- Department of Animal Biosciences, University of Guelph, Guelph, ON, Canada
Poultry egg and meat production continue to be optimized for productivity and efficiency. However, genetic selection focusing on production efficiency has overlooked other aspects critical to bird wellbeing, such as skeletal development. As a result, modern birds are more prone to leg weakness, osteoporosis, and, subsequently, fractures. Dietary omega-3 polyunsaturated fatty acid (n-3 PUFA) enrichment has been proposed to benefit bone development, quality, and strength. However, there is a lack of conclusive and quantitative results across studies. Therefore, a meta-analysis approach was used to evaluate published studies to determine the effects of dietary n-3 PUFA enrichment on bone quality in laying- and meat-type birds. Publications were retrieved from multiple sources (databases and hand searching), and ten studies were selected for inclusion in the final dataset. A model to predict tibial bone ash content (BAC) was developed in Proc MIXED of SAS, treating the study as a random effect. The dietary concentration of n-3 PUFA, n-3 PUFA:n-6 PUFA ratio, calcium (Ca), phosphorus and feeding duration (days) were used as independent variables to predict BAC. The final model included the dietary n-6:n-3 FA ratio and the calcium concentration in the diet. The final model was selected based on the corrected Akaike Information Criteria, the root mean square prediction error (0.999) and its components, and the concordance correlation coefficient (CCC) (0.99). In laying-type birds, BAC was reduced by n-3 PUFA (p = 0.001) but was increased by Ca (p = 0.014). In contrast, in broiler chickens, BAC was increased by n-3 PUFA (p = 0.001) and decreased by Ca (p = 0.014). The influence of n-3 PUFA:n-6 PUFA ratio on tibia BAC in laying-type birds was not statistically significant (p = 0.505), whereas in meat-type birds, the influence of PUFA ratio was significant (p < 0.05). These results may indicate a low biological significance in laying-type birds but not in meat-type birds.
Introduction
Due to intensive genetic selection for production parameters, both laying- and meat-type birds experience skeletal metabolic disorders throughout their lifetime. In laying hens, breeding companies have continued to pursue longer production cycles with persistent rates of lay, resulting in osteoporosis and an increased risk of fractures (Bain et al., 2016). Meat-type birds have been continually selected for fast growth rates, better feed efficiencies, and low-cost production cycles, resulting in skeletal frames that are incapable of supporting their heavy bodyweights (Siegel, 2014). Subsequently, nutrition and production strategies for modulating skeletal health have gained interest as a way of attenuating skeletal disorders (Venäläinen and Valaja, 2006; Campbell et al., 2019; Khanal et al., 2021). Based on mammalian research (Watkins et al., 2000), the inclusion of omega-3 polyunsaturated fatty acids (n-3 PUFA) in poultry diets is one route of interest due to its potential to enhance bone quality. Boeyens et al. (2014) reported a strong inhibition of osteoclastogenesis in murine cell lines treated with n-3 PUFA, resulting in increased bone mass retention. However, the effect of dietary n-3 PUFA on bone attributes varies in poultry studies. An increase in the bone ash content of the tibia and the femur was reported in laying hens fed a n-3 PUFA diet when compared with laying hens fed a short-chain fatty acid diet for 58 weeks (Josling et al., 2019). In broiler chickens, the inclusion of docosahexaenoic acid (DHA) at 1 or 2% significantly improved the breaking strength of the humerus, another indicator of bone quality (Ao et al., 2015). Liu et al. (2003) reported similar findings in growing quail fed a n-3 PUFA diet—the tibial bone mineral content was highest in n-3 PUFA supplemented birds. However, Baird et al. (2008) reported no significant effects on the tibial bone mineral content in laying hens fed a high n-3 PUFA diet.
The primary objective of this meta-analysis was to quantify and examine variables that influence bone quality in poultry fed n-3 PUFA enriched diets. For this study, bone quality will be defined by bone ash content (BAC), an indicator of bone mineralization and bone strength.
Materials and Methods
Literature Search and Dataset Development
The literature was systematically searched for relevant articles using the Web of Science, Omni, and Google Scholar in March 2021. For the dataset, the following combination of search terms was used: (omega 3 fatty acids OR n-3 OR PUFA OR polyunsaturated fatty acids OR long-chain polyunsaturated fatty acids) AND (skeletal OR bone OR tibia) AND (poultry OR avian OR layer OR broiler) for all fields. No limitations were set on language. However, studies involving broiler chickens were limited to the last 20 years to account for rapid genetic selection and evolution in the chicken industry (Tavárez and Santos, 2016). Highly cited articles and reviews were searched manually for relevant publications, and the reference lists were examined for additional sources.
Study designs with an intervention of n-3 fatty acid deficient diets were excluded in both datasets. Studies that did not report an adequate definition of the fatty acid profile of the diets were also excluded. Furthermore, the pieces of literature were screened for quantifying BAC in the tibia or the femur specifically, such that only studies following one of the two methods described by Hall et al. (2003) for quantifying bone ash were included. Lastly, studies examining keel bone quality in laying hens were excluded from the dataset to create consistency between the types of birds. The literature funnels the dataset is presented in Figure 1 and adapted from Moher et al. (2009). The final dataset is presented in Table 1.
