- 1School of Animal Sciences, Virginia Tech, Blacksburg, VA, United States
- 2Department of Animal Sciences, The Ohio State University, Columbus, OH, United States
Winter supplementation of gestating beef cows is often necessary to ensure energy and protein requirements remain satisfied. However, it is difficult to prevent over- or under-consumption by individual animals fed in a group. The objective of this study was to evaluate the intake limiting effects of 3 levels of tea saponin (TS) on pelleted feed consumption when compared with a TS-free control treatment. Commercial beef cows in late gestation (n = 24) were allocated to 1 of 4 treatments delivered via a pelleted feed supplement: 0% (A), 0.16% (B), 0.32% (C), or 0.64% (D) TS on a dry matter basis. Cows were assigned so that initial mean body weights and body condition scores were similar among treatment groups. Supplement was delivered once daily via Calan gates at a rate of 2.5% of BW for 42 days. Refusals were collected daily to calculate intake. Treatment differences were observed for pellet DMI, cow BW, and cow BCS (P < 0.0001). Cow hay intake, calf birth weight, and calf weaning weight were unaffected by treatment (P > 0.05). Dry matter intake of pellets as a percent of BW (DMIBW) was significantly different for all treatments (P < 0.0001) with intake declining as TS content increased. Considerable variability in DMIBW of all treatments was observed from day 0 to 15 but intakes plateaued between 1.75 and 2.5% DMIBW for the remainder of the trial with Treatment D intake remaining noticeably lower than the other treatments. Treatment D was found to be successful at limiting pellet intake to an average DMIBW of 1.51%. This study concluded that short-term pellet intake can be limited by inclusion of TS, highlighting it as a potential intake limiter product for beef cattle producers.
1. Introduction
The impact of bovine maternal nutrient status during gestation on offspring health and performance is well documented (Corah et al., 1975; Greenwood and Cafe, 2007; Larson et al., 2009; Long et al., 2010). Maternal nutrient restriction during late gestation has been associated with lower birth weights and decreased survival rates of calves (LeMaster et al., 2017; Perry et al., 2019). Despite conflicting reports of compensatory preweaning growth in calves that were severely nutrient restricted in utero (Greenwood and Cafe, 2007; López Valiente et al., 2022), nutritional supplementation of dams during late gestation has been demonstrated to increase weaning weights in both steer and heifer calves (Funston et al., 2010). Besides growth, nutrient status of pregnant females may also influence carcass characteristics of progeny, specifically carcass weight, tenderness, and fat deposition (Underwood et al., 2010). In the cow, inadequate prepartum nutrition is known to contribute to longer postpartum intervals and reduced pregnancy rates (D’Occhio et al., 2019). Supplementation is therefore occasionally required to address nutritional deficiencies in gestating females, particularly when they are to be maintained on low quality forage during the winter months. A common challenge for beef producers, however, is delivering a target amount of energy or protein supplement to individual gestating cows. Because group housing is standard, it is difficult to prevent over- or underconsumption of a provided supplement and nearly impossible to ensure that each animal consumes a specific target amount of feed. Fortunately, the use of an intake limiter can help ensure that each animal consumes only a target amount of supplement relative to its body weight. Common limiters include salt, gypsum, calcium chloride, and phosphoric acid. Although salt is a reasonably safe and inexpensive limiter, utilization of the other compounds can be accompanied by the challenges of sulfur toxicity (gypsum), corrosivity (calcium chloride), and expensive handling requirements (phosphoric acid) (Kunkle et al., 2000).
Saponins are bitter-tasting compounds found in plants that reduce plant palatability in high enough concentrations (Cheeke, 1996). Structurally, they are glycosides of either steroidal or triterpenoid aglycones with variable numbers of sugar side chains (Foerster, 2006). To date, the effects of saponins on feed intake and dietary preferences of ruminants have received little scientific attention. Applications of saponins in ruminant nutrition have focused instead on their antimicrobial properties and abilities to optimize rumen fermentation and enhance nutrient utilization (Patra and Saxena, 2009; McMurphy et al., 2014b; Liu et al., 2019). The central objective of this study was therefore to evaluate the intake limiting effects of 3 levels of tea saponin (TS), a triterpenoid saponin isolated from Camellia sinensis (the tea plant), on pelleted supplement consumption compared with a TS-free control supplement. Secondary objectives were to evaluate the effects of differing levels of limiter treatment on cow BW, cow BCS, forage intake, calf birth weight, and calf weaning weight.
2. Materials and methods
2.1. Animals and study design
All procedures in this study were approved by the Virginia Tech Institutional Animal Care and Use Committee (Protocol #17-164). Twenty-four commercial Angus beef cows (BW = 537 ± 33 kg) were maintained in a bare 1.33 ha pasture at Virginia Tech Kentland Farm, Blacksburg, VA. All cows were 210 days pregnant to timed artificial insemination relative to trial commencement. Cows were stratified by initial BW and BCS then randomly assigned to 1 of 4 supplemental treatments so that each group contained 6 cows. Treatments (Table 1) were custom grain pellets manufactured by Cooperative Research Farms (North Chesterfield, VA) containing varying levels of TS: 0% (A), 0.16% (B), 0.32% (C), or 0.64% (D). Treatments were delivered to cows daily via the Calan Broadbent Feeding System (American Calan Inc., Northwood, NH) at a rate of 2.5% of BW in DM for 42 days (November 29 to January 9). Calan feeders were mounted onto 2 covered flatbed trailers with 6 feeders on each side. Trailers were parked in a 13 × 25 m lot adjacent to the pasture and cows had 24 h access to the Calan feeders. Prior to day 0, cows were given a 12-day adaptation period to become accustomed to the Calan feeding system. First-cutting grass hay (Table 2), minerals, and fresh water were available ad libitum.
