- 1Departamento de Producción Animal y Pasturas, Facultad de Agronomía, Universidad de la República Uruguay, Paysandú, Uruguay
- 2Departamento de Producción Animal, Facultad de Agronomía de Azul, Universidad Nacional del Centro de la Provincia de Buenos Aires, Azul, Argentina
- 3Empresa Brasileira de Pesquisa Agropecuária, Bagé, Brazil
Sward height is strongly related to the daily dry matter intake of grazing dairy cows, which consequently determines animal performance. Despite that, few studies have explored the potential to increase milk production by managing post-grazing sward height. An experiment was carried out to evaluate the effect of three defoliation intensities on a Lolium arundinaceum-based pasture on frequency and length of grazing meals and ruminating bouts, daily grazing and ruminating time, feeding stations and patches exploration, and dry matter intake and milk production of dairy cows. The treatments imposed were three different post-grazing sward heights: control (TC), medium (TM), and lax (TL), which were managed with 6, 9, and 12 cm of post-grazing sward heights during autumn and winter, and 9, 12, and 15 cm of post-grazing sward heights during spring, respectively. Thirty-six autumn-calving Holstein cows were grouped by parity (2.6 ± 0.8), body weight (618 ± 48) kg, and body condition score (2.8 ± 0.2) and randomized to the treatments. The pasture was accessed from 08:00 to 14:00 and 17:00 to 03:00 during spring and no supplement was involved during the evaluation period. Daily grazing time averaged 508 ± 15 min and was not affected by treatment. The reduction of post-grazing sward height increased the length of the first grazing session in the morning and the afternoon. The number of grazing sessions was greater on TL than on TM, with no difference in TC. The number of feeding stations (the hypothetical semi-circle in front of an animal from which the bites were taken without moving the front forefeet) visited was less on TC than on TL, and neither of them differed from TM. Dry matter (DM) intake was lesser on TC than on TM and TL (14.7 vs. 17.8 kg DM). Milk production during the evaluation period was 13.1, 16.2, and 18.7 kg/day for TC, TM, and TL, respectively. The milk fat, protein, and lactose content did not differ between treatments. The cows on TC exhibited a lower intake rate, although they were less selective, probably as a consequence of the sward structure of TC treatment. The cows on TM adopted a compensation mechanism which allowed them to achieve the same dry matter intake as cows on TL, but lower milk production. The cows on TL were more selective than TC and TM resulting in higher digestible dry matter intake and consequently higher milk production. The intensity of defoliation impacts on the animal-plant interaction, and constitutes a valuable management tool that can be used to boost forage intake and milk production. The new developments on information technology would allow researchers to link behavioral data with response variables (e.g., milk production, health, welfare, etc.) at different spatio-temporal scales and support short and long-term management decisions.
Introduction
Pasture-based dairy systems are receiving special attention around the world because they may offer benefits to several environmental aspects (Aguirre-Villegas et al., 2017), may present profitability advantages (O'Brien et al.,2015), and are well-regarded by consumers in terms of animal welfare (Cardoso et al., 2019). Uruguayan pasture-based dairy farming systems have the potential for production growth and must achieve it to maintain international competitiveness (Fariña and Chilibroste, 2019). The optimized utilization of grazed pastures to reduce the costs associated with feeding dairy cows is a common goal for these dairy production systems (Finneran et al., 2010).
Grazing is a complex process that involves a set of decisions at different scales with bite mass as a basic unit. Hence, daily pasture dry matter intake by grazing animals is largely dependent on the mass of each bite and the frequency of them, which is affected by the sward height (Gibb et al., 1997) and herbage mass (Laca et al., 1992) available to animals. The daily grazing pattern adopted by the animals will depend mainly on their intrinsic behavior, photoperiod (Linnane et al., 2001), sward characteristics (Chapman et al., 2007), internal state (e.g., ruminal fill, energy demand, hormone release, physiological state, and adiposity, etc.; Chilibroste et al., 2005), spatial memory (ability of herbivores to use their previous experience to choose where to graze; Bailey et al., 1996), and anthropogenic management (Chilibroste et al., 2015).
The actions of a grazing animal can be divided at different spatio-temporal scales (Carvalho and Moraes, 2005). Considering the level of anthropogenic management, such as the use of fences to manage the forage allowance, in dairy farming could be identified at small spatial scales like patch, feeding stations, and bite scale. When the grazing animal has its front forefeet immobile and take bites in front of it, this area is defined as a feeding station (Ruyle and Dwyer, 1985). The selection of a feeding station is highly related to sward mass, structure, and quality, so it is a good indicator of grazing conditions (Carvalho and Moraes, 2005). In this context the animal behavior could be summarized as searching time and displacement rate between feeding stations and bite mass (Griffiths et al., 2003) and residence time in each feeding station (Gonçalves et al., 2009), as a general relationship has been postulated that large herbivores tend to spend more time exploring each feeding station as forage biomass increases (Searle et al., 2005).
Grazing management strategies will also have direct effects on pasture growth, where low post-grazing sward heights have an unfavorable impact on pasture regrowth and on the total forage production (Chapman, 2016). In Uruguay, previous research has indicated that increasing post-grazing sward height presents benefits for both pasture and animal performances (Mattiauda et al., 2009; Zibil et al., 2016). However, studies to understand the relationships between daily dry matter intake in eating behavior and milk production of dairy cows at different residual sward height management are scarce. The potential to increase milk production by increasing post-grazing sward height, and seeking an optimal point in the plant-animal interface, is still unknown in fescue. Therefore, an experiment was conducted to study the influence of post-grazing sward height on dry matter intake, milk production, daily grazing and ruminating time as well as frequency and length of grazing meals and ruminating bouts as well as exploration of feeding stations and patches. The hypothesis was that higher post-grazing sward height will increase intake rate, total dry matter intake, and milk production, through a more selective ingestive behavior, leading to a diet with a higher nutritive characteristic. These cows will do that by dividing the daily grazing time into shorter meals followed by a ruminating session and will devote more time to exploring each feeding station with fewer steps of searching between them.
Materials and Methods
The experiment was carried out at the Experimental Research Station “Dr. Mario A. Cassinoni” (EEMAC) of the Faculty of Agronomy–University of Uruguay (Paysandú, Uruguay, 32°S, 58°W), from late October to early November 2017. A pasture of tall fescue (Lolium arundinaceum cv. INIA Fortuna) was sown in May 2016 at 10 kg/ha. At the time of sowing, it was fertilized with 18 kg of N and 46 kg of P/ha and in the 2nd year, 180 kg of N/ha was applied in four equal applications from June to October. In February 2017, the sward was homogenized by mechanically mowing to 6 cm and the grazing treatments were implemented in April. Animal procedures were approved by the Animal Experimentation Committee of the University of Uruguay, application number 02174500019718 (Montevideo, Uruguay).
Treatments and Experimental Design
Three post-grazing sward heights were tested in a randomized complete block design with four spatial blocks of three paddocks each of 0.2 ha. Each paddock was grazed by the same three milking cows throughout the year. The criteria to start grazing in each treatment was when three new extended leaves developed (Fulkerson and Donaghy, 2001). From April to July, the post-grazing sward heights were 6, 9, and 12 cm for control (TC), medium (TM), and lax treatment (TL). During this period, three grazing cycles were implemented for TM and TL and two for TC. In August and September, two grazing cycles were implemented where all treatments were managed with a common post-grazing sward height of 5 cm grazed by dairy heifers at a high instantaneous stocking rate to prevent elongation of flowering stems (Jáuregui et al., 2017). Late October/early November was the evaluation period when all the pasture and animal measurements were undertaken. The treatments managed from April to July with 6, 9 and 12 cm post-grazing sward height were managed with 9 (TC), 12 (TM) and 15 cm (TL), respectively, during the evaluation period. The different post-grazing sward heights were generated by maintaining cows on the plot areas until the target sward heights were obtained.
Animals and Management
In early April, 36 autumn-calving Holstein cows were grouped by body weight (BW), body condition score (BCS), parity number, and days in milk (DIM), and randomized to the treatments. During the periods when cows were not grazing in the experimental paddocks they remained in a common herd with similar management. Prior to the evaluation period (late October/early November) cows were 618 ± 48 kg BW, with 2.8 ± 0.2 BCS (on a 1–5 scale), a 2.6 ± 0.8 parity number, and 224 ± 7 DIM.
