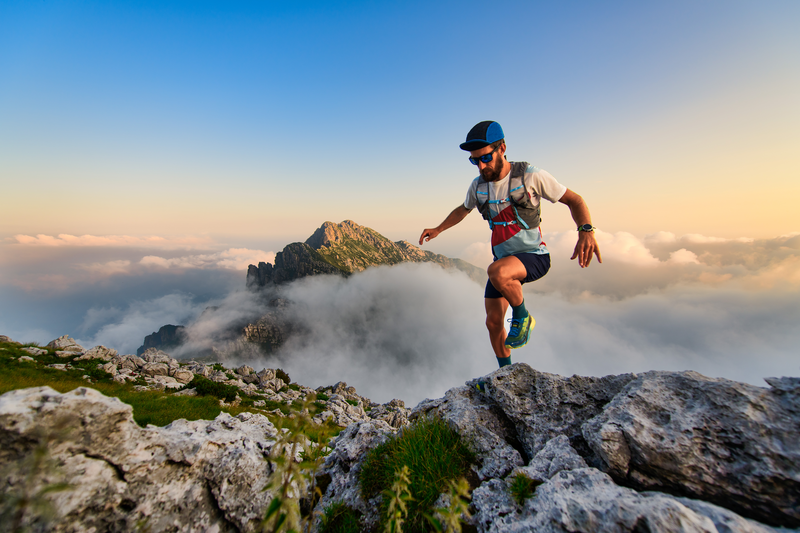
95% of researchers rate our articles as excellent or good
Learn more about the work of our research integrity team to safeguard the quality of each article we publish.
Find out more
ORIGINAL RESEARCH article
Front. Anim. Sci. , 07 April 2022
Sec. Animal Physiology and Management
Volume 3 - 2022 | https://doi.org/10.3389/fanim.2022.847574
This article is part of the Research Topic Embryonic Development, Pregnancy Establishment and Loss View all 9 articles
The objectives of this study were to evaluate the associations between residual dry matter (DM) intake or residual feed intake (RFI) from 1 to 15 weeks postpartum and concentrations of metabolites in plasma in early lactation and reproduction in Holstein cows. Data from 9 experiments, including 851 cows, were used. Intake of DM, milk yield, and body weight were evaluated daily, whereas milk composition and body condition were evaluated twice weekly for the first 105 days postpartum. Blood was sampled on the day of calving and again on days 7, 14, and 21 postpartum and analyzed for concentrations of non-esterified fatty acids (FA), β-hydroxybutyrate (BHB), and glucose. Reproduction was evaluated for the first 300 days postpartum. Residual DM intake was calculated as the observed minus the predicted intake, with intake predicted based on a model that accounted for major energy sinks. Cows were ranked and categorized into RFI quartiles, from the smallest (Q1) to the largest (Q4) RFI (−1.87, −0.46, 0.39, and 1.90 kg/day). Increasing efficiency (i.e., from Q4 to Q1) resulted in linear decreases in DM intake (Q1 to Q4; 18.9, 20.4, 21.3, and 22.7 kg/day), and median days open (132, 125, 135, and 147 d). Conversely, improving efficiency was associated with a linear increase in pregnancy per artificial insemination (AI, 31.4, 30.6, 31.2, and 24.5%) and quadratic increases in the 21-day cycle pregnancy rate (21.2, 21.1, 22.0, and 16.6%) and the proportion of pregnant cows (79.0, 80.7, 82.4, and 71.5%). The estimated net energy for lactation (NEL) content of diets increased linearly with improved RFI (1.88, 1.76, 1.71, and 1.58 Mcal/kg), resulting in no association between RFI and energy-corrected milk yield or body energy change. Nevertheless, increased feed efficiency was associated with a linear increase in concentrations of blood FA (0.68, 0.63, 0.60, and 0.59 mM), but a quadratic association with BHB (0.75, 0.64, 0.64, and 0.65 mM), with no association with glucose. Collectively, the most feed efficient cows ate 3.8 kg/day less DM, produced the same amount of energy-corrected milk, and had improved reproductive performance compared with the least efficient cows, thus suggesting that the underlying mechanisms responsible for improved feed efficiency might also be linked with improvements in reproduction.
Feed efficiency is an important measure of production efficiency because feed represents more than 50% of the cost of production in dairy farms (USDA-ERS, 2018). Improving the efficiency of nutrient utilization not only is economically beneficial, but also minimizes the footprint of dairy production. Therefore, implementing management practices that improve the efficiency of nutrient utilization is desirable because of the relationship between economic returns and improvement of land utilization (Bach et al., 2020). A common measure of feed efficiency is feed conversion ratio, typically expressed as kilograms of energy-corrected milk (ECM) per kilogram of dry matter (DM) intake. However, the feed conversion ratio is often high in the 1st month or two of lactation because the increment in ECM in the 1st weeks postpartum outpaces that of DM intake, and a portion of the nutrients required for the synthesis of milk originates from mobilized body tissues (Drackley, 1999). It is well-described that excessive loss of body weight (BW) or body condition score (BCS) in early lactation is negatively associated with pregnancy per AI or maintenance of pregnancy in dairy cows (Santos et al., 2010a; Carvalho et al., 2014), and improving feed efficiency at the expense of body reserves is expected to compromise reproduction. Thus, because the feed conversion ratio does not account for the energy accreted or released by changes in BW and BCS, it would be misleading to use such metrics to assess feed efficiency in dairy cows for selection purposes (VandeHaar et al., 2016).
A proposed method to measure feed efficiency is to use residual DM intake also called residual feed intake (RFI), which accounts for the energy needs for maintenance and milk synthesis, the energy accreted or released by gain or loss of body tissues, and adjusting for the cohort. As such, using RFI as a measure of feed efficiency is expected to minimize any impact on BW and BCS changes (Nehme Marinho et al., 2021). In fact, ranking cows for feed efficiency based on RFI was not associated with body energy changes in early lactation or with the risk of peripartum diseases or survival (Nehme Marinho et al., 2021). More efficient cows likely have reduced needs for maintenance, are capable of better digesting organic matter, and are more efficient at using absorbed nutrients for productive functions, which would reduce the net energy for lactation (NEL) needed for maintenance and increase the NEL content of the diet consumed. In such cases, one could postulate that reducing the maintenance needs and improving the use of consumed nutrients would improve feed efficiency without affecting reproduction, in spite of the calculated NEL balance to be reduced (Seymour et al., 2020; Nehme Marinho et al., 2021).
It was hypothesized that using RFI to rank cows for feed efficiency is not associated with reproduction in dairy cows. Thus, the objectives of this study were to evaluate the associations between RFI and important measures of reproduction during lactation in Holstein cows. Additional objectives were to evaluate the association between RFI and the estimated NEL content of the diet consumed and concentrations of metabolites in the blood in early lactation.
The study design was a retrospective cohort study. The exposure factor was the RFI category measured in the first 105 days postpartum. The sample size was a convenience sample based on the data available from nine experiments in which DM intake and production performance were evaluated daily in 851 postpartum Holstein cows and detailed information on blood metabolites and reproduction were collected from the study population.
Data from nine experiments conducted at the University of Florida were used for this study (Greco, 2014; Martinez et al., 2018a,b; Zenobi et al., 2018; Bollatti et al., 2020a,b; Lobo et al., 2021; Oyebade, 2021; Silva, 2021; Zimpel et al., 2021a,b; Nehme Marinho et al., 2022). The experimental procedures with cows were approved by the Institutional Animal Care and Use Committee of the University of Florida.