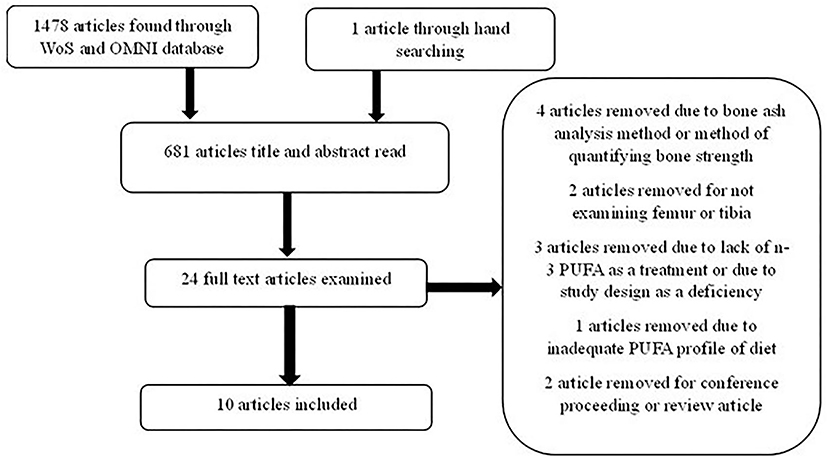
Figure 1. Literature funnel for dataset development examining direct omega-3 polyunsaturated fatty acid (n-3 PUFA) feeding effects on bone ash content (BAC).
Data Extraction and Model Development
Total dietary n-3 PUFA (g/kg diet), total dietary n-6 PUFA (g/kg diet), dietary Ca concentration (%), available dietary phosphorus concentration (%), treatment duration (days), number of birds, and tibia BAC (%) were extracted into Excel from the included studies (Figure 1). Where possible, standard error (SE) or standard deviation (SD) was also extracted and recorded as a metric of mean precision. In studies where data were presented in graphs, means and SE or SD were digitized and extracted using WebPlotDigitizer (Rohatgi, 2021). When dietary Ca and available phosphorus concentrations were not provided, the Poultry NRC (1994) values were used to estimate concentrations based on the ingredient lists provided by the authors (n = 2). To ensure consistency, the ratio of n-6 PUFA to n-3 PUFA was calculated manually within each study using the provided fatty acid composition by dividing the total n-6 PUFA by the total n-3 PUFA as a means of standardizing varying enrichment concentrations. In addition, data were identified by study type of the bird (laying type or meat birds) to account for genetic differences. For model development, a total of 40 treatment means from 10 studies were used.
All statistical analyses were performed using SAS Studio (SAS Inst. Inc., Cary, NC). Continuous independent X variables included in this meta-analysis were the ratio of dietary n-6 PUFA to n-3 PUFA and dietary Ca concentration, while the type of bird (laying or meat type) was a categorical independent X variable. Initial data assessment included examination of the means and SE (PROC MEANS) for continuous variables by the categorical variables (Table 2). The normality of data was also visually examined (PROC UNIVARIATE). PROC CORR was used to identify potential independent variables highly correlated according to Spearman's rank correlation (data not shown). After identifying variables that could be used in the model together (low collinearity), the PROC REG backward selection procedure was used for an initial assessment of potential continuous variable models. Based on these preliminary explorations of the data, potential continuous variables of interest (p < 0.05) included duration of treatment, dietary available phosphorus concentration, the ratio of n-6 PUFA to n-3 PUFA, and dietary Ca concentration.
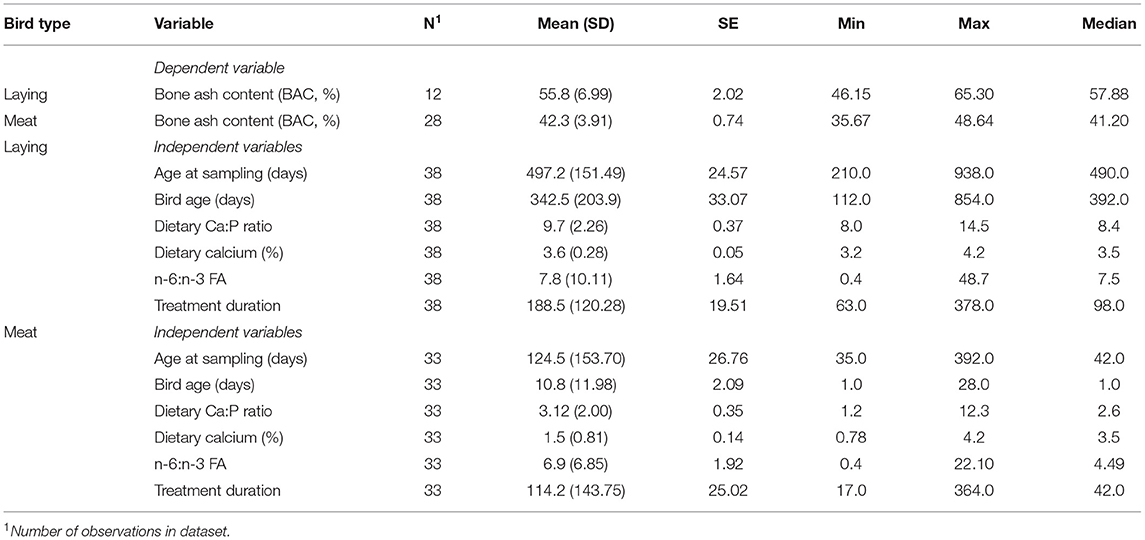
Table 2. Descriptive statistics of bone ash content (BAC, %) and independent variables (treatment duration (days), bird age (days), age at sampling (days), dietary calcium to phosphorus ratio, dietary n-6:n-3 ratio, and dietary calcium concentration (%) in the dataset.
