- 1Agricultural Research Council (ARC)-Animal Production Institute, Irene, South Africa
- 2Department of Animal, Grassland and Wildlife Sciences, University of the Free State, Bloemfontein, South Africa
Any reduction in the carbon footprint of beef production will contribute to future sustainability. This study investigates the environmental impact (carbon footprint) of the changes that occurred in cow productivity in the cowherds of four South African landrace breeds over a period of 25–30 years. Cow productivity, defined as kilogram calf weaned per large stock unit mated, increased by 18.3, 10.0, 14.2, and 10.4% in the Afrikaner, Bonsmara, Drakensberger, and Nguni, respectively. This resulted in a decrease in the carbon footprint, as defined by the enteric methane emissions factor, of between 6.6 and 12.0%. Changes in the cow productivity component traits, viz. weaning weight, cow weight, and fertility (as measured by inter-calving period) was also investigated. In all breeds, except the Nguni, the change in the environmental trends were less than that of the direct genetic or phenotypic trends. The genetic trends for direct weaning weight in the Afrikaner, Bonsmara, and Drakensberger were +6.7, +11.7, and +6.2 kg, respectively. In the case of the Bonsmara and Drakensberger breeds, the genetic trends were larger than the phenotypic trends, which may indicate that the environment cannot support the higher “genetic” weaning weights. The genetic trends for mature cow weight in Bonsmara and Drakensberger were +15.9 and +15.1 kg, respectively, whereas in the Afrikaner and Nguni it was not significantly different from zero (R2 ≤ 0.24). The trends in the phenotypic mature cow weights were −8.3, +17.5, +8.5, and −17.2 kg for in the Afrikaner, Bonsmara, Drakensberger, and Nguni, respectively. Although the observed inter-calving period of all breeds declined, there was no genetic change in inter-calving period for any of the breeds. It is proposed that selection indices are developed for cow productivity, which can be extended to a carbon footprint selection index. It is recommended that similar studies be done on all the major beef breeds in South Africa, especially those that rely on regular importation of genetic material.
Introduction
One of the responsibilities of the livestock industries is to reduce the carbon (release of greenhouse gases) and water footprints (i.e., the water use), which will contribute to future sustainability. One way to achieve this is through inter alia improved production efficiency. If cow productivity can be improved, it will have a mitigating effect on the production of greenhouse gases (GHG's), as higher cow productivity will probably lead to improved (higher) gross efficiency (Wall et al., 2010; Scholtz et al., 2011). An increase in cow productivity will lead to the generation of less GHG emissions per unit of product (Scholtz et al., 2013a), and in this case kilogram of calf weaned. It should be noted that the cow-calf production cycle is responsible for ~72% of the energy consumed in beef cattle from conception to slaughter (Ferrell and Jenkins, 1982).
A study by Capper (2011) compared the environmental impact of modern (2007) beef production in the USA with production practices of the beef production system in 1977. This study demonstrated that modern beef production in the USA requires considerably less resources than the equivalent production systems in 1977. The study indicated that improving productivity would be important if the environmental impact of beef production is to be reduced. Capper (2011) recommended further investigations into improving productivity, which can be used by the livestock industries to continue to provide sufficient animal protein to satisfy the market while the use of resources is reduced. It is therefore important to quantify the current cow productivity of the landrace beef breeds of South Africa, especially in the era of climate change, where GHG emissions from beef production is under the spotlight.
Over the last 50 years, emphasis was on breeding animals to meet specific, sometimes artificial, breed standards while using genetic information to select animals that conform to specific production norms. Each breed society has its own unique breed standards and therefore selection objectives between different breeds may differ. Furthermore, individual breeders, within breeds, may have their own objectives.
It is easy to achieve genetic change through selection (Garrick and Enns, 2010), which is the result of using animals as parents that depart from the average. However, it is much more difficult to achieve genetic improvement, since it requires that the combined value of all favorable changes exceed the negative effects caused by unfavorable changes. Some beef breeds have shown remarkable genetic change for specific traits, but whether this resulted in genetic improvement in efficiency of production and a lower environmental impact is not clear.
Since, the anticipated climate change will have a negative effect on the livestock production environments, it will become more important to match the genotype with the production environment. In order to ensure sustainable production, the selection of animals and genotypes that are better adapted to the anticipated environment, should be pursued (Scholtz et al., 2013b). In South Africa, the indigenous Sanga and Sanga derived cattle breeds can form the basis for increased productivity and product quality (Lepen, 1996; Mpofu, 2002; Scholtz and Theunissen, 2010). These breed types will also be increasingly used as dam lines, as they mostly have smaller frames with lower feed requirements, low birth weights and mortality at birth, and good maternal abilities (Scholtz, 1988; Schoeman, 1989; Pyoos et al., 2020); as well as good meat quality traits (Strydom, 2008). However, it is important to understand what happened with production in these breeds over the past number of years and what the levels of productivity are within these breeds.
Beef cattle in the Southern African countries will have to adapt to higher ambient temperatures, lower nutritional value of the grass in some cases, and expansion of diseases, especially ticks and tick borne diseases, because of global warming (Scholtz et al., 2013a). There is a large number of different indigenous (landrace) breeds in Southern Africa, which can survive the harsh local conditions, exhibit a low susceptibility to diseases, and with adaptation to other environmental conditions. In terms of the regulations of the Animal Improvement Act (Act 62 of 1998) of South Africa, the Afrikaner, Bonsmara, Drakensberger, and Nguni breeds are classified as landrace breeds.
