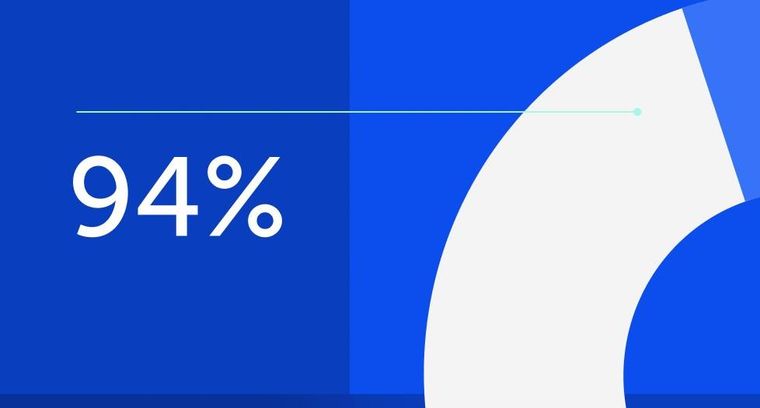
94% of researchers rate our articles as excellent or good
Learn more about the work of our research integrity team to safeguard the quality of each article we publish.
Find out more
BRIEF RESEARCH REPORT article
Front. Anim. Sci., 27 January 2022
Sec. Animal Physiology and Management
Volume 2 - 2021 | https://doi.org/10.3389/fanim.2021.799289
This article is part of the Research TopicMinimally Invasive Monitoring of Stress in Farm Animals, Volume IView all 8 articles
Heart rate variability (HRV), heart beat-to-beat variations, is a measure of cardiac autonomic functions, and HRV monitoring using sensor technology can be a non-invasive way to assess stress of animals. The objective of this study was to investigate the effect of dietary vitamin A (VA) restriction to enhance beef quality on the physiological status of fattening steers by HRV analysis. Six Japanese Black steers were equally allocated into VA-restricted (RES) and VA-supplemented (SUP) groups. The RES steers were fed VA-restricted diets from 11 to 20 months of age. The inter-beat intervals and blood VA concentration were measured at 18 and 24 months of age. HRV parameters in time, frequency, and non-linear domains were calculated using the inter-beat intervals. Blood VA concentration was significantly lower in RES steers than in SUP steers at 18 months of age (P < 0.05) but did not differ between the groups at 24 months of age. The HRV analysis indicated greater sympathetic and lower parasympathetic activities in RES steers than in SUP steers (P < 0.05). However, there were significant interactions of the group and age on HRV parameters (P < 0.05), indicating that although RES steers at 18 months of age might suffer from slight stress, the response could recover to a level similar to SUP steers at 24 months of age by the increase in blood VA concentration.
Healthy cardiac variation is characterized by irregular time intervals between heartbeats (von Borell et al., 2007). The fluctuation of inter-beat intervals is expressed as heart rate variability (HRV), which reflects the sympathetic and vagal activities of the autonomic nervous system. As a decrease in HRV can be caused by an increase in sympathetic activity and/or withdrawal of vagal activity, HRV is one of good indicators for assessing autonomic nervous system in response to various stressors (Acharya et al., 2006). Until now, a variety of studies have investigated internal and external stress factors that affect HRV in farm animals: e.g., diseases such as lameness (Kovács et al., 2015), diarrhea (Mohr et al., 2002), and bovine spongiform encephalopathy (Konold et al., 2011), and heat stress (Kitajima et al., 2021). Hence, HRV monitoring has gained notable attention as a non-invasive technique for evaluating the stress levels of animals from the point of view of animal welfare (Kovács et al., 2014).
