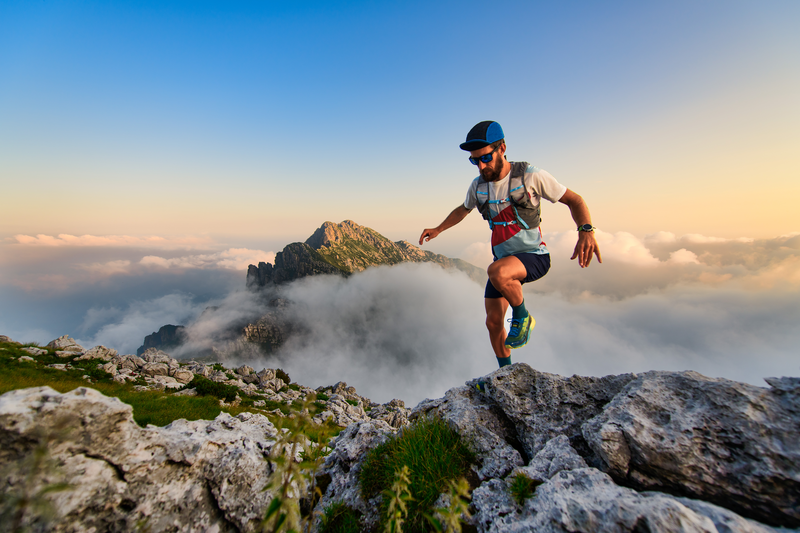
94% of researchers rate our articles as excellent or good
Learn more about the work of our research integrity team to safeguard the quality of each article we publish.
Find out more
ORIGINAL RESEARCH article
Front. Anim. Sci. , 20 October 2022
Sec. Animal Nutrition
Volume 3 - 2022 | https://doi.org/10.3389/fanim.2022.1000490
The aim of this study was to evaluate the effect of adding virginiamycin to either mineral mixture or multiple supplement on intake, digestion, ruminal fermentation profile, rumen microbial production, blood metabolites, and liver metabolism of zebu heifers fed a high-quality tropical forage. Eight Brahman heifers were assigned to a replicated 4 × 4 Latin Square design. The treatments were: mineral mixture, mineral mixture with virginiamycin, multiple supplement, and multiple supplement with virginiamycin. The basal diet consisted of a high-quality Tifton 85 hay (Cynodom sp.) chopped at 10-cm particle size and fed twice daily. The mineral mixture was provided daily at 120 g/animal. The multiple supplement was formulated to provide 300 g of crude protein (CP)/kg as fed, contained mineral mixture, corn grain, and urea: ammonium sulfate, and was daily provided at 200 g/animal. The mineral mixture and multiple supplement provided the same daily amount of minerals. The amount of supplemental virginiamycin was based on a maximum theoretical response on animal performance (50 mg/100 kg body weight) and daily mixed to the supplements types. The treatments were compared according to a 2 × 2 factorial arrangement (mineral mixture and multiple supplement, with or without virginiamycin). The virginiamycin supplementation did not alter either voluntary intake or digestibility (P≥0.44). Using the multiple supplement decreased forage (P<0.05) and digested organic matter (DOM, P<0.10) intake, but increased dietary CP : DOM ratio (P<0.01). The multiple supplement increased the ruminal ammonia concentration (P<0.04) and the acetate-to-propionate ratio (P<0.06). Virginiamycin increased urinary nitrogen (N) when provided along with multiple supplement (P<0.05). Fecal N was decreased by multiple supplement (P<0.06). Despite these effects, no effect was verified on either body N accretion (P≥0.48) or microbial N production in the rumen (P≥0.27). Virginiamycin decreased the blood IGF-1 (P<0.07). The hepatic gene expression for propionyl-CoA carboxylase was increased by virginiamycin (P<0.01). Virginiamycin increased the hepatic gene expression of both citrate synthase and pyrivate carboxylase when mineral mixture was provided (P<0.01), but decreased it when animals were fed multiple supplement (P<0.04). Using a low-intake multiple supplement with a high CP content for cattle fed high-quality forage causes a substitutive effect on forage intake, but keeps nitrogen accretion unchanged. That pattern indicates an improvement in feed efficiency. On the other hand, virginiamycin supplementation seems to cause some post-prandial influences, which may vary according to the type of supplement. Those influences apparently improve animal efficiency.
In tropical regions, most beef cattle production takes place under grazing conditions. However, tropical pastures cannot be considered balanced diets for an optimized production (Detmann et al., 2014). This particular aspect may result in a lowered beef production caused by low weight gain and advanced slaughtering age. In order to improve production, technologies must be adopted, such as adequate pasture management, supplementation, and, or using feed additives.
The application of nutritional technologies to grazing animal systems must be supported by understanding and exploring the interaction between forage and supplements aiming at optimizing the economic and productive variables of the system (Detmann et al., 2008). In the tropics, during the rainy season, the available forage undergoes intense growth and has better quality than during the dry-season (i.e., higher protein content and digestibility). However, despite the better quality, the utilization of basal nutritional resources is not thought to be optimized (Detmann et al., 2014), as nutritional unbalances may not allow an optimal rumen microbial growth or metabolizability of absorbed nutrients. In this context, there is a growth potential of approximately 200g/animal/d that is unused and can be achieved through supplementation with specific nutrients (Poppi and McLennan, 1995; Sousa et al., 2022). When animals are fed on medium- to high-quality tropical forages, it has been demonstrated that the supplementation with nitrogenous compounds is able to improve the metabolic efficiency of the animals and increase the nitrogen accretion in the body (Figueiras et al., 2016; Lazzarini et al., 2016; Reis et al., 2020).
Additives are substances without nutritional value, which are intentionally added to a diet aiming at improving either its nutritional characteristics or feeding efficiency. In terms of ruminant feeding, some additives can be used to change ruminal fermentation profile by altering the molar proportion of volatile fatty acids (VFA), mitigating methane production, and increasing the efficiency of microbial protein synthesis (Lemos et al., 2016). The main fermentation modifiers used in ruminant feeding are: ionophore antibiotics (e.g., monensin, lasalocid, salinomycin), non-ionophore antibiotics (e.g., virginiamycin, tylosin), essential oils, and probiotics.
