- 1Department of Animal and Dairy Sciences, University of Wisconsin-Madison, Madison, WI, United States
- 2Department of Animal Sciences, University of Florida, Gainesville, FL, United States
- 3Department of Animal Sciences, Universite Laval, Québec City, QC, Canada
- 4Department of Large Animal Clinical Sciences, School of Veterinary Medicine, University of Florida, Gainesville, FL, United States
Dairy calves exposed to late-gestation heat stress weigh less, have impaired immunity, produce less milk across multiple lactations, and have reduced productive life. However, less is known about the relationship between in utero heat stress and organ morphology and development. Herein, we characterized the consequences of late-gestation in utero heat stress on body and organ growth trajectories during early-life development. Holstein heifers were either in utero heat-stressed (IU-HT, n = 36, dams exposed to THI > 68) or cooled (IU-CL, n = 37, dams exposed to THI > 68 with access to active cooling) during late gestation (54 ± 5 d prepartum). All heifers were reared identically from birth to weaning. Upon birth, calves were weighed and fed 3.78 L of colostrum followed by 0.87 kg DM/d milk replacer (MR) over two feedings and ad libitum starter concentrate daily. Weaning began at 49 d and ended at 56 d of age. Feed intake was recorded daily, and body weight (BW) and other growth measures were recorded at 0, 28, 56, and 63 d. Blood was collected at d 1 then weekly. Subsets of heifers were selected for euthanasia at birth and 7 d after complete weaning (n = 8 per group each) to harvest and weigh major organs. Reduced BW and stature measures persisted in IU-HT heifers from 0 to 63 d of age with a 7% lower average daily gain and reduced starter consumption relative to IU-CL heifers. IU-HT heifers had lower hematocrit percentages and reduced apparent efficiency of absorption of IgG relative to IU-CL heifers. Additionally, IU-HT heifers had reduced gross thymus, spleen, thyroid gland, and heart weight at birth and larger adrenal glands and kidneys but smaller ovaries relative to BW at 63 d. The mammary gland of IU-HT heifers was smaller relative to IU-CL heifers at birth and 63 d adjusted for BW, suggesting mechanisms leading to impaired milk yield in mature IU-HT cows are initiated early in development. In summary, in utero heat stress reduces whole-body size and limits development of key organs with potential repercussions on dairy calf metabolic adaptation, immune function, and future productivity.
Introduction
Elevated ambient temperature and humidity negatively impact dairy cattle productivity, welfare, and economy (St-Pierre et al., 2003; Becker et al., 2020). While the impact of heat stress on lactating cows is well-understood and documented, recent literature has demonstrated that late gestation heat stress leads to a host of negative repercussions in both dam and offspring (Tao et al., 2019; Dado-Senn et al., 2020a) with long-lasting multigenerational consequences on herd performance and longevity (Laporta et al., 2020). Early-life experiences, including the intrauterine thermal environment, can profoundly influence fetal growth and development (i.e., organ size, structure and cell function) and exert long-term effects on postnatal disease and productivity (Barker, 1998; Van Eetvelde and Opsomer, 2017; Reynolds et al., 2019). In utero heat stress, for instance, adversely alters dairy cattle growth, immune response, and lactation even years after the initial event occurred. Heifers born to late gestation heat-stressed dams are born earlier, weigh less from birth to weaning, and have impaired passive transfer of IgG (Tao et al., 2012a; Monteiro et al., 2014, 2016a). These heifers are less likely to survive to lactation and produce less milk across their first, second, and third lactations relative to in utero cooled counterparts (Monteiro et al., 2016b; Laporta et al., 2020).
The underlying causes for the perturbations in heifer growth, immune function, and mammary synthetic capacity are multi-factorial. The reduced birth weight of late-gestation in utero heat-stressed calves has been attributed, in part, to a shorter gestation length, direct effects of fetal hyperthermia, and diminished placental function (Reynolds et al., 2006; Tao et al., 2012b). Further, prenatally heat-stressed calves have both impaired passive transfer of colostrum IgG and altered cell-mediated immunity, suggested to be linked to accelerated gut closure from increased calf jejunum apoptosis and reduction in immune organ weights at birth, respectively (Tao et al., 2012a; Monteiro et al., 2014; Ahmed et al., 2021). Research in mature heifers born to heat-stressed dams has found alterations in the lactating mammary microstructure and epigenome, which may help explain the milk production losses (Skibiel et al., 2018a,b).
Despite this emerging research, there is still much to understand regarding the consequences of late-gestation in utero heat stress on organ morphology, growth, development, and function. Organs and tissues, particularly those involved in adaptation to physiological stress, are regulated by growth factors and mediators of cell death, which may influence organ size (Penzo-Mendez and Stanger, 2015). An increase in organ size is expected to support a larger calf but may also be related to improvements in organ development, metabolic activity, and productivity (Jenkins et al., 1986; Kamiya et al., 2009; Geiger et al., 2016b). However, there is scarce research assessing organ weights in response to hyperthermia in cattle. One study of in utero heat-stressed dairy bull calves reported that organs pertaining to calf metabolism and immunity (i.e., thymus, spleen, heart, and liver) weighed less in prenatally heat-stressed calves at birth (Ahmed et al., 2021); though only the thymus and spleen tended to differ relative to body weight. However, that study only characterized one window of time and thus may not explain differences in growth and immunity throughout life. Further, changes in mammary development and body growth trajectories could not be assessed. Notably, previous studies from our group conducted on late-gestation in utero heat-stressed mammary glands have utilized mature lactating heifers and have not captured potential prior perturbations to mammary gland development (Skibiel et al., 2018a,b). The objective of the current study was to assess the impact of late-gestation in utero heat stress on heifer growth trajectory and specific organ systems at key stages of early-life development (i.e., birth and weaning). We hypothesized that exposure to intrauterine hyperthermia would reduce heifer body size and organ weights at birth and limit growth across the pre-weaning period and that body and organ differences would remain after weaning.
Materials and Methods
Dam Treatment
All procedures within the following study were approved by the University of Florida Institutional Animal Care and Use Committee (protocol #201910599). This study was conducted from August to December 2020 in Florida, USA. Cows were housed at a commercial dairy farm, and calves were transported within 24 h of birth to a campus facility at the University of Florida where they remained until two weeks post-weaning.
Eighty-two multiparous pregnant Holstein dams bred to sexed semen were blocked by parity (1.3 vs. 1.6 ± 0.5 lactations; P = 0.62), mature-equivalent milk production (11,977.1 vs. 11,504.2 ± 1,675.3 kg; P = 0.45), and offspring sire and enrolled simultaneously on August 5, 2020 into two dry period treatments at ~56 d before estimated calving date which ranged from September 18 to October 3, 2020 (i.e., 15 days) for both groups. Cows were on average 311 days in milk (DIM) at the time of enrollment (310.8 vs. 311.8 ± 36 DIM; P = 0.31). Prior to enrollment, dams were managed identically with access to shade of a freestall barn, fans, and soakers.
Upon enrollment, cows were housed in a compost-bedded free-stall barn in separate adjacent pens and either heat-stressed (HT; access to shade of the barn and natural ventilation; n = 41) or cooled (CL; access to shade of the barn plus forced ventilation via fans, and water soakers; n = 41) for the duration of their dry period (54 ± 5 d; Supplementary Figures 1A,B). Fans (Typhoon Power P-51, Seneca Dairy Systems, NY) ran continuously, and water soakers were activated every 5 min for 1.5-min intervals when ambient temperature met or exceeded 22.2°C. Ambient temperature and relative humidity were recorded every 15 min and averaged hourly from August to October using two Hobo Pro Series Temp Probes (Onset Computer Corp., Pocasset, MA) placed in the HT and CL pens. Temperature-humidity index (THI) was calculated using the equation established by the NRC and suggested for use in subtropical environments (NRC, 1971; Dikmen and Hansen, 2009). To characterize heat stress exposure for all dams in the previous lactation (i.e., prior to dry period treatment), ambient temperature, relative humidity, and THI from early to mid-gestation (i.e., December 2019 to August 2020) was determined from daily data provided by the National Oceanic and Atmospheric Administration (NOAA; Supplementary Figure 2); average THI was 63 across this period. Dam respiration rates (RR, flank movements per minute) and skin temperatures (ST, rump, Raytek MiniTemp MT6 Infrared Thermometer; Instrumart, South Burlington, VT) were measured twice weekly at 12 h during the experimental period. Environmental factors including air speed (AS, MS6252A Digital Anemometer System, Proster, Miller Place, NY) and particle matter (fine and coarse: PM2.5 and PM10; LKC-1000S + Air Quality Monitor, Temtop, Milpitas, CA) were collected once weekly at 13 h in six separate locations within the pens (Supplementary Figure 1A). Heat stress was considered achieved when environmental THI exceeded estimated thresholds for both lactating and dry cows (i.e., 68; Zimbelman et al., 2009 and 77; Ouellet et al., 2021, respectively) and RR and ST were elevated relative to the cows under the cooling treatment.
Cows were fed far-off and close-up total mixed rations (TMR) ad libitum for the first three weeks and the following four weeks before expected calving, respectively, according to the standard operating procedures of the commercial dairy. Samples of TMR from the HT and CL pens were collected once weekly for chemical analysis (Cumberland Valley Analytical Services Inc., Waynesboro, PA). Ration formulation and chemical composition can be found in Supplementary Table 1. One week prior to expected calving and every three h, cows were monitored for signs of calving then moved to the maternity area upon exhibiting signs. Calving ease scores were recorded by one trained study personnel according to Piwczyński et al. (2013). Upon birth, calf and dam were separated and the dam was milked in the maternity area to collect colostrum.