Intramuscular fat deposition and the desaturation of fat have a major influence on the quality of beef; high intramuscular fat and monounsaturated fatty acid content are properties that can attract premium prices for beef (Siebert et al., 2006). Some researchers have reported that restricting dietary vitamin A (VA) can enhance beef marbling performance (Torii et al., 1996; Oka et al., 1998; Ward et al., 2012) and increase monounsaturated fatty acid content in adipose tissue (Siebert et al., 2006; Gorocica-Buenfil et al., 2007). Consequently, dietary VA restriction for fattening cattle has become a well-known beef production technique especially in Japan (Irie et al., 2006; Oishi et al., 2011; Han et al., 2014). However, VA is an essential nutrient for the growth and development of animal tissues (Villarroya et al., 1999). In Japan, NARO (2008) indicated that blood vitamin A levels below 30 IU/dL increase the risk of vitamin A deficiency in beef cattle, and the animals can show VA deficiency symptoms when the blood VA concentration is too low (Nade et al., 2003; NARO, 2008). Moreover, vitamin A might also be a physiologically important antioxidant that reduces cardiovascular disease (Palace et al., 1999). The autonomic nervous system regulates heartbeats; therefore, it may be possible that vitamin A restriction affects the autonomic nervous system as a feedback control. Although clear clinical symptoms of VA deficiency are now less likely to be seen because of the establishment of dietary VA control in Japan, VA restriction might still be a cause of subclinical health problems in beef cattle (Uenishi et al., 2021). Therefore, the present study investigated the effect of dietary vitamin A restriction during the fattening period on the autonomic nervous system of Japanese Black (a breed of Wagyu) cattle using HRV analysis.
The experiment was conducted with the same design as that of our previously study (Uenishi et al., 2021) at the Aichi Agricultural Research Center (35° 16′ N, 137° 07′ E) in Japan. Six clinically healthy Japanese Black fattening steers with similar body weights (BW) at the start of the experiment were equally allocated into two experimental groups (three for each): VA-restricted (RES) and VA-supplemented (SUP) groups. Each group was managed in pens under conventional housing conditions and were fed individually with door feeder systems three times a day. The steers had ad libitum access to water and mineral blocks. The feeding regimens for the two groups were the same as those reported by Uenishi et al. (2021). During the growth stage (age 11–13 months), diets were gradually replaced by those fed during the fattening stage for adaptation. During the fattening stage, the steers were fed 10 kg of purchased concentrate for fattening cattle (12.5 and 74.0% of crude protein and total digestible nutrient per dry matter content, respectively) and 1.5 kg of rice straw per day from 14 to 19 months of age and 10 kg of the concentrate and 1 kg of rice straw per day after 20 months of age. The VA concentration in the concentrate was gradually reduced from 11 to 14 months of age during the growth stage for both groups. For RES steers, the VA concentration was maintained at a low level (316 IU/kg of the concentrate) until 20 months of age, and then increased to the same level as that of SUP steers for the rest of the fattening period. The SUP group, the steers were fed the concentrate mixed with supplemented VA with a higher level of VA (2,316 IU/kg of the concentrate) during the fattening period. At 21 months of age, the steers were intramuscularly administered with 1,500 IU/kg BW of VA. A trained technician and a veterinarian observed the steers daily to check their health condition. All of the animal experiments were approved by the Animal Use and Care Committee of the Aichi Agricultural Research Center (Permit Numbers: 29–95).
Blood VA concentrations, inter-beat intervals, and three-dimensional acceleration of the steers were measured at 18 months of age (RES: 573 ± 28.4 kg BW, SUP: 601 ± 31.6 kg BW) in spring 2017 and at 24 months of age (RES: 697 ± 48.0 kg BW, SUP: 749 ± 40.9 kg BW). Blood samples for steers in each group were collected by venipuncture, and after centrifugation the plasma was stored at −30°C with shading until VA analysis. Then, the plasma VA concentration was determined for each sample using high-performance liquid chromatography. The inter-beat interval data (ms) of the steers were measured for 24 h using heart rate sensors (myBeat WHS-1, Union Tool Co., Japan). Two sensor electrodes were placed on the steer's right shoulder and left anterior thorax, fixed to the body of the steer using a homemade chest belt, similar to the method described by Miwa et al. (2015). The electrode sites of the steers were roughly shaved and covered with a conductive gel to maintain electrode contact. Tri-axial acceleration data of the steers were collected simultaneously using acceleration loggers (USB Accelerometer X6-1A, X6-2A, and X6-1D, Gulf Coast Data Concepts, Waveland, MS, USA), placed on the back of the steers when the heart rate sensors were fixed. The data were recorded at 10 Hz (for X6-1A and X6-2A) or 12 Hz (for X6-1D) with 16-bit resolution. Usually, a change in physical activity levels is considered to be one of the major factors to affect HRV when the change occurs during the period of analysis (Bernardi et al., 1996). Therefore, in the present study, the effect of physical activity on HRV was considered using the vectorial dynamic body acceleration (VeDBA) (Qasem et al., 2012) calculated from the acceleration data.
