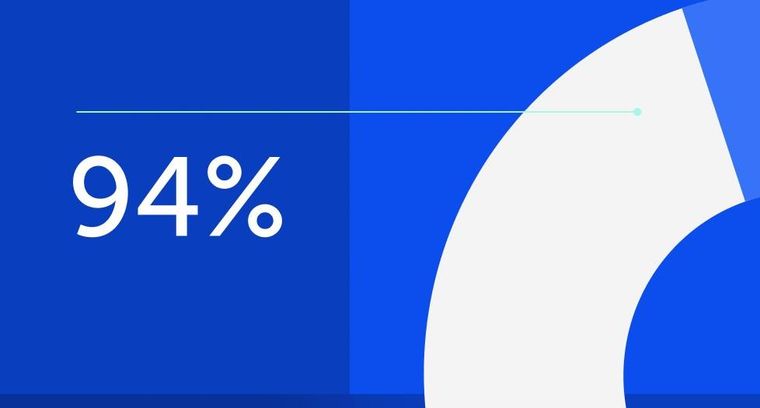
94% of researchers rate our articles as excellent or good
Learn more about the work of our research integrity team to safeguard the quality of each article we publish.
Find out more
ORIGINAL RESEARCH article
Front. Anim. Sci., 16 March 2022
Sec. Animal Physiology and Management
Volume 3 - 2022 | https://doi.org/10.3389/fanim.2022.852958
This article is part of the Research TopicAnimal Responses to Climatic Stress: Strategies for Coping with Harsh Climatic ConditionsView all 6 articles
The climate in northern latitude countries, such as Canada, are changing twice as fast as in lower latitude countries. This has resulted in an increased frequency of hot days and longer more frequent heat waves. Canadian dairy cattle are therefore at increased risk of heat stress, especially those in management systems without the infrastructure to properly cool animals. Cattle experiencing heat stress undergo numerous physiological changes. Previous research has shown dairy cattle classified as high immune responders have lower incidence of disease. Therefore, the objective of this study was to evaluate the variation in respiration rate, rectal temperature, and rumination activity in immune phenotyped dairy cattle during a natural heat stress challenge. Additionally, the relationship between physiological response and temperature humidity index was compared between free-stall and tie-stall management systems. A total of 27 immune phenotyped (nine high, nine average and nine low) lactating dairy cattle were housed in a free-stall during the summer months for a duration of 27 days. Concurrently, two groups of six (three high and three low) immune phenotyped lactating dairy cattle were housed in a tie-stall for a duration of 12 days. Rumination was measured for the duration of the study for all cattle using SCR Heatime rumination collars. Respiration was measured using EMKA respiration bands for cattle housed in the tie-stalls, and manually [once in the morning (a.m.) and once in the afternoon (p.m.)] for cattle in free-stall management. Rectal temperature was measured using a digital thermometer twice daily (a.m. and p.m.) in both free-stall and tie-stall management systems. The temperature humidity index was recorded every 15 min in both management systems for the duration of the study. The results showed that high responders had significantly lower respiration rates compared to low responders when the temperature humidity index was high in both free-stall and tie-stall management systems, but there was no difference in rectal temperature, or rumination activity between phenotypes. Temperature humidity index values in the free-stall were significantly lower than the tie-stall. These findings increase the evidence that high immune responders are more likely to be tolerant to heat stress than low immune responders.
The Intergovernmental Panel on Climate Change (IPCC) predicts that surface temperatures around the world will continue to increase until at least 2050, and this is under multiple different emissions scenarios, including reducing greenhouse gas emissions to below current levels (Masson-Delmotte et al., 2021). The rise in the earth's surface temperature has led to increasingly frequent and extreme changes in weather patterns. Recently, one of the major issues highlighted by an IPCC report was that hot temperature extremes, including heatwaves, are more frequent as well as more intense across the majority of land masses globally than they have been in the past (Masson-Delmotte et al., 2021). Additionally, areas of higher latitude, like Canada, are experiencing greater warming compared to mid or lower latitude regions [IPCC (Intergovermental Panel on Climate Change), 2014]. This poses an issue for Canadian livestock, and dairy cattle in particular, who generate greater amounts of metabolic heat compared to most other livestock species, making adaptation to increasingly hotter temperatures difficult (Kadzere et al., 2002; Carabaño et al., 2017). Consequently, dairy cattle are more prone to heat stress than other livestock species.
Heat stress can negatively affect many aspects of productivity in dairy cattle, including reduced milk production (Sánchez et al., 2009) and reduced concentration of milk components, namely protein and fat (Lambertz et al., 2014; Nasr and El-Tarabany, 2017). Furthermore, heat stress during late gestation can lead to reduced milk production for the entire subsequent lactation (Dahl et al., 2016). Similarly, heat stress has been shown to affect the reproductive capability of dairy cattle by reducing the ability of herd owners to detect active estrus (De Rensis et al., 2015; Schüller et al., 2017). Additionally, heat stress can damage the oocyte itself (Schüller et al., 2014; El-Tarabany and El-Bayoumi, 2015), which can negatively impact the survival of an embryo and lead to increased occurrence of abortion (De Rensis and Scaramuzzi, 2003; De Rensis et al., 2015). Disease incidence can also increase as a result of heat stress because the warmer environment in the barn is ideal for pathogen growth (Bett et al., 2017) and because of the deleterious effects on the immune system (Bagath et al., 2019).
Dairy cattle respond to increases in temperature and humidity with physiological mechanisms that dissipate heat from the body. Initially when dairy cattle start to experience heat stress, evaporative cooling mechanisms are engaged to remove heat from the body (Collier et al., 2019). These mechanisms include increasing respiration rate and sweating (Garner et al., 2016; Al-Qaisi et al., 2019; Collier et al., 2019), which produces moisture that is then evaporated into the environment resulting in a cooling effect (Berman, 2006). However, if the temperature and humidity reach a point beyond which evaporative cooling is no longer effective, an increase in core body temperature, hyperthermia, will occur (Garner et al., 2016; Collier et al., 2019). During hyperthermia other mechanisms to reduce heat load are engaged, such as the reduction of dry matter intake (Collier et al., 2019). This, in turn leads to reduced milk production and rumination activity (Soriani et al., 2013; Gilson et al., 2020), which in turn increases the risk of metabolic disorders (Soriani et al., 2013). Therefore, faced with a constantly changing and warming climate, the identification of dairy cattle naturally tolerant to increased temperature and humidity, and thereby at a reduced risk for heat stress, is essential.
Exposure to extreme high temperature and humidity, which are risk factors for heat stress, may not solely be a function of the environment, but the housing management system could also be a contributing factor. To the best of our knowledge, no studies to date have been conducted that compared how different management systems influence the exposure to heat stress. Currently, 73% of Canadian dairy cattle are housed in tie stall management systems while only 27% of Canadian dairy farms utilizing free stall management systems (Government of Canada, 2021). Historically, the structure and design of tie-stall barns are characteristically poor in terms of distribution of air flow and ventilation. Therefore, cattle in tie-stalls may be at greater risk of heat stress than animals housed in free-stall systems which was the hypothesis examined here.
