In this group, the L. plantarum treatment notably reduced the relative abundance of Pseudomonas, and such a finding was similar to a previous study (Zi et al., 2021). The LDA showed that, in the C group, Pseudomonadaceae was the top abundant family, Pseudomonas was the most abundant genus, and uncultured _bacterium_ g_ Pseudomonas was the most abundant species. In the CL group, Lactobacillus_ paraplantarum was the most abundant species.
In KL, the diversity and the richness were decreased. For β-diversity, Figure 1 indicated that a clear separation of bacterial communities was discovered in K and KL. Therefore, L. plantarum could affect the bacterial diversity and community structure of K silage. It could be explained that L. plantarum decreased the pH, inhibited the growth of harmful microorganisms, and promoted the growth of LAB species. At the phylum level, Firmicutes and Proteobacteria had absolute advantages in relative abundance in K and KL, and compared with K, the content of Firmicutes was decreased while Proteobacteria was increased. Besides, Cyanobacteria appeared in the KL group. At the genus level, Lactobacillus accounts for a very high proportion in K and KL groups. There were Gluconacetobacter and Acinetobacter in the KL group, and Gluconacetobacter is widely used in wine and vinegar production. It is a bacterial strain that consumes ethanol and sugar to produce acetic acid (Raspor and Goranovic, 2008). Therefore, the higher the relative abundance of Gluconacetobacter, the higher the acetic acid content. Acinetobacter species are aerobic bacteria. However, previous studies have found that some Acinetobacter can survive in an anaerobic environment with acetic acid as substrate (Fuhs and Chen, 1975), which consumes energy from carbohydrates and results in DM loss (Pitt, 1986). Another research has validated this finding, evidenced by increased the content of AA, the abundance of Acinetobacter, and the loss of DM in silage treated with Lactobacillus brucelli (Oliveira et al., 2017). In this study, when L. plantarum was added to K, and the relative abundance of Acinetobacter or Lactobacillus was increased or decreased, respectively. This finding might be attributed to the high content of acetic acid in the KL group, which provided a suitable growth environment for Acinetobacter. The microbial genus level of K had been a relatively single community mainly composed of Lactobacillus. When L. plantarum was added as an inoculant, the amount of available matrix remains unchanged, which may aggravate intraspecific and interspecific competition so that bacteria with stronger acid production, growth ability, and stress tolerance became dominant bacteria. However, the relative abundance of Lactobacillus decreased as a whole. To explore this problem, it is better to identify the community composition to the species level. The LDA showed that, in the K group, Firmicutes was the most abundant phylum, Bacilli was the most abundant class, Lactobacillales was the most abundant order, Lactobaillaceae was the most abundant family, Lactobacillus was the most abundant genus, and uncultured_ bacterium_ g_ Lactobailus and Lactobacillus_ panis were the most abundant species. Alphaproteobacteria was the most abundant class in the KL group, Acetobacterales was the most abundant order, Acetobacteraceae was the most abundant family, Gluconacetobacter was the most abundant genus, and Lactobacillus_ Casei, Giluconacetobacter_ Liquefaciens, and Lactobacillus_ ginsenosidimutans were the most abundant species.
Conclusions
Lactobacillus plantarum played a positive role in fermentation quality. The specific performance decreased the pH value, and the NH3-N content markedly in KL, CL, and BL inhibited the production of BA and increased the content of LA in KL and BL. That shows that the inoculant plays a positive role. Besides, L. plantarum were useful to change the bacterial community of C, but it had little effect on K and B. Lactobacillus accounts for a very high proportion in K and KL. Weissella and Enterobacter were the dominant genera in B and BL. The relative abundance of Lactobacillus in group C was also very high. In addition, the inoculant L. plantarum enriched it in the CL group. As the second dominant genus of group C, the relative abundance of Pseudomonas decreased significantly in CL.
Data Availability Statement
The datasets presented in this study can be found in online repositories. The names of the repository/repositories and accession number(s) can be found in the article/supplementary material.
Author Contributions
ML and XZ did the experimental design work. YL, TC, RS, XZ, and ML conducted the experiments. YL, ML, and XZ analyzed the data and wrote and revised the manuscript. All the authors read and approved the article.
Funding
This study was funded by the Natural Science Foundation of Hainan Province (No. 2019RC145), the National Natural Science Foundation of China (No. 31860680), the Ministry of Agriculture and Rural Affairs of the People's Republic of China (No. 16200159), and Integrated Demonstration of Key Techniques for the Industrial Development of Featured Crops in Rocky Desertification Areas of Yunnan-Guangxi-Guizhou Provinces.
