- Department of Animal Science, Iowa State University, Ames, IA, United States
Genetic selection for fast growth rate and high breast muscle yield in modern broilers has unintended effects on animal welfare and behavior, namely in terms of inactivity and leg disorders. We hypothesized that exercise stimulated through environmental enrichment could positively stimulate pen-wide activity and improve bird welfare. The study objectives were to implement a laser enrichment device to motivate active and feeding behaviors throughout the pen. Twelve hundred Ross 708 broilers were randomly assigned to enrichment (LASER; laser enrichment, or CON; no laser enrichment) for 49 d. Seventy focal birds were randomly assigned to 14 video-recorded pens for behavioral analysis, including focal bird home pen behavior and walking distance. Pen-wide activity was also measured during the 4-min laser periods, four times daily, d0–8, and 1 day weekly, wk 1–6. Focal birds were gait scored wk 1–6, and were euthanized on d42 for tibia bone mineral content, density, and bone breaking strength analysis. Time spent active was increased in LASER-enriched birds compared to CON on wk 3–5 by up to 214% (wk 4), and percent of time at the feeder was increased in LASER-enriched birds by 761% on wk 4 (P < 0.05). Peak percent of birds following the laser (LASER-enriched pens only) was observed on d0 (8.52%). Over wk 1–6, peak laser-following behavior was observed on wk 3 (3.07% of birds). Percent of birds moving during laser periods was increased in LASER-enriched pens on d0, 1, 2, 6, 7, and 8, with a percent increase of 68.7% observed on d1 (P < 0.05). Percent of birds moving (laser-following or not) was increased on wk 1, 3, and 4 in LASER-enriched pens, with an increase of 69.7% observed on wk 4 (P < 0.05). No differences were found in tibia measures. These data indicate that laser enrichment stimulated voluntary locomotion through wk 5 and laser-following behavior through wk 6, and that the relatively small percent of birds actively following the laser stimulated pen-wide movement above the level of the CON through wk 4 on study.
Introduction
Modern commercial broilers reach market weight in as little as 4–6 weeks due to genetic selection for feed efficiency and significantly improved growth rates, resulting in a high breast muscle-yielding phenotype five times heavier than birds 70 years ago (Havenstein et al., 2003; Zuidhof et al., 2014; Soglia et al., 2016). This growth rate has led to disproportionate upper body weight and an unnatural center of gravity in broiler birds (Havenstein et al., 2003). This outcome predisposes the birds to well-established animal welfare issues, and more recently, meat quality concerns. Primarily among broiler welfare issues are leg disorders affecting nearly one-third of commercial birds, who show reduced ability to walk and access resources (Knowles et al., 2008; Bassler et al., 2013). Lame broilers aged 6–7 weeks spend close to 90% of their daily time budget inactive, and spend only 1.5% of time active, compared to 3.3% of time active in sound birds (Weeks et al., 2000). This extended time spent lying in contact with the litter can result in contact dermatitis, and ultimately culls if the birds cannot access feed and water (Weeks et al., 2000; Nääs et al., 2009; Bassler et al., 2013). Culls are estimated to cause 2% loss in a $30 billion industry (Dunkley, 2007; USDA., 2019). Leg lameness is also recognized as a significant animal welfare concern by the National Chicken Council, whose guidelines recommend gait scoring 100 broilers/flock on-farm using a validated, three-point scoring system (National Chicken Council, 2020).
Leg disorders are exacerbated by characteristic inactivity (Weeks et al., 2000), and lack of exercise hinders leg skeletal development and weight-bearing ability (Lanyon, 1993; Rath et al., 2000). In other words, skeletal disorders partially caused by lack of exercise cause lameness, which itself causes inactivity. Hence, past broiler welfare research has studied tools to increase bird physical activity. Forced exercise for 20 min sessions utilizing treadmills was successful in improving broiler walking ability (Reiter and Bessei, 2009). Additionally, increasing distance or implementing barriers between resources has been used to necessitate increased locomotion (Reiter and Bessei, 2009; Ventura et al., 2012; Ruiz-Feria et al., 2014). Non-resource-based methods of environmental enrichment have also been tested with varying degrees of success. Ambient red lighting served to stimulate activity (Prayitno et al., 1997), but resulted in reduced growth performance. Moving, colorful spotlights projected onto the pen floor and scattered wheat were unsuccessful in stimulating broiler movement (Bizeray et al., 2002). Sand bedding provided to broilers as an alternative to wood shavings resulted in reduced active behaviors (Shields et al., 2005). Elevated platforms introduced by Tahamtani et al. (2020) reduced walking ability compared to other treatments. Platform perches at a height of 20 cm as well as dust baths held in steel rectangles 7.62 cm high were provided to broilers by Baxter et al. (2019), and while percent of birds perching was not reported and dust-baths were used, no effect of either enrichment was observed on broiler activity. Elevated platforms (30 cm) provided by Norring et al. (2016) were used more than high or low perch options (30 vs. 10 cm), but likewise did not increase activity. Overall, stimulating voluntary, active behavior in broilers has proven challenging.
A laser enrichment device was implemented in a previous study using 1,200 Ross 308 broilers fed a simple practical diet by the current authors with the hypothesis that insect-sized, red moving laser dots would visually motivate broiler exercise throughout grow-out. The device proved capable of increasing broiler locomotion through wk 5 of life, by up to 157% increased activity (wk 3) and 367.5 cm increased walking distance (wk 2) compared to the control (Meyer et al., 2020). Therefore, the current study objectives were to confirm the ability of the laser enrichment device to motivate broiler activity and feeding behavior, to document effects on pen-wide movement, and to quantify walking ability and tibia quality outcomes.
Materials and Methods
All live bird procedures were approved by the Iowa State University Institutional Animal Care and Use Committee, IACUC #19-322.
Animals
One thousand two hundred straight-run Ross 708 broiler chicks were obtained from a commercial hatchery (Welp Hatchery, Bancroft, IA, U.S.) on day of hatch and transported to the Poultry Research and Teaching Unit at Iowa State University for a 7-wk grow-out experiment in floor pens. Upon arrival, 30 birds were randomly assigned to each pen and weighed (average bird BW on d0: 35.98 ± 0.51 g). Seventy birds of the 1,200 total were randomly selected as focal birds (n = 5 birds/pen in 14 video-recorded pens; 7 pens/enrichment treatment), identified with wing-bands for tibia sampling, and marked with unique animal-safe gel food coloring for behavioral observations (red, blue, green, purple, and black; Wilton, Woodridge, IL, U.S.). Food coloring was applied to a cotton ball, rubbed on the back of the chick's head and neck, and reapplied on an as-needed basis (no more than once weekly was necessary). The red-colored bird was used for broiler home pen behavior analysis, the blue-colored bird for walking distance analysis, and remaining focal birds were used for gait scoring and tibia collection. This means of identifying focal birds has been used by the authors previously (Meyer et al., 2020) and proved an effective way to recognize birds on video that did not alter inter-bird behavior (did not induce pecking, aggression, injury).