The experiments were conducted between December 2008 and July 2021, and they all followed randomized block designs and involved dietary manipulations. Data were available from 851 lactating Holstein cows, 342 primiparous, and 509 multiparous with a mean (± SD) of 2.0 ± 1.1 lactations (1–8 lactations). Cows were housed in sand-bedded free-stall barns equipped with fans placed above the beds and a water soaker line with nozzles placed ~30 cm above the cows' withers on the feeding alley for evaporative cooling of cows. Fans and water spraying were controlled by thermostats and activated when the ambient temperature reached 18–20°C, depending on the experiment. Cows were randomly assigned to individual feeding gates (Calan Broadbent Feeding System, American Calan Inc., Northwood, NH) used to evaluate daily feed intake. Cows had ad libitum access to their respective diets that were fed as totally mixed rations (TMRs). The TMRs were weighed and fed twice daily at ~06:00 h and 13:00 h and refusals were weighed once daily, before the morning feeding, from 1 to 105 days postpartum. The amount of TMR offered to individual cows was adjusted daily to result in 5–10% refusals.
Dietary ingredients were sampled twice weekly and dried at 55°C for 48 h in a forced-air oven and dry weights were recorded. Dried feed samples were ground to pass a 1 mm screen of a Wiley mill (Thomas Scientific, Swedesboro, NJ), composited at each 4 to 8-week period, depending on the experiment, and analyzed according to AOAC (2012) methods for nitrogen (AOAC, 990.03), acid detergent fiber (AOAC, 973.18), neutral detergent fiber (AOAC, 2002-04), fat (AOAC, 2003.05), minerals (AOAC, 985.01), and DM at 105°C for 24 h. The DM content of diets was calculated by multiplying the DM obtained at 55°C by the DM obtained at 105°C. It was assumed that refusals had the same DM content as the diet offered. Intake of DM for individual cows was based on the amount of TMR offered minus the amount refused multiplied by the DM content of the TMR in a particular week.
In each experiment, cows were scored for body condition on the day of calving and again once or twice weekly by trained evaluators using a 1–5 scale with increments of 0.25 units as depicted in the Elanco BCS chart (Elanco Animal Health, 2009). Cows were weighed twice daily immediately after each milking on a walk-through scale (AfiWeigh, SAE Afikim, Israel) as they left the milking parlor, and the mean daily BW was calculated. Daily means were used to generate weekly means for BW. Weekly changes in BW were calculated by subtracting the mean BW in a given week minus the mean BW in the preceding week. For the 1st week postpartum, the value obtained was the difference between the mean value from 1 to 7 days postpartum minus the value of the day of calving. The obtained value was divided by the number of days between measurements to generate a daily change in BW in kg/day.
Milk yield was recorded using electronic milk flow meters (AfiFlo, SAE Afikim) at each milking and the daily yield was calculated by adding the individual milk weights per cow. Cows were milked twice daily at 12-h intervals in eight experiments, and 4 times daily, at 6-h intervals in one experiment. Samples of milk were taken at each milking 2 days per week until 105 days postpartum. Samples of milk were analyzed for fat, protein, and lactose at the Southeast Milk Inc. DHI laboratory (Belleview, FL). The amounts of fat, protein, and lactose produced on the sampling days were calculated according to the milk yield and composition of each milking, summed to obtain the daily yield, and then divided by the total milk produced to calculate the daily concentrations of fat, protein, and lactose. The values were then applied to the mean milk produced in the respective week to calculate the weekly yields of milk components.
Blood was collected on the days 0, 7, 14, and 21 postpartum by puncture of the coccygeal vessels into evacuated tubes (Vacutainer, Becton Dickinson, Franklin Lakes, NJ) containing either no anticoagulant or lithium heparin. Blood was sampled before the morning feeding, except for the day of calving in which blood was collected within the 1st hours after parturition regardless of the time of the day. Upon collection, tubes were placed on ice and then centrifuged within 30 min of collection at ~2,000 × g for 15 min at room temperature, serum or plasma harvested, and multiple aliquots of 1.5 ml were frozen at −30°C until analyses. Throughout the manuscript, samples will be denominated as plasma because most experiments used evacuated tubes with the anticoagulant. Plasma samples were assayed for concentrations of FA, β-hydroxybutyrate (BHB), and glucose. Details of each assay are presented in the respective manuscripts. Briefly, assays followed the initial randomization with blocks, such that samples from each block were analyzed in the same assay. In every assay, plasma samples were included multiple times at the beginning and end of the assay to calculate intra- and interassay coefficients of variations, which were all below 10%. Plasma concentrations of FA were assayed using a commercial kit (NEFA-C kit; Wako Diagnostics Inc., Richmond, VA) according to Johnson and Peters (1993). Plasma concentrations of BHB were assayed using a commercial kit (Wako Auto- kit 3-HB; Wako Diagnostics Inc.), and those of glucose were assayed using a colorimetric assay in microplates (TECO diagnostic, Anaheim, CA) or using a Technicon Autoanalyzer (Technicon Instruments Corp., Chauncey, NY) according to Gochman and Schmitz (1972).
All cows were subjected to timed AI for the first insemination. Briefly, cows had the estrous cycle pre-synchronized with either two doses of prostaglandin F2α (Moreira et al., 2001) administered 14 days apart or with the Ovsynch protocol (Souza et al., 2008). The timed AI protocol started either 14 or 7 days later, depending on whether cows received the pre-synchronization with prostaglandin F2α only or with the Ovsynch protocol, respectively. Cows were time inseminated following the Ovsynch protocol (Pursley et al., 1995) or the 5-d timed AI protocol (Santos et al., 2010b). Cows received the first postpartum AI between 70 and 80 days postpartum, depending on the experiment.
All cows were fitted with pedometers (Afi Act II, Afimilk), and those that returned and were detected in estrus after an AI were inseminated on the same day. Cows that were re-inseminated before any pregnancy diagnosis were considered not pregnant to the previous AI. Pregnancy was diagnosed on day 32 after each AI, based on the presence of an amniotic vesicle with an embryo with the heartbeat, detected by transrectal ultrasonography. Pregnant cows on day 32 after AI were reevaluated for pregnancy on day 74 after insemination. Cows diagnosed as non-pregnant had the estrous cycle resynchronized for timed AI with either the Ovsynch protocol (Pursley et al., 1995) or the 5-d timed AI protocol (Santos et al., 2010b).
For the statistical analyses, the diagnosis performed on day 74 after AI was used to determine whether a cow became pregnant to any AI within the first 300 days postpartum. Cows designated by the farm manager to become “do not inseminate,” or those that were sold or died, or remained non-pregnant by 300 days postpartum were censored on the respective dates during survival analysis, whichever happened first. For calculations of 21-day cycle insemination rate or 21-day cycle pregnancy rate, a cow that passed the voluntary waiting period of each experiment was considered eligible and it was assumed that the length of the estrous cycle was 21 days for calculation. Any eligible cow was expected to be inseminated each 21-day interval until becoming pregnant or being sold, dead, or designated “do not inseminate” by 300 days postpartum, whichever happened first. Measurements of reproduction were evaluated for the first 300 days postpartum or until the cow was diagnosed pregnant or was censored, whichever happened first. Responses measured included the proportion of cows receiving at least one AI, days postpartum at first AI, pregnancy per AI at first AI, pregnancy loss at first AI, days postpartum at second AI, pregnancy per AI at second AI, pregnancy loss at second AI, pregnancy per AI all AI, 21-day cycle insemination rate, 21-day pregnancy rate, pregnant by 300 days postpartum, days open, number of AI per pregnancy, and proportion of cows designated “do not inseminate” and the respective day postpartum.