Calf Treatments and Birth
There were n = 39 live calves born to HT dams over 24 days and n = 42 calves born to CL dams over 22 days. There were two aborted calves (n = 2 IU-HT) that were not included and one set of twin heifers (IU-CL) that were enrolled but excluded from euthanasia. Study exclusion criteria included bulls (n = 4 IU-CL; n = 3 IU-HT) and calves weighing <22.7 kg (n = 1 IU-CL). Thus, the remaining heifer calves born to these dams were considered either in utero heat-stressed (IU-HT; n = 36) or in utero cooled (IU-CL; n = 37) during late-gestation and enrolled in the study. The individual calf gestated in utero was considered the experimental unit, as the uterine environment would be specific to that dam.
After birth, calves were weighed, had their navels dipped prophylactically in 7% iodine tincture, and fed 3.78 L of high-quality colostrum via esophageal tubing within 2 h after birth (0.8 ± 0.5 h) unless assigned to birth euthanasia. Colostrum quality was determined by total protein concentration with a quality cut-off of 21% (digital BRIX refractometer; MA871, Milwaukee Instruments, Rocky Mount, NC; Quigley et al., 2013). A sample of colostrum fed to the calf was collected and stored at −20°C for analysis of IgG concentration. Vaccinations and other preventative health interventions were administered at birth and later at 35, 49, and 63 d of age (Supplementary Table 2).
Birth Euthanasia
A subset of heifers (n = 8 per in utero treatment) was euthanized at birth. Upon birth, these heifers were weighed (A and A Scales VS660; maximum capacity = 300 kg; precision = 0.10 kg) and had their navels dipped but did not receive colostrum nor vaccinations. Calves were euthanized 4.6 ± 2.3 h after birth via captive bolt and exsanguination. Organs and tissues of interest included: thyroid gland, adrenal gland, untrimmed (i.e., skin and hair remaining) and trimmed (i.e., skin and hair removed) mammary gland (MG), GI tract (stomach plus duodenum, jejunum, and ileum), ovaries, mesenteric lymph node, thymus, spleen, kidney, liver, pancreas, lungs, heart, and semitendinosus skeletal muscles. Time from euthanasia to completion of organ removal and tissue collection was approximately one h. Blood weight after exsanguination and carcass weight after organ removal were measured. All organs were weighed upon removal (Ohaus Scout STX223 Version 1.05; maximum capacity = 220 g; precision = 0.001 g or Houseables Industrial Platform Scale; maximum capacity = 300 kg; precision = 0.02 kg).
Calf Management From Birth to Weaning
Heifers not assigned to birth euthanasia (n = 28 IU-HT; n = 29 IUCL) were relocated within 24 h of birth to a University of Florida animal facility where they were managed identically and housed in individual sand-bedded pens under the shade of an open-sided barn with fans for ventilation (Supplementary Figures 1C,D). Calves were raised at this site from September to December of 2020 by personnel blinded to in utero treatment. Within 24 h of age, they were disbudded using caustic paste with analgesia (Supplementary Table 2). Calves were monitored daily and health status was assessed by trained study personnel and treated as needed by the university Food Animal Reproduction and Medicine Service on-call veterinarian. Health events, recorded as number of cases, and treatments were recorded daily at ~8 h by one trained study personnel for fever, bloat, diarrhea, respiratory disease, navel infections, localized infections, and total health events per calf (descriptions found in Supplementary Table 3). There was a 0% mortality rate among the managed calves herein. Ambient temperature and relative humidity were recorded every 15 min and averaged hourly (October to December, Hobo Pro Series Temp Probe), and THI of the postnatal environment was calculated.
Calves were fed 0.87 kg DM milk replacer per d (MR, UF Special 28/20 Bova Medicated, Southeast Milk, Okeechobee, FL) over two feeding periods at 6 and 17 h. The MR was offered as 0.45 kg MR mixed in 3.78 L warm water (mixing rate 120 g/L; 12.0% solids). Milk replacer intake (i.e., amount of MR refused subtracted from the amount of MR offered) was recorded daily and averaged weekly. Starter grain concentrate (Ampli-Calf Starter 20 Warm Weather, Purina Animal Nutrition LLC, Shoreview, MN) was offered ad libitum starting at 4 d of age, and concentrate intake was measured daily and averaged weekly from 28 to 63 d of age. Weaning began at 49 d of age and ended at 56 d of age whereby calves were fed 0.23 kg MR mixed in 1.89 L warm water for two feedings per d for one week then fully weaned off milk. Feed samples (MR and grain) were collected weekly and pooled biweekly for chemical analysis (Supplementary Table 1). At 60 d of age, calves were reassigned to group-housed pens where they received ad libitum starter grain concentrate and water.
Growth measures including body weight (BW), hip height, withers height, body length, chest girth, waist girth, and head circumference were measured from heifers at 0, 28 and 56 d of age, and BW was again recorded at 63 d of age (post-weaning). Average daily gain (ADG) was calculated as the difference in BW between 0 and 63 d of age, divided by 63. Blood was collected from a subset of calves (n = 15 per in utero treatment) at 23.6 ± 2 h after colostrum consumption and then weekly (7, 14, 21, 28, 35, 42, 49, 56, and 63 d of age) between 13 and 15 h. Blood was collected using serum and sodium heparin vacutainer blood collection tubes (BD catalog #366430 and 366480, Franklin Lakes, NJ). After collection, serum tubes clotted for 20 min at room temperature whereas sodium heparin tubes were placed on ice. Serum total protein (TP) was quantified from fresh plasma using a handheld Brix Refractometer (Brix Refractometer with ATC, Tiaoyeer, China; ±0.2% accuracy) and blood hematocrit was assessed via capillary tubes according to Dado-Senn et al. (2020b). Colostrum and d 1 serum were analyzed from a subset of calves (n = 15 per in utero treatment) for serum IgG concentration and apparent efficiency of absorption of IgG (% AEA; Quigley and Drewry, 1998) by radial immunodiffusion assay (Triple J Farms, Bellingham, WA) as described by Monteiro et al. (2014). The interassay CV was 6.8%.
Weaning Euthanasia
From the n = 57 heifers raised from birth to weaning, a subset of 16 (n = 8 per in utero treatment, born between September 26 and October 2) were randomly selected to be euthanized. Selected heifers remained individually housed from 60 to 63 d of age and were fasted for at least 15-h before euthanasia. Weaning euthanasia occurred over 2 days, and heifers were 62.9 ± 1.5 d old. Heifers were weighed then euthanized via captive bolt and exsanguination at the University of Florida abattoir. Organ harvest procedure was similar to that described for birth euthanasia.
Statistical Analysis
Overall sample size was determined assuming a 90% heifer-calf birthing rate and a power analysis for the primary outcome of interesting—birth body weight difference between birth or weaning-euthanized subgroups of calves (n = 8 per treatment per time; level of significance of 0.05; 85% power). Post-hoc power analyses for representative variables of interest, including liver organ weight, colostrum IgG, serum IgG and total protein, and specific health events were also run assuming a level of significance of 0.05 and 85% power. Sample size determination for these variables can be found in Supplementary Table 4, but in brief varied from n = 4 per treatment (liver organ weight) to n = 128 per treatment (total health events).
All data were analyzed in SAS (v. 9.4 SAS Institute, Cary, NC). Environmental data (temperature, humidity, and THI) were analyzed in PROC MEANS for descriptive statistics of daily average (average of all hours across one day) and hourly average (average of all days for each hour) for each respective collection period. Single timepoint variables including dam lactation number and mature equivalent milk production, calf birth weights (d 0), post-weaning weight (d 63), gestation length, organ weights at birth and weaning (both as gross weight and a per kg BW basis), and colostrum/serum IgG were analyzed as a two-sample t-test with the main effect of in utero treatment. Residuals were tested for normality, and block was included in the model. Birth and post-weaning weights were analyzed with the population of all heifers per treatment and for subgroups, including calves selected for birth euthanasia (n = 8 per in utero treatment, birth weight only), weaning euthanasia (n = 8 per in utero treatment), and remaining calves (n = 20 IU-HT, n = 21 IU-CL). Variables collected over time including dam respiration rates and skin temperatures and calf growth measures, intakes, and blood parameters were analyzed by PROC MIXED with the main effects of treatment, time (day or week, as repeated measure), and treatment by time interaction. Heifer ID within treatment was considered random, the first-order autoregressive covariance structure (AR-1) was used as the covariate structure, and residuals were tested for normality. Health events and calving ease were analyzed by PROC LOGISTIC to analyze the logistic regression and odds ratios, respectively. Unless otherwise stated, all birth data are reported from the population of all heifers and all other data are reported from the population of all heifers except those euthanized at birth. Data are presented as LSM ± SE, unless otherwise stated. Significance was declared at P ≤ 0.05 and tendency at 0.10 ≥ P > 0.05.