Housing and Feeding
Birds were housed in 40 pens (1.22 by 2.44 m), 30 birds per pen (0.10 m2/bird), replicated across two rooms in the barn. One room contained 20 enrichment device-containing pens (LASER), and the other contained 20 pens not containing laser enrichment (CON), with an anteroom separating the rooms so no crossover laser exposure to the CON was possible. Environmental conditions and management were kept the same across rooms. Approximately 10 cm deep fresh wood shavings provided bedding over the solid concrete floor, and PVC pipe dividers with mesh walls (1.22 m height) separated pens within each room. High and low temperatures and humidity were monitored daily. Birds were gradually adjusted from 24 h light on d0, defined as day of placement (30–40 lux) to 20 h light (20–30 lux) from d8–49. Chicks were brooded with 2-heat lamps/pen (22.9 cm reflectors with porcelain socket) using 125-watt heat bulbs (Sylvania, Wilmington, MA, U.S.) for the first week.
Broilers were assigned to one of two diets and evenly split across the LASER and the CON rooms of the barn (n = 20 pens/diet): a control diet and the control diet + 2.5% Spirulina algae ingredient. Effects of diet are not discussed in the current work as they did not significantly affect bird behavior or tibia quality outcomes, therefore diets are pooled by CON or LASER treatment. Dietary effects on performance and breast meal quality are reported in a companion paper (Meyer et al., unpublished companion paper, submitted to Frontiers). Diets were formulated according to Ross 708 broiler guidelines for starter (d0–d14), grower (d14–28), finisher 1 (d28–42), and finisher 2 (d42–49) performance phases. Birds were fed ad libitum out of a hanging feeder (BRHF151; Brower Equipment, Houghton, IA, U.S.) gradually raised to accommodate bird height. Water was provided ad libitum from a hanging nipple water line (~8 nipples/pen). Mortality throughout the trial was 3.8% in the CON room and 2.3% in the LASER room; increased mortality in the CON room was associated with waterline issues in a few pens during the first week on trial.
Laser Enrichment Device
Ten laser enrichment devices, previously described by Meyer et al. (2019, 2020), were placed over 20 pens; each device was designed and calibrated to cover two adjoining pens (approximate floorspace of 5.95 m2). The enrichment device consisted of two independent red 650 nm lasers contained within a 20.5 by 20.5 cm metal box with a glass bottom mounted on a custom-designed structure made of three wooden beams (2.4 m height) raised above the pens. The lasers projected in the direction of the pen floor and moved in a random pattern at a variable speed between 7.6 and 30.5 cm/s for 4-min “laser periods” at: 05:30, 11:30, 17:30, and 23:30 daily for the entirety of the trial. Barn and research staff did not enter the LASER nor CON rooms of the barn during the 4 h containing the four laser-periods each day (Image of laser device mounted over two pens available as a Supplementary Figure 1).
Video Recording
Seventy focal birds (n = 5/camera pen) were randomly assigned to seven pens per room of the barn (n = 14 camera pens: 7 LASER, 7 CON) equipped with 1 Sony HDR-CX440 Handycam (Sony Corp. of America, New York, NY, U.S.) each. The camera pens were randomly selected within rooms/enrichment treatments; hence the unequal distribution of diet treatments recorded. Cameras were affixed above each pen using brackets adjusted to capture the entire pen. Filming occurred in real-time (30 fps) for the first 10 d of the trial (d0–9) and once weekly for the remainder. Three video observers were trained to collect behavior data by an individual with 2 years animal behavior observation experience to 90% agreeability. During training, each observers spent one-on-one time with the trainer using the 4-min laser period video clips from any day recorded (d0–9, 16, 23, 30, and 37) and given additional practice videos to score. Practice videos were completed and scored against the trainer's answers for inter-reliability. All clips recorded were analyzed for the entirety of the enrichment period in LASER and CON pens. Video observers were not able to be blinded to enrichment treatment; either the laser dots or the supporting structure were visible in the videos.
Broiler Home Pen Behavior
Trained observers watched the red-colored focal bird (n = 14) during the 4-min laser periods on d0–9, 16, 23, 30, and 37, and categorized bird behavior continuously using a pre-determined ethogram (Meyer et al., 2020) for both frequency and duration (s; Table 1). Duration was converted to percent of time spent on each behavior per 4-min period.
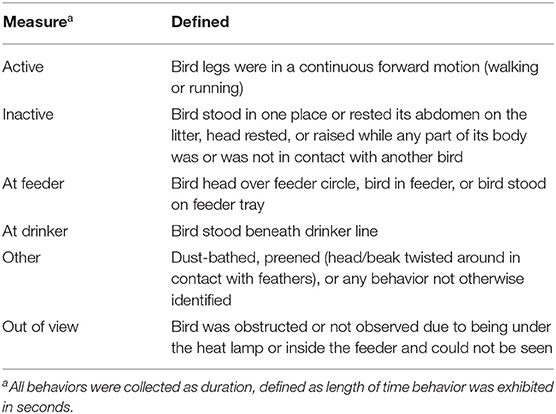
Table 1. Broiler home pen behavior ethogram; focal bird behavior was measured continuously during 4-min laser periods, 4 times daily at 05:30, 11:30, 17:30, and 23:30 for d0–9, 16, 23, 30, and 37.
Walking Distance
The distance walked by the blue-colored focal bird (n = 14) was measured over the 4-min laser periods (d0–9, 16, 23, 30, and 37), using methods described and validated in Meyer et al. (2020). Briefly, the focal bird movement was recorded by tracing the bird's route on screen using a clear sheet protector, and subsequently measured using the custom ruler tool on Adobe Photoshop (Adobe Systems Inc, San Jose, CA, U.S.). A known length within the pen (58.4 cm between 2 water line segments, measured on-farm) was used to standardize the custom ruler tool on Photoshop, which measures in pixels. The tool was used to convert the number of pixels measured on screen to approximate cm on-farm. This was repeated for each min and summed for the total distance walked per laser period.
Laser-Following Behavior and Pen-Wide Movement
All birds in each video-recorded pen (n = 30 birds pen, 7 pens/treatment) were categorized into two pen-wide behavior categories during 4-min laser periods (d0–9, 16, 23, 30, and 37), including percent of birds following the laser (in laser-enriched pens only) and percent of birds otherwise physically moving, both calculated using the total number of birds in each pen. “Laser-following” behavior was defined as “a bird with head and body orientated toward laser dot(s) on the floor of pen with legs in forward motion at least one time during the minute being analyzed.” A bird otherwise moving (in LASER and CON pens) was defined as “any bird with legs in forward motion; walking or running at least one time during minute being analyzed who is not directly following or moving in the direction of the laser dot(s). May be disturbed by another bird, walking to feeder or drinker, any bird moving while the laser is not visible in the pen being recorded, or any bird moving in a control pen.” In LASER-enriched pens, laser-following and otherwise moving behaviors were summed for total percent of birds moving in order to compare with total percent of CON birds moving during the same time periods (no laser exposure). For both measures, the percent of birds counted in each min was averaged over the 4 min of each laser period.
Gait Score
All focal birds (n = 70) were removed from their home pens once weekly, wk 1–6, and assessed for lameness. One researcher conducted the gait scores throughout. Birds were placed on a custom-designed plywood runway, described in Meyer et al. (2020). Briefly, birds either walked 1.5 m independently or were gently encouraged to walk by the researcher. Individual birds were considered to have completed the task when both feet had crossed into the finish section indicating 1.5 m. Scores were assigned using a 0–2 scale adapted from National Chicken Council guidelines (National Chicken Council, 2020) where 0 indicated the ability to walk 1.5 m with no signs of lameness, 1 indicated the ability to walk 1.5 m but broiler showed unevenness in steps or sat down at least once, and 2 indicated a bird that could not walk 1.5 m.
