- Department of Animal Science, Iowa State University, Ames, IA, United States
Sustainability in poultry production is evident in efforts to reduce inputs and a focus on bird welfare and livability. Dietary protein alternatives to traditional sources such as soybean meal aim to meet or exceed efficiency benchmarks and be cost-effective. Environmental enrichment encouraging activity may reduce the occurrence of the predominant breast muscle myopathy, woody breast (WB); interventions to minimize muscle damage and economic loss have yet to be established. The study objectives were to maintain or improve broiler performance and breast quality through environmental enrichment and partially replacing dietary soybean meal with Spirulina. Twelve hundred Ross 708 broilers were randomly assigned to enrichment (LASER; laser enrichment, or CON; no laser enrichment) and diet (algae; 2.5% Spirulina algae, or control) in a 2 × 2 factorial design for 49 days. The same 70 randomly selected birds were examined for contact dermatitis wk 1–6. Breast width was measured weekly on 200 growing broilers beginning on d22. On d42 and 49 slaughter, WB score was assigned using a tactile 0–3 scale and the right breast filet was weighed (n = 200). RNA isolated from 30 breast muscle samples each at d42 and 49 was analyzed using real-time qPCR. Laser enrichment increased body weight at all timepoints (d49: 0.148 kg, P < 0.001). Feed conversion ratio was improved in LASER-enriched birds by 3 points in the starter period (P = 0.003). Breast width was increased at all timepoints in LASER-enriched birds compared to CON (d49: 0.47 cm, P < 0.001). Algae inclusion increased body weight at d28 (0.059 kg, P = 0.005). At d42, 12% more LASER-enriched WB scores were 0 (normal) compared to CON, and at d49, 15% more enriched scores were 0. At d42, 5% more algae-fed broiler scores were 0 compared to control. LASER-enriched broiler breast tissue showed upregulated expression of myogenin, muscle regulatory factor 4, insulin-like growth factor 1, and myostatin compared to CON (P < 0.01). Both laser enrichment and algae inclusion improved broiler performance without negatively impacting environmental or physiological outcomes. LASER enrichment decreased severity of WB score and positively shifted myogenic gene expression in the breast muscle at slaughter.
Introduction
Today's commercial broiler is a highly feed efficient genetic hybrid selected for fast rate of growth and high breast yields (Soglia et al., 2016). This selection has been necessary to keep up with an increasing demand for poultry meat in recent decades, attributed to affordable cost, nutritional benefits, and production sustainability (Petracci et al., 2015). Swift growth and a disproportionate amount of breast muscle have inadvertently altered the bird's center of gravity (Havenstein et al., 2003) and led to welfare and meat quality issues. Predominant welfare concerns include leg lameness and lack of activity (Dunkley, 2007; Knowles et al., 2008; Bassler et al., 2013) which lead to contact dermatitis, further inactivity, and increased culls (Weeks et al., 2000; Nääs et al., 2009). Litter-contact driven dermatitis of the breasts and footpads results in a chemical burn effect that is likely painful (Haslam et al., 2006). Additionally, protein concentration as well as source in the diet can alter litter quality and increase dermatitis occurrence increasing moisture content (Swiatkiewicz et al., 2017). All-vegetable poultry diet formulations have been shown to increase footpad dermatitis, specifically, as well as litter moisture content, compared to diets containing 10% poultry by-product over a 42d grow-out (Eichner et al., 2007).
More recently, the breast muscle myopathy known as woody breast (WB) has been associated with high breast yielding, heavier birds (Petracci and Cavani, 2012; Kuttappan et al., 2016; Gall et al., 2019). Woody breast was first characterized in 2013 by Sihvo et al. and has since become the most prevalent, damaging meat quality concern in poultry meat production (Sihvo et al., 2013; Tijare et al., 2016). This disease of the pectoralis major muscle is broadly identified by pale, inflexible tissue, and a prominent caudal ridge. Woody breast leads to downgrades at slaughter, diminished raw and cooked poultry product quality, and in severe cases, rendering. Losses are significant, with downgrades or rejection costing the U.S. poultry industry up to $200 million/year (Jarvis et al., 2020) and affecting 48–73% of broilers (Velleman, 2019). While the etiology of WB is unknown and likely a combination of factors, the disorder is consistently observed in heavier birds (Kuttappan et al., 2016, 2017; Lilburn et al., 2019), and is hallmarked by measurably heavier, thicker breast filet products (Zhang et al., 2019; Hasegawa et al., 2020). Histologically, decreased circulatory supply and extensive collagen infiltration/fibrosis, along with giant, degraded muscle fibers, are consistently observed in afflicted muscles (Kuttappan et al., 2017; Velleman, 2019; Baldi et al., 2020; Hasegawa et al., 2020). Initially described as a meat quality concern, WB has been hypothesized to be a product and/or a cause of reduced animal welfare (Gall et al., 2019; Baldi et al., 2020). An understudied approach to mediating woody breast is using environmental enrichment to increase activity.
Increased exercise has been shown to improve tibia quality and gait in broilers (Prayitno et al., 1997; Reiter and Bessei, 2009), but stimulating this outcome voluntarily through environmental enrichment, without negatively alternating growth performance, has been limited with varying results. Environmental enrichment is widely defined as a modification to the environment of captive animals that improves biological functioning (Newberry, 1995). For enrichment to be considered truly effective and biologically relevant, it should measurably improve the animal's ability to cope with their environment (Newberry, 1995; Mason et al., 2007). Bizeray et al. (2002) projected multi-colored spotlights onto broiler pen floors but saw no increase in foraging behavior or physical activity. Tahamtani et al. (2020) introduced multiple forms of enrichment, including straw bales and elevated platforms, but saw increased footpad dermatitis due to straw and reduced walking ability due to platforms. Baxter et al. (2019) implemented platform perches and dust baths but saw no increase in broiler activity as a result. Similarly, platform enrichment researched by Norring et al. (2016) did not increase active behavior in broilers. A promising novel laser enrichment device designed by Meyer et al. (2019, 2020) successfully increased broiler movement by up to 157% without inducing fearfulness, lameness, or contact dermatitis (Meyer et al., 2020). Further, the laser stimulated increased feeding behavior, and this resulted in improved feed intake, weight gain, and feed conversion ratio, reduced by up to 18 points (Meyer et al., 2019).
Inducing exercise is of interest from a meat-quality perspective in addition to animal welfare. It is well-known that exercise can induce change in skeletal muscle including muscle fiber type, oxidative capacity, and blood supply (Fluck, 2006; Kalbe et al., 2018). Phenotypic skeletal muscle changes and improved meat quality traits as result of exercise obtained through free-range access have been previously observed in broilers (Yin et al., 2015; Guo et al., 2019). However, this outcome has not been reported in conventionally raised broilers. Interestingly, in pen-reared pigs studied by Kalbe et al. (2018), pigs with naturally/voluntarily higher exercise levels showed altered expression of myogenic genes of interest within the semitendinosus muscle including myostatin, insulin-like growth factor 2, myoblast determination protein 1, and muscle regulatory factor 4. Thus, voluntary exercise in conventionally reared commercial broilers could potentially mitigate WB severity as well as improve animal welfare.
A discussion of animal welfare is not complete without considering the bird's nutrition; a balanced diet is critical for broiler health and one of the five freedoms (Farm Animal Welfare Council Council, 1979). A well-used nutritional intervention to improve broiler growth performance and potentially reduce diet cost is implementing complementary protein products in the diet. Spirulina microalgae is increasing in popularity because it is a high quality, sustainable source of protein (Madeira et al., 2017). Through photosynthesis, microalgae synthesize and are a source of essential amino acids and are reported to be of comparable or superior protein quality to conventional plant sources of protein (Becker, 2007). Further, new sources of protein to replace/reduce corn and soybean inputs into broiler diets are necessary to increase sustainability and efficiency of production (Madeira et al., 2017).
Spirulina has been shown to stimulate positive immune responses and improve antioxidant status in broilers (Mirzaie et al., 2018; Pestana et al., 2020), and is also a well-established source of essential amino acids, lipids, vitamins, minerals, antioxidants, and especially protein (Spolaore et al., 2006; Soni et al., 2017). In growing broiler rations, this algae has improved weight gain at inclusion levels as low as ≤ 1% (Park et al., 2018), and as high as 16% (Evans et al., 2015). However, microalgae cannot completely replace soybean meal in the diet: 50% Spirulina substitution decreased feed intake, growth, and negatively affected feed conversion in broilers without amino acid supplementation above the control diet (Velten et al., 2018). Overall, microalgae are considered a sustainable protein source for animal diets that do not require farmland. With future advances in biomass harvesting technology and efficiency, microalgae may provide a cost-effective protein alternative that does not hinder growth performance (Saadaoui et al., 2021).
The study objectives were to validate the positive effects of the laser enrichment device first described in Meyer et al. (2019) on broiler growth performance, and to confirm that the laser does not negatively impact animal welfare in terms of contact dermatitis occurrence, independently and in conjunction with a Spirulina algae feed ingredient. This study included the additional component of measuring breast width and filet weight, WB score, and myogenic gene expression of the pectoralis major at slaughter to ensure that enrichment-induced exercise did not hinder breast yield or reduce breast muscle quality.
Materials and Methods
Animals
All live bird procedures were approved by the Iowa State University Institutional Animal Care and Use Committee, IACUC #19-322. 1200 mixed sex Ross 708 broiler chicks were obtained from a commercial hatchery (Welp Hatchery, Bancroft, IA, U.S.) and transported to the Poultry Research and Teaching Unit at Iowa State University for a 7-wk floor pen grow-out experiment. Birds were randomly assigned to pens of 30 birds and weighed as a pen upon arrival (average bird BW on d0: 35.98 ± 0.51 g). Seventy birds were randomly assigned on d0 as focal birds (n = 5 birds/pen in 14 pens; 7 pens/enrichment treatment and diet), identified with wing-bands, and marked with animal-safe gel food coloring (red, blue, green, purple, and black; Wilton, Woodridge, IL, U.S.). Food coloring was applied to a cotton ball, rubbed on the back of the bird's head and neck, and reapplied on an as-needed basis.