Before grazing in the treatment paddocks, all experimental cows grazed in an adjacent paddock at a similar herbage allowance. At this stage, milk production was equal between treatments (16.7 ± 0.89; P = 0.963). Cows were not supplemented and were removed from the paddock twice a day to be milked (04:00 and 15:00).
Measurements and Calculations
Sward Features
Weekly monitoring of the pasture's physiological state was performed to determine the start of grazing (Fulkerson and Donaghy, 2001). Sward height was measured pre and post-grazing, and every day during the paddock occupation period with a 1-m ruler, graduated in centimeters, using an adaptation of the technique described by Barthram (1986). The readings of sward height were taken from ground level at a minimum of 70 random sites in each paddock (performed along four transect lines). The sward mass was estimated pre and post-grazing using an adaption of the double sampling technique of Haydock and Shaw (1975) with a rising plate meter (RPM; Ashgrove Co., Palmerston North, New Zealand), as described by Mattiauda et al. (2013). Characterization of pre-grazing sward height was undertaken at ≈700 points per paddock (16 transects) with the RPM 1 day before grazing. The points were then classified according to their height in high (HH; >30 cm), medium (MH; between 15 and 30 cm), and low height (LH; < 15 cm). Two representative points were chosen in each paddock for each sward height type (HH, MH, and LH), and the leaf sheath height was measured on 5 tillers of fescue in 0.1 m2 (30 cm × 34 cm).
Grazing and Ruminating Behavior
The grazing behavior data were collected from 27 cows (three complete blocks) during 24 h at two moments: at the beginning (BM; day 3) and the end (EM; day 6 for treatment TC and day 5 for TM and TL) of the occupation period. The cows were equipped with behavioral recorders (IGER; Rutter et al., 1997) to measure grazing, ruminating, and idling times, as well as the number and length of grazing and rumination bouts. The raw data were processed using the Graze software (Rutter, 2000) following the definitions described by Gibb et al. (1999).
Displacement and Feeding Station and Patch Use
The number of feeding stations visited and the number of steps between feeding stations were registered by visual tests from 08:00 to 11:30 and from 17:00 to 19:30, at the beginning and the end of the paddock occupation period. The observations were performed by one observer for every three cows and the evaluation was sequential for 15 min for each individual cow. A feeding station was represented by the hypothetical semi-circle in front of an animal from which the bites were taken without moving the front forefeet (Ruyle and Dwyer, 1985). A new patch was counted when the cow did at least two consecutive searching steps (Gregorini et al., 2007).
Animal
Individual milk yield was measured twice a day at 04:00 and 15:00. On the third day of the occupation period, an individual milk sample was collected in both the morning and afternoon milking to determine milk fat, protein, and lactose concentration by mid infrared spectrophotometry (Milko-Scan, Foss Electric, Hillerød, Denmark).
Dosage of C32-Alkane and Collection of Feces and Forage
Individual herbage dry matter intake and digestibility were determined in 27 cows (three complete blocks) using the alkanes technique (Dove and Mayes, 2006), with hentriacontane (C31) as an internal marker, and dotriacontane (C32) and hexatriacontane (C36) as an external marker. For 10 consecutive days, the cows were dosed, after morning and evening milking, with a cellulose pellet containing 344 mg of C32 and 145 mg of C36. From days 5 to 10, feces samples were collected per rectum, after morning and evening milking, and immediately stored frozen at −20°C. Sample collection was performed in the last days of the occupation period. To achieve this, the alkane dosing started 3 days before grazing in the treatment paddocks, in an adjacent paddock with similar herbage allowance. At the end of the collection period, the samples from each animal were thawed and homogenized, dried until constant weight in a 60°C air-forced oven, and then milled through a 1-mm screen and stored until they were analyzed for alkanes concentrations. To characterize the selected diet by cows, herbage samples were collected on days 2 and 4 of the feces collection period by hand clipping (Coates and Penning, 2000) while following individual cows for 1 h in the first morning and evening grazing session. They were dried until constant weight in a 60°C air-forced oven and then milled through a 1-mm screen and stored until they were analyzed for concentration of alkanes and chemical composition.
Analytical Methods
The determination of alkanes present in the forage and feces followed the protocol proposed by Dove and Mayes (2006). The identification and quantification of alkanes were made by gas chromatography using a Shimadzu GC-2010 gas chromatograph equipped with a flame ionization detector, a spool auto-sampler AOC-20S, and an injector auto-injector AOC20i. The extracted n-alkanes were injected (1 μL) into a column Restek Rtx®-5 (30 m, 0.25 mm × 0.25 μm, absorbent composed by 5% diphenyl and 95% polysiloxane dimitil). For more details, please see Savian et al. (2014).
Herbage samples were also analyzed by ash and N according to AOAC (1990) (methods numbers 942.05 and 984.13, respectively). NDF and ADF were measured sequentially (Van Soest et al., 1991; without sodium sulfite in the neutral detergent solution) using an ANKOM200 Fiber Analyzer (ANKOM Technology Corp., Fairport, NY, USA). NDF was assayed with a heat stable amylase. Fiber concentrations were expressed following a correction for ash.
Calculations
The intake rate was obtained by the ratio between daily dry matter intake and daily grazing time, and ruminating index by the ratio between daily ruminating time and daily NDF intake (Harumoto and Kato, 1979).
From the number of feeding stations and patches visited, and the number of steps between feeding stations/patches, the following variables were determined to describe the process of grazing in the paddock. Feeding station scale: (i) number of feeding stations per minute: given by the quotient between the total number of feeding stations and the duration, in minutes, of the visual test; (ii) steps between feeding stations: quotient between the total number of steps by the number of feeding stations visited during the evaluation; (iii) displacement rate, in steps per minute: total number of steps divided by the duration in minutes by the visual test; (iv) time per feeding station: quotient between the duration of the test in seconds and the total number of feeding stations visited. Patch scale: (v) feeding stations per patch: given by the quotient between the total number of feeding stations and the total number of patches visited during the visual test; (vi) patches visited per hour: quotient between the total number of patches and the duration, in hours, of the visual test; (vii) time per patch: quotient between the duration of the test in minutes and the total number of patches visited, and (viii) steps between patches: quotient between the total number of steps by the number of patches visited during the evaluation (Ruyle and Dwyer, 1985; Gonçalves et al., 2009; Gregorini et al., 2009b).
The milk yield was calculated as the average of all days in the paddocks. Energy corrected milk was calculated according to Tyrrell and Reid (1965):
Dry matter intake (kg DM/cow per day) was estimated from the ratio of n-alkane concentrations found naturally in the forage and dosed alkane (C31:C32) in forage and feces samples, according to the equation proposed by De-Stefani Aguiar et al. (2013):
The digestibility was calculated using the following equation (Dove and Mayes, 2006):
where fecal output is the total fecal output estimated using C36 alkane by this equation:
Statistical Analyses
Data were analyzed as a complete randomized block design using the SAS System program (SAS® University Edition, SAS Institute Inc., Cary, NC, USA). The paddock was considered the experimental unit for the sward variables, but for animal variables, the cows within each paddock were considered the experimental unit. Univariate analyses were performed on all variables to identify outliers and inconsistencies, and to verify the normality of residuals. Most of the variables were normally distributed, except feeding stations per minute (fitted with a Poisson distribution), steps between feeding stations, displacement rate, time per feeding station, feeding stations per patch, patches per hour, time per patch, steps between patches, and leaf sheath height which were fitted with Log-normal distribution. For both Poisson and Log-normal distributions, a log transformation was specified in a model.
Sward height depletion rate was analyzed using the NLIN procedure. The mean, median, mode, range, variance, and standard deviation of sward height as well as grazing and ruminating behavior were analyzed as repeated measures using the GLIMMIX procedure with a mixed model that included treatment, moment, and their interaction as fixed effects, and block as the random effect. To analyze first grazing meal length as well as displacement, feeding station, and patch use, the variables time of the day (morning and evening) and their interactions were added to the model as fixed effects. The dry matter intake, milk production, depletion rate, and herbage chemical composition variables were analyzed using the GLIMMIX procedure with a mixed model that included treatment as the fixed effect and block as the random effect. The proportion of sward height type and leaf sheath height variables were analyzed with a mixed model that included treatment, sward height type, and their interaction as fixed effects, and block as the random effect.
Least square means were separated using Tukey–Kramer tests and a significant difference was accepted if P < 0.05 and tendencies to significance were accepted if 0.05 < P ≤ 0.10.
