- 1Prairie Research Unit, Mississippi State University, Prairie, MS, United States
- 2Department of Animal Science, Texas A&M University, College Station, TX, United States
- 3Range Cattle Research and Education Center, University of Florida, Gainesville, FL, United States
Effective early postnatal nutritional management is a crucial component of livestock production systems, and nutrient manipulation during this period has been shown to exert long-term consequences on beef cattle growth and physiology. Metabolic imprinting defines these biological responses to a nutritional intervention early in life that permanently alter physiological outcomes later in life. Early weaning has been used to study metabolic imprinting effects, given that it allows for nutritional manipulation of animals at a young age. This practice has been shown to enhance carcass characteristics in feedlot cattle and accelerate reproductive development of females. Another strategy to study the effects of metabolic imprinting without the need for early weaning is to provide supplements via creep feeding. Providing creep feed to nursing cattle has resulted in transient and long-term alterations in cattle metabolism, contributing to increased reproductive performance of developing heifers and enhanced carcass quality of feeder cattle. Collectively, results described herein demonstrate nutrient manipulation during early postnatal life exerts long-term consequences on beef cattle productivity and may be a strategy to optimize production efficiency in beef cattle systems.
Introduction
Food production will need to increase considerably in the next decades to meet the demand of a growing population [Food and Agricultural Organization (FAO), 2009]. As such, beef cattle are an integral component of achieving food security, and development of efficient and sustainable management strategies are fundamental to reach a level of animal production that can provide for the population by 2050 [Food and Agricultural Organization (FAO), 2009]. The success of each cow-calf operation depends upon proper establishment and maintenance of optimal developmental trajectory of the offspring (Bartol et al., 2013). Producers are also challenged to improve growth, efficiency, carcass weight and quality (Robinson et al., 2013); traits that benefit carcass value (USDA, 1997) and beef palatability. Moreover, efficient reproductive outcomes are essential for successful production systems, of which development of replacement beef heifers are a vital component. The embryonic, fetal, and neonatal periods are the stages of life in which most developmental processes occur, and manipulating nutritional status during these critical periods presents an opportunity to modulate future growth and performance trajectory (Fall, 2011; Koletzko et al., 2012). Therefore, understanding and exploiting metabolic cues responsible for programming offspring growth, health, and performance is warranted.
Developmental programming, also referred to as fetal programming, is the concept that alterations in nutrient or endocrine status during critical prenatal development stages may result in adaptations that permanently alter the trajectory of growth, physiology, and metabolism of the offspring (Barker and Clark, 1997; Wu et al., 2006). However, organ development and tissue differentiation are not complete at birth in most mammals and these processes extend into the early postnatal period. Several regulatory mechanisms and biological processes are also functionally immature at birth and continue development postnatally (Srinivasan and Patel, 2008). In fact, young animals can respond to environmental stimuli that are foreign to their normal development through adaptations at the cellular, biochemical, and molecular levels (Patel and Srinivasan, 2011). The ability of organisms to diverge from a developmental program leads to alternate developmental trajectories and therefore divergent phenotypes, metabolism, and physiology (Bateson et al., 2004). The potential for environmental stimuli to induce these changes tends to be greatest during early in life, when cells and tissues are still differentiating (Hochberg et al., 2011). The term metabolic imprinting defines these biological responses to a nutritional intervention occurring during a limited period of susceptibility in early postnatal life, which permanently alters physiological outcomes later in life (Patel and Srinivasan, 2011). Research described herein focuses on interventions applied before the animal reaches 9 months of age. This concept has substantial implications for animal agriculture, and nutritional strategies targeted at these critical periods of development should be explored to optimize production efficiency and profitability in beef cattle systems.