Conflict of Interest
The authors declare that the research was conducted in the absence of any commercial or financial relationships that could be construed as a potential conflict of interest.
Publisher's Note
All claims expressed in this article are solely those of the authors and do not necessarily represent those of their affiliated organizations, or those of the publisher, the editors and the reviewers. Any product that may be evaluated in this article, or claim that may be made by its manufacturer, is not guaranteed or endorsed by the publisher.
References
AOAC (2005). Official Methods of Analysis, 18th Edn. Arlington, VA: Association of Official Analytical Chemists.
Cai, Y., Benno, Y., Ogawa, M., Ohmomo, S., Kumai, S., and Nakase, T. (1998). Influence of Lactobacillus spp. from an inoculant and of Weissella and Leuconostoc spp. from forage crops on silage fermentation. Appl. Environ. Microbiol. 64, 2982–2987. doi: 10.1128/AEM.64.8.2982-2987.1998
Callahan, B. J., McMurdie, P. J., Rosen, M. J., Han, A. W., Johnson, A. J. A., and Holmes, S. P. (2016). DADA2: high-resolution sample inference from Illumina amplicon data. Nature methods: techniques for life scientists and chemists. Nat. Methods 13, 581–583. doi: 10.1038/nmeth.3869
Chen, D., Zheng, M., Guo, X., Chen, X., and Zhang, Q. (2021). Altering bacterial community: a possible way of lactic acid bacteria inoculants reducing CO2 production and nutrient loss during fermentation. Bioresour. Technol. 329, 124915. doi: 10.1016/j.biortech.2021.124915
Ding, Z., Bai, J., Xu, D., Li, F., Zhang, Y., and Guo, X. (2020). Microbial community dynamics and natural fermentation profiles of ensiled alpine grass Elymus nutans prepared from different regions of the Qinghai-Tibetan plateau. Front. Microbiol. 11, 855. doi: 10.3389/fmicb.2020.00855
Fuhs, G. W., and Chen, M. (1975). Microbiological basis of phosphate removal in the activated sludge process for the treatment of wastewater. Microb. Ecol. 2, 119–138. doi: 10.1007/BF02010434
He, L., Lv, H., Xing, Y., Wang, C., You, X., Chen, X., et al. (2020). The nutrients in Moringa oleifera leaf contribute to the improvement of stylo and alfalfa silage: fermentation, nutrition and bacterial community. Bioresour. Technol. 301, 122733. doi: 10.1016/j.biortech.2020.122733
Kung, L., Shaver, R. D., Grant, R. J., and Schmidt, R. J. (2018). Silage review: interpretation of chemical, microbial, and organoleptic components of silages. J. Dairy Sci. 101, 4020–4033. doi: 10.3168/jds.2017-13909
Li, M., Zhang, L., Zhang, Q., Zi, X., Lv, R., Tang, J., et al. (2020). Impacts of citric acid and malic acid on fermentation quality and bacterial community of cassava foliage silage. Front. Microbiol. 11, 595622. doi: 10.3389/fmicb.2020.595622
Li, M., Zi, X., Zhou, H., Hou, G., and Cai, Y. (2014). Effects of sucrose, glucose, molasses and cellulase on fermentation quality and in vitro gas production of king grass silage. Anim. Feed Sci. Technol. 197, 206–212. doi: 10.1016/j.anifeedsci.2014.06.016
Li, M., Zi, X., Zhou, H., Lv, R., Tang, J., and Cai, Y. (2019). Silage fermentation and ruminal degradation of cassava foliage prepared with microbial additive. AMB Express 9, 180. doi: 10.1186/s13568-019-0906-2
Li, P., Zhang, Y., Gou, W., Cheng, Q., Bai, S., and Cai, Y. (2018). Silage fermentation and bacterial community of bur clover, annual ryegrass and their mixtures prepared with microbial inoculant and chemical additive. Anim. Feed Sci. Technol.. 247, 285–293. doi: 10.1016/j.anifeedsci.2018.11.009
Lv, H., Pian, R., Xing, Y., Zhou, W., Yang, F., Chen, X., et al. (2020). Effects of citric acid on fermentation characteristics and bacterial diversity of Amomum villosum silage. Bioresour. Technol. 307, 123290. doi: 10.1016/j.biortech.2020.123290
Mao, L., Xuejuan, Z., Hanlin, Z., Guanyu, H., and Yimin, C. (2014). Effects of sucrose, glucose, molasses and cellulase on fermentation quality and in vitro gas production of king grass silage. Anim. Feed Sci. Technol. 197, 206–212.