The Afrikaner and Nguni breeds (Bos taurus africanus) have been closely associated with the people of South Africa (Scholtz, 2010). They are commonly known as Sanga cattle and possess a cardiothoracic hump which is likely derived from admixture between the thoracic-humped Zebu and hump-less African B. taurus cattle (Epstein, 1971; Hanotte et al., 2002). However, a recent study by Makina et al. (2016) indicated that there is very little evidence of B. indicus in the genetic makeup of these breeds, making them taurine tropical adapted breeds and fairly unique.
The Bonsmara breed was developed in the late 1930's using the locally adapted Afrikaner and the Hereford and Shorthorn breeds in a crossbreeding program. The optimal combination for the Bonsmara was found to be 5/8 Afrikaner and 3/8 Exotic (Shorthorn, Hereford) (Scholtz, 2010). No scientific documentary evidence is available on the earliest development or origin of the Drakensberger breed, but it is also classified as an indigenous breed.
The aim of this investigation is to study the environmental impact (carbon footprint) of the changes that occurred in the production/performance in the cowherds of South Africa's landrace breeds over a period of 25–30 years. It will be estimated if these changes had an effect on cow productivity, where cow productivity will be expressed as a ratio between outputs (calf weaning weight) and inputs (derived from the cow weight) and the resultant effect on the carbon footprint, which is defined as kg methane/kg calf weaned.
Materials and Methods
For the calculation of the annual performance/phenotypic trends, yearly breed average mature cow weight (MCW), weaning weight (WW), and inter-calving period (ICP) of all recorded animals were used. These trends will be influenced by genetic and environmental effects, as well as other unidentified effects (Garrick and Enns, 2010). In addition genotype x environment may also play a role (Neser et al., 2008).
There are various ways in which cow productivity or efficiency can be expressed, e.g., the calf/cow weight ratio and calf weight/cow weight0.75 (Dickerson and Grimes, 1947; Frahm and Marshall, 1985). For this study, the three component traits that influence cow productivity were identified as (1) weaning weight of the calf, (2) feed requirements to produce the calf, and (3) the frequency (calving percentage) at which a calf is produced. Weaning weight and fertility of beef cattle raised extensively can be measured fairly easy, but it is not possible to measure the feed requirements to produce the calf directly. However, the concept of a Large Stock Unit (LSU) can be linked to daily feed intake and use to estimate the feed requirements of cows. Likewise, the weaning percentage can be derived from the ICP.
In South Africa, the official definition of a LSU is the equivalent of an ox with a live weight of 450 kg, which gains 500 g per day on grass pasture with a mean digestible energy of 55%. To maintain this 75 MJ per day is required, which translates to a 9 kg dry matter intake per day (Meissner et al., 1983). This is similar to the Animal Unit used in North America (Thorne and Stevenson, 2007). The principles of LSU, the feed requirements of the different frame sizes and the classification of the breeds into the different frame sizes in South Africa is described in Scholtz et al. (2016) and Mokolobate et al. (2017). It is important to note that the LSU equivalent of cows with the same body weight but different frame sizes is different. The reasons for this may be linked to the size of the visceral organs, basal metabolic rate and mitochondrial function (Mokolobate et al., 2017). The relationship between cow weight and LSU is also not linear.
Cow productivity was thus defined as:
Calving Percentage [derived from ICP] × (205-day corrected weaning weight of calf/cow LSU)
Frame size specific equations, developed by Mokolobate (2015), were used to calculate the LSU for lactating cows of different weights (CW). The equation for small frame breeds used to estimate cow LSU units for lactating Afrikaner and Nguni cows is:
The equation for medium frame cows used to estimate the LSU units for lactating Bonsmara and Drakensberger cows is:
The predicted calving percentage was calculated according to Roux and Scholtz (1984) using the following equation:
Cow productivity, expressed as kg calf weaned (205-day corrected weaning weight) per LSU mated (KgC/LSU), was estimated and averaged per year of birth, using the yearly average mature cow- and 205-day corrected weaning weights and calving percentage derived from ICP. Du Toit et al. (2013) estimated the direct methane and nitrous oxide emissions of South African emerging and commercial beef cattle, using the Intergovernmental Panel on Climate Change (IPCC) Tier 2 approach. His study estimated that the enteric methane emissions factor (MEFenteric) of a LSU in the commercial beef sector of South Africa is 94 kg methane/year.
The carbon footprint for this study was thus defined as kg methane/kg calf weaned and estimated as indicated below.
Carbon footprint = 94/(Calving percentage × 205-day weaning weight/LSU)
It is also important to partition the phenotypic observed performance into its respective components in order to determine the extent that genetic or environmental trends or both are influencing the observed trends; as well as climate change.
The performance and pedigree data of seed stock animals of the four landrace breeds (Afrikaner, Bonsmara, Drakensberger, and Nguni), over a period of 25–30 years were used to estimate the genetic and environmental trends. In the case of WW a period of 30 years were studied and in the case of MCW and ICP a period of 25 years, since MCW was taken as the first weight after for years of age and ICP up to the third calf. More information can be obtained from Jordaan (2015). A summary of the performance data of the four breeds is presented in Table 1 and indicates the total number of herds, birth weights (BW), weaning weights (WW), MCW, and number of sires and dams, that was used in the study.

