- 1Department of Animal and Range Sciences, Montana State University, Bozeman, MT, United States
- 2North Florida Research and Education Center, University of Florida, Marianna, FL, United States
- 3College of Agricultural Sciences and Natural Resources, Texas A&M University Commerce, Commerce, TX, United States
- 4Department of Animal and Veterinary Sciences, Clemson University, Clemson, SC, United States
- 5Department of Animal Science, Texas A&M University, College Station, TX, United States
- 6Department of Animal and Dairy Science, University of Georgia, Athens, GA, United States
- 7Department of Animal and Dairy Sciences, Mississippi State University, Starkville, MS, United States
- 8Department of Animal Sciences, University of Florida, Gainesville, FL, United States
The objective of this study was to examine the effects of recombinant bovine somatotropin (bST) administration on uterine hemodynamics and subsequent fetal programming in suckled cows during the first trimester of gestation. Crossbred beef cows (n = 152) were stratified by breed, days postpartum, parity, cyclicity status, and body condition score (BCS) before being assigned to either receive injections of bST every other week (BST; 500-mg/14 d) starting at fixed-time artificial insemination (TAI; d 0) until d 97 or to receive no bST (CTL). Blood samples were collected until d 97 for analysis of plasma concentrations of insulin-like growth factor 1 (IGF-1). Pregnancy was assessed via transrectal ultrasonography on d 41 and 173. A subset of pregnant cows (BST, n = 24; CTL, n = 28) were selected for assessment of uterine arterial blood flow (BF), pulsatility index, and resistance index (RI) of the uterine arteries ipsilateral and contralateral to the conceptus via color Doppler ultrasonography on d 97 and 233 of gestation. No differences (P = 0.99) were detected in body weight (BW) or BCS of dams; however, plasma concentrations of IGF-1 were greater (P < 0.001) in BST-treated cows. Color Doppler ultrasonography parameters differed whereby a treatment × day interaction (P = 0.007) was detected for RI on d 97 (P = 0.048); however, on d 233, RI did not differ (P > 0.10) but ipsilateral BF for BST-treated cows was greater (P = 0.0319) than controls. Mean heart girth diameter, crown-to-rump length, and neonate BW at 7 ± 5 d of calf age did not differ (P > 0.10). Liver tissue samples from each calf were collected for analysis of mRNA expression of target insulin-like growth factor system ligands. There was no difference in gene expression of hepatic IGF-1 between treatments (P = 0.99). A treatment × sex interaction was determined, where BST heifers had increased mRNA expression of IGFR1 compared to BST bulls (P = 0.03). Bi-weekly administration of bST until d 97 of pregnancy increased plasma concentrations of IGF-1, altered uterine hemodynamics in dams, induced sex-specific changes in liver gene expression of the offspring but failed to alter calf morphometries or calf performance until weaning.
Introduction
Gestation is a dynamic process that relies on extensive adaptation of the maternal system to partition nutrients and oxygen to the developing conceptus. Livestock production systems typically focus on genetic selection and nutritional management to achieve optimal growth and performance of offspring. However, researchers are now evaluating how hormonal, nutritional, and metabolic environments during gestation may have lasting impacts on livestock offspring based on the concept of fetal, or developmental programming (Godfrey and Robinson, 1998). Alterations to maternal nutrition and circulating hormonal concentrations during gestation can impact fetal growth and cause lifelong modifications of the endocrine and vasoregulative systems (Nüsken et al., 2011).
Nutrient delivery to the growing fetus is dependent upon numerous factors including placental growth and development, uteroplacental blood flow (BF), nutrient availability, placental metabolism, and transport capacity (reviewed by Dunlap et al., 2015). Maternal and fetal nutrient availability is particularly important in times of suboptimal nutrition, as fetuses from dams exposed to nutrient restriction may never achieve their maximal genetic potential postnatally (Gluckman et al., 2013). Efficiency of placental nutrient transport is directly related to uteroplacental BF, which is critical for fetal growth (Ferrell, 1991; Reynolds and Redmer, 1995; Vonnahme, 2012). Fetal demand on the uteroplacenta requires adaptation and the production of growth hormones, such as insulin-like growth factor-I (IGF-1), as well as an increase in placental and fetal BF (Murphy et al., 2006; Gluckman et al., 2007; Nüsken et al., 2011). Insulin-like growth factor-1 is particularly important for both prenatal and postnatal growth (Lawrence et al., 2012), whereas growth hormone (GH), or somatotropin, regulates metabolism, growth and development of tissues and controls IGF-1 levels.
Fetal growth, development, and subsequent performance are critical to livestock producers, but few studies have investigated the impact of the maternal insulin-like growth factor (IGF) system on intrauterine programming in beef cattle. When recombinant bovine somatotropin (bST) was administered to beef heifers during the first third of gestation there was increased plasma concentrations of IGF-1, an increase in fetal fluid, decrease in placentome number and greater umbilical diameter (Sanford et al., 2021). The objective of this experiment was to determine the effects of bST administration during early gestation on uterine hemodynamics in suckled cows and subsequent calf growth. It was hypothesized that the bST-induced increase in maternal IGF-1 from the time of breeding through the first trimester of gestation would benefit conceptus development and subsequent calf performance due to improved uterine hemodynamics.