Previously studies have shown that dairy cattle identified as high antibody and high cell-mediated immune responders demonstrate health-related benefits, including an overall reduction in the incidence of disease (Thompson-Crispi et al., 2012; Larmer and Mallard, 2017) and improved response to vaccination (Wagter et al., 2000). The advantages of high immune response genetics suggest that selectively breeding for high antibody and cell-mediated immune responders can also lead to an improvement in hoof health (Cartwright et al., 2017). More recently it has been shown that blood mononuclear cells (BMC) isolated from high immune responder dairy cattle produce greater concentrations of molecules that aid in thermotolerance and display an increased capability to proliferate after exposure to an in-vitro heat stress challenge, compared to BMC from average and low responders (Cartwright et al., 2021). Additionally, a recent study revealed DNA methylation patterns that suggested an increased expression of genes involved in cellular protection in high immune responding Holsteins compared to low immune responders, after an in-vitro heat stress challenge (Livernois et al., 2021).
Therefore, the objective of this study was to evaluate the physiological response to heat stress in immune phenotyped Canadian dairy cattle housed in either free-stall or tie-stall management systems. The hypothesis for this study is that the temperature humidity index (THI) will be higher in a tie-stall vs. free-stall management system and that dairy cattle classified as high immune responders will have lower respiration rates, rectal temperatures, and increased rumination activity in both free-stall and tie-stall management systems at high THI values.
All procedures performed were approved by the Animal Care Committee at the University of Guelph (Animal Utilization Protocol 4449).
All cattle were housed at the University of Guelph Elora Dairy Research Station. For the purposes of this study a total of 27 lactating cattle that were housed in the free-stall area of the facility were used and 12 lactating cattle were housed in the tie-stall area of the facility. Cattle housed in the tie-stall area were placed at the back of the barn away from the door. All cattle in the tie-stall area were housed on the same side of the barn in stalls next to one another. Cattle were randomly assigned to a stall and an even distribution between phenotypes was maintained. Cattle remained in the same stall for the duration of the study. These numbers were chosen based on availability of equipment and sample size calculation (sample size calculated using one-way ANOVA in G*power 3.1.9.7 (Faul et al., 2007), α = 0.05, power = 0.95, obtained standard deviation and means from data evaluated in Cartwright et al. (2022) The free-stall area was similar to a modern free-stall barn, where curtains were fully open on both sides of the barn and large fans are mounted above to facilitate maximum airflow. Cattle housed in the free-stall area were milked twice daily via a rotary parlor. The tie-stall area was similar to traditional tie-stall barns which contained ventilation fans (as the only source of outside air) and cooling fans mounted on the ceiling which served as the main source of air flow. There was no curtain in the tie-stall to facilitate additional flow of air from the outside as the tie-stall area is contained within the main barn. Therefore, outside air did not circulate in the tie-stall area. Cows in the tie-stall were milked twice daily using individual pipeline milkers. Cattle evaluated in this study were from various parities (parities ranged from 1 to 5), production yields (average daily milk production ranged from 31 to 119 L/day) and days in milk (days in milk ranged from 61 to 302).
All cattle in the study were previously evaluated for immune response using a patented test protocol (Wagter et al., 2000; Hernandez et al., 2005; Wagter and Mallard, 2007). Briefly, blood samples were obtained via the tail vein on test day 0 and an intramuscular injection of type-1 and type-2 antigens were given. On test day 14 another blood sample was obtained via the tail vein. Serum was collected from blood samples obtained on days 0 and 14 and used to assess antibody-mediated immune response by enzyme linked immunosorbent assay. Additionally, on day 14, a delayed type hypersensitivity test was initiated. Triplicate measurements of each side of the tail-fold were made using calipers followed by intra-dermal injections of control and type-1 antigens on either side of the tail-fold. On day 15 additional skin fold measurements were taken to assess delayed type hypersensitivity, an indicator of cell-mediated immune response. Cattle were classified based on their estimated breeding value as high, average or low for both antibody and cell mediated-immune response (Cartwright et al., 2021).
Of the 27 dairy cattle housed in the free-stall, nine were high for antibody and cell-mediated immune response, nine were average for antibody and cell-mediated immune response and nine were low for antibody and cell-mediated immune response. Of the 12 cattle that were housed in the tie-stall, six were high for antibody and cell-mediated immune response and six were low for antibody and cell-mediated immune response. This study took place during the summer (July 30 to August 25, 2020). Data on production parameters for each animal was obtained from DairyComp 305 management software.
The respiration rate of cattle housed in the free-stall area was assessed over a 27-day period from July 30, 2020 to August 25, 2020, which is a similar timeframe to that reported in other studies evaluating respiration rate and rectal temperature in Holstein cattle (Srikandakumar and Johnson, 2004; Shwartz et al., 2009). Respiration rates were recorded twice per day (at 9 a.m. and 2 p.m.) by manually counting the number of breaths taken in a 15 s period and multiplying by 4 to obtain the number of breaths per minute. Cattle housed in the tie-stall area were assessed for respiration rate in 2 groups over a 12-day period, which is the maximum length of time the respiration bands could be worn and is also in line with other studies in dairy cattle (Huhnke and Monty, 1976; Trout et al., 1998). Each group contained 3 high and 3 low immune responders. The respiration rate of cattle in the tie-stall area were obtained every 15 min using an emkaPACK4G respiration rate band (EMKA Technologies, Sterling, VA, USA). These measurements were verified with manual respiration rate measurements taken twice daily at 9 a.m. and 2 p.m. The emkaPACK4G respiration rate bands were also tested with cattle housed in the free-stall area, but the band did not remain secure on the cattle for more than 24 h and the connection between the bands and the receiver was lost every time the cows were milked. For this reason, manual respiration rates were taken on cattle housed in the free-stall instead.
Rectal temperatures of cattle housed in both the free-stall and tie-stall areas were measured manually using a digital thermometer (Life Brand, Alberta, CA) twice daily at 9 a.m. and 2 p.m. for the duration of the 27-day study and the 12-day study as described above.
All cattle housed in both the free-stall and tie-stall were equipped with SCR Heatime rumination collars (Micro Technologies, Amarillo TX, USA) for the duration of the study. The collars measured rumination activity every 2 h. Rumination activity data was extracted from the SCR Heatime system and analyzed for associations with THI and difference between immune phenotypes.
Both the free-stall and tie-stall areas of the barn were equipped with sensors that measured temperature and humidity at 15-min interval throughout the day. Temperature and humidity were used to calculate THI using the following equation:
Where temp is temperature in degrees C and humidity is a percentage (Zimbelman et al., 2009). Temperature humidity index was calculated for corresponding time when respiration rate, rectal temperature, and rumination activity data were recorded.