The Kubios HRV 2.2 (Kubios LTD, Finland; Tarvainen et al., 2014) was used for the analysis of inter-beat interval data, and HRV parameters were calculated with 5 min intervals based on the Task Force of the European Society of Cardiology, North American Society of Pacing and Electrophysiology (1996). Artifacts of the data were removed using the artifact correction algorithm of the software (Tarvainen et al., 2014). In addition, the 5 min interval data including 5 min averaged inter-beat interval values outside the three-sigma range of individual datasets were removed. A total of 3,335 inter-beat interval data, 827 and 843 intervals for RES steers, and 823 and 842 intervals for SUP steers at 18 and 24 months of age, respectively, were obtained for the analysis. From the preprocessed data, the mean heart rate (HR) and the following three HRV parameters per 5 min were calculated: the root mean square of successive inter-beat interval differences (RMSSD) as a time domain parameter, the high-frequency component (HF) from fast Fourier transformation as a frequency domain parameter, and the percentage of determinism (%DET) of recurrence quantification analysis (Eckmann et al., 1987) as a non-linear parameter. The RMSSD is a primary measure of time domain analysis, and the increase in RMSSD can be interpreted as an activation of the vagal tone. Similarly, an increase in HF is shown under the predominance of vagal activity. In the present study, the range of HF was defined as 0.20–0.58 Hz with reference to von Borell et al. (2007). The %DET can show the richness of deterministic structures of inter-beat intervals, and an increase in this parameter expresses a decrease in HRV (Dabiré et al., 1998), which can be interpreted as the predominance of the sympathetic activity.
Blood VA concentration was analyzed using a linear model with the fixed effects of the groups of VA treatment, months of age, their interaction, and animals nested within the groups. In addition, the effects of the difference in VA groups on HR and HRV parameters were analyzed using a similar model, adding naturally log-transformed VeDBA value as a covariate. Differences were evaluated by the least squares means with the Tukey–Kramer comparison test with significant level (P <0.05). All analyses were performed using PROC GLM in SAS 9.3 (SAS Institute Inc, 2008).
Blood VA concentration for steers was significantly lower in RES steers (54.7 IU/dL) than in SUP steers (92.0 IU/dL) at 18 months of age (P < 0.05) but was not significantly different between the two groups at 24 months of age (77.7 and 68.7 IU/dL for RES and SUP, respectively). All VA concentrations were above the recommended minimum value given in the Japanese Feeding Standard for Beef Cattle (30 IU/dL; NARO, 2008) and no steers showed any clinical symptoms of VA deficiency, indicating that dietary VA restriction was able to be controlled as expected.
The effects of VA treatment and age on HR and HRV parameters are presented in Table 1. The effect of VA treatment was significant for HR, RMSSD, and %DET (P < 0.05). Lower RMSSD and higher %DET in RES steers than in SUP steers indicated higher sympathetic and lower vagal activities, whereas HF was not significantly different between the VA treatments. As for the effect of age, HR and RMSSD were significantly higher at 24 months than at 18 months of age (P < 0.05). In addition, the covariate of VeDBA was significant for HR, RMSSD, and %DET (P < 0.05). In previous studies, the effect of physical activity quantified by VeDBA was significant for HR and all HRV parameters when HRV of animals was evaluated under free-moving conditions (Oishi et al., 2018; Kitajima et al., 2021). Except for HF, the present results were similar to those of the previous studies, despite the fact that all steers were managed in relatively small feedlot pens under housing conditions in the present study.
Table 1. The effects of low vitamin A feeding and age on mean heart rate (HR) and heart rate variability (HRV) parameters in the experimental Japanese Black fattening steers.