Materials and Methods
All procedures for the study conducted at the North Florida Research and Education Center in Marianna, FL were reviewed and approved by the University of Florida Institutional Animal Care and Use Committee (number 201709780).
Experimental Design
A total of 152 primiparous (n = 29; 462 ± 42 kg, BCS 5.6 ± 0.9 at DPP 68 ± 14 d) and multiparous (n = 123; 561 ± 85 kg, BCS 5.9 ± 0.3 at DPP 74 ± 17 d) suckled beef cows composed of Angus (n =78), Brangus (n = 56), and SimAngus (n = 18) breeds were enrolled in this experiment. Cow-calf pairs were maintained together according to standard operating procedures of the facility and housed on Pensacola bahiagrass (Paspalum notatum) pasture for the duration of the experiment with ad libitum access to bahiagrass hay, bahiagrass pasture, and water. On d −22 and −10, body condition score (BCS) was assessed, and blood samples were collected via jugular venipuncture to determine estrous cyclicity status of all cows. Body condition score was individually assigned using a scale of 1 to 9 (Whitman, 1975). Cows were then stratified by breed, days postpartum, parity, estrous cyclicity status, and BCS before being randomly assigned to either receive bi-weekly injections of recombinant bST (BST; 500-mg/14 d; Posilac; Elanco Animal Health, Greenville, IN) starting at fixed-time artificial insemination (TAI; d 0) through d 97, or to receive no bST treatment as untreated controls (CTL). On d −10, cows were exposed to the 7-d CO-Synch + controlled internal progesterone releasing (CIDR) estrus synchronization protocol. In brief, all cows received a 100-μg injection of GnRH (2-mL Factrel; Zoetis Animal Health, Parsippany, NJ) concurrent with the insertion of a CIDR (1.38-g Progesterone [P4]; Zoetis Animal Health), followed by a 25-mg injection of Prostaglandin F2α (5-mL Lutalyse; Zoetis Animal Health) at the time of CIDR removal. Cows received an injection of 100-μg GnRH and TAI 66 ± 2 h after CIDR removal. Following TAI, individual body weight (BW) was recorded and BCS was assessed on d 13, 27, 41, 55, 69, 83, 97, 173, and 233. The administration of bST was performed by subcutaneous injection in the neck every 14 d throughout the first trimester of gestation. Calf BW and sex were recorded at parturition. Moreover, liver samples were collected and calf morphometrics (heart girth [HG] and crown-to-rump length [CRL]) were measured at 7 ± 5 d of age using a measuring tape. At weaning, all calves were weighed and an adjusted 205-d weaning weight (WW) was estimated.
Blood Sampling Analysis
Blood samples from all cows were collected on d −22, −10, 0, 13, 27, 41, 55, 69, 83, and 97 relative to TAI via jugular venipuncture into 10-mL evacuated tubes spray-coated with 143-IU of sodium heparin (BD Diagnostics, Franklin Lakes, NJ) then immediately placed on ice, and later centrifuged at 1,500 × g for 15 min at 4°C. Plasma from each blood sample was aliquoted into polypropylene vials and stored at −20°C until further analysis. Samples collected on d −22 and −10 were used to determine the circulating concentrations of P4 and the results were used to assess cyclicity status prior to estrus synchronization. Cows were considered cyclic when one or both samples had a P4 concentration of ≥ 1 ng/mL. Blood samples were also collected every 14 d from 0 to 97 d to determine the concentrations of IGF-1 in the circulation of all cows. Concentrations of plasma P4 and total IGF-1 were determined with an immunoassay system (Immulite 1000 Version 5.22; Siemens Healthcare Diagnostics, Malvern, PA) as previously reported for bovine samples (Mercadante et al., 2016; Leiva et al., 2017; Fontes et al., 2019).
Ultrasonography Evaluation
Pregnancy status was determined via transrectal ultrasonography (Ibex Pro portable ultrasound; 5.0-MHz linear multi-frequency transducer; E.I. Medical Imaging, Loveland, CO) on d 41 and 173 post TAI. Uteroplacental hemodynamic measurements were determined using color Doppler ultrasonography (MicroMaxx, Sonosite, Inc., Bothell, WA) and a transrectal probe (Linear Endorectal L52x probe, Sonosite, Inc.). Uterine perfusion measurements were obtained on d 97, while uterine artery hemodynamics ipsilateral and contralateral to the conceptus were measured on d 233 (BST, n = 24; CTL, n = 28) of gestation following the techniques described by Brockus et al. (2016). Briefly, the uterine artery was located transrectally by following the abdominal aorta toward the origin of the external iliac artery and moving the probe caudally to locate the internal iliac artery. The left and right uterine arteries were palpated and were identified as a major branch of the iliac arteries. On d 233, the left and right uterine arteries noticeably differed to assure pliability and pulsatility. Hemodynamic examinations took ~30 min per cow. Cardiac cycle waveforms from two independent ultrasound scans were used to calculate systolic velocity (S; cm/s), diastolic velocity (Dv; cm/s), maximal velocity (Vmax; cm/s), S:Dv ratio, pulsatility index (PI), and resistance index (RI) using preset functions on the color Doppler ultrasound device (Tables 1, 2). Mean velocity (MnV) was calculated using the equation: (S – Dv)/PI. Uterine BF was calculated using the following equation: (MnV × vessel area × 60 s). Total uterine artery BF was calculated by addition of BF in both the right and left uterine arteries. Ipsilateral and contralateral uterine artery BF is presented as the vessels on the same and opposite side of the fetus, respectively.