Housing and Feeding
Birds were housed in 40 pens (1.22 by 2.44 m/pen) divided across two rooms in the barn. One room contained 20 LASER pens (+ enrichment device), and the second contained 20 CON pens (no enrichment device), with doors and an anteroom separating the rooms so no crossover laser exposure was possible. Environmental conditions and management were kept the same across rooms. Approximately 10 cm deep fresh wood shavings provided bedding over solid concrete floor, and PVC pipe dividers with mesh walls (1.22 m height) separated pens. High and low temperatures and humidity were monitored daily. Birds were gradually adjusted from 24 h light on d0, defined as day of placement (30–40 lux), to 20 h light (20–30 lux) from d8-49. Chicks were brooded with 2-heat lamps/pen (22.9 cm reflectors with porcelain socket) using 125-watt heat bulbs (Sylvania, Wilmington, MA, U.S.) for the 1st week.
Broilers were assigned to one of two diets and evenly split across the LASER and the CON rooms of the barn (n = 20 pens/diet): control diet (control), and the control diet + 2.5% Spirulina algae ingredient (Arthrospira platensis; algae). Diets were formulated to Ross 708 guidelines for starter (d0-d14), grower (d14-28), finisher 1 (d28-42) and finisher 2 (d42-49) performance phases (Table 1). Birds were fed ad libitum out of a hanging feeder (BRHF151, Brower Equipment, Houghton, IA, U.S.) gradually raised to accommodate bird height. Water was provided ad libitum from a hanging nipple water line (~8 nipples/pen). Mortality throughout the trial was 6.1% total; 3.8% in CON birds and 2.3% in LASER-enriched birds, or if separated by diet, 3.1% in algae diet and 3.0% in control diet.
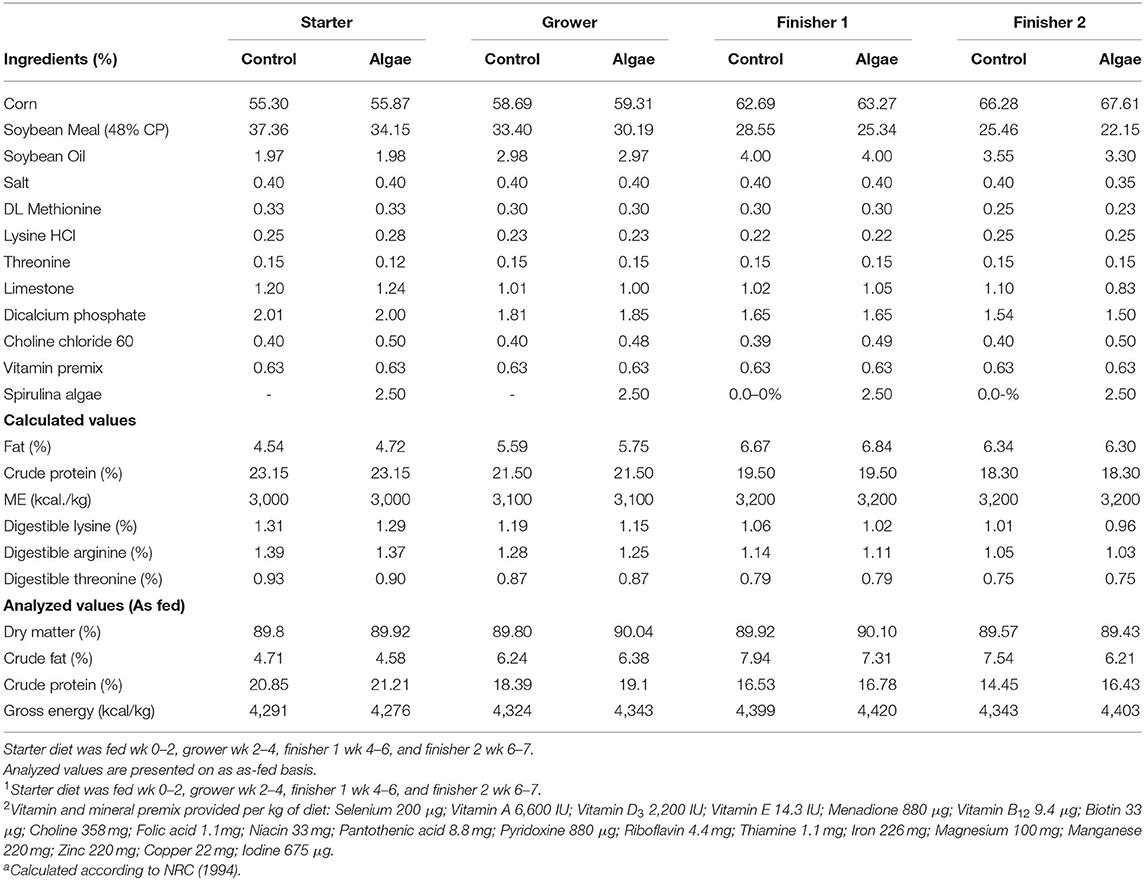
Table 1. Starter, grower, finisher 1, and finisher 2 dietsa provided ad libitum to Ross 708 broilers.
Laser Enrichment Device
Ten laser enrichment devices, previously described by Meyer et al. (2019, 2020), were designed and calibrated to cover two adjoining pens (approximate floorspace of 5.95 m2). The enrichment device consisted of a pair of independent red 650 nm lasers contained within a 20.5 by 20.5 cm metal box with a glass front mounted on a custom-designed structure made of three wooden beams (2.4 m height) placed above the pens. The lasers projected in the direction of the pen floor and moved in a random pattern at a variable speed between 7.6 and 30.5 cm/s for 4-min “laser periods” at: 05:30, 11:30, 17:30, and 23:30 daily for the entirety of the trial. Barn and research staff did not enter the LASER nor CON rooms of the barn during the 4 h daily containing the 4-min laser periods.
Performance
The 7-wk trial was separated into starter, grower, finisher 1, and finisher 2 performance periods. All birds in each pen were weighed as a pen, and focal birds were weighed individually, at the trial's start and at the conclusion of each period. Feed intake (FI) was recorded by measuring disappearance throughout. Feed conversion ratio (FCR) was calculated by pen and averaged by number of birds in the pen.
Breast Blisters and Footpad Dermatitis
Focal birds (n = 70) were examined weekly for breast blisters and footpad dermatitis through wk 1–6. Contact dermatitis examinations were not carried out on wk 7 as focal birds were euthanized at d42 for tibia quality measures (described in Meyer et al., unpublished paper, also submitted to Frontiers). Both examinations took place at the same day and time each week by one researcher. Footpad dermatitis was scored pass/fail using the American Association of Avian Pathologists (2015); a normal yellow color or slight discoloration with hyperkeratosis on an area less than half of the footpad was scored “pass,” and erosions, ulcerations, scabs, hemorrhages, or swelling on an area greater than half of the footpad are scored “fail.” Breast blisters were scored present/absent based on methods by Greene et al. (1985); blisters were considered present when equal to or larger than 1.27 cm2, when there are one or more burns, or when there were scabs on breast skin. A brownish-colored scab was considered “mild” and an ulcer with black exudates was considered “severe.”
Breast Muscle Width and Weight
On d22, 29, 36, 42, and 49, a subset of 100 birds/treatment (n = 200 live birds) were measured for breast width. The width of the entire pectoralis major muscle from beneath wing to wing was measured using a soft seamstress tape measure (reported in cm). On d42 and 49, following euthanasia by carbon dioxide (American Veterinary Medical Association, 2020), the whole right breast filet was dissected from 100 birds/timepoint and weighed individually (n = 200 breast weights total, reported in kg).
Woody Breast Score
On d42, and 49, WB score was assigned by one researcher using the tactile 0-3 scale described by Kuttappan et al. (2016) on a subset of 100 birds/timepoint (n = 200 filets). Scores were assigned on right breast filets post-mortem. Scores were assigned on a 0-3 scale where 0 represented a normal filet that was soft and flexible throughout, 1 represented a mild WB filet with hardness through cranial and caudal region but remained flexible in the medial/caudal region, 2 represented a moderate WB filet that was hard and rigid but maintained some flexibility in the mid to caudal region, and 3 represented a severe WB filet with no flexibility. Increments of 0.5 were used to identify intermediate scores based on the degree of woodiness observed.
Breast Muscle MRNA Isolation and QPCR
On d42 and 49 at ~12:00 each timepoint following WB scoring, the cranial region (~52 cm2 segment) of each right filet was collected (n = 100 filets/timepoint). Samples were flash frozen in liquid nitrogen and stored at −80°C for future analysis. RNA was isolated from 60 breast muscle samples across both timepoints using the RNeasy Fibrous Tissue Kit (Qiagen, MD, U.S.). Fifteen breast muscle samples/treatment/timepoint were thus analyzed. Isolated RNA was DNAse treated and diluted to 50 ng/ul for One-Step SYBR Green quantitative PCR (Qiagen, MD, U.S.), tested against 6 muscle-growth and regulation primers of interest and the ubiquitously expressed chicken housekeeping gene, 28s. Primers included: myoblast determination protein 1 (MyoD); myogenin (MyoG); muscle regulatory factor 4 (MRF4); insulin-like growth factor 1 (IGF1); insulin-like growth factor 2 (IGF2); and myostatin (MSTN). Means are reported as adjusted Ct values: [(40-MeanCttestgene + [Median 28s]-(MeanCttest gene)].
Air and Litter Quality
Ammonia was measured at bird height in the front, middle, and back of each room of the barn on 1 day/week, wk 1–7 with a hand-held ammonia monitor (GasAlert Extreme, BW Technologies, Schaumburg, IL, U.S.) and ammonia test strips. The ammonia monitor was titrated every 14d with an ammonia tank and provided an exact value in ppm, while the strips provide a range of 5 ppm. Litter quality was analyzed weekly according to the National Chicken Council Audit Guidelines (National Chicken Council, 2020). Litter moisture was evaluated in three different, randomly selected pens in the front, middle, and back of each room of the barn. One handful of litter sample was gathered from 3 sections; litter within 15 cm of the water line of each pen was excluded. Litter quality was scored pass/fail by the same researcher weekly; to pass litter must be “loosely compacted when squeezed in the hand. If the litter remains in a clump when it is squeezed in the hand, it is too wet” (National Chicken Council, 2020).