In Brazil, monensin and virginiamycin are the most used additives for beef cattle production, including their utilization in supplements for grazing cattle. Both of them have antimicrobial action mainly on gram-positive bacteria, whose species are responsible for decreasing rumen pH and, consequently, causing metabolic disorders (Nagaraja et al., 1987). By controlling gram-positive bacteria growth, we may change the equilibrium among ruminal microbial populations and benefit the proliferation of gram-negative bacteria.
Produced by Streptomyces virginae, virginiamycin is a cyclic depsipeptides antibiotic that has a strong synergistic bactericidal activity against a wide range of gram-positive bacteria. Its structural formula comprises two factors: factor M (responsible for 80% of the molecular weight) and factor S. The factor M is known for its action on Streptococus spp. and Staphlycoccus spp. However, its action is enhanced by the presence of factor S, which, alone, is not effective against most microorganisms. The factors M and S pass through cell membrane of the gram-positive bacteria and reach the cytoplasm, where they exert their effect. Both factors irreversibly link to the 50S subunit of bacterial ribosomes, preventing the formation of the peptide bounds. Thus, they act at tRNA level, avoiding the transcription of new proteins. Consequently, there would be either a decreased growth (bacteriostatic effect) or death (bactericidal effect) of the bacterial cells (Cocito et al., 1974).
In the tropics, feeding virginiamycin to Nellore cattle was able to increase the ruminal degradation of dry matter (DM), neutral detergent fiber (NDF), and starch compared to the use of monensin and the combination of monensin and virginiamycin. Simultaneously, virginiamycin caused a decrease in the rumen protozoa population, which may imply in a decreased predation activity (Squizatti et al., 2019a; Squizatti et al., 2019b). Moreover, studies on the inclusion of virginiamycin in grazing cattle diets indicated either an improvement in animal performance (Alves Neto et al., 2018; Costa et al., 2018) or a maintenance of performance but with improvement in feed efficiency (Maciel et al., 2019).
Since tropical forages cannot be considered as balanced diets when fed exclusively for cattle, multiple supplementation constitutes the main nutritional tool to improve diet quality and nutrient utilization (Detmann et al., 2014). In this sense, the additives for modulating ruminal fermentation should be associated with either multiple supplements or mineral mixtures in order to improve the utilization of supplemental nutrients for animals under grazing system during rainy season in tropical regions.
However, the literature is still scarce of studies that evaluate any possible metabolic alteration in animals fed mineral mixtures or multiple supplements associated or not with virginiamycin. Such evidence could add to the current knowledge about the mechanisms of action of virginiamycin in animal production and efficiency.
Thus, considering the aforementioned potential effects caused by virginiamycin supplementation, we hypothesize that its effects on the equilibrium of rumen microbial populations and on rumen fermentation pattern may affect the animal metabolism. In addition, this effect can be altered when an additional supply of nutrients is provided to the animal as a mineral mixture or a multiple supplement.
Therefore, our objective was to evaluate the effects of adding virginiamycin to either mineral mixture or multiple supplement on intake, digestion, ruminal fermentation profile, microbial production in the rumen, blood metabolites, and liver metabolism in zebu heifers fed a high-quality tropical forage.
The experiment was carried out at the Animal Science Department of the Universidade Federal de Viçosa, Minas Gerais, Brazil. All surgical and animal care procedures were approved by the Institutional Animal Care and Use Committee of the Universidade Federal de Viçosa (CEUAP, protocol 57/2020).
Eight 3-years old Brahman heifers, averaging 520 ± 24.6 kg of body weight (BW), were used. The animals were housed in individual stalls (2 × 5 m) equipped with concrete floors, individual feeders, and water drinkers, which assured unrestricted access to feed and water.
The animals were previously adapted to the experimental facilities, management, and feeding for 20 d prior the experiment. The basal diet consisted of a high-quality Tifton 85 hay (Cynodom sp.) chopped at 10-cm particle size and fed twice daily at 0600 and 1800 h. To ensure ad libitum forage intake, the amount of hay was monitored daily in order to provide, at least, 300 g orts/kg of offered forage. The orts of forage were daily discarded.
The experiment was performed according to a complete and duplicated 4 × 4 Latin square design, balanced for residual effects (Cochran and Cox, 1957). Animals were allocated to the different squares according to their BW. Each experimental period lasted 25 d, with 14 d for adaptation to the treatments (Machado et al., 2016) and 11 d for sample collections. Heifers were weighed at the beginning and the end of each experimental period in order to calculate the average BW and relative intake.
The four treatments were: mineral mixture (MM), mineral mixture with virginiamycin (MM+V), multiple supplement (MSUP), and multiple supplement with virginiamycin (MSUP+V). The mineral mixture contained a blend of macro and micro minerals (Table 1) and was provided daily at 120 g/animal for the treatments MM and MM+V. This specific daily amount of mineral mixture was provided so that the animals could achieve an ad libitum intake. The multiple supplement was planned to present 300 g of crude protein (CP)/kg as fed and contained mineral mixture (600 g/kg as fed), corn grain (300 g/kg as fed), and urea: ammonium sulfate (9:1, 100 g/kg as fed). The multiple supplement was daily provided at 200 g/animal so the amount of offered mineral mixture was the same for all treatments. The amount of supplemental virginiamycin (VMAX 10®, Phibro Animal Health Corporation, Teaneck, New Jersey, USA) was based on the findings by Alves Neto et al. (2018) aiming at a maximum theoretical response on animal performance (50 mg/100 kg BW or 250 mg/d assuming an average BW of 500 kg). The virginiamycin was daily mixed to the mineral mixture or multiple supplement just before offering to the animals. The supplements (mineral and multiple) were offered at 0600 h in a separate feeder and their intake was monitored daily.
Voluntary forage intake was quantified from d 15 to d 21 of each period. The calculations considered the amount of forage offered from d 15 to d 20, and the orts obtained from d 16 to d 21. Representative samples of hay and orts were collected daily, stored in plastic bags, and blended manually at the end of each period in order to obtain pooled samples per animal. Samples of the supplements were collected after each batching in the mill of the Universidade Federal de Viçosa. All samples were ground in a knife mill to pass through a 1-mm screen sieve.
