- 1Faculty of Arts and Science, Kyushu University, Fukuoka, Japan
- 2Laboratory of Regulation in Metabolism and Behavior, Graduate School of Bioresource and Bioenvironmental Science, Kyushu University, Fukuoka, Japan
- 3Department of Animal and Poultry Behaviour and Management, Faculty of Veterinary Medicine, Aswan University, Aswan, Egypt
- 4Department of Bioresource Science, Graduate School of Biosphere Science, Hiroshima University, Hiroshima, Japan
- 5Department of Biochemistry, Showa University School of Medicine, Tokyo, Japan
- 6School of Veterinary Science, Massey University, Palmerston North, New Zealand
High ambient temperatures (HT) in summer are becoming more severe due to global warming, leading to severe adverse effects on poultry production. Recently, we have reported that oral administration of L-citrulline (L-Cit) can minimize hyperthermia in chickens under HT. However, whether oral L-Cit can enter the brain, the center for thermoregulation, has not been studied. We investigated the effects of oral administration of L-Cit on free amino acids and monoamines in the diencephalon region of the brain of heat-exposed broilers. Broilers were treated with L-Cit (40 mmol/20 ml/bird), then moved to a chamber at HT (30 ± 1°C) or to a thermoneutral temperature (CT: 22 ± 1°C) chamber for 2 h. Control groups were given methyl cellulose solution and placed in the CT or HT chambers. After 2 h of exposure to HT, there were increased brain concentrations of Cit in comparison with concentrations in broilers exposed to CT, whereas brain ornithine (Orn) concentrations were decreased, and arginine (Arg) concentrations were not changed. Interestingly, oral administration of L-Cit increased brain concentration of Cit, Arg, and Orn under both CT and HT. Tryptophan and its metabolite, serotonin (5-HT) concentrations were lower in the brain under HT than under CT. HT did not change brain concentrations of tyrosine, but dopamine (DA, a metabolite of tyrosine) concentrations decreased, and methoxyhydroxyphenylglycol (MHPG, a metabolite of DA) concentrations increased in comparison with CT. Oral administration of L-Cit decreased brain concentrations of both tryptophan and tyrosine under CT and HT without changing 5-HT; however, DA levels declined under HT. Moreover, MHPG concentrations increased. In conclusion, these results suggest that metabolism of amino acids and metabolism of DA can be enhanced in the brain by oral administration of L-Cit. Metabolic changes in the brain in response to oral administration of L-Cit may influence the thermoregulatory center in the brain, leading to a reduction in body temperature and conferring thermotolerance in heat-exposed broiler chickens.
Introduction
Environmental conditions with high ambient temperatures (HT) are becoming a growing global threat for rearing animals (Kjellstrom and McMichael, 2013; Tanizawa et al., 2014). Livestock need to live at temperatures within their thermoneutral zone as otherwise their well-being and productivity will be compromised and they may die (Daramola et al., 2012). In poultry, chickens do not have sweat glands, the body is covered with feathers, and they have a high metabolic rate which makes them very sensitive to heat stress with HT (Yahav and Hurwitz, 1996; Bartlett and Smith, 2003; Soleimani et al., 2010; Chowdhury, 2019). Although management (Saeed et al., 2019) and nutritional approaches (Lin et al., 2006) are being adopted to overcome heat stress concerns at chicken farms, novel approaches are needed based on physiological responses of chickens to heat stress. It has been reported that the blood content of citrulline (Cit) declined in heat-exposed chicks (Chowdhury et al., 2014), and oral administration of L-Cit, but not L-arginine (L-Arg) and L-ornithine (L-Orn), lowered rectal temperature in chicks (Chowdhury et al., 2015). It was further found that oral administration of L-Cit increased thermotolerance in heat-exposed chicks (Chowdhury et al., 2017) and broilers (Chowdhury et al., 2021). However, chickens cannot produce sufficient Cit because chickens are lacking a necessary enzyme (carbamoyl phosphate synthetase) which is required for the synthesis of carbamoyl phosphate which in turn is required for the synthesis of Cit from Orn (Tamir and Ratner, 1963). Therefore, a small amount of Cit is produced as a byproduct when Arg is converted to nitric oxide (NO) by the action of the enzyme NO synthase (NOS). This is the basis for Arg being an essential requirement for inclusion in poultry diets. However, less attention has been given to Cit supplementation, which could be beneficial for chickens under heat stress. Effects of oral administration of L-Cit on overall amino acid metabolism in chickens are not known. Also, it is not known if orally administered Cit can bypass the blood brain-barrier and enter the brain.
In most animals, L-Cit plays roles from cellular metabolism to organ function (Curis et al., 2005). L-Cit, is produced from L-Arg by the enzymatic action of NOS when NO is produced (Palmer et al., 1987). NO is well-known as a regulator of body temperature (De Luca et al., 1995; Osaka, 2010). However, orally administered L-Cit reduced body temperature in chicks without changing plasma NO concentration in chicks (Chowdhury et al., 2017). To clarify the function of L-Cit, therefore, we focused on brain metabolism in the present study since the hypothalamus is the center of regulation of body temperature (Romanovsky, 2007).
There are several reports showing that amino acids are also important in the regulation of body temperature (Erwan et al., 2014; Chowdhury et al., 2015, 2017; Han et al., 2017; Elhussiny et al., 2021). Brain monoamines can regulate different behaviors including thermoregulation (Denbow et al., 1981). It was reported that intracerebroventricular (ICV) injection of epinephrine (E) and norepinephrine (NE) decreased rectal temperature in chicks (Denbow et al., 1981). Bahry et al. (2017) showed that ICV injection of neuropeptide Y, which is a hypothermic agent, stimulated catecholamine metabolism in chicks. Therefore, the aim of this study was to examine amino acid and monoamine metabolism in chicks treated with oral administration of L-Cit.