Statistical Analysis
The study followed a 2 × 2 factorial design with two enrichment (LASER vs. CON) and two dietary (algae vs. control) treatments. Room within barn was confounded by enrichment treatment, thus was not included in the model, but environmental conditions, management, and feeding were kept as identical as possible between both rooms. All data were analyzed using SAS software version 9.4 (SAS Institute Inc.; 2016, Carey, NC. U.S.). PROC UNIVARIATE was used to assess the distribution of data prior to analysis. Woody breast scores, due to the descriptive nature of the scale used, were analyzed for percent distribution of scores by enrichment and by diet, with a Likelihood Ratio Chi-Square P-value reported for prediction of score by treatment and diet. The remaining data were normally distributed, hence were analyzed using PROC MIXED, a mixed linear model, with enrichment and diet as main effects, and the resulting enrichment*diet interaction. The majority of broilers in this study were scored “absent”/“pass” for contact dermatitis, hence the data could not be statistically analyzed and are presented descriptively, as percent distribution of scores within each treatment. Principal Component Analysis (PROC PRIN QUAL) was used to test for redundancy and correlation within the qPCR outcomes and PROC CORR was used to provide Pearson Correlation Coefficients between variables. For all measures, a value of P ≤ 0.05 was considered significant.
Results
Performance
Feed intake in the first 2 weeks was unaffected by either treatment, but in the grower period (wk 2–4), there was a significant enrichment by diet interaction (P = 0.031), with both the LASER treatment and algae diet increasing FI (Table 2). LASER treatment increased feed intake by 0.07 kg, while the effect of dietary algae increased FI by 0.08 kg in the grower. In the finisher 1 period (wk 4–6), the effect of enrichment remained significant (P = 0.011), with LASER-enriched birds eating an average of 0.09 kg greater than their control counterparts. While there were no effects of diet on FI during finisher 1, in this period the algae-fed birds ate numerically less than birds fed the control diet (0.006 kg decrease). In wk 6–7 (finisher 2), neither enrichment nor dietary treatment affected broiler FI, although LASER-enriched birds maintained a numerically increased consumption of 0.03 kg (enrichment P = 0.246).
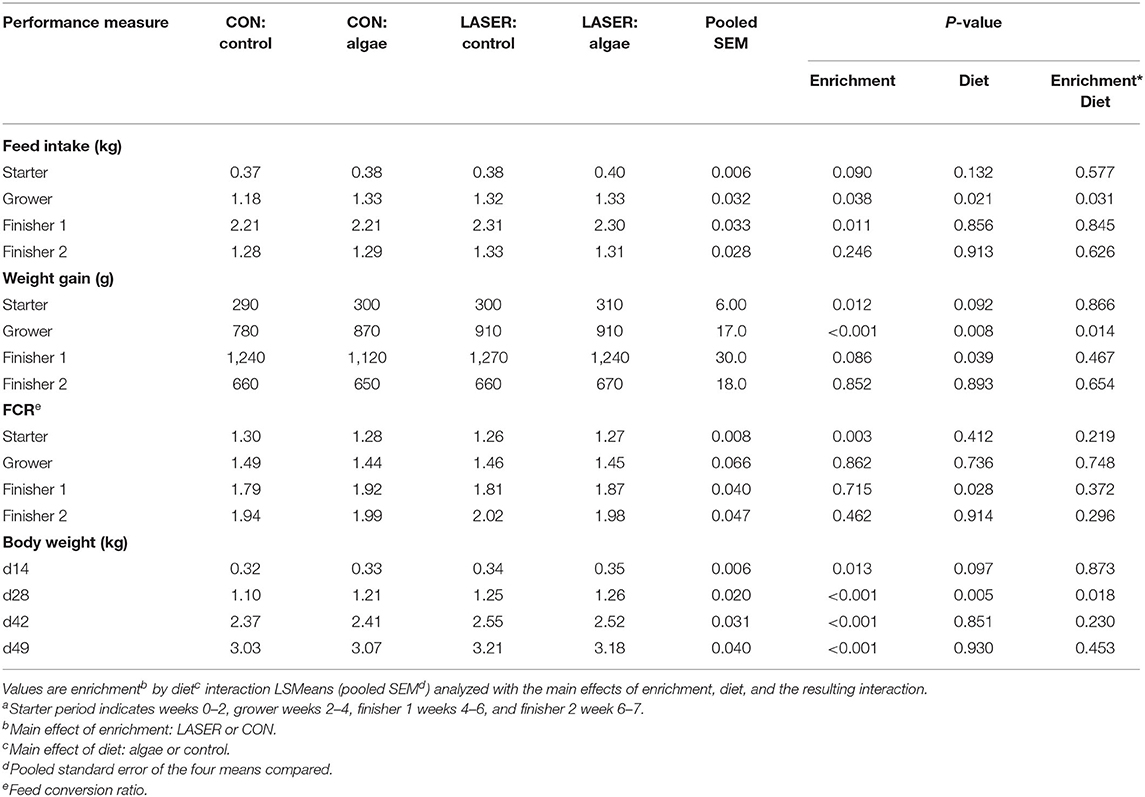
Table 2. Performance outcomes of Ross 708 straight run broilers averaged per bird by performance perioda.
Body weight at the end of the starter period (d14) was positively impacted by enrichment alone (P = 0.013), with LASER-enriched birds weighing 0.017 kg greater than CON; diet did not significantly impact weight gain. However, at this timepoint, algae-fed birds weighed 0.011 kg more than control-fed birds (diet P = 0.097). In the grower period, the enrichment by diet interaction was significant (P = 0.014), and by d28, average bird body weight was increased both due to enrichment and diet (interaction P = 0.018). LASER-enriched birds gained 0.083 kg more than the CON birds (enrichment P < 0.001), and algae-fed birds gained 0.049 more than the control-fed birds, irrespective of enrichment (diet P = 0.008). LASER-enriched birds weighed a significant 0.10 kg more than their CON counterparts at the end of wk 4 (enrichment P < 0.001), while birds fed the algae diet weighed 0.059 kg greater than those fed the control diet (diet P = 0.005).
During finisher 1 (wk 4–6), diet had a significant impact on body weight gain (diet P = 0.039); birds fed the algae ingredient gained 0.064 kg less than birds fed the control diet. LASER-enriched birds maintained a numerically increased gain above the CON in this period (averaged over diet) of 0.053 kg (enrichment P = 0.086). Upon finisher 1 conclusion (d42), enrichment alone impacted body weight (P < 0.001), with LASER-enriched birds weighing 0.144 kg more than CON broilers; there were no differences detected due to algae inclusion. Similarly, at d49, only enrichment affected end body weight (P < 0.001), with LASER-enriched birds reaching a 7-wk slaughter weight 0.148 kg above the CON; there were no differences due to dietary treatment at d49 (P = 0.453; Table 2). In the final study week, neither enrichment nor diet affected weight gain (P = 0.654; Table 2).
Feed conversion ratio (kg of feed consumed/kg of weight gain) was impacted only by the main effect of enrichment in the starter period (P = 0.003); with a 3-point decrease observed in LASER-enriched birds. In the grower period, there was no significant treatment effect on FCR, but CON birds fed the control diet showed the numerically highest or least efficient feed conversion (P = 0.731). In wk 4–6 (finisher 1), diet alone affected FCR (P = 0.028), with a significant 9-point increase due to algae inclusion. In this period, birds fed the control diet in the CON enrichment treatment had the numerically lowest FCR, along with lowest body weights. In wk 6–7 (finisher 2 period), neither enrichment nor diet impacted FCR, but the lightest treatment group, CON birds fed the control diet, maintained the numerically lowest FCR (P = 0.296; Table 2).
Breast Blisters and Footpad Dermatitis
Breast blisters were scored “absent” on all focal birds through wk 1–6 of the current study. Footpad dermatitis was scored “pass” on all focal birds, wk 1–5. On wk 6, 12 out of 70 focal birds scored a “fail” for footpad dermatitis. Two affected broilers were in the CON treatment, and 10 were broilers in the LASER treatment. Four of the affected broilers were fed control diets and 8 were supplemented with algae.
Breast Muscle Width and Weight
Breast width was significantly affected by enrichment (P < 0.001) at all timepoints measured, beginning at wk 3. At d22, LASER broiler breast widths measured 0.49 cm greater than CON (P < 0.001), and at d29 the LASER breasts were 0.43 cm greater (P < 0.001). This enrichment effect on width was maintained throughout d36 (0.48 cm increase; P < 0.001), d42 (0.48 cm increase; P < 0.001), and d49 (0.47 cm increase; P = 0.006). The main effect of algae diet was significant only at d49 (P = 0.015), where algae-fed broiler breasts widths were 0.41 cm smaller than control-fed. The right breast filet weight, removed from 100 broilers/timepoint at 6 and 7-wk slaughter, was not affected by enrichment or diet at either endpoint (P = 0.054; Table 3).
Woody Breast Score
Woody breast scores assigned on a 0–3 scale by 0.5 increments are presented as percent distribution of score. At the 6-wk timepoint, scores in the current study ranged from 0 to 1 (normal to mild WB). A greater percentage of LASER-enriched broiler breast scores fell into the normal category; of the 100 breasts scored at d42, 72% of LASER-enriched broiler breasts and 60.4% of CON broiler breasts received a score of 0 (no WB). Twenty percent of LASER-enriched broiler breasts and 27.1% of CON broiler breasts received a normal to mild score of 0.5. Eight percent of LASER-enriched broiler breasts and 12.5% of CON broiler breasts were scored 1 (mild WB; P = 0.4701, Figure 1A).
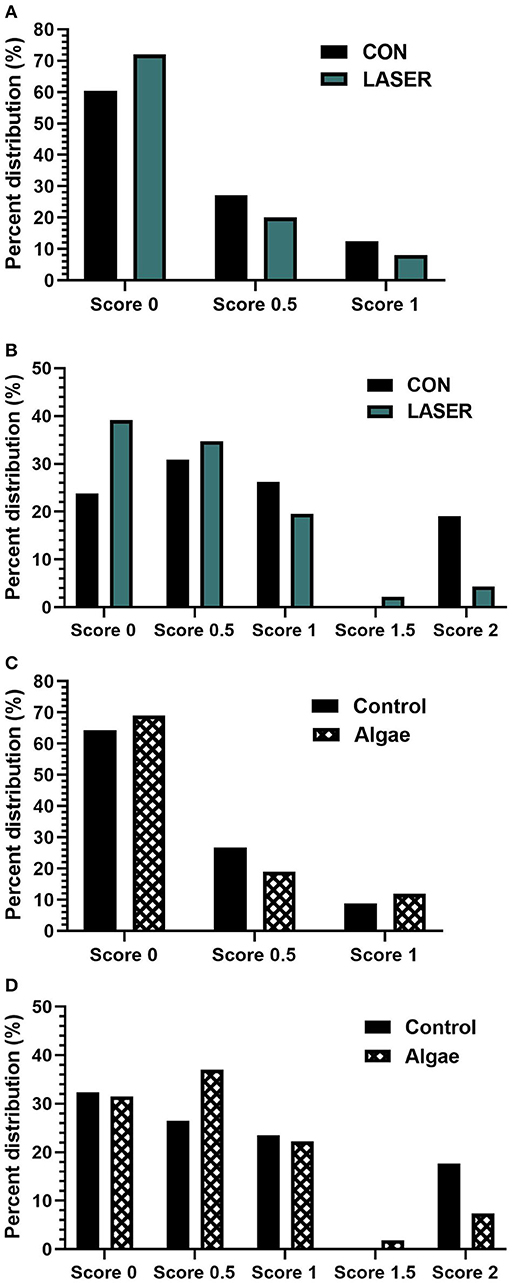
Figure 1. (A–D) Distribution of Ross 708 broiler woody breast score (n = 100) on (A) d42 and (B) d49 by enrichment and (C) d42 and (D) d49 by dietary treatment.