Production parameter data were checked for normality using the Shapiro-Wilks test in R 3.6 (R Core Team, 2019). Data not normally distributed was log transformed and re-checked for normality. Variation in production parameters between immune response phenotyped cattle housed in the two management systems was assessed using general linear models in R 3.6 (R Core Team, 2019) using the following models:
where yij = average daily milk production, μ = overall mean, ii = effect immune response phenotype housed in the tie-stall or free-stall (high in tie-stall, low in tie-stall, high in free-stall, average in free-stall, low in free-stall), pj = effect of parity, dk = effect of days in milk and eijk = residual error.
where yij = parity, μ = overall mean, ii = effect immune response phenotype housed in the tie-stall or free-stall (high in tie-stall, low in tie-stall, high in free-stall, average in free-stall, low in free-stall), lj = effect of average daily milk production, dk = effect of days in milk and eijk = residual error.
where yij = day in milk, μ = overall mean, ii = effect immune response phenotype housed in the tie-stall or free-stall (high in tie-stall, low in tie-stall, high in free-stall, average in free-stall, low in free-stall), lj = effect of average daily milk production, pk = effect of parity and eijk = residual error.
To assess the variation in THI between free-stall and tie-stall areas a repeated measures model with an autoregressive co-variance structure was used in R 3.6 (R Core Team, 2019) using the following model:
where yij = THI in free-stall and tie-stall, μ = overall mean, li = effect of location (free-stall or tie-stall), dj = effect of time of day (either a.m. or p.m., which are defined the same as mentioned above for differences in physiological measurements or night which is defined as 12 p.m. to 5 a.m.) and eij = residual error.
Physiological data were checked for normality using the Shapiro-Wilks test in R 3.6 (R Core Team, 2019). If data was not normally distributed it was log transformed and re-checked for normality. Variation in respiration rate, rectal temperature, and rumination activity between immune response phenotypes for were assessed separately for free-stall and tie-stall areas. To assess the variation in respiration rate, rectal temperature and rumination activity between phenotypes, for each area of the facility, repeated measures models with an autoregressive co-variance structure were used in R 3.6 (R Core Team, 2019) with the following model:
where yijkl = either respiration rate, rectal temperature or rumination activity in either free-stall or tie-stall area, μ = overall mean, ii = effect of immune response phenotype (high, average, or low for free-stall or high, low for tie-stall), tj = effect of THI at each time point, pk = effect of parity (1, 2, 3 and ≥4), ml = average daily production throughout study period and eijkl = residual error.
To assess variation in respiration rate, rectal temperature and rumination activity between free-stall and tie-stall areas of the research facility repeated measures models with an autoregressive co-variance structure were used in R 3.6 (R Core Team, 2019) using the following model:
where yijklmn = either respiration rate, rectal temperature or rumination activity, μ = overall mean, ii = effect of immune response phenotype (high, average or low), tj = effect of THI at each time point, pk = effect of parity (1, 2, 3 and ≥4), ml = average daily production throughout study period, lm = effect of location (either free-stall or tie-stall), dn = effect of time of day: either a.m. or p.m. For rumination activity and tie-stall respiration rate, a.m. is defined as measurements from 12 a.m. to 11:59 a.m. For free-stall respiration rate and rectal temperature and tie-stall rectal temperature, a.m. is defined at measurements taken at 9 a.m. For rumination activity and tie-stall respiration rate, p.m. is defined as measurements from 12 p.m. to 11:59 p.m. For free-stall respiration rate and rectal temperature and tie-stall rectal temperature, p.m. is defined at measurements taken at 2 p.m. Finally, eijklmn = residual error. Results are presented as least squared means (LSM) of untransformed data; however, significance and trends are based on normalized data. Significant differences are defined at p ≤ 0.05 and trends are defined at p ≤ 0.10.
Data on the comparison of THI in the a.m. vs. p.m. vs. night for free-stall and tie-stall management systems can be seen in Figure 1. Differences were observed for both free-stall (p < 0.001) and tie-stall (p < 0.001) management systems when comparing a.m. vs. p.m., with elevated THI values being observed in the p.m. Differences were also observed within tie-stall and free-stall management systems when comparing a.m. vs. night (tie-stall p = 0.011, free-stall p < 0.001) and p.m. vs. night (p < 0.001), with a.m. and p.m. THI values increased relative to night THI values. Additionally, the THI was reduced in the free-stall at the a.m. (p < 0.001), p.m. (p < 0.001) and night (p < 0.001) time points compared to the tie-stall.
Figure 1. Average temperature humidity index for free-stall vs. tie-stall. Data are presented as least squared means of untransformed data, with error bars representing standard error for each mean. Significance (P < 0.001 for all comparisons except tie-stall a.m. vs. night p = 0.011) is represented by double stars above the error bars.
No difference in production parameters were observed between immune response phenotyped cattle housed in both the tie-stall and free-stall management system. The LSM for average daily production was 71.8 L/day [standard error of mean (SEM) = 7.92] for high responders in the tie-stall, 73.1 L/day (SEM = 7.71) for low responders in the tie-stall, 78.6 L/day (SEM = 5.73) for high responders in the free-stall, 74.2 (SEM = 6.47) L/day for average responders in the free-stall and 74.1 L per day (SEM = 7.43) for low responders in the free-stall. The LSM for days in milk was 205 (SEM = 38.2) for high responders in the tie-stall, 175 (SEM = 37.5) for low responders in the tie-stall, 193 (SEM = 27.9) for high responders in the free-stall, 151 (SEM = 31.2) for average responders in the free-stall and 146 (SEM = 35.8) for low responders in the free-stall. The LSM parity was 1.42 (SEM = 0.437) for high responders in the tie-stall, 1.83 (SEM = 0.432) for low responders in the tie-stall, 1.94 (SEM = 0.322) for high responders in the free-stall, 1.54 (SEM = 0.358) for average responders in the free-stall and 2.18 (SEM = 0.4) for low responders in the free-stall.
In the free-stall area differences between phenotypes for respiration rate were not observed until a THI of 75. At a THI of 75 (p = 0.027), 76 (p = 0.015) and 77 (p = 0.026), high immune responders had reduced respiration rates compared to low immune responders (Figure 2). Respiration rates measured for high immune responders ranged from 34.1 to 50.6 breaths per minute across all THI values, which is within the normal respiration rate of dairy cattle [26–50 breaths per minute (Becker et al., 2020)]. In contrast, low immune responders displayed respiration rates above normal at THI of 75 and higher, ranging from 34 to 63.8 breaths per minute. Average immune responders did not differ from high or low immune responders for respiration rate. However, average responders had respiration rates above normal ranging from 30.2 to 58.3 breaths per minute at THI of 76.
Figure 2. Respiration rate in breaths per minute vs. temperature humidity index for high, average, and low immune responding dairy cattle housed in the free-stall. Data are presented as least squared means of untransformed data, with error bars representing standard error for each mean. Double stars above the error bars indicate that a significant difference [75 (p = 0.027), 76 (p = 0.015) and 77 (p = 0.026)] was observed between low and high immune responders. The line at 50 breaths per minute represents the maximum normal respiration rate for dairy cattle.