Tibia Outcomes
On d42, all focal birds (n = 70) were euthanized using carbon dioxide and the right tibia was collected from each bird and frozen at −20°C until further analysis. Tibias were thawed, weighed, and scanned using dual energy x-ray absorptiometry (DXA, Hologic, Marlborough, MA). The bones were scanned in groups of seven using the “infant whole body” protocol for bone mineral density (BMD) and bone mineral content (BMC).
Bone breaking strength (BBS) of focal bird tibia was measured using the tensile test and compression method on an Instron 3369 Universal Test Machine (Instron; Norwood, MA, U.S.) with a 50 kN load capacity. Two platons were controlled to fracture the bone between them. Each tibia was individually fractured in a plastic bag to prevent contamination of the machine. Each tibia was placed with the lateral/medial condyle end of the bone placed over the edge of the bottom platon, and the bend of the tibia facing down. The test method was set so that the top platon moved vertically downwards toward the bone at a rate of 10 mm/min and a 15% rate of load. Each test was either stopped manually, by the operator at the distinct sound of the bone fracturing with simultaneous visualized decline in force on the monitor, or automatically, by the Instron following a 40% decline in force. Load (kgf) was recorded at the point of break, and divided by area of tibia (cm2, obtained from DXA scanning) to calculate BBS per manufacturer recommendations (Instron; Norwood, MA, U.S.).
Statistical Analysis
Room within barn was confounded by enrichment, thus was not included in the statistical model, but environmental conditions, management, and feeding were kept as identical as possible between rooms. The model included the random effect of pen within treatment, as birds were randomly assigned to pens. All data were analyzed using SAS software version 9.4 (SAS Institute Inc., 2016, Carey, NC. U.S.) with the main effect of enrichment. PROC UNIVARIATE was used to assess the distribution of data prior to analysis. Home pen behavior, pen-wide movement, and walking distance data were abnormally distributed (Poisson distribution), hence were analyzed using PROC GLIMMIX with the fixed effect of enrichment (LASER vs. CON), week or day, and the enrichment × week/day interaction. Laser-following behavior was analyzed with the main effect of day/week only, as it was only possible to record in LASER-enriched pens and could hence not be analyzed with the effect of enrichment. For walking distance analysis, distance walked by each laser minute was summed to create a total distance walked per laser period. Gait scores were unable to be analyzed statistically, as scores other than 0 rarely occurred. Tibia data were normally distributed, hence were analyzed using PROC MIXED, a mixed linear model. PROC CORR was used to provide Pearson Correlation Coefficients for tibia outcomes. For all measures, a value of P ≤ 0.05 was considered significant.
Results
Broiler Home Pen Behavior
d0–8
The enrichment by day interaction was significant for active (P < 0.001), inactive (P < 0.001), at feeder (P < 0.001), and at drinker (P < 0.001) behaviors. Behaviors categorized as “other” occurred so infrequently on d0–8 that the data could not be analyzed. LASER enriched birds were significantly more active on d6 (7.8% greater) and d8 (3.6% greater), while CON birds displayed greater active behaviors on d3 by 2.6% (Figure 1A). LASER-enriched birds spent more time inactive than the CON on d3 (22.1% greater) and d4 (25.5% greater), but showed significantly reduced time spent inactive on d0 (10.4% less) and d6 (9.5% less; Figure 1B). Percent of time at feeder was increased in LASER-enriched birds on d5 (12.4% greater) and d7 (9.7% greater) and was increased in CON birds on d0 (10.1% greater) and d3 (21.7% greater; Figure 1C). Time spent at drinker during laser periods was increased in LASER-enriched birds on d2 by 4.4% and was increased in CON birds on d4 (by 9%), d5 (by 5.3%), and d7 (by 10.7%; Figure 1D).
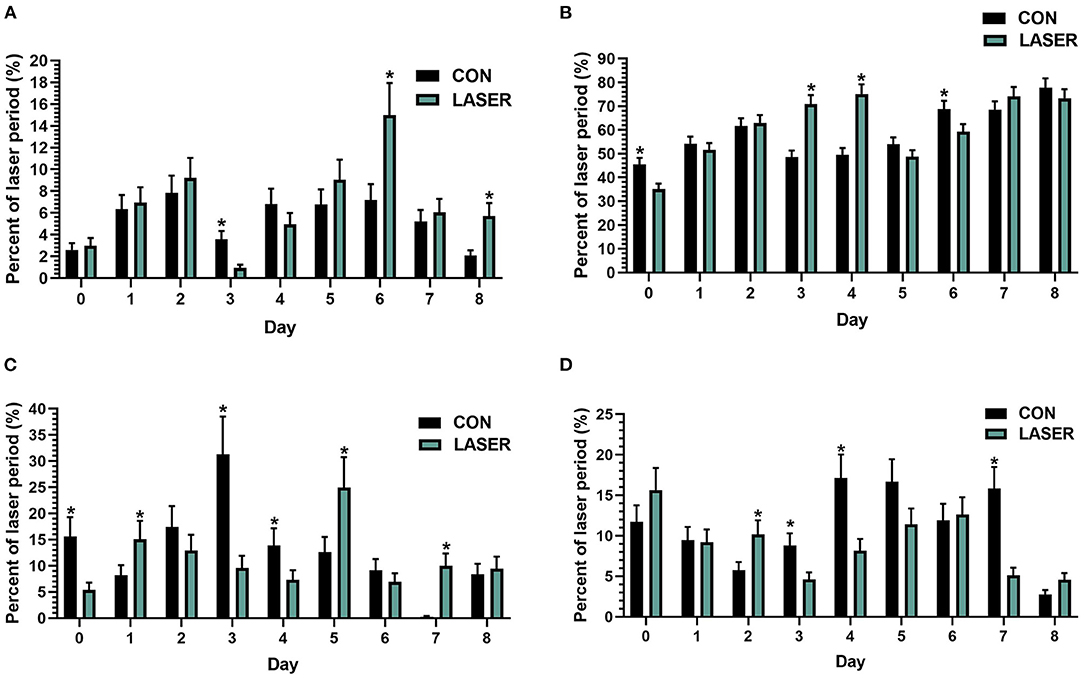
Figure 1. (A–D) Ross 708 focal broiler home pen behavior over d0–8 during 4-min laser periods: enrichment × day LSMeans (±SEM)a. Percent of time spent: (A) active; (B) inactive; (C) at feeder; and (D) at drinker. aBars denoted * indicate means that are significantly different within the same day/week (P ≤ 0.05).
Wk 1–6
When broiler behavior was analyzed 1 day/week (every Thursday) over wk 1–6, the enrichment × week interaction was significant for active (P < 0.001), inactive (P < 0.001), at feeder (P < 0.001), and at drinker (P < 0.001) behaviors. “Other” behaviors occurred so infrequently that the data could not be statistically analyzed (convergence criteria attempted to 10−4), hence results are not included here. Increased time spent active was observed in LASER-enriched birds on wk 3 (5.6% greater), wk 4 (3.7% greater), and wk 5 (1.8% greater) compared to CON birds (Figure 2A). Percent of time inactive was significantly increased in CON birds on wk 4 by 22.7% but was unaffected by enrichment at all other timepoints (Figure 2B). Percent of time at feeder was increased in LASER-enriched birds on week 4 by 13.3% (Figure 2C). Time spent at drinker was increased in LASER birds on wk 3 by 7.8% (Figure 2D).