Analyzed by diet, at d42 a greater percent of algae-fed broiler breasts fell into the normal (score 0) and mild WB (score 1) categories, while a greater percentage of control birds fell into the normal to mild (0.5) category. Sixty nine percent of algae-fed broiler breasts scored 0 compared to 64.3% in the control-fed broilers. Nineteen percent of algae-fed broiler breasts and 26.8% of control-fed broiler breasts scored 0.5. In the mild WB category, 11.9% of algae-fed broiler breasts and 8.9% of control-fed broiler breasts were scored 1 (P = 0.6335; Figure 1C).
At wk 7, WB scores ranged between 0 and 2. Consistent with the 6-wk results, a greater percentage of LASER-enriched broiler breasts were scored 0: 39.1% of LASER-enriched broiler breasts and 23.8% of CON broiler breasts. This is an outcome of 15.3% greater unaffected filet scores in the LASER-enriched broiler breasts compared to the CON. In the 0.5 normal to mild category, indicating hardness beginning in the cranial region of the filet only, 34.8% of LASER-enriched broiler breasts and 31% of CON broiler breasts received this score. 19.6% of LASER-enriched broiler and 26.2% of CON broiler breasts received a score of 1 (mild). No CON bird breasts were scored 1.5, while 2.2% of LASER-enriched broiler breasts fell into this category. In the moderate WB category, 4.4% of LASER-enriched broiler breasts and 19.1% of CON broiler breasts were scored 2. This indicates that roughly 15% less LASER-enriched broiler breasts scored were affected with moderate WB (P = 0.0958, Figure 1B).
When analyzed by diet, a comparable percent of algae and control-fed broiler breasts were scored normal at d49; 31.5 and 32.4%, respectively. In the normal to mild category, there was a greater percentage of algae-fed broiler breasts, with 37% of algae-fed and 26.5% of control-fed broiler breasts receiving the score 0.5. Within the mild WB category, 22.2% of algae-fed and 23.5% of control-fed broiler breasts received a score of 1. No control-fed broiler breasts received a score of 1.5 (mild to moderate WB); 1.85% of algae-fed broiler breasts fell into this category. A greater percentage of control-fed broiler breasts fell into the moderate WB category, with 17.7% of control and 7.41% of algae broiler breasts receiving a score of 2, indicating a 10.3% reduction in moderate WB scores at d49 in the algae diet (P = 0.4653; Figure 1D).
Breast Muscle QPCR
Results obtained from qPCR are presented as Adjusted Ct values, calculated relative to the ubiquitously expressed chicken 28s gene. LASER enrichment significantly upregulated gene expression of MyoG (P = 0.009), MRF4 (P < 0.001), IGF1 (P = 0.001), and MSTN (P = 0.006), compared to the control (Figure 2A). MyoG was upregulated by 4.7%, MRF4 by 8.5%, IGF1 by 4.5%, and MSTN by 3.3% in the LASER treatment. Expression of MyoD trended toward significance to be upregulated in LASER broiler breast tissue (P = 0.072). The diet effect was significant in the expression of MRF4 (P = 0.013) and IGF1 (P = 0.015), with the control-fed birds showing an upregulation of 5% in MRF4 expression and 3.3% in IGF1 expression (Figure 2B). The expression of IGF2 was trending to be upregulated in the control diet (P = 0.089). The enrichment*diet interaction significantly affected expression of MyoD only (P = 0.012), but differences existed between means within each gene, apart from IGF2 (Figure 2C).
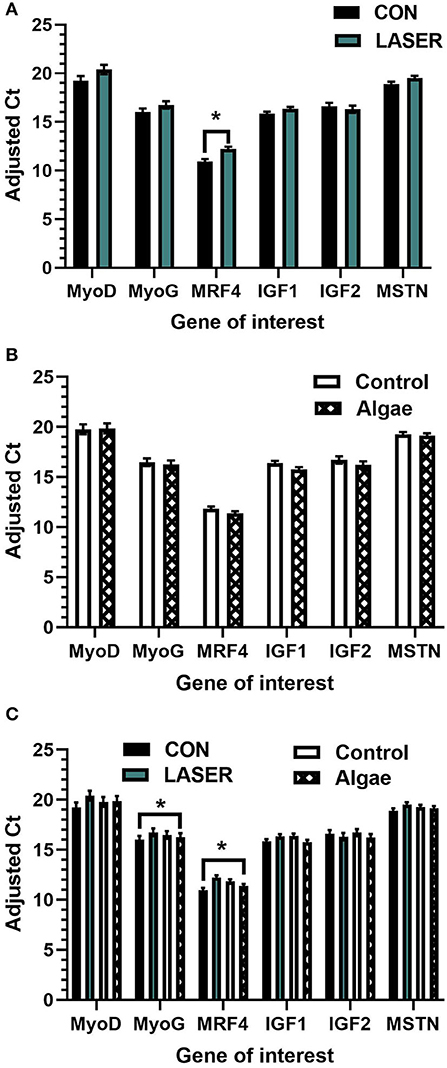
Figure 2. (A–C) Relative adjusted Ct values obtained from real-time qPCR using mRNA extracted from cranial portion of Ross 708 broiler pectoralis major tissue on d42 (n = 30 breast muscle samples). LSMeans (± SEM) are presented by main effect of (A) enrichment (B) diet, and (C) the enrichment*diet interaction1. *indicates statistical differences within each gene (P ≤ 0.05).
Breast tissue was collected and analyzed from two study timepoints, d42 and d49, hence main effect of day of slaughter (age) was analyzed. Day of slaughter significantly impacted the expression of IGF1 (P < 0.001) and IGF2 (P < 0.001), with 6.8 and 8.6% increased expression, respectively, on d49 (Figure 3A). Neither the enrichment x day nor diet x day interactions were significant across any of the genes tested, but differences existed between means within individual genes, presented for reference (Figures 3B,C).
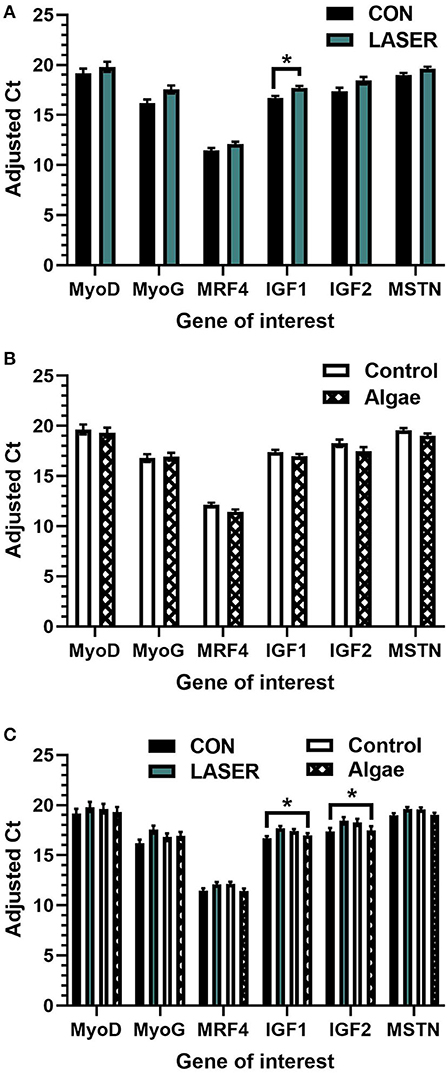
Figure 3. (A–C) Relative adjusted ct values obtained from real-time qPCR using mRNA extracted from cranial portion of Ross 708 broiler pectoralis major tissue on d49 (n = 30 breast muscle samples). LSMeans (± SEM) are presented main effect of (A) enrichment (B) diet, and (C) the enrichment*diet interaction1. *indicates statistical differences within each gene (P ≤ 0.05).
A principal component analysis was performed to test for redundancy and correlation within the qPCR data. A moderate positive correlation exists between MRF4 and IGF1 (r = 0.69) and IGF1 and IGF2 (r = 0.61) expression. A weak positive correlation exists between MyoD and MyoG expression (r = 0.54), MyoD and MRF4 expression (r = 0.46), MyoD and IGF1 expression (0.36), MyoG and MRF4 expression (r = 0.59), MyoG and IGF1 expression (r = 0.49), MyoG and IGF2 expression (r = 0.50), MyoG and MSTN expression (r = 0.53), MRF4 and IGF2 expression (r = 0.43), MRF4 and MSTN expression (r = 0.55), IGF1 and MSTN expression (r = 0.46), and IGF2 and MSTN expression (r = 0.57). Remaining correlation coefficients were non-significant.
Air and Litter Quality
Litter scored “pass” for friability throughout wk 1–7 of the study in both rooms. Ammonia test strip ranges were the same in both rooms on wk 1–7, respectively: 5, 5, 5, 5, 10, 20, and 20 ppm. When utilizing a handheld ammonia monitor at bird height, the readings remained similar between each room of the barn. In the LASER room, readings were detected at: 0, 3, 4, 4, 4, 7, and 9 ppm, wk 1–7, respectively. In the CON room, readings were detected at: 0, 4, 4, 4, 4, 9, and 9 ppm, wk 1–7, respectively.