Mu, L., Xie, Z., Hu, L., Chen, G., and Zhang, Z. (2020). Cellulase interacts with Lactobacillus plantarum to affect chemical composition, bacterial communities, and aerobic stability in mixed silage of high-moisture amaranth and rice straw. Bioresour. Technol. 315, 123772. doi: 10.1016/j.biortech.2020.123772
Muck, R. E., Nadeau, E. M G., Mcallister, T. A., Contreras-Govea, F. E., Santos, M. C., King Jr., L., et al. (2018). Silage review: Recent advances and future uses of silage additives. J. Dairy Sci. 101, 3980–4000. doi: 10.3168/jds.2017-13839
Napasirth, V., Napasirth, P., Sulinthone, T., Phommachanh, K., and Cai, Y. (2015). Microbial population, chemical composition and silage fermentation of cassava residues. Anim. Sci. J. 86, 842–848. doi: 10.1111/asj.12362
Ni, K., Zhao, J., Zhu, B., Su, R., Pan, Y., Ma, J., et al. (2018). Assessing the fermentation quality and microbial community of the mixed silage of forage soybean with crop corn or sorghum. Bioresour. Technol. 265, 563–567. doi: 10.1016/j.biortech.2018.05.097
Oliveira, A. S., Weinberg, Z. G., Ogunade, I. M., Cervantes, A. A. P., Arriola, K. G., Jiang, Y., et al. (2017). Meta-analysis of effects of inoculation with homofermentative and facultative heterofermentative lactic acid bacteria on silage fermentation, aerobic stability, and the performance of dairy cows. J. Dairy Sci. 100, 4587–4603. doi: 10.3168/jds.2016-11815
Pitt, R. E. (1986). Dry matter losses due to oxygen infiltration in silos. J. Agric. Eng. Res. 35, 193–205. doi: 10.1016/S0021-8634(86)80056-7
Rabelo, C. H. S., Härter, C. J., Ávila CLd, S., and Reis, R. A. (2019). Meta-analysis of the effects of Lactobacillus plantarum and Lactobacillus buchneri on fermentation, chemical composition and aerobic stability of sugarcane silage. Grassland Sci. 65, 3–12.doi: 10.1111/grs.12215
Raspor, P., and Goranovic, D. (2008). Biotechnological applications of acetic acid bacteria. Crit. Rev. Biotechnol. 28, 101–124. doi: 10.1080/07388550802046749
Ren, H., Feng, Y., Pei, J., Li, J., Wang, Z., Fu, S., et al. (2020). Effects of Lactobacillus plantarum additive and temperature on the ensiling quality and microbial community dynamics of cauliflower leaf silages. Bioresour. Technol. 307, 123238. doi: 10.1016/j.biortech.2020.123238
Santos, A. O., Ávila, C. L. S., Pinto, JC, Carvalho, B. F., Dias, D. R., and Schwan, R. F. (2016). Fermentative profile and bacterial diversity of corn silages inoculated with new tropical lactic acid bacteria. J. Appl. Microbiol. 120, 266–279. doi: 10.1111/jam.12980
Schloss, P. D., Westcott, S. L., Ryabin, T., Hall, J. R., Hartmann, M., Hollister, E. B., et al. (2009). Introducing mothur: open-source, platform-independent, community-supported software for describing and comparing microbial communities. Appl. Environ. Microbiol. 75, 7537–7541. doi: 10.1128/AEM.01541-09
Segata, N., Izard, J., Waldron, L., Gevers, D., Miropolsky, L., Garrett, W. S., et al. (2011). Metagenomic biomarker discovery and explanation. Front. Microbiol. 12, R60. doi: 10.1186/gb-2011-12-6-r60
Si, B., Tao, H., Zhang, X., Guo, J., Cui, K., Tu, Y., et al. (2018). Effect of Broussonetia papyrifera L. (paper mulberry). silage on dry matter intake, milk composition, antioxidant capacity and milk fatty acid profile in dairy cows. Asian Aust. J. Anim. Sci. 31, 1259–1266. doi: 10.5713/ajas.17.0847
Silva, V. P., Pereira, O. G., Leandro, E. S., Silva, T. C. D., Ribeiro, K. G., Mantovani, H. C., et al. (2016). Effects of lactic acid bacteria with bacteriocinogenic potential on the fermentation profile and chemical composition of alfalfa silage in tropical conditions. J. Dairy Sci. 99, 1895–1902. doi: 10.3168/jds.2015-9792