Diseases were recorded for each cow for the first 90 days postpartum and included milk fever, retained placenta, metritis, mastitis, displaced abomasum, lameness, and pneumonia. Details of disease diagnosis were provided elsewhere (Nehme Marinho et al., 2021). A cow was considered to have morbidity if diagnosed with at least one clinical disease in the first 90 days postpartum. Morbidity was used as a covariate in all statistical analyses.
All calculations were performed using the mean values for each week postpartum. The cow was the unit of analysis. ECM yield and the NEL content in milk were calculated according to National Research Council (NRC) nutrient requirements of dairy cattle (NRC, 2001) as follows: ECM, kg/day = [(0.3246 × milk yield) + (12.86 × fat yield) + (7.04 × protein yield)]; NEL in milk, Mcal/kg = [(0.0929 × fat %)] + [(0.0563 × protein %)] + [(0.0395 × lactose %)]. The NEL content in milk was multiplied by milk yield to calculate the amount of NEL secreted in milk daily.
Body energy that was accreted or mobilized as body tissue reserves was calculated from changes in BW as daily loss or gain in kg/day, and considering BCS (1–5) measurements to adjust the NEL content in tissue gained or mobilized using an equation from NRC (2001) as follows: body energy change (Mcal/day) = [(1.88 + 1.036 × BCS) × change in BW)]. Values for body energy change are expressed as Mcal of NEL per day. The NEL required for maintenance was calculated based on metabolic BW as follows: NEL for maintenance (Mcal/day) = BW0.75 × 0.086 (Moraes et al., 2015).
The content of NEL of the diet consumed by each cow was estimated as follows: diet NEL content, Mcal/kg = (NEL required for maintenance + NEL required for milk synthesis + NEL required for body energy change)/DM intake. The individual values were analyzed to determine differences in estimated dietary NEL content according to RFI.
Data were analyzed by the MIXED, GLIMMIX, and PHREG procedures of SAS 9.4 (SAS/STAT version 9.4; SAS Institute Inc., Cary, NC) for continuous, binomial, and time to event data, respectively. The associations calculated in this study were all based on phenotypic measurements.
Predicted DM intakes were calculated for each cow fitting a linear mixed-effects model, using the MIXED procedure or SAS (SAS/STAT; SAS Institute Inc.), that regressed the mean DM intake with major energy sinks. The statistical model included fixed effects of NEL secreted in milk, metabolic BW, body energy change, parity (primiparous vs. multiparous), the random effect of treatment nested within the experiment, and the residual error term. Then, RFI (kg/day) was calculated as the residuals based on the difference between observed and predicted DM intake. The coefficient of determination (R2) of the model was 0.615. All variables included in the model significantly (P < 0.05) contributed to explain the variance in DM intake. The R2 values for the fixed effects were 0.289 for NEL secreted in milk, 0.0886 for metabolic BW, 0.121 for body energy change, and 0.0034 for the parity group. The random effect of treatment nested within the experiment resulted in R2 of 0.1135. The root mean square of the error for the model was 1.59, and the model equation was (SE): DM intake = 0.869 (0.7924) + 0.3482 (0.01406) × NEL secreted + 0.08542 (0.00618) × BW0.75 + 0.2715 (0.01569) × body energy change + 0.6706 (0.1998) multiparous.
Cows were ranked by RFI and then grouped into quartiles, with quartile 1 being the most efficient (Q1, smallest RFI) and quartile 4 being the least efficient cows (Q4, largest RFI). The rationale to use quartiles and not the linear covariate of RFI was because the analyses performed using RFI as a continuous or categorical variable resulted in the same inferences relative to the associations with the reproduction or plasma metabolites. By categorizing RFI into quartiles and using orthogonal polynomials, it allowed the presentation of results with least-squares means (LSM) and respective SEM without altering the inferences relative to the results of the analyses. A similar approach has been previously used (Connor et al., 2013; Fischer et al., 2018; Nehme Marinho et al., 2021).
Intake of DM, changes in body energy, NEL secreted in milk, ECM, and NEL of diet in the first 15 weeks of lactation were analyzed with mixed-effects models with the MIXED procedure of SAS (SAS/STAT; SAS Institute Inc.). The models included the fixed effects of quartile of RFI (Q1–Q4), week postpartum (1–15), the interaction between RFI quartile and week postpartum, morbidity (no vs. yes), and the random effects of month–year of calving, cow nested within RFI quartile, and the residual error. Plasma concentrations of FA, BHB, and glucose were analyzed with mixed-effects models that included the fixed effects of RFI quartile, day postpartum, the interaction between RFI quartile and day postpartum, morbidity, and the random effects of month–year of calving, cow nested within RFI quartile, and the residual error. In all repeated measures models, the day or week postpartum was the term included in the REPEATED statement. The covariance structure (unstructured, autoregressive 1, autoregressive heterogeneous 1, Toeplitz) with the smallest Akaike's information criterion was selected for each variable analyzed. The Kenward–Roger method was used to calculate the approximate denominator degrees of freedom (DF) for the F tests. When an interaction between quartile and day postpartum resulted in P < 0.10, then means at different time points were partitioned using the SLICE command of SAS. Data are presented as LSM and respective SEM.
Binary data, such as the proportion of cows that received AI, the proportion of cows that became do not inseminate, pregnancy per AI, pregnancy loss, and the proportion of cows pregnant by 300 days postpartum, were analyzed by generalized linear mixed-effects models using logistic regression with the GLIMMIX procedure of SAS (SAS/STAT, SAS Institute Inc.). The statistical models included the fixed effects of RFI quartile and morbidity and the random effect of month–year of calving. For responses with more than one measurement per cow, such as 21-day cycle insemination rate and 21-day cycle pregnancy rate, the fixed effect of cycle number and the random effect of the cow also, were included in the statistical models. Dispersion of the data for the generalized linear mixed models was assessed by the ratio of the generalized chi-square statistic DF (χ2/DF). In all models, except for pregnancy loss at second AI, the generalized χ2/DF was between 0.95 and 1.05 indicating that the variability in the data was properly modeled, and that only minor residual under- or over-dispersion was present. For pregnancy loss at the second AI, residual under-dispersion was present based on the χ2/DF of 0.85, and a multiplicative dispersion parameter estimated from Pearson's statistic was added to the model. Data are presented as LSM and respective SEM.
The number of AI per pregnancy was analyzed with the GLIMMIX procedure of SAS (SAS/STAT, SAS Institute Inc.) fitting a negative binomial distribution. The statistical model included the fixed effects of RFI quartile and morbidity and the random effect of month–year of calving.