In this meta-analysis, the study was treated as a random effect, accounting for sources of between-study variance (St-Pierre, 2001; Sauvant et al., 2008). The PROC MIXED procedure in SAS was used for model development while considering the random study effect, such that:
where i is the number of studies, j is the number of observations, Yij is the value of the response variable (BAC, %), b0 is the overall intercept, b1 are the coefficients of independent predictive variable 1 (X1ij, N6:N3 ratio), b3 is the species level (meat-type or layer-type), b2 are the coefficients of independent predictive variable 2 (X2ij, Dietary Ca, %), si is the intercept of the random effect portion of the model, and eij is the unexplained residual error (St-Pierre, 2001). Variables in the model were considered statistically significant at p < 0.05 to be included in the model.
The Cook's Distance test was used in PROC MIXED to identify influential outliers, which were subsequently examined and considered for exclusion. Conditional studentized residual plots were visually assessed to ensure homogeneous and normally distributed residual errors. The homogeneity and normality of the random effect of the study (on model intercept) were also visually assessed, using a Q–Q plot (PROC SGPLOT). The optimal variance-covariance structure was determined by comparing the corrected Akaike's Information Criterion (AICc) of various random-effects statement structures.
Model Evaluation
The developed model was assessed for accuracy and precision of predictions using the original dataset, using methods described by Tedeschi (2006). The mean square prediction error (MSPE) assesses the mean bias, the error due to regression slope deviation from unity and random errors, and can be calculated as:
where is the mean of the model predicted values, Y is the mean of the observed values, sf(X1, …Xp) is the SD of the model predicted values, r is the Pearson correlation coefficient, sy is the SD of the observed values, and r2 is the coefficient of determination. The root MSPE (RMSPE) was also calculated, expressed as a percentage of the observed mean, estimating the overall prediction error relative to the data scale.
The model was also evaluated via the concordance correlation coefficient (CCC) to account for both accuracy and precision simultaneously (Lin, 1989) and calculated as:
where r is the Pearson correlation coefficient, a measure of precision and Cb is a measure of accuracy. The Cb formula contains multiple useful metrics, such as measures of scale shift (V) and location shift (μ) about the data scale. The scale shift represents the change in SD between predicted and observed data; therefore, a large shift indicates a large difference in variance captured in predictions compared with the observations. Location shift indicates underprediction or overprediction by the model as indicated by a negative or a positive value, respectively.
Finally, the models developed were visually assessed using (conditional) predicted vs. observed plots and (conditional) residuals vs. predicted plots.
Results and Discussion
Influence of Dietary n-3 PUFA on Bone Development in Laying- and Meat-Type Birds
To the best of our knowledge, this is the first meta-analysis conducted regarding the dietary enrichment of n-3 PUFA and its effects on bone strength in poultry. Bone strength is a concern in poultry production as genetic selection for production goals, such as high rates of lay in hens and fast growth in broilers, has neglected bone quality and can lead to skeletal disorders (Julian, 2005). In broiler- or meat-type birds, rapid muscle tissue accretion supersedes the rate of bone development, resulting in a wide and thick tibial growth plate unable to support the bird's heavy body weight (Butterworth, 1999; Julian, 2005). In laying hens, high egg production rates and lay persistency in conjunction with inadequate dietary Ca supply can lead to osteoporosis (Bain et al., 2016; Rufener et al., 2019). Bone strength is affected by bone mineralization, where poor mineralization has been associated with increased fracture risks (Onyango et al., 2003). Bone mineralization can be evaluated by bone ash, the mineral portion of the bone remaining after incineration, and is the dependent variable in the models developed here (Rao et al., 1993).
Models Predicting the Influence of Dietary n-3 PUFA on Bone Development in Laying- and Meat-Type Birds
The final model for predicting bone strength, as represented by BAC in avian species fed diets enriched with n-3 PUFA, is as follows:
Laying-Type Birds
Meat-Type Birds
While the n-6:n-3 × bird type interaction was significant, the coefficient for the n-6:n-3 ratio in laying-type birds (0.03 ± 0.049, parameter estimate) was not significantly different from zero (p = 0.505), indicating a low biological significance. Similarly, the coefficient for dietary Ca in meat-type birds (−0.07 ± 2.781, parameter estimate) was not significantly different from zero (p = 0.979), indicating a small influence of Ca on BAC in these birds. Interestingly, the model did not consider phosphorus or the ratio of calcium to phosphorus to be significant.
Model evaluation was conducted using conditional predictions (such as, the fixed and random components of the model developed) and is presented in Table 3. The majority of errors (MSPE) were random errors, indicating a high precision and accuracy of the model (Table 3). Furthermore, the CCC of the model was 0.99, indicating a high agreement between predictions and observations. Predicted vs. observed and residual vs. predicted plots are also presented in Figure 2, with both “raw” (considering just the fixed effect portion of the model) and conditional adjusted predictions. The difference between the two sets of plots highlights the variation accounted for in the “study effect.”