Results
Herbage Mass, Height, and Depletion Dynamics
Seven days of grazing were needed on TC, and six on TM and TL treatment to achieve the targeted post-grazing sward height. No differences were found between treatments (P = 0.086) on pre-grazing herbage mass (2,700 ± 130 kg/ha), pre-grazing sward height (18.8 ± 0.8 cm), and post-grazing herbage mass (1,700 ± 110 kg/ha). Post-grazing sward height were 9.0 ± 0.23 cm, 12.0 ± 0.23 cm, and 13.5 ± 0.27 cm (P < 0.001) for target heights of 9, 12, and 15 cm, respectively.
The characterization of sward the day before grazing is presented in Table 1. The proportion of LH was similar between treatments, while MH was lower on TL and HH was greater on TL (interaction sward height type × treatment; P < 0.001). The leaf sheath height increased from LH to HH and from TC to TL treatment, presenting significance on all tested effects (P < 0.001).
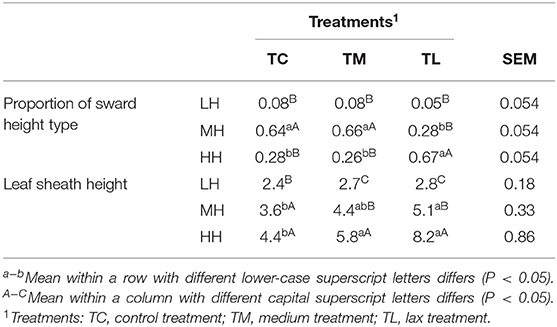
Table 1. Proportion of low height (LH), medium height (MH), and high height (HH) of sward and leaf sheath height (cm) 1 day before grazing according to the treatments.
The dynamics of sward height depletion throughout the grazing period is presented in Figure 1. An exponential decay was fitted for each treatment with a fractional rate of −0.052, −0.096, and −0.116 cm/day for TL, TM, and TC, respectively. The depletion rate was lower on TL than TM and TC (P < 0.001), with a trend (P < 0.055) between the last two.
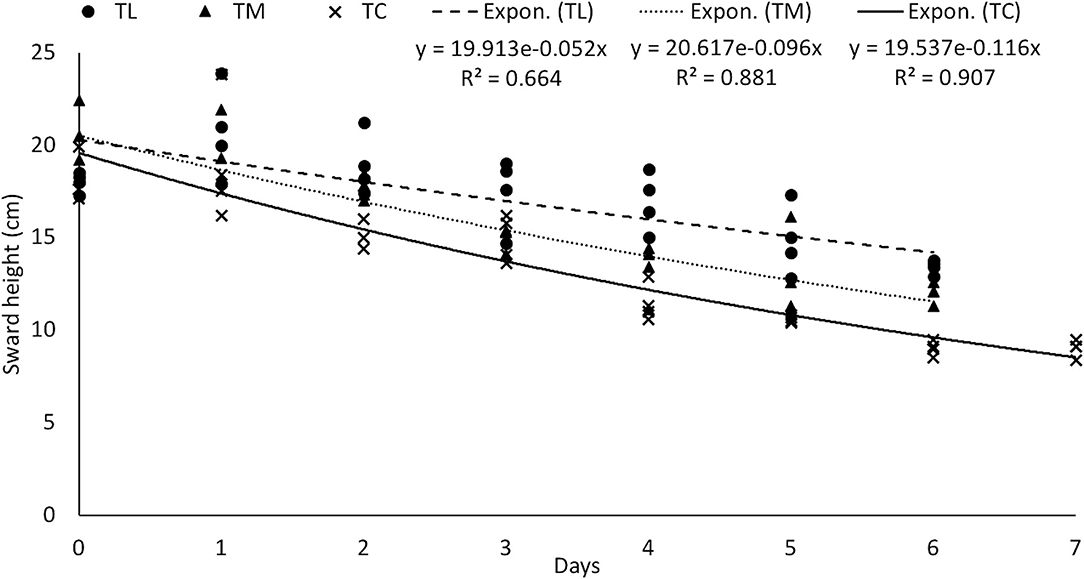
Figure 1. Depletion dynamics of height throughout the grazing down by cows to post-grazing sward heights of 9 (TC), 12 (TM), or 15 cm (TL). Fitted exponential equations for each treatment are shown within the figures under the series identification.
The sward height distribution during the 2 days of behavioral evaluation is presented in Table 2. All the variables presented were affected by treatment and moment. The sward height median and mode remained lower than the sward height mean on TC and TM and above on TL. The range, variance, and standard deviation increased from TC to TL and in general decreased from the beginning to the end of the occupation period.
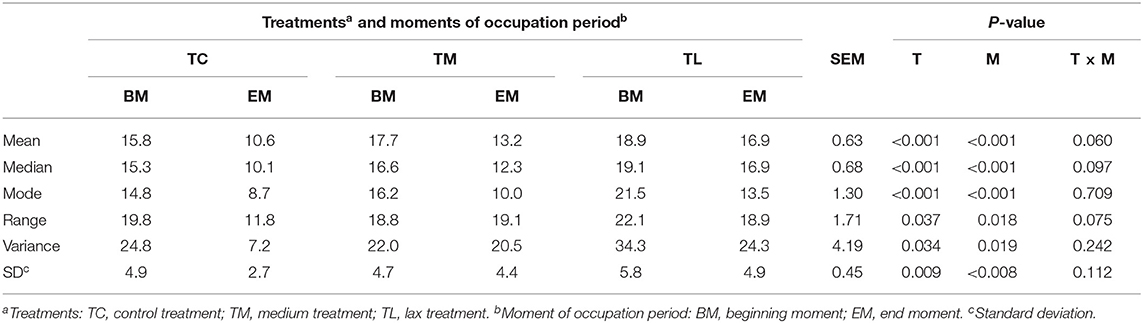
Table 2. Effect of post-grazing sward height (T) and moment of occupation period (M) on sward height distribution.
Ingestive and Ruminating Behavior
Daily grazing time was unaffected by treatment or moment, but there was an interaction (Table 3). The cows on TM spent more time grazing at the beginning than at the end of the occupation period (551 vs. 489 min of daily grazing), while TL tended to have a shorter grazing time than TM at the beginning moment (481 vs. 551 min of daily grazing). The morning's first grazing meal duration was greater at the end than at the beginning of the occupation period (169 vs. 147 min). TC and TM did not change the morning's first grazing meal duration between the beginning and the end moment of the occupation period while TL increased the duration at the end of the occupation period (58 vs. 126 min for the beginning and the end, respectively). The number of grazing meals was greater on TL than on TM, with no differences with TC. The number of grazing meals was greater at the beginning (6.3) than at the end moment (4.8) for TL. Moreover, at the beginning, TL cows grazed in 6.3 meals while both TM and TC did it in 4.6 meals. The mean grazing meal duration was greater on TM than on TL, and tended to be greater than TC (P = 0.090). The time spent ruminating was greater on TC and TL than on TM, and lower at the end than at the beginning (480 vs. 521 min). Nevertheless, the number of ruminating bouts was greater on TL and TM than on TC. On the other hand, the mean ruminating bout length was greater on TC than on the other ones.
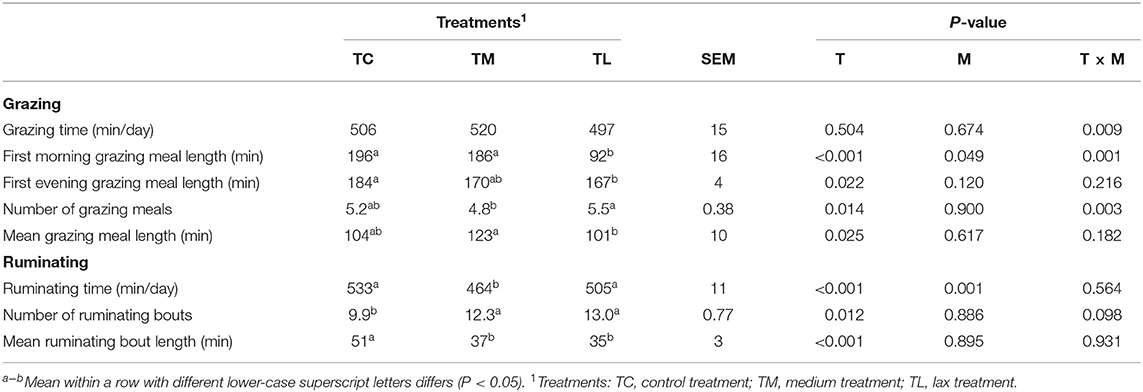
Table 3. Effect of post-grazing sward height (T) and moment of occupation period (M) on ingestive and ruminating behavior by lactating cows.