Days to pregnancy were analyzed with Cox's proportional hazard regression using the PHREG procedure of SAS (SAS/STAT, SAS Institute Inc.). The statistical model included the fixed effects of RFI quartile and morbidity and the random effect of month–year of calving. The adjusted hazard ratios and respective 95% CI were calculated. Survival curves were generated adjusted for covariates in the statistical models using the BASELINE statement in PHREG of SAS (SAS/STAT, SAS Institute Inc.). Model fit was assessed by plotting the martingale and deviance residuals against the estimates of the linear predictor. The proportionality of hazards among RFI quartiles was evaluated using the ASSESS statement for proportional hazard in PHREG. The LSM ± SEM and median days open (95% CI) were calculated with the LIFETEST procedure of SAS (SAS/STAT, SAS Institute Inc.).
In all the statistical models, orthogonal polynomial contrasts were used to determine linear, quadratic, or cubic associations of quartile of RFI and the response analyzed. The interactive matrix language procedure of SAS (PROC IML; SAS/STAT, SAS Institute Inc.) was used to generate the orthogonal coefficients to adjust for the unequal spacing of the median values for RFI among quartiles.
Statistical significance was considered at P ≤ 0.05, and tendency when 0.05 < P ≤ 0.10.
A total of 851 cows were included in the study and contributed data for statistical analyses for production and reproduction. Four of the nine experiments did not have plasma samples analyzed for FA, BHB, and glucose concentrations; therefore, the number of cows included in the analyses of plasma metabolites was 473. Descriptive statistics of RFI and productive performance of cows according to quartiles are presented in Table 1.
Table 1. Descriptive statistics of performance of dairy cows from 1 to 105 days postpartum according to residual dry matter (DM) intake [residual feed intake (RFI)] quartile.
The difference in RFI between the most and least efficient quartiles was 3.8 kg/day, and the estimated NEL of the diet increased linearly (P < 0.001) as the RFI quartile decreased. Cows in Q1 consumed diets that provided 19% more NEL than those in Q4 (1.88 vs. 1.58 Mcal/kg). No difference was observed for the yield of ECM (38.6 kg/d) or body energy change (−1.48 Mcal/d) among quartiles.
Improving efficiency, based on reduced RFI quartile, was linearly associated (P < 0.001) with an increase in concentrations of FA and quadratically (P = 0.01) associated with the concentration of BHB in plasma, although for BHB differences among groups were observed only on day 7 postpartum (Figure 1; Table 2). Cows in Q1 had greater (P < 0.01) BHB concentration on day 7 than those in the other three quartiles (Figure 1B). Concentrations of glucose only differed (P = 0.02) among groups on day 0 and cows in Q4 had the smallest concentration of glucose (Figure 1C).
Figure 1. Concentrations of fatty acids (A), β- hydroxybutyrate (B), and glucose (C) in the plasma of Holstein cows according to quartile of residual dry matter (DM) intake [residual feed intake (RFI)]. Residual DM intake was calculated from 1 to 105 days postpartum based on the difference between observed and predicted DM intake, and cows were grouped into quartiles (Q1, the smallest RFI, to Q4, the largest RFI). Predicted DM intake was calculated following a statistical model that considered net energy for lactation (NEL) secreted as milk, metabolic BW, body energy change, parity group, and treatment nested within the experiment. (A) The effect of RFI quartile (P = 0.003) and interaction between RFI quartile and day postpartum (P = 0.26). (B) The effect of RFI quartile (P = 0.001) and interaction between RFI quartile and day postpartum (P = 0.002). (C) The effect of RFI quartile (P = 0.47) and interaction between RFI quartile and day postpartum (P = 0.06). Error bars represent the SEM. Within day postpartum, *denotes difference among quartiles (P < 0.05).
Table 2. Association of quartiles of residual DM intake (RFI) and plasma metabolites in Holstein cows.
The proportions of cows that received at least one AI in the study did not differ with the RFI quartile, with a mean of 98.5% (Table 3). The day postpartum at the first AI did not differ among quartiles of RFI and had a mean of 77.9 d. Similarly, the proportion of cows that became pregnant to the first AI or lost pregnancy between 32 and 74 days after the first AI did not differ with the RFI quartile. Cows received the second postpartum AI with a mean of 115.5 days in lactation. Pregnancy per AI at the second AI linearly increased (P < 0.001) as efficiency increased. For instance, as the RFI quartile changed from Q4 to Q1, pregnancy per AI on day 74 after the second AI increased from 17.6 to 38.5% (Table 3). No association was observed between RFI and pregnancy loss at the second AI.
Table 3. Association between quartiles of residual DM intake (RFI) and reproduction in the first two postpartum AI in Holstein cows.
The proportions of cows designated as “do not inseminate” and the day postpartum when inseminations stopped did not differ among RFI quartiles (Table 4). The 21-day cycle rate of insemination did not differ with RFI resulting in a mean of 67.9% in the first 300 days postpartum. Pregnancy per AI at all postpartum inseminations increased linearly (P = 0.01) with reducing RFI. Cows in Q4 had the smallest pregnancy per AI at all AI, whereas no difference was observed among cows in Q1 to Q3. Similarly, the 21-day cycle pregnancy rate increased linearly (P = 0.03) with improved feed efficiency, and cows in Q4 had the longest days open and the slowest rate of pregnancy (Figure 2; Table 4). The differences in the rate of pregnancy resulted in a quadratic association (P = 0.04) between RFI and the proportion of cows pregnant by 300 days postpartum, and cows in Q4 had the smallest proportion of pregnancy. In fact, the mean number of AI per pregnancy increased linearly (P = 0.02) as RFI increased (Table 4).
Table 4. Association between quartiles of residual DM intake (RFI) and reproduction in the first 300 days postpartum in Holstein cows.
Figure 2. Survival curves for time to pregnancy in Holstein cows according to quartile of residual DM intake (RFI). Residual DM intake was calculated from 1 to 105 days postpartum based on the difference between observed and predicted DM intake, and cows were grouped into quartiles (Q1, smallest RFI, to Q4, largest RFI). Predicted DM intake was calculated following a statistical model that considered NEL secreted as milk, metabolic BW, body energy change, parity group, and treatment nested within the experiment. Cows from quartile 4 had extended time to pregnancy (P < 0.05) compared with cows from quartiles 1, 2, or 3. The adjusted hazard ratios for the daily rate of pregnancy were greater for Q1 (1.29; 95% CI = 1.03–1.62), Q2 (1.25; 95% CI = 1.00–1.57), and Q3 (1.32; 95% CI = 1.05–1.65) than cows in Q4.
Selection for feed efficiency in dairy cattle is not a novel concept (Veerkamp, 1998), but concerns exist with imposing selection methods that favor animals that consume less DM because of reduced energy balance that could compromise reproduction (Santos et al., 2010a). In this study, ranking cows for RFI, a measure of feed efficiency that accounts for the major energy sinks, was associated with reduced DM intake, but no impacts on yield of ECM, secretion of NEL in milk, or body energy change were observed in the first 105 days postpartum. The lack of associations between RFI and ECM and body energy change was expected because residuals have independence from the variables used to predict the response. In spite of small increases in concentrations of plasma metabolites linked to lipomobilization as efficiency increased, more efficient cows had improved pregnancy per AI and increased rate of pregnancy compared with the quartile of least efficient cows, which resulted in a reduction in median days open and increased proportion of pregnant cows by 300 days postpartum.