Materials and Methods
Animals
One-day-old chunky male broiler chicks (Chunky: Ross 308; Gallus gallus domesticus) were purchased from a local hatchery (Fukuda Hatchery, Okayama, Japan) and housed in groups of 7–12 chicks in polypropylene boxes (50 × 35 × 33 cm) at a constant temperature of 30 ± 1°C and with continuous light until the subjects reached 3 days of age. The temperature of the room was gradually decreased to 24°C when the chicks reached 14 days of age. The chicks were housed without an adult present. A commercial starter diet (22% CP, 3100 kcal/kg ME; Nichiwa Sangyo Co. Ltd., Kobe, Japan) and water were provided ad libitum. For acclimation, broilers were isolated in individual cages (23 × 40 × 45 cm) on day 26. Birds were assigned to treatment and control groups 48 h before the start of the experiment so that mean body weights of chicks were similar for both groups. The broilers had free access to food and water throughout the experimental period. This study was performed in accordance with the guidelines of the Animal Experiments Committee at Hiroshima University, Japan (authorization No. C19–15).
Preparation of L-Cit Solution
L-Cit was purchased from Kyowa Hakko Bio Co., Ltd. (Yamaguchi, Japan). L-Cit was suspended in 0.25% methyl cellulose solution, as described elsewhere (Chowdhury et al., 2017), because it is difficult to dissolve L-Cit in water. The suspensions were stirred well in a vortexer before oral administration. A 0.25% methyl cellulose solution was used as the control treatment. The solutions were kept at room temperature (22 ± 1°C) during the experiments.
Experimental Design
This manuscript describes results from the analyses of samples collected in the animal experiment published recently (Chowdhury et al., 2021). We have separated the amino acid analyses in the current paper from previously published data in order to focus on metabolism after considering behavior in the previous paper. Therefore, the experimental design is the same as reported recently (Chowdhury et al., 2021). Briefly, a total of 32 broilers (28 days old; n = 8/group; average body weight: 1.8 kg) were divided into four groups based on their initial body weight to produce uniform groups. One group of broilers received two oral doses of L-Cit based on our previous findings in chicks (Chowdhury et al., 2015, 2017) using an elastic–plastic needle on a syringe. Two doses were used as described in a previous study (Chowdhury et al., 2021) to provide thermotolerance. Broilers were given two oral doses of L-Cit (20 mmol/10 ml/bird/dose) before being moved to HT (30 ± 1°C) or control thermoneutral temperature (CT: 22 ± 1°C) chambers. After the first oral dose of L-Cit, the broilers remained at room temperature for 60 min then the second oral dose was given. Thirty minutes later, birds were moved to individual temperature-controlled chambers (inner chamber size: 90 × 90 × 115 cm) at HT or CT for 120 min. The control group was administered 10 ml of 0.25% methyl cellulose solution twice in the same manner as the treatment group before exposure to HT or CT in temperature-controlled chambers (inner chamber size: 90 × 90 × 115 cm) for 120 min. At the end of the experiment, broilers were anesthetized using isoflurane (FUJIFILM Wako Pure Chemical Corporation, Osaka, Japan) then trunk blood was collected into a heparinized tube. Blood samples were centrifuged at 10,000× g at 4°C for 4 min to separate the plasma, which was then stored at − 80 °C until further analysis. After euthanasia, the brains were dissected and the diencephalons (thalamus and hypothalamus) were collected as described elsewhere (Chowdhury et al., 2014). Brain samples were snap-frozen and stored at – 80°C until free amino acids and monoamines analyses were performed.
Analysis of Free Amino Acids in the Diencephalon and Plasma
Free amino acid concentrations were measured by high-performance liquid chromatography (HPLC) following the method of Boogers et al. (2008) with some modifications. The diencephalon was homogenized in ice-cold 0.2 M perchloric acid solution containing 0.01 mM ethylenediaminetetraacetic acid disodium salt (EDTA-2Na) and left on ice for deproteinization. Thirty minutes later, homogenates were centrifuged at 20,000 g for 15 min at 4°C, then supernatants were adjusted to pH 7 with 1 M sodium hydroxide. Plasma was prepared by centrifuging at 12,000 g for 10 min at 4°C followed by ultrafiltration (Millipore, Bedford, USA). Twenty μl of each diencephalon supernatant and 10 μl of each plasma sample were dried under reduced pressure. The dried residues were dissolved in 10 μl 1 M sodium acetate-methanol-triethylamine (2:2:1), dried again under reduced pressure, then converted to their phenylthiocarbamoyl derivatives by dissolving each sample in 20 μl methanol-distilled water-triethylamine-phenylisothiocyanate (7:1:1:1) and allowing them to react for 20 min at room temperature. The samples were dried again and dissolved in 200 μl of Pico-Tag Diluent (Waters, Milford, CT, USA). These diluted samples were filtered through a 0.20 μm filter (Millipore, Bedford, USA). The same methods were followed for standard solutions prepared by dilution of an L-amino acid solution (type ANII, type B, L-asparagine, L-glutamine and L-tryptophan; Wako, Osaka, Japan) with distilled water. Each solution of sample or standard containing the derivatives was applied to a Waters HPLC system. The system was equilibrated with buffer A (70 mM sodium acetate adjusted to pH 6.45 with 10% acetic acid-acetonitrile, ratio 975:25) and eluted with a linear gradient of buffer B (water-acetonitrile-methanol (40:45:15) at 0, 3, 6, 9, 40, and 100%) at a flow rate of 1 ml/min at 46°C. Concentrations of free amino acids were determined by absorbance at a wavelength of 254 nm. The amino acid concentrations in the diencephalon were expressed as pmol/mg wet tissue and plasma amino acid concentrations were expressed as pmol/μl. The system did not distinguish between L- and D-isomers; thus, only names of amino acids are provided in the obtained results.