Discussion
The current study aimed to identify independent and cumulative effects of two tools used to improve broiler welfare and sustainable production: environmental enrichment and an algae feed ingredient. Environmental enrichment is designed to improve animal welfare indicators, particularly in terms of increased natural behavior expression, and should improve biological outcomes (Newberry, 1995; Riber et al., 2018). Enrichment is of growing interest to consumers and is being researched and implemented by poultry producers as a sustainable strategy to improve animal welfare, and is now required in some auditing guidelines (USDA, 2014; United Egg Producers, 2017; Perdue Foods, 2018; Christensen, 2020). Inclusion of Spirulina algae (Arthrospira platensis) in the diet is growing in popularity as a natural, antibiotic alternative, growth promotant, immunostimulant, and protein replacer (Mirzaie et al., 2018; Pestana et al., 2020).
Laser enrichment alone increased broiler live weight at the conclusion of each performance period, notably at the 6- and 7-wk timepoints, with LASER-enriched birds finishing at 0.14 (d42) and 0.15 (d49) kg greater body weight than CON broilers. Importantly, birds in the LASER treatment managed this increased BW without sacrificing FCR, arguably the most critical production outcome for improved sustainability. FCR was reduced by 3 points in the starter period in LASER-enriched birds; feed alone accounts for >70% of broiler production costs, hence feed efficiency from placement is a major selection outcome to promote profitability (Chen et al., 2021). While economic value of FCR may fluctuate due to its multifactorial nature, a 17-point FCR reduction could reduce feed costs by 5% (Emmerson, 1997). Thus, the maintenance of FCR with increased marketable body weight is a relevant production outcome of LASER enrichment.
The improved growth performance observed in LASER-enriched birds agrees with previous work utilizing these laser devices, where enrichment resulted in an increased body weight of 0.24 kg/bird at d42 (Meyer et al., 2019). In contrast to the findings of the current study, in the initial laser work FCR was improved in each performance period and overall (7-point overall decrease). This difference may be due to the genetic strain and differing phenotype of growth rate/weight accretion (Ross 308 broilers used in the pilot study vs. Ross 708 broilers used in the current study) or the inclusion of the dietary algae component in the current work, which also produced differences in growth and feed intake. Ross 708 broilers (Aviagen, 2019a) have lower estimated body weight and FCR at d42 compared to Ross 308 (Aviagen, 2019b), as well as different estimated weight gain curves, which could certainly have impacted production outcomes. However, the improved growth performance across both studies and two genetic lines in LASER-enriched birds is a notable, consistent outcome that may be considered unexpected due to the increased exercise, attributed to innate foraging behavior, observed in both trials (Meyer et al., 2020, 2021).
Exercise has previously been associated with poorer feed conversion in egg-laying chicken strains (Akbar et al., 1985), and has been shown to have no effect on body weight or FCR in broilers, whether stimulated by lighting (Prayitno et al., 1997) or by increasing distance between resources (Reiter and Bessei, 2009; Ruiz-Feria et al., 2014). Modern broilers are reported to spend the vast majority of their time inactive, and only 3.3% of the day walking as they near market weight (Weeks et al., 2000). This is believed to be the result of an unenriched environment (Newberry, 1999), exercise-limiting stocking density (Sorensen et al., 2000), and genetic selection for a large proportion of breast and body weight associated with skeletal disorders (Kestin et al., 1992). The widely accepted theory is that selection for improved growth rate and FCR inadvertently favored birds “which were able to reduce subordinate energy expenditure (walking, exploring litter, running, etc.) because productivity traits sometimes conflict with behavioral traits” (Bizeray et al., 2000).
Thus, improved performance in LASER-enriched birds with simultaneously increased active behavior and distance walked observed over two studies (Meyer et al., 2020; Meyer et al., unpublished companion paper), is a relatively unique outcome. We hypothesize that the level of locomotion achieved by laser enrichment in our research is within an ideal range: beyond the minimum to access feed and water (Lewis and Hurnik, 1990), but not to the point of growth-inhibiting energy expenditure, as evidenced in performance outcomes. This outcome was similarly observed in a study involving free-range chickens of high- and low-exercise levels, where the high-exercise birds weighed more (Guo et al., 2019). However, these birds were an indigenous Chinese breed raised for a significantly longer period, 140 d, compared to our typical commercial birds raised only 35–70 d.
A performance benefit due to dietary algae inclusion was observed in the grower period, with increased FI and BW averaging 0.06 kg more in algae vs. control-fed birds at d28. Interestingly, this was the only performance period in which there was an enrichment*diet interaction, with LASER-enriched birds of both diets gaining and weighing significantly more than CON birds of either diet (Table 2). In contrast, in wk 4–6 (finisher 1 period), there was an increased weight gain and improved FCR in the control over algae-fed birds. This ultimately did not culminate in differing BW at d42 nor 49 due to diet, but was unexpected nonetheless. It is possible that main effects of laser enrichment outweighed detectable improvement due to diet in the finishing periods. Regardless, growth advantages during the grower period could be crucial to the smaller bird markets for improving weight gain, FCR, and sustainability.
Spirulina microalgae is rich in essential amino acids, lipids, vitamins, minerals, and antioxidants, and is considered to be a good source of protein (Spolaore et al., 2006; Soni et al., 2017). A lack of stronger performance response due to algae over 49 d in the current study is in contrast to some previous work, where broiler growth performance over 35 d was improved at lower inclusion levels, ≤ 1% (Park et al., 2018), and over 21 d at significantly higher inclusion levels, ≤ 16% (Evans et al., 2015). The Evans study reported that inclusion at 21% was detrimental to bird feed intake and performance, determining 16% inclusion of Spirulina at the upper limit (2015). In agreement with our data, work by Ross and Dominy (1990) with a more comparable grow-out period length showed no performance effects in broilers fed up to 12% Spirulina over 41d, and the authors reported slower growth in 12%-fed birds compared to 1.5, 3, and 6% inclusion. Similar to the outcomes reported here, Spirulina inclusion of 0.5–2% in the diet did not significantly impact broiler growth in a more recent study, but a numerically improved FCR was observed in a 1% inclusion treatment (Mirzaie et al., 2018).
Overall, LASER-enriched birds consistently outperformed CON birds, and apart from the finisher 1 period, algae-fed birds generally (numerically) outperformed birds fed the control diet. The effects of Spirulina in the diet were more subtle and potentially overshadowed by the exercise-inducing effects of laser enrichment on production parameters, but our data critically show that performance was not negatively impacted due to Spirulina partially replacing soybean meal as a dietary protein source. Based on market costs and availability, Spirulina could be used to reduce overall practical diet costs without a loss in performance, based on data reported here and the work of others.
Contact dermatitis is both an animal welfare and carcass quality issue associated with increased time spent in contact with poor quality litter (Bassler et al., 2013). Dermatitis of the breasts and feet affect 15–30% of broiler carcasses/week (Greene et al., 1985), causing considerable economic loss of two high value retail products. Breast dermatitis was not observed in the current study, likely due to both a lower stocking density than is typical commercially, as well as a relatively clean research setting. Additionally, litter remained dry and friable throughout wk 7 of the study. Footpad dermatitis costs the industry $280 million/year due to the value of broiler paws (Shepherd and Fairchild, 2010). In agreement with previous work in our lab, footpad dermatitis was not observed on wk 1–5 of the study (Meyer et al., 2019). However, a small number of affected footpads were observed on wk 6 (12 focal birds out of 70). These scores occurred in a limited number of pens, and it is unclear why they alone were affected. One potential explanation for dermatitis is ammonia, deriving from urea in the litter combining with moisture from excreta or drinkers, creating a chemical burn effect on the bird's skin (Haslam et al., 2006). However, ammonia measured at bird height in both rooms of the barn at wk 7 did not surpass 9 ppm using a titrated ammonia monitor, well below the NCC audit maximum of 25 ppm. A definitive correlation between footpad dermatitis and litter moisture has also been identified (Haslam et al., 2006), but litter quality in the current study was maintained through wk 7. The shift in protein source included in the diet may have played a role in dermatitis; only 4 of the affected broilers were control-fed compared to 8 algae-fed birds. Dietary protein source and all-vegetable diets in particular have been shown to affect litter moisture, and may hence increase footpad dermatitis with increased moisture (Eichner et al., 2007; Swiatkiewicz et al., 2017). Overall, it is unclear why a small proportion of pens in the same room/barn were affected by contact dermatitis and others were not, but the frequency of fail scores (12 out of 490 total scores) was too low to analyze statistically.
Pectoralis major muscle growth is an outcome relevant to broiler production and energy partitioning, measured in the current work by whole breast diameter on growing broilers and right breast filet weight post-mortem. LASER-enriched birds maintained consistently greater breast width than the CON from d22-d49, irrespective of dietary treatment, but no differences were detected due to enrichment or diet in filet weight at d42 or 49. This outcome may be important in terms of meat quality and saleable product. Despite the whole chicken breast muscle being the highest valued broiler meat cut (USDA, 2021), there are limited data exploring the effects of exercise on breast meat quality and proportion of breast muscle in broilers. These outcomes are important, because breast muscle myopathies leading to downgrades at slaughter, namely woody breast and white striping, have been repeatedly associated with heavier, thicker breast filets (Kuttappan et al., 2017; Hasegawa et al., 2020). In the current study, it appears that phenotypically, the breast dimension/shape was altered by laser enrichment, but resulting product weight was not increased.
Woody breast is most prevalent in heavier birds with correspondingly heavier breast filets (Sun et al., 2018; Zhang et al., 2019; Hasegawa et al., 2020). The negative effects on meat quality observed in woody breast filets are caused by muscle fiber degeneration and excessive connective tissue infiltration in an attempt to support the disproportionately large muscle (Tijare et al., 2016), but the underlying cause of woody breast remains unknown. However, it is well-established that prolonged exercise can alter the phenotype of skeletal muscle fibers. This effect has been observed in free-range vs. conventionally raised broilers by Yin et al. (2015). Further, exercise has been shown to stimulate capillary development, hence increasing blood supply, which leads to increased nutrient and oxygen delivery to skeletal muscles (Fluck, 2006). A major concern with the WB phenotype is lack of adequate blood supply due to muscle fiber breakdown, and a resulting lack of oxygen leading to metabolically starved tissue (Pampouille and C. Hennequet-Antier, 2019; Velleman, 2019; Baldi et al., 2020). Hence, movement motivated by laser enrichment described in the companion work (Meyer et al., 2021) may have helped to improve breast meat quality and reduce WB severity by stimulating more healthy muscle growth and circulation. This is evidenced by the reduction in WB scores due to laser enrichment. At wk 6, LASER-enriched broiler breasts had a 20% increase in normal filet scores compared to the control, and a 56% reduction in mildly affected filets. By wk 7, all WB scores had increased in severity, shifting toward moderate scores, but LASER-enriched broiler breasts maintained a 64% increase in normal scores compared to CON, and a striking 334% reduction in moderate scores compared to the CON. This outcome provides evidence that the degree of muscle damage was limited in the laser enrichment treatment.