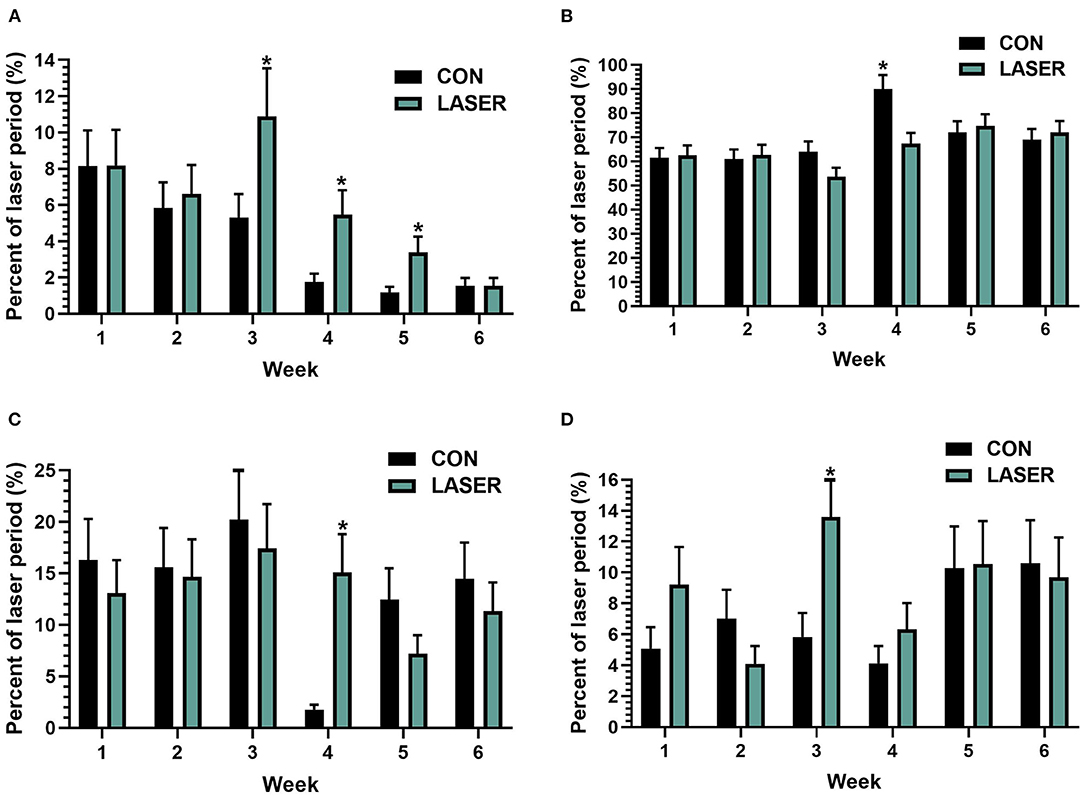
Figure 2. (A–D) Ross 708 focal broiler home pen behavior over wk 1–6 during 4-min laser periods. Enrichment × week LSMeans (±SEM)a showing percent of time spent: (A) active; (B) inactive; (C) at feeder; and (D) at drinker. The same day each week was used for video recording. aBars denoted * indicate means that are significantly different within the same day/week (P ≤ 0.05).
Walking Distance
d0–8
The enrichment × day interaction was significant for distance walked by each individual minute of the 4-min laser period (P < 0.0001), and for the total distance walked per period from d0–8 (P < 0.001; Figure 3A). Total distance walked during laser periods was significantly increased in LASER birds on d1 by 109 cm (126.1% increase) and increased in CON birds on d4 by 73 cm (67.9% increase; Figure 3A).
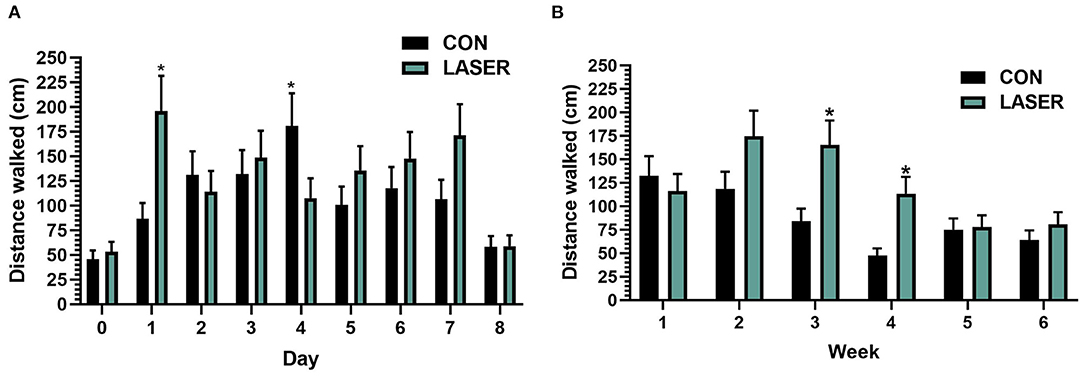
Figure 3. (A,B) Mean walking distancea (cm) of focal birds during 4-min laser periods over: (A) d0–8 (enrichment × day LSMeans ± SEM); and (B) wk 1–6 (enrichment × week LSMeans ± SEM)b. Following the first d0–8, Thursdays of each week were selected for behavior video recording. aTotal distance convergence criteria relaxed to 10−6. bBars denoted * indicate means that are significantly different within the same day/week (P ≤ 0.05).
Wk 1–6
The enrichment × week interaction was significant for walking distance in minutes 1–4 individually (P < 0.001) and the total distance per period (P < 0.001; Figure 3B). Laser period total walking distance was increased in LASER birds by 81 cm on wk 3 (96.7% increase) and by 66 cm on wk 4 (139% increase; Figure 3B).
Laser-Following Behavior and Pen-Wide Movement
d0–8
Percent of birds following the laser (LASER-enriched pens only) was significantly affected by day of study (P < 0.0001), with maximum laser-following occurring on d0 (8.52%), and a gradual reduction but not cessation of the behavior observed over the first 9d (Figure 4A). Laser-following in 1–10% of total birds continued on a daily basis through d8 and weekly through wk 6 (Figures 4A,B).
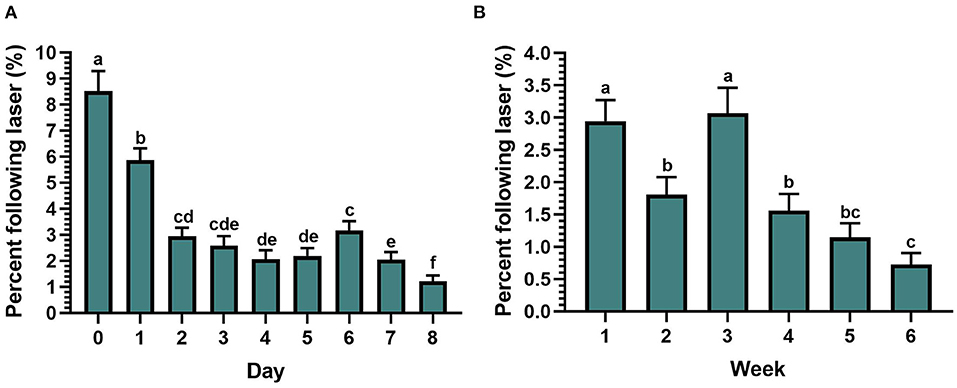
Figure 4. Laser-following behavior over (A) d0–8 (day LSMeans ± SEM) and (B) wk 1–6 (week LSMeans ± SEM) in LASER-enriched pens onlya. Following the first d0–8, Thursdays of each week were selected for behavior video recording. aBars lacking common letters indicate means that are significantly different (P ≤ 0.05).