Results
Dam Outcomes
Pregnant dry HT dams had elevated RR (77.36 vs. 53.51 ± 0.63 bpm, HT vs. CL respectively, P < 0.001, Figure 1A) and ST (35.95 vs. 34.09 ± 0.05 °C, P < 0.001, Figure 1A) relative to CL dams across the dry period. Air speed was significantly lower in the HT pen (2.09 vs. 3.91 ± 0.13 m/s, P < 0.001, Figure 1B) and there was reduced air particle matter (P ≤ 0.05, Figure 1B) in the HT pen compared with the CL pen. Pen daily THI remained above 68 for 95% of the dam exposure (i.e., prenatal environment, Figure 2A) and above a more stringent threshold of 77 for 75% of the dam exposure (Zimbelman et al., 2009; Ouellet et al., 2021). Average hourly THI ranged from 75 to 83 (Figure 2C).
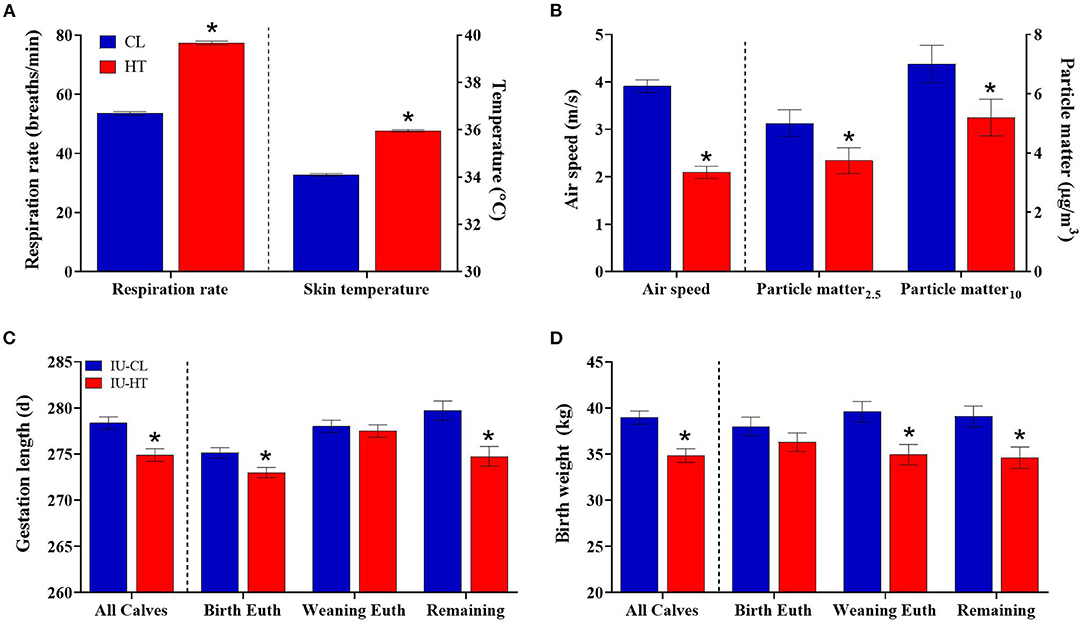
Figure 1. Dam thermoregulatory and birth outcomes upon exposure to late-gestation heat stress (HT, red bars; n = 41) or cooling (CL, fans and soakers, blue bars; n = 41). (A) Respiration rate and skin temperature across the dry period of HT or CL dams, and (B) environmental measures of HT and CL pens including air speed and particle matter. Gestation length (C) and birth weight (D) of heifers exposed to late gestation in utero HT (IU-HT, red bars, n = 36) or CL (IU-CL, blue bars, n = 37). The graphs depict all heifers per treatment plus subgroups of heifers euthanized at birth (n = 8 per in utero treatment), euthanized at weaning (n = 8 per in utero treatment), and the remaining calves (n = 20, IU-HT; n = 21, IUCL). Data is presented as LSM ± SE of the main effect of in utero treatment. *Indicates significance (P ≤ 0.05).
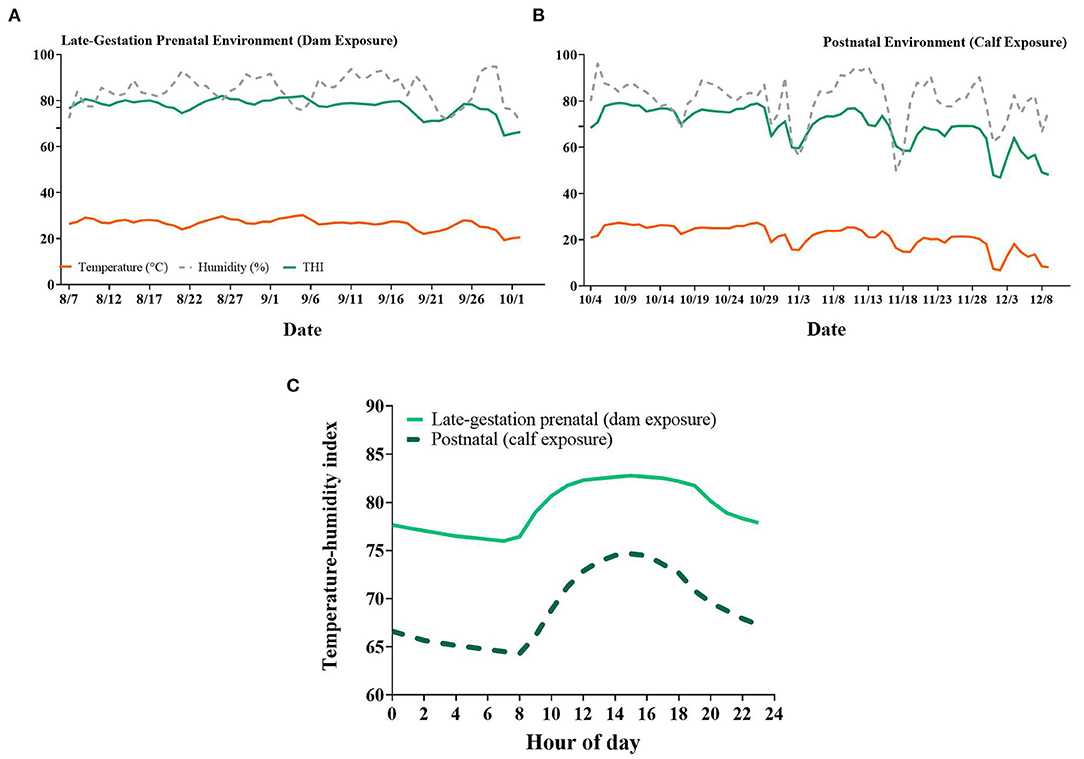
Figure 2. Daily average ambient temperature, relative humidity, and temperature-humidity index (THI) of the surrounding environment during the (A) late-gestation prenatal (dam exposure) and (B) postnatal (calf exposure) periods of the present study. (C) Hourly average THI for prenatal and postnatal exposures. Data are presented as the average of all hours across one day (A,B) or the average of all days for each hour (C). Additional ticks denote thresholds for cows set at 68 and 77 [(A); Zimbelman et al., 2009; Ouellet et al., 2021] and for calves at 69 [(B); Dado-Senn et al., 2020c].
Birth Outcomes
There was a difference in gestation length whereby IU-HT heifers were born approximately 3 d earlier than IU-CL heifers (274.97 vs. 278.35 ± 0.68 d, P < 0.001, Figure 1C), and IU-HT heifers weighed 4 kg less at birth relative to IU-CL heifers (34.84 vs. 38.95 ± 0.72 kg, P < 0.001, Figure 1D). Of the subgroups analyzed, IU-HT heifers selected for birth euthanasia were born earlier than their IU-CL counterparts (P < 0.02, Figure 1C), but there was no significant difference in birth weight (P = 0.25, Figure 1D). The heifers selected for weaning euthanasia had no difference between treatments for gestation length (P = 0.90, Figure 1C), but the IU-HT heifers weighed ~4.5 kg less at birth than weaning-euthanasia IU-CL heifers (P = 0.01, Figure 1D). Finally, of the remaining calves not selected for euthanasia, IU-HT heifers had a shorter gestation length and were born lighter than their IU-CL counterparts (P ≤ 0.005, Figures 1C,D). There was no difference between treatments for calving ease (P = 0.72), and calving ease was scored at 1 for 74% of calving events (data not shown).
Birth Organ Weights
Despite the lack of birth weight difference between IU-CL and IU-HT birth-euthanized heifers, heifers born to HT dams had smaller trimmed MG on a per kg BW basis compared with heifers born to CL dams (P = 0.02, Table 1). In utero HT heifers tended to have reduced thymus and thyroid gland gross organ weights (P ≤ 0.07, Table 1) and had reduced spleen and heart gross organ weights at birth (P < 0.02, Table 1) relative to IU-CL heifers, but these effects were negated when assessed on a per kg BW basis. There were no other differences in organ, blood, or carcass weights at birth (P > 0.13, Table 1).