Potential Mechanisms of Developmental Programming
Nutrient supply may permanently affect an organism's structure or function of tissues through a variety of mechanisms, such as induced variations in organ structure, altering cell number or rate of proliferation, clonal selection, and epigenetics (Lucas, 1991). Modifications in organ vascularization due to altered nutrient supply during organogenesis may affect the ability of cells to generate and respond properly to stimuli including circulating nutrients or hormonal signals (Waterland and Garza, 1999). Therefore, local concentrations of nutrients and metabolites present during organogenesis may result in morphologic alterations, permanently affecting body function. Rates of cellular proliferation and growth has been shown to be tissue-specific, indicating that different tissues have diverse and limited periods of hyperplastic and hypertrophic growth (Winick and Noble, 1966; MacInstosh et al., 2006). Given that cell growth is dependent on nutrient supply, nutrient deprivation or excess during essential periods of proliferation may affect rate of cell division, leading to permanent changes in cell number. For example, maternal nutrient restriction results in a reduction in the total number of secondary muscle fibers (Zhu et al., 2004), whereas maternal overnutrition results in increased number and size of adipocytes in postnatal skeletal muscle (Yan et al., 2013). The process of myogenesis occurs in utero, and postnatal muscle growth is dictated by an increase in muscle fiber size, absent of formation of new muscle fibers (Stickland, 1978). Hence, nutrient supply during critical periods of development exerts profound effects on muscle growth and development during postnatal life (Neibergs and Johnson, 2012).
Proliferation of cells within all organs involves the initial multiplication of a finite population of founder cells. As this process un-folds, early genetic and epigenetic modifications occur within individual cells, distinguishing them from subpopulations of rapidly dividing cells (Waterland and Garza, 1999). However, nutrients such as vitamins and minerals are vital components of enzymes and proteins involved in DNA synthesis, repair, and maintenance of genome integrity (Neibergs and Johnson, 2012). Therefore, altered nutrient supply may result in incorrect base pairing during replication and subsequent effects on cellular metabolism which will be inherited by daughter cells (Waterland and Garza, 1999; Fenech, 2010). These differences among proliferating cells are the basis for clonal selection, resulting in similar populations of rapidly dividing cells that develop distinct metabolic characteristics resulting from diverse microenvironmental conditions (Waterland and Garza, 1999). The phenomenon of metabolic imprinting can also occur through epigenetics referring to alterations in gene expression due to changes in chromatin structure (Wu et al., 2006). Similar to the epigenetic alterations associated with fetal programming, metabolic imprinting also results in postnatal silencing or activation of genes occurring independent of changes in DNA sequence (Lucas, 1998; Funston and Summers, 2013). These changes can pass from one cell generation to another via mitotic inheritance, as well as from one generation of a species to the next via meiotic inheritance (Ling and Groop, 2009). More specifically, epigenetic mechanisms elicited by dietary factors include DNA methylation, histone modifications, and non-coding microRNA (Canani et al., 2011). As examples, dietary restriction of folate, vitamin B12, and methionine, which serve as methyl donor molecules, in the diet of the gestating ewe resulted in widespread alterations in DNA methylation patterns and impaired immune function in the offspring (Sinclair et al., 2007). Dietary restriction of protein in maternal diets results in reduced DNA methylation and histone modification in offspring, persisting into adulthood (Lillycrop et al., 2007, 2008).
Evidence of Metabolic Imprinting in Cattle
Evidence of metabolic imprinting has been well-established in multiple species including mice, humans, pigs, and dairy cattle. Postnatal nutrient restriction impairs skeletal muscle growth and delays muscle fiber maturation in piglets (Hu et al., 2020), whereas newborn rodents reared on high carbohydrate formulas display altered expression of hypothalamic genes that regulate appetite later in life, leading to persistent body weight gain and intake (Srinivasan et al., 2008). In humans, increased energy intake at four months of age lead to greater weight gain and body mass index later in childhood (Ong et al., 2006). Additionally, research in dairy cattle shows enhancing nutrient intake of calves during the pre-weaning phase increased milk production during their first lactation compared to calves that were limit fed (Soberon and Van Amburgh, 2013).