A possible reason for the reduced DM intake by efficient cows might be related to their ability to extract nutrients from the diet. The estimated NEL density of the diets consumed provided 19% more energy for cows in Q1 than those in Q4. The estimated differences suggest that more efficient cows might have increased digestion and absorption of nutrients (Tyrrell and Moe, 1975), thus attending the nutrients demanded lactation with less intake. Obviously, the differences in estimated NEL content for the diets consumed assumed that maintenance requirements remained constant among cows with the same metabolic BW in different quartiles of RFI, which might not necessarily be true. Variability exists in maintenance requirements of groups of cows (Ellis et al., 2006), and changes in maintenance likely help explain differences in RFI among cows (Dong et al., 2015; Meale et al., 2017). The body composition, such as organs size and tissue composition, likely affects the energy used for basal metabolic rate, and studies with beef bulls reported smaller rumen, bladder, and heart associated with low RFI (Fitzsimons et al., 2014; Meale et al., 2017). The differences among organs sizes can indicate less energy expenditure for maintenance, therefore, an expected larger fraction of absorbed nutrients become available to be partitioned to production.
Increments in concentrations of FA and BHB are often observed in early lactation cows during periods of negative nutrient balance associated with losses in BW and BCS as part of the homeorhetic mechanisms to support lactation. Extensive lipomobilization is linked with a delayed resumption of estrous cyclicity and depression in pregnancy per AI in dairy cows (Butler, 2000; Santos et al., 2010a). Nevertheless, although cows with negative RFI have reduced DM intake, as observed in this study, which decreased net energy balance (Nehme Marinho et al., 2021), they did not experience differences in changes in body energy compared with cows with positive RFI. The literature has indicated that dairy or beef cattle selected for negative RFI have increased lipolytic signals based on increased concentrations of FA in the blood (Mäntysaari et al., 2012) and increased concentration of growth hormone (Dechow et al., 2017), and decreased lipogenic signals, such as concentrations of leptin (Xi et al., 2016) and insulin (Richardson and Herd, 2004). However, the increased lipolysis in cows with reduced RFI seems to not influence daily changes in BW or BCS (Fischer et al., 2018; Nehme Marinho et al., 2021) or disease incidence (Martin et al., 2021; Nehme Marinho et al., 2021). Although the reduced DM intake observed in more efficient cows likely explains the minor increases in lipomobilization and ketogenesis in early lactation, no differences were observed in body energy change either because the NEL content of the diet consumed differed as estimated herein, or the maintenance requirements differed among cows in different quartiles of RFI.
Conserved mechanisms exist across species to regulate reproductive cycles when nutrient availability and endocrine signals are inadequate during periods of negative nutrient balance (Butler, 2000; Schneider, 2004). The loss of BW or BCS and the increased risk of diseases that are common in early lactation are known to depress reproduction in dairy cows (Santos et al., 2010a; Carvalho et al., 2014; Ribeiro et al., 2016). Energy balance is linked with reproduction in dairy cows and other species (Butler, 2000; Schneider, 2004; Santos et al., 2010a) and it is expected that energy balance should reflect changes in body energy; however, energy balance calculation assumes a fixed value for NEL of a diet with some discount for DM intake (NRC, 2001), and a constant NEL required for maintenance for a given metabolic BW, which results in an inaccurate assessment of the energy status of cows (Nehme Marinho et al., 2021).
Residual DM intake has been shown not to be associated with changes in body energy or risk of diseases in early lactation (Nehme Marinho et al., 2021), which are known risk factors linked with reproduction in dairy cows (Butler, 2000; Santos et al., 2010a; Ribeiro et al., 2016). Nehme Marinho et al. (2021) showed that NEL balance is positively and linearly associated with RFI, but RFI had no association with body energy changes in early lactation suggesting that NEL balance, as calculated, penalizes more efficient cows. Therefore, the estimated 19% increase in NEL content of diets consumed by cows in Q1 compared with cows in Q4 might reflect differences in ruminal and total tract organic matter digestibility (Tyrrell and Moe, 1975), differences in post-absorptive metabolism of nutrients, or differences in maintenance requirements often observed in different groups of cows (Ellis et al., 2006).
Reproduction in all experiments that contributed data to this study was managed with a combination of AI upon detection of estrus and timed AI. Subjecting cows to timed AI at first AI likely abolished some of the potential associations of RFI with estrous cyclicity and interval to first insemination postpartum (Santos et al., 2010a), although it might not completely abolish the potential effects of differences in energy balance with pregnancy per AI (Bisinotto et al., 2010; Santos et al., 2016). Because of the management used for first postpartum insemination, no difference in days to the first AI was expected or observed in this study. On the other hand, it was surprising to note that more efficient cows had increased pregnancy per AI at all postpartum inseminations. More efficient cows had a greater 21-day cycle pregnancy rate and, therefore, an increased hazard of pregnancy in the first 300 days postpartum that resulted in a tendency for a larger proportion of pregnant cows at the end of the study compared with cows in Q4. Obviously, these results would not agree with the commonly discussed mechanisms of energy balance affecting reproduction when NEL balance is calculated assuming constant values for the energy density of diets fed.
Studies assessing RFI and reproduction in cattle are scarce. Macdonald et al. (2016) evaluated reproduction in grazing dairy cows according to their RFI evaluated between 5 and 9 months of age. The authors showed that divergence in RFI as heifers was not associated with production, BW, BCS, or reproduction as lactating cows. In beef heifers, improved feed efficiency, based on reduced RFI, was associated with extended age at puberty, but no associations were observed between RFI and pregnancy per AI or rate of pregnancy (Shaffer et al., 2011). The authors attributed the differences in age at puberty related to the reduced degree of fatness in more efficient heifers. In beef cattle, heifers with negative RFI typically contain 2–5% less body fat than heifers with positive RFI (Basarab et al., 2011), and the degree of fatness is positively associated with reproduction in beef cattle (Randel and Welsh, 2013). In general, reducing the degree of fatness in more efficient beef heifers is associated with delayed puberty (Basarab et al., 2011) and, in some cases, reduced proportion of pregnancy (Basarab et al., 2011), which was not observed once fatness was included in the statistical model. Fatness, based on BCS, was not altered with RFI in lactating dairy cows (Nehme Marinho et al., 2021), and body energy changes did not differ in this study in cows in the different quartiles of RFI. Not only reproduction was not depressed with improved efficiency in dairy cows, the least efficient cows, those in Q4, had the smallest hazard of daily pregnancy and longest median days open. It is very likely that the reproductive management imposed eliminated potential differences in detection of estrus and insemination rate, as evidenced by the lack of differences in 21-day cycle insemination rate and pregnancy at first AI. Thus, differences in the hazard of pregnancy in the first 300 days postpartum were mediated by the differences in pregnancy per AI that were observed after the first postpartum insemination.