Table 1. Summary of the data set, indicating the number of herds, birth-, weaning- and mature cow weights, sires, and dams used in the genetic analyses.
The Agricultural Research Council (ARC) developed models for the routine genetic evaluation of beef breeds in South Africa in 2010 and these models were used it in a multi trait analysis using PEST (Groeneveld et al., 1990) for this study. The aim of the study was not to develop or evaluate these models. Heritability estimates for the traits and the genetic correlations between them, published by Van der Westhuizen et al. (2010) were used in all the analyses. The traits analyzed included birth-, weaning-, yearling, 18 months, and mature cow weight (1st cow weight after 4 years of age). The genetic- and environmental trends were estimated for 205-day Weaning Weight (direct and maternal) of the calf (WW205) over a period of 30 years and for MCW over a period of 25 years, for the four landrace breeds (Afrikaner, Bonsmara, Drakensberger, and Nguni) using the model indicated below.
where: y = vector of observations b = vector of fixed effects where the following factors were fitted for all traits: sex, contemporary group that consists of herd-year-season, parity (defined as 1 for first calves and 2 for parity 2 and higher), mature cow weight contemporary group only for MCW, linear and quadric regressions for dam age and linear regression for age at weighing a = vector of random animal effects m = vector of random maternal (dam) effects for all traits c1 = vector of random permanent maternal environment for WW, yearling, 18 month, and mature cow weight c2 = vector of random sire by herd year effects fitted for birth and WW e = vector of random residual effects X & Z = incidence matrices relating records to fixed and random animal effects, respectively, Simplified descriptions of the models used for each growth trait are given below:
The traits are as follows: BW, birth weight; WW, weaning weight; YW, yearling weight; 18M, eighteen month weight; and MCW, mature weight.
The factors are as follows: damage, age of the dam at birth of the calf; sex, sex of the calf; parity, fixed effect, first calf or subsequent calves; ccg, fixed effect, contemporary group for birth-, weaning-, year-, 18 month, and mature weight; shy, sire-herd-year-season; pe, permanent environment of the dam, dam = dam of the calf; and animal, calf.
In the case of fertility, the traits included in the multi-trait PEST analysis (Groeneveld et al., 1990) were Inter-Calving Period for the first three calving's (ICP1-3), measured in days and Age at First Calving (AFC) measured in months. Only the results for ICP1-3 are reported here and no environmental trends were estimated. Since the genetic trends in ICP for all the breeds were very low for the period of 25 years, the environmental trend is largely a reflection of the phenotypic trend.
The following model was used:
where:
y = vector of observations
b = vector of fixed effects where the following factors were fitted for Age at First Calving (AFC): Weaning Weight Code (WWC) where 1 = own weaning weight <179 kg, 2 = own weaning weight 179–254 kg, 3 = own weaning weight >254 kg. Weaning weight contemporary group consisted of herd-year-season of weaning weight measurement and contemporary group for Age at First Calving (AFC) consisting of herd-year-season. For ICP the dam age at consecutive calving's, were used as a linear regression.
a = vector of random animal effects
e = vector of random residual effects
X & Z = incidence matrices relating records to fixed and random animal effects, respectively,
A simplified description of the models used for each fertility trait are given below:
where:
The traits are as follows: AFC, age at first calving; ICP1, inter calving period one, ICP2, inter calving period two; ICP3, inter calving period three.
The fixed factors are as follows: damage, age of the dam at birth of the calf; ccg, contemporary group (herd, year, and season); wwc, weaning weight of the cow.
The individual BLUP (Best Linear Unbiased Prediction) breeding values and BLUE (Best Linear Unbiased Estimate) environmental values were derived from the outputs of the models specified above. These values were averaged within birth years and used for the estimation of the genetic and environmental trends, by regressing the average values on year of birth (Zishiri et al., 2010). Standard linear regression techniques were used to estimate the trends of the four breeds over time and the results are summarized in Table 2.
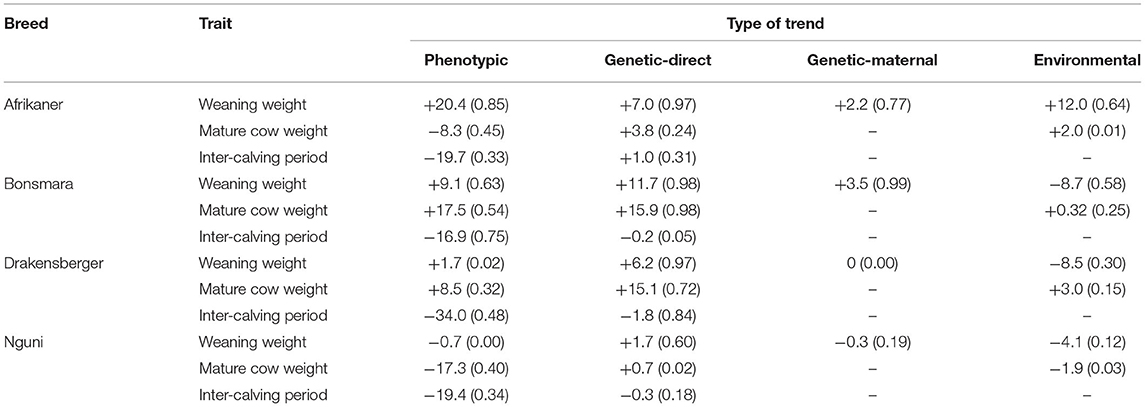
Table 2. Summarized phenotypic, direct, and maternal genetic and environmental trends (change) for the two weight traits (in kg) and inter-calving period (in days) for the four landrace breeds (R2 in brackets).