Tissue Collection
Liver tissue samples were harvested from calves at 7 ± 5 d of age. Samples were collected via needle biopsy, following the techniques described by Corah et al. (1994). Immediately following collection, ~100 mg of wet liver tissue was placed into 1 mL of RNA stabilization solution (RNAlater, Ambion Inc., Austin, TX), placed on ice for 6 h and stored at either −20 or −80°C until analysis.
RNA Extraction and Quantitative Reverse Transcription Polymerase Chain Reaction
Approximately 50 mg of liver tissue from each biopsy was homogenized with a mechanical homogenizer in 1 mL of TRIzol (Ambion Inc., Austin, TX) followed by phase separation with 0.2 mL of chloroform. Subsequent RNA was precipitated in isopropanol and pelleted by centrifugation (12,000 × g for 15 min at 4°C). Resultant RNA was washed in ethanol by centrifugation and finally resuspended in molecular grade RNase-free water. Extracted total RNA was quantified using a NanoDrop 2000 spectrophotometer (Thermo Scientific, Waltham, MA). All samples resulted in 260 nm/280 nm ratios between 1.9 and 2.0 and were deemed acceptable for downstream procedures. Using the Verso cDNA Synthesis Kit (Thermo Fisher Scientific, Waltham, MA) complementary DNA was produced by 1 μg of RNA being reverse transcribed and then amplified in duplicate using iTaq Universal SYBR Green Supermix (Bio-Rad Laboratories, Hercules, CA). The cDNA and SYBR based master mix were pipetted in duplicate for each sample into a 96-well plate and used for quantitative real-time reverse transcription polymerase chain reaction (PCR, CFX Connect, Bio-Rad Laboratories). Assays for the genes of interest, insulin-like growth factor 1 (IGF1), insulin-like growth factor 2 (IGF2), insulin-like growth factor 1 receptor (IGFR1), and insulin-like factor binding protein 3 (IGFBP3) were validated for efficiency and specificity prior to PCR (Table 3). The geometric mean of the two reference genes (CYP1 and RPS9) were calculated and relative expression for the established genes of interest were calculated using the 2−ΔCt method relative to the housekeeping genes.
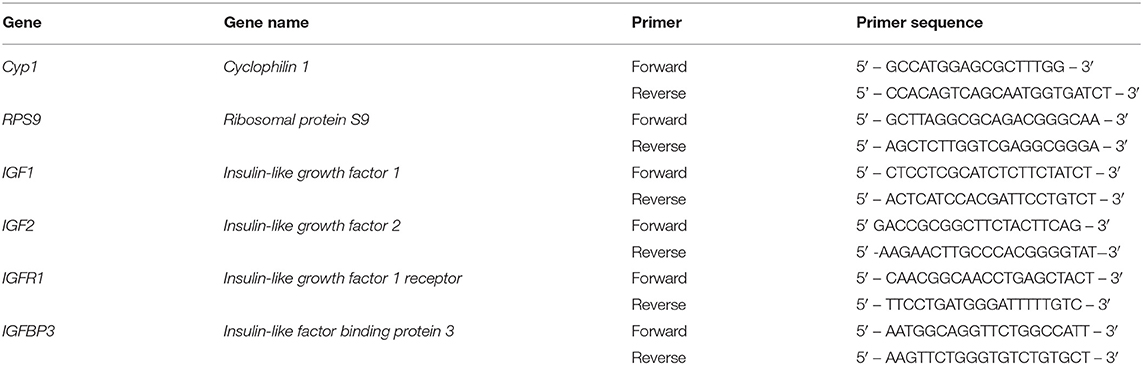
Table 3. Nucleotide sequence of bovine-specific primers used for real-time quantitative reverse transcription PCR to determine the hepatic expression of target genes.