Perhaps what makes dairy cows less efficient from the production perspective, such as differences in post-absorptive metabolism of nutrients (Guinguina et al., 2020), metabolic and hormonal regulation, (Cantalapiedra-Hijar et al., 2018; Clemmons et al., 2019), thermoregulation (Guinguina et al., 2020), and behavior (Connor et al., 2013), might also influence aspects of reproduction that compromise pregnancy per AI. For instance, mitochondrial metabolism of pyruvate and fatty acids play critical roles in mammalian oocyte and embryo development. Although oxidative metabolism is critical for gametes and embryos, it generates reactive oxygen species that can damage cells (Bradley and Swann, 2019). Mitochondria from beef cattle with increased feed efficiency had a greater degree of coupling between respiration and oxidative phosphorylation observed in hepatic (Casal et al., 2018) and skeletal muscle (Kolath et al., 2006). Increased capture of cellular energy might reduce proton leakage and the risk of damaging cellular membranes in reproductive tissues (Bradley and Swann, 2019). Furthermore, evidence exists that more efficient animals have increased antioxidant capacity in hepatic cells (Casal et al., 2019). It is possible that the differences observed in liver and skeletal muscle might be present in other tissues, including ovaries, endometrium, and gametes, thus favoring the development of oocytes, early embryos, and the conceptus that improves pregnancy per AI and increases the rate of pregnancy. Also, it is possible that the supply of oxidizable fuels to the reproductive axis differs among cows of diverging RFI. The hypothalamic–pituitary–gonadal axis is sensitive to the availability of oxidizable metabolic fuels, in particular glucose and fatty acids (Schneider, 2004). Genetic correlations exist between RFI and fertility traits in Holstein cattle, and the genomic predicted transmitting ability for RFI and daughter pregnancy rate (−0.09), heifer conception rate (−0.02), and cow conception rate (−0.14) all favor improved genetic measures of fertility as genetics for feed efficiency improved based on genomic RFI values (Li et al., 2020). Perhaps, the phenotypic associations of improved reproduction in more feed-efficient cows observed herein have a genetic link. Also, it is possible that more efficient cows sense endocrine cues differently than less efficient cows, which might be linked with genetic variability or environmental factors taking place in early life. An example in bovine is that altered nutrition in early life affects the hypothalamic transcriptome linked with accelerated puberty that favors reproduction (Sánchez et al., 2021).
Cows with reduced RFI in the first 15 weeks of lactation had similar milk energy output or changes in body energy, but a small increase in plasma concentrations of FA and BHB suggesting increased lipomobilization in the 1st weeks of lactation. An intriguing finding of this study was the association between RFI and reproduction, in which the least efficient cows also had reduced hazard of pregnancy and extended days open mediated by the reduced pregnancy per AI. Although the study does not allow mechanisms to be drawn, it is postulated that cows with positive RFI not only have less efficient use of nutrients based on the marked reduction in NEL content of the diets consumed, but potential changes in post-absorptive metabolism of nutrients that might impact their ability to establish or maintain pregnancy.
The raw data supporting the conclusions of this article will be made available by the authors, without undue reservation.
The animal study was reviewed and approved by the University of Florida Institutional Animal Care and Use Committee.
MN and JS developed the study design, performed the data collection, statistical analyses, manuscript preparation, and revised the manuscript. All authors contributed to the article and approved the submitted version.
The authors are grateful for the financial support for this study provided by grants from the Southeast Milk Check-Off Program (Southeast Milk Inc., Belleview, FL), Balchem Animal Nutrition and Health (New Hampton, NY), Arm and Hammer Animal and Food Production (Trenton, NJ), the Foundation for Food and Agriculture Research (FFAR), and the Council on Dairy Cattle Breeding (CDCB, Bowie, MD), with the last two sponsors listed providing the grant number CA18-SS-0000000236.
The authors declare that the research was conducted in the absence of any commercial or financial relationships that could be construed as a potential conflict of interest.
The reviewer PP declared a past collaboration with one of the authors JS to the handling editor.
All claims expressed in this article are solely those of the authors and do not necessarily represent those of their affiliated organizations, or those of the publisher, the editors and the reviewers. Any product that may be evaluated in this article, or claim that may be made by its manufacturer, is not guaranteed or endorsed by the publisher.
The authors thank Juan M. Bollatti, Leandro F. Greco, Richard Lobo, Natalia Martinez, Ana Carolina M. Silva, Adeoye Oyebade, Marcos Zenobi, and Roney Zimpel (University of Florida) for providing data for this study.
AOAC (2012). Official Methods of Analysis. 19th Edn. Gaithersburg, MD: Association of Official Agricultural Chemists International.
Bach, A., Terré, M., and Vida, M. (2020). Symposium review: decomposing efficiency of milk production and maximizing profit. J. Dairy Sci. 103, 5709–5725. doi: 10.3168/jds.2019-17304
Basarab, J. A., Colazo, M. G., Ambrose, D. J., Novak, S., McCartney, D., and Baron, V. S. (2011). Residual feed intake adjusted for backfat thickness and feeding frequency is independent of fertility in beef heifers. Can. J. Anim. Sci. 91, 573–584. doi: 10.4141/cjas2011-010
Bisinotto, R. S., Chebel, R. C., and Santos, J. E. P. (2010). Follicular wave of the ovulatory follicle and not cyclic status influences fertility of dairy cows. J. Dairy Sci. 93, 3578–87. doi: 10.3168/jds.2010-3047
Bollatti, J. M., Zenobi, M. G., Artusso, N. A., Alfaro, G. F., Lopez, A. M., Barton, B. A., et al. (2020a). Timing of initiation and duration of feeding rumen-protected choline affects performance of lactating Holstein cows. J. Dairy Sci. 103, 4174–4191. doi: 10.3168/jds.2019-17293
Bollatti, J. M., Zenobi, M. G., Artusso, N. A., Lopez, A. M., Nelson, C. D., Barton, B. A., et al. (2020b). Effects of rumen-protected choline on the inflammatory and metabolic status and health of dairy cows during the transition period. J. Dairy Sci. 103, 4192–4205. doi: 10.3168/jds.2019-17294
Bradley, J., and Swann, K. (2019). Mitochondria and lipid metabolism in mammalian oocytes and early embryos. Int. J. Dev. Biol. 63, 93–103. doi: 10.1387/ijdb.180355ks
Butler, W. R. (2000). Nutritional interactions with reproductive performance in dairy cattle. Anim. Reprod. Sci. 60-61, 449–457. doi: 10.1016/S0378-4320(00)00076-2
Cantalapiedra-Hijar, G., Abo-Ismail, M., Carstens, G., Guan, L., Hegarty, R., Kenny, D., et al. (2018). Review: biological determinants of between-animal variation in feed efficiency of growing beef cattle. Animal 12, s321–s335. doi: 10.1017/S1751731118001489
Carvalho, P. D., Souza, A. H., Amundson, M. C., Hackbart, K. S., Fuenzalida, M. J., Herlihy, M. M., et al. (2014). Relationships between fertility and postpartum changes in body condition and body weight in lactating dairy cows. J. Dairy Sci. 97, 3666–3683. doi: 10.3168/jds.2013-7809
Casal, A., Garcia-Roche, M., Navajas, E. A., Cassina, A., and Carriquiry, M. (2018). Hepatic mitochondrial function in Hereford steers with divergent residual feed intake phenotypes. J. Anim. Sci. 96, 4431–4443. doi: 10.1093/jas/sky285
Casal, A., Garcia-Roche, M., Navajas, E. A., Cassina, A., and Carriquiry, M. (2019). Differential hepatic oxidative status in steers with divergent residual feed intake phenotype. Animal 14, 78–85. doi: 10.1017/S1751731119001332
Clemmons, B. A., Martino, C., Powers, J. B., Campagna, S. R., Voy, B. H., Donohoe, D. R., et al. (2019). Rumen bacteria and serum metabolites predictive of feed efficiency phenotypes in beef cattle. Sci. Rep. 9:19265. doi: 10.1038/s41598-019-55978-y
Connor, E. E., Hutchison, J. L., Norman, H. D., Olson, K. M., Van Tassell, C. P., Leith, J. M., et al. (2013). Use of residual feed intake in Holsteins during early lactation shows potential to improve feed efficiency through genetic selection. J. Anim. Sci. 91, 3978–3988. doi: 10.2527/jas.2012-5977
Dechow, C. D., Baumrucker, C. R., Bruckmaier, R. M., and Blum, J. W. (2017). Blood plasma traits associated with genetic merit for feed utilization in Holstein cows. J. Dairy Sci. 100, 8232–8238. doi: 10.3168/jds.2016-12502
Dong, L. F., Yan, T., Ferris, C. P., and McDowell, D. A. (2015). Comparison of maintenance energy requirement and energetic efficiency between lactating Holstein-Friesian and other groups of dairy cows. J. Dairy Sci. 98, 1136–1144. doi: 10.3168/jds.2014-8629
Drackley, J. K. (1999). Biology of dairy cows during the transition period: the final frontier? J. Dairy Sci. 82, 2259–2273. doi: 10.3168/jds.S0022-0302(99)75474-3
Elanco Animal Health (2009). The 5-Point Body Condition Scoring System. Bulletin AI 10752. Greenfield, IN: Elanco Animal Health.