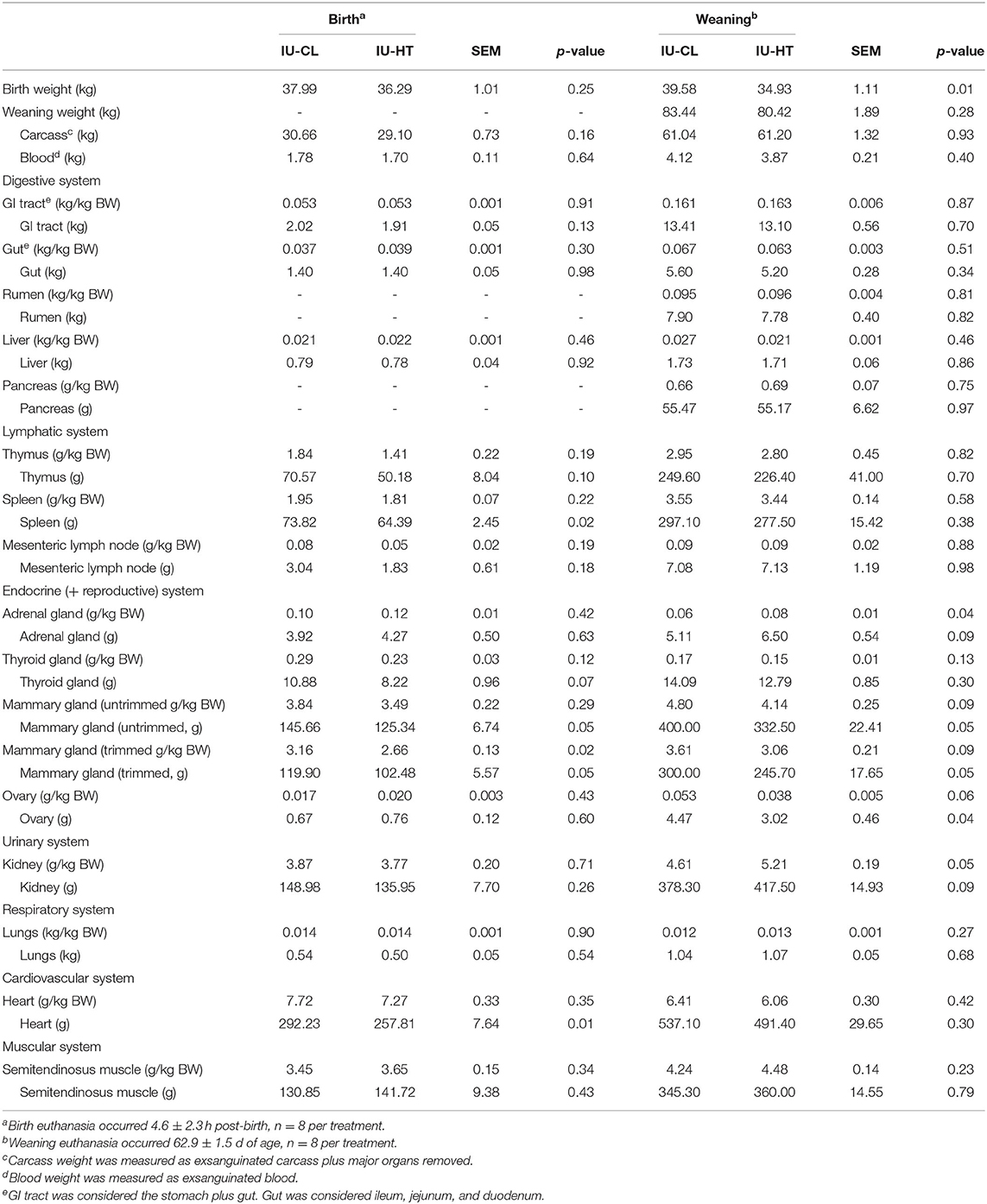
Table 1. Organ weights of heifers exposed to late gestation in utero heat stress (IU-HT) or cooling (IU-CL), assessed at either birth or after weaning.
Calf Environment
The postnatal environment to which growing heifers were exposed encompassed the months of October to December and was more temperate compared to the prenatal environment that the pregnant dams experienced (Figures 2B,C). The environmental THI remained above a heat stress threshold of 69 throughout most of October and the first half of November, then dropped below 69 for the rest of November and December (Figure 2B; Dado-Senn et al., 2020c). The average hourly THI during the postnatal period ranged from 64 to 75 (Figure 2C).
Calf Growth and Intake
There was an in utero treatment effect for BW, whereby IU-HT heifers weighed less than the IU-CL counterparts from 0 to 63 d of age (P < 0.001, Figures 3A,B). There was a day effect (P < 0.001) but the treatment × day interaction was not significant (P = 0.37). Further, IU-HT heifers had a reduced ADG relative to IU-CL heifers during this time-period (0.71 vs. 0.76 ± 0.05 kg/d, P = 0.04, Figure 3C). The pattern of lower post-weaning BW and ADG in IU-HT heifers was similar for the remaining calves (non-euthanized; P ≤ 0.007, Figures 3B,C), but the weaning-euthanasia heifers had similar BW and ADG at 63 d of age (Figures 3B,C).
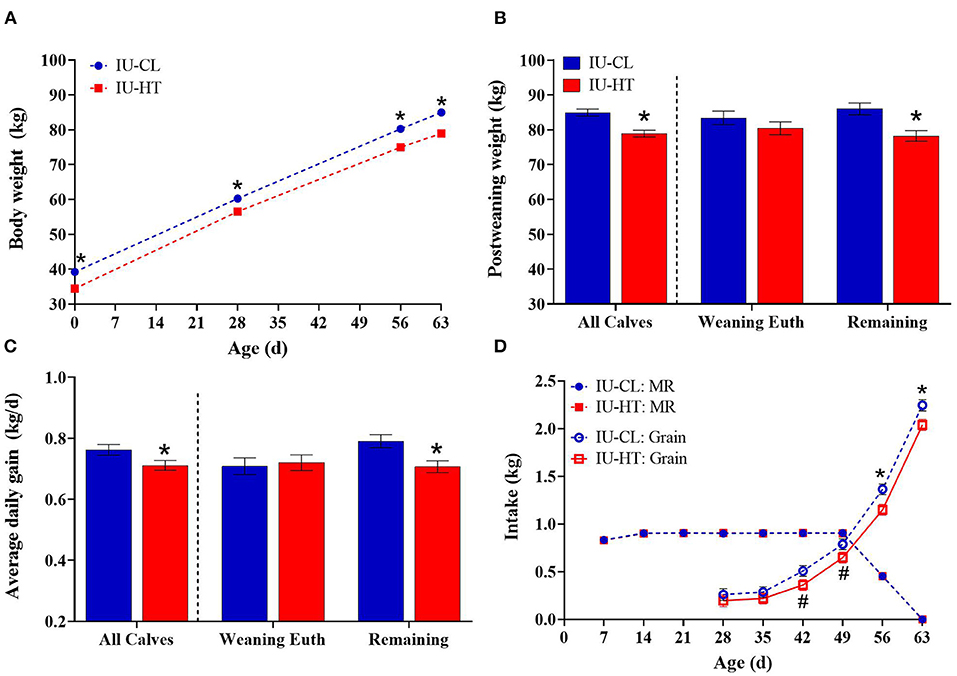
Figure 3. Growth parameters and feed intake of heifers exposed to late-gestation in utero heat stress (IU-HT, red bars/symbols, n = 36) or cooling (IU-CL, blue bars/symbols, n = 37). (A) Body weights (BW) from birth (d 0) to post-weaning (d 63). Post-weaning BW (B) and average daily gain from 0 to 63 d (C) depicted as all heifers per treatment plus subgroups of heifers euthanized at weaning (n = 8 per in utero treatment) and remaining calves (n = 20, IU-HT; n = 21, IUCL). (D) Milk replacer (MR, closed symbols) and starter grain concentrate (open symbols) intake from 1 to 9 weeks of age. Data is presented as LSM ± SE for the main effect of in utero treatment (B,C) or treatment × time (A,D). *Indicates significance (P ≤ 0.05) and #indicates tendency (0.10 ≥ P > 0.05).
Similar to BW over time, there were treatment and day main effects from 0 to 56 d of age for other growth measures. Heifers exposed to IU-HT had reduced hip height, withers height, body length, chest girth, waist girth, and head circumference compared to IU-CL heifers (P ≤ 0.03, Table 2). There was also a treatment × day interaction and tendency for an interaction for chest girth (P = 0.02) and withers height (P = 0.09), as measures were less disparate for these specific parameters at 28 d of age (Table 2).