2.2. Data collection
Refusals were collected and weighed each day at 0800 h to calculate pellet DMI, after which the daily allotment of supplement was weighed and delivered. Cows were weighed on days 0, 14, 28, and 42 using a digital scale (Tru-Test ID5000, Carbine Aginvest, Auckland, New Zealand) located under a squeeze chute. Cows were body condition scored immediately after weighing using a scale of 1 to 9, with 1 being emaciated and 9 being obese. Calves were born approximately 30 days after trial completion and calf birth weights were taken within 24 h of birth. Calves were weaned and weighed at 6 months of age. Titanium dioxide was utilized as an external marker for estimation of forage DMI and was added to the pellets during milling at a concentration of 200 g/T. A fecal sample (approximately 100 g) was collected rectally from each cow every 6 h starting on day 38 for a total of 9 collection times evenly distributed over the day. Fecal samples were dried for 36 h at 55°C in a forced-air oven (Thermo Scientific Heratherm Advanced Protocol Oven Model 51028115, Fisher Scientific, Waltham, MA) and ground to pass through a 1 mm screen of a Wiley mill (Model 4, Thomas Scientific, Swedesboro, NJ). Samples were pooled by animal, ashed in a muffle furnace (Sybron Thermolyne FA1730, Fisher Scientific, Waltham, MA) for 12 h at 500°C, and digested in concentrated sulfuric acid. Titanium concentrations were determined on an inductively coupled plasma atomic emission spectrometer (SPECTRO ARCOS SOP, SPECTRO Analytical Instruments, Inc., Kleve, Germany). Estimated hay DMI was calculated following the method of de Souza et al. (2015).
2.3. Nutrient analysis
Nutrient composition data of treatment pellets and grassy hay is given in Table 2. One sample of each treatment pellet was collected at the end of the study. Hay samples were collected twice weekly and pooled to create a representative sample. Pellet and hay samples were dried at 55°C in a forced-air oven (Thermo Scientific Heratherm Advanced Protocol Ovens Model 51028115, Fisher Scientific, Waltham, MA) for 24 h and ground to pass through a 1 mm screen of a Wiley mill (Model 4, Thomas Scientific, Swedesboro, NJ). Dry matter percentage was determined by drying for 12 h at 100°C. Ash percent was assessed through combustion in a muffle furnace (Sybron Thermolyne FA1730, Fisher Scientific, Waltham, MA) for 12 h at 500°C. Neutral detergent fiber and ADF concentrations were determined using the Ankom200 Fiber Analyzer (Ankom Technology, Macedon, NY). Alpha-amylase from Bacillus licheniformis (Thermostable Amylase HTL, BIO-CAT, Troy, VA) and sodium sulfite (Sodium Sulfite, Anhydrous, Fisher Scientific, Waltham, MA) were utilized in the NDF analysis (Van Soest et al., 1991). Acid detergent lignin content was obtained by agitating ADF residues in 72% sulfuric acid on a rocking platform (Flask Dancer, Boekel Scientific, Feasterville-Trevose, PA) for 3 h (AOAC, 2000). Samples were combusted using a Vario El Cube CN analyzer (Elementar Americas Inc., Mount Laurel, NJ) to measure N content. Crude protein concentration was then calculated as %N × 6.25. Starch content was assessed following the acetate buffer method described by Hall (2015) with α-amylase and amyloglucosidase from Aspergillus niger (E-AMGDF, Megazyme International, Wicklow, Ireland). In vitro dry matter digestibility (DMD) was performed on each feed sample by Cumberland Valley Analytical Services (Waynesboro, PA).
2.4. Statistical analysis
All data were analyzed in R version 4.2.1 (R Core Team, 2022) using a linear mixed-effects model and the nlme package (Pinheiro et al., 2013). Response variables included pellet DMI, pellet DMI as a percent of BW (DMIBW), estimated forage DMI, cow BW, cow BCS, calf birth weight, and calf weaning weight. Treatment was used as a fixed effect while cow and Calan feeder trailer (1 of 2) were included as random effects. A 1st order autoregressive residual error variance structure was used for each of the response variables. Analysis of variance was performed on each model and least square means calculated. Significance was considered when P < 0.05 and a tendency when 0.05 ≤ P < 0.10.
3. Results
Least square means and standard errors for each treatment are given in Supplementary Table S1. P-values for contrasts between treatment means are given in Supplementary Table S2. Pellet DMI was affected by TS concentration, with cows on the highest limiter percentage (0.64%) consuming 3.3 kg less pellet on average than those receiving the control with no limiter (Figure 1A). Pellet DMI of the lowest TS percentage (0.16%) tended (P = 0.066) to be lower than the control pellet DMI. All TS concentrations resulted in significantly different pellet DMIBW compared to the control (P < 0.012; Figure 1B), with pellet DMIBW decreasing with increasing TS concentration (Figure 2). With a mean pellet DMIBW of 1.51%, Treatment D (0.64% TS) resulted in the lowest average pellet intake as a percent of body weight (Figure 2). Estimated grass hay intake was similar (P > 0.81) between the treatment groups (Figure 3).
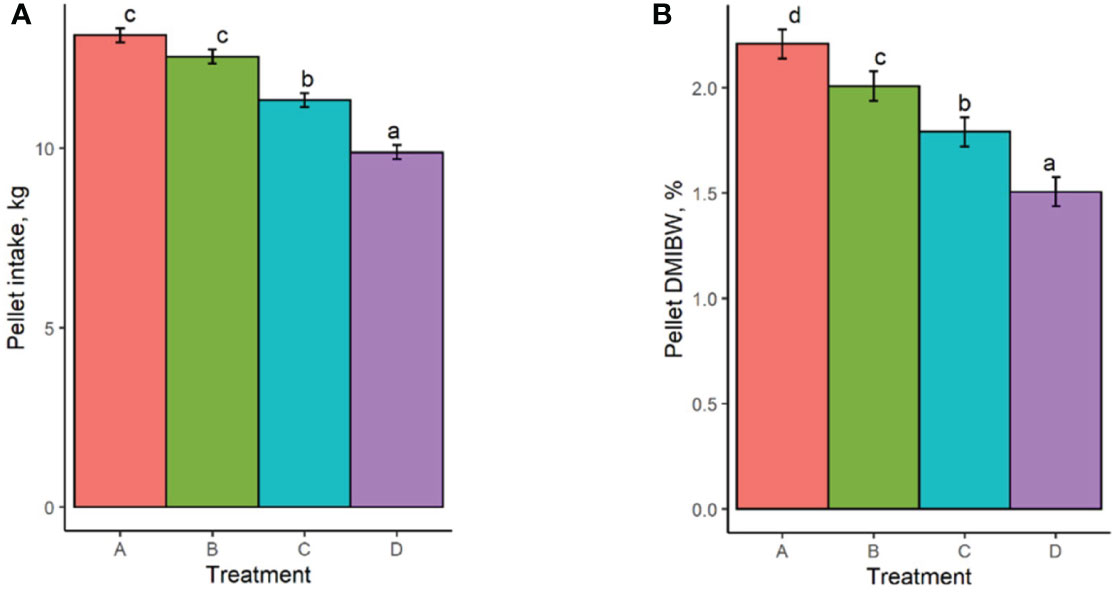
Figure 1 (A) Pellet dry matter intake of treatment groups. (B) Pellet dry matter intake as a percent of body weight. Treatment A contained 0.00% tea saponin (TS), B contained 0.16% TS, C contained 0.32% TS, and D contained 0.64% TS. Means bearing different letters differ significantly (P < 0.05).