Statistical Analysis
All data were analyzed as a completely randomized block design with either cow or calf considered the experimental unit. The SAS (version 9.4; SAS/STAT, SAS Inst. Inc., Cary, NC) statistical package was used for all statistical analyses. Continuous response variables collected over time (plasma concentrations of IGF-1, BW and BCS) were analyzed as repeated measures using the PROC MIXED procedure. The model included the fixed effects of parity, treatment, day, and their interactions, using first-order autoregressive as the covariance structure. In addition, the fixed effect of treatment, day, and parity were included in the model. Uterine hemodynamics were also analyzed using the PROC MIXED procedure. The model included the fixed effect of treatment and the random effect of calf sex. Gestational length, calf BW and WW, HG, and CRL were analyzed using the PROC MIXED procedure. The model included the fixed effects of parity, treatment, and the interaction between treatment and parity. Relative mRNA expression was analyzed using the PROC MIXED procedure with Kenward–Roger's adjusted degrees of freedom. Statistical significance was declared at P ≤ 0.05, and tendencies discussed when 0.05 < P ≤ 0.10.
Results and Discussion
This study examined the effects of bST administration during the first trimester of gestation on uterine hemodynamics and subsequent calf performance in beef cattle. Growth hormone and the IGF system are components of the somatotropic axis and are essential for controlling growth and reproduction (Lucy et al., 1991; Le Roith et al., 2001). The liver secretes IGF-1 and IGF-2 into circulation in response to GH stimulation (Liu and LeRoith, 1999; Duan et al., 2010). The IGF system is expressed throughout the body, including the reproductive tract (Rhoads et al., 2008) where it regulates preimplantation embryo and placental development (Wathes et al., 1998). The bioavailability of IGF-1 in the bloodstream is regulated by IGF binding proteins (IGFBP) which are expressed in the endometrium prior to implantation and in the placenta (Wathes et al., 1998). During gestation, uterine IGF-1 concentrations decline, and IGF2 is expressed in caruncle and fetal placental mesoderm (Wathes et al., 1998). However, few studies have been conducted to investigate the impact of stimulating the maternal IGF system in gestating beef cows on blood perfusion and intrauterine programming in beef calves.
At TAI (d 0), prior to the initial bST treatment, plasma concentrations of IGF-1 did not differ between treatments (P = 0.936). However, the 500-mg injections of bST administered every 14 d from TAI until d 97 of gestation increased plasma concentrations of IGF-1 in those females receiving recombinant bST (P < 0.001; Figure 1). While past reports have noted a dose response of bST, this data agrees with research conducted in beef cows receiving 325 mg of bST either at TAI, two wk post TAI, or at TAI and two wk post TAI (Mercadante et al., 2016). Furthermore, research in dairy cows receiving 325 mg of bST at TAI and then two wk post TAI reported an increase in plasma concentrations of IGF-1 (Ribeiro et al., 2013). While bST administration concluded on d 97 in this study, it is likely that concentrations of IGF-1 remained elevated for several days after administration. Research in dairy cows reported that IGF-1 peaks on d 7 post-injection and can persist for 31 d when consecutive injections are given bi-weekly (Ribeiro et al., 2013). However, other data indicate that bST mediated IGF-1 stimulation continues for ~14 d in beef and dairy females (Bilby et al., 1999; Cooke et al., 2013; Ribeiro et al., 2013; Mercadante et al., 2016).
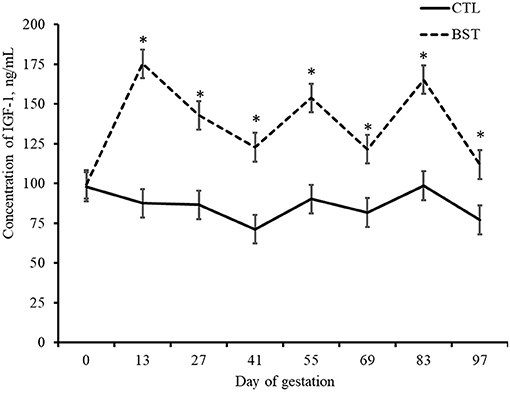
Figure 1. Plasma concentrations of insulin-like growth factor (IGF-1) of beef cows during early gestation. BST: cows received 500-mg of recombinant bovine somatotropin bi-weekly via subcutaneous injection from insemination until d 97 of gestation; CTL: Angus-based crossbred cows received no injections. All cows were exposed to estrus synchronization protocol and fixed-time artificial insemination. *P < 0.001.