Analysis of Diencephalic Monoamines
Monoamine concentrations of dopamine (DA), 3,4-dihydroxyphenylacetic acid (DOPAC), homovanillic acid (HVA), E, NE, 3-methoxy-4-hydroxyphenylglycol (MHPG), serotonin (5-HT), and 5 hydroxyindoleacetic acid (5-HIAA) in the diencephalon were measured using HPLC following the method described elsewhere (Bahry et al., 2017; Elhussiny et al., 2021). Briefly, the diencephalon samples were homogenized in ice-cold 0.2 M perchloric acid and left for 30 min on ice for deproteinization. Homogenates were then centrifuged at 20,000 g at 4°C for 15 min, then filtered through 0.2 μm hydrophilic polytetrafluoroethylene filters (Millipore, Bedford, USA). 50 ul of filtrate from each diencephalon sample was adjusted to approximately pH 3 by adding 1 M sodium acetate and was then applied to a HPLC system (Eicom Co. wLtd., Kyoto, Japan) with a 150 mm × 3 mm octadecylsilane (ODS) column (EICOMPAK SC-5ODS; Eicom) and an electrochemical detector (ECD-300, Eicom) at an applied potential of +0.75 V vs. an Ag/AgCl reference analytical electrode. Electric current (nA) changes were recorded on a computer using an interface system (Power Chromver 2.3.2. J: AD Instruments, Tokyo, Japan). The mobile phase (0.1 M sodium acetate, 0.1 M citric acid, 100 mg/ml sodium 1-octane sulfonate, and 5 mg/ml disodium ethylenediaminetetraacetic acid and methanol) was used at a flow rate of 0.5 ml/min. External standard solutions of each monoamine (100 pg/μl) were serially diluted with 0.01 N HCl. Brain monoamine concentrations were expressed as pg/mg wet tissue.
Statistical Analysis
Mean amino acid and monoamine concentrations were compared between treatments and temperatures using two-way ANOVA followed by Tukey's multiple comparison tests when interactions were significant. Significant differences were denoted as P < 0.05. Statistical analyses were performed using GraphPad Prism 8 (GraphPad Software Inc., San Diego, CA, USA). Values are presented as mean ± SEM. All data in each group were first subjected to a Thompson's rejection test as described by Kobayashi and Pillai (2013) to eliminate outliers (P < 0.01), with remaining data were used for the analyses.
Results
Effects of L-Cit on Brain and Plasma Free Amino Acid Concentrations
Free amino acid concentrations in the brain and plasma of broiler chickens are shown in Tables 1, 2. Brain and plasma concentrations of Cit were significantly higher (P < 0.05) in broilers exposed to HT compared with CT. Plasma Arg was higher and brain Orn was lower in HT compared with CT conditions. Brain and plasma concentrations of many free amino acid concentrations differed between HT and CT conditions (see Tables 1, 2). Interestingly, brain and plasma concentrations of Cit, Arg, and Orn were higher (P < 0.0001) after oral administration of L-Cit under both CT and HT. Brain concentrations of phenylalanine (Phe, P < 0.0001), tryptophan (Trp, P < 0.05) and tyrosine (Tyr, P < 0.0001) were lower, and cystathionine and taurine were significantly (P < 0.0001) higher after oral administration of L-Cit (Table 1). Plasma taurine was also higher (P < 0.0001) after L-Cit treatment. Cystathionine was lower in the brain and higher in plasma after exposure to HT. Both brain and plasma taurine concentrations were significantly (P < 0.0001) increased by oral L-Cit. Brain and plasma concentrations of other free amino acids were increased, decreased or not changed by the L-Cit treatment or by exposure to HT (Tables 1, 2).
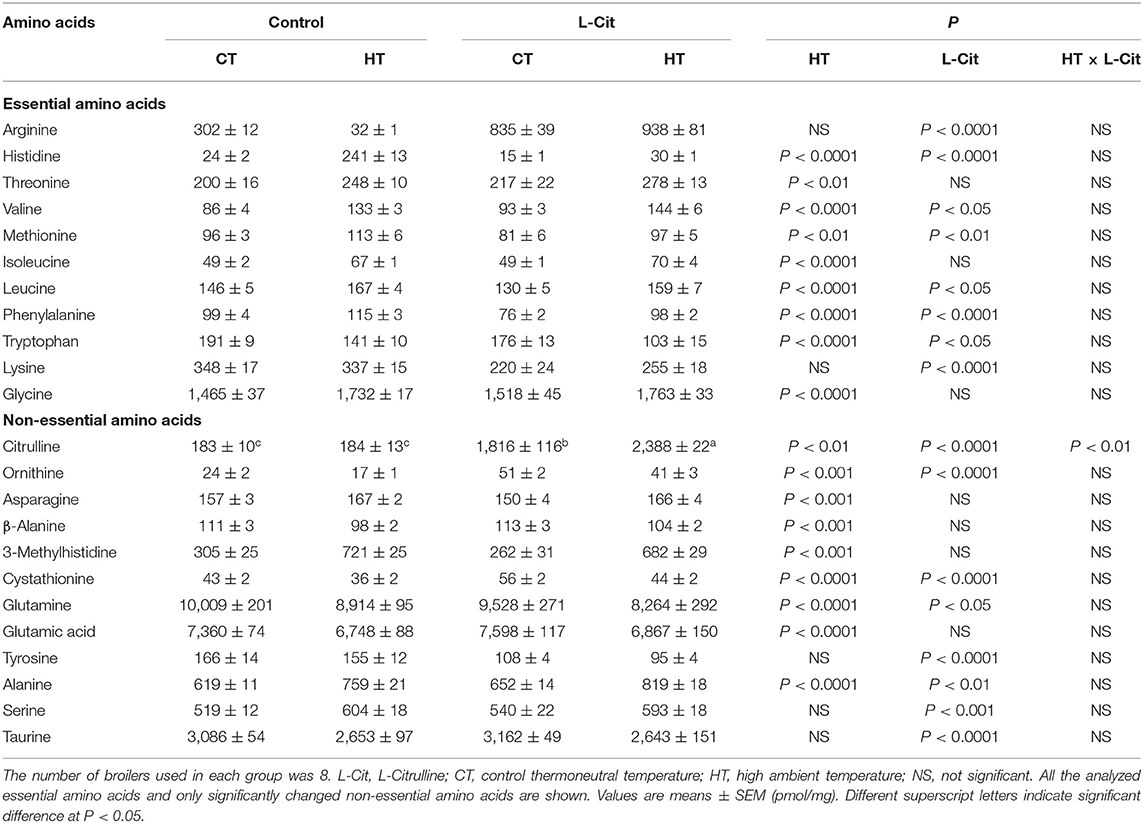
Table 1. Effects of oral administration of L-Cit on free amino acid concentrations in the diencephalon of broilers.