Wk 1–6
The effect of week was significant (P < 0.0001), with the maximum laser-following observed on wk 3 (3.07% of birds). Total percent of birds moving in LASER-enriched and CON pens was affected by enrichment, day, and the enrichment × day interaction (P < 0.0001). Percent of birds moving was increased in LASER-enriched pens on d0, 1, 2, 6, 7, and 8 (Figure 5A), with a peak percent increase of 68.7% observed on d1. Over wk 1–6, enrichment, week, and the enrichment × week interaction were significant (P < 0.0001). Percent of birds moving (laser-following or not) was increased on wk 1, 3, and 4 in LASER-enriched pens, with a peak increase of 69.7% observed on wk 4 (Figure 5B).
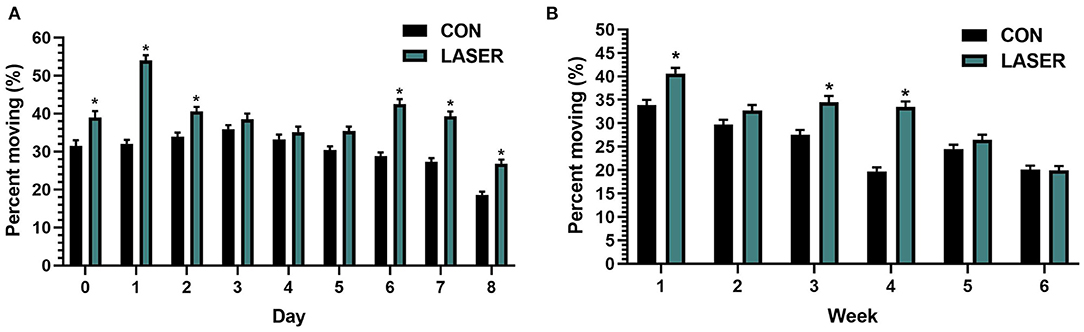
Figure 5. Total percent of birds moving in LASER-enriched and CON pens over (A) d0–8 (enrichment × day LSMeans ± SEM) and (B) wk 1–6 (enrichment × week LSMeans ± SEM)a. Following the first d0–8, Thursdays of each week were selected for behavior video recording. aBars denoted * indicate means that are significantly different within the same day/week (P ≤ 0.05).
Gait Score
Gait scores are presented descriptively by occurrence of score other than 0 (a bird able to walk 1.5 m with no signs of lameness). A single score of 1 was observed in the CON focal birds on wk 3, a single score of 1 was observed in the LASER-enriched focal birds on wk 4, and two CON birds and one LASER bird received a score of 1 on wk 6. No scores of 2 were observed in either treatment.
Tibia Outcomes
Right tibia bone mineral content and density were not significantly affected by enrichment. Mean BMC was 0.17 g ± 0.02 in the LASER treatment and 0.19 g ± 0.03 in the CON treatment. Bone mineral density (BMC/area of tibia), a measure indicative of tibia quality, showed identical means of 0.08 g/cm2 ± 0.08. Bone breaking strength (reported as force at point of break/area of tibia), was also unaffected by enrichment, but was numerically increased in the LASER treatment. Mean BBS was 30.48 kgf/cm2 ± 2.33 in the LASER-enriched birds and 24.89 kgf/cm2 ± 2.60 in the CON.
The data show that there is a moderate, positive relationship between bird weight and tibia weight (r = 0.59), and between BMC and BMD (r = 0.64). There is a strong positive correlation between BMC and tibia area (r = 0.95). Fairly weak positive correlations exist between bird body weight at d42 and tibia area (r = 0.41), bird body weight and BMC (r = 0.34), tibia weight and tibia area (r = 0.53), and BMD and tibia area (r = 0.44). Remaining correlations were non-significant.
Discussion
Environmental enrichment was implemented with the aim to motivate broiler locomotion and foraging behaviors, and to ultimately improve walking ability. Animal motivation, generally understood as goal-oriented action caused by both environmental and physiological variables (Toates, 1986), is not well-defined in broilers. Previous research indicates that chickens, as foragers, are naturally motivated by food (Bokkers et al., 2004), but are also inclined to investigate novelty in their environment (Newberry, 1999), and are reportedly stimulated to peck at particle-sized objects (Hogan, 1973). In the current study, LASER-enriched broilers spent more time active during laser periods by up to 109% in the first week (d6) and up to 214% in wk 4. In terms of distance walked, LASER-enriched broilers walked 126% further in the first week (d1) and 139% further on wk 4. Due to lack of success in motivating physical activity using large, moving, multi-colored spotlights in a study by Bizeray et al. (2002), we can surmise that the size, color, and speed of lasers utilized in the current study were more effective in visually stimulating the broilers. This increased activity agrees with data from the proof-of-concept study testing the laser devices, where broiler activity was also significantly increased. In the previous study utilizing Ross 308 broilers grown to 6 wk, time spent active was increased during laser periods wk 2–5 by up to 157% on wk 3 (Meyer et al., 2020). On d3, CON birds were observed spending a greater percent of time active, at the feeder, and at the drinker, while LASER birds spent a greater percent of time inactive on this day. It is unclear why CON birds were more active overall on d3 but may have been due to difficult to control factors such as increased barn or research staff presence in the control room on that day. Regardless, no staff were permitted to enter either room of the barn during the hour of laser periods/video-recording.
A novel measure utilized in this study was measuring both specific laser-following behavior as well as pen-wide activity. Our data showed that while a relatively small percent of broilers was observed actively following the laser dots during recorded laser periods, this specific behavior was maintained through wk 6, indicating that complete habituation to the laser did not occur. Furthermore, the overall pen movement was significantly higher in LASER-enriched pens. Previous research in broiler breeder enrichment reported habituation to bunches of string, but the length of time it took for birds to lose interest is unclear (Hocking and Jones, 2006). Habituation to visual contact with humans for 10 min twice/d was established in broilers within 3–6 weeks (Zulkifli et al., 2002), and broiler and layer chicks became habituated to humans through visual contact for 30 s/d for 10 d (Jones, 1995). A study incorporating hanging strings for 10 min/d for five consecutive days showed that laying hens maintained interest in pecking the strings for 14 wk, a promising result indicating that novel enrichment may remain relevant to chickens with limited (10-min periods) exposure, even over multiple days (Jones et al., 2000).
An additional interesting outcome observed in the current study was the increase between percent of birds following the laser vs. otherwise moving in the laser-enriched pens. For example, while only 5.86% of birds were recorded following the laser on d1, over half of the pen (53.99%) was observed moving during the same laser periods. Additionally, while on wk 5 only 1.15% of birds were observed following the laser, 26.49% were observed moving, an 8% increase in overall activity compared to CON pens during the same periods. A likely explanation for this result is the documented disturbance of resting broilers by fellow birds (Yngvesson et al., 2017; Forslind et al., 2021), largely considered a negative welfare outcome in terms of interrupting rest. However, fast-growing strains of broilers are also reported to spend more time inactive and to use physical environmental enrichment in the form of platforms, scales, mineral blocks, and hanging rope less than slow-growing strains of birds (Dawson et al., 2021), behavioral traits both considered to be inherent to the individual strain of bird's growth rate and negative in terms of animal welfare. Therefore, the authors of the current work consider that increasing activity, specifically without observing negative impacts on animal welfare or performance, should be considered positive and unique in terms of environmental enrichment impact provided by the laser. Furthermore, the significant outcome of increased activity above the level of CON birds was observed through wk 4, particularly considering the increasing inactivity observed in broilers with age (Weeks et al., 2000), and the difficulty reported in stimulating activity through broiler enrichment (Bizeray et al., 2002; Shields et al., 2005; Jordan et al., 2011; Pichova et al., 2016). Regardless, measuring incidences of rest disturbance due to laser-induced activity would be of interest in future work using laser enrichment devices.