In the tie-stall area differences in respiration rate between high and low immune responders were observed across most measured THI values [65 (p = 0.036), 67 (p = 0.006), 70 (p < 0.001), 71 (p = 0.016), 72 (p = 0.006), 73 (p < 0.001), 74 (p < 0.001), 75 (p = 0.019), 76 (p = 0.007), 77 (p = 0.001), 78 (p < 0.001), 79 (p < 0.001), 81 (p < 0.001) and 84 (p = 0.046)] and trends were observed at a THI of 64 (p = 0.06) and 66 (p = 0.056), where high responders exhibited reduced respiration rates compared to low immune responders. Respiration rate ranged from 34.4 to 55.4 breaths per minute for high responders and between 32.3 and 60 breaths per minute for low responders across all measured THI values (Figure 3). Low immune responders maintained respiration rates within normal range until THI of 77, at which point the respiration rate remained above normal for all remaining higher THI values. High immune responders maintained respiration rates within normal range until a THI of 80. High immune responders had respiration rates above normal at a THI of 80, 82 and 83, however at a THI of 81 and 84 respiration rates returned to within the normal range.
Figure 3. Respiration rate in breaths per minute vs. temperature humidity index for high and low immune responding cattle housed in the tie-stall. Data are presented as least squared means of untransformed data, with error bars representing standard error for each mean. Double stars above the error bars indicate that a significant difference [65 (p = 0.036), 67 (p = 0.006), 70 (p < 0.001), 71 (p = 0.016), 72 (p = 0.006), 73 (p < 0.001), 74 (p < 0.001), 75 (p = 0.019), 76 (p = 0.007), 77 (p = 0.001), 78 (p < 0.001), 79 (p < 0.001), 81 (p < 0.001)] was observed and a single star above the error bar represents a trend [64 (p = 0.06), 66 (p = 0.056)] was observed. The line at 50 breaths per minute represents the maximum normal respiration rate for dairy cattle.
There was no difference in the average respiration rate between the free-stall vs. the tie-stall areas. However, the average respiration rate of cattle increased in both the free-stall (p = 0.01) and tie-stall (p < 0.001) areas when comparing measurements taken in the morning vs. the afternoon (Figure 4).
Figure 4. Average respiration rate in breathes per minute for free-stall vs. tie-stall. All data is presented as least squared means of untransformed data, with error bars representing standard error for each mean. Significance [free-stall (p = 0.01), tie-stall (p < 0.001)] is represented by double stars above the error bars.
There were no differences in rectal temperature between immune response phenotypes for cattle housed in the free-stall management system. All phenotypes remained within normal range for rectal temperature [between 38.5°C and 39.3°C (Fielder, 2015)] throughout the duration of the study. High immune responders had rectal temperatures ranging from 38.5°C to 39.3°C. Average immune responders had rectal temperatures ranging from 38.5°C to 38.9°C. Low immune responders had rectal temperatures ranging from 38.5°C to 39.2°C.
Similarly, there were no differences, across THI values, in rectal temperature between high and low responders housed in the tie-stall management system. Both high and low immune responders maintained rectal temperatures within the normal range throughout the duration of the study. However, in general, high immune responders (mean rectal temperature = 38.8°C) had a tendency (p = 0.03) to have reduced rectal temperatures across THI values between 74 and 83 compared to low responders (mean rectal temperature 39°C) (Figure 5). Overall, the rectal temperatures for high responders housed in the tie-stall area ranged from 38.7°C to 39.0°C, whereas the rectal temperature for low responders ranged from 38.7°C to 39.3°C.
Figure 5. Rectal temperature in degrees Celsius vs. temperature humidity index for high and low immune responding cattle housed in the tie-stall. Data are presented as least squared means of untransformed data, with error bars representing standard error for each mean. The line at 39.3◦C represents the maximum normal rectal temperature for dairy cattle. No difference was observed between phenotypes at THI values, however there was a tendency (p = 0.03) for high immune responder to have reduced rectal temperature across THI values compared to lows.
Additionally, no difference in average rectal temperature was observed between free-stall and tie-stall areas. A trend was observed in both the free-stall (p = 0.09) and tie-stall (p = 0.10) where rectal temperature was higher in the afternoon vs. morning (Figure 6).
Figure 6. Average rectal temperature in degrees Celsius for free-stall vs. tie-stall. Data are presented as least squared means of untransformed data, with error bars representing standard error for each mean. Trends [free-stall (p = 0.09), tie-stall (p = 0.10)] are represented by single stars above the error bars.
There was no difference in rumination activity between immune response phenotypes of cattle housed in the free-stall or tie-stall areas, across all THI values. Similarly, when comparing between the free-stall and tie-stall management systems, there was no significant difference in rumination activity for all cattle, across all THI values. In both management systems rumination activity remained within the normal range throughout the duration of the study, except at THI values of 77 and 82 rumination activity was below normal for low responders in the tie-stall area. In the free-stall area, average rumination activity for high, average, and low immune responders ranged from 40.7 to 58.4 min, 37.8 to 74.9 min, and 37.3 to 63.4 min in a two-hour span, respectively. In the tie-stall area average rumination activity in a two-hour span for highs ranged from 37.4 to 71.5 min and for lows it ranged from 33.3 to 68.4 min.
One of the first indicators of heat stress in dairy cattle is an increase in respiration rate (Dairy Austrailia, 2019). Dairy cattle increase their respiration rate to dissipate body heat in order to maintain normal internal body temperature (Polsky and von Keyserlingk, 2017). Results from this study showed that in both free-stall and tie-stall management systems, Holstein cattle classified as high immune responders had reduced respiration rates at high THI values compared to cattle classified as low immune responders. Additionally, it was observed in both free-stall and tie-stall management systems that low immune responders had respiration rates above the normal expected range at a lower THI than high immune responders. Taken together, these results may indicate that cattle identified as high immune responders are more tolerant to increased temperature and humidity compared to low immune responders, and therefore, may be more resistant to heat stress. This study also showed that low immune responders had above normal respiration rates at a THI of 75 (free-stall management system) and 77 (tie-stall management system), whereas high immune responders didn't experience respiration rates above normal until a THI of 80 (in the tie-stall management system). These observations are in agreement with a similar study evaluating respiration rate between immune phenotyped dairy cattle, where low immune responders had respiration rates above normal at a THI of 77, whereas high immune responders did not exhibit elevated respiration rate until a THI of 79 (Cartwright et al., 2022). Other studies examining the effect of heat challenge on cattle consider THI values between 73 and 77 as mild heat stress, and THI values between 78 and 89 as moderate heat stress (Mishra, 2021). Consequently, the results of this study indicate low immune responders are more susceptible to mild heat stress than high responders. Further, high responders did not show signs of heat stress until THI reached the moderate heat stress range. In this study, since high responders did not show signs of heat stress until moderate THI values were reached, it is possible they are more tolerant to heat stress compared to low immune responders.