Total fecal output was measured at d 16, d 18, and d 20 of each period. The feces were collected immediately after spontaneous defecation and stored in 35-L polyethylene containers. After 24 hours, the collected feces were weighted and manually homogenized. Then, a representative sample (50 g/kg) was collected and oven-dried (55°C). The samples were ground as previously described and pooled samples (weighted according to the amount of feces obtained in each collection) were produced per animal.
The urine was fully collected from d 21 to d 23 of each period using a 2-way Foley catheter (n° 26, Rush Amber, Kamuting, Malaysia) which has a 30-mL balloon. A polyethylene tube was connected to the free end of the catheter for conducting the urine to clean containers (20-L). To avoid N losses, the containers were kept into Styrofoam boxes with ice (Van Niekerk et al., 1963). At the end of each 24-h period, the urine was weighed and thoroughly mixed. Two aliquots (10 mL/L) were taken and filtered through four layers of cheesecloth. The first one was immediately analyzed for the total N concentration (method N-001/2; Detmann et al., 2021). The second aliquot had its pH corrected down to 3.0 with the addition of concentrated sulfuric acid (H2SO4) and frozen (-20°C) for later analyses.
On d 24 of each period, blood samples were collected at 0600, 1200, 1800, and 2400 h using needles and 4-mL vacuum tubes (BD vacutainer, Bencton Dickson Franklin Lakes, New Jersey, USA) with sodium fluoride/EDTA (for glucose analyses) and with clot activator (for the other analyses). The samples were then centrifuged (1.200 × g, 15 min at 4°C) for separation of plasma (floride/EDTA tubes) and serum (clotted tubes). The material was kept refrigerated (4°C). At the end of the collections, a pooled sample was prepared per animal and frozen (-20°C) for later analyses.
On d 24 of each period, sampling of rumen liquid was performed at 1200 h using an oral probe. The liquid was filtrated through a triple layer of cheesecloth and two 40-mL aliquots were taken. The first was fixed with 1 mL of a H2SO4 solution (500 mL/L) and frozen (-20°C) for ruminal ammonia N (RAN) analyses. The second aliquot was fixed with 0.4 mL of H2SO4 solution (500 mL/L) and kept at -20°C for subsequent VFA analyses. This procedure was performed in only one of the Latin squares (four animals and four periods).
On the last day of each period (d 25), hepatic biopsy was performed in only one of the Latin squares (opposite to the one used for rumen liquid sampling). The sampling procedure took place in the intercostal space between the 11th and 12th ribs on the right side of the animals. A 5 × 5-cm area was shaved, cleaned with ethanol (700 mL/L), and anesthetized with lidocaine (20 g/L). After this procedure, a 1.0-cm incision was made with a scalpel, and through it the tru-cut needle was inserted to extract the liver sample (Herdt, 2013). The liver samples were washed with sterile saline solution (NaCl, 9 g/L) and immediately frozen in liquid nitrogen. After that, the samples were kept in an ultra-freezer (-80°C) for subsequent analyses of gene expression.
All feed, ort, and fecal samples were analyzed according to the standard analytical procedures of the Instituto Nacional de Ciência e Tecnologia de Ciência Animal (INCT-CA; Detmann et al., 2021) for dry matter (DM; dried overnight at 105°C; method G003/1), ash (complete combustion in a muffle furnace at 550°C; method M-001/2), and N (Kjeldahl procedure; method N-001/2) contents. The neutral detergent fiber (NDF) contents were evaluated using a heat-stable α-amylase and omitting sodium sulfite according to Mertens (2002). The NDF contents were expressed exclusive of residual ash and protein (NDFap, Table 1).
The urinary concentrations of urea (colorimetric kinetic test, Bioclin® K056), creatinine (enzymatic-colorimetric method, Bioclin® K067), and uric acid (enzymatic-colorimetric method, Bioclin® K139) were quantified with an automated chemistry analyzer (BS200E, Mindray, China). A colorimetric method was used to measure the concentration of urinary allantoin (Chen and Gomes, 1992). The microbial production in the rumen was estimated using the equations proposed by Barbosa et al. (2011).
Blood plasma samples were analyzed for glucose (enzymatic glucose oxidase-peroxidase method, Bioclin® K082), urea (enzymatic-colorimetric method, Bioclin® K056), total protein (colorimetric kinetic test, Bioclin® K031), albumin (bromecresol green method, Bioclin® K040), aspartate transaminase (AST, U.V. kinetic – IFCC, Bioclin® K048), alanine transaminase (ALT, U.V. kinetic – IFCC, Bioclin® K049), and gamma-glutamyl transferase (GGT, SZASZ IFCC method, Bioclin® K080). The blood concentration of insulin-like growth factor (IGF-1) was analyzed by chemiluminescence in a commercial laboratory (ViçosaLab, Viçosa, Minas Gerais, Brazil). The blood globulins were estimated as the difference between blood total protein and albumin.
The concentration of RAN was quantified using the colorimetric technique described by Detmann et al. (2021), method N-006/1). For VFA analyses, rumen fluid samples were centrifuged (12,000 × g, 10 min, 4°C) and supernatants were treated as described by Siegfried et al. (1984). Ruminal VFA were analyzed by HPLC (Shimadzu HPLC class VP series, model SPD 10A; Shimadzu Corporation, Kyoto, Japan) using a reverse-phase column (mobile phase 0.15 M ortho-phosphoric acid) and UV detector at a wavelength of 210 nm, as described by Batista et al. (2016).