Displacement Pattern and Feeding Station and Patch Exploration
The displacement pattern, feeding station, and patch use are shown in Table 4. The number of feeding stations per minute was greater on TL than on TC, and both did not differ from TM. The cows explored a greater number of feeding stations at the beginning than at the end of the occupation period (4.8 ± 0.10 vs. 4.3 ± 0.10), as well as grazing in the evening than in the morning (4.7 ± 0.09 vs. 4.4 ± 0.09). The time that cows explored each feeding station was greater on TC and decreased at higher post-grazing sward height, which was affected by the moment of occupation period (12.8 ± 0.31 vs. 14.2 ± 0.35 for the beginning and the end, respectively) as well as the time of the day (14.0 ± 0.31 vs. 13.0 ± 0.30 for morning and evening grazing, respectively). Steps between feeding stations and displacement rates were not affected by treatment, the moment of the occupation period, or time of the day. The number of steps between feeding stations at morning grazing was higher on TC (0.36 ± 0.06) than on TL (0.21 ± 0.04). The cows grazing on TC, at the beginning of the occupation period, had more steps between feeding stations (0.44 ± 0.09 vs. 0.21 ± 0.04) and per minute (1.86 ± 0.39 vs. 0.91 ± 0.20) at morning grazing than in the evening. The treatment × moment interaction was not significant for all the variables tested.
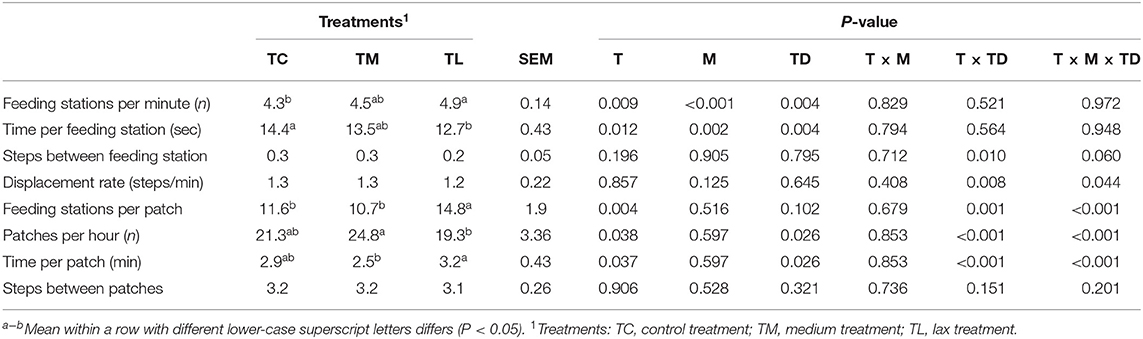
Table 4. Effect of post-grazing sward height (T), moment of occupation period (M), and time of the day (TD) on displacement pattern and exploration of feeding stations and patches by lactating cows.
The number of feeding stations per patch was lesser on TC and TM than on TL, and in morning grazing the difference between them was highlighted (10.7 ± 1.48 and 11.2 ± 1.55 vs. 18.2 ± 2.58 for TC, TM, and TL, respectively). At the beginning of the occupation period, TC increased the number of feeding stations explored from morning to evening grazing (8.3 ± 1.35 vs. 16.0 ± 2.68). The patches explored per hour were higher on TM than on TL, and in evening grazing (23.3 ± 2.99) than in the morning (20.1 ± 2.57). In the morning grazing sessions, TC and TM cows explored more patches per hour (23.4 ± 3.41 and 23.3 ± 3.4, respectively) than TL ones (15.0 ± 2.23), and fewer than in the evening grazing sessions (24.9 ± 3.7). At the beginning, TC explored double the patches per hour in morning grazing (31.1 ± 5.28) than in the evening (15.0 ± 2.58), which differed from TM (25.6 ± 4.51) at the same moment. On the other hand, TL cows at the end explored double the patches per hour in evening grazing (25.8 ± 4.48) than in the morning (13.3 ± 2.21). The residence time per patch was greater on TL than on TM without differing from TC; however, it had an interaction with the effect of the time of the day (3.0 ± 0.38 vs. 2.6 ± 0.33 for morning and evening grazing, respectively), and in morning grazing, the TC (2.6 ± 0.38) and TL (4.09 ± 0.6) differed from each other. At the beginning moment, the TC had a greater time per patch in the evening (4.1 ± 0.7) than in morning grazing (1.9 ± 0.33), while at the end, TL had a greater time per patch in morning grazing (4.7 ± 0.8 vs. 2.3 ± 0.41). The variable steps between patches was not significant for all the tested effects.
Dry Matter Intake, Ingestive Process, and Selected Diet
The dry matter intake was lesser on TC than on TM and TL. The intake rate was lesser on TC than on TL (Table 5). The rumination index was greater on TC than on TM and TL. The digestibility of the diet was lesser on TC and TM than on TL. The selected diet by cows presented lesser crude protein and neutral detergent fiber content on TC and TM than on TL. The acid detergent fiber tended (P = 0.066) to be greater on TC than on the other ones.
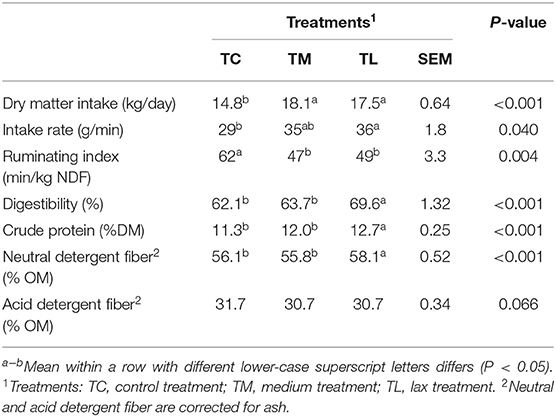
Table 5. Effect of post-grazing sward height on dry matter intake, ingestive process, and nutritional characteristics of selected diet by lactating cows.
Milk Production and Composition
Milk yield, energy corrected milk yield, fat, protein, and lactose yield increased with post-grazing sward height (Table 6). Fat, protein, and lactose content did not differ between treatments.
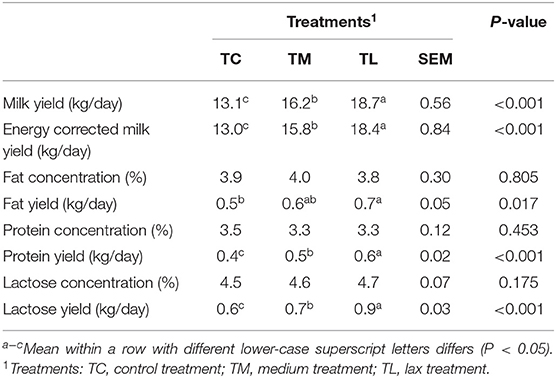
Table 6. Effect of post-grazing sward height on milk yield and milk component yields and concentration by lactating cows.
Discussion
We have found that the establishment and maintenance of treatments for 8 months before the period of measurement generated a different spatial arrangement of the height (proportion of HH, MH, and LH) and a distinctive canopy vertical structure in each treatment that affected the behavioral pattern of the animals. The grazing started with equal sward height and herbage mass between treatments, but the different depletion rate during grazing down resulted in a different post-grazing sward height, although no differences in herbage mass, probably because the different vertical structures of the canopy between treatments (sheath height) changed the density of the residual strata. Therefore, as suggested by Gibb et al. (1997), the productive and behavioral performance of the animals should be analyzed in a context of a complex environment, because mean values of biomass or sward surface height would be poor predictors of cattle foraging behavioral responses (Laca et al., 1992). For a better correlation of sward characteristics and animal behavior, an analysis of sward height distribution during the beginning and the end of the occupation period was performed. The heterogeneity increased from TC to TL, given that it had an increase in the range, variance, and standard deviation of sward height distribution. Although heterogeneity is a complex, rich, and multidimensional concept (Laca, 2008), we refer to it as the variation on sward surface height across the paddock and days (space and time scale). When a large variance is found, it means that both good and poor bites are relatively abundant; in the opposite, when a small variance is found, most of the bites are similar (Laca, 2008). The variance was almost double on TL than on TC (29.3 vs. 16.1) and, despite no effect of treatment by moment interaction, the variance decreased 71% from the beginning to the end of the occupation period on TC, whereas the same reduction was about 7% on TM and 29% on TL. Heterogeneity can affect intake through effects on bite formation and dimensions, as well as selectivity, which will be beneficial when searching costs do not increase because of it (Utsumi et al., 2009).