Tang, T., Tong, F., Zhao, S., Bai, J., Wei, Z., Hu, Y., et al. (2021). Effects of fermented Broussonetia papyrifera on growth, intestinal antioxidant, inflammation and microbiota of grass carp. (Ctenopharyngodon idella). Aquacult. Rep. 20, 100673. doi: 10.1016/j.aqrep.2021.100673
Vázquez-Baeza, Y., Pirrung, M., Gonzalez, A., and Knight, R. J. G. (2013). EMPeror: a tool for visualizing high-throughput microbial community data. GigaScience 2, 16. doi: 10.1186/2047-217X-2-16
Wang, Y., He, L., Xing, Y., Zhou, W., Pian, R., Yang, F., et al. (2019). Bacterial diversity and fermentation quality of Moringa oleifera leaves silage prepared with lactic acid bacteria inoculants and stored at different temperatures. Bioresource Technology 284, 349–358. doi: 10.1016/j.biortech.2019.03.139
Wang, Y., Wang, C., Zhou, W., Yang, F. Y, Chen, X. Y, and Zhang, Q. (2018). Effects of Wilting and Lactobacillus plantarum addition on the fermentation quality and microbial community of Moringa oleifera leaf silage. Front. Microbiol. 9, 1817. doi: 10.3389/fmicb.2018.01817
Wüstholz, J., Carrasco, S., Berger, U., Sundrum, A., and Bellof, G. (2017). Fattening and slaughtering performance of growing pigs consuming high levels of alfalfa silage (Medicago sativa) in organic pig production. Livestock Sci. 200, 46–52. doi: 10.1016/j.livsci.2017.04.004
Yan, Y., Li, X., Guan, H., Huang, L., Ma, X., Peng, Y., et al. (2019). Microbial community and fermentation characteristic of Italian ryegrass silage prepared with corn stover and lactic acid bacteria. Bioresour. Technol. 279:166–173. doi: 10.1016/j.biortech.2019.01.107
Yang, L., Yuan, X., Li, J., Dong, Z., and Shao, T. (2019). Dynamics of microbial community and fermentation quality during ensiling of sterile and nonsterile alfalfa with or without Lactobacillus plantarum inoculant. Bioresour. Technol. 275:280–287. doi: 10.1016/j.biortech.2018.12.067
Zhao, J., Dong, Z., Li, J., Chen, L., Bai, Y., Jia, Y., et al. (2019). Effects of lactic acid bacteria and molasses on fermentation dynamics, structural and nonstructural carbohydrate composition and ruminal fermentation of rice straw silage. Asian-Australas J. Anim. Sci. 32, 783–791. doi: 10.5713/ajas.18.0543
Zheng, M., Mao, P., Tian, X., Guo, Q., and Meng, L. (2019). Effects of dietary supplementation of alfalfa meal on growth performance, carcass characteristics, meat and egg quality, and intestinal microbiota in Beijing-you chicken. Poult. Sci. 98, 2250–2259. doi: 10.3382/ps/pey550
Keywords: Lactobacillus plantarum, king grass, cassava foliage, Broussonetia papyrifera, silage fermentation, bacterial community
Citation: Liu Y, Chen T, Sun R, Zi X and Li M (2022) Effects of Lactobacillus plantarum on Silage Fermentation and Bacterial Community of Three Tropical Forages. Front. Anim. Sci. 3:878909. doi: 10.3389/fanim.2022.878909
Received: 18 February 2022; Accepted: 05 April 2022;
Published: 16 May 2022.
Edited by:
Qing Zhang, South China Agricultural University, ChinaReviewed by:
Yanhong Yan, Sichuan Agricultural University, ChinaHao Guan, Southwest Minzu University, China
Siran Wang, Nanjing Agricultural University, China
Copyright © 2022 Liu, Chen, Sun, Zi and Li. This is an open-access article distributed under the terms of the Creative Commons Attribution License (CC BY). The use, distribution or reproduction in other forums is permitted, provided the original author(s) and the copyright owner(s) are credited and that the original publication in this journal is cited, in accordance with accepted academic practice. No use, distribution or reproduction is permitted which does not comply with these terms.
*Correspondence: Xuejuan Zi, eml4dWVqdWFuJiN4MDAwNDA7MTYzLmNvbQ==; Mao Li, bGltYW9obiYjeDAwMDQwOzE2My5jb20=