The interaction effect of VA treatment and age was also significant for HR and all HRV parameters investigated (P < 0.05), thus simple main effects of VA treatment and age on HR and HRV parameters were analyzed (Figure 1). At 18 months of age, HR and RMSSD was lower and %DET was higher in RES steers than in SUP steers (P < 0.05). However, no significant differences in RMSSD and %DET were found between the VA treatment groups at 24 months of age. Furthermore, HR, RMSSD, and HF significantly increased (P < 0.05) from 18 to 24 months of age in RES steers, whereas no significant differences in the parameters were found between the two months of age in SUP steers. The results of the present study indicated that HRV was reduced in RES steers relative to SUP steers during the VA restriction period, but HRV was increased to a level similar to that in SUP steers at 24 months of age. In contrast, our previous study showed that the influence of latent VA deficiency caused by dietary VA restriction on the physical activity of fattening steers at 18 months of age still remained at 24 months of age despite the increase in blood VA concentration (Uenishi et al., 2021). One possible reason for the different results is that RES steers in the present study might be desensitized to long-term mild stress caused by the latent VA deficiency. Exposure to a stressor of low intensity causes desensitization to that particular stressor; it is possible for a stress system to stop responding, not due to actual adaptation, but because of some mechanism that suppresses the response (Ladewig, 2000). For example, Ladewig and Smidt (1989) reported that increased secretion of cortisol in bulls due to tethering disappeared and the return of the basic secretion to normal occurred at the adrenal level, which could involve desensitization of the cells, although the bulls did not show any observable behavioral adaptations. Therefore, the difference between the results of the present study and those of Uenishi et al. (2021) implied that, in cattle recovered from some physiological stress such as latent VA deficiency, the physiological stress response shown by a decrease in HRV might be diminished more quickly than the behavioral stress response shown by a change in physical activity.
Figure 1. Interaction effects of month of age and vitamin A treatment on mean heart rate (HR) and heart rate variability (HRV) parameters of the experimental Japanese Black fattening steers. RES: vitamin A restricted group, SUP: vitamin A supplemented group. RMSSD: square root of the mean squared differences of successive inter-beat intervals (ms), HF: normalized power of the high frequency band (n.u.), and %DET: the percentage of recurrent points that appear in sequence (%). Least square means and standard error bars with different symbols differ as **P < 0.01, *0.01 ≤ P < 0.05, and † 0.05 ≤ P < 0.10.
The results of the present study indicated that feeding VA-restricted diets could lead to a decrease in HRV in fattening steers, suggesting the effect of VA intake on the autonomic nervous system of animals. Hence, it can be hypothesized that based on the characteristics of VA, it may act on the autonomic nervous system through several pathways. First, β-carotene (VA precursor) has antioxidant properties similar to vitamin E, and it acts as an oxidative stress inhibitor (Miller et al., 1993). Oxidative stress is a major factor that leads to inflammatory responses in cattle (Mavangira and Sordillo, 2018). From a meta-analysis of human studies, Williams et al. (2019) reported that there were negative relationships between HRV and markers of inflammation, indicating the importance of the autonomic nervous system in regulating inflammatory responses. Therefore, VA deficiency likely has a negative effect on the autonomic nervous system, with an increase in inflammatory responses caused by oxidative stress. Second, it has been reported that VA metabolites can affect some aspects of adaptive immune response (Mora et al., 2008), and that both the autonomic nervous system and neuroendocrine outflow via the pituitary provide biologically active molecules capable of interacting with cells of the immune system (Ader et al., 1995). Therefore, there may also be some inter-relationships between VA metabolites and the autonomic nervous system via neural-immune interactions. Due to the very small sample size of the present study, further studies will be needed to explore the physiological response of VA restriction on the autonomic nervous system in fattening cattle.
The present study firstly investigated the effects of VA restriction on HRV in fattening steers. During the VA restriction period, steers under VA restriction showed significantly lower RMSSD and higher %DET, indicating that the steers had slightly higher sympathetic activity and lower vagal activity than steers supplemented VA. However, after the restriction and recovery of blood VA status, the autonomic function could return to a similar level between the steers. Thus, it can be concluded that feeding VA-restricted diets during mid-fattening period for the purpose of producing meat with high intramuscular fat and monounsaturated fatty acid content is one of the potential factors that decreases the HRV of steers, but the physiological stress evaluated by HRV status can be relieved after the end of the VA restricted feeding during the late-fattening period.