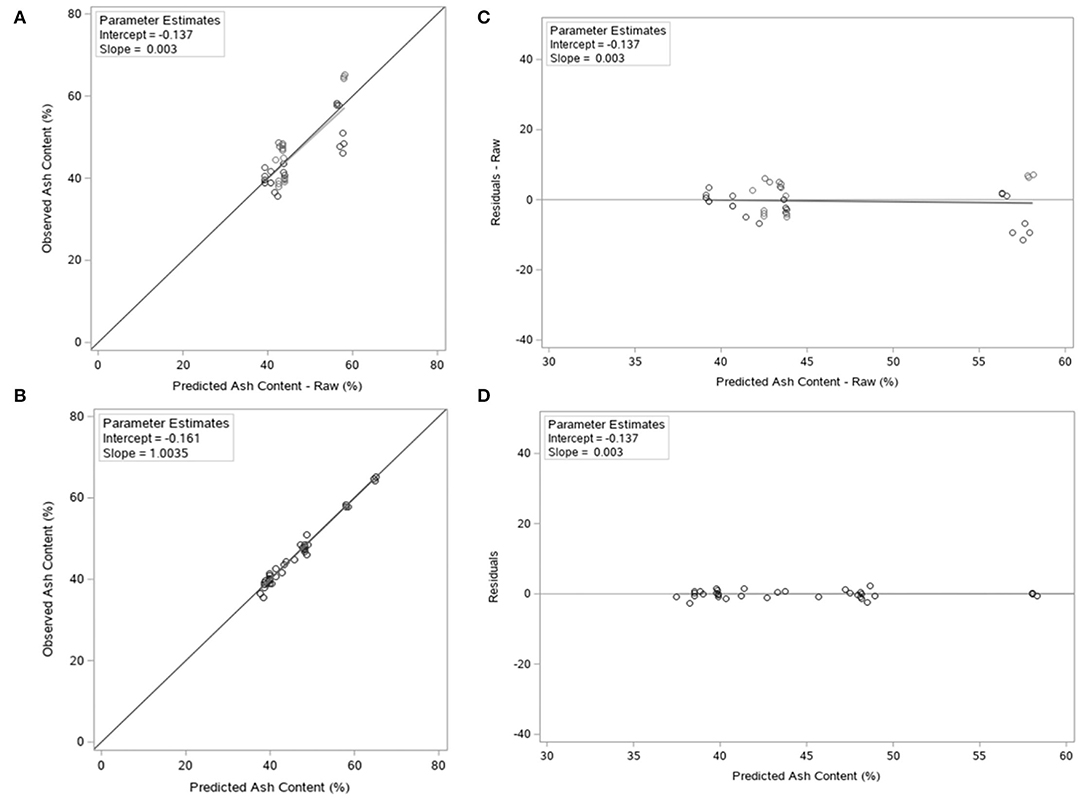
Figure 2. (A) Observed vs. raw predicted values plot for the model equation. (B) Observed vs. conditional predicted values plot for the model equation. (C) Raw residuals vs. predicted plot for the model equation. (D) Conditional residuals (predicted – observed) vs. predicted plot for the model equation.
Influence of Dietary n-3 PUFA on Bone Development in Laying Birds
In the database used for model development, the reduction in the n-6:n-3 ratio is primarily a result of increased n-3 content in the diet. Therefore, the coefficient estimate in the model (b1 = 0.03) for laying hens indicates that increasing the inclusion of dietary n-3 reduces BAC. While the size of the database is limited (n = 12 treatment means for layers and n = 25 for meat birds), within each study, BAC did decrease numerically with n-3 PUFA enrichment. The meta-analysis approach has been shown to increase statistical power (Cohn, 2003), and thus a (perhaps) smaller, previously non-significant effect was picked up as significant herein.
Toscano et al. (2015) previously reported that changes in bone properties due to n-3 PUFA are dependent on the type of n-3 PUFA provided. Furthermore, while only studied in mammals, dietary n-3 PUFA enrichment is thought to attenuate osteoporosis through the receptor activator of the nuclear factor-kappa-B ligand (RANKL)/receptor activator of nuclear factor-kappa-B (RANK)/Osteoprotegerin (OPG) pathway, which is responsible for osteoclastogenesis, the process wherein bone-resorbing cells known as osteoclasts are formed (Kajarabille et al., 2013). The main regulatory cytokine involved in this process is arachidonic acid (AA) (which is derived from n-6 PUFA, LA—it is present in quantities in soy oil) derived lipid mediator prostaglandin E2 (PGE2) and acts to stimulate RANKL and RANK, leading to maturation of osteoclast precursors into activated osteoclasts, causing bones to enter a resorptive state (Boyce and Xing, 2008; Shen et al., 2008). Manipulation of dietary fatty acids results in higher levels of n-3 PUFA in cellular membranes, increasing production of EPA and DHA-derived eicosanoids and decreasing AA-derived eicosanoid production—suppressing osteoclastogenesis (Kajarabille et al., 2013). The conversion of alpha-linolenic acid (ALA) to eicosapentaenoic acid (EPA) and DHA, the active metabolites, is relatively poor, and therefore, the authors hypothesize that the source of dietary n-3 PUFA in the studies analyzed may have influenced the results. However, due to little variance in the source of PUFA (studies provided flaxseed oil, a source of ALA or soybean oil, a source of LA), the source or type (e.g., ALA, e.g., ALA vs. DHA) of PUFA could not be considered in the model(s) developed.