Supporting our hypothesis that the laser induced foraging-based behaviors, time spent at the feeder was significantly increased in the current study during the first week by up to 3,427% (d7; Figure 1C) and by 761% on week 4 (Figure 2C). These results are in agreement with results from our previous study, where percent of time spent at the feeder was increased by up to 247% on wk 5 (Meyer et al., 2020). Although foraging and general activity have largely been removed from the behavioral repertoire of today's broiler approaching market weight (Weeks et al., 2000), continuous movement while foraging, motivated by visual stimuli, is a behavior natural to the chicken's junglefowl ancestor (Arshad et al., 2000; Fernandez-Juricic et al., 2004). However, on days 0, 3, and 4, CON birds spent a greater percent of time at the feeder. This was not reflected in increased feed intake in CON birds during this time period (Meyer et al., unpublished companion paper), and may be due LASER-enriched bird time spent following the laser in the first week.
Ultimately, increased feed intake was observed in LASER-enriched birds wk 2–6 (Meyer et al., unpublished companion paper). Notably, bird body weight was increased in LASER-enriched birds at the end of all performance periods, which an increased harvest weight of 0.14 kg at wk 6 and 0.15 kg at wk 7. Further, FCR was reduced in LASER-enriched birds in the starter period by 3 points (Meyer et al., unpublished companion paper). These data indicate that increased voluntary exercise observed due to laser enrichment did not hinder growth performance of Ross 708 broilers. This outcome agrees with performance data from the laser proof of concept study utilizing Ross 308 birds, where feed intake was increased in all performance periods and birds finished at a live weight 0.24 kg greater than the control (Meyer et al., 2019). Taken together, these behavior and performance results from both studies provide evidence that the degree of exercise stimulated by laser enrichment was not to an extent of energy-expenditure that detrimentally affected body weight gain nor feed conversion. This outcome is perhaps in contrast to the current understanding that modern broilers are inherently inactive partially as a result of genetic selection for growth-focused energy partitioning (Bizeray et al., 2000) as well as due to stocking density and generally unenriched environments (Newberry, 1999; Sorensen et al., 2000).
One of the study aims was to improve walking ability through laser-induced locomotion. Improved gait score with percent of time spent walking is an outcome previously reported in broilers by Dawkins et al. (2009). Ultimately, under our research conditions, lameness typical to a commercial scale was not observed; the overwhelming majority of gait scores were 0, or birds showing no signs of lameness. The highest score identified was 1, indicating mild lameness in a bird able to walk 1.5 m; hence, we did not see an induction or improvement in gait score due to laser enrichment. This reflects the lack of lameness previously observed in our research conditions using Ross 308 broilers exposed to laser enrichment (Meyer et al., 2020), and is a positive outcome validating that the increased body weight consistently observed in LASER-enriched broilers either did not predispose them to lameness or was counter-acted by laser-induced exercise.
Leg lameness causing pain and difficulty walking is a multifactorial welfare issue affecting up to 30–35% of commercial broilers (Kestin et al., 1992; Sanotra et al., 2007). Severe lameness inhibiting birds from accessing resources necessitates ~2% of culls in the broiler industry (Dunkley, 2007). These walking difficulties are primarily caused by rapid growth rates, in conjunction with lack of activity, leading to skeletal disorders of the legs (Knowles et al., 2008; Bassler et al., 2013). It has been well-established that exercise and weight-bearing during early growth are critical for successful bone development (Lanyon, 1993), and although collagen cross-linking contributing to bone strength increases with age, this may not play a meaningful role in broilers due to the 6–7 wk harvest age (Rath et al., 2000).
Previous broiler work has shown that physical activity can increase tibia strength and decrease the lameness severity (Reiter and Bessei, 2009). Hence, in the current study, tibia quality measures were collected at d42 with the hypothesis that LASER-enriched birds with significantly increased locomotory behavior would have improved BMC, BMD, and BBS, an outcome observed numerically in the initial laser study (Meyer et al., 2019). DXA scanning has been validated previously in broilers to determine BMC and BMD (Swennen et al., 2004; Castro et al., 2019), as has BBS (McDevitt et al., 2006; Shim et al., 2012). Data suggest in the research setting, BMD and BMC outcomes were not significantly altered by enrichment. These data are in contrast to findings by Wang et al. (2021), who reported that birds exhibiting increased exercise had improved tibial strength, but is in agreement with enrichment work by Pedersen et al. (2020), where broilers that walked more to access resources did not have improved tibia strength. Also in agreement with our results, a lack of significance in tibia outcomes was observed in free-range hens with reported increased exercise by Kolakshyapati et al. (2019).
Despite the lack of enrichment effect on tibia outcomes in the current study, a positive relationship was identified between bird weight and tibia weight, BMC and BMD, and BMC and tibia area. The correlation between body and tibia weight agrees with similar findings in free-range laying hens (Kolakshyapati et al., 2019). As bone mineral density is reflective of both mineral quality and content (Rath et al., 2000), the observed relationship between BMC and BMD was expected. While bird body weight, tibia weight, tibia area, BMC, and BMD together contributed to 63.6% of the variation in tibia-related outcomes, BBS was not correlated with any of the other observations, and rather, independently contributed to 22.8% of variation. The BBS test used was a compression force method with a constant rate of load, thus BBS should have been reflective of BMC and BMD (Rath et al., 2000). However, this is not always the case, as observed in non-human primates (Vahle et al., 2015) and in our previous broiler work (Meyer et al., 2019).
In summary, these data validate successful laser device-induced locomotion in a different genetic line of broilers than tested previously and provide a novel environmental enrichment tool that can be used to increase pen-wide activity without altering bird management or sacrificing performance. Importantly, in the work reported here, this increased locomotion was achieved through visually stimulating a small number of birds that then promoted movement throughout the pen without hindering measures of animal welfare such as gait score or tibia quality. In the future, the laser device should be tested and implemented on a commercial scale to ultimately improve animal welfare and enhance active behavior.
Data Availability Statement
The raw data supporting the conclusions of this article will be made available by the authors, without undue reservation.
Ethics Statement
The animal study was reviewed and approved by Iowa State University Institutional Animal Care and Use Committee.
Author Contributions
MM, AJ, and EB contributed to conception and design of the study. MM and EB contributed to on-farm animal care, data collection, statistical analysis, and manuscript drafts. All authors contributed to manuscript revision, read, and approved the submitted version.
Funding
This work was supported by the Department of Animal Science, College of Agriculture and Life Sciences at Iowa State University and USDA for partial funding of AJ and EB's salary, and from USDA Grant # 2019-69012-29905 for Ph.D., student and project funding.
Conflict of Interest
The authors declare that the research was conducted in the absence of any commercial or financial relationships that could be construed as a potential conflict of interest.
Publisher's Note
All claims expressed in this article are solely those of the authors and do not necessarily represent those of their affiliated organizations, or those of the publisher, the editors and the reviewers. Any product that may be evaluated in this article, or claim that may be made by its manufacturer, is not guaranteed or endorsed by the publisher.