Blood flow to the utero-placental unit is critical for optimal fetal growth and development, as well as postnatal performance of the calf. Blood perfusion of the reproductive tract was investigated on d 97 and again on d 233 (Tables 1, 2). On d 97, uterine hemodynamic parameters of BF of the uterine artery ipsilateral to the conceptus (P = 0.809) and diameter of the vessel (P = 0.881) did not differ. However, RI was different (P = 0.048) and there was a tendency for PI (P = 0.079) to be decreased in BST cows, which indicates a drop in blood vessel resistance in the uterine vasculature. Although overall BF on d 97 was not different among treatments, this drop in resistance could highlight early changes in vascularity. Furthermore, there was no difference (P = 0.161) in the angle used to perform the measurement of the blood vessel or heartrate of the cows at the time of examination (P = 0.309). A treatment × d interaction (P = 0.007) was detected for maximal systolic velocity (Vmax), where no differences were observed on d 97 between treatments; however, on d 233, Vmax was greater (P < 0.001; Table 2) for BST-treated cows. Similar to Vmax, ipsilateral blood flow was increased by 30% in BST vs. CTL cows. Therefore, increased plasma concentrations of IGF-1 may have altered uterine hemodynamics. Past reports in cattle have indicated that bST can stimulate embryonic development directly (Moreira et al., 2000) or in vitro through its actions on IGF-1 (Palma et al., 1997). Maternal plasma IGFs play a role in maternal tissue growth and metabolism, resulting in the modulation of nutrient availability for adequate conceptus growth and have been positively correlated with fetal growth in several livestock species (Sferruzzi-Perri et al., 2011). As a result, maternal plasma IGF-1 could potentially affect fetal growth indirectly and is itself altered by both BCS and diet during gestation (Osgerby et al., 2002, 2003a,b).
While bi-weekly bST administration increased plasma concentration of IGF-1 in maternal circulation in the present study, there were no differences detected in gestational length, calf birth weight, mean HG diameter, or CRL length (Table 4; P > 0.10) when assessed at 7 ± 5 d of calf age. In addition, cow BW and BCS were not different (P < 0.10) between treatments. The administration of bST during the periconception period in sheep resulted in improved postnatal offspring performance (Costine et al., 2005; Koch et al., 2010). When calves were assessed at the time of weaning for performance, WW of calves was not affected by treatment (CTL = 214.0 kg; BST = 211.8 kg). This concurs with previous work in beef calves that reported no differences in BW or adjusted WW of calves born to dams that received a single 500-mg injection of bST at the time of AI compared to those not receiving bST (Starbuck et al., 2006). A more recent study involving beef cattle that included 325-mg injections of bST at either TAI, two weeks prior to TAI, or both at TAI and two weeks prior had no effect on calf BW when assessed monthly until 150 days of age (Mercadante et al., 2016).
To evaluate the potential effects of maternal bST administration on the somatotropic axis of offspring, expression of hepatic IGF1, IGF2, IGFR1, and IGFBP3 were analyzed in each calf (Figure 2). There was no difference in relative gene expression of hepatic IGF1 between treatments (P = 0.99) which supports previous findings of no difference in calf liver mRNA expression when dams received 325 mg of bST at TAI and bi-weekly up to 14 d after TAI (Mercadante et al., 2016). There was a treatment × sex interaction, where heifers of BST cows had increased expression of IGFR1 compared to bulls born from BST cows (P = 0.03). However, research has indicated that GH stimulates liver expression of IGF-1 (Kim et al., 2006; Jiang et al., 2007). While the current study did not measure mRNA expression in the maternal system, research has indicated that administration of 500-mg of bST increases serum IGF-1 and hepatic expression of IGF1 in beef cows fed concentrate compared to those fed only hay (Wu et al., 2010).
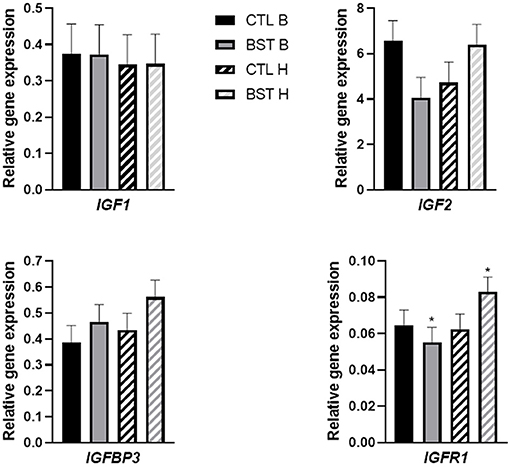
Figure 2. Relative gene expression of hepatic insulin-like growth factor 1 (IGF-1) mRNA of heifer and bull calves that were either exposed to or the absence of the treatment during intrauterine development. CTL B: bull calf born to dam that received no bovine somatotropin (bST) administration, BST B: bull calf born to dam that received a 500-mg injection of bST bi-weekly during early to mid-gestation, CTL H: heifer calf born to dam that received no bovine somatotropin (bST) administration, or BST H: heifer calf born to dam that received a 500-mg injection of bST bi-weekly during early to mid-gestation. *Effect of treatment × gender (P = 0.03) interaction.
In conclusion, beef cows that received bi-weekly injections of 500-mg of bST from the time of breeding through early gestation had increased plasma circulating concentrations of IGF-1, decreased vascular resistance and increased blood flow to the ipsilateral uterine horn. Heifers born to cows treated with bST had increased expression of IGFR1 compared to bull calves born from bST-treated cows. Even though blood flow was altered, bST administration failed to alter subsequent calf performance assessed from birth to the time of weaning. Further investigation is required into the effects of induced IGF signaling on uterine hemodynamics and sex-specific postnatal calf growth performance in beef cattle.