The frozen samples of liver were powdered in liquid N and total RNA was extracted from 0.07 g of tissue using Trizol® (Invitrogen TM, Thermo Fischer Scientific®, Oregon USA) following the manufacturer’s recommendations. Subsequently, washing and RNA isolation were performed using RNase free silica membrane columns (PureLink TM RNA Mini Kit – Invitrogen TM, Fischer Scientific®, Oregon USA). After extraction, the RNA was quantified by a NanoVue spectrophotometer (GE Healthcare Life Sciences Inc.) and its integrity was verified using an agarose gel (1%). The RNA samples were then reverse transcribed into cDNA using the GoScriptTM Reverse Transcription System Kit (Promega Corporation, Madison, Wisconsin, USA). The primers (Table 2) for amplification of target and endogenous genes were designed using PrimerQuest software (www.idtdna.com/Scitools/Applications/PrimerQuest) with sequences obtained using GenBank (www.ncbi.nlm.nih.gov). Real-time quantitative PCR was performed in thermal cycler ABI Prism 7300 Sequence Detection System (Applied Biosystems, Foster City, CA, USA) using the detection method SYBR Green (Applied Biosystems – Foster City, CA, USA) with GoTaq® qPCR Master Mix kit (Promega Corporation, Madison, WI, USA) and the following cycle parameters:. 50°C for 2 min, 95°C for 2 min, followed by 40 cycles of 95°C for 15 s, 60°C for 15 s, and 72°C for 1 min. The mRNA expression was quantified by the 2−ΔΔCt method (Livak and Schmittgen, 2001).
The experiment was carried out according to a replicated 4 × 4 Latin Square design. The response variables measured in both squares were analyzed according to the model:
where Yijkl is the response measured on the animal k and period l within the Latin square i, and submitted to the treatment j; μ is the general constant; Qi is the random effect of the Latin square i; Tj is the fixed effect of the treatment j; A(i)k is the random effect of the animal k nested within the Latin square i; P(i)l is random the effect of experimental period l nested within the Latin square i; QTij is the interaction effect between Latin square i and treatment j (random); and ϵijkl is the random error, which is supposed to be NIID (μ, ).
We performed a first analysis of variance in order to evaluate the interaction between Latin squares and treatments. We anticipate that we did not find any significant interaction (P>0.10). Then, the interaction was removed from the model as it does not interfere on randomization process and its removal increases the residual degrees of freedom. For the response variables measured in only one Latin square, the model (1) was re-parameterized by excluding the Latin square effect and its interaction with treatments.
The treatments were compared according to a 2 × 2 factorial arrangement (mineral mixture and multiple supplement, with or without virginiamycin). All analyses were performed using the MIXED procedure of SAS 9.4. Significant effects were declared at P<0.10.
On average, the mineral mixture intake was 86 and 91 g/d without and with virginiamycin inclusion, respectively. Moreover, the multiple supplement intake averaged 173 and 168 g/d without and with virginiamycin inclusion, respectively (Table 3). We planned that the animals should consume 250 mg/d of virginiamycin. However, as the supplement intake was lower than the amount offered, the estimated virginiamycin intake was 186 and 207 mg/d for mineral mixture and multiple supplement, respectively.
Table 3 Effects of feeding multiple supplement and, or virginiamycin on voluntary intake of Brahman heifers fed a high-quality tropical forage.
There was no interaction between virginiamycin and supplement type (P≥0.58) nor effects of virginiamycin (P≥0.66) on voluntary intake characteristics (Table 3). However, using the multiple supplement decreased voluntary DM intake in both kg/d (P<0.07) and g/kg BW (P<0.05). This pattern was a direct response to a decrease in forage intake (P<0.05), despite the fact that supplement intake was slightly higher for animals fed the multiple supplement. Due to the decreased forage intake, the multiple supplementation did not affect the CP intake (P=0.60). Thus, from those effects, providing multiple supplement decreased digested organic matter (DOM) intake (P<0.10), but increased dietary CP : DOM ratio (P<0.01).
There were no effects of virginiamycin (P≥0.44) or interaction between virginiamycin and supplement type (P≥0.61) on total digestibility and dietary DOM content (Table 4). The multiple supplementation increased (P<0.08) the CP digestibility, but did not affect the other digestion characteristics (P≥0.34). The average dietary DOM across treatments (P≥0.44) was 562 g/kg DM.
Table 4 Effects of feeding multiple supplement and, or virginiamycin on total digestibility (g/g) and total dietary content of digested organic matter (g/kg dry matter) in Brahman heifers fed a high-quality tropical forage.
There were no effects (P≥0.46) on rumen pH and VFA concentrations (Table 5). The RAN concentration was only affect by supplement type (P<0.04). On average, when compared to mineral mixture, multiple supplement increased RAN from 6.16 to 8.16 mg/dL. The molar proportions of individual VFA were only affected by providing multiple supplement, which increased the proportion of acetate (P<0.07) and acetate-to-propionate ratio (P<0.06), but decreased the molar proportion of propionate (P<0.06). No effect was verified on molar proportion of butyrate (P≥0.46).
Table 5 Effects of feeding multiple supplement and, or virginiamycin on ruminal fermentation characteristics of Brahman heifers fed a high-quality tropical forage.
Even though there was no difference in N intake, there was an interaction between virginiamycin and supplement type on urine N excretion (P<0.08, Table 6). Virginiamycin did not affect urine N when animals received mineral mixture (P>0.64), but increased urinary N excretion when multiple supplementation was used (P<0.05). On the other hand, the fecal-N excretion was decreased on average by multiple supplementation (P<0.06). Despite of these patterns, there were no effects on N retention (P≥0.48) or efficiency of N utilization (P≥0.27). The same was verified for microbial N production and efficiency (P≥0.24), whose average values were 99 g N/d, 0.75 g N/g N intake, and 137 g microbial CP/kg DOM.
Table 6 Effects of feeding multiple supplement and, or virginiamycin on N utilization characteristics of heifers fed a high-quality tropical forage.
Providing virginiamycin decreased the blood IGF-1 (P<0.07), whereas multiple supplementation increased blood urea-N (P<0.01). The other blood characteristics and liver function indicators shown at Table 7 were not altered by any effect (P≥0.11).
Table 7 Effects of feeding multiple supplement and, or virginiamycin on blood characteristics of heifers fed a high-quality tropical forage.