Metabolic Imprinting in Early-Weaned Beef Calves
In beef cattle research, early-weaning (EW) is defined as separating calves from their dams prior to 180 days of age (Rasby, 2007), and has been used to study the effects of metabolic imprinting, given that it allows for nutritional manipulation of animals at a young age. This management practice is usually applied during periods of forage shortage to alleviate the amount of nutrients required by postpartum cows to support lactation (Arthington and Minton, 2004). This decrease in nutritional requirements combined with the removal of the negative feedback of calf suckling leads to improved cow reproductive efficiency, particularly for first and second parity females (Arthington and Kalmbacher, 2003; Arthington and Minton, 2004). Calves older than 60–90 days of age can acquire most of their nutrient requirements from solid feed (Maddox, 1965), thus providing nutrient dense diets at the time of EW may optimize calf development as well. Research demonstrates enhanced growth performance of EW calves compared to normally weaned cohorts (Gasser et al., 2006a; Waterman et al., 2012a,b; Scheffler et al., 2014).
Long-term effects of calf-management following EW on growth and carcass quality of beef steers have been reported (Myers et al., 1999; Meyer et al., 2005; Waterman et al., 2012b; Moriel et al., 2014a; Scheffler et al., 2014), primarily due to early exposure to a high-concentrate diet. Calves provided a high-concentrate diet at 177 vs. 231 days of age had improved feed efficiency while in the feedlot, enhanced marbling scores, heavier carcasses, and a greater percentage of carcasses grading Choice or better (Myers et al., 1999). Likewise, calves that were early-weaned at 3 to 4 months of age and consuming a high-concentrate diet produced heavier carcasses with enhanced marbling scores compared to forage-fed, normally weaned cohorts (Scheffler et al., 2014). These outcomes can be attributed to enhanced expression of adipogenic genes, resulting in early differentiation of adipocytes and fatty acid accumulation (Schoonmaker et al., 2003). To further explore this rationale, Moriel et al. (2014a) investigated the impact of duration of exposure to a high-concentrate diet following EW at 2 to 3 months of age on growth performance, muscle gene expression, and carcass traits of Bos indicus-influenced beef steers. Steers were either normally weaned at 250 days of age (NW; day 180 of the study), or EW at 70 days of age (day 0 of the study) and then randomly assigned to 1 of 3 EW management systems: (1) EW and grazed on pasture for 180 days (EWRG); (2) EW and limit-fed a high-concentrate diet for 180 days (EW180); EW and limit-fed a high-concentrate diet for 90 days and then grazed on pasture for 90 days (EW90).
In muscle samples collected on day 90, Moriel et al. (2014a) reported greater mRNA expression of IGF-I receptor (IGF1R) for EW180 and EW90 steers compared to EWRG and NW steers (Table 1). Additionally, in muscle samples collected on d 180, IGF1R mRNA expression was greater for EW180 vs. EW90 steers and EWRG steers and intermediate for NW steers (Table 1) (Moriel et al., 2014a) As cattle grow, muscle accretion is positively correlated with circulating concentrations of IGF-1 and IGF1R density (Oksbjerg et al., 2004), therefore, the increased mRNA expression of IGF1R in muscle of EW steers exposed to a high concentrate diet immediately after weaning provided additional binding sites for local and systemic IGF-1, resulting in enhanced growth performance of EW vs. NW steers (Moriel et al., 2014a). Moreover, in muscle samples collected on day 90, Moriel et al. (2014a) reported mRNA expression of peroxisome proliferator-activated receptor γ (PPAR-γ) was greater for EW180 and EW90 steers compared to EWRG steers, but similar between EW180 and NW steers, whereas muscle mRNA expression of PPAR-γ on day 180 was greater for EW180 vs. NW, EW90, and EWRG steers (Table 1) (Moriel et al., 2014a). Expression of PPAR-γ is associated with adipogenesis of bovine intramuscular adipose tissue (Lim et al., 2011) and plays a pivotal role regulating adipogenesis through induction of genes mediating lipid metabolism (Houseknecht et al., 2002). Hence, Moriel et al. (2014a) concluded that early exposure to high-concentrate diets not only enhanced growth performance of EW steers, but also induced early differentiation of adipocytes as evidenced by greater mRNA expression of PPAR-γ compared to normal weaning. Corroborating these results, Graugnard et al. (2010) reported exposure to diets rich in starch for 112 days following EW induced precocious intramuscular preadipocyte differentiation and lipid filling in muscle tissue of steers, through elevated expression of PPAR-γ and its targets. More specifically, these authors reported greater mRNA expression of PPAR-γ targets including sterol regulatory element binding factor 1 over 100 days after steers were fed a common diet, suggesting a nutritional imprinting effect elicited by early exposure to high dietary starch, leading to enrichment of intramuscular adipocytes as the animal matured (Graugnard et al., 2010). Conversely, steers fed a diet low in starch showed a blunted lipogenic response through upregulated expression of anti-adipogenic factors, and delayed insulin sensitivity (Graugnard et al., 2010).