The daily grazing time was not affected by treatment, but was due to the interaction with the time of day and moment of the occupation period. It is argued that cows on each treatment adopted a different behavioral strategy during the grazing process as a result of the changing sward structure. The daily grazing time is defined by Gibb et al. (1998) as a sum of all grazing meals. At a post-grazing sward height of 12 cm (TM), the fact that cows increased grazing time by ≈20 min, although this was not statistically significant, is highlighted by the lower ruminating time found in this treatment when compared with TC and TL (69 and 41 min lower, respectively). The time spent on ruminating and grazing could have had a compensatory relationship, that is, when the grazing time is longer, the ruminating time tends to decrease (Dado and Allen, 1994), given that the total chewing time is less variable (Beauchemin, 2018). The number of grazing meals found in this study is in accordance with that expected for dairy cattle (Gregorini, 2012), presenting a maximum value on TL at the beginning moment (6.3), where forage was abundant. The length of each grazing meal is highly dependent on rumen fill (Chilibroste et al., 1997), although it has been related to a multi-factorial response (Gregorini, 2012; Chilibroste et al., 2015). Normally, between two consecutive grazing meals, the animal ruminates (Gregorini, 2012) to release soluble nutrients from feeds (Chilibroste et al., 2007). On TC, the ruminating bout length was greater than other treatments, and, according to Amaral et al. (2013), by decreasing post-grazing sward height the animal is forced to explore strata with a predominance of stems and senescent material, increasing the intervals between meals.
In the literature there is evidence that the dusk grazing event is the longest and most intense (Gibb et al., 1998; Gregorini, 2012; Chilibroste et al., 2015); however, only cows managed on TL in our experiment followed this grazing pattern. This is possibly due to the less favorable grazing conditions on both TC and TM, because the cows increased both morning and evening grazing meal length to compensate for low intake rates. On both TC and TM the compensation was limited by requirements of rumination and idling time (Chilibroste et al., 2015), but on TC the low intake rate and lower estimated digestibility of the diet resulted in a reduced dry matter intake and consequently milk production. Furthermore, sward height depletion has been associated with short-term behavior adaptations, such as bite mass and intake rate reductions (Gregorini et al., 2009a). On TM, the sward structure allowed a more efficient compensation mechanism, that resulted in intermediary milk production which was achieved through the same dry matter intake as TL from a diet with lower digestibility.
The chemical composition of sward changes through the day through photosynthates accumulation, with the maximum levels of dry matter and non-structural carbohydrate concentrations in the evening (Griggs et al., 2005) and in the upper layers of the sward (Delagarde et al., 2000). Therefore, analyzing the two most important grazing events (first morning and evening meal; Gibb et al., 1998), the TL cows grazed double in the evening compared to the morning, while on TC and TM grazing time was equal across the day. In addition, the higher intake rate achieved on TL occurred at the time when the nutritional value of the sward was highest (Abrahamse et al., 2008). The theoretically higher non-structural carbohydrate concentrations in the evening, associated with the highest crude protein level in herbage samples on TL led to higher dry matter intake and milk production. Controversially, the neutral detergent fiber and the diet digestibility was higher on TL. It is possible that a better match of nutrients along the day in the rumen of TL cows allowed a better diet digestibility despite the higher level of NDF. In other words, a higher crude protein and probably soluble carbohydrate intake added to shorter grazing meals followed by a ruminating session determined a better ruminal environment and thus a higher extent of fermentation. According to Filho et al. (2012), the pattern of ruminal fermentation clearly depends on changes in nutritional values that occur in different sward layers during grazing.
The information about feeding station exploration is widely variable between studies (Roguet et al., 1998b; Searle et al., 2005; Gregorini et al., 2007, 2009b, 2011; Gonçalves et al., 2009; Da Trindade et al., 2012; Amaral et al., 2013; Mezzalira et al., 2013, 2014), but there is a consensus that it is highly dependent of sward vertical structure and spatial distribution, herbage mass, and the internal state of the animals, resulting in a trade-off determining the behavior pattern adopted throughout the grazing down process within the day and between days.
The exploration of feeding stations enhanced the adaptation of the behavioral patterns of cows to adapt to different scenarios. According to Gregorini et al. (2007), feeding station behavior is partially controlled by ruminal fill and their interaction with external factors such as sward characteristics. The decision to move to a new feeding station will depend of the profitability of each bite (trade-off between the benefit and cost of moving on) which is highly connected to the animal perception of resource availability (Roguet et al., 1998a). We found that cows on TL explored a higher number of feeding stations but spent less time at each feeding station, probably because the benefit outweighed the cost of travel (Utsumi et al., 2009).
Utsumi et al. (2009) using artificial patches of fescue and lucerne found that the cows increased their patch residence time when the distance between patches was increased, leading to increased speed between patches. The authors suggest that the animals adopted a strategy linearly related to the maximization of intake rate. In this study, the cows on TC remained at each feeding station longer but took more steps between feeding stations than TL cows. According to Utsumi et al. (2009), foragers maximizing intake rate increase travel time between patches by increasing the residence time in each patch. We, however, did not find an increase in travel time between patches, but the residence time was lower on TM compared with TL, resulting in a greater number of patches explored by TM cows. On the other hand, the feeding stations explored per patch was greater on TL. These results suggest that TL achieved the selectivity at the feeding station and bite scale while TM cows did it at the patch scale. The patch can be defined as a spatial aggregation of feeding stations (and bites, consequently) over which intake or movement rate remains relatively constant (Bailey et al., 1996). Therefore, considering that the number of patches per hour was lower on TL than on TM, the TL cows had a more constant intake rate.
If departure decisions at patch scale are influenced by trade-offs between maximizing intake rate and food quality (Searle et al., 2005), we can think that TL patches were more attractive to the cows, because on this treatment the animals spent more time at each patch with greater diet digestibility. On the other hand, TM cows explored more patches achieving the same dry matter intake as TL cows, but the selected diet was inferior in terms of quality and, consequently, the milk production was lower as well.
A general relationship has been postulated that large herbivores tend to spend more time in feeding stations as forage biomass increases (Searle et al., 2005). However, in this case, the mean herbage mass did not differ between treatments, giving spatial arrangement and a distinctive canopy vertical structure. The cows on TL grazed more “high structures” (Giles et al., 2018) than on TM and on TC and increased the residence time per feeding station, conversely conforming to our hypothesis but still generating a greater nutrients intake. Similar results about structure selection were reported by Faber (2012) with the same pasture species. In a short-term study on natural grasslands, (Gonçalves et al. 2009) found the opposite. The animals increased the residence time and decreased the number of feeding stations visited when sward height increased. In accordance with our results, Mezzalira et al. (2013) observed that under low sward heights, animals explored more feeding stations, and they argue that probably grazing patterns were affected by spatial memory, giving that it was a large study (more than 4 months). On the same line, according to Roguet et al. (1998a) and Searle et al. (2005), grazing herbivores tend to utilize feeding stations in such a way as to remove more digestible forage before moving on; however, the animal can prioritize intake rate rather than selection when the transmission of signals to the brain in response to low amounts of digesta in the rumen occurs (Gregorini et al., 2009a). The latter probably occurred on TC and TM, but with a different trade-off, in response to the different canopy structures.
Studying the effect of manipulated ruminal fill on beef heifers, Gregorini et al. (2007) observed that the “hungrier” (fewer ruminal fill) the animals were, the greater the time invested in each feeding station was, and the faster they searched. This can help explain why TC cows explored for a longer time at each feeding station, since dry matter intake on this treatment remained below potential. This is in agreement with the assumption that cattle can reduce forage manipulation time during feeding to increase the intake rate (Parsons et al., 1994). On the opposite side, TL cows with a large bite mass (short-term measurement; Oborsky et al., 2019) and finally greater dry matter intake, probably spent more time manipulating each bite while searching for a new feeding station to explore. This leads us to believe that the difference in time spent ruminating per kg of NDF (15 min per kg of NDF less on TL than on TC) can be influenced by differences in processing during eating.