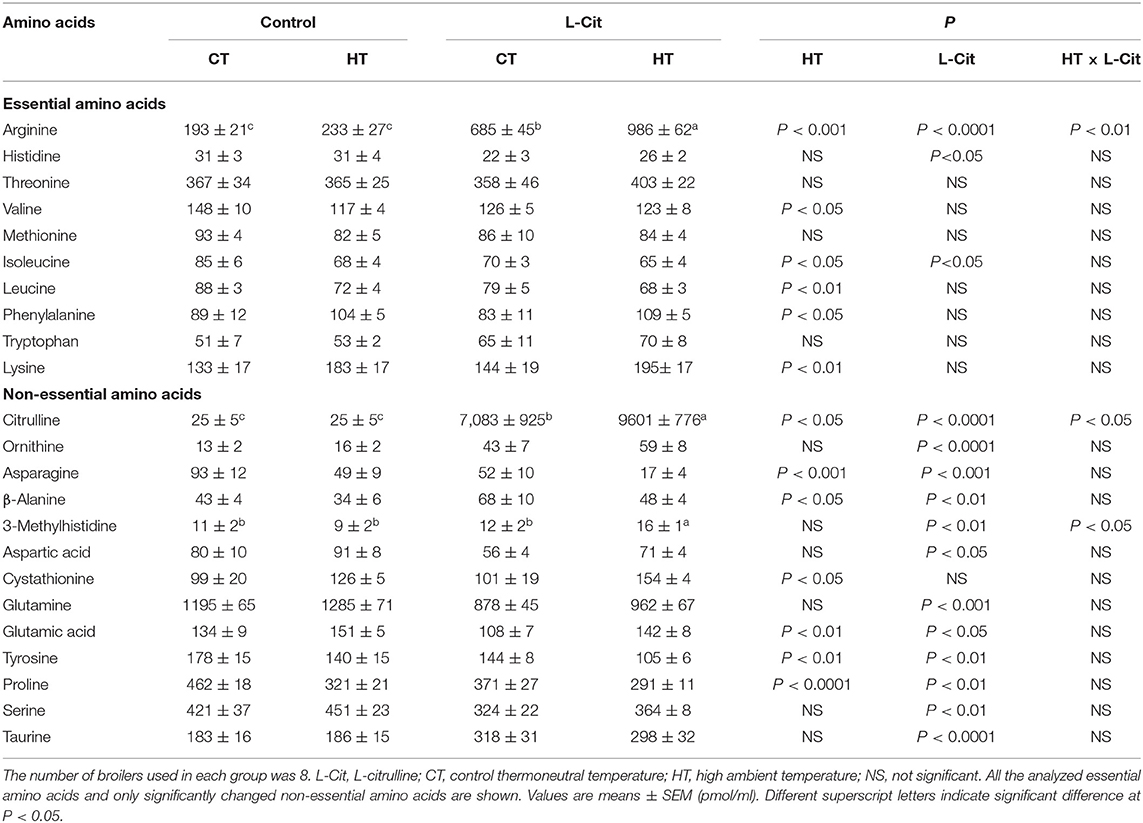
Table 2. Effects of oral administration of L-Cit on free amino acid concentrations in the plasma of broilers.
Effects of L-Cit on Brain Monoamine Concentrations
Concentrations of monoamines and their metabolites in the diencephalon are shown in Table 3. Brain E (P < 0.0001), NE (P < 0.0001), DA (P < 0.0001), DOPAC (P < 0.05), 5-HT (P < 0.0001), and 5-HIAA (P < 0.0001) concentrations were significantly lower after exposure to HT, while MHPG (P < 0.05) concentrations were significantly higher. The MHPG/NE and 5-HIAA/5-HT ratios were significantly increased by HT. Oral administration of L-Cit caused higher (P < 0.01) diencephalic MHPG, with a difference in the MHPG/NE ratio that approached being significantly lower (P = 0.0502). There were significant interactions between L-Cit treatment and temperature for DA, HVA and 5-HIAA concentrations, indicating that differences between groups did not follow the same patterns for the two experimental variables. DA and HVA were lower after L-Cit treatment under HT but higher under CT; however, reverse was true for 5-HIAA.