Acknowledgments
The authors would like to thank the Poultry Research and Teaching Farm staff for help in animal husbandry and barn management, as well as M.S. student Emiline Sundman for help gait-scoring birds, and undergraduate students Tyler Loew, Kayla Elmore, and Kaitlyn Olson for behavioral analysis.
Supplementary Material
The Supplementary Material for this article can be found online at: https://www.frontiersin.org/articles/10.3389/fanim.2021.784408/full#supplementary-material
References
Arshad, M., Zakaria, M., Sajap, A. S., and Ismail, A. (2000). Food and feeding habits of red junglefowl. Pak. J. Biol. Sci. 3, 1024–2026. doi: 10.3923/pjbs.2000.1024.1026
Bassler, A. W., Arnould, C., Butterworth, A., Colin, L., De Jong, I. C., Ferrante, V., et al. (2013). Potential risk factors associated with contact dermatitis, lameness, negative emotional state, and fear of humans in broiler chicken flocks. Poult. Sci. 92, 2811–2826. doi: 10.3382/ps.2013-03208
Baxter, M., Bailie, C. L., and O'Connell, N. E. (2019). Play behaviour, fear responses and activity levels in commercial broiler chickens provided with preferred environmental enrichments. Animal 13, 171–179. doi: 10.1017/S1751731118001118
Bizeray, D., Estevez, I., Leterrier, C., and Faure, J. M. (2002). Effects of increasing environmental complexity on the physical activity of broiler chickens. Appl. Anim. Behav. Sci. 79, 27–41. doi: 10.1016/S0168-1591(02)00083-7
Bizeray, D., Leterrier, C., Constantin, P., Picard, M., and Faure, J. M. (2000). Early locomotor behaviour in genetic stocks of chickens with different growth rates. Appl. Anim. Behav. Sci. 68, 231–242. doi: 10.1016/S0168-1591(00)00105-2
Bokkers, E. A. M., Koene, P., Rodenburg, T. B., Zimmerman, P. H., and Spruijt, B. M. (2004). Working for food under conditions of varying motivation in broilers. Anim. Behav. 68, 105–113. doi: 10.1016/j.anbehav.2003.10.013
Castro, F. L. S., Su, S., Choi, H., Koo, E., and Kim, W. K. (2019). L-Arginine supplementation enhances growth performance, lean muscle, and bone density but not fat in broiler chickens. Poult. Sci. 98, 1716–1722. doi: 10.3382/ps/pey504
Dawkins, M. S., Lee, H., Waitt, C. D., and Roberts, S. J. (2009). Optical flow patterns in broiler chicken flocks as automated measures of behaviour and gait. Appl. Anim. Behav. Sci. 119, 203–209. doi: 10.1016/j.applanim.2009.04.009
Dawson, L. C., Widowski, T. M., Liu, Z., Edwards, A. M., and Torrey, S. (2021). In pursuit of a better broiler: a comparison of the inactivity, behavior, and enrichment use of fast- and slower-growing broiler chickens. Poult. Sci. 100:101451. doi: 10.1016/j.psj.2021.101451
Dunkley, C. S. (2007). The Poultry Site. Leg Problems in Broilers. Available online at: https://www.thepoultrysite.com/articles/leg-problems-in-broilers (accessed September, 2021).
Fernandez-Juricic, E., Erichsen, J. T., and Kacelnik, A. (2004). Visual perception and social foraging in birds. Trends Ecol. Evol. 19, 25–31. doi: 10.1016/j.tree.2003.10.003
Forslind, S., Blokhuis, H., and Riber, A. B. (2021). Disturbance of resting behaviour of broilers under different environmental conditions. Appl. Anim. Behav. Sci. 242:105425. doi: 10.1016/j.applanim.2021.105425
Havenstein, G. B., Ferket, P. R., and Qureshi, M. A. (2003). Growth, livability, and feed conversion of 1957 versus 2001 broilers when fed representative 1957 and 2001 broiler diets. Poult. Sci. 82, 1500–1508. doi: 10.1093/ps/82.10.1500
Hocking, P. M., and Jones, E. K. M. (2006). On-farm assessment of environmental enrichment for broiler breeders. Br. Poult. Sci. 47, 418–425. doi: 10.1080/00071660600825074
Hogan, J. A. (1973). Development of food recognition in young chicks: I. Maturation and nutrition. J. Comp. Physiol. Psychol. 83, 355–366. doi: 10.1037/h0034669
Jones, R. B. (1995). Habituation to human beings via visual contact in docile and flighty strains of domestic chicks. Int. J. Comp. Psychol. 8, 88–98.
Jones, R. B., Carmichael, N. L., and Rayner, E. (2000). Pecking preferences and pre-dispositions in domestic chicks: implications for the development of environmental enrichment devices. Appl. Anim. Behav. Sci. 69, 291–312. doi: 10.1016/S0168-1591(00)00138-6
Jordan, D., Stuhec, I., and Bessei, W. (2011). Effect of whole wheat and feed pellets disribution in the litter on broilers' activity and performance. Eur. Poult. Sci. 75, 98–103.
Kestin, S. C., Knowles, T. G., Tinch, A. E., and Gregory, N. G. (1992). Prevalence of leg weakness in broiler chickens and its relationship with genotype. Vet. Rec. 131, 190–194. doi: 10.1136/vr.131.9.190
Knowles, T. G., Kestin, S. C., Haslam, S. M., Brown, S. N., Green, L. E., Butterworth, A., et al. (2008). Leg disorders in broiler chickens: prevalence, risk factors and prevention. PLoS ONE 3:e1545. doi: 10.1371/journal.pone.0001545
Kolakshyapati, M. R., Flavel, J., Sibanda, T. Z., Schneider, D., Welch, M. C., and Ruhnke, I. (2019). Various bone parameters are positively correlated with hen body weight whilerange access has no beneficial effect on tibia health of free-range layers. Poult. Sci. 98, 6241–6250. doi: 10.3382/ps/pez487
Lanyon, L. E. (1993). “Skeletal responses to physical loading” in Physiology and Pharmacology of Bone. Handbook of Experimental Pharmacology, eds G. V. R. Born, P. Cuatrecasas, H. Herken, and K. Melmon (Berlin: Springer), 107. doi: 10.1007/978-3-642-77991-6_14
McDevitt, R. M., McEntee, G. M., and Rance, K. A. (2006). Bone breaking strength and apparent metabolisability of calcium and phosphorus in selected and unselected broiler chicken genotypes. Br. Poult. Sci. 47, 613–621. doi: 10.1080/00071660600963560
Meyer, M. M., Johnson, A. K., and Bobeck, E. A. (2019). A novel environmental enrichment device improved broiler performance without sacrificing bird physiological or environmental quality measures. Poult. Sci. 98, 5247–5256. doi: 10.3382/ps/pez417
Meyer, M. M., Johnson, A. K., and Bobeck, E. A. (2020). A novel environmental enrichment device increased physical activity and walking distance in broilers. Poult. Sci. 99, 48–60. doi: 10.3382/ps/pez581
Nääs, I. A., Paz, I. C. L. A., Baracho, M. S., Menezes, A. G., Bueno, L. G. F., Almeida, I. C. L., et al. (2009). Impact of lameness on broiler well-being. J. Appl. Poult. Res. 18, 432–439. doi: 10.3382/japr.2008-00061
National Chicken Council (2020). National Chicken Council Animal Welfare Guidelines and Audit Checklist For Broilers. Available online at: https://www.nationalchickencouncil.org/wp-content/uploads/2021/02/NCC-Animal-Welfare-Guidelines_Broilers_Sept2020.pdf (accessed September, 2021).