Results and Discussion
The phenotypic, genetic and environmental trends of all the traits, for the four breeds are summarized in Table 2. The R2 for some of the traits are low or almost zero. One possible reason for this may be a lack of data, especially in the case of the Nguni where the level of recording is low.
The genetic trends in Weaning Weight-direct (WW205-D) and the observed trends in the phenotypic performance for all four breeds are illustrated in Figures 1, 2. The Afrikaner, Bonsmara and Drakensberger breeds all show positive genetic trends for WW205-D with an increase of 7.0, 11.7, and 6.2 kg, respectively, over the 30-year period. In the case of the Nguni, there was no genetic change for WW205-D as demonstrated in Table 2 and Figure 1. This is probably the result of not selecting breeding stock based on performance but rather on type. The aim of some Nguni breeders is also not to change the breed, but keep it as nature has formed it.
In the case of Weaning Weight-maternal (WW205-M), there were small positive genetic trends in the case of the Afrikaner (+2.2 kg) and the Bonsmara (+3.5 kg), indicating that there was some genetic improvement in the milk production of these two breeds; in contrast, no changes were observed for the Drakensberger and Nguni (Table 2).
There is a large positive phenotypic trend in WW205 in the Afrikaner (+20.4 kg) and Bonsmara (+9.1 kg) breeds, as indicated in Table 2 and Figure 2; while virtually no change was observed in the case of the Drakensberger (+1.7 kg) and Nguni (−0.7 kg) breeds. It is interesting to note that in the case of the Bonsmara and Drakensberger breeds, the genetic trends were larger than the phenotypic trends. This may be an indication that the environment cannot support the higher weaning weights implicated by the genetic trends.
In all breeds, except for the Afrikaner, the environmental trends for WW205 were negative. In the case of the Bonsmara, the phenotypic trend was +9.1 kg and the environmental trend −8.7 kg. There may be many reasons for this antagonistic trend, including poorer adaptation due to a possible diminishing influence of Afrikaner genes in the Bonsmara (Makina et al., 2016), or the decrease in the number of breeders (farmers) from 510 to 353, which may imply that the production environments have changed, which induced a possible genotype x environment interaction.
The low R2 found for some of the traits (Table 2) may be due to a lack of sufficient data and/or demonstrates that there was no trend for the specific trait. In the case of the Nguni, six out of the nine R2's are <0.20. In this breed, <20% of the animals are the performance recorded and there is no clear breeding objective. Only one of the trends for both the Afrikaner (environmental trend for mature cow weight) and Bonsmara (Genetic-direct for ICP) has a R2 value below 0.20.
A comparison of the changes in weaning weight (phenotypic, genetic-direct, genetic-maternal, and environmental) of the four breeds is presented in Figure 3. In the case of the Afrikaner, all the changes are positive with the phenotypic change the largest. This indicates that the phenotypic trend is a result of an improvement in all the other components (genetic and environmental). It is also important to note that a large number of genes associated with adaptation has recently been discovered in the Afrikaner (Mdyogolo, 2021). As early as 1981, Frisch (1981) indicted that selection for growth rate under stressful environments, may result in selection for adaptation to the environment, which may explain this result.
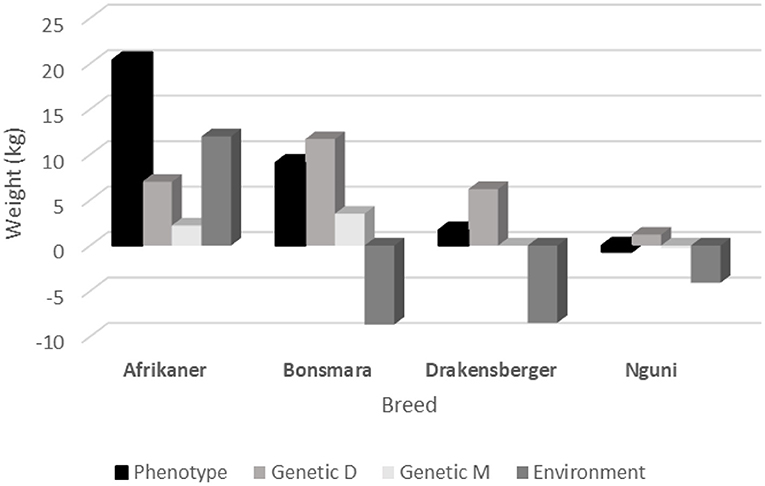
Figure 3. A comparison of the different trends in weaning weight (phenotypic, direct, maternal, and environmental) of the four breeds.