This result is corroborated by a recent study where free-range chickens with more exercise culminated in carcasses with improved meat quality (increased water-holding capacity and decreased shear force values), as well as a decreased percentage of breast weight (Guo et al., 2019). Work by Yin et al. (2015) showed that free-range exercise increased the muscle fiber diameter of the pectoralis major. In another study, increased broiler activity due to vertical panel enrichment increased muscle width of the lower leg, but width of the breast was not measured (Tahamtani et al., 2020). To the authors' knowledge, there are no current data available evaluating the effects of exercise on WB directly.
The effect of dietary Spirulina algae on WB severity was less clear; at wk 6 slaughter, algae-fed broiler breasts had 6% increased normal scores compared to control-fed birds, however, algae-fed broiler breasts showed 34% increased mild scores compared to control-fed, with more control-fed broiler breasts falling into the normal to mild (0.5) category. At wk 7, percentage of algae- and control-fed WB scores of normal were nearly identical, but there was a 139% reduction in moderate scores in algae-fed broiler breasts compared to the control. Overall, an increase in normal scores was observed at 6 weeks of age and a reduction in moderate scores were observed at 7 weeks of age in the algae diet, a positive outcome that has not been previously studied and requires further validation irrespective of environmental enrichment studied here.
mRNA expression of specific genes involved in skeletal muscle metabolism and myogenesis was examined to determine effects of voluntary exercise using breast tissue collected at wk 6 and 7 slaughter. Yin et al. (2015) collected myogenic regulatory factor gene expression data in thigh and breast muscles of broilers raised conventionally vs. free-range but saw no differences in gene expression within the pectoralis major. In the current study, gene expression of MyoG was upregulated due to laser enrichment alone. Myogenin is a transcription factor involved in skeletal muscle myogenesis and repair that is necessary to form myotubes and mature muscle fibers (Ganassi et al., 2020), and has been shown to be increased in the thigh muscles of free-range chickens compared to pen-raised birds (Yin et al., 2015). An increase in this transcription factor could be considered a sign of muscle homeostasis, as MyoG regulates fusion of myocytes, thus determining muscle fiber characteristics (Ganassi et al., 2020).
Myogenic-regulatory factor 4, a gene solely expressed in skeletal muscle that regulates muscle mass (Schiaffino et al., 2018), was upregulated by 8.5% in the breast tissue of LASER-enriched broilers. Expression of MRF4 is related to myoblast differentiation and hypertrophy along with exercise; lack of this protein causes myopathies (Hernandez-Hernandez et al., 2017). Although MRF4 is a negative regulator of skeletal muscle growth (Moretti et al., 2016), this gene has also been upregulated in thigh muscles of broilers with free-range access (Yin et al., 2015), and was increased in the semitendinosus of pigs with increased exercise (Kalbe et al., 2018). In the data reported here, MRF4 expression was reduced in algae-fed broiler breast tissue compared to the control diet, an unexpected outcome that was likely reflected in the reduced breast width observed in algae-fed broilers at d49.
Insulin-like growth factor 1 expression was increased in LASER-enriched broiler breast tissue; IGF1 is a key growth factor in skeletal muscle believed to regulate muscle fiber hypertrophy and regeneration (Philippou et al., 2007). mRNA expression of IGF1 has been previously associated with post-hatch muscle growth in chickens (Zhang et al., 2019). Like MRF4, IGF1 expression was increased in control-fed broiler breast tissue compared to algae, which may again have been reflected in the reduced breast width observed in algae-fed birds at d49. Additionally, IGF1 expression was increased at the d49 timepoint compared to d42, irrespective of treatment, an outcome that likely reflects the growth and increased broiler breast muscle mass between wk 6 and 7 of slaughter (Velloso, 2008). IGF2, another factor necessary for skeletal muscle growth in chickens (Zhang et al., 2019) was likewise increased at d49, irrespective of treatment. This evidence of continued growth was supported by increased breast width between d42-49. We did not observe an increase in IGF2 gene expression in LASER-enriched broiler breast tissue, in contrast to findings by Kalbe et al. (2018), where IGF2 was upregulated in semitendinosus muscles of pigs with increased locomotion.
Myostatin is a negative regulator of muscle growth, and a lack of this protein results in excessive muscle size lacking corresponding increase in force (Amthor et al., 2007). In contrast to our findings, MSTN expression was decreased in semitendinosus muscles of pigs with increased exercise (Kalbe et al., 2018). As breast muscle width was significantly increased in LASER-enriched broilers with the same overall filet weight, the increased MSTN expression observed may be an indicator of healthy muscle growth, as MSTN is a key regulator of muscle mass. Lack of MSTN has been associated with decreased muscle function in mice (Amthor et al., 2007). Alternatively, MSTN expression in broiler breast muscle has been negatively correlated with bird and muscle weight at 90–150 days of age (Dou et al., 2018). This differs from the current results, where MSTN expression was positively increased with breast muscle width and body weight (breast filet weight unaffected by laser enrichment). Differences in both myogenic growth potential measured through mRNA expression as well as changes in macroscopic texture to the breast tissue observed here are likely due to laser-induced exercise (Meyer et al., 2021). Dietary algae did not impact breast width nor weight, apart from a notable exception at the 7-wk slaughter timepoint. On d49, control-fed birds had significantly greater breast width compared to the algae treatment. It is unclear why algae-fed birds would have a lower proportion of breast tissue on d49; to the authors' knowledge, there are no existing data indicating that Spirulina in the diet would alter breast muscle yield. Most likely, body weight, impacted largely by laser enrichment, skewed results when observed by main effect of diet alone.
In summary, these data provide further evidence of the laser enrichment device's effectiveness in improving broiler performance in hybrid, fast-growing commercial genetic lines. A novel outcome of this study was consistently increased breast width in broilers exposed to laser enrichment, without an increase in breast weight that may be associated with the damaging woody breast myopathy. Increased expression of myogenic growth-related genes and transcription factors in LASER-enriched broiler breast tissue, along with reduced severity of WB tactile score through week 7, indicate that voluntary exercise visually stimulated throughout week 5 by the laser device (Meyer et al., unpublished companion paper) had a positive impact on pectoralis major muscle growth in Ross 708 broilers. Dietary Spirulina algae also appeared to reduce severity of WB scores at d42 and 49, but decreased gene expression of muscle-growth and regulatory factors MRF4 and IGF2. Further, this study showed a positive effect of 2.5% Spirulina algae inclusion in the diet in grower period performance. This additive ultimately did not increase broiler body weight and may have been fed at an inclusion level lower than necessary for optimal results.
The increased activity and weight gain observed in LASER-enriched birds did not negatively impact animal welfare through the measures collected, with no effects detected on contact dermatitis or environmental conditions. Future research utilizing the laser enrichment with a focus on breast meat quality as well as growth and welfare, particularly in a commercial setting, is warranted. Additional work studying increased inclusion of Spirulina algae in the broiler diet is also necessary. The laser enrichment device described here is suitable for commercial application, as it could be used over multiple flocks without disrupting biosecurity, animal husbandry, or mortality. With refinement and validation in a commercial-scale setting, it may serve as an enrichment option that improves activity and performance simultaneously.
Data Availability Statement
The raw data supporting the conclusions of this article will be made available by the authors, without undue reservation.
Ethics Statement
The animal study was reviewed and approved by Iowa State University Institutional Animal Care and Use Committee IACUC #19–322.
Author Contributions
MM, AJ, and EB contributed to conception and design of the study. MM and EB contributed to on-farm animal care, data collection, statistical analysis, and manuscript drafts. All authors contributed to manuscript revision, read, and approved the submitted version.
Funding
This study was support from the Department of Animal Science, College of Agriculture and Life Sciences at Iowa State University and USDA for partial funding of EB and AJ salary, and from USDA Grant # 2019-69012-29905 for partial funding of EB salary, PhD student, and project funding.
Conflict of Interest
The authors declare that the research was conducted in the absence of any commercial or financial relationships that could be construed as a potential conflict of interest.
Publisher's Note
All claims expressed in this article are solely those of the authors and do not necessarily represent those of their affiliated organizations, or those of the publisher, the editors and the reviewers. Any product that may be evaluated in this article, or claim that may be made by its manufacturer, is not guaranteed or endorsed by the publisher.
Acknowledgments
The authors would like to thank the Poultry Research and Teaching Farm staff for help in animal husbandry and barn management, as well as M. S. student Emiline Sundman and Dr. Cassandra Stambuk and various undergraduate students for help on-farm mixing diets and weighing birds.
References
Akbar, M. K., Gavora, J. S., and Fairfull, R. W. (1985). Relationship between activity at a young age and feed efficiency in chickens. Poult. Sci. 64, 1402–1404. doi: 10.3382/ps.0641402
American Association of Avian Pathologists (2015). Animal Welfare and Management Committee. Available online at: https://www.aaap.info/animal-welfare (accessed September, 2021).
American Veterinary Medical Association (2020). AVMA Guidelines for the Euthanasia of Animals. Available online at: https://www.avma.org/sites/default/files/2020-01/2020-Euthanasia-Final-1-17-20.pdf (accessed September, 2021).
Amthor, H., Macharia, R., Navarrete, R., Schuelke, M., Brown, S. C., Otto, A., et al. (2007). Lack of myostatin results in excessive muscle growth but impaired force generation. Proc. Natl. Acad. Sci. USA. 104, 1835–1840. doi: 10.1073/pnas.0604893104
Aviagen (2019a). Ross 708 As-Hatched Performance. Aviagen Ross 708 Performance Objectives. Available online at: https://en.aviagen.com/assets/Tech_Center/Specialty_Males/EPxRoss708-BroilerPO2019-EN.pdf (accessed September, 2021).
Aviagen (2019b). Ross 308 As-Hatched Performance. Aviagen Ross 308 Performance Objectives. Available online at: https://en.aviagen.com/assets/Tech_Center/Ross_Broiler/Ross308-308FF-BroilerPO2019-EN.pdf (accessed September, 2021).