The mechanisms that lead to lower respiration rate in high immune responders vs. average and low immune responders have yet to be evaluated and future studies in this area are warranted. However, several studies evaluating the effects of heat stress on immune response have been conducted that may provide an indication as to why high immune responders have reduced respiration rate during a heat challenge. Studies have shown that heat stress typically results in a shift toward a type-2 immune response (associated with antibody-mediated immune response) and a down regulation of type-1 responses (associated with cell-mediated immune response) (Salak-Johnson and McGlone, 2007; Bagath et al., 2019). Additionally, studies have shown heat stress reduces lymphocyte proliferation (Bhanuprakash et al., 2016) and can impair cell functions (Kansas, 1996; Evans et al., 2016). A previous study evaluating blood mononuclear cell (BMC) function of immune phenotyped dairy cattle during an in-vitro heat challenge showed that BMCs from high immune responders displayed increased ability to proliferate compared to BMCs under thermoneutral conditions. Blood mononuclear cells from low immune responders displayed reduced ability to proliferate when heat challenged compared to cells in thermoneutral conditions (Cartwright et al., 2021). Cartwright et al. (2021) also showed that BMCs from high immune responders displayed greater cell proliferation compared to average and low immune responders. The impairment of cell-mediated responses during heat stress is typically associated with cortisol production, since cortisol can bind to DNA and inhibit the expression of genes involved in activation of type-1 responses (Bagath et al., 2019). Therefore, as cortisol levels are associated with impaired cellular responses, it is possible that high immune responders may produce less cortisol during heat stress compared to average and low responders resulting in an increased tolerance to stress and the ability to maintain a normal respiration rate. Moreover, studies in sheep have revealed an increased expression of heat shock proteins during heat stress is associated with reduced respiration rate (Abdelnour et al., 2019). Cartwright et al. (2021) observed an increased concentration of heat shock protein 70 from BMC after in-vitro heat challenge in high immune responder dairy cattle compared to low immune responders. Therefore, increased concentration of heat shock protein 70 may be an additional mechanism that contributes to the reduced respiration rate observed during heat stress for high immune responders.
Evaporative cooling mechanisms, like increased respiration rate, are an effective way for dairy cattle to dissipate heat from their body. However, if ambient temperatures increase beyond the capacity of evaporative cooling mechanisms, body temperature will begin to rise (Mishra, 2021). Studies have shown varied results for the effect of heat stress on rectal temperature of dairy cattle. Some studies have shown that the rectal temperature of dairy cattle increases beyond normal thresholds when experiencing heat stress (Spiers et al., 2004; Wheelock et al., 2010; Chen et al., 2018), whereas others demonstrated elevated rectal temperatures within the normal range during heat stress (Guzeloglu et al., 2001; Koubkova et al., 2002; Park et al., 2019). The results from this study revealed no difference in rectal temperature between immune response phenotypes at all THI values. However, in the tie-stall management system, where THI values were higher, dairy cattle classified as low immune responders tended to have elevated rectal temperatures at a THI of 76 and above. This corresponds to another study evaluating rectal temperature between immune phenotyped dairy cattle where rectal temperature increased at a THI of 76 (Cartwright et al., 2022). As expected, dairy cattle classified as high immune responders and housed in the tie-stall management system tended to have reduced rectal temperatures at higher THI values compared to low immune responders housed in the tie-stall management. These results on rectal temperature agree with studies showing elevated rectal temperatures during heat stress but remaining within normal ranges (Guzeloglu et al., 2001; Koubkova et al., 2002; Park et al., 2019). The THI values observed in the experimental period for the tie-stall and free-stall management systems indicate a decrease in THI overnight (Figure 1). Therefore, overnight cooling may have prevented cattle from experiencing severe heat stress maintaining rectal temperature within normal range.
Previous studies have shown that dairy cattle reduce dry matter intake to reduce metabolic heat load when body temperature increase beyond the normal range (Collier et al., 2019; Lees et al., 2019). Decreased dry matter intake leads to a reduction in rumination activity and milk production and also increases the risk of metabolic disorders (Soriani et al., 2013; Tao et al., 2018; Gilson et al., 2020). In this study there was no difference in rumination activity between high and low responders housed in both the free-stall and tie-stall management systems. Indeed, rumination activity remained within the normal range for all animals for the duration of the study. Since there was no elevation in body temperature beyond normal physiological range in this study for both phenotypes, this may contribute to rumination activity remaining within normal ranges Additionally, the THI values in the free-stall management system did not increase above the mild heat stress range (Mishra, 2021) and therefore its likely that conditions were not extreme enough to increase body temperatures to a level that would negatively affect dry matter intake and reduce rumination activity. Indeed, a previous study demonstrated that rumination activity in lactating Holsteins remained within normal ranges until the THI was above 82, at which point it decreased (Park et al., 2019). Similarly, in a study evaluating the effects of a controlled heat challenge on physiological parameters and function of blood mononuclear cells, that was also conducted in the same tie-stall area as this study, the THI only reached a maximum of 81 (Cartwright et al., 2022). Based on these findings from Park et al. (2019) and Cartwright et al. (2022), it is clear that the THI did not reach a high enough value in the tie-stall management system in this study to observe a decrease in rumination activity.
The THI was different between a.m. and p.m., as well as between a.m. and p.m. vs. night within each management system. Additionally, the THI in the tie-stall management system was elevated compared to that of the free-stall management system in the a.m., p.m. and night. To date there are no studies comparing THI values between free-stall and tie-stall management systems. Tie-stall barns typically do not allow for even distribution of air flow compared to free-stall barns. Therefore, it is more difficult to manage increased temperatures and excessive humidity in tie-stall barns compared to most free-stall barns. It follows that in this study the tie-stall management system experienced elevated THI values overall compared to the free-stall management system. Although both respiration rate and rectal temperature increased from a.m. to p.m. within each management system, there was no difference in a.m. and p.m. values across management systems. Additionally, there was no difference in rumination activity between a.m. and p.m. within each management system or between management systems. Other studies have presented similar results for the physiological response to heat challenge in the a.m. vs. p.m., where an increase in respiration rate and rectal temperature was also observed in the p.m. (Honig et al., 2012; Arias et al., 2018; Kaufman et al., 2018). Finally, these results suggest that differences observed in physiological measurements, respiration rate in particular, are likely controlled by immune response phenotype rather than management system.