The dataset analyzed in this study will be made available on request to the corresponding author.
All of the animal experiments were approved by the Animal Use and Care Committee of the Aichi Agricultural Research Center (Permit Numbers: 29–95).
KK and KO designed the study, collected and analyzed the data, and wrote the manuscript. TK collected the data and helped to write the manuscript. SU, YY, and KS collected the data. HK and HH helped to write the manuscript. All authors discussed the results, contributed to manuscript revisions, and approved the manuscript for publication.
This study was supported by Grants-in-Aid for Scientific Research (C) (grant numbers: 15K07705 and 19K06352) from the Japan's Society for the Promotion of Science.
The authors declare that the research was conducted in the absence of any commercial or financial relationships that could be construed as a potential conflict of interest.
All claims expressed in this article are solely those of the authors and do not necessarily represent those of their affiliated organizations, or those of the publisher, the editors and the reviewers. Any product that may be evaluated in this article, or claim that may be made by its manufacturer, is not guaranteed or endorsed by the publisher.
Acharya, U. R., Joseph, K. P., Kannathal, N., Lim, C. M., and Suri, J. S. (2006). Heart rate variability: a review. Med. Biol. Eng. Comput. 44, 1031–1051. doi: 10.1007/s11517-006-0119-0
Ader, R., Cohen, N., and Felten, D. (1995). Phychoneuroimmunology: interactions between the nervous system and the immune system. Lancet 345, 99–103. doi: 10.1016/S0140-6736(95)90066-7
Bernardi, L., Valle, F., Coco, M., Calciati, A., and Sleight, P. (1996). Physical activity influences heart rate variability and very-low-frequency components in Holter electrocardiograms. Cardiovasc. Res. 32, 234–237. doi: 10.1016/0008-6363(96)00081-8
Dabiré, H., Mestivier, D., Jarnet, J., Safar, M. E., and Chau, N. P. (1998). Quantification of sympathetic and parasympathetic tones by nonlinear indexes in normotensive rats. Am. J. Physiol. Heart Circ. Physiol. 275, 1290–1297. doi: 10.1152/ajpheart.1998.275.4.H1290
Eckmann, J. P., Kamphorst, S. O., and Ruelle, D. (1987). Recurrence plots of dynamical systems. Europhys. Lett. 4, 973–977. doi: 10.1209/0295-5075/4/9/004
Gorocica-Buenfil, M. A., Fluharty, F. L., and Loerch, S. C. (2007). Effect of vitamin A restriction on carcass characteristics and immune status of beef steers J. Anim. Sci. 86, 1609–1616. doi: 10.2527/jas.2007-0241
Han, S., Kondo, N., Ogawa, Y., Fujiura, T., Tanigawa, S., Shiigi, T., et al. (2014). Feasibility of pupillary light reflex analysis to identify vitamin A deficiency in Japanese black cattle. Comput. Electron. Agric. 108, 80–86. doi: 10.1016/j.compag.2014.07.005
Irie, M., Inno, Y., Ishizuka, Y., Nishioka, T., and Morita, T. (2006). Vitamins A and E in carcass fat from Japanese Black and F1 cross cattle. Asian Aust. J. Anim. Sci. 19, 1266–1270. doi: 10.5713/ajas.2006.1266
Kitajima, K., Oishi, K., Miwa, M., Anzai, H., Setoguchi, A., Yasunaka, Y., et al. (2021). Effects of heat stress on heart rate variability in free-moving sheep and goats assessed with correction for physical activity. Front. Vet. Sci. 8:658763. doi: 10.3389/fvets.2021.658763
Konold, T., Bone, G. E., and Simmons, M. M. (2011). Time and frequency domain analysis of heart rate variability in cattle affected by bovine spongiform encephalopathy. BMC Res Notes. 4:259. doi: 10.1186/1756-0500-4-259
Kovács, L., Jurkovich, V., Bakony, M., Póti, P., Szenci, O., and Tözsér, J. (2014). Welfare assessment in dairy cattle by heart rate and heart rate variability- Literature review and implications for future research. Animal 8, 316–330. doi: 10.1017/S1751731113002140
Kovács, L., Kézér, F. L., Jurkovich, V., Kulcsár-Huszenicza, M., and Tozsér, J. (2015). Heart rate variability as an indicator of chronic stress caused by lameness in dairy cows. PLoS ONE 10:e0134792. doi: 10.1371/journal.pone.0134792