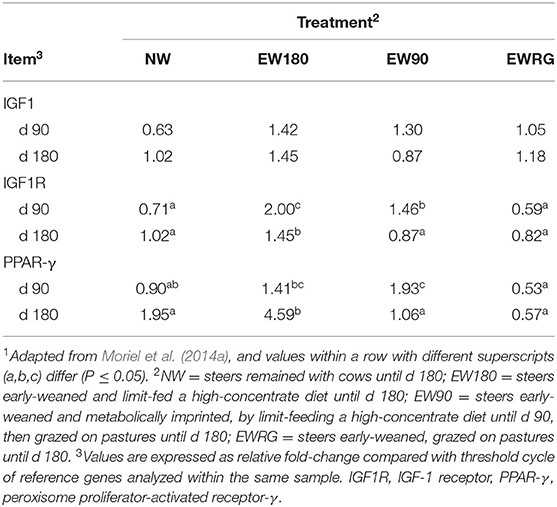
Table 1. Muscle mRNA expression of genes associated with growth and adipose tissue development in beef steers developed in different calf management systems from the time of early weaning (d 0)1.
Recently, specific nutrient inputs provided to EW calves have been investigated including lipid supplements rich in omega-6 fatty acids. Mangrum et al. (2016) demonstrated early-weaned beef steers supplemented with calcium salts of soybean oil (CSSO) had greater percentage of lipid in striploin steaks, and enhanced marbling scores upon slaughter. Further, supplemented steers had decreased average adipocyte diameter in intramuscular adipose tissue, with a greater percentage of intramuscular adipocytes in the small diameter range (Mangrum et al., 2016). Essential fatty acids such as linoleic acid are precursors for activation of receptor/ligand systems that initiate expression of binding proteins (Gaillard et al., 1989), subsequently enhancing expression of PPAR-γ (Xu et al., 1999; Thoennes et al., 2000), thereby regulating adipogenesis. Adipocytes grow by both hyperplasia and hypertrophy, with the latter occurring as cattle age and terminally differentiated cells fill with lipid (Cianzio et al., 1985). Hence, these authors concluded that CSSO supplementation to EW steers enhanced early adipocyte hyperplasia and differentiation (Mangrum et al., 2016). Accordingly, Tipton et al. (2020) also reported increased adipocyte hyperplasia in intramuscular adipose tissue of EW steers supplemented with CSSO for 90 days, evidenced by decreased adipocyte diameter. The same result was not evident in traditionally weaned steers provided the same supplementation, indicating that providing CSSO supplementation earlier in life may result in a greater number of undifferentiated stem cells driven toward adipogenic confirmation and differentiation (Tipton et al., 2020).