Ellis, J. L., Qiao, F., and Cant, J. P. (2006). Evaluation of net energy expenditures of dairy cows according to body weight changes over a full lactation. J. Dairy Sci. 89, 1546–1557. doi: 10.3168/jds.S0022-0302(06)72222-6
Fischer, A., Delagarde, R., and Faverdin, P. (2018). Identification of biological traits associated with differences in residual energy intake among lactating Holstein cows. J. Dairy Sci. 101, 4193–4211. doi: 10.3168/jds.2017-12636
Fitzsimons, C., Kenny, D. A., and McGee, M. (2014). Visceral organ weights, digestion and carcass characteristics of beef bulls differing in residual feed intake offered a high concentrate diet. Anim. 8, 949–959. doi: 10.1017/S1751731114000652
Gochman, N., and Schmitz, J. M. (1972). Application of a new peroxide indicator reaction to the specific automated determination of glucose with glucose oxidase. Clin. Chem. 18, 943–950. doi: 10.1093/clinchem/18.9.943
Greco, L. F. (2014). Effects of Dietary Polyunsaturated Fatty Acids on Lactation Performance, Tissue Gene Expression, and Reproduction in Dairy Cows. (PhD Dissertation), Department of Animal Sciences, University of Florida, Gainesville, FL, United States, 270.
Guinguina, A., Yan, T., Lund, P., Bayat, A. R., Hellwing, A. L. F., and Huhtanen, P. (2020). Between-cow variation in the components of feed efficiency. J. Dairy Sci. 103, 7968–7982. doi: 10.3168/jds.2020-18257
Johnson, M. M., and Peters, J. P. (1993). Technical note: an improved method to quantify nonesterified fatty acids in bovine plasma. J. Anim. Sci. 71, 753–756. doi: 10.2527/1993.713753x
Kolath, W. H., Kerley, M. S., Golden, J. W., and Keister, D. H. (2006). The relationship between mitochondrial function and residual feed intake in steers. J. Anim. Sci. 84, 861–865. doi: 10.2527/2006.844861x
Li, B., VanRaden, P. M., Guduk, E., O'Connell, J. R., Null, D. J., Connor, E. E., et al. (2020). Genomic prediction of residual feed intake in US Holstein dairy cattle. J. Dairy Sci. 103, 2477–2486. doi: 10.3168/jds.2019-17332
Lobo, R. R., Arce-Cordero, J. A., Marinho, M. N., So, S., Ravelo, A., Agustinho, B. C., et al. (2021). Effects of Mg sources and buffer inclusion on high-producing dairy cows' performance. J. Dairy Sci. 104(Suppl.1):21. doi: 10.3168/jds.2020-20020
Macdonald, K. A., Thomson, B. P., and Waghorn, G. C. (2016). Divergence for residual feed intake of Holstein-Friesian cattle during growth did not affect production and reproduction during lactation. J. Anim. 10, 1890–1898. doi: 10.1017/S1751731116000641
Mäntysaari, P., Liinamo, A-E., and Mäntysaari, E. A. (2012). Energy efficiency and its relationship with milk, body, and intake traits and energy status among primiparous Nordic Red dairy cattle. J. Dairy Sci. 95, 3200–3211. doi: 10.3168/jds.2011-4685
Martin, M. J., Weigel, K. A., and White, H. M. (2021). Assessment of the relationship between postpartum health and mid-lactation performance, behavior, and feed efficiency in Holstein dairy cows. Animal 11:1385. doi: 10.3390/ani11051385
Martinez, N, Rodney, R. M., Block, E., Hernandez, L. L., Nelson, C. D., Lean, I. J., et al. (2018a). Effects of prepartum dietary cation-anion difference and source of vitamin D in dairy cows: health and reproductive responses. J. Dairy Sci. 101, 2563–2578. doi: 10.3168/jds.2017-13740
Martinez, N, Rodney, R. M., Block, E., Hernandez, L. L., Nelson, C. D., Lean, I. J., et al. (2018b). Effects of prepartum dietary cation-anion difference and source of vitamin D in dairy cows: Lactation performance and energy metabolism J. Dairy Sci. 101, 2544–2562. doi: 10.3168/jds.2017-13739
Meale, S. J., Morgavi, D. P., Cassar-Malek, I., Andueza, D., Ortigues-Marty, I., Robins, R. J., et al. (2017). Exploration of biological markers of feed efficiency in young bulls. J. Agric. Food Chem. 65, 9817–9827. doi: 10.1021/acs.jafc.7b03503
Moraes, L. E., Kebreab, E., Strathe, A. B., Dijkstra, J., France, J., Casper, D. P., et al. (2015). Multivariate and univariate analysis of energy balance data from lactating dairy cows. J. Dairy Sci. 98, 4012–4029. doi: 10.3168/jds.2014-8995
Moreira, F., Orlandi, C., Risco, C. A., Mattos, R., Lopes, F., and Thatcher, W. W. (2001). Effects of presynchronization and bovine somatotropin on pregnancy rates to a timed artificial insemination protocol in lactating dairy cows. J. Dairy Sci. 84, 1646–1659. doi: 10.3168/jds.S0022-0302(01)74600-0
Nehme Marinho, M., Perdomo, M. C., Simões, B. S., Husnain, A., Arshard, U., Figueiredo, C. C., et al. (2022). Lactation performance in dairy cows supplemented with microbial additives. J. Dairy Sci. 105 (Suppl. 1): Abstr.
Nehme Marinho, M., Zimpel, R., Peñagaricano, F., and Santos, J. E. P. (2021). Assessing feed efficiency in early and mid lactation and its associations with performance and health in Holstein cows. J. Dairy Sci. 104, 5493–5507. doi: 10.3168/jds.2020-19652
Oyebade, A. O. (2021). Effects of Bacterial Direct-Fed Microbial Supplementation on Rumen Fermentation, Rumen and Plasma Metabolome, and Performance of Lactating Dairy Cows. (PhD Dissertation), Department of Animal Sciences, University of Florida, Gainesville, FL, United States, 186.