In conclusion, this study shows that Holstein dairy cattle classified as high immune responders displayed reduced respiration rate compared to cattle classified as low immune responders in both free-stall and tie-stall management systems at elevated THI values, indicating that high immune responders may be more tolerant to increases in temperature and humidity in multiple types of management systems. Although no difference in rectal temperature was observed between phenotypes at THI values there was a tendency for high immune responders, housed in the tie-stall, to have reduced rectal temperatures across THI values compared to low immune responders housed in the tie-stall management system. Additionally, although THI was elevated in the tie-stall management system vs. the free-stall management system, differences in respiration rate and rectal temperature were observed only within management systems between a.m. vs. p.m. measurements. This may indicate that immune response phenotype has a greater impact on differences in physiological response to heat stress than the management system itself. Results of this study provide some evidence that high immune responders may be more tolerant to heat stress. Future studies involving more severe heat stress may better elucidate the differences in the physiological and production parameters evaluated here between heat-stressed immune phenotyped dairy cattle.
The raw data supporting the conclusions of this article will be made available by the authors, without undue reservation.
The animal study was reviewed and approved by University of Guelph Animal Care Committee (AUP #4449).
SC obtained manual respiration rate, rectal temperature measurements as well as equipped all cows with telemetry equipment, obtained, organized, prepared all data for analysis, completed all statistical analysis, and prepared the initial manuscript. JS assisted with installation of telemetry equipment, provided both intellectual and technical support, and edited and reviewed the manuscript. AL assisted with installation of telemetry equipment and edited and reviewed the manuscript. BM oversaw entire study, provided intellectual support in all aspects of the research, and also helped with manuscript preparation. All authors have read and approved the final manuscript.
Animal use was supported by the Ontario Ministry of Agriculture and Rural Affairs Tier II grant. Collection and analysis of manual data measurements was supported by the Canadian First Research Excellence Fund (302-021000-499122-000000). Purchase of telemetry equipment was supported by the Canada Foundation for Innovation equipment grant. Dairy Farmers of Ontario, the Ontario Veterinary College and Food from thought provided support for the corresponding author to perform analysis of data and to write the manuscript.
The authors declare that the research was conducted in the absence of any commercial or financial relationships that could be construed as a potential conflict of interest.
All claims expressed in this article are solely those of the authors and do not necessarily represent those of their affiliated organizations, or those of the publisher, the editors and the reviewers. Any product that may be evaluated in this article, or claim that may be made by its manufacturer, is not guaranteed or endorsed by the publisher.
The authors would like to thank Laura Wright and the staff at the Elora Research Station for providing care for the animals throughout the study. The authors would also like to thank Matthew Vermy for his assistance in collection of respiration rate and rectal temperature data. The authors would like to acknowledge the staff at EMKA Technologies for their assistance in set-up an extraction of telemetry equipment data. Finally, the authors would like to thank Kaitlyn Dancy and Dr. Trevor Devries for providing access to the rumination collars and HeatPro system.
Abdelnour, S. A., Abd El-Hack, M. E., Khafaga, A. F., Arif, M., Taha, A. E., and Noreldin, A. E. (2019). Stress biomarkers and proteomics alteration to thermal stress in ruminants: a review. J. Therm. Biol. 79, 120–134. doi: 10.1016/j.jtherbio.2018.12.013
Al-Qaisi, M., Horst, E. A., Kvidera, S. K., Mayorga, E. J., Timms, L. L., and Baumgard, L. H. (2019). Technical note: developing a heat stress model in dairy cows using an electric heat blanket. J. Dairy Sci. 102, 684–689. doi: 10.3168/jds.2018-15128
Arias, R. A., Herrera, C., Larrain, R., Gonzalez, F., Mader, T. L., and Velasquez, A. (2018). Physiological and behavioural response of two dairy cows' genotypes during summertime in the central region of Chile. Austral J. Vet. Sci. 50, 9-14. doi: 10.4067/S0719-81322018000100103
Bagath, M., Krishnan, G., Devaraj, C., Rashamol, V. P., Pragna, P., Lees, A. M., et al. (2019). The impact of heat stress on the immune system in dairy cattle: a review. Res. Vet. Sci. 126, 94–102. doi: 10.1016/j.rvsc.2019.08.011
Becker, C. A., Woolums, A. R., and Stone, A. E. (2020). Recognizing Heat Stress in Dairy Cattle: How to Visually Record Respiration Rate. Available at: http://extension.msstate.edu/publications/recognizing-heat-stress-dairy-cattle-how-visually-record-respiration-rate
Berman, A (2006). Extending the potential of evaporative cooling for heatstress relief. J. Dairy Sci. 89, 3817–3825. doi: 10.3168/jds.S0022-0302(06)72423-7
Bett, B., Kiunga, P., Gachohi, J., Sindato, C., Mbotha, D., Robinson, T., et al. (2017). Effects of climate change on the occurrence and distribution of livestock diseases. Prev. Vet. Med. 137, 119–129. doi: 10.1016/j.prevetmed.2016.11.019
Bhanuprakash, V., Singh, U., Sengar, G., Sajjanar, B., Bhusan, B., Raja, T. V., et al. (2016). Differential effect of thermal stress on HSP70 expression, nitric oxide production and cell proliferation among native and crossbred dairy cattle. J. Therm. Biol. 59, 18–25. doi: 10.1016/j.jtherbio.2016.04.012
Carabaño, M. J., Ramón, M., Díaz, C., Molina, A., Pérez-Guzmán, M. D., and Serradilla, J. M. (2017). Breeding and genetics symposium: breeding for resilience to heat stress effects in dairy ruminants. A comprehensive review. J. Anim. Sci. 95, 1813. doi: 10.2527/jas2016.1114
Cartwright, S. L., Malchiodi, F., Thompson-Crispi, K., Miglior, F., and Mallard, B. A. (2017). Short communication: prevalence of digital dermatitis in Canadian dairy cattle classified as high, average, or low antibody- and cell-mediated immune responders. J. Dairy Sci. 100, 8409–8413. doi: 10.3168/jds.2016-12157
Cartwright, S. L., McKechnie, M., Schmied, J., Livernois, A. M., and Mallard, B. A. (2021). Effect of in-vitro heat stress challenge on the function blood mononuclear cells from dairy cattle ranked as high, average and low immune responders. BMC Vet. Res. 17:233. doi: 10.1186/s12917-021-02940-8
Cartwright, S. L., Schmied, J., Livernois, A., and Mallard, B. A. (2022). Effect of in-vivo heat stress on physiological parameters and function of blood mononuclear cells in immune phenotyped dairy cattle. Vet. Immunol. Immunpathol. (Under Review).
Chen, S., Wang, J., Peng, D., Li, G., Chen, J., and Gu, X. (2018). Exposure to heat-stress environment affects the physiology, circulation levels of cytokines, and microbiome in dairy cows. Sci. Rep. 8, 14606. doi: 10.1038/s41598-018-32886-1
Collier, R. J., Baumgard, L. H., Zimbelman, R. B., and Xiao, Y. (2019). Heat stress: physiology of acclimation and adaptation. Anim. Front. 9, 12–19. doi: 10.1093/af/vfy031
Dahl, G. E., Tao, S., and Monteiro, A. P. A. (2016). Effects of late-gestation heat stress on immunity and performance of calves. J. Dairy Sci. 99, 3193–3198. doi: 10.3168/jds.2015-9990
Dairy Austrailia (2019). Heat Stress in Cows. Czech J. Anim. Sci. Available online at: https://www.dairyaustralia.com.au/animal-management-and-milk-quality/animal-health/heat-stress#.YLjw-qjYrIU (accessed October 15, 2021).