Unfortunately, this database (and thus the model) could not consider the time of intervention (growing, pre-lay, or laying phase) or the treatment duration as most studies in the dataset were considered long-term feeding and after the onset of production. At the onset of lay, there is a shift from structural cortical bone formation to the medullary bone, a reservoir of Ca for eggshell production (Whitehead, 2004). In cases where dietary Ca is insufficient, the medullary bone is replenished by resorption of structural bones, which may lead to osteoporosis (Khanal et al., 2021). Radiographic density reports indicated a higher bone mineral density and a higher amount of medullary bone in the keel bone of egg-laying birds (Eusemann et al., 2020). While the medullary bone has a high level of mineralization, it does not contribute to bone strength due to its structure (Whitehead, 2004). The keel bone, a structural bone, is widely studied in laying-type birds as a measure of skeletal health. Ultimately, studies examining keel bone quality in laying hens were excluded from the dataset to create consistency between the types of birds in this meta-analysis. However, it would be beneficial to examine the effects of feeding omega-3 FA perhaps separately on keel bone BAC in laying hens, specifically due to the availability of literature (Tarlton et al., 2011; Toscano et al., 2012).
To put the effect of n-3 on egg-laying birds into perspective, compared with the relatively larger impact of dietary Ca concentration, the influence of n-3 PUFA enrichment is minimal and may not cause detrimental effects on bone ash (Table 4). Table 4 demonstrates the model predictions using the egg-type model—the change in dietary Ca concentration influenced the BAC much greater than the dietary n-6 PUFA:n-3 PUFA within each Ca concentration.
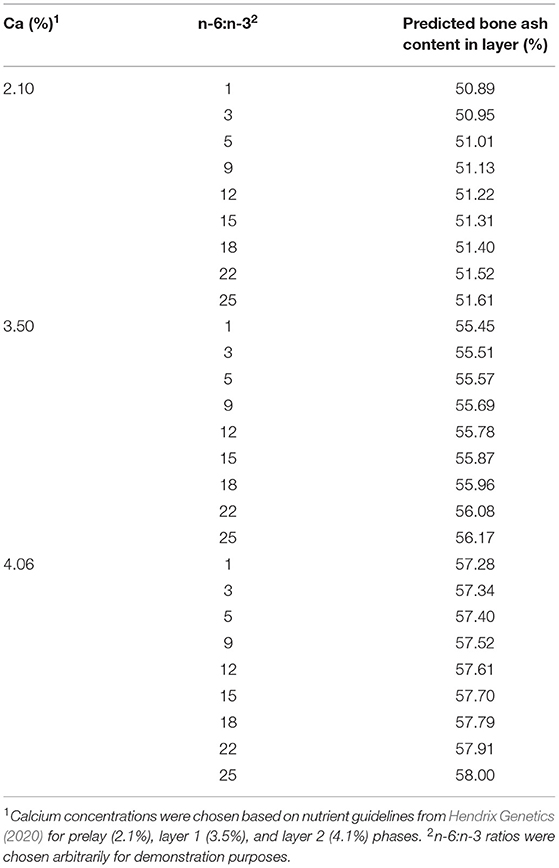
Table 4. Predicted BAC (%) for layers using the developed model, where BAC (%) = 44.01 + 0.03 (dietary n-6:n-3) + 3.26 (Ca concentration diet, %).
Influence of Dietary n-3 PUFA on Bone Development in Meat-Type Birds
In contrast, the meat bird model indicated increased BAC as diets were increasingly enriched with n-3 PUFA despite the negative Ca coefficient (Table 5). The negative calcium coefficient may be because of a decreased concentration of calcium in the diet as meat birds age, indicating decreasing importance metabolically. In the meat-type bird studies, the broilers were fed starting from their hatching, and therefore dietary influence on developing birds could be more significant. For example, growing rats fed a n-3 PUFA diet had an increased Ca balance and small intestine absorption, translating to increased intestinal availability of Ca, potentially increasing incorporation into the bone matrix (Lau et al., 2013). Another proposed mechanism of action involving n-3 PUFA and bone development involves bone marrow containing mesenchymal stem cells that can differentiate into osteoblasts (bone-forming cells) and adipocytes, suggesting that the higher the number of bone marrow cells available, the higher the potential for osteoblastogenesis (Kruger et al., 2010). However, as previously mentioned, the effect of treatment duration was not significant (perhaps due to limited data representation) and therefore not included in the model.
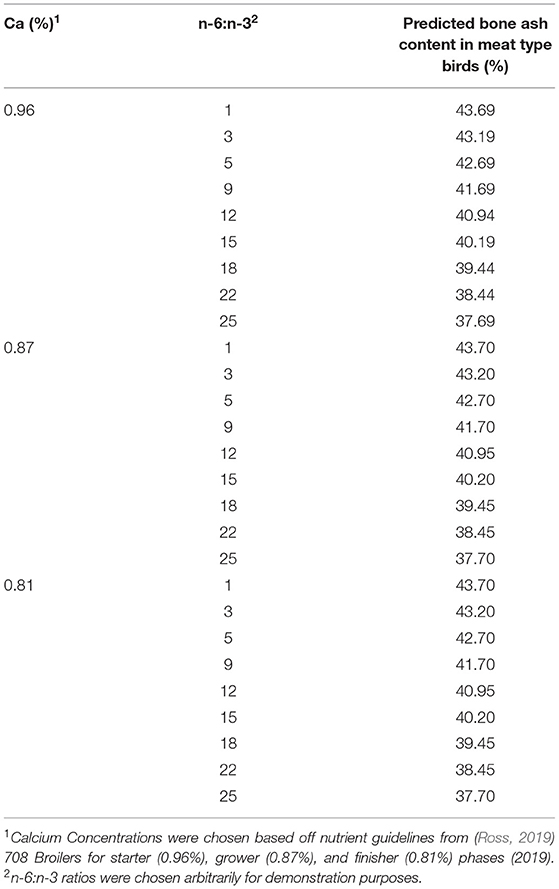
Table 5. Predicted BAC (%) for meat birds using the developed model, where BAC (%) = 44.01 – 0.25 (dietary n-6:n-3) – 0.07 (Ca concentration diet, %).