Pursley, J. R., Mee, M. O., and Wiltbank, M. C. (1995). Synchronization of ovulation in dairy cows using PGF2alpha and GnRH. Theriogenology 44, 915–923. doi: 10.1016/0093-691X(95)00279-H
Randel, R. D., and Welsh, T. H. Jr (2013). Joint Alpharma-beef species symposium: interactions of feed efficiency with beef heifer reproductive development. J. Anim. Sci. 91, 1323–1328. doi: 10.2527/jas.2012-5679
Ribeiro, E. S., Gomes, G., Greco, L. F., Cerri, R. L. A., Vieira-Neto, A., Monteiro, P. L. J. Jr, et al. (2016). Carryover effect of postpartum inflammatory diseases on developmental biology and fertility in lactating dairy cows. J. Dairy Sci. 99, 2201–2220. doi: 10.3168/jds.2015-10337
Richardson, E. C., and Herd, R. M. (2004). Biological basis for variation in residual feed intake in beef cattle. 2. Synthesis of results following divergent selection. Aust. J. Exp. Agric. 44, 431–440. doi: 10.1071/EA02221
Sánchez, J. M., Keogh, K., Kelly, A. K., Byrne, C. J., Lonergan, P., and Kenny, D. A. (2021). A high plane of nutrition during early life alters the hypothalamic transcriptome of heifer calves. Sci. Rep. 11:13978. doi: 10.1038/s41598-021-93080-4
Santos, J. E. P., Bisinotto, R. S., and Ribeiro, E. S. (2016). Mechanisms underlying reduced fertility in anovular dairy cows. Theriogenology 86, 254–262. doi: 10.1016/j.theriogenology.2016.04.038
Santos, J. E. P., Bisinotto, R. S., Ribeiro, E. S., Lima, F. S., Greco, L. F., Staples, C. R., et al. (2010a). Applying nutrition and physiology to improve reproduction in dairy cattle. Soc. Reprod. Fertil. Suppl. 67, 387–403 doi: 10.7313/UPO9781907284991.030
Santos, J. E. P., Narciso, C. D., Rivera, F., Thatcher, W. W., and Chebel, R. C. (2010b). Effect of reducing the period of follicle dominance in a timed artificial insemination protocol on reproduction of dairy cows. J. Dairy Sci. 93, 2976–2988. doi: 10.3168/jds.2009-2870
Schneider, J. E. (2004). Energy balance and reproduction. Physiol. Behav. 81, 289–317. doi: 10.1016/j.physbeh.2004.02.007
Seymour, D. J., Cánovas, A., Chud, T. C. S., Cant, J. P., Osborne, V. R., Baes, C. F., et al. (2020). The dynamic behavior of feed efficiency in primiparous dairy cattle. J. Dairy Sci. 103, 1528–1540. doi: 10.3168/jds.2019-17414
Shaffer, K. S., Turk, P., Wagner, W. R., and Felton, E. E. (2011). Residual feed intake, body composition, and fertility in yearling beef heifers. J Anim. Sci. 89, 1028–34. doi: 10.2527/jas.2010-3322
Silva, A. M. C. (2021). Nutrition Strategies to Improve Immunity and Productivity of Nulliparous Dairy Cows. (Master of Science thesis), Department of Animal Sciences, University of Florida, Gainesville, FL, United States, 94.
Souza, A. H., Ayres, H., Ferreira, R. M., and Wiltbank, M. C. (2008). A new presynchronization system (Double-Ovsynch) increases fertility at first postpartum timed AI in lactating dairy cows. Theriogenology 70, 208–215. doi: 10.1016/j.theriogenology.2008.03.014
Tyrrell, H. F., and Moe, P. W. (1975). Effect of intake on digestive efficiency. J. Dairy Sci. 58, 1151–1163. doi: 10.3168/JDS.S0022-0302(75)84694-7
USDA-ERS (2018). Milk Production Costs and Returns per Hundredweight (cwt) Sold, by State. (US Department of Agriculture Economic Research Service). Available online at: https://www.ers.usda.gov/data-products/milk-cost-of-production-estimates/ (accessed July 30, 2020).
VandeHaar, M. J, Armentano, J. E., Weigel, K., Spurlock, D.M., Tempelman, R. J., and Veerkamp, R. (2016). Harnessing the genetics of the modern dairy cow to continue improvements in feed efficiency. J. Dairy Sci. 99, 4941–4954. doi: 10.3168/jds.2015-10352
Veerkamp, R. F. (1998). Selection for economic efficiency of dairy cattle using information on live weight and feed intake: a review. J. Dairy Sci. 81, 1109–1119. doi: 10.3168/jds.S0022-0302(98)75673-5
Xi, Y. M., Wu, F., Yang, Z., Li, L., Han, Z. Y., and Wang, G. L. (2016). Biological mechanisms related to differences in residual feed intake in dairy cows. Animal 10, 1311–1318. doi: 10.1017/S1751731116000343
Zenobi, M. G., Zuniga, J. E., Dias, A. L. G., Nelson, C. D., Driver, J. P., Barton, B. A., et al. (2018). Effects of supplementation with ruminally protected choline on performance of multiparous Holstein cows did not depend upon prepartum caloric intake. J. Dairy Sci. 101, 1088–1110. doi: 10.3168/jds.2017-13327
Zimpel, R., Almeida, K. V., Nehme Marinho, M., Revilla, A. R., Perdomo, M. C., Poindexter, M. B., et al. (2021a). Prepartum level of dietary cation-anion difference fed to nulliparous cows: lactation and reproductive responses J. Dairy Sci. 104, 11699–11714. doi: 10.3168/jds.2021-20485
Zimpel, R., Nehme Marinho, M., Almeida, K. V., Revilla, A. R., Perdomo, M. C., Poindexter, M. B., et al. (2021b). Effect of level of dietary cation-anion difference prepartum in nulliparous cows: Acid-base balance, mineral metabolism, and health responses. J. Dairy Sci. 104, 12580–12599. doi: 10.3168/jds.2021-20486
Keywords: dairy cows, energy balance (EB), feed efficiency (FE), reproduction, residual feed intake (RFI)
Citation: Nehme Marinho M and Santos JEP (2022) Association of Residual Feed Intake With Blood Metabolites and Reproduction in Holstein Cows. Front. Anim. Sci. 3:847574. doi: 10.3389/fanim.2022.847574
Received: 02 January 2022; Accepted: 28 February 2022;
Published: 07 April 2022.
Edited by:
Graham Cliff Lamb, Texas A&M University, United StatesReviewed by:
Pablo Pinedo, Colorado State University, United StatesCopyright © 2022 Nehme Marinho and Santos. This is an open-access article distributed under the terms of the Creative Commons Attribution License (CC BY). The use, distribution or reproduction in other forums is permitted, provided the original author(s) and the copyright owner(s) are credited and that the original publication in this journal is cited, in accordance with accepted academic practice. No use, distribution or reproduction is permitted which does not comply with these terms.
*Correspondence: José E. P. Santos, amVwc2FudG9zQHVmbC5lZHU=
Disclaimer: All claims expressed in this article are solely those of the authors and do not necessarily represent those of their affiliated organizations, or those of the publisher, the editors and the reviewers. Any product that may be evaluated in this article or claim that may be made by its manufacturer is not guaranteed or endorsed by the publisher.
Research integrity at Frontiers
Learn more about the work of our research integrity team to safeguard the quality of each article we publish.