Baldi, G., Soglia, F., and Petracci, M. (2020). Current status of poultry meat abnormalities. Meat Muscle Biol. 4, 1–7. doi: 10.22175/mmb.9503
Bassler, A. W., Arnould, C., Butterworth, A., Colin, L., De Jong, I. C., Ferrante, V., et al. (2013). Potential risk factors associated with contact dermatitis, lameness, negative emotional state, and fear of humans in broiler chicken flocks. Poult. Sci. 92, 2811–2826. doi: 10.3382/ps.2013-03208
Baxter, M., Bailie, C. L., and O'Connell, N. E. (2019). Play behaviour, fear responses and activity levels in commercial broiler chickens provided with preferred environmental enrichments. Animal 13, 171–179. doi: 10.1017/S1751731118001118
Becker, E. W.. (2007). Micro-algae as a source of protein. Biotech. Adv. 25, 207–210. doi: 10.1016/j.biotechadv.2006.11.002
Bizeray, D., Estevez, I., Leterrier, C., and Faure, J. M. (2002). Effects of increasing environmental complexity on the physical activity of broiler chickens. Appl. Anim. Behav. Sci. 79, 27–41. doi: 10.1016/S0168-1591(02)00083-7
Bizeray, D., Leterrier, C., Constantin, P., Picard, M., and Faure, J. M. (2000). Early locomotor behaviour in genetic stocks of chickens with different growth rates. Appl. Anim. Behav. Sci. 68, 231–242. doi: 10.1016/s0168-1591(00)00105-2
Chen, C., Su, Z., Li, Y., Luan, P., Wang, S., Zhang, H., et al. (2021). Estimation of the genetic parameters of traits relevant to feed efficiency: result from broiler lines divergent for high or low abdominal fat content. Poult. Sci. 100, 461–466. doi: 10.1016/j.psj.2020.10.028
Christensen, K.. (2020). Producing Food for the World and Caring for the Animals We Depend On. Available online at: https://thefeed.blog/2020/09/30/animal-welfare-broiler-research-farm/ (accessed September, 2021).
Dou, T., Li, Z., Wang, K., Liu, L., Rong, H., Xu, Z., et al. (2018). Regulation of myostatin expression is associated with growth and muscle development in commercial broiler and DMC muscle. Mol. Biol. Rep. 45, 511–522. doi: 10.1007/s11033-018-4187-7
Dunkley, C. S.. (2007). The Poultry Site. Leg Problems in Broilers. Available online at: https://www.thepoultrysite.com/articles/leg-problems-in-broilers (accessed September, 2021).
Eichner, G., Vieira, S. L., Torres, C. C., Coneglian, J. L. B., Freitas, D. M., and Oyarzabal, O. A. (2007). Litter moisture and footpad dermatitis as affected by diets formulated on an all-vegetable basis or having the inclusion of poultry by-product. J. Appl. Poult. Res. 16:3. doi: 10.1093/japr/16.3.344
Emmerson, D. A.. (1997). Commercial approaches to genetic selection for growth and feed conversion in domestic poultry. Poult. Sci. 76:8. doi: 10.1093/ps/76.8.1121
Evans, A. M., Smith, D. L., and Moritz, J. S. (2015). Effects of algae incorporation into broiler starter diet formulations on nutrient digestibility and 3 to 21 d bird performance. J. Appl. Poult. Res. 24, 344–350. doi: 10.3382/japr/pfv027
Farm Animal Welfare Council Council (1979). Five Freedoms in The National Archives. Available online at: https://webarchive.nationalarchives.gov.uk/ukgwa/20121010012427/http://www.fawc.org.uk/freedoms.htm (accessed September, 2021).
Fluck, M.. (2006). Functional, structural and molecular plasticity of mammalian skeletal muscle in response to exercise stimuli. J. Exp. Biol. 209, 2239–2248. doi: 10.1242/jeb.02149
Gall, S., Suyemoto, M. M., Sather, H. M. L., Sharpton, A. R., Barnes, H. J., and Borst, L. B. (2019). Wooden breast in commercial broilers associated with mortality, dorsal recumbency, and pulmonary disease. Avian Dis. 63, 514–519. doi: 10.1637/11995-111218-Case.1
Ganassi, M., Badodi, S., Wanders, K., Zammit, P. S., and Hughes, S. M. (2020). Myogenin is an essential regulator of adult myofibre growth and muscle stem cell homeostasis. eLife. 9:e60445. doi: 10.7554/eLife.60445
Greene, J. A., McCracken, R. M., and Evans, R. T. (1985). A contact dermatitis of broilers -clinical and pathological findings. Avian. Path. 14, 23–38. doi: 10.1080/03079458508436205
Guo, X., Wang, J., Chen, H., Su, H., Wang, Z., Wan, Y., et al. (2019). Effects of exercise on carcass composition, meat quality, and mRNA expression profiles in breast muscle of a Chinese indigenous chicken breed. Poult. Sci. 98, 5241–5246. doi: 10.3382/ps/pez415
Hasegawa, Y., Hara, T., Kawasaki, T., Yamada, M., Watanabe, T., and Iwasaki, T. (2020). Effect of wooden breast on postmortem changes in chicken meat. Food Chem. 315:126285. doi: 10.1016/j.foodchem.2020.126285
Haslam, S. M., Brown, S. N., Wilkins, L. J., Kestin, S. C., Warriss, P. D., and Nicol, C. J. (2006). Preliminary study to examine the utility of using foot burn or hock burn to assess aspects of housing conditions for broiler chicken. Br. Poult. Sci. 47, 13–18. doi: 10.1080/00071660500475046
Havenstein, G. B., Ferket, P. R., and Qureshi, M. A. (2003). Growth, livability, and feed conversion of 1957 versus 2001 broilers when fed representative 1957 and 2001 broiler diets. Poult. Sci. 82, 1500–1508. doi: 10.1093/ps/82.10.1500
Hernandez-Hernandez, J. M., Garcia-Gonzales, E. G., Brun, C. E., and Rudnicki, M. A. (2017). the myogenic regulatory factors, determinants of muscle development, cell identity and regeneration. Semin. Cell Dev. Biol. 72, 10–18. doi: 10.1016/j.semcdb.2017.11.010
Jarvis, T., Byron, M., Von Staden, M., Crist, C., Zhang, X., Rowe, C., et al. (2020). Quality differences in wooden and normal breast meat marinated with traditional and clean label marinades. Meat and Muscle Biol. 4:1. doi: 10.22175/mmb.9458
Kalbe, C., Zebunke, M., Losel, D., J. Brendle, J., Hoy, S., and Puppe, B. (2018). Voluntary locomotor activity promotes myogenic growth potential in domestic pigs. Nature. 8:2533. doi: 10.1038/s4159-018-20652-2
Kestin, S. C., Knowles, T. G., Tinch, A. E., and Gregory, N. G. (1992). Prevalence of leg weakness in broiler chickens and its relationship with genotype. Vet. Rec. 131, 190–194. doi: 10.1136/vr.131.9.190
Knowles, T. G., Kestin, S. C., Haslam, S. M., Brown, S. N., Green, L. E., Butterworth, A., et al. (2008). Leg disorders in broiler chickens: prevalence, risk factors and prevention. PLoS ONE 3:e1545. doi: 10.1371/journal.pone.0001545
Kuttappan, V. A., Hargis, B. M., and Owens, C. M. (2016). White striping and woody breast myopathies in the modern poultry industry: a review. Poult. Sci. 95, 2724–2733. doi: 10.3382/ps/pew216
Kuttappan, V. A., Owens, C. M., Coon, C., Hargis, B. M., and Vazquez-Anon, M. (2017). Incidence of broiler breast myopathies at 2 different ages and its impact on selected raw meat quality parameters. Poult. Sci. 96, 3005–3009. doi: 10.3382/ps/pex072
Lewis, N. J., and Hurnik, J. F. (1990). Locomotion of broiler chickens in floor pens. Poult. Sci. 69, 1087–1093. doi: 10.3382/ps.0691087
Lilburn, M. S., Griffin, J. R., and Wick, M. (2019). From muscle to food: oxidative challenges and developmental anomalies in poultry breast muscle. Poult. Sci. 98, 4255–4260. doi: 10.3382/ps/pey409
Madeira, M. S., Cardoso, C., Lopes, P. A., Coelho, D., Afonso, C., Bandarra, N. M., et al. (2017). Microalgae as feed ingredients for livestock production and meat quality: a review. Livestock Sci. 205, 111–121. doi: 10.1016/j.livsci.2017.09.020
Mason, G., Clubb, R., Latham, N., and Vickery, S. (2007). Why and how should we use environmental enrichment to tackle stereotypic behaviour? Appl. Anim. Behav. Sci. 102, 163–188. doi: 10.1016/j.applanim.2006.05.041
Meyer, M. M., Johnson, A. K., and Bobeck, E. A. (2019). A novel environmental enrichment device improved broiler performance without sacrificing bird physiological or environmental quality measures. Poult. Sci. 98, 5247–5256. doi: 10.3382/ps/pez417
Meyer, M. M., Johnson, A. K., and Bobeck, E. A. (2020). A novel environmental enrichment device increased physical activity and walking distance in broilers. Poult. Sci. 99, 48–60. doi: 10.3382/ps/pez581
Meyer, M. M., Johnson, A. K., and Bobeck, E. A. (2021). Laser enrichment device stimulates broiler laser-following behavior while increasing individual bird locomotion and pen-wide movement. In Proof. doi: 10.3389/fanim.2021.784408
Mirzaie, S., Zirak-Khattab, F., Hosseini, S. A., and Donyaei-Darian, H. (2018). Effects of dietary Spirulina on antioxidant status, lipid profile, immune response and performance characteristics of broiler chickens reared under high ambient temperature. Asian-Australas J. Anim. Sci. 31, 556–563. doi: 10.5713/ajas.17.0483
Moretti, I., Ciciliot, S., Dyar, K. A., Abraham, R., Murgia, M., Agatea, L., et al. (2016). MRF4 negatively regulates adult skeletal muscle growth by repressing MEF2 activity. Nat. Commun. 7, 1–12. doi: 10.1038/ncomms12397
Nääs, I. A., Paz, I. C. L. A., Baracho, M. S., Menezes, A. G., Bueno, L. G. F., Almeida, I. C. L., et al. (2009). Impact of lameness on broiler well-being. J. Appl. Poult. Res. 18, 432–439. doi: 10.3382/japr.2008-00061
National Chicken Council (2020). National Chicken Council Animal Welfare Guidelines and Audit Checklist For Broilers. Available online at: https://www.nationalchickencouncil.org/wp-content/uploads/2021/02/NCC-Animal-Welfare-Guidelines_Broilers_Sept2020.pdf (accessed September, 2021).