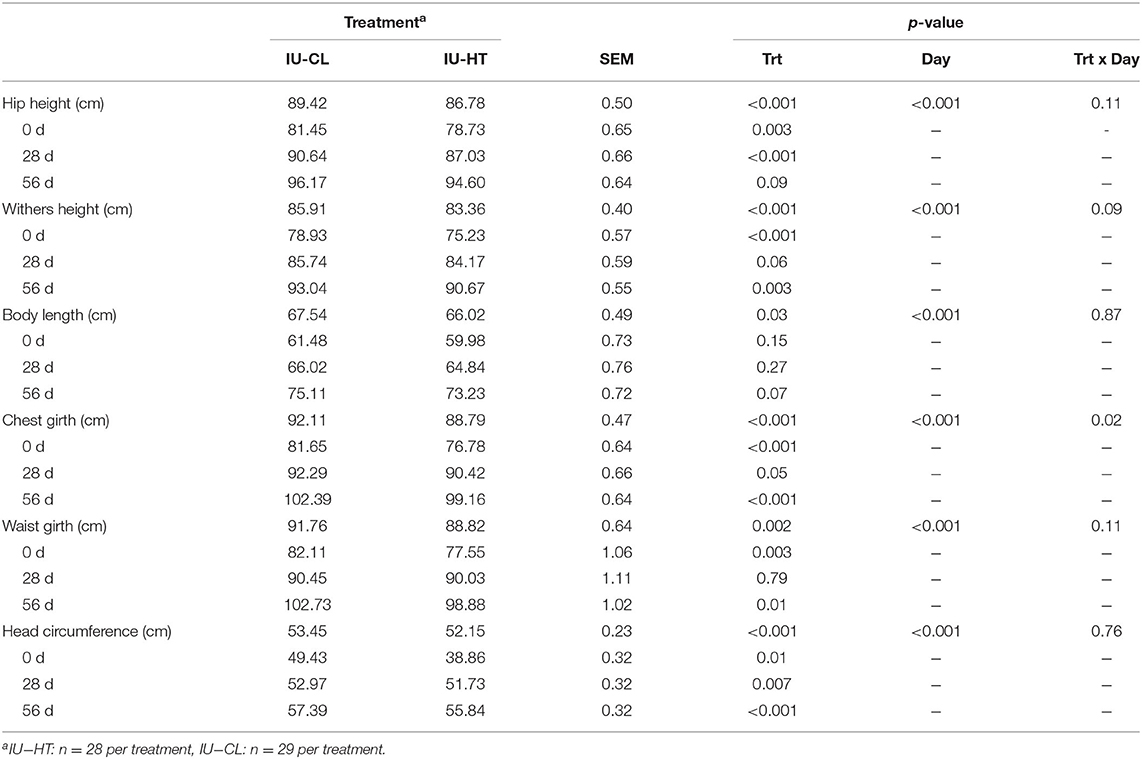
Table 2. Growth and stature parameters of dairy heifers exposed to late gestation in utero heat stress (IU-HT) or cooling (IU-CL) measured at 0, 28, and 56 d of age.
Pre-weaning calf MR intake did not differ between treatments and remained near allotted levels of 0.87 kg DM MR/d until weaning (P = 0.60, Figure 3D). However, IU-HT heifers consumed less starter grain concentrate relative to IU-CL heifers, particularly from 42 to 63 d of age (P = 0.02, Figure 3D). On a component basis, IU-HT heifers had reduced grain and total dry matter (DM), metabolizable energy (ME), crude protein (CP), and crude fat intake (Table 3). There was a main effect of week for both MR and grain intake (P < 0.001), but no treatment × week interaction (P > 0.62).
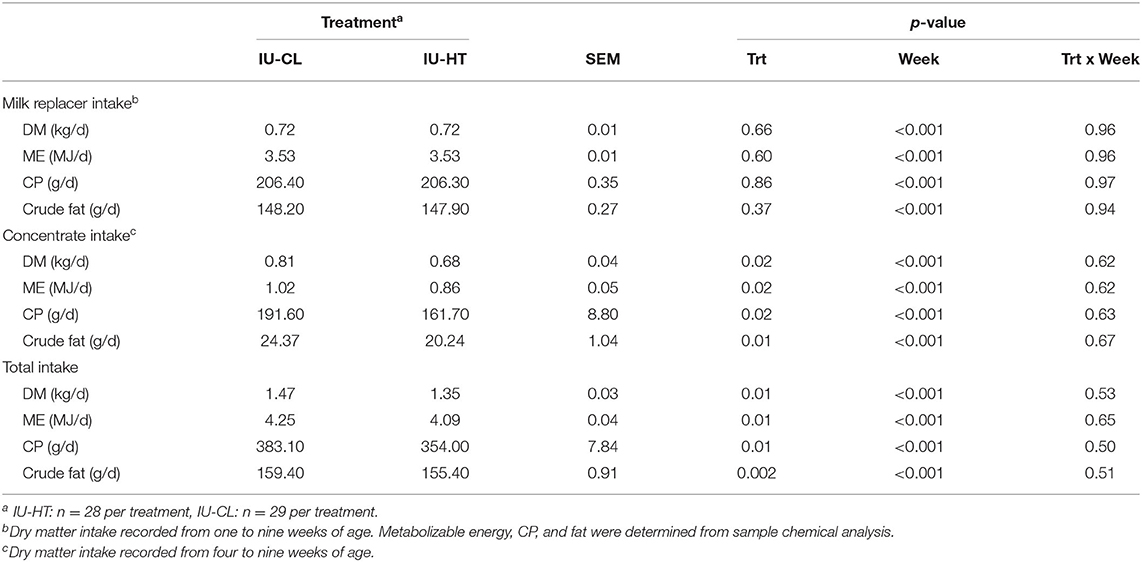
Table 3. Milk replacer, concentrate, and total feed intake of dairy heifers exposed to late gestation in utero heat stress (IU-HT) or cooling (IU-CL) recorded daily and averaged at weekly.
Calf Colostrum IgG, Blood Parameters, and Health Events
There was no difference between treatments in colostrum TP or IgG concentration nor calf 24-h serum IgG concentration (P ≥ 0.32, Figures 4A,B). However, IU-HT heifers had significantly reduced % AEA of IgG at 24-h after colostrum consumption relative to IU-CL counterparts (23.49 vs. 33.26 ± 2.16%, P = 0.004, Figure 4B). Further, heifers exposed to IU-HT had reduced hematocrit percentages (P < 0.001) but no difference in serum TP compared with IU-CL heifers across the pre-weaning period (P = 0.90, Figure 4C). The odds of specific health event occurring was not different between IU-HT and IU-CL calves for fever, bloat, diarrhea, respiratory disease, navel infections, and localized infections (P > 0.18). However, the odds of any health event occurring was greater for IU-HT heifers relative to IU-CL heifers (odds ratio: 3.501, 95% confidence interval: 1.28–9.57, P = 0.01).
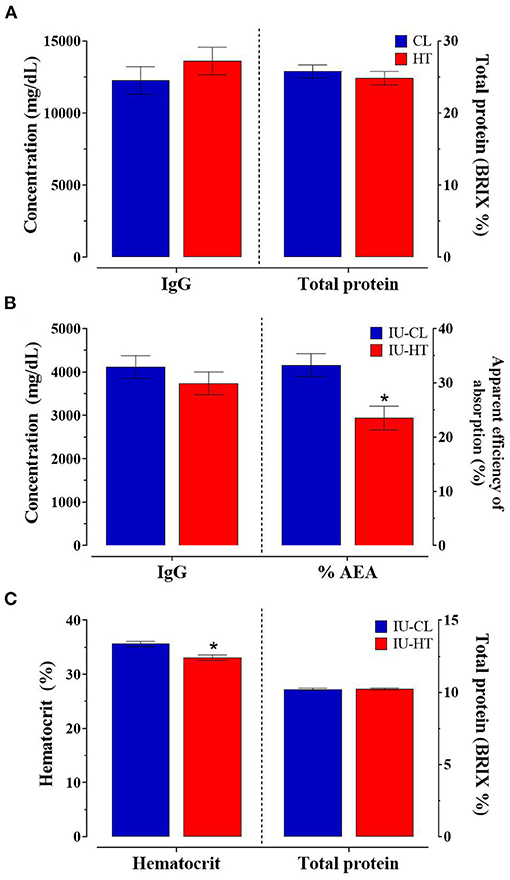
Figure 4. Colostrum and blood parameters of a subset of heifers exposed to late-gestation in utero heat stress (IU-HT, red bars, n = 15) or cooling (IU-CL, blue bars, n = 15). (A) Colostrum total IgG and total protein (TP) concentration (0.8 h after birth) and (B) serum IgG concentration and apparent efficiency of absorption (% AEA) 24-h after colostrum consumption. (C) Whole blood hematocrit and serum TP of heifers from 0 to 63 d of age, collected between 13 and 15 h. Data is presented as LSM ± SE for the main effect of in utero treatment. *Indicates significance (P ≤ 0.05).
Weaning Organ Weights
Regardless of lack of gross BW difference for the subset of heifers euthanized at weaning, there were alterations in specific organ weights relative to BW. Heifers gestated under IU-HT during late gestation had larger adrenal glands and kidneys at 63 d of age relative to IU-CL heifers when adjusted for BW (P ≤ 0.05, Table 1). Additionally, IU-HT heifers tended to have reduced ovary weights compared to IU-CL counterparts after weaning on a per kg BW basis (P = 0.06, Table 1). Finally, consistent with birth outcomes, IU-HT heifers had smaller trimmed MG after weaning (P = 0.05) and tended to have smaller untrimmed and trimmed MG when adjusted for BW (P = 0.09, Table 1).
Discussion
Dairy calves exposed to in utero heat stress are subject to altered growth, immunity, and productivity from birth through lactation with negative consequences on welfare and economy (Tao et al., 2019; Dado-Senn et al., 2020a; Laporta et al., 2020). However, less is known about the impact of prenatal thermal environment on dairy calf fetal organ growth, development, and function postnatally as the animal grows. Herein, we investigated the effect of in utero environmental perturbations on heifer body and organ growth that may contribute to an impaired developmental trajectory in early life. We confirm and further establish that in utero heat-stressed heifers are smaller in weight, stature, and other body size measures from birth to weaning, and we demonstrate that impaired development may partially be attributed to reduced feed intake and alterations in immune, metabolic, and endocrine organ development.