In the Bonsmara and Drakensberger breeds the phenotypic and environmental changes are in opposite directions. This may suggest a problem with adaptation in these two breeds. This is especially remarkable in the case of the Bonsmara, where the phenotypic trend is +9.1 kg and the environmental trend −8.7 kg. These results also supports the way back suggestion by Neser et al. (1996) that herd-year-season by sire interaction should be included in the estimation of genetic parameters in Bonsmara cattle and that of Neser et al. (2008) that a cluster analysis should be used in the genetic evaluation of the Bonsmara breed.
The genetic and phenotypic trends for MCW for all four breeds are summarized in Table 2 and illustrated in Figures 4, 5, respectively. In the case of the Bonsmara and Drakensberger, there are positive genetic trends in MCW. It is only in the case of the Nguni that there is a clear negative phenotypic trend in MCW, indicating the cows became lighter.
In Figure 6, a comparison of the different trends in MCW (phenotypic, genetic direct, and environmental) of the four breeds are presented. The breed that showed the largest genetic change for MCW is the Bonsmara (+15.9), followed by the Drakensberger (+15.1 kg). This increase in MCW could possibly be attributed to correlated responses, which is the result of selection for other growth traits such as weaning weight and post-weaning growth. The Bonsmara (+17.5 kg) and Drakensberger (+8.5 kg) also showed a positive phenotypic trend for MCW, in contrast to the Afrikaner (−8.3 kg) and Nguni (−17.3 kg).
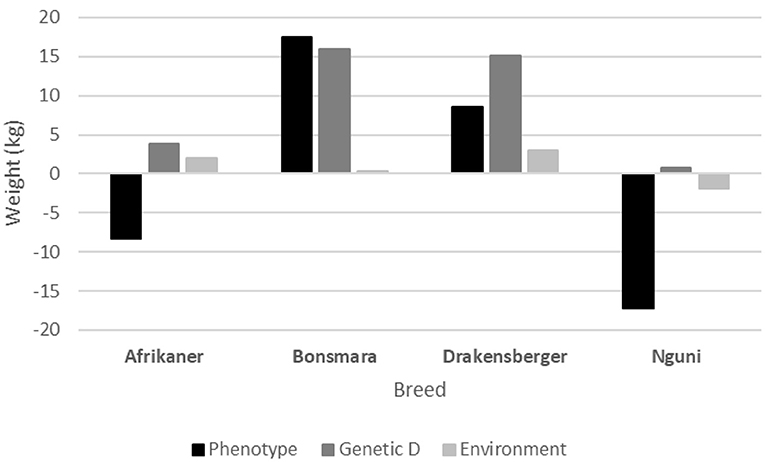
Figure 6. A comparison of the different trends in mature cow weight (genetic direct, environmental, and phenotypic) of the four breeds.
It is important to note that in the case of the Afrikaner, both the genetic (+3.8 kg) and environmental (+2.0 kg) trends for MCW are positive, but the phenotypic trend for MCW is negative (−8.3 kg). The fact that the phenotypic trend for WW205 is +20.4 kg may be partially the result of higher milk production (+2.2 kg), that put strain on the cow's resources, resulting in a lower phenotypic MCW.
The genetic (ICP1-3) and phenotypic trends for ICP for all four breeds are summarized in Table 2 and illustrated in Figures 7, 8, respectively. None of the breeds showed a significant genetic trend for ICP1-3, indicating that there was no genetic improvement in this fertility trait. In spite of this, there was a large negative phenotypic trend in ICP for all the breeds (Table 2 and Figure 8), ranging from −19.9 days in the case of the Bonsmara to −34.0 days in the case of the Drakensberger. This can probably be attributed to the culling of cows that exceeded a certain ICP, or better management and the application of breed standards with respect to maximum ICP's.
Interestingly, in the weight traits, the genetic tend is larger than the phenotypic trend in a number of traits (Figures 3, 6). The question that may be asked is whether these breeds have reached the limit of their performance for the specific environment. It is possible that climate change may have an effect in this phenomena. Climate can effect beef cattle in a number of ways. Firstly, there is the direct effect of weather and extreme events on animal health, growth and reproduction. Secondly, it impacts on pasture production and quality (Scholtz et al., 2013b).
The phenotypic changes in cow productivity (KgC/LSU) and its component traits are summarized in Table 3. In addition, the changes carbon footprint, using the enteric methane emissions factor (MEFenteric), was also estimated and summarized in the table. In all breeds the cow productivity increased by between 10 and 18.3%, with a reduction in the carbon footprint of between −12 and −6.6%. In the case of the Afrikaner, where the increase in cow productivity was the highest (18.3%), it resulted in more efficient breeding cows with less maintenance requirements per unit of product and thus reducing the carbon footprint of weaner calf production by 12%. Selection that only focus on growth traits will result in an increase in mature weight and an increase in maintenance requirements. However, this did not happen in the Afrikaner. Selection should be used as a tool to increase profitability, and not just revenues (Enns et al., 2001), as was the case in the Afrikaner. As mentioned previously selection for growth rate in the Afrikaner under stressful environments, may have lead to selection for adaptation to the environment.

Table 3. The phenotypic changes in the component traits of cow productivity, cow productivity, and the carbon footprint in the four landrace breeds.