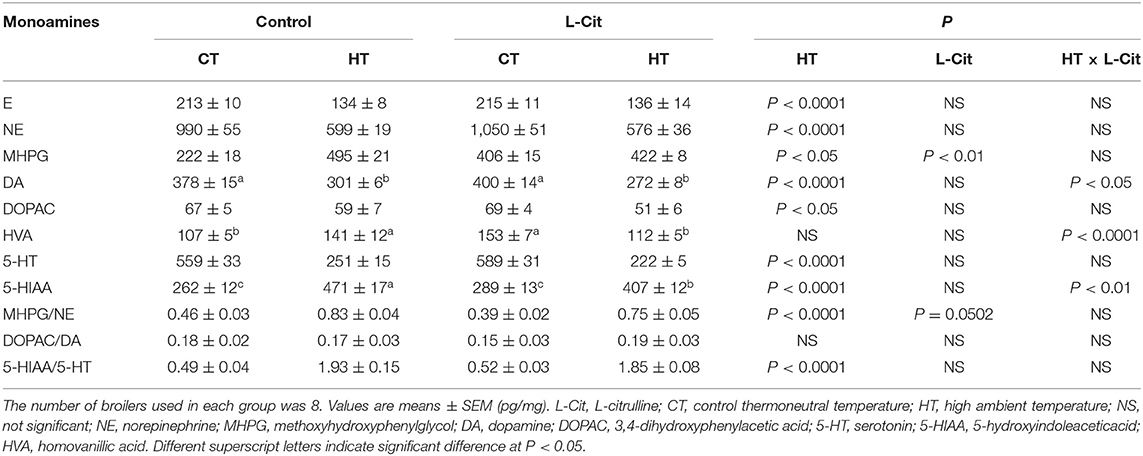
Table 3. Effects of oral administration of L-Cit on concentrations of monoamines and their metabolites in the diencephalon of broilers.
Discussion
The aim of the current study was to clarify the mechanism by which oral administration of L-Cit has hypothermic effects and to explain the involvement of brain metabolism of amino acids and monoamines in these effects. Chowdhury et al. (2021) showed that a similar dose of L-Cit afforded thermotolerance in broiler chickens. The hypothalamus is involved in body temperature regulation in pigeons (Akerman et al., 1960; Hissa and Rautenberg, 1974, 1975) and chickens (Kanematsu et al., 1967), so it is important to examine metabolism of functional factors such as amino acids and monoamines in the brain. The present results show that the so-called urea-cycle amino acids, Cit, Arg, and Orn, all increased in the diencephalon and plasma after L-Cit treatment (Tables 1, 2). On the other hand, Arg produces Cit and Orn in pathways other than the urea cycle. When Arg produces Cit, then NO is synthesized. Orn produced from Arg can be in the first step of creatine synthesis. Arg also produces polyamines through Orn, and polyamines are associated with cell proliferation, cell repair and wound healing (Wu and Morris, 1998). A recent study showed that the kidney may be a major peripheral tissue of polyamine synthesis in broiler chickens (Furukawa et al., 2021). Further study of brain NO and polyamines in heat-exposed chickens subjected to oral administration of L-Cit, including isonitrogenous control groups, is needed.
Since oral administration of L-Cit greatly enhanced both plasma and diencephalic Cit, it is clear that L-Cit can be transported through the blood brain-barrier and can regulate brain neuronal functions. Lee and Kang (2017) reported that L-Cit was transported via the large neutral amino acid transporter 1 (LAT1) in a rat brain capillary endothelial cell line and suggested the possibility that L-Cit to serve as therapeutic approaches to improve its neuroprotective effects. Oral L-Cit might have been transported via LAT1 in chickens, leading to increased brain content of Cit. Arg was present in plasma and increased after oral administration of L-Cit. This Arg increase in plasma clearly influenced the increment of brain Arg. On the other hand, plasma Orn was also increased with oral administration of L-Cit, but the increment was small compared with those of Cit and Arg. Levels of brain Orn were also lower than those of Cit and Arg in control birds. The increase in brain Orn after oral administration of L-Cit was small and this was due to small changes in plasma Orn. Furthermore, brain Orn was decreased by HT with or without oral administration of L-Cit. The reason for this is unknown, but brain Orn levels can be an indicator of heat stress.
In the current study, free amino acid concentrations in brain and blood plasma were influenced by 2 h of HT. In particular, several essential amino acids showed an upward tendency in the brain, namely histidine, threonine, valine, methionine, isoleucine, leucine, Phe, lysine, and glycine, whereas only Trp declined. The reason for the increase of all essential amino acids in connection to thermoregulation is unknown, but we suggest that increased concentrations of essential amino acid may be the result of an increased rate of the brain protein breakdown. 3-methyhistidine, a marker of proteolysis (Young and Munro, 1978; Nishizawa, 1983), was greatly increased in the brain by HT. Interestingly, most of the increases of brain essential amino acids were minimized by oral administration of L-Cit. These findings suggest that oral administration of L-Cit may reduce brain protein breakdown and could confer neuroprotection under heat stress. The pattern of changes in free amino acids in the plasma had some dissimilarities with that of the brain, which indicate that brain-dependent amino acid metabolism occurred during heat stress and oral administration of L-Cit.
Trp is the precursor of 5-HT, and both brain Trp and 5-HT concentrations were decreased by HT in the present study (Tables 1, 3). Yoshida et al. (2015) demonstrated that ICV injection of Trp attenuated stress behaviors, and ICV injection of 5-HT caused sedative effects in chicks. Recently, it was found that intraperitoneal injection of L-Trp reduced rectal temperature and serum testosterone in heat-exposed chicks (Badakhshan et al., 2021). Therefore, it could be postulated that heat stress might stimulate 5-HT metabolism in the brain, leading to the activation of mechanisms to cope with high ambient temperatures. L-Cit might have further enhanced this process, as brain Trp concentrations declined after oral administration of L-Cit, although 5-HT concentrations were not changed by the L-Cit treatment.
Tyr is the precursor of DA. Brain Tyr concentrations did not change after HT, whereas brain DA and its metabolites, E and NE decreased. It has been reported that injection of E and NE into the lateral ventricle of chickens can decrease rectal temperature (Grunden and Marley, 1970; Scott and van Tienhoven, 1974; Hillman et al., 1977; Denbow et al., 1981). The experimental HT in the present study might have activated catecholamine metabolism to limit the heat stress response. In the present study, oral administration of L-Cit reduced brain concentrations of Tyr, and reduced brain DA concentrations under HT. Infusion of DA into the hypothalamus induced sleep and lowering of body temperate in chickens (Marley and Nistico, 1972). It can be suggested that oral administration of L-Cit could enhance the utilization of brain DA to afford thermotolerance.