Assessment of dam thermoregulation and the surrounding environment confirmed that the dam, and consequently the in utero calf, were under heat stress. Dams without access to fans and soakers during late gestation had elevated ST and RR relative to actively cooled cows, and the RR was above the heat stress benchmark of 61 bpm for dry cows (Toledo et al., 2020). Further, THI was consistently higher than 68 (Zimbelman et al., 2009) across virtually the entire late gestation and higher than 77 (Ouellet et al., 2021) for 75% of the late gestation. Airspeed in the CL environment was higher than the HT environment, and the mean air speed met or exceeded recommendations necessary to promote heat abatement in lactating dairy cows (Berman, 2005). Notably, this elevated airspeed likely contributed to the increased particle matter in the CL pen relative to the HT pen, although within safe levels as determined by the U.S. EPA National Ambient Air Quality Standards (U. S. Environmental Protection Agency, 2020).
Heifers born to heat-stressed dams weighed ~4 kg less at birth with a 3 d shorter gestation length, consistent with previous studies of late-gestation heat stress and literature review averages (Monteiro et al., 2014; Laporta et al., 2017; Dado-Senn et al., 2020a). Further, IU-HT heifers had a reduced pre-weaning BW, weighed approximately 6 kg less one week post-weaning, and had a 7% reduction in ADG relative to IU-CL heifers. This weight disparity was paired with a reduction in overall size (i.e., height, girth, body length, and head circumference). Whereas previous studies also reported lower weaning weights and ADG in prenatally heat-stressed calves, only one (Almoosavi et al., 2020) reported a difference in calf stature (Monteiro et al., 2016a; Almoosavi et al., 2020; Dado-Senn et al., 2020b). This discrepancy may be due to differences in sample size or the exclusion of bull calves in the present study. While rapid body growth in post-weaned heifers impairs future milk potential (Zanton and Heinrichs, 2005), increased pre-weaning ADG and stature have been associated with decreased age at puberty, conception, and calving and greater milk yield upon lactation (Moallem et al., 2010; Davis Rincker et al., 2011; Soberon et al., 2012). Improved stature (i.e., skeletal growth) may be particularly relevant, as accelerated body growth without disproportionate adiposity leads to enhanced skeletal growth and maximized milk production (Capuco et al., 2003). Balanced growth is achieved through sufficient energy and protein intake (Capuco et al., 2003; Akins, 2016), thus the reduction in ME and CP intake in IU-HT heifers may have contributed to both reduced body weight and stature. Further work will target pre- and post-pubertal stages to assess growth and body composition from heifers gestated under hyperthermic conditions.
The subsets of heifers euthanized at birth and weaning did not have significant BW differences at time of euthanasia, which might be attributed to the smaller sub-sample size or tighter relative calving window selected. Regardless, variations in organ weights were evident at birth and weaning. Most organ weights at birth differed only as gross measures, and thus results must be interpreted with caution. Calves born to heat-stressed dams had smaller gross immune organs (thymus and spleen), thyroid glands, and hearts at birth relative to those born to cooled dams, similar to in utero heat-stressed bulls euthanized between 0 and 2 d of age (Ahmed et al., 2021). These differences, along with physiological outcomes such as reduced apparent efficiency of IgG absorption and increased odds of a health event, could contribute to altered metabolic profiles and diminished immune system development of IU-HT heifers, as reported in similar studies (Tao et al., 2012a; Monteiro et al., 2014; Guo et al., 2016). Notably, there was not enough power in the present study sample size (n = 28–29 per treatment) to detect differences in specific health events herein. Colostrum IgG sampling was also slightly under power, which could have influenced the non-significant findings.
Most organ weight differences observed at birth did not persist to weaning, but alternative organ weight differences, relative to BW, emerged. For example, heifers exposed to in utero heat stress had larger adrenal glands and kidneys and smaller ovaries at weaning relative to calves that were cooled in utero. The mammary gland was the only organ that was consistently lighter at both birth and weaning in IU-HT heifers.
The increase in adrenal gland weight in IU-HT heifers might be related to chronic stress adaptation. As the primary source of stress hormones, adrenal glands play a key role in response and adaptation to physiological challenges, including chronic hyperthermia (Berger et al., 2019). Direct prolonged heat stress exposure in rodents increased adrenal weight, likely due to adaptation of the glands to a continuous challenge (Wang et al., 2015). The impact of prenatal stressors on adrenal gland weights in rodent and cattle models are contradictory with studies reporting increased adrenal size or no difference at birth after in utero stress exposure (Lay et al., 1997; Kapoor and Matthews, 2008; Ashworth et al., 2016). Notably, those studies neither assessed hyperthermic stress nor weights at a later stage of development (i.e., after weaning). Increases in adrenal gland weight can cause maladaptation or hyperactivation of the gland (Berger et al., 2019), thus in utero heat-stress could lead to impaired future stress responses or metabolic disorders in heifers.
The reduced ovary size of IU-HT heifers may pose implications in future reproductive performance. Extensive literature has established that environmental thermal stress negatively influences dairy cattle reproduction. Physiological alterations under heat stress include compromised growth dynamics of the ovarian follicles, reduced circulating concentrations of estradiol and luteinizing hormone, damaged oocyte development, and impaired embryonic survival (Hansen and Aréchiga, 1999; Roth, 2020). Further consequences can arise due to prenatal stressors, as dairy cattle conceived in summer months or born to malnourished dams have smaller pre-pubertal follicles, decreased ovulation rates, reduced follicle numbers, and an increased number of days to breeding and conception (Dupont et al., 2012; Pinedo and De Vries, 2017). It is possible that the smaller body weights combined with the reduced ovarian size of IU-HT heifers at weaning could lead to negative outcomes in follicular development and steroidogenesis.
Heifers exposed to late gestation heat stress had smaller mammary glands at birth and weaning, relative to IU-CL heifers. Importantly, these reduced mammary weights of IU-HT heifers were captured at birth and days after weaning, corresponding to the isometric growth phase of the mammary gland (i.e., same rate as the body). Feeding pre-weaned calves at a lower plane of nutrition negatively impacts body and mammary gland weight at weaning (Daniels et al., 2009; Geiger et al., 2016b). Further exploration of the mammary gland parenchyma (i.e., future milk synthesizing tissue) in those studies found decreased total protein and DNA content, reduced cellular proliferation and impaired response to mammogenic stimuli in the restricted-fed calves, together indicative of diminished future synthetic capacity (Soberon et al., 2012; Geiger et al., 2016a, 2017). Emerging data from our group demonstrates that dairy cattle born to heat-stressed dams produce less milk across multiple lactations, partially attributed to alterations in the lactating gland microstructure and epigenome (Skibiel et al., 2018a,b; Laporta et al., 2020). The reduced mammary gland size of IU-HT heifers in the present study is the first evidence to suggest that the milk synthesis impairment documented in mature in utero heat-stressed cows could be prompted by perturbations that begin at or even before birth.
In the present study, it is difficult to ascertain whether in utero heat stress directly caused alterations in organ development, growth, and feed intake, as additional factors such as the shortened gestation length or smaller birth weights could have also contributed. However, these external factors are also implicated and might result from late gestation heat stress (Tao et al., 2019; Ouellet et al., 2020). Additionally, alterations in maternal feed intake are reported to decrease slightly under late-gestation heat stress, on average by 13% (Ouellet et al., 2020), which could influence offspring growth outcomes. Yet, when accounting for the confounding effect of dissimilar feed intakes, pair-fed studies assessing late-gestation heat stress in both rodents and dairy cattle still identify reductions in offspring size (i.e., birth weight and stature) and passive immune transfer relative to both thermoneutral pair-fed and ad libitum fed counterparts (Almoosavi et al., 2020; Xiao et al., 2020).
An additional limitation to the present study is lack of control over dam early-life experiences, as this study was conducted on a commercial farm that raises youngstock at a different facility. We have previously reported that late-gestation in utero heat stress has multigenerational consequences (Laporta et al., 2020), thus potential dissimilarities in dams' gestation or early life could have influenced the offspring outcomes. Additionally, despite efforts taken to provide a homogenous, thermoneutral postnatal environment, it is possible that exposure to heat stress after birth could have occurred and could have influenced calf physiology and feeding behaviors (Dado-Senn et al., 2020c). However, the combination of fan provision for the calves in this study as well as a lower mean daily THI relative to Dado-Senn et al. (2020b,c) should have minimized any effect of postnatal hyperthermia.
Conclusion
Heifers that experienced heat-stress in utero during late gestation were smaller in weight, stature and size with reduced concentrate intake relative to in utero cooled heifers. This growth retardation was not transient, remaining at least through 63 d of age. Prenatal heat stress led to subtle differences in immune and metabolic organs, while stress-related and reproductive organs were altered in size after weaning. Heifers exposed to in utero heat stress had reduced mammary gland weight across the isometric growth phase. Together, these data suggest that intrauterine hyperthermia experienced during late gestation impairs growth and development at the whole-body and organ level with potential repercussions on developmental trajectory and future functionality. Future investigation will characterize molecular aspects of metabolic, immune, and endocrine organs to determine contribution toward documented impairments in heifer calf growth, immunity, and lactation.
Data Availability Statement
The raw data supporting the conclusions of this article will be made available by the authors, without undue reservation.
Ethics Statement
The animal study was reviewed and approved by University of Florida Institutional Animal Care and Use Committee (protocol #201910599). Written informed consent was obtained from the owners for the participation of their animals in this study.