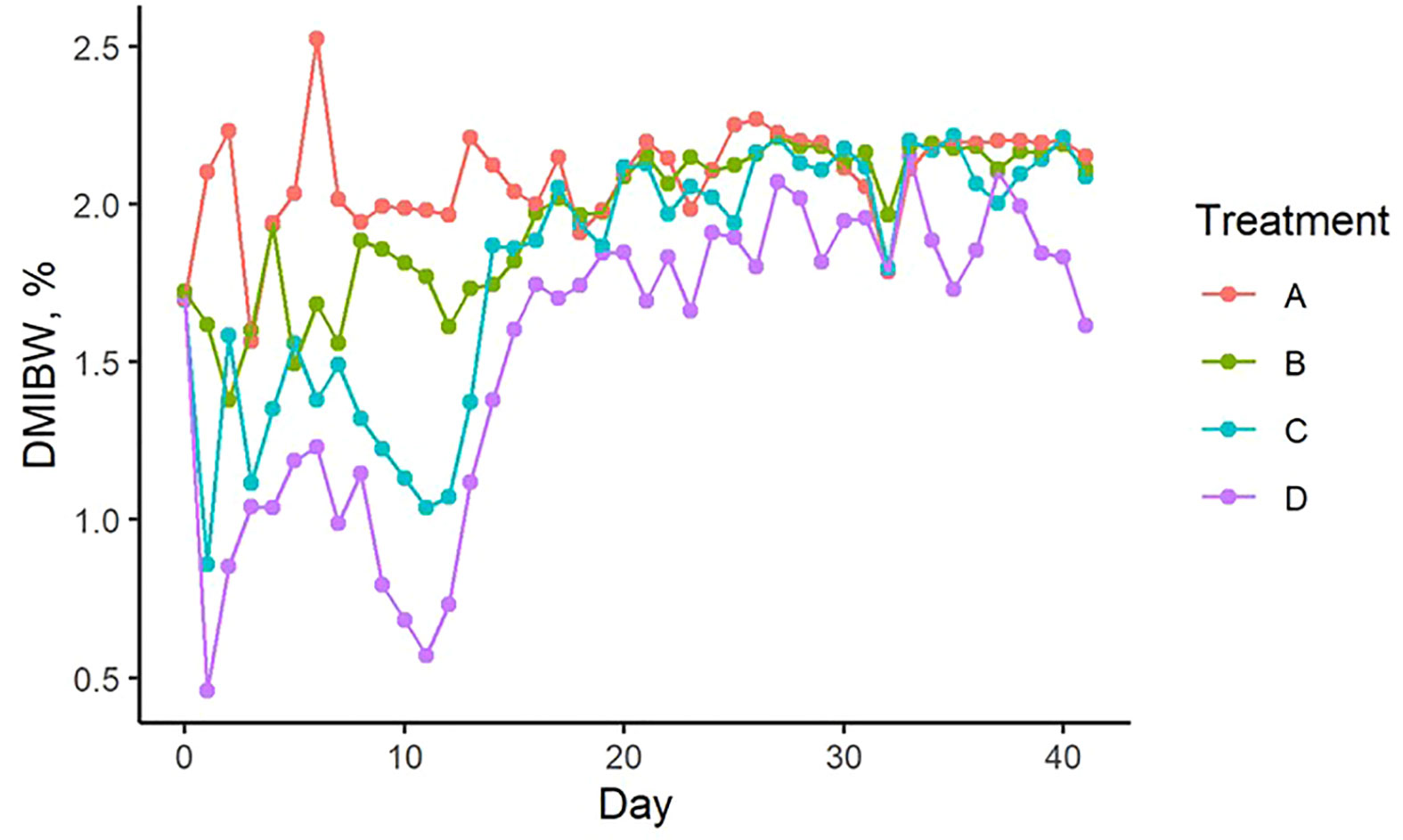
Figure 2 Change in pellet dry matter intake as a percent of body weight over time from experimental day 0 to day 41 (corresponding to day 210 to day 251 of gestation, respectively). Treatment A contained 0.00% tea saponin (TS), B contained 0.16% TS, C contained 0.32% TS, and D contained 0.64% TS.