In the diencephalon, HT increased the MHPG/NE and 5-HIAA/5-HT ratios compared to CT conditions. These increases imply that the release of NE and 5-HT may have increased, and that metabolism of NE to MHPG and of 5-HT to 5-HIAA may have been activated. It can be considered that these changes reflect activation of brain serotonergic and dopaminergic systems. Oral administration of L-Cit showed a tendency to decrease the MHPG/NE ratio compared to the control group, which indicates that release of NE may have been reduced and the metabolism of NE to MHPG may have been decreased.
Brain and plasma taurine concentrations were increased by oral administration of L-Cit in the present study. Recently, Elhussiny et al. (2021, 2022) reported that ICV injection of taurine lowered rectal temperature and attenuated stress responses in chicks. Therefore, it could be thought that increased brain and plasma taurine, as a result of oral L-Cit treatment, could help to ameliorate the heat stress response in chickens.
Brain and plasma concentrations of several other free amino acids, including cystathionine, differed between control and L-Cit treatment groups or between CT and HT conditions (see Tables 1, 2). In the present study, cystathionine concentrations were lower after HT, and oral L-Cit increased concentrations of cystathionine. Cystathionine is synthesized from serine and homocysteine in a reaction catalyzed by cystathionine β-synthase (Aitken et al., 2011; Yamada et al., 2012). Brain concentrations of serine were elevated by the oral administration of L-Cit. Oral L-Cit may have enhanced cystathionine synthesis, which might provide protection from brain injury under HT as it was found that cystathionine β-synthase expression increased in the mouse brain to protect it after traumatic injury (Zhang et al., 2013).
In conclusion, these results suggest that metabolism of amino acids and metabolism of DA can be enhanced in the brain by oral administration of L-Cit. The current results further suggest that, after oral administration, L-Cit can be transported through the blood-brain-barrier and can change brain free amino acid concentrations and monoamine metabolism. Metabolic changes in the brain in response to oral administration of L-Cit may influence the thermoregulatory center in the brain, leading to a reduction in body temperature and conferring thermotolerance in heat-exposed broiler chickens.
Data Availability Statement
The original contributions presented in the study are included in the article, further inquiries can be directed to the corresponding author.
Ethics Statement
This study was performed in accordance with the guidelines of the Animal Experiments Committee at Hiroshima University, Japan (authorization No. C19–15).
Author Contributions
VC and TB designed the study. GH, VC, TB, and YO collected and analyzed the data. VC and ME wrote the manuscript. JC, TB, MF, GH, PT, HN, and SH edited the manuscript. All authors discussed the results, contributed to manuscript revisions, and approved the manuscript for publication.
Funding
This study was supported by Grants-in-Aid for Scientific Research (Grant Nos. JP18K19271 and JP19H03110 to VC) from the Japan's Society for the Promotion of Science.
Conflict of Interest
The authors declare that the research was conducted in the absence of any commercial or financial relationships that could be construed as a potential conflict of interest.
Publisher's Note
All claims expressed in this article are solely those of the authors and do not necessarily represent those of their affiliated organizations, or those of the publisher, the editors and the reviewers. Any product that may be evaluated in this article, or claim that may be made by its manufacturer, is not guaranteed or endorsed by the publisher.
Acknowledgments
The authors acknowledge the staff of the Laboratory of Animal Behavior and Physiology, Hiroshima University, for their technical support in maintaining the broilers.
References
Aitken, S. M., Lodha, P. H., and Morneau, D. J. (2011). The enzymes of the transsulfuration pathways: active-site characterizations. Biochim. Biophys. Acta 1814, 1511–1517. doi: 10.1016/j.bbapap.2011.03.006
Akerman, B., Anderson, B., Fabricius, E., and Svensson, L. (1960). Observations on central regulation of body temperature and of food and water intake in the pigeon (Columba livia). Acta Physiol. Scand. 50, 328–336. doi: 10.1111/j.1748-1716.1960.tb00187.x
Badakhshan, Y., Emadi, L., Esmaeili-Mahani, S., and Nazifi, S. (2021). The effect of L-tryptophan on the food intake, rectal temperature, and blood metabolic parameters of 7-day-old chicks during feeding, fasting, and acute heat stress. Iran. J. Vet. Res. 22, 55–64. doi: 10.22099/ijvr.2020.37266.5428
Bahry, M. A., Chowdhury, V. S., Yang, H., Tran, P. V., Do, P. H., Han, G., et al. (2017). Central administration of neuropeptide Y differentially regulates monoamines and corticosterone in heat-exposed fed and fasted chicks. Neuropeptides 62, 93–100. doi: 10.1016/j.npep.2016.11.008
Bartlett, J. R., and Smith, M. O. (2003). Effects of different levels of zinc on the performance and immunocompetence of broilers under heat stress. Poult. Sci. 82, 1580–1588. doi: 10.1093/ps/82.10.1580