Author Contributions
JL and GD: conceptualization, supervision, project administration, and funding acquisition. JL, GD, and FM: resources. BD-S, BD, and VO: methodology. BD-S, SF, BD, LC, MM, VO, FC, MS, and CR: investigation. BD-S, VO, and JL: formal analysis. BD-S and JL: writing—original draft preparation. SF, BD, LC, MM, VO, FC, MS, CR, FM, GD: writing—review and editing. All authors have read and agreed to the published version.
Funding
This study was funded by USDA-NIFA AFRI Foundational Program Award 2019-67015-29445 and University of Wisconsin Foundation MSN1882.
Conflict of Interest
The authors declare that the research was conducted in the absence of any commercial or financial relationships that could be construed as a potential conflict of interest.
Publisher's Note
All claims expressed in this article are solely those of the authors and do not necessarily represent those of their affiliated organizations, or those of the publisher, the editors and the reviewers. Any product that may be evaluated in this article, or claim that may be made by its manufacturer, is not guaranteed or endorsed by the publisher.
Acknowledgments
We thank the commercial farm staff and managers and UF Animal Sciences staff for their assistance in cow and calf care, respectively. Further acknowledgment goes to the numerous research interns and volunteers who assisted with data collection.
Supplementary Material
The Supplementary Material for this article can be found online at: https://www.frontiersin.org/articles/10.3389/fanim.2021.750390/full#supplementary-material
References
Ahmed, B. M. S., Younas, U., Asar, T. O., Monteiro, A. P. A., Hayen, M. J., Tao, S., et al. (2021). Maternal heat stress reduces body and organ growth in calves: relationship to immune status. JDS Commun. 2, 295–299. doi: 10.3168/jdsc.2021-0098
Akins, M. S. (2016). Dairy heifer development and nutrition management. Vet. Clin. North Am. Food Anim. Pract. 32, 303–317. doi: 10.1016/j.cvfa.2016.01.004
Almoosavi, S. M. M. S., Ghoorchi, T., Naserian, A. A., Ramezanpor, S. S., and Ghaffari, M. H. (2020). Long-term impacts of late-gestation maternal heat stress on growth performance, blood hormones and metabolites of newborn calves independent of maternal reduced feed intake. Domest. Anim. Endocrinol. 72:106433. doi: 10.1016/j.domaniend.2019.106433
Ashworth, C. J., George, S. O., Hogg, C. O., Lai, Y. T., and Brunton, P. J. (2016). Sex-specific prenatal stress effects on the rat reproductive axis and adrenal gland structure. Reproduction 151, 709–717. doi: 10.1530/REP-16-0097
Barker, D. J. (1998). In utero programming of chronic disease. Clin. Sci. 95, 115–128. doi: 10.1042/CS19980019
Becker, C. A., Collier, R. J., and Stone, A. E. (2020). Invited review: physiological and behavioral effects of heat stress in dairy cows. J. Dairy Sci. 103, 6751–6770. doi: 10.3168/jds.2019-17929
Berger, I., Werdermann, M., Bornstein, S. R., and Steenblock, C. (2019). The adrenal gland in stress – adaptation on a cellular level. J. Steroid Biochem. Mol. Biol. 190, 198–206. doi: 10.1016/j.jsbmb.2019.04.006
Berman, A. (2005). Estimates of heat stress relief needs for Holstein dairy cows. J. Anim. Sci. 83, 1377–1384. doi: 10.2527/2005.8361377x
Capuco, A. V., Erdman, R., Dahl, G., Meyer, M., and Vanamburgh, M. (2003). “Heifer nutrition - prepubertal growth and development,” in Proceedings of the Maryland Nutrition Conference (College Park, MD).
Dado-Senn, B., Laporta, J., and Dahl, G. E. (2020a). Carry over effects of late-gestational heat stress on dairy cattle progeny. Theriogenology 154, 17–23. doi: 10.1016/j.theriogenology.2020.05.012
Dado-Senn, B., Ouellet, V., Dahl, G. E., and Laporta, J. (2020b). Methods for assessing heat stress in pre-weaned dairy calves exposed to chronic heat stress or continous cooling. J. Dairy Sci. 103, 8587–8600. doi: 10.3168/jds.2020-18381
Dado-Senn, B., Vega Acosta, L., Torres Rivera, M., Field, S. L., Marrero, M. G., Davidson, B. D., et al. (2020c). Pre- and postnatal heat stress abatement affects dairy calf thermoregulation and performance. J. Dairy Sci. 103, 4822–4837. doi: 10.3168/jds.2019-17926
Daniels, K. M., Capuco, A. V., McGilliard, M. L., James, R. E., and Akers, R. M. (2009). Effects of milk replacer formulation on measures of mammary growth and composition in Holstein heifers. J. Dairy Sci. 92, 5937–5950. doi: 10.3168/jds.2008-1959
Davis Rincker, L. E., Vandehaar, M. J., Wolf, C. A., Liesman, J. S., Chapin, L. T., and Weber Nielsen, M. S. (2011). Effect of intensified feeding of heifer calves on growth, pubertal age, calving age, milk yield, and economics. J. Dairy Sci. 94, 3554–3567. doi: 10.3168/jds.2010-3923
Dikmen, S., and Hansen, P. J. (2009). Is the temperature-humidity index the best indicator of heat stress in lactating dairy cows in a subtropical environment? J. Dairy Sci. 92, 109–116. doi: 10.3168/jds.2008-1370
Dupont, C., Cordier, A. G., Junien, C., Mandon-Pépin, B., Levy, R., and Chavatte-Palmer, P. (2012). Maternal environment and the reproductive function of the offspring. Theriogenology 78, 1405–1414. doi: 10.1016/j.theriogenology.2012.06.016
Geiger, A. J., Parsons, C. L. M., and Akers, R. M. (2016a). Feeding a higher plane of nutrition and providing exogenous estrogen increases mammary gland development in Holstein heifer calves. J. Dairy Sci. 99, 7642–7653. doi: 10.3168/jds.2016-11283
Geiger, A. J., Parsons, C. L. M., and Akers, R. M. (2017). Feeding an enhanced diet to Holstein heifers during the preweaning period alters steroid receptor expression and increases cellular proliferation. J. Dairy Sci. 100, 8534–8543. doi: 10.3168/jds.2017-12791
Geiger, A. J., Parsons, C. L. M., James, R. E., and Akers, R. M. (2016b). Growth, intake, and health of Holstein heifer calves fed an enhanced preweaning diet with or without postweaning exogenous estrogen. J. Dairy Sci. 99, 3995–4004. doi: 10.3168/jds.2015-10405
Guo, J., Monteiro, A. P. A., Weng, X., Ahmed, B. M., Laporta, J., Hayen, M. J., et al. (2016). Short communication : effect of maternal heat stress in late gestation on blood hormones and metabolites of newborn calves. 6804–6807. doi: 10.3168/jds.2016-11088
Hansen, P. J., and Aréchiga, C. F. (1999). Strategies for managing reproduction in the heat-stressed dairy cow1. J. Anim. Sci. 77, 36–50. doi: 10.2527/1997.77suppl_236x
Jenkins, T. G., Ferrell, C. L., and Cundiff, L. V. (1986). Relationship of components of the body among mature cows as related to size, lactation potential and possible effects on productivity. Anim. Sci. 43, 245–254. doi: 10.1017/S0003356100002427
Kamiya, M., Matsuzaki, M., Orito, H., Kamiya, Y., Nakamura, Y. N., and Tsuneishi, E. (2009). Effects of feeding level of milk replacer on body growth, plasma metabolite and insulin concentrations, and visceral organ growth of suckling calves. Anim. Sci. J. 80, 662–668. doi: 10.1111/j.1740-0929.2009.00690.x
Kapoor, A., and Matthews, S. G. (2008). Prenatal stress modifies behavior and hypothalamic-pituitary-adrenal function in female guinea pig offspring: effects of timing of prenatal stress and stage of reproductive cycle. Endocrinology 149, 6406–6415. doi: 10.1210/en.2008-0347
Laporta, J., Fabris, T. F., Skibiel, A. L., Powell, J. L., Hayen, M. J., Horvath, K., et al. (2017). In utero exposure to heat stress during late gestation has prolonged effects on the activity patterns and growth of dairy calves. J. Dairy Sci. 100, 2976–2984. doi: 10.3168/jds.2016-11993
Laporta, J., Ferreira, F. C., Ouellet, V., Dado-Senn, B., Almeida, A. K., De Vries, A., et al. (2020). Late-gestation heat stress impairs daughter and granddaughter lifetime performance. J. Dairy Sci. 103, 7555–7568. doi: 10.3168/jds.2020-18154
Lay, D. C., Randel, R. D., Friend, T. H., Carroll, J. A., Welsh, T. H., Jenkins, O. C., et al. (1997). Effects of prenatal stress on the fetal calf. Domest. Anim. Endocrinol. 14, 73–80. doi: 10.1016/S0739-7240(96)00115-4
Moallem, U., Werner, D., Lehrer, H., Zachut, M., Livshitz, L., Yakoby, S., et al. (2010). Long-term effects of ad libitum whole milk prior to weaning and prepubertal protein supplementation on skeletal growth rate and first-lactation milk production. J. Dairy Sci. 93, 2639–2650. doi: 10.3168/jds.2009-3007
Monteiro, A. P. A., Guo, J., Weng, X., Ahmed, B. M., Hayen, M. J., Dahl, G. E., et al. (2016a). Effect of maternal heat stress during the dry period on growth and metabolism of calves. J. Dairy Sci. 99, 3896–3907. doi: 10.3168/jds.2015-10699
Monteiro, A. P. A., Tao, S., Thompson, I. M., and Dahl, G. E. (2014). Effect of heat stress during late gestation on immune function and growth performance of calves : isolation of altered colostral and calf factors. J. Dairy Sci. 97, 6426–6439. doi: 10.3168/jds.2013-7891
Monteiro, A. P. A., Tao, S., Thompson, I. M. T., and Dahl, G. E. (2016b). In utero heat stress decreases calf survival and performance through the first lactation. J. Dairy Sci. 99, 8443–8450. doi: 10.3168/jds.2016-11072
NRC (1971). A Guide to Environmental Research on Animals. Washington, DC: National Academy of Sciences.