Long-term effects of calf management following EW on growth and reproductive performance of beef heifers have been investigated as well (Gasser et al., 2006a,b,c,d; Moriel et al., 2014b). Cow-calf systems rely on replacement heifers to initiate and maintain estrous cyclicity, and in order for heifers to be efficacious, they need to attain puberty by 12 months of age and conceive early in the breeding season (Perry and Cushman, 2013). A plethora of factors play a role in heifer development and puberty attainment, the most notable of which are genetics and nutritional management (Perry, 2016). Most of the research evaluating the impact of nutrition on age at puberty has focused on nutritional inputs after weaning at 7 months of age (Funston et al., 2012). However, the potency of nutritional interventions may be greater earlier in life during critical developmental windows (Day and Anderson, 1998; Hochberg et al., 2011). For example, the effects of nutrition during the early maturation period were evaluated by Gasser et al. (2006a,b,c,d). This model consisted of EW at 2 to 4 months of age and feeding a high-concentrate diet. These authors demonstrated that enhancing the average daily gain (ADG) of EW heifers resulted in hastened puberty attainment and increased the percentage of heifers achieving precocious puberty (Gasser et al., 2006d). Further, ovarian maturation was accelerated in EW heifers offered a high-concentrate diet, evidenced by increased frequency of LH pulses, dominant follicle diameter, and follicular wave duration (Gasser et al., 2006c). These studies were conducted using B. taurus breeds, whereas few studies have investigated the influence of nutritional status during early postnatal development on reproductive maturation in B. indicus-influenced breeds, which often reach puberty at older ages compared to B. taurus heifers (Rodrigues et al., 2002).
To address this gap in knowledge, Moriel et al. (2014b) investigated the impact of post-EW nutritional management strategy on growth and puberty attainment of B. indicus-influenced heifers. Heifers were either normally weaned at 250 days of age (NW; day 180 of the study) or EW at 70 days of age (day 0 of the study) and randomly assigned to 1 of 3 EW management systems: (1) EW and grazed on pasture for 180 days (EWRG); (2) EW and limit-fed a high-concentrate diet for 180 days (EW180); EW and limit-fed a high-concentrate diet for 90 days and then grazed on pasture for 90 days (EW90). These authors reported body weight (BW) was greater for EW180 and EW90 heifers compared to EWRG and NW heifers until day 90 after EW. However, at the time of normal weaning (day 180 of the study), EW180 heifers were the heaviest, EWGR were the lightest, and EW90 and NW were intermediate and did not differ between each other (Moriel et al., 2014b). Consequently, cumulative percentage of pubertal heifers at the start of the breeding season was greatest for EW180 heifers and did not differ between EWRG and NW heifers (Figure 1) (Moriel et al., 2014b). More importantly, the cumulative percentage of pubertal heifers at the start of the breeding season was nearly 2-fold greater for EW90 compared to NW heifers, despite their similar BW and ADG from day 180 until 390 of the study, which provides further evidence of the metabolic imprinting effects of early exposure to high-concentrate diets in beef cattle. Similar findings have been reported using B. indicus-influenced heifers, weaned at approximately 4 months of age and fed a high concentrate diet to promote a body weight gain of 1 kg daily (Cardoso et al., 2014a,b). Cardoso et al. (2014b) employed a stair-step dietary strategy involving alternating periods of feed restriction and re-feeding in heifers weaned at 3.5 months of age. These authors reported heifers exhibiting high rates of body weight gain between 4 and 6.5 months of age, followed by feed restriction between 6.5 to 9 months of age attained puberty at similar rates compared to cohorts receiving a high-concentrate diet continuously Cardoso et al. (2014b). Alternatively, the same results were not observed in heifers that were subjected to feed restriction from 4 to 6.5 months of age, followed by access to a high-concentrate diet Cardoso et al. (2014b). Moriel et al. (2014b) concluded that the enhanced nutrient intake and ADG of EW90 heifers during a period of developmental plasticity, hastened follicular development and LH secretion (Gasser et al., 2006c,d), contributing to accelerated reproductive maturation.