Data Availability Statement
The raw data supporting the conclusions of this article will be made available by the authors, without undue reservation.
Ethics Statement
The animal study was reviewed and approved by University of Florida Institutional Animal Care and Use Committee.
Author Contributions
GL, ND, CS, PF, NO, and LC conceived and designed the experiment. CS, CL, ND, TS, and MO wrote the manuscript, reviewed and edited the manuscript. GL, ND, CL, and JB provided experimental and laboratorial resources. CS, LC, MO, and KM conducted sample collection. CS, LC, and TS performed the lab analysis. ND and NO performed data analysis. ND, CL, and CS interpreted results. All authors have read and approved the final manuscript.
Funding
The authors wish to thank Zoetis Animal Health (Parsippany, NJ) for donating the following products: PGF2α (Lutalyse), GnRH (Factrel), CIDR inserts (EAZI-BREED CIDR), and ABS Global (DeForest, WI) for their donation of frozen semen.
Conflict of Interest
The authors declare that the research was conducted in the absence of any commercial or financial relationships that could be construed as a potential conflict of interest.
Publisher's Note
All claims expressed in this article are solely those of the authors and do not necessarily represent those of their affiliated organizations, or those of the publisher, the editors and the reviewers. Any product that may be evaluated in this article, or claim that may be made by its manufacturer, is not guaranteed or endorsed by the publisher.
Acknowledgments
The authors would like to acknowledge V. Mercadante, J. Rizo, D. Henry, and F. Ciriaco for their assistance with data collection and analysis; and P. Folsom, M. Foran, D. Jones, D. Thomas, and C. Wood for their assistance with data collection.
Abbreviations
BCS, body condition score; BF, blood flow; bST, recombinant bovine somatotropin; BW, body weight; CIDR, controlled internal progesterone releasing insert; CRL, crown-to-rump length; Cyp1, cyclophilin-1; Dv, diastolic velocity; GH, growth hormone; HG, heart girth; IGF, insulin-like growth factor system; IGF-1, insulin-like growth factor 1; IGF1, insulin-like growth factor 1 gene; IGF2, insulin-like growth factor 2 gene; IGFBP, insulin-like growth factor binding proteins; IGFBP3, insulin-like growth factor binding protein 3; IGFR1, insulin-like growth factor 1 receptor; MnV, mean velocity; P4, progesterone; PI, pulsatility index; PCR, real-time reverse transcription polymerase chain reaction; RI, resistance index; RPS9, ribosomal protein S9; S, systolic velocity; TAI, fixed-time artificial insemination; Vmax, maximal systolic velocity; WW, weaning weight.
References
Bilby, C. R., Bader, J. F., Salfen, B. E., Youngquist, R. S., Murphy, C. N., Garverick, H. A., et al. (1999). Plasma GH, IGF-I, and conception rate in cattle treated with low doses of recombinant bovine GH. Theriogenology 51, 1285–1296. doi: 10.1016/S0093-691X(99)00072-2
Brockus, K. E., Hart, C. G., Gilfeather, C. L., Fleming, B. O., and Lemley, C. O. (2016). Dietary melatonin alters uterine artery hemodynamics in pregnant Holstein heifers. Domest. Anim. Endocrinol. 55, 1–10. doi: 10.1016/j.domaniend.2015.10.006
Cooke, R. F., Bohnert, D. W., Francisco, C. L., Marques, R. S., Mueller, C. J., and Keisler, D. H. (2013). Effects of bovine somatotropin administration on growth, physiological, and reproductive responses of replacement beef heifers. J. Anim. Sci. 91, 2894–2901. doi: 10.2527/jas.2012-6082
Corah, L. R., Arthington, J. D., Frank, R., and Pryor, D. (1994). Kansas State University Cooperative Extension Service, and Kansas State University Department of Communications, Elanco Animal Health. Liver Biopsy Procedures for Determining Trace Mineral Status in Beef Cows. Manhattan: Cooperative Extension Service, Kansas State University.