Despite the phenotypic increase in MCW (+8.5 kg), the cow productivity in the Drakensberger improved as indicated in Table 3; the reason being the decrease in ICP of 34 days (higher estimated fertility). The net result is a remarkable increase of 14.2% in cow productivity (Table 3). In the case of the Bonsmara there was an increase of 17.5 kg in the MCW. However, the ICP decreased by almost 17 days, resulting in an improvement of 10% in cow productivity. In the case of the Nguni cow productivity increased by 10.4% (Table 3). Since there was no significant change in WW205 (R2 = 0 from Table 2), this increase is the result of the decrease in MCW and ICP. In all the breeds it is evident that an improvement in fertility (shorter ICP) increase cow productivity and reduce the carbon footprint. The 76% calving percentage of seed stock breeds and the 61% in the total South African commercial beef cattle sector (Scholtz et al., 2016) is thus a matter of concern.
Factors such as temperature, solar radiation, humidity and wind all have direct effects on livestock. Ambient temperature is the factor that has the largest direct effect on ruminant production. Most ruminants perform at their best at temperatures between 4 and 24°C (Scholtz et al., 2013b). MacNeil and Vermeire (2012) indicated that heat stress in cows during late pregnancy can have downstream effects on the performance of the progeny during the suckling period and that heat stress per se negatively affect the growth of suckling calves. Factors such as digestibility of feed, quality and quantity of grazing all have indirect effects on animals. As temperature increases, the vegetation may change from C3 to C4 grasses and in some areas, the grazing capacity is expected to decline (Scholtz et al., 2013a). This will result in natural pasture with both a lower nutritional value and lower tiller density than in temperate regions. These effects, because of climate change, may explain some of the antagonism found between the genetic, environmental, and phenotypic trends in the weight traits.
Conclusion
All breeds showed an improvement in cow productivity, but it seems that some breed societies and breeders will have to reconsider their breeding strategies. Differences between the phenotypic and genetic trends were demonstrated between breeds. There are three possible reasons for this. Firstly, there may be changes in the production environment, production system, and production region. In the case of Nguni cattle, for example, they are currently farmed with in areas that were traditionally regarded as unsuitable for cattle farming. Secondly, the relative emphasis on pre-weaning and post-weaning traits between the breeds may be a reason for the differences demonstrated. Lastly, the effects of climate change on breed adaptation, and the availability and quality of the nutrition may be an explanation.
The fact that there was no detectable genetic change for ICP1-3 in all the breeds is an issue of concern. It is therefore important that technologies for the genetic improvement of fertility in beef cattle in South Africa must be researched properly, developed or refined. The decrease in the phenotypic trend for ICP found in this study can be attributed of many factors; including, but not limited to better record keeping by both farmers and the breeder's societies, management decisions to cull cows that do not reconceive regularly or within a specified period of time and sticker application of breed standards in respect of fertility. However, these management decisions did not result in any genetic improvement.
This study demonstrated the genetic and phenotypic changes in South Africa's landrace beef breeds over a period of 25–30 years and the effect of these changes on the cow productivity of these breeds. It is important to note that what is reported in this study are trends over time and the direct connection with selection is weak. Future studies should attempt to collect data that will enable the estimation of selection differentials. This can even include a more in-depth analyses by considering the four paths of selection (sires of sires, sires of dams, dams of sires, and dams of dams).
The study also demonstrated that cow productivity improved in all the landrace breeds. The improvement in cow productivity is a key component if the environmental impact of beef production is to be reduced, while sufficient animal protein is produced to satisfy the growing demand. To achieve this, multi trait selection indices will have to be developed that increase cow productivity for South Africa's landrace breeds. This can even be extended to a carbon footprint selection index.
It is important that similar studies be carried out on all the major beef breeds that are present in South Africa. It is important to get an indication of the changes that occurred in the different breeds and breed types in South Africa and how this affect cow productivity. This may be of particular relevance in the case of breeds that import genetic material regularly.
Data Availability Statement
The data analysed in this study was subject to the following licences/restrictions: the dataset forms part of the National Beef Recording and Improvement Scheme and is only available on request. Requests to access these datasets should be directed to Corrie Van Zyl, VanZylC@arc.agric.za.
Ethics Statement
Ethical review and approval was not required for the animal study because the datasets were obtained from the INTERGIS, which stores all data from the National Beef Recording and Improvement Scheme. Data for this scheme was collected according to approved standard operating procedures and was accredited with the International Committee for Animal Recording (ICAR).
Author Contributions
This study was partially based on the MSc dissertation of FJ. MS, FN, and AM conceived the investigation. ZK assisted with the preparation, data analysis, and preparation of the figures and manuscript. FJ prepared the data for analysis and analyzed the data under the guidance of FN and MS. FJ, MS, FN, and AM contributed to interpretation of the results. FJ drafted the initial manuscript, which was subsequently edited by MS. All authors read and approved the final manuscript.
Funding
This work was based on research supported in part by Red Meat Research and Development South Africa and the National Research Foundation of South Africa (NRF), under grant UID 75121.
Author Disclaimer
The Grant holder acknowledges that opinions, findings, and conclusions or recommendations expressed in any publication generated by the NRF supported research are that of the authors and that the NRF accepts no liability whatsoever in this regard.