De Rensis, F., Garcia-Ispierto, I., and López-Gatius, F. (2015). Seasonal heat stress: clinical implications and hormone treatments for the fertility of dairy cows. Theriogenology 84, 659–666. doi: 10.1016/j.theriogenology.2015.04.021
De Rensis, F., and Scaramuzzi, R. J. (2003). Heat stress and seasonal effects on reproduction in the dairy cow: a review. Theriogenology 60, 1139–1151. doi: 10.1016/S0093-691X(03)00126-2
El-Tarabany, M. S., and El-Bayoumi, K. M. (2015). Reproductive Performance of backcross Holstein x Brown Swiss and their Holstein contemporaries under subtropical environmental conditions. Theriogenology 83, 444–448. doi: 10.1016/j.theriogenology.2014.10.010
Evans, S. S., Repasky, E. A., and Fisher, D. T. (2016). System feels the heat. Nat. Rev. Immunol. 15, 335–349. doi: 10.1038/nri3843
Faul, F., Erdfelder, E., Lang, A.-G., and Buchner, A. (2007). G*Power 3: a flexible statistical power analysis program for the social, behavioral and biomedical sciences. Behav. Res. Methods 39, 175–191. doi: 10.3758/BF03193146
Fielder, S. E (2015). Normal Rectal Temperature Ranges. Merck Veteerinary Manual. Available online at: https://www.merckvetmanual.com/special-subjects/reference-guides/normal-rectal-temperature-ranges (accessed December 6, 2021).
Garner, J. B., Douglas, M. L., Williams, S. R. O., Wales, W. J., Marett, L. C., Nguyen, T. T. T., et al. (2016). Genomic selection improves heat tolerance in dairy cattle. Sci. Rep. 6, 34114. doi: 10.1038/srep34114
Gilson, G. M., Siqueira, L. G, Vasconcelos, C. O. d., Tomich, T. R., Camargo, L. S., et al. (2020). Effects of heat stress on rumination activity in Holstein-Gyr dry cows. Livest. Sci. 239, 104092. doi: 10.1016/j.livsci.2020.104092
Government of Canada (2021). Dairy Barns by Type in Canada 2020. Available online at: https://agriculture.canada.ca/en/canadas-agriculture-sectors/animal-industry/canadian-dairy-information-centre/dairy-statistics-and-market-information/farm-statistics/dairy-barns-type (accessed September 27, 2021).
Guzeloglu, A., Ambrose, J. D., Kassa, T., Diaz, T., Thatcher, M. J., and Thatcher, W. W. (2001). Long term follicular dynamics and biochemical characteristics of dominant follicles in dairy cows subjected to acute heat stress. Anim. Reprod. Sci. 66, 15–34. doi: 10.1016/S0378-4320(01)00082-3
Hernandez, A., Yager, J. A., Wilkie, B. N., Leslie, K. E., and Mallard, B. A. (2005). Evaluation of bovine cutaneous delayed-type hypersensitivity (DTH) to various test antigens and a mitogen using several adjuvants. Vet. Immunol Immunpathol 104, 45–58. doi: 10.1016/j.vetimm.2004.10.006
Honig, H., Miron, J., Lehere, H., Jackoby, S., Zachut, M., Zinou, A., et al. (2012). Performance and welfare of high-yeidling dairy cows subjected to 5 or 8 cooling sessions daily under hot and humid climate. J. Dairy Sci. 95, 3736–3742. doi: 10.3168/jds.2011-5054
Huhnke, M. R., and Monty, D. E. Jr. (1976). Physiologic responses of preparturient and postparturient Holstein-Friesian cow to summer heat stress in Arizona. Am. J. Vet. Res. 37, 1301–1304.
IPCC (Intergovermental Panel on Climate Change) (2014). Climate Change 2014: Mitigation of Climate Change. Available online at: https://www.ipcc.ch/report/ar5/wg3/ (accessed accessed October 01, 2021).
Kadzere, C. T., Murphy, M. R., Silanikove, N., and Maltz, E. (2002). Heat stress in lactating diary cows: a review. J. Anim. Sci. 77, 59–91. doi: 10.1016/S0301-6226(01)00330-X
Kansas, G. S (1996). Selectins and their ligands: current concepts and controversies. Blood 88, 3259–3287. doi: 10.1182/blood.V88.9.3259.bloodjournal8893259
Kaufman, J. D., Saxton, A. M., and Rius, A. G. (2018). Short communication: relationships among temperature-humidity index with rectal, udder surface and vaginal temperatures in lactating dairy cows experiencing heat stress. J. Dairy Sci. 101, 6424–6429. doi: 10.3168/jds.2017-13799
Koubkova, M., Kuizkova, I., Kunc, P., Hartlova, H., Flusser, J., and Dolezal, O. (2002). Influence of high environmental temperature and evaporative cooling on some physiological, haematological and biochemical parameters in high yielding dairy cows. J. Anim. Sci. 47, 309–318.
Lambertz, C., Sanker, C., and Gauly, M. (2014). Climatic effects on milk production traits and somatic cell score in lactating Holstein-Friesian cows in different housing systems. J. Dairy Sci. 97, 319–329. doi: 10.3168/jds.2013-7217
Larmer, S. G., and Mallard, B. A. (2017). High immune response sires reduce disease incidence in North American large commercial dairy populatons. Cattle Pract. 25, 74-81.
Lees, A. M., Sejian, V., Wallage, A. L., Steel, C. C., Mader, T. L., Lees, J. C., et al. (2019). The impact of heat load on cattle. Animals 9, 1–20. doi: 10.3390/ani9060322
Livernois, A. M., Mallard, B. A., Cartwright, S. L., and Canovas, A. (2021). Heat stress and immune response phenotype affect DNA methylation in blood mononuclear cells from Holstein dairy cows. Sci. Rep. 11, 1–12. doi: 10.1038/s41598-021-89951-5
Masson-Delmotte, V., Zhai, P., Pirani, A., Connors, S. L., Pean, C., Berger, S., et al (2021). IPCC 2021: Summary for Policymakers. Climate Change 2021: The Physical Science Basis. Contribution of Working Group 1 to the Sixth Assessment Report of the Intergovernmental Panel on Climate Change. Available online at: https://www.ipcc.ch/report/ar6/wg1/#SPM (accessed October 01, 2021).