Ladewig, J. (2000). “Chronic intermittent stress: a model for the study of long-term stressors,” in The Biology of Animal Stress, eds G. P. Moberg, and J. A. Mench (Wallingford: CABI Publishing), 159–169. doi: 10.1079/9780851993591.0159
Ladewig, J., and Smidt, D. (1989). Behavior, episodic secretion of cortisol, and adrenocortical reactivity in bulls subjected to tethering. Horm. Behav. 23, 344–360. doi: 10.1016/0018-506X(89)90048-2
Mavangira, V., and Sordillo, L. M. (2018). Role of lipid mediators in the regulation of oxidative stress and inflammatory responses in dairy cattle. Res. Vet. Sci. 116, 4–14. doi: 10.1016/j.rvsc.2017.08.002
Miller, J. K., Brzezinska-Slebodzinska, E., and Madsen, F. C. (1993). Oxidative stress, antioxidants, and animal function. J. Dairy Sci. 76, 2812–2823. doi: 10.3168/jds.S0022-0302(93)77620-1
Miwa, M., Oishi, K., Nakagawa, Y., Maeno, H., Anzai, H., Kumagai, H., et al. (2015). Application of overall dynamic body acceleration as a proxy for estimating the energy expenditure of grazing farm animals: relationship with heart rate. PLoS ONE 10:e0128042. doi: 10.1371/journal.pone.0128042
Mohr, E., Langbein, J., and Nürnberg, G. (2002). A noninvasive approach to measure stress in calves and cows. Physiol. Behav. 75, 251–259. doi: 10.1016/S0031-9384(01)00651-5
Mora, J. R., Iwata, M., and von Andrian, U. H. (2008). Vitamin effects on the immune system: vitamins A and D take centre stage. Nat. Rev. Immunol. 8, 685–698. doi: 10.1038/nri2378
Nade, T., Hirabara, S., Okumura, T., and Fujita, K. (2003). Effects of vitamin A on carcass composition concerning younger steer fattening of Wagyu cattle. Asian Aust. J. Anim. Sci. 16, 353–358. doi: 10.5713/ajas.2003.353
NARO (2008). Japanese Feeding Standard for Beef Cattle. National Agriculture and Food Research Organization. Tokyo: Livestock Industry Association.
Oishi, K., Himeno, Y., Miwa, M., Anzai, H., Kitajima, K., Yasunaka, Y., et al. (2018). Correcting the activity-specific component of heart rate variability using dynamic body acceleration under free-moving conditions. Front. Physiol. 9:1063. doi: 10.3389/fphys.2018.01063
Oishi, K., Kumagai, H., and Hirooka, H. (2011). Application of the modified feed formulation to optimize economic and environmental criteria in beef cattle fattening systems with food by-products. Anim. Feed Sci. Technol. 165, 38–50. doi: 10.1016/j.anifeedsci.2011.02.015
Oka, A., Maruo, Y., Miki, T., Yamasaki, T., and Saito, T. (1998). Influence of vitamin A on the quality of beef from the Tajima strain of Japanese Black cattle. Meat Sci. 48, 159–167. doi: 10.1016/S0309-1740(97)00086-7
Palace, V. P., Khaper, N., Qin, Q., and Signal, P. K. (1999). Antioxidant potentials of vitamin A and carotenoids and their relevance to heart disease. Free Radical Biol. Med. 26, 746–761. doi: 10.1016/S0891-5849(98)00266-4
Qasem, L., Cardew, A., Wilson, A., Griffiths, I., Halsey, L. G., Shepard, E. L. C., et al. (2012). Tri-axial dynamic acceleration as a proxy for animal energy expenditure; should we be summing values or calculating the vector? PLoS ONE 7:e31187. doi: 10.1371/journal.pone.0031187
Siebert, B. D., Kruk, Z. A., Davis, J., Pitchford, W. S., Harper, G. S., and Bottema, C. D. K. (2006). Effect of low vitamin A status on fat deposition and fatty acid desaturation in beef cattle. Lipids 41, 365–370. doi: 10.1007/s11745-006-5107-5
Tarvainen, M. P., Niskanen, J.-P., Lipponen, J. A., Ranta-acho, P. O., and Karjalainen, P. A. (2014). Kubios HRV – Heart rate variability analysis software. Comput. Methods Programs Biomed. 113, 210–220. doi: 10.1016/j.cmpb.2013.07.024
Task Force of the European Society of Cardiology North American Society of Pacing and Electrophysiology. (1996). Heart rate variability: standards of measurement, physiological interpretation, and clinical use. Circulation 93, 1043–1065.