Ouellet, V., Laporta, J., and Dahl, G. E. (2020). Late gestation heat stress in dairy cows: effects on dam and daughter. Theriogenology 150, 471–479. doi: 10.1016/j.theriogenology.2020.03.011
Ouellet, V., Toledo, I. M., Dado-Senn, B., Dahl, G. E., and Laporta, J. (2021). Critical temperature-humidity index thresholds for dry cows in a subtropical climate. Front. Anim. Sci. 2:706636. doi: 10.3389/fanim.2021.706636
Penzo-Mendez, A. I., and Stanger, B. Z. (2015). Organ-size regulation in mammals. Cold Springs Harb. Perspect. Biol. 7, 1–11. doi: 10.1101/cshperspect.a019240
Pinedo, P. J., and De Vries, A. (2017). Season of conception is associated with future survival, fertility, and milk yield of Holstein cows. J. Dairy Sci. 100, 6631–6639. doi: 10.3168/jds.2017-12662
Piwczyński, D., Nogalski, Z., and Sitkowska, B. (2013). Statistical modeling of calving ease and stillbirths in dairy cattle using the classification tree technique. Livest. Sci. 154, 19–27. doi: 10.1016/j.livsci.2013.02.013
Quigley, J. D., and Drewry, J. J. (1998). Nutrient and immunity transfer from cow to calf pre- and postcalving. J. Dairy Sci. 81, 2779–2790. doi: 10.3168/jds.S0022-0302(98)75836-9
Quigley, J. D., Lago, A., Chapman, C., Erickson, P., and Polo, J. (2013). Evaluation of the brix refractometer to estimate immunoglobulin G concentration in bovine colostrum. J. Dairy Sci. 96, 1148–1155. doi: 10.3168/jds.2012-5823
Reynolds, L. P., Borowicz, P. P., Caton, J. S., Crouse, M. S., Dahlen, C. R., and Ward, A. K. (2019). Developmental programming of fetal growth and development. Vet. Clin. NA Food Anim. Pract. 35, 229–247. doi: 10.1016/j.cvfa.2019.02.006
Reynolds, L. P., Caton, J. S., Redmer, D. A., Grazul-Bilska, A. T., Vonnahme, K. A., Borowicz, P. P., et al. (2006). Evidence for altered placental blood flow and vascularity in compromised pregnancies. J. Physiol. 572, 51–58. doi: 10.1113/jphysiol.2005.104430
Roth, Z. (2020). Reproductive physiology and endocrinology responses of cows exposed to environmental heat stress - experiences from the past and lessons for the present. Theriogenology 155, 150–156. doi: 10.1016/j.theriogenology.2020.05.040
Skibiel, A. L., Dado-Senn, B., Fabris, T. F., Dahl, G. E., and Laporta, J. (2018a). In utero exposure to thermal stress has longterm effects on mammary gland microstructure and function in dairy cattle. PLoS ONE 13, 1–13. doi: 10.1371/journal.pone.0206046
Skibiel, A. L., Peñagaricano, F., Amorín, R., Ahmed, B. M., Dahl, G. E., and Laporta, J. (2018b). In utero heat stress alters the offspring epigenome. Sci. Rep. 8, 1–15. doi: 10.1038/s41598-018-32975-1
Soberon, F., Raffrenato, E., Everett, R. W., and Van Amburgh, M. E. (2012). Preweaning milk replacer intake and effects on long-term productivity of dairy calves. J. Dairy Sci. 95, 783–793. doi: 10.3168/jds.2011-4391
St-Pierre, N. R., Cobanov, B., and Schnitkey, G. (2003). Economic losses from heat stress by US livestock industries. J. Dairy Sci. 86, E52–E77. doi: 10.3168/jds.S0022-0302(03)74040-5
Tao, S., Dahl, G. E., Laporta, J., Bernard, J. K., Orellana Rivas, R. M., and Marins, T. N. (2019). Physiology symposium: effects of heat stress during late gestation on the dam and its calf. J. Anim. Sci. 97, 2245–2257. doi: 10.1093/jas/skz061
Tao, S., Monteiro, A. P. A., Thompson, I. M., Hayen, M. J., and Dahl, G. E. (2012a). Effect of late-gestation maternal heat stress on growth and immune function of dairy calves. J. Dairy Sci. 95, 7128–7136. doi: 10.3168/jds.2012-5697
Tao, S., Thompson, I. M., Monteiro, A. P. A., Hayen, M. J., Young, L. J., and Dahl, G. E. (2012b). Effect of cooling heat-stressed dairy cows during the dry period on insulin response. J. Dairy Sci. 95, 5035–5046. doi: 10.3168/jds.2012-5405
Toledo, I. M., Fabris, T. F., Tao, S., and Dahl, G. E. (2020). When do dry cows get heat stressed? Correlations of rectal temperature, respiration rate, and performance. JDS Commun. 1, 21–24. doi: 10.3168/jdsc.2019-18019
U. S. Environmental Protection Agency (2020). National Ambient Air Quality Standards (NAAQS) for PM. Particulate Matter (PM) Pollution. Available online at: https://www.epa.gov/pm-pollution/national-ambient-air-quality-standards-naaqs-pm (accessed January 4, 2021).
Van Eetvelde, M., and Opsomer, G. (2017). Innovative look at dairy heifer rearing: effect of prenatal and natal environment on later performance. Reprod. Domest. Anim. 52, 30–36. doi: 10.1111/rda.13019
Wang, L., Liu, F., Luo, Y., Zhu, L., and Li, G. (2015). Effect of acute heat stress on adrenocorticotropic hormone, cortisol, interleukin-2, interleukin-12 and apoptosis gene expression in rats. Biomed Rep 3, 425–429. doi: 10.3892/br.2015.445
Xiao, Y., Kronenfeld, J. M., and Renquist, B. J. (2020). Feed intake–dependent and –independent effects of heat stress on lactation and mammary gland development. J. Dairy Sci. 103, 12003–12014. doi: 10.3168/jds.2020-18675
Zanton, G. I., and Heinrichs, A. J. (2005). Meta-analysis to assess effect of prepubertal average daily gain of Holstein heifers on first-lactation production*. J. Dairy Sci. 88, 3860–3867. doi: 10.3168/jds.S0022-0302(05)73071-X
Zimbelman, R. B., Rhoads, R. P. M. L, Rhoads, M. L., Duff, G. C., Baumgard, L. H., et al. (2009). “A re-evaluation of the impact of temperature humidity index (THI) and black globe humidity index (BGHI) on milk production in high producing dairy cows,” in Southwest Nutrition & Management Conference (Tempe, AZ), 158–169. Available at: https://www.researchgate.net/profile/Lance-Baumgard/publication/251735409_A_Re-evaluation_of_the_Impact_of_Temperature_Humidity_Index_THI_and_Black_Globe_Humidity_Index_BGHI_on_Milk_Production_in_High_Producing_Dairy_Cows/links/5877d22608aebf17d3bbc528/A-Re-evaluation-of-the-Impact-of-Temperature-Humidity-Index-THI-and-Black-Globe-Humidity-Index-BGHI-on-Milk-Production-in-High-Producing-Dairy-Cows.pdf (accessed October 29, 2021).
Keywords: prenatal, hyperthermia, intrauterine, environment, productivity, organ, growth
Citation: Dado-Senn B, Field SL, Davidson BD, Casarotto LT, Marrero MG, Ouellet V, Cunha F, Sacher MA, Rice CL, Maunsell FP, Dahl GE and Laporta J (2021) Late-Gestation in utero Heat Stress Limits Dairy Heifer Early-Life Growth and Organ Development. Front. Anim. Sci. 2:750390. doi: 10.3389/fanim.2021.750390
Received: 30 July 2021; Accepted: 25 October 2021;
Published: 17 November 2021.
Edited by:
Edward Narayan, The University of Queensland, AustraliaReviewed by:
Ana Meikle, University of the Republic, UruguayFernanda Carolina Ferreira, University of California, Davis, United States
Copyright © 2021 Dado-Senn, Field, Davidson, Casarotto, Marrero, Ouellet, Cunha, Sacher, Rice, Maunsell, Dahl and Laporta. This is an open-access article distributed under the terms of the Creative Commons Attribution License (CC BY). The use, distribution or reproduction in other forums is permitted, provided the original author(s) and the copyright owner(s) are credited and that the original publication in this journal is cited, in accordance with accepted academic practice. No use, distribution or reproduction is permitted which does not comply with these terms.
*Correspondence: Jimena Laporta, amxhcG9ydGFAd2lzYy5lZHU=