Costine, B. A., Inskeep, E. K., and Wilson, M. E. (2005). Growth hormone at breeding modifies conceptus development and postnatal growth in sheep. J. Anim. Sci. 83, 810–815. doi: 10.2527/2005.834810x
Duan, C., Ren, H., and Gao, S. (2010). Insulin-like growth factors (IGFs), IGF receptors, and IGF-binding proteins: roles in skeletal muscle growth and differentiation. Gen. Comp. Endocrinol. 167, 344–351. doi: 10.1016/j.ygcen.2010.04.009
Dunlap, K. A., Brown, J. D., Keith, A. B., and Satterfield, M. C. (2015). Factors controlling nutrient availability to the developing fetus in ruminants. J. Anim. Sci. Biotechnol. 6:16. doi: 10.1186/s40104-015-0012-5
Ferrell, C. L. (1991). Maternal and fetal influences on uterine and conceptus development in the cow: I. Growth of tissues of the gravid uterus. J. Anim. Sci. 69, 1945–1953. doi: 10.2527/1991.6951945x
Fontes, P. L. P., Cooke, R. F., Oosthuizen, N., Timlin, C. L., Dias, N. W., Currin, J. F., et al. (2019). Impacts of administering prostaglandin F2α analogue 24 h prior to progesterone insert removal on expression of estrus in beef females. Livestock Sci. 226, 82–86. doi: 10.1016/j.livsci.2019.06.007
Gluckman, P. D., Beedle, A. S., Hanson, M. A., and Low, F. M. (2013). Human growth: evolutionary and life history perspectives. Nestle Nutr. Inst. Workshop Ser. 71:89–102. doi: 10.1159/000342572
Gluckman, P. D., Lillycrop, K. A., Vickers, M. H., Pleasants, A. B., Phillips, E. S., Beedle, A. S., et al. (2007). Metabolic plasticity during mammalian development is directionally dependent on early nutritional status. Proc. Natl. Acad. Sci. U. S. A. 104, 12796–12800. doi: 10.1073/pnas.0705667104
Godfrey, K., and Robinson, S. (1998). Maternal nutrition, placental growth and fetal programming. Proc. Nutr. Soc. 57, 105–111. doi: 10.1079/PNS19980016
Jiang, H., Wang, Y., Wu, M., Gu, Z., Frank, S. J., and Torres-Diaz, R. (2007). Growth hormone stimulates hepatic expression of bovine growth hormone receptor messenger ribonucleic acid through signal transducer and activator of transcription 5 activation of a major growth hormone receptor gene promoter. Endocrinology 148, 3307–3315. doi: 10.1210/en.2006-1738
Kim, J. W., Rhoads, R. P., Segoale, N., Kristensen, N. B., Bauman, D. E., and Boisclair, Y. R. (2006). Isolation of the cDNA encoding the acid labile subunit (ALS) of the 150 kDa IGF-binding protein complex in cattle and ALS regulation during the transition from pregnancy to lactation. J. Endocrinol. 189, 583–593. doi: 10.1677/joe.1.06824
Koch, J. M., Wilmoth, T. A., and Wilson, M. E. (2010). Periconceptional growth hormone treatment alters fetal growth and development in lambs. J. Anim. Sci. 88, 1619–1625. doi: 10.2527/jas.2009-2392
Lawrence, T., Fowler, V., and Novakofski, J. (2012). Growth of Farm Animals, 3rd Edn. Oxford: CABI. doi: 10.1079/9781780641461.0000
Le Roith, D., Bondy, C., Yakar, S., Liu, J. L., and Butler, A. (2001). The somatomedin hypothesis: 2001. Endo. Rev. 22, 53–74. doi: 10.1210/edrv.22.1.0419
Leiva, T., Cooke, R. F., Brandao, A. P., Schubach, K. M., Batista, L. F. D., Miranda, M. F., et al. (2017). Supplementing an immunomodulatory feed ingredient to modulate thermoregulation, physiologic, and production responses in lactating dairy cows under heat stress conditions. J. Dairy Sci. 100, 4829–4838. doi: 10.3168/jds.2016-12258
Liu, J. L., and LeRoith, D. (1999). Insulin-like growth factor I is essential for postnatal growth in response to growth hormone. Endocrinology 140, 5178–5184. doi: 10.1210/endo.140.11.7151
Lucy, M., Beck, J., Staples, C., Head, H., De La Sota, R., and Thatcher, W. (1991). Follicular dynamics, plasma metabolites, and growth factors in lactating cows with positive or negative energy balance during the periestrual period. Reprod. Nutr. Devel. 32, 331–341. doi: 10.1051/rnd:19920403
Mercadante, V. R. G., Fontes, P. L. P., Ciriaco, F. M., Henry, D. D., Moriel, P., Ealy, A. D., et al. (2016). Effects of recombinant bovine somatotropin administration at breeding on cow, conceptus, and subsequent offspring performance of beef cattle. J. Anim. Sci. 94, 2128–2138. doi: 10.2527/jas.2015-0217
Moreira, F., Risco, C. A., Pires, M. F., Ambrose, J. D., Drost, M., and Thatcher, W. W. (2000). Use of bovine somatotropin in lactating dairy cows receiving timed artificial insemination. J. Dairy Sci. 83, 1237–1247. doi: 10.3168/jds.S0022-0302(00)74990-3
Murphy, V. E., Smith, R., Giles, W. B., and Clifton, V. L. (2006). Endocrine regulation of human fetal growth: the role of the mother, placenta, and fetus. Endocr. Rev. 27, 141–169. doi: 10.1210/er.2005-0011