Newberry, R. C.. (1995). Environmental enrichment: Increasing the biological relevance of captive environments. Appl. Anim. Behav. Sci. 44, 229–243. doi: 10.1016/0168-1591(95)00616-Z
Newberry, R. C.. (1999). Exploratory behaviour of young domestic fowl. Appl. Anim. Behav. Sci. 63, 311–321. doi: 10.1016/S0168-1591(99)00016-7
Norring, M., Kaukonen, E., and Valros, A. (2016). The use of perches and platforms by broiler chickens. Appl. Anim. Behav. Sci. 184, 91–96. doi: 10.1016/j.applanim.2016.07.012
Pampouille, E., C. Hennequet-Antier, C., Praud, A., Juanchich, A., Brionne, E., Godet, T., et al. (2019). Differential expression and co-expression gene network analyses reveal molecular mechanisms and candidate biomarkers involved in breast muscle myopathies in chicken. Nature. 9:14905. doi: 10.1038/s41598-019-51521-1
Park, J. H., Lee, S. I., and Kim, I. H. (2018). Effect of dietary Spirulina (Arthrospira) platensis on the growth performance, antioxidant enzyme activity, nutrient digestibility, cecal microflora, excreta noxious gas emission, and breast meat quality of broiler chickens. Poult. Sci. 97, 2451–2459. doi: 10.3382/ps/pey093
Perdue Foods (2018). Commitments to Animal Care. Perdue Foods Commitments to Animal Care Program. Available online at: https://corporate.perduefarms.com/media/1920/commitments-to-animal-care-2018.pdf (accessed September, 2021).
Pestana, J. M., Puerta, B., Santos, H., Madeira, M. S., Alfaia, C. M., Lopes, P. A., et al. (2020). Impact of dietary incorporation of Spirulina (Arthrospira platensis) and exogenous enzymes on broiler performance, carcass traits, and meat quality. Poult. Sci. 99, 2519–2532. doi: 10.1016/j.psj.2019.11.069
Petracci, M., and Cavani, C. (2012). Muscle growth and poultry meat quality issues. Nutrients 4, 1–12. doi: 10.3390/nu4010001
Petracci, M., Mudalal, S., Soglia, F., and Cavani, C. (2015). Meat quality in fast-growing broiler chickens. World's Poult. Sci. J. 71, 363–374. doi: 10.1017/S0043933915000367
Philippou, A., Maridaki, M., Halapas, A., and Koutsilieris, M. (2007). The role of the insulin-like growth factor 1 (IGF-1) in skeletal muscle physiology. In Vivo. 21, 45–54.
Prayitno, D. S., Phillips, C. J., and Stokes, D. K. (1997). The effects of color and intensity of light on behavior and leg disorders in broiler chickens. Poult. Sci. 76, 1674–1681. doi: 10.1093/ps/76.12.1674
Reiter, K., and Bessei, W. (2009). Effect of locomotor activity on leg disorder in fattening chicken. Berl Munch Tierarztl Wochenschr 122, 264–270.
Riber, A. B., van de Weerd, H. A., de Jong, I. C., and Steenfeldt, S. (2018). Review of environmental enrichment for broiler chickens. Poult. Sci. 97, 378–296. doi: 10.3382/ps/pex344
Ross, E., and Dominy, W. (1990). The nutritional value of dehydrated, blue-green algae (Spirulina platensis) for poultry. Poult. Sci. 69, 794–800. doi: 10.3382/ps.0690794
Ruiz-Feria, C. A., Arroyo-Villegas, J. J., Pro-Martinez, A., Bautista-Ortega, J., Cortes-Cuevas, A., Narciso-Gaytan, C., et al. (2014). Effects of distance and barriers between resources on bone and tendon strength and productive performance of broiler chickens. Poult. Sci. 93, 1608–1617. doi: 10.3382/ps.2013-03421
Saadaoui, I., Rasheed, R., Aguilar, A., Cherif, M., Al Jabri, H., Sayadi, S., et al. (2021). Microalgal-based feed: promising alternative feedstocks for livestock and poultry production. J. Anim. Sci. Biotechnol. 12:76. doi: 10.1186/s40104-021-00593-z
Schiaffino, S., Dyar, K. A., and Calabria, E. (2018). Skeletal muscle mass is controlled by thr MRF4-MEF2 axis. Clin. Nutr. Metab. Care. 21, 164–167. doi: 10.1097/MCO.0000000000000456
Shepherd, E. M., and Fairchild, B. D. (2010). Footpad dermatitis in poultry. Poult. Sci. 89, 2043–2051. doi: 10.3382/ps.2010-00770
Sihvo, H.-K., Immonen, K., and Puolanne, E. (2013). Myodegenration with fibrosis and regeneration in pectoralis major muscle of broilers. Vet. Path. 51, 619–623. doi: 10.1177/0300985813497488
Soglia, F., Laghi, L., Canonico, L., Cavani, C., and Petracci, M. (2016). Functional property issues in broiler breast meat related to emerging muscle abnormalities. Food Res. Inter. 89, 1071–1076. doi: 10.1016/j.foodres.2016.04.042
Soni, R. A., Sudhakar, K., and Rana, R. S. (2017). Spirulina– From growth to nutritional product: A review. Tr. Food Sci. Technol. 69, 157–171. doi: 10.1016/j.tifs.2017.09.010
Sorensen, P., Su, G., and Kestin, S. C. (2000). Effects of age and stocking density on leg weakness in broiler chickens. Poult. Sci. 79, 864–870. doi: 10.1093/ps/79.6.864
Spolaore, P., Joannis-Cassan, C., Duran, E., and Isambert, A. (2006). Commercial applications of microalgae. J. Biosci. Bioengineer. 101, 87–96. doi: 10.1263/jbb.101.87
Sun, X., Koltes, D., Coon, C., Chen, K., and Owens, C. (2018). Instrumental compression force and meat attribute changes in woody broiler breast fillets during short-term storage. Poult. Sci. 97, 2600–2606. doi: 10.3382/ps/pey107
Swiatkiewicz, S., Archzewska-Wlosek, A., and Josefiak, D. (2017). The nutrition of poultry as a factor affecting litter quality and foot pad dermatitis- an updated review. J. Anim. Physiol. Anim. Nut. 101, e14–e20. doi: 10.1111/jpn.12630
Tahamtani, F. M., Pedersen, I. J., and Riber, A. B. (2020). Effects of environmental complexity on welfare indicators of fast-growing broiler chickens. Poult. Sci. 99, 21–29. doi: 10.3382/ps/pez510
Tijare, V. V., Yang, F. L., Kuttappan, V. A., Alvarado, C. Z., Coon, C. N., and Owens, C. M. (2016). Meat quality of broiler breast fillets with white striping and woody breast muscle myopathies. Poult. Sci. 95, 2167–2173. doi: 10.3382/ps/pew129
United Egg Producers (2017). Animal Husbandry Guidelines for U.S. Egg-Laying Flocks. UEP Certified Cage-Free Guidelines. Available online at: https://uepcertified.com/wp-content/uploads/2019/09/CF-UEP-Guidelines_17-3.pdf (accessed September, 2021).
USDA (2014). Humane Farm Animal Care; Animal Care Standards for Chickens. USDA Certified Humane: Standards and Forms. Available online at: https://2gn8ag2k4ou3ll8b41b7v2qp-wpengine.netdna-ssl.com/wp-content/uploads/Std19.Chickens.5M.pdf (accessed September, 2021).
USDA (2021). Retail Prices for Beef, Pork, Poultry Cuts, Eggs, and Dairy Products. USDA Economic Research Service; Meat Price Spreads. Available online at: https://www.ers.usda.gov/data-products/meat-price-spreads/ (accessed September, 2021).
Velleman, S. G.. (2019). Recent Developments in Breast Muscle Myopathies Associated with Growth in Poultry. Annu. Rev. Anim. Biosci. 7, 289–308. doi: 10.1146/annurev-animal-020518-115311
Velten, S., Neumann, C., Bleyer, M., Gruber-Dujardin, E., Hanuszewsja, M., Przybylska-Gornowicz, B., et al. (2018). Effects of 50 percent substitution of soybean meal by alternative proteins from Hermetia illucens or Spirulina platensis in meat-type chicken diets with graded amino acid supply. Open J. Anim. Sci. 82, 119–136. doi: 10.4236/ojas.2018.82009
Weeks, C. A., Danbury, T. D., Davies, H. C., Hunt, P., and Kestin, S. C. (2000). The behaviour of broiler chickens and its modification by lameness. Appl. Anim. Behav. Sci. 67, 111–125. doi: 10.1016/S0168-1591(99)00102-1
Yin, H., Li, D., Wang, Y., Zhao, X., Liu, Y., Yang, Z., et al. (2015). Myogenic regulatory factor (MRF) expression is affected by exercise in postnatal chicken skeletal muscles. Gene. 561, 292–299. doi: 10.1016/j.gene.2015.02.044
Keywords: broiler, environmental enrichment, breast muscle myopathy, woody breast, Spirulina algae
Citation: Meyer MM, Johnson AK and Bobeck EA (2021) Laser Environmental Enrichment and Spirulina Algae Improve Broiler Growth Performance and Alter Myogenic Gene Expression and pectoralis major Dimensions. Front. Anim. Sci. 2:784294. doi: 10.3389/fanim.2021.784294
Received: 27 September 2021; Accepted: 16 November 2021;
Published: 14 December 2021.
Edited by:
Vishwajit S. Chowdhury, Kyushu University, JapanReviewed by:
Arda Yildirim, Gaziosmanpaşa University, TurkeyTakashi Bungo, Hiroshima University, Japan
Copyright © 2021 Meyer, Johnson and Bobeck. This is an open-access article distributed under the terms of the Creative Commons Attribution License (CC BY). The use, distribution or reproduction in other forums is permitted, provided the original author(s) and the copyright owner(s) are credited and that the original publication in this journal is cited, in accordance with accepted academic practice. No use, distribution or reproduction is permitted which does not comply with these terms.
*Correspondence: Elizabeth A. Bobeck, ZWFib2JlY2smI3gwMDA0MDtpYXN0YXRlLmVkdQ==