Hepatic gene expressions for phosphoenolpyruvate carboxykinase (P≥0.23) and carbamoyl phosphate synthetase (P≥0.77) were not affected viriginiamycin, multiple supplement, or their interaction (Table 8). The gene expression for propionyl-CoA carboxylase was not affected by either multiple supplementation (P>0.23) or interaction between multiple supplementation and virginiamycin (P>0.67). However, it was increased by virginiamycin (P<0.01). Interaction between supplement type and virginiamycin was observed for the gene expression of pyruvate carboxylase (P<0.01). Virginiamycin increased the gene expression when mineral mixture was provided (P<0.01), but decreased it when animals were fed multiple supplement (P<0.04). The interaction effect was also observed for citrate synthase gene expression (P<0.02), which showed the same pattern observed for pyruvate carboxylase.
Table 8 Effects of feeding multiple supplement and, or virginiamycin on the hepatic gene expression of some key enzymes of energy metabolism and urea cycle of heifers fed a high-quality tropical forage.
Generally, virginiamycin did not cause any effect on voluntary intake and digestibility. This pattern agrees with several experiments carried out in the tropics (Monção, 2017; Alves Neto et al., 2018; Costa et al., 2018). On the other hand, some authors stated that virginiamycin could decrease the voluntary intake of supplements (Maciel et al., 2019), which was not verified in our study, as the multiple supplement intake was approximately the same with or without virginiamycin inclusion. According to Monção (2017), the voluntary intake should not be affected by the virginiamycin because the nutritive value of the diet would not be altered by its inclusion.
However, the forage intake was depressed by the multiple supplementation with an average substitutive effect of 10 g of forage per g of supplement (Table 3). Such a negative effect reflected a lack of compensation on total DM intake, which was also decreased when compared to the animals fed mineral mixture. Overall, the control of voluntary intake in ruminants cannot be attributed to a single factor as it has a multifactorial influence (Forbes, 2003). In the tropics, the protein-to-energy ratio (CP : DOM) in the total diet has been pointed out as valuable indicator of the animal metabolic discomfort towards the voluntary intake behavior. This ratio allows integrating the supplemental effects on both rumen and body metabolism (Poppi and McLennan, 1995; Detmann et al., 2014). In this aspect, the intake of tropical forages would be maximized when CP : DOM of the diet is adjusted close to 210 g/kg through supplementation (Reis et al., 2016). In our experiment, the average CP : DOM with mineral mixture supplementation was 176 g/kg and was increased by the multiple supplement, on average, up to 187 g/kg. Despite these values being lower than the optimal ratio (Reis et al., 2016), the multiple supplementation slightly changed CP : DOM towards a more comfortable ratio, and a decrease in voluntary intake should not have occurred. An excessive ruminal ammonia caused by protein supplements also can impair forage intake (Reis et al., 2020) due to its possible neurotoxicity (Kertz et al., 1982) or impairment in liver metabolism (Allen et al., 2009). However, the increase in RAN caused by the multiple supplement was only marginal and any constraint to intake caused by ammonia is unlikely. It is known that substitutive effect caused by supplementation increases as the forage quality increases (Minson, 1990). It seems to be the case we observed.
The only effect detected on digestibility was the increased CP digestion when the multiple supplement was provided to the animals. Considering the substitution of forage by supplements, part of the protein from forage (mainly fiber-bound protein) was replaced by urea, which increased, on average, the urinary N excretion. Therefore, a lower amount of N from forage escaped to the hindgut (indicated by the lowered fecal N) and, then, the apparent digestion of CP increased.
Despite of its expected action on gram-positive bacteria (Cocito et al., 1974), we did not observe any apparent impact of virginiamycin on rumen fermentation profile or microbial production. According to Salinas-Chavira et al. (2009), virginiamycin tends to improve feed efficiency in cattle, but its effects on ruminal fermentation and digestion are small and cannot be responsible for improvements in performance. Several researches also have demonstrated either absence of impacts or only marginal effects of virginiamycin on rumen fermentation characteristics (Nagaraja et al., 1987; Monção, 2017; Alves Neto et al., 2018; Costa et al., 2018). Costa et al. (2018) found an increment in microbial N production in the rumen with virginiamycin supplementation, which was probably caused by a decreased predation activity. However, we did not find a similar pattern in our study.
Nevertheless, ruminal fermentation characteristics were affected by multiple supplementation. The RAN concentration was increased by the multiple supplement, on average, from 6.2 to 8.2 mg/dL, making the rumen ammonia availability more adequate for action of fibrolytic microorganisms (Detmann et al., 2009). Despite of virginiamycin has no effect on total VFA concentration, multiple supplementation increased the molar proportion of acetate and decreased molar proportion of propionate. The positive effects of supplemental protein on molar proportion of acetate were also described by Figueiras et al. (2016).
Nonetheless, multiple supplementation did not affect the microbial N production in the rumen. However, this pattern should not be seen as a complete absence of effects of supplemental N on rumen microorganisms. The protein supplementation is able to increase the contribution of fibrolytic species in the total bacterial population in the rumen, even though a concomitant improvement in fiber digestion cannot be observed (Silva-Marques et al., 2019). The change in the fermentation profile from propionate to acetate seems to indicate an increased growth of fibrolytic bacteria, which was caused by supplemental N. However, microbial growth in the rumen and microbial flow could not necessarily be related to each other (Reis et al., 2020) and an increased growth of particular species cannot reflect an increased microbial flow to intestines (Belanche et al., 2012; Fanchone et al., 2013). On the other hand, an increased fiber degradation in the rumen could not be detected by total digestion measurements, as it is not able to account for the influence of hindgut fermentation. An increased escape of potentially degradable NDF from rumen increases the amount of potentially degradable fiber in the hindgut (Oliveira et al., 2020). Therefore, based on this, it is possible that multiple supplement affected bacterial growth in the rumen. However, due to integration with other nutritional events, it was not able to improve total microbial flow to intestines and the amount of fiber digested in the gastrointestinal tract.
At first glance, the treatments here evaluated would have no potential action on animal performance, as no effect was detected on body N accretion. However, that result must be evaluated in an integrated way by considering simultaneously other evidences obtained in our work. First, the multiple supplementation decreased voluntary intake, but did not affect N accretion. It constitutes a clear evidence that low-intake protein supplements can improve feed efficiency of cattle fed high-quality forages. Our basic reasoning relies on the fact that total energy intake decreased (which is here represented by DOM intake), but efficiency of N utilization remained unchanged.