Mishra, S. R (2021). Behavioural, physiological, neuro-endocrine and molecular responses of cattle against heat stress: an updated review. Trop. Anim. Health Prod. 53, 400. doi: 10.1007/s11250-021-02790-4
Nasr, M. A. F., and El-Tarabany, M. S. (2017). Impact of three THI levels on somatic cell count, milk yield and composition of multiparous Holstein cows in a subtropical region. J. Therm. Biol. 64, 73–77. doi: 10.1016/j.jtherbio.2017.01.004
Park, J. H., Choi, H. C., Lee, H. J., Kim, E. T., Son, J. K., and Kim, D. H. (2019). A study on the effect of temperature humidity index on the respiration rate, rectal temperature and rumination time of lactating holstein cows in summer season. J. Korea Acad. Ind. Coop. Soc. 20, 136–143. doi: 10.5762/KAIS.2019.20.11.136
Polsky, L., and von Keyserlingk, M. A. G. (2017). Effects of heat stress on dairy cattle welfare. J. Dairy Sci. 100, 8645–8657. doi: 10.3168/jds.2017-12651
R Core Team (2019). R: A Language and Environment for Statistical Computing. Vienna: R Foundation for Statistical Compututing. Available at: https://www.r-project.org/ (accessed September 15, 2021).
Salak-Johnson, J. L., and McGlone, J. J. (2007). Making sense of apparently conflicting data: stress and immunity in swine and cattle. J. Anim. Sci. 85, 81–88. doi: 10.2527/jas.2006-538
Sánchez, J. P., Misztal, I., Aguilar, I., Zumbach, B., and Rekaya, R. (2009). Genetic determination of the onset of heat stress on daily milk production in the US Holstein cattle. J. Dairy Sci. 92, 4035–4045. doi: 10.3168/jds.2008-1626
Schüller, L. K., Burfeind, O., and Heuwieser, W. (2014). Impact of heat stress on conception rate of dairy cows in the moderate climate considering different temperature-humidity index thresholds, periods relative to breeding, and heat load indices. Theriogenology 81, 1050–1057. doi: 10.1016/j.theriogenology.2014.01.029
Schüller, L. K., Michaelis, I., and Heuwieser, W. (2017). Impact of heat stress on estrus expression and follicle size in estrus under field conditions in dairy cows. Theriogenology 102, 48–53. doi: 10.1016/j.theriogenology.2017.07.004
Shwartz, G., Rhoads, M. L., VanBaale, M. J., Rhoads, R. P., and Baumgard, L. H. (2009). Effects of a supplemental yeast culture on heat-stressed lactating Holstein cows. J. Dairy Sci. 92, 935–942. doi: 10.3168/jds.2008-1496
Soriani, N., Panella, G., and Calamari, L. (2013). Rumination time during the summer season and its relationship with metabolic conditions and milk production. J. Dairy Sci. 96, 5082–5094. doi: 10.3168/jds.2013-6620
Spiers, D. E., Spain, J. N., Sampson, J. D., and Rhoads, R. P. (2004). Use of physiological parameters to predict milk yield and feed intake in heat stressed dairy cows. J. Therm. Biol. 29, 759–764. doi: 10.1016/j.jtherbio.2004.08.051
Srikandakumar, A., and Johnson, E. H. (2004). Effect of heat stress on milk production, rectal temperature, respiratory rate and blood chemistry in Holstein, Jersey and Australian Milking Zebu cows. Trop. Anim. Health Prod. 36, 685–692. doi: 10.1023/B:TROP.0000042868.76914.a9
Tao, S., Orellana, R. M., Weng, X., Marins, T. N., Dahl, G. E., and Bernard, J. K. (2018). Symposium review: the influences of heat stress on bovine mammary gland function 1. J. Dairy Sci. 101, 5642–5654. doi: 10.3168/jds.2017-13727
Thompson-Crispi, K. A., Hine, B., Quinton, M., Miglior, F., and Mallard, B. A. (2012). Short communication: association of disease incidence and adaptive immune response in Holstein dairy cows. J. Dairy Sci. 95, 3888–3893. doi: 10.3168/jds.2011-5201
Trout, J. P., McDowewll, L. R., and Hansen, P. J. (1998). Characteristics of the estrus cycle and antioxidant status of lactating holstein cows exposed to heat stress. J. Dairy Sci. 81, 1244–1250. doi: 10.3168/jds.S0022-0302(98)75685-1
Wagter, L., and Mallard, B. A. (2007). Method of Identifying High Immune Response Animals. Available at: https://patents.google.com/patent/US7258858B2/en?oq=US+Patent.+%237%2C258%2C858 (accessed September 30, 2021).
Wagter, L. C., Mallard, B. A., Wilkie, B. N., Leslie, K. E., Boettche, P. J., and Dekkers, J. C. M. (2000). A quantitative approach to classifying Holstein cows based on antibody responsiveness and its relationship to peripartum mastitis occurrence. J. Dairy Sci. 83, 488–498. doi: 10.3168/jds.S0022-0302(00)74908-3
Wheelock, J. B., Rhoads, R. P., Vanbaale, M. J., Sanders, S. R., and Baumgard, L. H. (2010). Effects of heat stress on energetic matabolism in lactating Holstein cows. J. Dairy Sci. 93, 644–655. doi: 10.3168/jds.2009-2295
Zimbelman, R. B., Rhoads, R. P., Collier, R. J., and Duff, G. C. (2009). A Re-Evaluation of the Impact of Temperature Humidity Index (THI) and Black Globe Humidity Index (BGHI) on Milk Production in High Producing Dairy Cows. Available online at: http://animal.cals.arizona.edu/swnmc/Proceedings/2009/14Collier_09.pdf (accessed September 01, 2021).
Keywords: heat stress, physiological response, immune response, dairy cattle, thermoregulation, management system
Citation: Cartwright S, Schmied J, Livernois A and Mallard BA (2022) Physiological Response to Heat Stress in Immune Phenotyped Canadian Holstein Dairy Cattle in Free-Stall and Tie-Stall Management Systems. Front. Anim. Sci. 3:852958. doi: 10.3389/fanim.2022.852958
Received: 11 January 2022; Accepted: 21 February 2022;
Published: 16 March 2022.
Edited by:
David Renaudeau, Institut National de Recherche pour l'Agriculture, l'Alimentation et l'Environnement (INRAE), FranceReviewed by:
Michelle L. Rhoads, Virginia Tech, United StatesCopyright © 2022 Cartwright, Schmied, Livernois and Mallard. This is an open-access article distributed under the terms of the Creative Commons Attribution License (CC BY). The use, distribution or reproduction in other forums is permitted, provided the original author(s) and the copyright owner(s) are credited and that the original publication in this journal is cited, in accordance with accepted academic practice. No use, distribution or reproduction is permitted which does not comply with these terms.
*Correspondence: Shannon Cartwright, Y2FydHdyaXNAdW9ndWVscGguY2E=
Disclaimer: All claims expressed in this article are solely those of the authors and do not necessarily represent those of their affiliated organizations, or those of the publisher, the editors and the reviewers. Any product that may be evaluated in this article or claim that may be made by its manufacturer is not guaranteed or endorsed by the publisher.
Research integrity at Frontiers
Learn more about the work of our research integrity team to safeguard the quality of each article we publish.