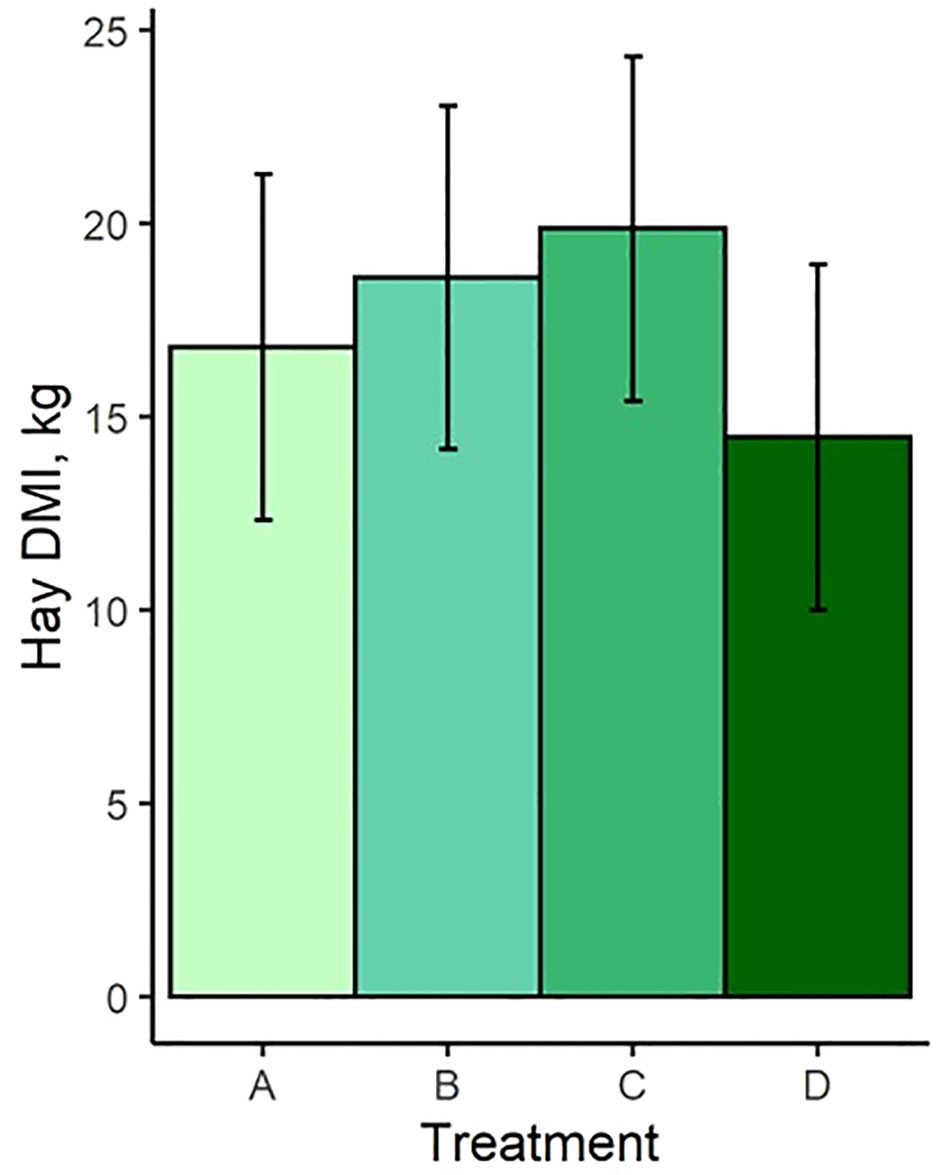
Figure 3 Hay dry matter intake of treatment groups. Treatment A contained 0.00% tea saponin (TS), B contained 0.16% TS, C contained 0.32% TS, and D contained 0.64% TS.
Both mean cow BW and BCS increased with increasing TS concentration (Figure 4A; Figure 5A). Mean BW differed (P < 0.0006; Figure 4A) between all treatment groups with the exception of groups B and C (P = 0.23). Similarly, Mean BCS differed (P < 0.009; Figure 5A) between all treatment groups with the exception of groups B and C (P = 0.92). Initial cow BW (Figure 4B) and initial cow BCS (Figure 5B) were not different between groups (P > 0.93 and P > 0.82, respectively). Final cow BW was different (P < 0.0001; Figure 4C) between groups except for groups B and C (P = 0.99). Final cow BCS was different for all treatment groups (P < 0.0002; Figure 5C). Calf birth weights (Figure 6A) were not different between treatments (P > 0.19). Calf weaning weights (Figure 6B) were also similar between treatments (P > 0.33).
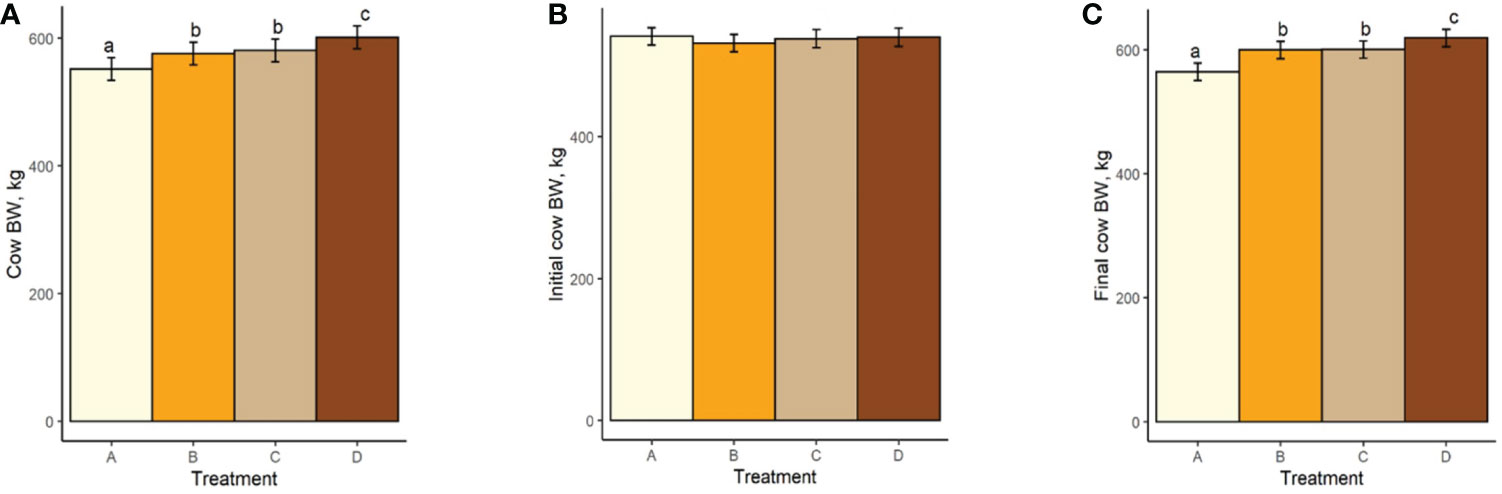
Figure 4 (A) Mean cow body weight of treatment groups. (B) Initial cow body weight. (C) Final cow body weight. Treatment A contained 0.00% tea saponin (TS), B contained 0.16% TS, C contained 0.32% TS, and D contained 0.64% TS. Means bearing different letters differ significantly (P < 0.05).