Newberry, R. C. (1999). Exploratory behaviour of young domestic fowl. Appl. Anim. Behav. Sci. 63, 311–321. doi: 10.1016/S0168-1591(99)00016-7
Norring, M., Kaukonen, E., and Valros, A. (2016). The use of perches and platforms by broiler chickens. Appl. Anim. Behav. Sci. 184, 91–96. doi: 10.1016/j.applanim.2016.07.012
Pedersen, I. J., Tahamtani, F. M., Forkman, B., Young, J. F., Pulsen, H. D., and Riber, A. B. (2020). Effects of environmental enrichment on health and bone characteristics of fast growing broiler chickens. Poult. Sci. 99, 1946–1955. doi: 10.1016/j.psj.2019.11.061
Pichova, K., Nordgreen, K., Leterrier, C., Kostal, L., and Oppermann, M. R. (2016). The effects of a food-related environmental complexity on litter directed behaviour, fear, and exploration of novel stimuli in young broiler chicks. Appl. Anim. Behav. Sci. 174, 83–89. doi: 10.1016/j.applanim.2015.11.007
Prayitno, D. S., Phillips, C. J., and Stokes, D. K. (1997). The effects of color and intensity of light on behavior and leg disorders in broiler chickens. Poult. Sci. 76, 1674–1681. doi: 10.1093/ps/76.12.1674
Rath, N. C., Huff, G. R., Huff, W. E., and Balog, J. M. (2000). Factors regulating bone maturity and strength in poultry. Poult. Sci. 79, 1024–1032. doi: 10.1093/ps/79.7.1024
Reiter, K., and Bessei, W. (2009). Effect of locomotor activity on leg disorder in fattening chicken. Berl. Munch. Tierarztl. Wochenschr. 122, 264–270.
Ruiz-Feria, C. A., Arroyo-Villegas, J. J., Pro-Martinez, A., Bautista-Ortega, J., Cortes-Cuevas, A., Narciso-Gaytan, C., et al. (2014). Effects of distance and barriers between resources on bone and tendon strength and productive performance of broiler chickens. Poult. Sci. 93, 1608–1617. doi: 10.3382/ps.2013-03421
Sanotra, G. S., Lund, J. D., Ersboll, A. K., Petersen, J. S., and Vestergaard, K. S. (2007). Monitoring leg problems in broilers: a survey of commercial broiler production in Denmark. Worlds Poult. Sci. J. 57, 655–659.
Shields, S. J., Garner, J. P., and Mench, J. A. (2005). Effect of sand and wood-shavings bedding on the behavior of broiler chickens. Poult. Sci. 84, 1816–1824. doi: 10.1093/ps/84.12.1816
Shim, M. Y., Karnuah, A. B., Mitchell, A. D., Anthony, N. B., Pesti, G. M., and Aggrey, S. E. (2012). The effects of growth rate on leg morphology and tibia breaking strength, mineral density, mineral content, and bone ash in broilers. Poult. Sci. 91, 1790–1795. doi: 10.3382/ps.2011-01968
Soglia, F., Laghi, L., Canonico, L., Cavani, C., and Petracci, M. (2016). Functional property issues in broiler breast meat related to emerging muscle abnormalities. Food Res. Int. 89, 1071–1076. doi: 10.1016/j.foodres.2016.04.042
Sorensen, P., Su, G., and Kestin, S. C. (2000). Effects of age and stocking density on leg weakness in broiler chickens. Poult. Sci. 79, 864–870. doi: 10.1093/ps/79.6.864
Swennen, Q., Janssens, G. P., Geers, R., Decuypere, E., and Buyse, J. (2004). Validation of dual-energy x-ray absorptiometry for determining in vivo body composition of chickens. Poult. Sci. 83, 1348–1357. doi: 10.1093/ps/83.8.1348
Tahamtani, F. M., Pedersen, I. J., and Riber, A. B. (2020). Effects of environmental complexity on welfare indicators of fast-growing broiler chickens. Poult. Sci. 99, 21–29. doi: 10.3382/ps/pez510
USDA. (2019). USDA National Retail Report-Chicken. USDA Livestock, Poultry, & Grain Market News. Available online at: http://www.ams.usda.gov/market-news/livestock-poultry-grain (accessed September, 2021).
Vahle, J. L., Ma, Y. L., and Burr, D. B. (2015). “The nonhuman primate,” in Nonclinical Drug Development and Safety Assessment, eds J. Bluemel, E. Schenck, S. Korte, and G. Weinbauer (New York, NY: Academic Press), 605–625. doi: 10.1016/B978-0-12-417144-2.00032-9
Ventura, B. A., Siewerdt, F., and Estevez, I. (2012). Access to barrier perches improves behavior repertoire in broilers. PLoS ONE 7:e29826. doi: 10.1371/journal.pone.0029826
Wang, J.-C., He, X. X., Zhao, Y. C., Wang, Y., Wang, J. X., Guo, X., et al. (2021). Exercise profile and effect on growth traits, carcass yield, meat quality, and tibial strength in Chinese Wannan chickens. Poult. Sci. 100, 721–727. doi: 10.1016/j.psj.2020.11.044
Weeks, C. A., Danbury, T. D., Davies, H. C., Hunt, P., and Kestin, S. C. (2000). The behaviour of broiler chickens and its modification by lameness. Appl. Anim. Behav. Sci. 67, 111–125. doi: 10.1016/S0168-1591(99)00102-1
Yngvesson, J., Wedin, M., Gunnarsson, S., Jönsson, L., Blokhuis, H., and Wallenbeck, A. (2017). Let me sleep! Welfare of broilers (Gallus gallus domesticus) with disrupted resting behaviour. Acta Agric. Scand. 67, 123–133. doi: 10.1080/09064702.2018.1485729
Zuidhof, M. J., Schneider, B. L., Carney, V. L., Korver, D. R., and Robinson, F. E. (2014). Growth, efficiency, and yield of commercial broilers from 1957, 1978, and 2005. Poult. Sci. 93, 2970–2982. doi: 10.3382/ps.2014-04291
Keywords: broiler, environmental enrichment, activity, welfare, walking distance
Citation: Meyer MM, Johnson AK and Bobeck EA (2021) Laser Enrichment Device Stimulates Broiler Laser-Following Behavior While Increasing Individual Bird Locomotion and Pen-Wide Movement. Front. Anim. Sci. 2:784408. doi: 10.3389/fanim.2021.784408
Received: 27 September 2021; Accepted: 28 October 2021;
Published: 29 November 2021.
Edited by:
Edward Narayan, The University of Queensland, AustraliaReviewed by:
Sezen Özkan, Ege University, TurkeyBeth Ventura, University of Minnesota Twin Cities, United States
Copyright © 2021 Meyer, Johnson and Bobeck. This is an open-access article distributed under the terms of the Creative Commons Attribution License (CC BY). The use, distribution or reproduction in other forums is permitted, provided the original author(s) and the copyright owner(s) are credited and that the original publication in this journal is cited, in accordance with accepted academic practice. No use, distribution or reproduction is permitted which does not comply with these terms.
*Correspondence: Elizabeth A. Bobeck, ZWFib2JlY2tAaWFzdGF0ZS5lZHU=