The results from the current study confirm our hypothesis that dry matter intake and milk production increases with a reduction in defoliation intensity through more selective ingestive behavior leading to a higher digestibility diet. Given that the first limitation on grazing animal performance is the feed intake (Holmes et al., 2002), the main reason the milk production increased from TC to TM and TL was the difference in the dry matter intake (3 kg of DM per cow per day). From TC to TM the dry matter intake increased 1 kg per extra cm in post-grazing sward height and milk yield response was 0.9 kg of energy corrected milk per extra cm in post-grazing sward height. The same milk yield response was found from TC to TL and from TM to TL. However, an increase on dry matter intake between TM and TL was not found, but it was on digestible dry matter intake, which was 1 kg lesser on TM than on TL. Ganche et al. (2013) contrasting 2.7 vs. 3.5 cm of post-grazing sward height, with supplemented cows, found a 30% increase on grass digestible organic matter intake, while in this study we found an increase of 23, 35, and 10% for TC vs. TM, TC vs. TL, and TM vs. TL, respectively on digestible dry matter intake. McEvoy et al. (2008), comparing 3.8 vs. 4.6 cm of post-grazing sward height reported a higher milk yield response (2.1 kg of milk per extra cm in post-grazing sward height) than we found. A similar milk yield response was reported by Delaby et al. (2001; 0.8 kg of milk per extra cm in compressed plate meter post-grazing sward height; 5.7 vs. 6.8 cm). The increase in milk production was related to a higher energy supply from an increasing digestible dry matter intake.
The management of the defoliation intensity determined different levels of heterogeneity in the pasture, resulting in the adoption of different behavior patterns of the animals at different spatio-temporal scales. This indicates that animal behavior is the basis of knowledge required for the decision-making process based on animal and pasture monitoring. The integration of the different behavioral scales evaluated in this experiment (feeding station, patch, grazing and ruminating session, and daily grazing and ruminating time) allowed us to understand the how and why of cow's grazing behavior.
The behavior data must be hierarchically integrated to gain a better understanding of the processes and allow the development of decision-making support models. In this sense, technological innovations that allow real-time monitoring of the animals in their pastoral environment should be used to capitalize the animal and pasture heterogeneity more than trying to homogenize it.
The intensity of defoliation modulates the animal-plant interaction and constitutes a valuable management tool that can be used to boost forage intake and milk production. The new developments on information and communication technology would allow for linking behavioral data with response variables (e.g., milk production, health, welfare, etc.) at different spatio-temporal scales and support either short (day-to-day) or long (months to years) term management decisions.
Conclusions
The height and structure of the pasture on control treatment resulted in lower intake rate with less selectivity as cows' strategy to compensate for the lower sward height. In the medium treatment, cows adopted a behavioral strategy different from the others, achieving the same dry matter intake than TL cows but without increases in milk production. The lax management of the grass resulted in a more digestible dry matter intake and therefore higher milk production, achieved through behavioral adaptations throughout the grazing process expressing higher selectivity. The cows' response to changes in mean residual sward height and their associated heterogeneity is not the adoption of a uniformed pattern since we observed changes throughout the occupation period and throughout the day. The dairy grazing cow has great capacity to adapt its behavior to different scenarios and does it at different scales of time and space.
Data Availability Statement
The original contributions presented in the study are included in the article, further inquiries can be directed to the corresponding author.
Ethics Statement
The animal study was reviewed and approved by Animal Experimentation Committee of the University of Uruguay, application number 02174500019718 (Montevideo, Uruguay).
Author Contributions
GM participated in experiment design, performed the field experiment, analyzed the results, promoted data analysis discussions, wrote the original draft, and revised versions of the manuscript. PG conceptualized and designed the field experiment, performed the field experiment, participated in data analysis discussions, and reviewed and edited the manuscript. MO participated in experiment design, performed the field experiment, and participated in data analysis discussions. OF participated in data analysis discussions. DM conceived, designed, supervised field experiments, and participated in data analysis discussions. TG designed and supervised field experiments, alkane lab analysis, and participated in data analysis discussions. PC conceived, designed, obtained funding, administrated the project, supervised field experiments, results analyses, and participated in data analysis discussions. All authors reviewed the manuscript and approved the final version of the manuscript.
Conflict of Interest
The authors declare that the research was conducted in the absence of any commercial or financial relationships that could be construed as a potential conflict of interest.
Publisher's Note
All claims expressed in this article are solely those of the authors and do not necessarily represent those of their affiliated organizations, or those of the publisher, the editors and the reviewers. Any product that may be evaluated in this article, or claim that may be made by its manufacturer, is not guaranteed or endorsed by the publisher.
Acknowledgments
The authors thank the Agencia Nacional de Investigación e Innovación (ANII) and Red Tecnológica Sectorial de Lechería (RTS) for supporting the project (ANII-RTS_X_2014_1_3) and the Comisión Académica de Posgrado (CAP–Udelar) for funding the post-graduate scholarship awarded to GM (BFPM_2019_1#63324394).
References
Abrahamse, P. A., Dijkstra, J., Vlaeminck, B., and Tamminga, S. (2008). Frequent allocation of rotationally grazed dairy cows changes grazing behavior and improves productivity. J. Dairy Sci. 91, 2033–2045. doi: 10.3168/jds.2007-0579
Aguirre-Villegas, H. A., Passos-Fonseca, T. H., Reinemann, D. J., and Larson, R. (2017). Grazing intensity affects the environmental impact of dairy systems. J. Dairy Sci. 100, 6804–6821. doi: 10.3168/jds.2016-12325
Amaral, M. F., Mezzalira, J. C., Bremm, C., Da Trindade, J. K., Gibb, M. J., Suñe, R. W. M., et al. (2013). Sward structure management for a maximum short-term intake rate in annual ryegrass. Grass Forage Sci. 68, 271–277. doi: 10.1111/j.1365-2494.2012.00898.x
AOAC (1990). Official Methods of Analysis, 15th Edn. Arlington, VA: Association of Official Analytical Chemists.
Bailey, D. W., Gross, J. E., Laca, E. A., Rittenhouse, L. R., Coughenour, M. B., Swift, D. M., et al. (1996). Mechanisms that result in large herbivore grazing distribution patterns. J. Range Manag. 49:386. doi: 10.2307/4002919
Barthram, G. T. (1986). “Experimental techniques: the HFRO sward stick,” in Biennial Report of the Hill Farming Research Organization, ed M. M. Alcock (Midlothian: HFRO), 29–30.
Beauchemin, K. A. (2018). Invited review: current perspectives on eating and rumination activity in dairy cows. J. Dairy Sci. 101, 4762–4784. doi: 10.3168/jds.2017-13706
Cardoso, C. S., von Keyserlingk, M. A. G., and Hötzel, M. J. (2019). Views of dairy farmers, agricultural advisors, and lay citizens on the ideal dairy farm. J. Dairy Sci. 102, 1811–1821. doi: 10.3168/jds.2018-14688
Carvalho, P. C. F., and Moraes, A. (2005). “Comportamento ingestivo de Ruminantes: bases para o manejo sustentável do pasto,” in Manejo Sustentável em Pastagem, eds U. Cecato, and C. C. Jobim (Maringá-PR: UEM), 1–20.