Boogers, I., Plugge, W., Stokkermans, Y. Q., and Duchateau, A. L. (2008). Ultra-performance liquid chromatographic analysis of amino acids in protein hydrolysates using an automated pre-column derivatisation method. J. Chromatogr. A 1189, 406–409. doi: 10.1016/j.chroma.2007.11.052
Chowdhury, V. S.. (2019). Heat stress biomarker amino acids and neuropeptide afford thermotolerance in chicks. J. Poult. Sci. 56, 1–11. doi: 10.2141/jpsa.0180024
Chowdhury, V. S., Han, G., Bahry, M. A., Tran, P. V., Do, P. H., Yang, H., et al. (2017). L-Citrulline acts as potential hypothermic agent to afford thermotolerance in chicks. J. Therm. Biol. 69, 163–170. doi: 10.1016/j.jtherbio.2017.07.007
Chowdhury, V. S., Ouchi, Y., Han, G., Eltahan, H. M., Haraguchi, S., Miyazaki, T., et al. (2021). Oral administration of L-citrulline changes the concentrations of plasma hormones and biochemical profile in heat-exposed broilers. Anim. Sci. J. 92:e13578. doi: 10.1111/asj.13578
Chowdhury, V. S., Shigemura, A., Erwan, E., Ito, K., Bahry, M. A., Tran, P. V., et al. (2015). Oral administration of L-citrulline, but not L-arginine or L-ornithine, acts as a hypothermic agent in chicks. J. Poult. Sci. 52, 331–335. doi: 10.2141/jpsa.0150014
Chowdhury, V. S., Tomonaga, S., Ikegami, T., Erwan, E., Ito, K., Cockrem, J. F., et al. (2014). Oxidative damage and brain concentrations of free amino acid in chicks exposed to high ambient temperature. Comp. Biochem. Physiol. A. Mol. Integr. Physiol. 169, 70–76. doi: 10.1016/j.cbpa.2013.12.020
Curis, E., Nicolis, I., Moinard, C., Osowska, S., Zerrouk, N., Bénazeth, S., et al. (2005). Almost all about citrulline in mammals. Amino Acids 29, 177–205. doi: 10.1007/s00726-005-0235-4
Daramola, J. O., Abioja, M. O., and Onagbesan, O. M. (2012). “Heat Stress Impact on Livestock, Production,” in Environmental Stress and Amelioration in Livestock Production, eds V., Sejian, S. M. K. Naqvi, T. Ezeji, J. Lakritz, and R. Lal (Berlin; Hiedelberg: Springer), 53–73. doi: 10.1007/978-3-642-29205-7_3
De Luca, B., Monda, M., and Sullo, A. (1995). Changes in eating behavior and thermogenic activity following inhibition of nitric oxide formation. Am. J. Physiol. 268, R1533–R1538. doi: 10.1152/ajpregu.1995.268.6.R1533
Denbow, D. M., Cherry, J. A., Siegel, P. B., and Van Krey, H. P. (1981). Eating, drinking and temperature response of chicks to brain catecholamine injections. Physiol. Behav. 27, 265–269. doi: 10.1016/0031-9384(81)90268-7
Elhussiny, M. Z., Tran, P. V., Pham, C. V., Nguyen, L. T. N., Haraguchi, S., Gilbert, E. R., et al. (2021). Central GABAA receptor mediates taurine-induced hypothermia and possibly reduces food intake in thermo-neutral chicks and regulates plasma metabolites in heat-exposed chicks. J. Therm. Biol. 98:102905. doi: 10.1016/j.jtherbio.2021.102905
Elhussiny, M. Z., Tran, P. V., Tsuru, Y., Haraguchi, S., Gilbert, E. R., Cline, M. A., et al. (2022). Central taurine attenuates hyperthermia and isolation stress behaviors augmented by corticotropin-releasing factor with modifying brain amino acid metabolism in neonatal chicks. Metabolites 12:83. doi: 10.3390/metabo12010083
Erwan, E., Chowdhury, V. S., Nagasawa, M., Goda, R., Otsuka, T., Yasuo, S., et al. (2014). Oral administration of D-aspartate, but not L-aspartate, depresses rectal temperature and alters plasma metabolites in chicks. Life Sci. 109, 65–71. doi: 10.1016/j.lfs.2014.05.015
Furukawa, K., He, W., Bailey, C. A., Bazer, F. W., Toyomizu, M., and Wu, G. (2021). Polyamine synthesis from arginine and proline in tissues of developing chickens. Amino Acids 53, 1739–1748. doi: 10.1007/s00726-021-03084-7
Grunden, L. R., and Marley, E. (1970). Effects of sympathomimetic amines injected into the third cerebral ventricle in adult chickens. Neuropharmacology 9, 119–128. doi: 10.1016/0028-3908(70)90055-9
Han, G., Yang, H., Bahry, M. A., Tran, P. V., Do, P. H., Ikeda, H., et al. (2017). L-Leucine acts as a potential agent in reducing body temperature at hatching and affords thermotolerance in broiler chicks. Comp. Biochem. Physiol. A. Mol. Integr. Physiol. 204, 48–56. doi: 10.1016/j.cbpa.2016.10.013
Hillman, P. E., Scott, N. R., and van Tienhoven, A. (1977). Impact of centrally applied biogenic amines upon the energy balance of fowl. Am. J. Physiol. 232, R137–R144. doi: 10.1152/ajpregu.1977.232.5.R137
Hissa, R., and Rautenberg, W. (1974). The influence of centrally applied noradrenaline on shivering and body temperature in the pigeon. J. Physiol. 238, 427–435. doi: 10.1113/jphysiol.1974.sp010534
Hissa, R., and Rautenberg, W. (1975). Thermoregulatory effects of intrahypothalamic injections of neurotransmitters and their inhibitors in the pigeon. Comp. Biochem. Physiol. A. Comp. Physiol. 51, 319–326. doi: 10.1016/0300-9629(75)90376-x
Kanematsu, S., Kii, M., Sonoda, T., and Kato, Y. (1967). Effects of hypothalamic lesions on body temperature in the chicken. Jpn. J. Vet. Sci. 29, 95–104. doi: 10.1292/jvms1939.29.95
Kjellstrom, T., and McMichael, A. J. (2013). Climate change threats to population health and well-being: the imperative of protective solutions that will last. Glob. Health Action 6:20816. doi: 10.3402/gha.v6i0.20816