Torii, S., Matsui, T., and Yano, H. (1996). Development of intramuscular fat in Wagyu beef cattle depends on adipogenic or antiadipogenic substances present in serum. Anim. Sci. 63, 73–78. doi: 10.1017/S1357729800028307
Uenishi, S., Oishi, K., Kojima, T., Kitajima, K., Yasunaka, Y., Sakai, K., et al. (2021). A novel accelerometry approach combining information on classified behaviors and quantified physical activity for assessing health status of cattle: a preliminary study. Appl. Anim. Behav. Sci. 235:105220. doi: 10.1016/j.applanim.2021.105220
Villarroya, F., Giralt, M., and Iglesias, R. (1999). Retinoids and adipose tissues: metabolism, cell differentiation and gene expression. Int. J. Obes. 23, 1–6. doi: 10.1038/sj.ijo.0800799
von Borell, E., Langbein, J., Després, G., Hansen, S., Leterrier, C., Marchant-Forde, J., et al. (2007). Heart rate variability as a measure of autonomic regulation of cardiac activity for assessing stress and welfare in farm animals – a review. Physiol. Behav. 92, 293–316. doi: 10.1016/j.physbeh.2007.01.007
Ward, A. K., McKinnon, J. J., Hendrick, S., and Buchanan, F. C. (2012). The impact of vitamin A restriction and ADH1C genotype on marbling in feedlot steers. J. Anim. Sci. 90, 2476–2483. doi: 10.2527/jas.2011-4404
Keywords: fattening steers, heart rate variability, Japanese Black, marbling, vitamin A
Citation: Kitajima K, Oishi K, Kojima T, Uenishi S, Yasunaka Y, Sakai K, Kumagai H and Hirooka H (2022) An Assessment of Stress Status in Fattening Steers by Monitoring Heart Rate Variability: A Case of Dietary Vitamin A Restriction. Front. Anim. Sci. 2:799289. doi: 10.3389/fanim.2021.799289
Received: 21 October 2021; Accepted: 29 November 2021;
Published: 27 January 2022.
Edited by:
Edward Narayan, The University of Queensland, AustraliaReviewed by:
Igor Victorovich Lakhno, V. N. Karazin Kharkiv National University, UkraineCopyright © 2022 Kitajima, Oishi, Kojima, Uenishi, Yasunaka, Sakai, Kumagai and Hirooka. This is an open-access article distributed under the terms of the Creative Commons Attribution License (CC BY). The use, distribution or reproduction in other forums is permitted, provided the original author(s) and the copyright owner(s) are credited and that the original publication in this journal is cited, in accordance with accepted academic practice. No use, distribution or reproduction is permitted which does not comply with these terms.
*Correspondence: Kazato Oishi, b2lzaGkua2F6YXRvLjNtQGt5b3RvLXUuYWMuanA=
Disclaimer: All claims expressed in this article are solely those of the authors and do not necessarily represent those of their affiliated organizations, or those of the publisher, the editors and the reviewers. Any product that may be evaluated in this article or claim that may be made by its manufacturer is not guaranteed or endorsed by the publisher.
Research integrity at Frontiers
Learn more about the work of our research integrity team to safeguard the quality of each article we publish.