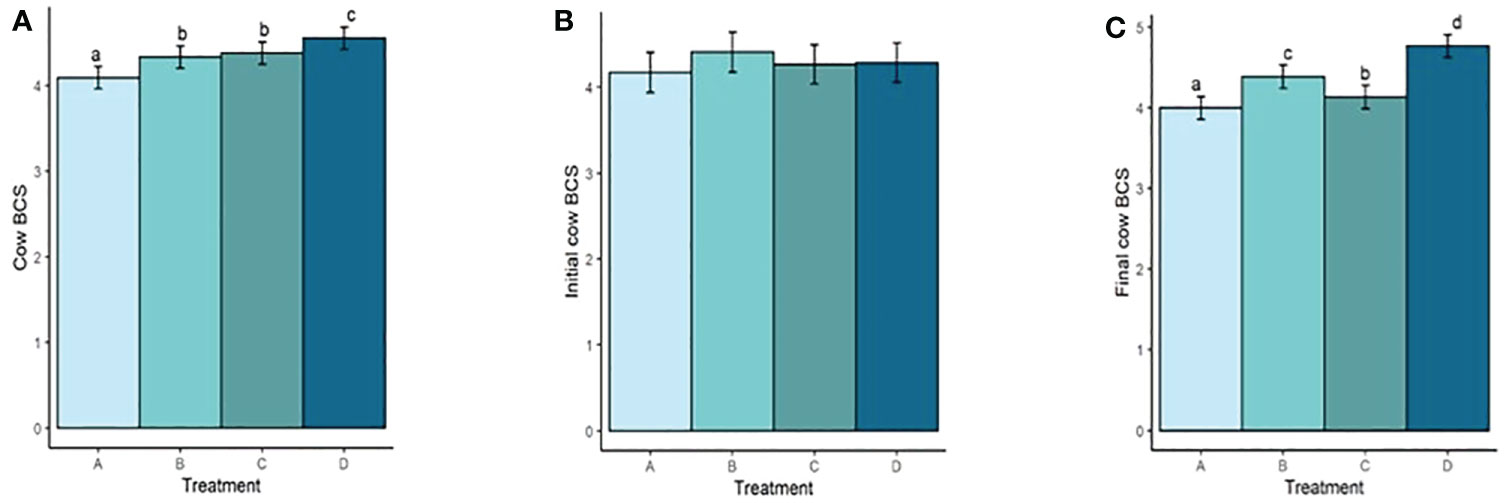
Figure 5 (A) Mean cow BCS of treatment groups. (B) Initial cow BCS. (C) Final cow BCS. Treatment A contained 0.00% tea saponin (TS), B contained 0.16% TS, C contained 0.32% TS, and D contained 0.64% TS. Means bearing different letters differ significantly (P < 0.05).
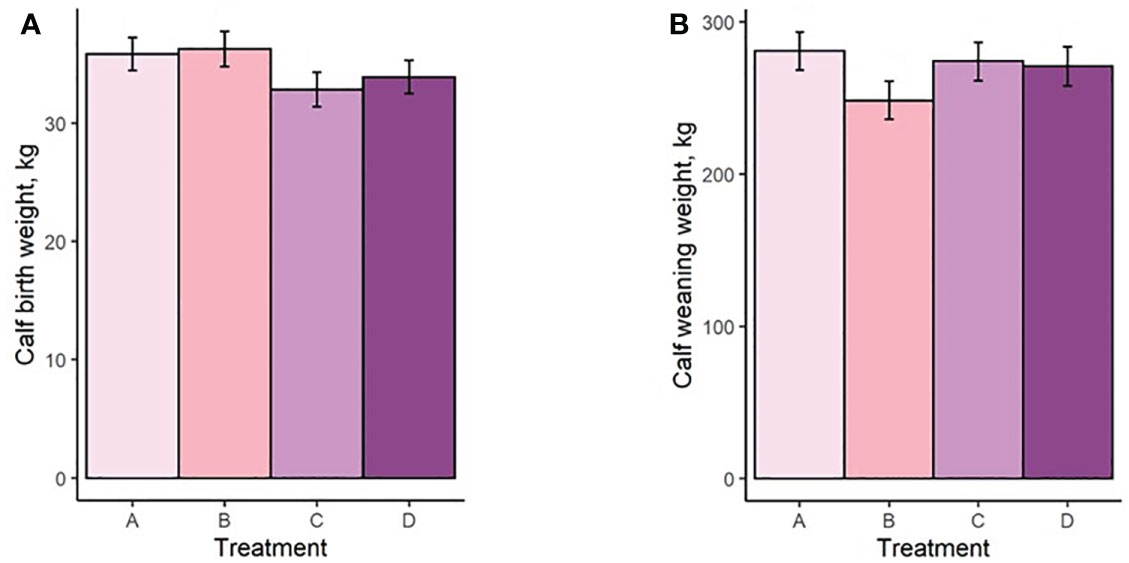
Figure 6 (A) Calf birth weight of treatment groups. (B) Calf weaning weight of treatment groups. Treatment A contained 0.00% tea saponin (TS), B contained 0.16% TS, C contained 0.32% TS, and D contained 0.64% TS.
4. Discussion
4.1. Pellet and forage intake
As anticipated, TS concentration influenced pellet DMI in that cows receiving the greatest TS percentage consumed less pellet on average than those receiving no TS treatment. All groups receiving the TS treatment had significantly different pellet DMIBW compared to the control and to each other, demonstrating the ability of TS to function effectively as a limiter and to exert greater limiting power over intake as its dosage is increased. Cows receiving Treatment D (0.64% TS) consumed an average of 1.51% of BW in pellet and were most successfully limited by this concentration. Interestingly, estimated grass hay intake did not vary between the treatment groups despite the differences observed in pellet intake. This was consistent with the observations of McMurphy et al. (2014a), who noted no effect of the saponin from Yucca schidigera (known as sarsaponin or Micro-Aid commercially) on hay DMI in spring-calving beef cows. This indicates that cows restricted from high pellet consumption due to the limiter’s effects did not compensate by increasing their hay intake. Further discussion of this effect is presented in Section 4.4 below.
Few reports of saponin effect on feed intake in cattle are present in the literature, and no published studies utilizing saponin sourced from Camellia sinensis in beef brood cows appear to exist. In dairy cows, however, TMR intake has been shown to decrease in response to inclusion of sarsaponin in a number of studies (Wu et al., 1994; Lovett et al., 2006; Benchaar et al., 2008). Reported sarsaponin concentrations were similar to or lower than those used in the present study: 0.31% (Lovett et al., 2006), 0.28% (Benchaar et al., 2008), and 0.007% (Wu et al., 1994). McMurphy et al. (2014b) reported that administration of sarsaponin to beef steers fed a protein supplement and low quality hay did not affect DMI (P = 0.40). However, the sarsaponin dosages administered were 1 and 2 g/d, which may have been insufficient to stimulate a significant intake response in these animals. Our pellet intake results therefore appear to be consistent with previous work where comparable saponin levels were utilized.