Conflict of Interest
The authors declare that the research was conducted in the absence of any commercial or financial relationships that could be construed as a potential conflict of interest.
Publisher's Note
All claims expressed in this article are solely those of the authors and do not necessarily represent those of their affiliated organizations, or those of the publisher, the editors and the reviewers. Any product that may be evaluated in this article, or claim that may be made by its manufacturer, is not guaranteed or endorsed by the publisher.
References
Capper, J. L. (2011). The environmental impact of beef production in the United States: 1977 compared with 2007. J. Anim. Sci. 89, 4249–4261. doi: 10.2527/jas.2010-3784
Dickerson, G. E., and Grimes, J. C. (1947). Effectiveness of selection for efficiency of gain in Duroc swine. J. Anim. Sci. 6, 265–287. doi: 10.2527/jas1947.63265x
Du Toit, C. J. L., Meissner, H. H., and van Niekerk, W. A. (2013). Direct methane and nitrous emissions of South African dairy and beef. S. Afr. J. Anim. Sci. 43, 320–339. doi: 10.4314/sajas.v43i3.7
Enns, M. R., Speidel, S. E., and McAllister, C. M. (2001). Genetic Trend for American Tarentaise. Fort Collins: Colorado State University; Center for Genetic Evaluation of Livestock.
Epstein, H. (1971). The Origin of the Domestic Animals of Africa, Vol. 1. Edinburgh: The Hebrew University of Jerusalem. Institute of Animal Genetics.
Ferrell, C. L., and Jenkins, T. G. (1982). Efficiency of Cows of Different Size and Milk Production Potential. RLHUSMARC Germ Plasm Evaluation Report 10, 12.
Frahm, R. R., and Marshall, D. M. (1985). Comparisons among two-breed cross cow groups. I. Cow productivity and calf performance to weaning. J. Anim. Sci. 61, 844–855. doi: 10.2527/jas1985.614844x
Frisch, J. E. (1981). Changes occurring in cattle as a consequence of selection for growth rate in a stressful environment. J. Agric. Sci. 96, 23–38. doi: 10.1017/S0021859600031841
Garrick, D. J., and Enns, R. M. (2010). How Best to Achieve Genetic Change. Available online at: http://www.sbmaonline.org.br/~anais/v/palestras/pdfs/palest08.pdf (accessed April 25, 2016).
Groeneveld, E., Kovac, M., and Wang, T. (1990). “PEST, a general purpose BLUP package for multivariate prediction and estimation,” in Proceedings of the World Congress on Genetics Applied to Livestock Production, Edinburgh 23-27 July 1990. XIII. Plenary Lectures, Molecular Genetics and Mapping, Selection, Prediction and Estimation (Edinburgh), 488–491.
Hanotte, O., Bradley, D. G., Ochieng, J. W., Verjee, Y., Hill, E. W., and Rege, E. O. (2002). African pastoralism: genetic imprints of origins and migrations Science 296, 336–339. doi: 10.1126/science.1069878
Jordaan, F. J. (2015). Genetic and environmental trends in landrace beef breeds and the effect on cow productivity [master's thesis]. University of the Free State, Bloemfontein South Africa.
Lepen, J. M. (1996). Breed Characterization Studies in Namibia. Available online at: www.nbri.org.na/old/agricola_files/Agricola1996
MacNeil, M. D., and Vermeire, L. T. (2012). Effect of weather patterns on preweaning growth of beef calves in the Northern Great Plains. Agric. Sci. 3, 929–935. doi: 10.4236/as.2012.37113
Makina, S. O., Whitacre, L. K., Decker, J. E., Taylor, J. F., MacNeil, M. D., Scholtz, M. M., et al. (2016). Insight into the genetic composition of South African Sanga cattle using SNP data from cattle breeds worldwide. Genet. Sel. Evol. 48:88. doi: 10.1186/s12711-016-0266-1
Mdyogolo, S. (2021). Detection of selection signatures and genes associated with fitness in South African Afrikaner and Brahman cattle [doctoral dissertation]. University of the Free State, Bloemfontein, South Africa.
Meissner, H. H., Hofmeyr, H. S., Van Rensburg, W. J. J., and Pienaar, J. P. (1983). Classification of Livestock for Realistic Prediction of Substitution Values in Terms of a Biologically Defined Large Stock Unit. Technical Communication No. 175. Department of Agriculture, Pretoria.
Mokolobate, M. C. (2015). Novelty traits to improve cow-calf efficiency in climate smart beef production systems [master's thesis]. University of the Free State, Bloemfontein, South Africa.
Mokolobate, M. C., Scholtz, M. M., and Calitz, F. J. (2017). Explaining the principle of large stock units and its implications on grazing capacity. Appl. Anim. Husb. Rural Dev. 10, 17–20. Available online at: https://www.sasas.co.za/wp-content/uploads/2017/11/Mokolobate_vol-10_Issue_1
Mpofu, N. (2002). The Multiplication of Africa's Indigenous Cattle Breeds Internationally: The Story of the Tuli and Boran Breeds. Available online at: http://agtr.ilri.cgiar.org/Casestudy/case-mpofu-1-TuliBoran-31.htm (accessed January 7, 2020).