Nüsken, K.-D., Schneider, H., Plank, C., Trollmann, R., Nüsken, E., Rascher, W., et al. (2011). Fetal programming of gene expression in growth-restricted rats depends on the cause of low birth weight. Endocrinology 152, 1327–1335. doi: 10.1210/en.2010-1116
Osgerby, J., Wathes, D. C., Howard, D., and Gadd, T. (2002). The effect of maternal undernutrition on ovine fetal growth. J. Endocr. 173, 131–141. doi: 10.1677/joe.0.1730131
Osgerby, J. C., Gadd, T. S., and Wathes, D. C. (2003a). Effect of maternal body condition on placental and fetal growth and the insulin-like growth factor axis in Dorset ewes. Reproduction 125, 717–731. doi: 10.1530/reprod/125.5.717
Osgerby, J. C., Gadd, T. S., and Wathes, D. C. (2003b). The effects of maternal nutrition and body condition on placental and foetal growth in the ewe. Placenta 24, 236–247. doi: 10.1053/plac.2002.0902
Palma, G., Muller, M., and Brem, G. (1997). Effect of insulin-like growth factor-I (IGF-I) at high concentrations on blastocyst development of bovine embryos produced in vitro. J. Reprod. Fertil. 110, 347–353. doi: 10.1530/jrf.0.1100347
Reynolds, L. P., and Redmer, D. A. (1995). uteroplacental vascular development and placental function. J. Anim. Sci. 73, 1839–1851. doi: 10.2527/1995.7361839x
Rhoads, M. L., Meyer, J. P., Kolath, S. J., Lamberson, W. R., and Lucy, M. C. (2008). Growth hormone receptor, insulin-like growth factor (IGF)-1, and IGF-binding protein-2 expression in the reproductive tissues of early postpartum dairy cows. J. Dairy Sci. 91, 1802–1813. doi: 10.3168/jds.2007-0664
Ribeiro, E., Bruno, R., Farias, A., Hernández Rivera, J., Gomes, G., Surjus, R., et al. (2013). Low doses of bovine somatotropin enhance conceptus development and fertility in lactating dairy cows. Biol. Reprod. 90, 1–12. doi: 10.1095/biolreprod.113.114694
Sanford, C. D., Owen, M. P. T., Oosthuizen, N., Fontes, P. L. P., Vonnahme, K. A., Nelson, M., et al. (2021). Effects of administering exogenous bovine somatotropin to beef heifers during the first trimester on conceptus development as well as steroid- and eicosanoid-metabolizing enzymes. J. Anim. Sci. 99, 1–7. doi: 10.1093/jas/skab050
Sferruzzi-Perri, A. N., Owens, J. A., Pringle, K. G., and Roberts, C. T. (2011). The neglected role of insulin-like growth factors in the maternal circulation regulating fetal growth. J. Physiol.-London 589, 7–20. doi: 10.1113/jphysiol.2010.198622
Starbuck, M. J., Inskeep, E. K., and Dailey, R. A. (2006). Effect of a single growth hormone (rbST) treatment at breeding on conception rates and pregnancy retention in dairy and beef cattle. Anim. Reprod. Sci. 93, 349–359. doi: 10.1016/j.anireprosci.2005.08.010
Vonnahme, K. A. (2012). How the maternal environment impacts fetal and placental development: implications for livestock production. Anim. Reprod. 9, 789–797. doi: 10.1071/RD11910
Wathes, D. C., Reynolds, T. S., Robinson, R. S., and Stevenson, K. R. (1998). Role of the insulin-like growth factor system in uterine function and placental development in ruminants. J. Dairy Sci. 81, 1778–1789. doi: 10.3168/jds.S0022-0302(98)75747-9
Whitman, R. W. (1975). Weight Change, Body Condition, and Beef Cow Reproduction. Fort Collins, CO: Colorado State University.
Keywords: beef cow, Doppler ultrasonography, insulin-like growth factor I, recombinant bovine somatotropin, Doppler ultrasound
Citation: Sanford CD, Owen MPT, McCarty K, Canal LB, Schulmeister TM, Oosthuizen N, Fontes PLP, Lemley CO, Bromfield JJ, DiLorenzo N and Lamb GC (2021) Effects of Administering Exogenous Bovine Somatotropin During the First Trimester of Pregnancy Altered Uterine Hemodynamics in Suckled Beef Cows. Front. Anim. Sci. 2:744790. doi: 10.3389/fanim.2021.744790
Received: 20 July 2021; Accepted: 24 September 2021;
Published: 20 October 2021.
Edited by:
Edward Narayan, The University of Queensland, AustraliaReviewed by:
M. Sofia Ortega, University of Missouri, United StatesHatem Eltahan, Agricultural Research Center, Egypt
Copyright © 2021 Sanford, Owen, McCarty, Canal, Schulmeister, Oosthuizen, Fontes, Lemley, Bromfield, DiLorenzo and Lamb. This is an open-access article distributed under the terms of the Creative Commons Attribution License (CC BY). The use, distribution or reproduction in other forums is permitted, provided the original author(s) and the copyright owner(s) are credited and that the original publication in this journal is cited, in accordance with accepted academic practice. No use, distribution or reproduction is permitted which does not comply with these terms.
*Correspondence: G. Cliff Lamb, Z2NsYW1iQHRhbXUuZWR1