Second, virginiamycin supplementation decreased blood IGF-1, but also did not affect N accretion. The IGF-1 is an endocrine regulator of muscle growth and it is also an important link between growth hormone and the metabolic processes of growth (Drewnoski et al., 2014; Barclay et al., 2019). Blood IGF-1 has been positively correlated with N accretion in cattle fed tropical forages (Reis et al., 2020; Rufino et al., 2020; Franco et al., 2021). In this sense, the decreased IGF-1 caused by virginiamycin should have implied a decreased N accretion, which did not happen. There are two connected implications here. First, the virginiamycin may have improved metabolic efficiency of the animals, which corroborate the results obtained by Salinas-Chavira et al. (2009). Second, virginiamycin could change the metabolic regulation in the animals and prioritizes other ways to keep anabolism unaltered. These effects could not be caused directly by supplemental virginiamycin. Rather, they could be attributed to any change in digestion process that signals those possible changes in animal metabolism.
We may support our hypothesis from the results obtained for gene expression of key enzymes for hepatic energy metabolism. Virginiamycin increased gene expression for propionyl-CoA-carboxylase, which is a key enzyme to insert propionate into the Krebs cycle (Nelson and Cox, 2014) for either oxidation or gluconeogenesis. This is particularly intriguing, as virginiamycin had no effect on VFA concentration and molar proportion. However, we measured only concentration of VFA, which represents a static picture of rumen fermentation and not necessarily is associated with VFA production. The amount of VFA absorbed is influenced by substrate type, fermentation rate and routes, absorption through rumen wall, and outflow to abomasum. Thus, this dynamic process cannot be accurately described by a single and statical measurement of concentrations in rumen fluid. Therefore, the pattern of propionyl-CoA-carboxylase caused by virginiamycin seems to indicate a significant impact on rumen fermentation and, consequently, an alteration of metabolite profile for intermediate metabolism and synthesis processes in the animal body. This could have, at least partially, some influence on the improvement of the animal efficiency caused by virginiamycin supplementation.
On the other hand, the virginiamycin effects on pyruvate carboxylase, which interacted with the type of supplement, seems to indicate another post-prandial influence of this additive. Even though the expression of PEPCK was not effected (P>0.23), it followed the same interaction pattern (Table 8). Both enzymes are key for the gluconeogenesis (Nelson and Cox, 2014) and their gene-expression pattern may indicate some indirect influence of virginamycin (perhaps from ruminal fermentation as well) on glucose production in ruminant. Interestingly, the same influence was observed on gene expression for citrate synthase, which indicates some influence in the hepatic oxidation of acetate. However, the actual meaning of the interaction between virginiamycin and supplement type could not be understood from our results and further studies are suggested in order to obtain a clearer understanding of this post-prandial virginiamycin influence.
Using a low-intake multiple supplement with a high CP content for cattle fed high-quality forage causes a substitutive effect on forage intake, but keeps nitrogen accretion unchanged. That pattern indicates an improvement in feed efficiency. On the other hand, virginiamycin supplementation seems to cause some post-prandial influences, which may vary according to the type of supplement. Those influences apparently improve animal efficiency. However, this statement deserves further studies in order to obtain a clearer understanding.
The original contributions presented in the study are included in the article/supplementary material. Further inquiries can be directed to the corresponding author.
The animal study was reviewed and approved by Animal Care and Use Committee of the Universidade Federal de Viçosa.
RV: investigation, writing - original draft. NL: investigation, writing – review and editing. CS: writing – review and editing. MD: methodology, writing – review and editing. ED: conceptualization, formal analysis, supervision, funding acquisition, project administration, writing – review and editing. All authors contributed to the article and approved the submitted version.
This project has finnacial support from Insitituto Nacional de Ciência e Tecnologia de Ciência Animal (INCT-CA), Conselho Nacional de Pesquisa e Desenvolvimento Científico e Tecnológico (CNPq), Coordenação de Aperfeiçoamento de Pessoal de Nível Superior (CAPES), Fundação de Amparo à Pesquisa do Estado de Minas Gerais (FAPEMIG), and Swedish University of Agricultural Sciences (SLU).
The authors declare that the research was conducted in the absence of any commercial or financial relationships that could be construed as a potential conflict of interest.
All claims expressed in this article are solely those of the authors and do not necessarily represent those of their affiliated organizations, or those of the publisher, the editors and the reviewers. Any product that may be evaluated in this article, or claim that may be made by its manufacturer, is not guaranteed or endorsed by the publisher.
Allen M. S., Bradforf B. J., Oba M. (2009). The hepatic oxidation theory of the control of feed intake and its application to ruminants. J. Anim. Sci. 87, 3317–3334. doi: 10.2527/jas.2009-1779
Alves Neto J. A., Oliveira I. M., Moretti M. H., Gonçalves P. H., Alves M. A. P., Fernandes J. R., et al. (2018). Determining the optimal dose of virginiamycin for ruminal parameters and performance of nellore cattle on pasture. Semina-Cienc. Agrar. 39, 1749–1758. doi: 10.5433/1679-0359.2018v39n4p1749
Barbosa A. M., Valadares R. F. D., Valadares Filho S. C., Pina D. S., Detmann E., Leão M. I. (2011). Endogenous fraction and urinary recovery of purine derivates obtained by different methods in nellore cattle. J. Anim. Sci. 89, 510–519. doi: 10.2527/jas.2009-2366
Barclay R. B., Burd N. A., Tyler C., Tillin N. A., MacKenzie R. W. (2019). The role of the IGF-1 signaling cascade in muscle protein synthesis and anabolic resistance in aging skeletal muscle. Front. Nutr. 6. doi: 10.3389/fnut.2019.00146
Batista E. D., Detmann E., Titgemeyer E. C., Valadares Filho S. C., Valadares R. F. D., Prates L. L., et al. (2016). Effects of varying ruminally undegradable protein supplementation on forage digestion, nitrogen metabolism, and urea kinetics in nellore cattle fed low-quality tropical forage. J. Anim. Sci. 94, 201–216. doi: 10.2527/jas2015-9493
Belanche A., Doreau M., Edwards J. E., Moorby J. M., Pinloche E., Newbold C. J. (2012). Shifts in the rumen microbiota due to the type of carbohydrate and level of protein ingested by dairy cattle are associated with changes in rumen fermentation. J. Nutr. 142, 684–1692. doi: 10.3945/jn.112.159574
Chen X. B., Gomes M. J. (1992). Estimation of microbial protein supply to sheep and cattle based on urinary excretion of purine derivates – an overview of technical details (Aberdeen: Roweet Research Institute).