Furthermore, in a typical broiler ration, LA constitutes over 50% of total PUFA compared with the ~3–3.5% ALA, due to the predominance of corn, soybean, and other sources of dietary fat high in n-6 PUFA, generally leading to a pro-inflammatory state in broilers (Cherian, 2011). Enrichment with n-3 PUFA while decreasing the n-6 PUFA to n−3 PUFA ratio results in the decrease of proinflammatory n-6 PUFA-derived cytokines, such as IL-6 (Tarlton et al., 2013). Cytokines, such as interleukin-6 (IL-6), and PGE2 induce each other's production and prompt osteoclastogenesis by inhibiting OPG production and upregulating RANK production, indicating that an inflamed state could perpetuate bone loss and weakening (Liu et al., 2003). The relationship between immune response, dietary n-3 PUFA, and bone quality is another mechanism that could explain our model. Ultimately, this model suggests that enriching meat-type birds' diets with n-3 PUFA could attenuate skeletal disorders.
Conclusion and Future Directions
The foregoing meta-analysis developed models to predict the bone quality of both egg- and meat-type birds when fed a n-3 PUFA diet with consideration of dietary Ca concentration as an important covariate. In laying-type birds, the enrichment of dietary n-3 PUFA resulted in decreased BAC whereas, in meat-type birds, the enrichment increased BAC. It is important to note that this exercise was not able to account for treatment duration, source of FA, or genetic breed differences (which may all influence BAC) due to a lack of available data. Future studies should consider examining and reporting the age of dietary intervention. Furthermore, BAC incorporates both Ca and P; however, our models did not have dietary phosphorus as a significant fixed effect. The developed models suggest the relationship between n-3 PUFA inclusion and BAC in broiler and layer diets; however, more data is needed to account for all possible influencing variables.
Data Availability Statement
The original contributions presented in the study are included in the article/supplementary material, further inquiries can be directed to the corresponding author/s.
Author Contributions
AT is a graduate student of EK and conducted the meta-analysis under the supervision of JE. All authors reviewed and edited the manuscript. All authors contributed to the article and approved the submitted version.
Conflict of Interest
The authors declare that the research was conducted in the absence of any commercial or financial relationships that could be construed as a potential conflict of interest.
Publisher's Note
All claims expressed in this article are solely those of the authors and do not necessarily represent those of their affiliated organizations, or those of the publisher, the editors and the reviewers. Any product that may be evaluated in this article, or claim that may be made by its manufacturer, is not guaranteed or endorsed by the publisher.
Acknowledgments
We would like to thank the contributions of current and former graduate students and research associates of EK Monogastric Nutrition Research Laboratory at the University of Guelph.
References
Abdulla, N. R., Loh, T. C., Akit, H., Sazili, A. Q., Foo, H. L., Kareem, K. Y., et al. (2016). Effects of dietary oil sources, calcium and phosphorus levels on growth performance, carcass characteristics and bone quality of broiler chickens. J. Appl. Animal Res. 45, 423–429. doi: 10.1080/09712119.2016.1206903
Ao, T., Macalintal, L. M., Paul, M. A., Pescatore, A. J., Cantor, A. A., and Glenney, P. (2015). Poultry Science Association Annual Meeting, Poultry Science Association. Kentucky, US: Microalgae supplementation in broiler diets enriches docosahexaenoic acid content of meat and improves bone strength.
Bain, M. M., Nys, Y., and Dunn, I. C. (2016). Increasing persistency in lay and stabilising egg quality in longer laying cycles. What are the challenges? Br. Poultry Sci. 57, 330–338. doi: 10.1080/00071668.2016.1161727
Baird, H. T., Eggett, D. L., and Fullmer, S. (2008). Varying ratios of omega-6:omega-3 fatty acids on the pre-and postmortem bone mineral density, bone ash, and bone breaking strength of laying chickens. Poultry Sci. 87, 323–328. doi: 10.3382/ps.2007-00186
Boeyens, J. A. C., Deepak, V., Chua, W., Kruger, M. C., Joubert, A. M., and Coetzee, M. (2014). Effects of ω3- and ω6-polyunsaturated fatty acids on RANKL-induced osteoclast differentiation of RAW264, 7. cells: a comparative in vitro study. Nutrients. 6, 2584–2601. doi: 10.3390/nu6072584
Boyce, B. F., and Xing, L. (2008). Functions of RANKL/RANK/OPG in bone modeling and remodeling. Arch. Biochem Biophys. 473, 139–146. doi: 10.1016/j.abb.2008.03.018
Butterworth, A. (1999). Infectious components of broiler lameness: a review. World's Poultry Sci. J. 55, 327–352. doi: 10.1079/WPS19990024
Campbell, D. L. M., Haas, E. N., and Lee, C. (2019). A review of environmental enrichment for laying hens during rearing in relation to their behavioural and physiological development. Poult. Sci. 98, 9–28. doi: 10.3382/ps/pey319
Cherian, G. (2011). Essential fatty acids and early life programming in meat-type birds. World's Poult. Sci. J. 67, 599–614. doi: 10.1017/S0043933911000705
Cohn, L. D. (2003). How meta-analysis increases statistical power. Psychol. Method. 8, 243–253. doi: 10.1037/1082-989X.8.3.243
Ebeid, T., Fayoud, A., Abou El-Soud, S., Eid, Y., and El-Habbak, M. (2011). The effect of omega-3 enriched meat production on lipid peroxidation, antioxidative status, immune response and tibia bone characteristics in Japanese quail. Czech J. Animal Sci. 56, 314–324. doi: 10.17221/1293-CJAS
Ebeid, T. A. (2011). The impact of incorporation of n-3 fatty acids into eggs on ovarian follicular development, immune response, antioxidative status and tibial bone characteristics in aged laying hens. Animal 5, 1554–1562. doi: 10.1017/S1751731111000619
Ehr, I. J. (2017). Production and health responses of laying hens and growing broilers to dietary omega-3 fatty acid supplementation (Ph. D. thesis). Iowa State University, Ames, IA, United States. p. 1–215. Available online at: https://dr.lib.iastate.edu/handle/20.500.12876/31363
Eusemann, K. B., Patt, A., Schrader, L., Weigend, S., Thone-Reineke, C., and Petow, S. (2020). The role of egg production in the etiology of keel bone damage in laying hens. Front. Veter. Sci. 7, 81. doi: 10.3389/fvets.2020.00081
Hall, L. E., Shirley, R. B., Bakalli, R. I., Aggrey, S. E., Pesti, G. M., and Edwards, H. M. (2003). Power of two methods for the estimation of bone ash of broilers. Poult. Sci. 82, 414–418. doi: 10.1093/ps/82.3.414
Hendrix Genetics (2020). Nutrition Guide. Available online at: https://layinghens.hendrix-genetics.com/documents/883/Nutrition_Guide_English_vs2.pdf (accessed April, 2021).