4.2. Limiter efficacy over time
A considerable degree of variation in pellet DMIBW was noted for all treatments from day 0 to 15 of the trial (Figure 2). This large degree of variation may have been due to the cows responding to the presence of the limiter and becoming accustomed to its effects, which can include gastrointestinal irritation (Wen et al., 2015). An instance of initial overeating may have led to internal discomfort, prompting the cow to decrease pellet intake the following day before increasing consumption again when the effects subsided, and potentially explains the intake variability observed during the beginning of the trial. After day 15, mean pellet intakes on all treatments plateaued between 1.75 and 2.5% DMIBW for the rest of the trial. This loss of limiter efficacy was likely the result of the cows developing a tolerance to the TS treatment. Cattle are also known to increase their intake of salt limiters over time, prompting the need to increase the limiter dosage to maintain intakes at desirable levels (Kunkle et al., 2000). Successful long-term applications of saponin limiters will likely require dosage increases as well. Although also increasing from low levels initially, mean pellet DMIBW of Treatment D remained visibly lower compared to the other 3 treatments and averaged 1.51% DMIBW across time, indicating that a concentration of 0.64% TS is likely sufficient to limit intake for short feeding periods.
4.3. Body weight, body condition score, calf birth weight, and calf weaning weight
Both mean cow BW and BCS increased with increasing TS concentration. Mean BW on all TS treatments were significantly different from the control and from each other, with the exception of B and C. These significant differences were also observed for BCS. Calf birth weights were not different between treatments so did not likely contribute to the differences in cow BW. Although reports on saponin effects on weight change and body condition in adult cows are scarce, our BW and BCS results are consistent with those of McMurphy et al. (2014a), who also noted BW and BCS increases in gestating, spring-calving cows (Angus and Angus × Hereford) in response to sarsaponin supplementation. The increased level of condition (and by extension BW) seen in our cows receiving higher TS concentrations may have been partially attributed to enhanced fermentation and digestibility, and therefore greater energy uptake by these animals. This could not be confirmed, however, because the cows were not cannulated and total-tract digestibility was not assessed, being outside the scope of the experiment. The higher degree of body fat observed in high-limiter cows may also have contributed to the decrease in feed consumption through the intake regulatory effects of released leptin or unsaturated fatty acids (NRC, 2016). Although mean BCS scores were higher for the cows receiving higher limiter dosages, they are still considered suboptimal (<5; Figure 5). Decreased palatability of the TS-containing diets may therefore have been a larger contributor to the lower pellet DMI observed. McMurphy et al. (2014a) also reported no treatment effect on calf birth weights or weaning weights, in agreement with our findings.
Further comparisons with the literature are challenging due to the deficiency of beef brood cow data; however, a number of studies report similar findings with saponin applications in other ruminant models. Mader and Brumm (1987), for instance, observed both an increase in ADG and feed efficiency in growing crossbred beef steers supplemented with sarsaponin. Greater ADG was also reported in sheep by several studies where saponins from Enterolobium cyclocarpum (Leng et al., 1992; Navas-Camacho et al., 1993) or Sapindus saponaria (Hess et al., 2004) were utilized. As mentioned, saponins have been studied in cattle for their desirable manipulations of rumen function. Their major action in the rumen is depopulation of protozoa (Patra and Saxena, 2009). This defaunation results in a decrease in bacterial proteolysis, which improves N conservation and increases efficiency of microbial protein synthesis (McMurphy et al., 2014b). There is evidence that saponins impose selective pressure on bacterial populations and archaeal activities as well (Hess et al., 2003; Guo et al., 2008; Wang et al., 2019). Methanogens have demonstrated decreases in methane production, but not always in total numbers, in response to saponin administration (Hess et al., 2003). This may be at least partially attributed to the defaunating effect of the treatment, which would remove protozoal support of methane production. Tea saponin has also been shown to reduce methyl coenzyme‐M reductase subunit A gene expression and methane production in cultured rumen methanogens (Guo et al., 2008). Wang et al. (2000) noted that sarsaponin negatively impacted cellulolytic bacterial populations but did not affect amylolytic bacteria, indicating a useful application of saponin in high concentrate diets. In agreement with this finding, a number of studies report lower ruminal acetate:propionate ratios on saponin treatments of varying sources (Hristov et al., 1999; Abreu et al., 2004; Hess et al., 2004; Santoso et al., 2007). Dietary inclusion of saponins has also been observed to improve rumen degradability of DM and NDF (attributed to a slower particulate passage rate) as well as increase microbial protein flow to the small intestine (McMurphy et al., 2014b). Taken together, these various effects on the rumen environment may collectively promote efficiency of energy and nitrogen usage through the favoring of metabolically efficient pathways, activities, and participants. Our results appear to reflect this logic because, despite consuming less pelleted supplement and a similar amount of hay, cows on higher TS treatments were able to maintain a more desirable BCS with no difference in calf birth weights. The inclusion of tea saponin in the diets of beef cattle may therefore provide a number of metabolic, production, and environmental benefits beyond simply limiting intake. However, further research is necessary to confirm this hypothesis.
4.4. Conclusions
This study offered a novel demonstration of the intake-limiting effects of tea saponin in gestating beef brood cows consuming grain pellets as well as its impact on forage consumption, body weight, and body condition. A saponin concentration of 0.64% limited mean supplement intake to 1.51% of cow body weight with no effect on calf birth weight or weaning weight, underscoring the potential value of tea saponin as a commercial product for beef cattle producers. The observation of increased tolerance to saponin administration prompts further investigation of strategies to maintain a target supplement intake long-term, likely involving an increase in limiter dosage over time. Additional research is also required to evaluate the role of tea saponin as an optimizer of rumen function and nutrient utilization as our observations indicate tea saponin may be a valuable tool to enhance feed efficiency.
Data availability statement
The raw data supporting the conclusions of this article will be made available by the authors, without undue reservation.
Ethics statement
The animal study was reviewed and approved by the Virginia Tech Institutional Animal Care and Use Committee.