Cocito C., Voorma H., Bosch L. (1974). Interference of virginiamycin m with the initiation and the elongation of peptide chains in cell-free systems. Biochim. Biophys. Acta – Nucleic Acids Protein Synthesis 340, 285–298. doi: 10.1016/0005-2787(74)90274-3
Costa J. P. R., Jesus R. B., Oliveira I. M., Resende F. D., Siqueira G. R., Malheiros E. B. (2018). Does virginiamycin supplementation affect the metabolism and performance of nellore bulls grazing under low and high gain rates? Anim. Sci. J. 10, 1432–1441. doi: 10.1111/asj.13052
Detmann E., Costa e Silva L. F., Rocha G. C., Palma M. N. N., Rodrigues J. P. P. (2021). Métodos para análise de alimentos. 2nd ed (Visconde do Rio Branco: Suprema).
Detmann E., Paulino M. F., Mantovani H. C., Valadares Filho S. C., Sampaio C. B., Souza M. A., et al. (2009). Parameterization of ruminal fibre degradation in low-quality tropical forage using michaelis-menten kinectis. Livest. Sci. 126, 136–146. doi: 10.1016/j.livsci.2009.06.013
Detmann E., Paulino M. F., Valadares Filho S. C. (2008). “Avaliação de alimentos ou de dietas? uma abordagem conceitual,” in 6th simpósio de produção de gado de corte (Viçosa: Departamento de Zootecnia/ UFV), pp. 21–pp. 52.
Detmann E., Paulino M. F., Valadares Filho S. C., Huhtanen P. (2014). Nutritional aspects applied to grazing cattle in the tropics: a review based on Brazilian results. Semina-Cienc. Agrar. 35, 2829–2854. doi: 10.5433/1679-0359.2014v35n4Suplp2829
Drewnoski M. E., Huntington G. B., Poore M. H. (2014). Reduced supplementation frequency increased insulin-like growth factor 1 in beef steers fed medium quality hay and supplemented with a soybean hull and corn gluten feed blend. J. Anim. Sci. 92, 2546–2553. doi: 10.2527/jas.2013-7372
Fanchone A., Nozière P., Portelli J., Duriot B., Largeau V., Doreau M. (2013). Effects of nitrogen underfeeding and energy source on nitrogen ruminal metabolism, digestion, and nitrogen partitioning in dairy cows. J. Anim. Sci. 91, 895–906. doi: 10.2527/jas.2012-5296
Figueiras J. F., Detmann E., Franco M. O., Batista E. D., Reis W. L. S., Paulino M. F., et al. (2016). Effects of supplements with different protein contents on nutritional performance of grazing cattle during the rainy season. Asian austral. J. Anim. Sci. 29, 1710–1718. doi: 10.5713/ajas.16.0125
Forbes J. M. (2003). The multifactorial nature of food intake control. J. Anim. Sci. 81, E139–E144. doi: 10.2527/2003.8114_suppl_2E139x
Franco M. O., Detmann E., Batista E. D., Rufino L. M. A., Paulino M. F., Valares Filho S. C. (2021). Nutritional performance and metabolic characteristics of cattle fed tropical forages with protein and starch supplementation. An. Acad. Bras. Ciênc. 93, 20190487. doi: 10.1590/0001-3765202120190487
Herdt T. H. (2013). Liver biopsy procedure in cattle. Lansing Diagn. Center Population e Anim. Health. Available at: https://cvm.msu.edu/vdl/laboratory-sections/nutrition/mineral-and-vitamin-testing-sample-collection-and-handling/liver-biopsy-procedure-in-cattle.
Kertz A. F., Koepke M. K., Davidson L. E., Betz N. L., Norris J. R., Skoch L. V., et al. (1982). Factors influencing intake of high urea-containing rations by lactating dairy cows. J. Dairy Sci. 65, 587–604. doi: 10.3168/jds.S0022-0302(82)82236-4
Lazzarini I., Detmann E., Valadares Filho S. C., Paulino M. F., Batista E. D., Rufino L. M. A., et al. (2016). Nutritional performance of cattle grazing during rainy season with nitrogen and starch supplementation. Asian Austral. J. Anim. Sci. 29, 1120–1128. doi: 10.5713/ajas.15.0514
Lemos B. J. M., Castro F. G. F., Santos L. S., Mendonça B. P. C., Couto V. R. M., Fernandes J. J. R. (2016). Monensin, virginiamycin, and flavomycin in a no-roughage finishing diet fed to zebu cattle. J. Anim. Sci. 94, 4307–4314. doi: 10.2527/jas.2016-0504
Livak K. J., Schmittgen T. D. (2001). Analysis of relative gene expression data using real-time quantitative PCR and the 2-ΔΔCT method. Methods 25, 402–408. doi: 10.1006/meth.2001.1262
Machado M. G., Detmann E., Mantovani H. C., Valadares Filho S. C., Bento C. B., Marcondes M. I., et al. (2016). Evaluation of the length of adaptation period fir changeover and crossover nutritional experiments with cattle fed tropical forage-based diets. Anim. Feed Sci. Technol. 222, 132–148. doi: 10.1016/j.anifeedsci.2016.10.009
Maciel I. C. F., Saturnino H. M., Barbosa F. A., Malacco V. M. R., Andrade Jr. J.M.C., Maia Filho G. H. B., et al. (2019). Virginiamycin and sodium monensin supplementation for beef cattle on pasture. Arq. Bras. Med. Vet. Zootec. 71, 1999–2008. doi: 10.1590/1678-4162-10659
Mertens D. R. (2002). Gravimetric determination of amylase-treated neutral detergent fiber in feeds with refluxing in beakers or crucibles: collaborative study. J. AOAC Inter. 85, 1217–1240. doi: 10.1093/jaoac/85.6.1217
Monção F. P. (2017). Suplementação e uso da virginiamicina como moduladores do desempenho de bovinos nelore na recria e seus efeitos na terminação em confinamento (Jaboticabal: Universidade Estadual Paulista).