Kobayashi, K., and Pillai, K. S. (2013). “Transformation of data and outliers,” in A Handbook of Applied Statistics in Pharmacology, eds K. Kobayashi and K. S. Pillai (New York, NY: Taylor & Francis Group; CRC Press), 37–46. doi: 10.1201/b13152-7
Lee, K., and Kang, Y. (2017). Characteristics of L-citrulline transport through blood-brain barrier in the brain capillary endothelial cell line (TR-BBB cells). J. Biomed. Sci. 24:28. doi: 10.1186/s12929-017-0336-x
Lin, H., Jiao, H., Buyse, J., and Decuypere, E. (2006). Strategies for preventing heat stress in poultry. World's Poult. Sci. J. 62, 71–86. doi: 10.1079/WPS200585
Marley, E., and Nistico, G. (1972). Effects of catecholamines and adenosine derivatives given into the brain of fowls. Br. J. Pharmacol. 46, 619–636. doi: 10.1111/j.1476-5381.1972.tb06888.x
Nishizawa, N.. (1983). Development and its application of a method to estimate catabolic rate of myofibrillar proteins by measuring urinary excretion of N-methylhistidine. J. Jpn. Soc. Nutr. Food Sci. 36, 409–423. doi: 10.4327/jsnfs.36.409
Osaka, T.. (2010). Nitric oxide mediates noradrenaline-induced hypothermic responses and opposes prostaglandin E2-induced fever in the rostromedial preoptic area. Neuroscience 165, 976–983. doi: 10.1016/j.neuroscience.2009.10.069
Palmer, R. M., Ferrige, A. G., and Moncada, S. (1987). Nitric oxide release accounts for the biological activity of endothelium-derived relaxing factor. Nature 327, 524–526. doi: 10.1038/327524a0
Romanovsky, A. A.. (2007). Thermoregulation: some concepts have changed. Functional architecture of the thermoregulatory system. Am. J. Physiol. Regul. Integr. Comp. Physiol. 292, R37–R46. doi: 10.1152/ajpregu.00668.2006
Saeed, M., Abbas, G., Alagawany, M., Kamboh, A. A., Abd El-Hack, M. E., Khafaga, A. F., et al. (2019). Heat stress management in poultry farms: a comprehensive overview. J. Therm. Biol. 84, 414–425. doi: 10.1016/j.jtherbio.2019.07.025
Scott, N. R., and van Tienhoven, A. (1974). Biogenic amines and body temperature in the hen Gallus domesticus. Am. J. Physiol. 227, 1399–1405. doi: 10.1152/ajplegacy.1974.227.6.1399
Soleimani, A. F., Kasim, A., Alimon, A. R., Meimandipour, A., and Zulkifli, I. (2010). Ileal endogenous amino acid flow of broiler chickens under high ambient temperature. J. Anim. Physiol. Anim. Nutr. 94, 641–647. doi: 10.1111/j.1439-0396.2009.00951.x
Tamir, H., and Ratner, S. (1963). Enzymes of arginine metabolism in chicks. Arch. Biochem. Biophys. 102, 249–258. doi: 10.1016/0003-9861(63)90178-4
Tanizawa, H., Shiraishi, J., Kawakami, S., Tsudzuki, M., and Bungo, T. (2014). Effect of short-term thermal conditioning on physiological and behavioral responses to subsequent acute heat exposure in chicks. J. Poult. Sci. 51, 80–86. doi: 10.2141/jpsa.0130040
Wu, G., and Morris, S. M. Jr. (1998). Arginine metabolism: nitric oxide and beyond. Biochem. J. 336, 1–17. doi: 10.1042/bj3360001
Yahav, S., and Hurwitz, S. (1996). Induction of thermotolerance in male broiler chickens by temperature conditioning at an early age. Poult. Sci. 75, 402–406. doi: 10.3382/ps.0750402
Yamada, H., Akahoshi, N., Kamata, S., Hagiya, Y., Hishiki, T., Nagahata, Y., et al. (2012). Methionine excess in diet induces acute lethal hepatitis in mice lacking cystathionine γ-lyase, an animal model of cystathioninuria. Free Radic. Biol. Med. 52, 1716–1726. doi: 10.1016/j.freeradbiomed.2012.02.033
Yoshida, J., Erwan, E., Chowdhury, V. S., Ogino, Y., Shigemura, A., Denbow, D. M., et al. (2015). Comparison of centrally injected tryptophan-related substances inducing sedation in acute isolation stress-induced neonatal chicks. Pharmacol. Biochem. Behav. 129, 1–6. doi: 10.1016/j.pbb.2014.11.016
Young, V. R., and Munro, H. N. (1978). Ntau-methylhistidine (3-methylhistidine) and muscle protein turnover: an overview. Fed. Proc. 37, 2291–2300.
Keywords: amino acid metabolism, brain, heat stress, L-citrulline, poultry
Citation: Chowdhury VS, Han G, Elhussiny MZ, Ouchi Y, Tran PV, Nishimura H, Haraguchi S, Cockrem JF, Bungo T and Furuse M (2022) Oral Administration of L-Citrulline Changes Brain Free Amino Acid and Monoamine Metabolism in Heat-Exposed Broiler Chickens. Front. Anim. Sci. 3:875572. doi: 10.3389/fanim.2022.875572
Received: 14 February 2022; Accepted: 02 March 2022;
Published: 05 April 2022.
Edited by:
Graham Cliff Lamb, Texas A&M University College Station, United StatesReviewed by:
Michael Carey Satterfield, Texas A&M University, United StatesGuoyao Wu, Texas A&M University, United States
Copyright © 2022 Chowdhury, Han, Elhussiny, Ouchi, Tran, Nishimura, Haraguchi, Cockrem, Bungo and Furuse. This is an open-access article distributed under the terms of the Creative Commons Attribution License (CC BY). The use, distribution or reproduction in other forums is permitted, provided the original author(s) and the copyright owner(s) are credited and that the original publication in this journal is cited, in accordance with accepted academic practice. No use, distribution or reproduction is permitted which does not comply with these terms.
*Correspondence: Vishwajit S. Chowdhury, vc-sur@artsci.kyushu-u.ac.jp
†Present address: Guofeng Han, Department of Animal Nutrition and Food Science, College of Animal Science and Technology, Nanjing Agricultural University, Nanjing, China