Author contributions
CG was responsible for animal monitoring, sample collection, data analysis, and manuscript preparation. TW assisted with study design and contributed to manuscript editing. VM assisted with study design and contributed to manuscript editing. RW served as principal investigator, determined study design, guided data analysis, and contributed to manuscript editing. All authors contributed to the article and approved the submitted version.
Funding
This research was supported by funding from Cooperative Research Farms (North Chesterfield, VA). Publication of this manuscript was supported by Virginia Tech's Open Access Subvention Fund.
Acknowledgments
The authors would like to thank Dr. Kevin Herkelman for his assistance with study coordination.
Conflict of interest
The authors declare that the research was conducted in the absence of any commercial or financial relationships that could be constructed as a potential conflict of interest.
Cooperative Research Farms provided the test product (limiter pellets) and funded the research but did not participate in the collection, handling, analysis, or interpretation of any data.
Publisher’s note
All claims expressed in this article are solely those of the authors and do not necessarily represent those of their affiliated organizations, or those of the publisher, the editors and the reviewers. Any product that may be evaluated in this article, or claim that may be made by its manufacturer, is not guaranteed or endorsed by the publisher.
Supplementary material
The Supplementary Material for this article can be found online at: https://www.frontiersin.org/articles/10.3389/fanim.2022.1080115/full#supplementary-material
References
AOAC (2000). Official methods of analysis. 17th ed. Arlington, VA: Association of Official Analytical Chemists
Abreu A., Carulla J. E., Lascano C. E., Diaz T. E., Kreuzer M., Hess H.-D. (2004). Effects of sapindus saponaria fruits on ruminal fermentation and duodenal nitrogen flow of sheep fed a tropical grass diet with and without legume. J. Anim. Sci. 82, 1392–1400. doi: 10.2527/2004.8251392x
Benchaar C., McAllister T. A., Chouinard P. Y. (2008). Digestion, ruminal fermentation, ciliate protozoal populations, and milk production from dairy cows fed cinnamaldehyde, quebracho condensed tannin, or yucca schidigera saponin extracts. J. Dairy Sci. 91, 4765–4777. doi: 10.3168/jds.2008-1338
Cheeke P. R. (1996). “Biological effects of feed and forage saponins and their impacts on animal production,” in Saponins used in food and agriculture (New York, NY: Springer), 377–385.
Corah L. R., Dunn T. G., Kaltenbach C. C. (1975). Influence of prepartum nutrition on the reproductive performance of beef females and the performance of their progeny. J. Anim. Sci. 41, 819–824. doi: 10.2527/jas1975.413819x
de Souza J., Batistel F., Welter K. C., Silva M. M., Costa D. F., Portela Santos F. A. (2015). Evaluation of external markers to estimate fecal excretion, intake, and digestibility in dairy cows. Trop. Anim. Health Prod. 47, 265–268. doi: 10.1007/s11250-014-0674-6
D’Occhio M. J., Baruselli P. S., Campanile G. (2019). Influence of nutrition, body condition, and metabolic status on reproduction in female beef cattle: A review. Theriogenology 125, 277–284. doi: 10.1016/j.theriogenology.2018.11.010
Funston R. N., Larson D. M., Vonnahme K. A. (2010). Effects of maternal nutrition on conceptus growth and offspring performance: Implications for beef cattle production. J. Anim. Sci. 88, E205–E215. doi: 10.2527/jas.2009-2351
Greenwood P. L., Cafe L. M. (2007). Prenatal and pre-weaning growth and nutrition of cattle: long-term consequences for beef production. Animal 1, 1283–1296. doi: 10.1017/S175173110700050X
Guo Y. Q., Liu J.-X., Lu Y., Zhu W. Y., Denman S. E., McSweeney C. S. (2008). Effect of tea saponin on methanogenesis, microbial community structure and expression of mcrA gene, in cultures of rumen micro-organisms. Lett. Appl. Microbiol. 47, 421–426. doi: 10.1111/j.1472-765X.2008.02459.x
Hall M. B. (2015). Determination of dietary starch in animal feeds and pet food by an enzymatic-colorimetric method: Collaborative study. J. AOAC Int. 98, 397–409. doi: 10.5740/jaoacint.15-012
Hess H.-D., Beuret R. A., Lötscher M., Hindrichsen I. K., Machmüller A., Carulla J. E., et al. (2004). Ruminal fermentation, methanogenesis and nitrogen utilization of sheep receiving tropical grass hay-concentrate diets offered with sapindus saponaria fruits and cratylia argentea foliage. Anim. Sci. 79, 177–189. doi: 10.1017/S1357729800054643
Hess H.-D., Monsalve L. M., Lascano C. E., Carulla J. E., Diaz T. E., Kreuzer M. (2003). Supplementation of a tropical grass diet with forage legumes and sapindus saponaria fruits: Effects on in vitro ruminal nitrogen turnover and methanogenesis. Aust. J. Agric. Res. 54, 703–713. doi: 10.1071/AR02241
Hristov A. N., McAllister T. A., Van Herk F. H., Cheng K.-J., Newbold C. J., Cheeke P. R. (1999). Effect of yucca schidigera on ruminal fermentation and nutrient digestion in heifers. J. Anim. Sci. 77, 2554–2563. doi: 10.2527/1999.7792554x
Kunkle W. E., Johns J. T., Poore M. H., Herd D. B. (2000). Designing supplementation programs for beef cattle fed forage-based diets. J. Anim. Sci. 77, 1-11. doi: 10.2527/jas2000.00218812007700ES0012x
Larson D. M., Martin J. L., Adams D. C., Funston R. N. (2009). Winter grazing system and supplementation during late gestation influence performance of beef cows and steer progeny. J. Anim. Sci. 87, 1147–1155. doi: 10.2527/jas.2008-1323
LeMaster C. T., Taylor R. K., Ricks R. E., Long N. M. (2017). The effects of late gestation maternal nutrient restriction with or without protein supplementation on endocrine regulation of newborn and postnatal beef calves. Theriogenology 87, 64–71. doi: 10.1016/j.theriogenology.2016.08.004
Leng R. A., Bird S. H., Klieve A. (1992). “Legume trees and other fodder trees as protein sources for livestock,” in Proceedings of the FAO expert consultation held at the Malaysian agricultural research and development institute 633.3/S742. Eds. Pugliese P.-L., Speedy A. (Kuala Lumpur, Malaysia: FAO).