Chapman, D. F. (2016). Using ecophysiology to improve farm efficiency: application in temperate dairy grazing systems. Agriculture 6:17. doi: 10.3390/agriculture6020017
Chapman, D. F., Parsons, A. J., Cosgrove, G. P., Barker, D. J., Marotti, D. M., Venning, K. J., et al. (2007). Impacts of spatial patterns in pasture on animal grazing behavior, intake, and performance. Crop Sci. 47, 399–415. doi: 10.2135/cropsci2006.01.0036
Chilibroste, P., Gibb, M., and Tamminga, S. (2005). “Pasture characteristics and animal performance,” in Quantitative Aspects of Ruminant Digestion and Metabolism, eds J. Dijkstra, J. Forbes, and J. France (Wallingford: CAB International), 681–706. doi: 10.1079/9780851998145.0681
Chilibroste, P., Gibb, M. J., Soca, P., and Mattiauda, D. A. (2015). Behavioural adaptation of grazing dairy cows to changes in feeding management: do they follow a predictable pattern? Anim. Prod. Sci. 55, 328–338. doi: 10.1071/AN14484
Chilibroste, P., Soca, P., Mattiauda, D. A., Bentancur, O., and Robinson, P. H. (2007). Short term fasting as a tool to design effective grazing strategies for lactating dairy cattle: a review. Aust. J. Exp. Agric. 47:1075. doi: 10.1071/EA06130
Chilibroste, P., Tamminga, S., and Boer, H. (1997). Effects of length of grazing session, rumen fill and starvation time before grazing on dry-matter intake, ingestive behaviour and dry-matter rumen pool sizes of grazing lactating dairy cows. Grass Forage Sci. 52, 249–257. doi: 10.1111/j.1365-2494.1997.tb02355.x
Coates, D. B., and Penning, P. (2000). “Measuring animal performance,” in Field and Laboratory Methods for Grassland and Animal Production research, eds L. 't Mannetje, and R. M. Jones (Wallingford: CABI), 353–402. doi: 10.1079/9780851993515.0353
Da Trindade, J. K., Pinto, C. E., Neves, F. P., Mezzalira, J. C., Bremm, C., Genro, T. C. M., et al. (2012). Forage allowance as a target of grazing management: implications on grazing time and forage searching. Rangel. Ecol. Manag. 65, 382–393. doi: 10.2111/REM-D-11-00204.1
Dado, R. G., and Allen, M. S. (1994). Variation in and relationships among feeding, chewing, and drinking variables for lactating dairy cows. J. Dairy Sci. 77, 132–144. doi: 10.3168/jds.S0022-0302(94)76936-8
Delaby, L., Peyraud, J. L., and Delagarde, R. (2001). Effect of the level of concentrate supplementation, herbage allowance and milk yield at turn-out on the performance of dairy cows in mid lactation at grazing. Anim. Sci. 73, 171–181. doi: 10.1017/S1357729800058161
Delagarde, R., Peyraud, J. L., Delaby, L., and Faverdin, P. (2000). Vertical distribution of biomass, chemical composition and pepsin-cellulase digestibility in a perennial ryegrass sward: interaction with month of year, regrowth age and time of day. Anim. Feed Sci. Tech. 84, 49–68. doi: 10.1016/S0377-8401(00)00114-0
De-Stefani Aguiar, A., Forbes, T. D. A., Rouquette, F. M., Tedeschi, L. O., and Randel, R. D. (2013). Evaluating the statistical variation in estimating forage dry matter intake of grazing Brahman bulls using n-alkanes. J. Agric. Sci. 151, 129–140. doi: 10.1017/S0021859612000354
Dove, H., and Mayes, R. W. (2006). Protocol for the analysis of n-alkanes and other plant-wax compounds and for their use as markers for quantifying the nutrient supply of large mammalian herbivores. Nat. Protoc. 1, 1680–1697. doi: 10.1038/nprot.2006.225
Faber, A. C. (2012). Estructura espacial y selectivadad de parches en pasturas de festuca alta pastoreadas a diferente altura remanente (MSc. thesis), Universidad de la República, Montevideo, Uruguay.
Fariña, S. R., and Chilibroste, P. (2019). Opportunities and challenges for the growth of milk production from pasture: the case of farm systems in Uruguay. Agric. Syst. 176:102631. doi: 10.1016/j.agsy.2019.05.001
Filho, H. M. N. R., Peyraud, J. L., and Delagarde, R. (2012). Foraging behavior and ruminal fermentation of dairy cows grazing ryegrass pasture alone or with white clover. Pesqui. Agropecu. Bras. 47, 458–465. doi: 10.1590/S0100-204X2012000300019
Finneran, E., Crosson, P., O'Kiely, P., Shalloo, L., Forristal, D., and Wallace, M. (2010). Simulation modelling of the cost of producing and utilising feeds for ruminants on Irish farms. J. farm Manag. 14, 95–116.
Fulkerson, W. J., and Donaghy, D. J. (2001). Plant-soluble carbohydrate reserves and senescence-key criteria for developing an effective grazing management system for ryegrass-based pastures: a review. Aust. J. Exp. Agric. 41, 261–275. doi: 10.1071/EA00062
Ganche, E., Delaby, L., O'Donovan, M., Boland, T. M., and Kennedy, E. (2013). Direct and carryover effect of post-grazing sward height on total lactation dairy cow performance. Animal 7, 1390–1400. doi: 10.1017/S1751731113000451
Gibb, M. J., Huckle, C. A., and Nuthall, R. (1998). Effect of time of day on grazing behaviour by lactating dairy cows. Grass Forage Sci. 53, 41–46. doi: 10.1046/j.1365-2494.1998.00102.x
Gibb, M. J., Huckle, C. A., Nuthall, R., and Rook, A. J. (1997). Effect of sward surface height on intake and grazing behaviour by lactating Holstein Friesian cows. Grass Forage Sci. 52, 309–321. doi: 10.1111/j.1365-2494.1997.tb02361.x
Gibb, M. J., Huckle, C. A., Nuthall, R., and Rook, A. J. (1999). The effect of physiological state (lactating or dry) and sward surface height on grazing behaviour and intake by dairy cows. Appl. Anim. Behav. Sci. 63, 269–287. doi: 10.1016/S0168-1591(99)00014-3
Giles, P. Y., Oborsky, M., Menegazzi, G., Genro, T. C. M., Soca, P., Mattiauda, D. A., et al. (2018). “Proporción de parches de pastoreo y su efecto en el comportamiento animal según la intensidad de defoliación,” in 41o Congreso Argentino de Producción Animal, ed Asociación Argentina de Producción Animal (Mar del Plata: Revista Argentina de Producción Animal), 181.
Gonçalves, E. N., Carvalho, P. C., de, F., Devincenzi, T., Lopes, M. L. T., Freitas, F. K., and de Jacques, A. V. Á. (2009). Plant-animal relationships in a heterogeneous pastoral environment: displacement patterns and feeding station use. Rev. Bras. Zootec. 38, 2121–2126. doi: 10.1590/S1516-35982009001100008
Gregorini, P. (2012). Diurnal grazing pattern: its physiological basis and strategic management. Anim. Prod. Sci. 52:416. doi: 10.1071/AN11250
Gregorini, P., Gunter, S. A., Beck, P. A., Caldwell, J., Bowman, M. T., and Coblentz, W. K. (2009a). Short-term foraging dynamics of cattle grazing swards with different canopy structures. J. Anim. Sci. 87, 3817–3824. doi: 10.2527/jas.2009-2094
Gregorini, P., Gunter, S. A., Bowman, M. T., Caldwell, J. D., Masino, C. A., Coblentz, W. K., et al. (2011). Effect of herbage depletion on short-term foraging dynamics and diet quality of steers grazing wheat pastures. J. Anim. Sci. 89, 3824–3830. doi: 10.2527/jas.2010-3725
Gregorini, P., Gunter, S. A., Masino, C. A., and Beck, P. A. (2007). Effects of ruminal fill on short-term herbage intake rate and grazing dynamics of beef heifers. Grass Forage Sci. 62, 346–354. doi: 10.1111/j.1365-2494.2007.00589.x
Gregorini, P., Soder, K. J., and Kensinger, R. S. (2009b). Effects of rumen fill on short-term ingestive behavior and circulating concentrations of ghrelin, insulin, and glucose of dairy cows foraging vegetative micro-swards. J. Dairy Sci. 92, 2095–2105. doi: 10.3168/jds.2008-1803
Griffiths, W. M., Hodgson, J., and Arnold, G. C. (2003). The influence of sward canopy structure on foraging decisions by grazing cattle. I. Patch selection. Grass Forage Sci. 58, 112–124. doi: 10.1046/j.1365-2494.2003.00360.x
Griggs, T. C., MacAdam, J. W., Mayland, H. F., and Burns, J. C. (2005). Non-structural carbohydrate and digestibility patterns in orchardgrass swards during daily defoliation sequences initiated in evening and morning. Crop Sci. 45, 1295–1304. doi: 10.2135/cropsci2003.0613
Harumoto, T., and Kato, M. (1979). Use of ruminating time as an index of the herbage intake by grazing animals. J. Japanese Soc. Grassl. Sci. 24, 232–238.