Josling, G. C., Hugo, A., Fair, M. D., and de Witt, F. H. (2019). Long term effect of dietary lipid saturation on eggshell quality and bone characteristics of laying hens. Poultry Sci. 98, 3593–3601. doi: 10.3382/ps/pez127
Julian, R. J. (2005). Production and growth related disorders and other metabolic diseases of poultry – a review. Veter. J. 169, 350–369. doi: 10.1016/j.tvjl.2004.04.015
Kajarabille, N., Díaz-Castro, J., Hijano, S., López-Frías, M., López-Aliaga, I., and Ochoa, J. J. (2013). A new insight to bone turnover: role of−3 polyunsaturated fatty acids. Sci. World J. 2013, 1–16. doi: 10.1155/2013/589641
Khanal, T., Bedecarrats, G. Y., and Kiarie, E. G. (2021). Cage type and mineral nutrition had independent impact on skeletal development in Lohmann LSL-Lite pullets from hatch to 16 weeks of age. Animal Nutr. 7, 631–640. doi: 10.1016/j.aninu.2020.11.013
Kruger, M. C., Coetzee, M., Haag, M., and Weiler, H. (2010). Long-chain polyunsaturated fatty acids: selected mechanisms of action on bone. Prog. Lipid Res. 49, 438–449. doi: 10.1016/j.plipres.2010.06.002
Lau, B., Cohen, D., Ward, W., and Ma, D. (2013). Investigating the role of polyunsaturated fatty acids in bone development using animal models. Molecules 18, 14203–14227. doi: 10.3390/molecules181114203
Leskovec, J., Levart, A., Žgur, S., Jordan, D., Pirman, T., Salobir, J., et al. (2018). Effects of olive leaf and marigold extracts on the utilization of nutrients and on bone mineralization using two different oil sources in broilers. J. Poultry Sci. 55, 17–27. doi: 10.2141/jpsa.0170059
Lin, L. I. K. (1989). A concordance correlation coefficient to evaluate reproducibility. Biometrics. 45, 255. doi: 10.2307/2532051
Liu, D., Veit, H. P., and Denbow, D. M. (2004). Effects of long-term dietary lipids on mature bone mineral content, collagen, crosslinks, and prostaglandin E2 production in Japanese quail. Poultry Sci. 83, 1876–1883. doi: 10.1093/ps/83.11.1876
Liu, D., Veit, H. P., Wilson, J. H., and Denbow, D. M. (2003). Long-term supplementation of various dietary lipids alters bone mineral content, mechanical properties and histological characteristics of Japanese quail. Poultry Sci. 82, 831–839. doi: 10.1093/ps/82.5.831
Moher, D., Liberati, A., Tetzlaff, J., and Altman, D. G. (2009). Preferred reporting items for systematic reviews and meta-analyses: the PRISMA statement. BMJ. 339. doi: 10.1136/bmj.b2535
NRC (1994). “Nutrient Requirements of Poultry”, 9th revision ed. ational Academy of Sciences Press, Washington, DC.
Onyango, E. M., Hester, P. Y., Stroshine, R., and Adeola, O. (2003). Bone densitometry as an indicator of percentage tibia ash in broiler chicks fed varying dietary calcium and phosphorus levels. Poultry Sci. 82, 1787–1791. doi: 10.1093/ps/82.11.1787
Puzio, I., Jaśkiewicz, T., Sagan, A., Bieńko, M., and Graboś, D. (2012). Effects of cla and camelina sativa seed oil on bone properties in broiler chickens. Bull. Veter. Inst. Pulawy. 56, 93–97. doi: 10.2478/v10213-012-0017-1
Rao, S. K., West, M. S., Frost, T. J., Orban, J. I., Bryant, M. M., and Roland, D. A. (1993). Sample size required for various methods of assessing bone status in commercial leghorn hens. Poultry Sci. 72, 229–235. doi: 10.3382/ps.0720229
Rohatgi, A. (2021). WebPlotDigitizer - Extract data from plots, images, and maps. WebPlotDigitzer. Available online at: https://automeris.io/WebPlotDigitizer (accessed April, 2021).