Neser, F. W. C., Erasmus, G. J., and Scholtz, M. M. (2008). The use of a cluster analysis in across herd genetic evaluation for beef cattle. S. Afr. J. Anim. Sci. 38, 51–57. doi: 10.4314/sajas.v38i1.4109
Neser, F. W. C., Konstantinov, K. V., and Erasmus, G. J. (1996). The inclusion of herd-year-season by sire interaction in the estimation of genetic parameters in Bonsmara cattle. S. Afr. J. Anim. Sci. 26, 75–78. doi: 10.4314/sajas.v26i3.44322
Pyoos, G. M., Scholtz, M. M., Macneil, M. D., Theunissen, A., and Neser, F. W. C. (2020). Genetic effects from an Afrikaner, Bonsmara, and Nguni three-breed diallel and top-crosses of Angus and Simmental sires. S. Afr. J. Anim. Sci. 50, 366–377. doi: 10.4314/sajas.v50i3.3
Roux, C. Z., and Scholtz, M. M. (1984). “Breeding for optimal total life cycle production systems,” in Proceedings of the 2nd World Congress on Sheep and Beef Cattle Breeding (Pretoria), 444–454.
Schoeman, S. J. (1989). Recent research into production potential of indigenous cattle with special reference to Sanga (Review). S. Afr. J. Anim. Sci. 19, 55–61.
Scholtz, M. M. (1988). “Selection possibilities for hardy beef breeds in Africa: the Nguni example,” in Proceedings of the 3rd World Congress on Sheep and Beef Cattle Breeding (Paris), 303–319.
Scholtz, M. M., (ed.). (2010). Beef Breeding in South Africa, 2nd Edn. Pretoria: Agricultural Research Council.
Scholtz, M. M., Maiwashe, A., Neser, F. W. C., Theunissen, A., Olivier, W. J., Mokolobate, M. C., et al. (2013a). Livestock breeding for sustainability to mitigate global warming, with the emphasis on developing countries. S. Afr. J. Anim. Sci. 43, 269–281. doi: 10.4314/sajas.v43i3.4
Scholtz, M. M., McManus, C., Leeuw, K.-J., Louvandini, H., Seixas, L., Demelo, C. B., et al. (2013b). The effect of global warming on beef production in developing countries of the southern hemisphere. Nat. Sci. 5, 106–119. doi: 10.4236/ns.2013.51A017
Scholtz, M. M., McManus, C., Okeyo, A. M., and Theunissen, A. (2011). Opportunities for beef production in developing countries of the southern hemisphere. Livest. Sci. 142, 195–202. doi: 10.1016/j.livsci.2011.07.014
Scholtz, M. M., Mokolobate, M. C., Jordaan, F. J., Neser, F. W. C., and Theunissen, A. (2016). A critical analysis of cow-calf efficiency in extensive beef production systems. Appl. Anim. Husb. Rural Dev. 9, 11–20. doi: 10.10520/EJC94774
Scholtz, M. M., and Theunissen, A. (2010). The use of indigenous cattle in terminal crossbreeding to improve beef cattle production in Sub-Saharan Africa. Anim. Genet. Res. 46, 33–36. doi: 10.1017/S2078633610000676
Strydom, P. E. (2008). Do indigenous Southern African cattle breeds have the right genetics for commercial production of quality meat? Meat Sci. 80, 86–93. doi: 10.1016/j.meatsci.2008.04.017
Thorne, M. S., and Stevenson, M. H. (2007). Stocking Rate: The Most Important Tool in the Toolbox. College of Tropical Agriculture and Human Resources. University of Hawaii, Manoa. Available online at: http://www.ctahr.hawaii.edu/oc/freepubs/pdf/PRM-4.pdf (assessed April 27, 2016).
Van der Westhuizen, R. R., Theron, H. E., Van der Westhuizen, J., Maiwaishe, A., and Jordaan, F. (2010). National beef recording and improvement scheme. Newsletter 99:39.
Wall, E., Simm, G., and Moran, D. (2010). Developing breeding schemes to assist mitigation of greenhouse gas emissions. Animal 4, 1–11. doi: 10.1017/S175173110999070X
Keywords: mature cow weight, fertility, large stock unit, production trends, weaning weight
Citation: Jordaan FJ, Neser FWC, Maiwashe A, King Z and Scholtz MM (2021) The Environmental Impact of Changes in Cow Productivity and Its Component Traits in South Africa's Landrace Beef Breeds. Front. Anim. Sci. 2:743229. doi: 10.3389/fanim.2021.743229
Received: 17 July 2021; Accepted: 09 September 2021;
Published: 04 October 2021.
Edited by:
Pasquale De Palo, University of Bari Aldo Moro, ItalyReviewed by:
Yuzo Kurokawa, Hiroshima University, JapanFabio Abeni, Consiglio per la Ricerca in Agricoltura e l'Analisi dell'Economia Agraria (CREA), Italy
Copyright © 2021 Jordaan, Neser, Maiwashe, King and Scholtz. This is an open-access article distributed under the terms of the Creative Commons Attribution License (CC BY). The use, distribution or reproduction in other forums is permitted, provided the original author(s) and the copyright owner(s) are credited and that the original publication in this journal is cited, in accordance with accepted academic practice. No use, distribution or reproduction is permitted which does not comply with these terms.
*Correspondence: Frans J. Jordaan, fransj@arc.agric.za