Haydock, K. P., and Shaw, N. H. H. (1975). The Comparative yield method for estimating dry matter yield of pasture.\rMétodo de rendimiento comparativo. Aust. J. Exp. Agric. Anim. Husb. 15, 663–670. doi: 10.1071/EA9750663c
Holmes, C. W., Brookes, I. M., Garrick, D. J., MacKenzie, D. D. S., Parkinson, T. J., and Wilson, G. F. (2002). “Principles of nutrition and feeding,” in Milk Production from Pasture: Principles and Practices, ed D. Swain (Palmerston North: Massey University), 209–313.
Jáuregui, J. M., Michelini, D. F., Agnusdei, M. G., Baudracco, J., Sevilla, G. H., Chilibroste, P., et al. (2017). Persistence of tall fescue in a subtropical environment: tiller survival over summer in response to flowering control and nitrogen supply. Grass Forage Sci. 72, 454–466. doi: 10.1111/gfs.12252
Laca, E. A. (2008). “Foraging in a heterogeneous environment: intake and diet selection,” in Resource Ecology: Spatial and Temporal Dynamics of Foraging, eds H. H. T. Prins, and F. van Langeveld (Dordrecht: Springer), 81–100. doi: 10.1007/978-1-4020-6850-8_8
Laca, E. A., Ungar, E. D., Seligman, N., and Demment, M. W. (1992). Effects of sward height and bulk density on bite dimensions of cattle grazing homogeneous swards. Grass Forage Sci. 47, 91–102. doi: 10.1111/j.1365-2494.1992.tb02251.x
Linnane, M. I., Brereton, A. J., and Giller, P. S. (2001). Seasonal changes in circadian grazing patterns of Kerry cows (Bos Taurus) in semi-feral conditions in Killarney National Park, Co. Kerry, Ireland. Appl. Anim. Behav. Sci. 71, 277–292. doi: 10.1016/S0168-1591(00)00188-X
Mattiauda, D. A., Chilibroste, P., Bentancur, O., and Soca, P. (2009). “Intensidad de pastoreo y utilización de pasturas perennes en sistemas de producción de leche: ‘qué niveles de producción permite y que problemas contribuye a solucionar?” in XXXVII Jornadas Uruguayas de Buiatría, ed Centro Médico Veterinario de Paysandú (Paysandú: CENTRAL DE IMPRESIONES Ltda), 96–110.
Mattiauda, D. A., Tamminga, S., Gibb, M. J., Soca, P., Bentancur, O., and Chilibroste, P. (2013). Restricting access time at pasture and time of grazing allocation for Holstein dairy cows: ingestive behaviour, dry matter intake and milk production. Livest. Sci. 152, 53–62. doi: 10.1016/j.livsci.2012.12.010
McEvoy, M., Kennedy, E., Murphy, J. P., Boland, T. M., Delaby, L., and O'Donovan, M. (2008). The effect of herbage allowance and concentrate supplementation on milk production performance and dry matter intake of spring-calving dairy cows in early lactation. J. Dairy Sci. 91, 1258–1269. doi: 10.3168/jds.2007-0710
Mezzalira, J., Bremm, C., Trindade, J. K. D., Gonda, H. L., Vieira, P. C., and Carvalho, P. C. D. F. (2013). Ingestive behaviour from the feeding station to patch level in heterogeneous environments. J. Anim. Sci. Adv. 3, 613–623.
Mezzalira, J. C., De Faccio Carvalho, P. C., Fonseca, L., Bremm, C., Cangiano, C., Gonda, H. L., et al. (2014). Behavioural mechanisms of intake rate by heifers grazing swards of contrasting structures. Appl. Anim. Behav. Sci. 153, 1–9. doi: 10.1016/j.applanim.2013.12.014
Oborsky, M., Menegazzi, G., Giles, P. Y., Trujillo, A. I., Mattiauda, D. A., and Chilibroste, P. (2019). “Tasa de consumo y patrón de ingestión de vacas Holando pastoreando festuca a diferentes intensidades de defoliación,” in 42o Congreso Argentino de Producción Animal, ed Asociación Argentina de Producción Animal (Bahia Blanca: Revista Argentina de Producción Animal), 27–67.
O'Brien, D., Hennessy, T., Moran, B., and Shalloo, L. (2015). Relating the carbon footprint of milk from Irish dairy farms to economic performance. J. Dairy Sci. 98, 7394–7407. doi: 10.3168/jds.2014-9222
Parsons, A. J., Newman, J. A., Penning, P. D., Harvey, A., and Orr, R. J. (1994). Diet preference of sheep: effects of recent diet, physiological state and species abundance. J. Anim. Ecol. 63:465. doi: 10.2307/5563
Roguet, C., Dumont, B., and Prache, S. (1998a). Selection and use of feeding sites and feeding stations by herbivores: a review. Anim. Res. 47, 225–244. doi: 10.1051/animres:19980401
Roguet, C., Prache, S., and Petit, M. (1998b). Feeding station behaviour of ewes in response to forage availability and sward phenological stage. Appl. Anim. Behav. Sci. 56, 187–201. doi: 10.1016/S0168-1591(97)00104-4
Rutter, S. M. (2000). Graze: a program to analyze recordings of the jaw movements of ruminants. Behav. Res. Methods Instr. Comput. 32, 86–92. doi: 10.3758/BF03200791
Rutter, S. M., Champion, R. A., and Penning, P. D. (1997). An automatic system to record foraging behaviour in free-ranging ruminants. Appl. Anim. Behav. Sci. 54, 185–195. doi: 10.1016/S0168-1591(96)01191-4
Ruyle, G. B., and Dwyer, D. D. (1985). Feeding stations of sheep as an indicator of diminished forage supply. J. Anim. Sci. 61, 349–353. doi: 10.2527/jas1985.612349x
Savian, J. V., Neto, A. B., de David, D. B., Bremm, C., Schons, R. M. T., Genro, T. C. M., et al. (2014). Grazing intensity and stocking methods on animal production and methane emission by grazing sheep: implications for integrated crop-livestock system. Agric. Ecosyst. Environ. 190, 112–119. doi: 10.1016/j.agee.2014.02.008
Searle, K. R., Hobbs, N. T., and Shipley, L. A. (2005). Should I stay or should I go? Patch departure decisions by herbivores at multiple scales. Oikos 111, 417–424. doi: 10.1111/j.0030-1299.2005.13918.x
Tyrrell, H. F., and Reid, J. T. (1965). Prediction of the energy value of cow's milk. J. Dairy Sci. 48, 1215–1223. doi: 10.3168/jds.S0022-0302(65)88430-2
Utsumi, S. A., Cangiano, C. A., RGalli, J., McEachern, M., Demment, M. W., and Laca, E. (2009). Resource heterogeneity and foraging behaviour of cattle across spatial scales. BMC Ecol. 9:9. doi: 10.1186/1472-6785-9-9
Van Soest, P. J., Robertson, J. B., and Lewis, B. A. (1991). Methods for dietary fiber, neutral detergent fiber, and non-starch polysaccharides in relation to animal nutrition. J. Dairy Sci. 74, 3583–3597. doi: 10.3168/jds.S0022-0302(91)78551-2
Keywords: dairy cow, grazing behavior, defoliation intensity, heterogeneity, foraging scale
Citation: Menegazzi G, Giles PY, Oborsky M, Fast O, Mattiauda DA, Genro TCM and Chilibroste P (2021) Effect of Post-grazing Sward Height on Ingestive Behavior, Dry Matter Intake, and Milk Production of Holstein Dairy Cows. Front. Anim. Sci. 2:742685. doi: 10.3389/fanim.2021.742685
Received: 16 July 2021; Accepted: 04 October 2021;
Published: 04 November 2021.
Edited by:
Oleksiy Guzhva, Swedish University of Agricultural Sciences, SwedenReviewed by:
Bill Wales, Agriculture Victoria Ellinbank, AustraliaCarlos Gomez, National Agrarian University, Peru
Copyright © 2021 Menegazzi, Giles, Oborsky, Fast, Mattiauda, Genro and Chilibroste. This is an open-access article distributed under the terms of the Creative Commons Attribution License (CC BY). The use, distribution or reproduction in other forums is permitted, provided the original author(s) and the copyright owner(s) are credited and that the original publication in this journal is cited, in accordance with accepted academic practice. No use, distribution or reproduction is permitted which does not comply with these terms.
*Correspondence: Gabriel Menegazzi, Z21lbmVnYXp6aUBmYWdyby5lZHUudXk=