Ross (2019). Ross. Nutrient Specifications. Available online at http://en.aviagen.com/assets/Tech_Center/Ross_Broiler/RossBroilerNutritionSpecs2019-EN.pdf (accessed April, 2021).
Rufener, C., Baur, S., Stratmann, A., and Toscano, M. J. (2019). Keel bone fractures affect egg laying performance but not egg quality in laying hens housed in a commercial aviary system. Poult. Sci. 98, 1589–1600. doi: 10.3382/ps/pey544
Sauvant, D., Schmidely, P., Daudin, J. J., and St-Pierre, N. R. (2008). Meta-analyses of experimental data in animal nutrition. Animal. 2, 1203–1214. doi: 10.1017/S1751731108002280
Shen, C. L., Yeh, J. K., Rasty, J., Li, Y., and Watkins, B. A. (2006). Protective effect of dietary long-chainn-3 polyunsaturated fatty acids on bone loss in gonad-intact middle-aged male rats. Br. J. Nutr. 95, 462–468. doi: 10.1079/BJN20051664
Siegel, P. B. (2014). Evolution of the modern broiler and feed efficiency. Ann. Rev. Animal Biosci. 2, 375–385. doi: 10.1146/annurev-animal-022513-114132
Skrivan, M., Englmaierová, M., Taubner, T., and Skrivanová, E. (2020). Effects of dietary hemp seed and flaxseed on growth performance, meat fatty acid compositions, liver tocopherol concentration and bone strength of cockerels. Animals 10, 458. doi: 10.3390/ani10030458
St-Pierre, N. R. (2001). Invited review: integrating quantitative findings from multiple studies using mixed model methodology. J. Dairy Sci. 84, 741–755. doi: 10.3168/jds.S0022-0302(01)74530-4
Tarlton, J. F., Avery, N. C., Wilkins, L. J., and Knott, L. (2011). Omega-3 (n3) Fatty Acid Supplemented Diet Reduced Bone Breakage and Increases Bone Sregnth in Free Range Laying Hens. World's Poultry Science Association (UK Meeting) Annual Meeting.
Tarlton, J. F., Wilkins, L. J., Toscano, M. J., Avery, N. C., and Knott, L. (2013). Reduced bone breakage and increased bone strength in free range laying hens fed omega-3 polyunsaturated fatty acid supplemented diets. Bone. 52, 578–586. doi: 10.1016/j.bone.2012.11.003
Tavárez, M. A., and Santos, F. S. (2016). Impact of genetics and breeding on broiler production performance: A look into the past, present, and future of the industry. Anim Front. 6, 37–41. doi: 10.2527/af.2016-0042
Tedeschi, L. O. (2006). Assessment of the adequacy of mathematical models. Agric. Syst. 89, 225–247. doi: 10.1016/j.agsy.2005.11.004
Toscano, M. J., Booth, F., Wilkins, L. J., Avery, N. C., Brown, S. B., Richards, G., et al. (2015). The effects of long (C20/22) and short (C18) chain omega-3 fatty acids on keel bone fractures, bone biomechanics, behavior, and egg production in free-range laying hens. Poultry Sci. 94, 823–835. doi: 10.3382/ps/pev048
Toscano, M. J., Wilkins, L. J., and Tarlton, J. F. (2012). Impact of a mixed chain length omega-3 fatty acid diet on production variables in commercial free-range laying hens. Bri. Poult. Sci. 53, 360–365. doi: 10.1080/00071668.2012.698726
Venäläinen, E., and Valaja, J. (2006). Effects of dietary metabolisable energy, calcium and phosphorus on bone mineralisation, leg weakness and performance of broiler chickens. Br. Poult. Sci. 47, 301–310. doi: 10.1080/00071660600741776
Watkins, B. A., Li, Y., Allen, K. G., Hoffmann, W. E., and Seifert, M. F. (2000). Dietary ratio of (n-6)/(n-3) polyunsaturated fatty acids alters the fatty acid composition of bone compartments and biomarkers of bone formation in rats. J. Nutr. 130, 2274–2284. doi: 10.1093/jn/130.9.2274
Keywords: layer, broiler chickens, omega-3 fatty acids, bone quality, meta-analysis
Citation: Thanabalan A, Ellis J and Kiarie EG (2022) A Meta-Analysis on the Significance of Dietary Omega-3 Fatty Acids on Bone Development and Quality in Egg- and Meat-Type Chickens. Front. Anim. Sci. 3:875944. doi: 10.3389/fanim.2022.875944
Received: 14 February 2022; Accepted: 19 April 2022;
Published: 17 May 2022.
Edited by:
Kendall C. Swanson, North Dakota State University, United StatesReviewed by:
Lynn Weber, University of Saskatchewan, CanadaAlexandru Vlaicu, University of Agronomic Sciences and Veterinary Medicine, Romania
Copyright © 2022 Thanabalan, Ellis and Kiarie. This is an open-access article distributed under the terms of the Creative Commons Attribution License (CC BY). The use, distribution or reproduction in other forums is permitted, provided the original author(s) and the copyright owner(s) are credited and that the original publication in this journal is cited, in accordance with accepted academic practice. No use, distribution or reproduction is permitted which does not comply with these terms.
*Correspondence: Elijah G. Kiarie, ekiarie@uoguelph.ca