Nagaraja T. G., Taylor M. B., Harmon D. L., Boyer J. E. (1987). In vitro lactic acid inhibition and alterations in volatile fatty acid production by antimicrobial feed additives. J. Anim. Sci. 65, 1064–1076. doi: 10.2527/jas1987.6541064x
Nelson D. L., Cox M. M. (2014). Lehninger principles of biochemistry. 6th ed (New York: W.H. Freeman and Company).
Oliveira C. V. R., Silva T. E., Batista E. D., Rennó L. N., Silva F. F., Carvalho I. P. C., et al. (2020). Urea supplementation in rumen and post-rumen for cattle fed a low-quality tropical forage. Br. J. Nutr. 124, 1166–1178. doi: 10.1017/S0007114520002251
Poppi D. P., McLennan S. R. (1995). Protein and energy utilization by ruminants at pasture. J. Anim. Sci. 73, 278–290. doi: 10.2527/1995.731278x
Reis W. L. S., Detmann E., Batista E. D., Rufino L. M. A., Gomes D. I. C., Bento C. B. P., et al. (2016). Effects of ruminal and post-ruminal protein supplementation in cattle fed tropical forages on insoluble fiber degradation, activity of fibrolytic enzymes, and the ruminal microbial community profile. Anim. Feed Sci. Technol. 218, 1–16. doi: 10.1016/j.anifeedsci.2016.05.001
Reis W. L. S., Palma M. N. N., Paulino M. F., Rennó L. N., Detmann E. (2020). Investigation on daily or every three days supplementation with protein or protein and starch of cattle fed tropical forage. Anim. Feed Sci. Technol. 269, 114650. doi: 10.1016/j.anifeedsci.2020.114650
Rufino L. M. A., Batista E. D., Rodrigues J. P. P., Valadares Filho S. C., Paulino M. F., Costa e Silva L. F., et al. (2020). Effects of the amount and frequency of nitrogen supplementation on intake, digestion, and metabolism in cattle fed low-quality tropical grass. Anim. Feed Sci. Technol. 260, 114367. doi: 10.1016/j.anifeedsci.2019.114367
Salinas-Chavira J., Lenin J., Ponce E., Sanchez U., Torrenta N., Zinn R. A. (2009). Comparative effects of virginiamycin supplementation on characteristics of growth-performance, dietary energetics, and digestion of calf-fed Holstein steers. J. Anim. Sci. 87, 4101–4108. doi: 10.2527/jas.2009-1959
Siegfried R., Ruckemann H., Stumpf G., Siegfried V. R., Ruckemann H., Siegfried B. D. (1984). Method for the determination of organic acids in silage by high performed liquid chromatograpy. Landwirt. Frosch. 37, 298–304.
Silva-Marques R. P., Zervoudarkis J. T., Nakazato L., Hatamoto-Zervoudakis L. K., Cabral L. S., Matos N. B. N., et al. (2019). Ruminal microbial populations and fermentation characteristics in beef cattle grazing tropical forage in dry season and supplemented with different protein levels. Curr. Microbiol. 76, 270–278. doi: 10.1007/s00284-019-01631-w
Sousa L. C. O., Palma M. N. N., Franco M. O., Detmann E. (2022). Does frequency of protein supplementation affect performance of cattle under grazing in tropical pastures? Anim. Feed Sci. Technol. 289, 115316. doi: 10.1016/j.anifeedsci.2022.115316
Squizatti M. M., Rigueiro A. L. N., Assumpção A. H. P. M., Dias E. F. F., Lirian K., Silva L. A. F., et al. (2019a). “Reduction of adaptation to high-energy diets using virginiamycin: effects on ruminal fermentation,” in EAAP international symposium on energy and protein metabolism and nutrition (Belo Horizonte: Wageningen Academic Publishers), p. 255–256.
Squizatti M. M., Rigueiro A. L. N., Soares C. H. G., Nunes A. B. C. P., Dermartini B. L., Felizari L. D., et al. (2019b). “Reduction of adaptation to high-energy diets using virginiamycin: protozoa and rumen degradability,” in EAAP international symposium on energy and protein metabolism and nutrition (Belo Horizonte: Wageningen Academic Publishers), p. 255–256.
Keywords: additives, Brahman, nitrogen balance, supplementation, voluntary intake
Citation: Vidal RC, Lima NSA, Sampaio CB, Duarte MS and Detmann E (2022) Association of virginiamycin and multiple supplement for cattle fed a high-quality tropical forage. Front. Anim. Sci. 3:1000490. doi: 10.3389/fanim.2022.1000490
Received: 22 July 2022; Accepted: 06 October 2022;
Published: 20 October 2022.
Edited by:
Sarita Bonagurio Gallo, Faculty of Animal Science and Food Engineering, University of São Paulo, BrazilReviewed by:
Fabricia Miotto, Universidade Federal do Norte do Tocantins, BrazilCopyright © 2022 Vidal, Lima, Sampaio, Duarte and Detmann. This is an open-access article distributed under the terms of the Creative Commons Attribution License (CC BY). The use, distribution or reproduction in other forums is permitted, provided the original author(s) and the copyright owner(s) are credited and that the original publication in this journal is cited, in accordance with accepted academic practice. No use, distribution or reproduction is permitted which does not comply with these terms.
*Correspondence: Edenio Detmann, ZWRlbmlvLmRldG1hbm5Ac2x1LnNl
Disclaimer: All claims expressed in this article are solely those of the authors and do not necessarily represent those of their affiliated organizations, or those of the publisher, the editors and the reviewers. Any product that may be evaluated in this article or claim that may be made by its manufacturer is not guaranteed or endorsed by the publisher.
Research integrity at Frontiers
Learn more about the work of our research integrity team to safeguard the quality of each article we publish.