Liu Y., Ma T., Chen D., Zhang N., Si B., Deng K., et al. (2019). Effects of tea saponin supplementation on nutrient digestibility, methanogenesis, and ruminal microbial flora in dorper crossbred ewe. Animals 9, 29. doi: 10.3390/ani9010029
Long N. M., Prado-Cooper M. J., Krehbiel C. R., DeSilva U., Wettemann R. P. (2010). Effects of nutrient restriction of bovine dams during early gestation on postnatal growth, carcass and organ characteristics, and gene expression in adipose tissue and muscle. J. Anim. Sci. 88, 3251–3261. doi: 10.2527/jas.2009-2512
López Valiente S., Rodriguez A. M., Long N. M., Lacau-Mengido I. M., Maresca S. (2022). The degree of maternal nutrient restriction during late gestation influences the growth and endocrine profiles of offspring from beef cows. Anim. Prod. Sci. 62, 163. doi: 10.1071/AN20527
Lovett D. K., Stack L., Lovell S., Callan J., Flynn B., Hawkins M., et al. (2006). Effect of feeding yucca schidigera extract on performance of lactating dairy cows and ruminal fermentation parameters in steers. Livest. Sci. 102, 23–32. doi: 10.1016/j.livsci.2005.11.005
Mader T. L., Brumm M. C. (1987). Effect of feeding sarsaponin in cattle and swine diets. J. Anim. Sci. 65, 9–15. doi: 10.2527/jas1987.6519
McMurphy C. P., Sexten A. J., Mourer G. L., Rincker M. J., Lalman D. L. (2014a). Effects of including saponins (Micro-aid®) in a protein supplement on performance of growing steers and spring-calving cows. Anim. Feed Sci. Technol. 190, 19–29. doi: 10.1016/j.anifeedsci.2014.01.002
McMurphy C. P., Sexten A. J., Mourer G. L., Sharman E. D., Trojan S. J., Rincker M. J., et al. (2014b). Effects of including saponins (Micro-aid®) on intake, rumen fermentation and digestibility in steers fed low-quality prairie hay. Anim. Feed Sci. Technol. 190, 47–58. doi: 10.1016/j.anifeedsci.2014.01.007
Navas-Camacho A., Laredo M. A., Cuesta A., Anzola H., Leon J. C. (1993). Effect of supplementation with a tree legume forage on rumen function. Livest. Res. Rural Dev. 5, 60–74. Available at: http://www.lrrd.org/lrrd5/2/navas.htm
NRC (2016). Nutrient requirements of beef cattle. 8th ed (Washington, DC: National Academies Press).
Patra A. K., Saxena J. (2009). The effect and mode of action of saponins on the microbial populations and fermentation in the rumen and ruminant production. Nutr. Res. Rev. 22, 204–219. doi: 10.1017/S0954422409990163
Perry V. E. A., Copping K. J., Miguel-Pacheco G., Hernandez- Medrano J. (2019). The effects of developmental programming upon neonatal mortality. Vet. Clin. North Am. Food Anim. Pract. 35, 289–302. doi: 10.1016/j.cvfa.2019.02.002
Pinheiro J., Bates D., DebRoy S., Sarkar D., R Core Team (2013). Nlme: linear and nonlinear mixed effects models. (Vienna, Austria: R Foundation for Statistical Computing)
R Core Team (2022). R: a language and environment for statistical computing. (Vienna, Austria: R Foundation for Statistical Computing)
Santoso B., Kilmaskossu A., Sambodo P. (2007). Effects of saponin from biophytum petersianum klotzsch on ruminal fermentation, microbial protein synthesis and nitrogen utilization in goats. Anim. Feed Sci. Technol. 137, 58–68. doi: 10.1016/j.anifeedsci.2006.10.005
Underwood K. R., Tong J. F., Price P. L., Roberts A. J., Grings E. E., Hess B. W., et al. (2010). Nutrition during mid to late gestation affects growth, adipose tissue deposition, and tenderness in cross-bred beef steers. Meat Sci. 86, 588–593. doi: 10.1016/j.meatsci.2010.04.008
Van Soest P. J., Robertson J. B., Lewis B. A. (1991). Methods for dietary fiber, neutral detergent fiber, and nonstarch polysaccharides in relation to animal nutrition. J. Dairy Sci. 74, 3583–3597. doi: 10.3168/jds.s0022-0302(91)78551-2
Wang B., Ma M. P., Diao Q. Y., Tu Y. (2019). Saponin-induced shifts in the rumen microbiome and metabolome of young cattle. Front. Microbiol. 10. doi: 10.3389/fmicb.2019.00356
Wang Y., McAllister T. A., Yanke L. J., Cheeke P. R. (2000). Effect of steroidal saponin from yucca schidigera extract on ruminal microbes. J. Appl. Microbiol. 88, 887–896. doi: 10.1046/j.1365-2672.2000.01054.x
Wen L., Xia N., Tang P., Hong Y., Wang Z., Liu Y., et al. (2015). The gastrointestinal irritation of polygala saponins and its potential mechanism in vitro and in vivo. BioMed. Res. Int. 2015, 1–8. doi: 10.1155/2015/918048
Keywords: beef cattle, feed intake, rumen modifier, saponin, winter supplementation
Citation: Gleason CB, Wilson TB, Mercadante VRG and White RR (2022) Evaluation of a rumen modifier to limit pellet intake in beef brood cows. Front. Anim. Sci. 3:1080115. doi: 10.3389/fanim.2022.1080115
Received: 25 October 2022; Accepted: 05 December 2022;
Published: 16 December 2022.
Edited by:
James Levi Klotz, Agricultural Research Service (USDA), United StatesReviewed by:
Othusitse Ricky Madibela, Botswana University of Agriculture and Natural Resources, BotswanaGibson Maswayi Alugongo, China Agricultural University, China
Copyright © 2022 Gleason, Wilson, Mercadante and White. This is an open-access article distributed under the terms of the Creative Commons Attribution License (CC BY). The use, distribution or reproduction in other forums is permitted, provided the original author(s) and the copyright owner(s) are credited and that the original publication in this journal is cited, in accordance with accepted academic practice. No use, distribution or reproduction is permitted which does not comply with these terms.
*Correspondence: Robin R. White, rrwhite@vt.edu