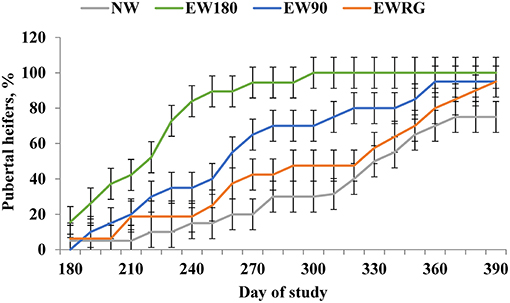
Figure 1. Puberty achievement of replacement heifers developed on different calf management systems from the time of early-weaning (d 0) until the time of normal weaning (d 180). NW = heifers remained with cows until d 180; EW180 = heifers early-weaned and limit-fed a high- concentrate diet until d 180; EW90 = heifers early-weaned and metabolically imprinted, by limit- feeding a high-concentrate diet until d 90, then grazed on pastures until d 180; EWRG = heifers early-weaned, grazed on pastures until d 180. A treatment x day interaction (P < 0.01) was detected for puberty attainment. Adapted from Moriel et al. (2014b).
Moriel et al. (2014b) also reported that hepatic mRNA abundance of IGF-1 on d 90 was positively correlated (P ≤ 0.05) with the ADG from d 0 to 90, whereas liver IGF-1 mRNA expression on d 180 was positively correlated (P = 0.0002) with the ADG from d 90 to 180. Moreover, liver IGF-1 mRNA expression of EW90 heifers on d 270 (90 days after all heifers were provided similar nutritional management) was greater than NW and EW180 heifers (Figure 2), despite the similar post-weaning ADG, which further supports the concept of metabolic imprinting effects on puberty achievement of beef heifers. Dietary regimens that promote high rates of body weight gain result in greater adiposity and increased circulating concentrations of leptin, insulin, and IGF-1 compared with heifers achieving lower rates of body weight gain (Allen et al., 2012; Cardoso et al., 2014b; Alves et al., 2015). These changes in circulating metabolites result in modifications in the reproductive neuroendocrine axis such as increased pulse frequency of GnRH and LH (Cardoso et al., 2014a) and enhance steroidogenesis within the ovaries (Spicer and Echternkamp, 1995). Moriel et al. (2014b) reported age at puberty decreased approximately 0.5 days for every 1 ng/mL increase in plasma concentrations of IGF-1 on d 90 and 180, providing evidence that the metabolic imprinting process, likely via IGF-1 hastened puberty attainment of beef heifers. Further, increased rates of body weight gain in heifers following EW has resulted in altered DNA methylation patterns in the hypothalamic arcuate nucleus, with significant modifications in the methylation status of genes associated with growth and puberty attainment (Alves et al., 2017), indicating the potential for neuronal plasticity. Collectively, these results demonstrate there exists a critical period in which nutrient inputs may induce early activation of the reproductive axis, thus programming puberty attainment in beef heifers.
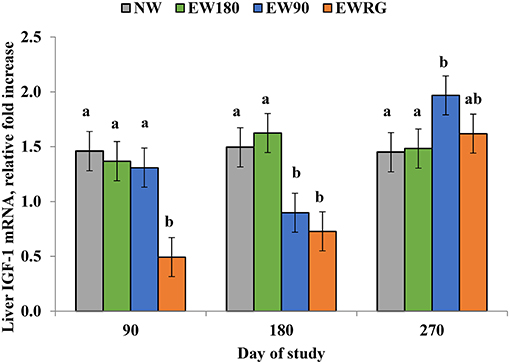
Figure 2. Liver IGF-1 mRNA expression of heifers developed on different calf management systems from the time of early-weaning (d 0) until the time of normal weaning (d 180). NW = heifers remained with cows until d 180; EW180 = heifers early-weaned and limit-fed a high-concentrate diet until d 180; EW90 = heifers early-weaned and metabolically imprinted, by limit- feeding a high-concentrate diet until d 90, then grazed on pastures until d 180; EWRG = heifers early-weaned, grazed on pastures until d 180. Adapted from Moriel et al. (2014b). A treatment × day interaction was detected (P < 0.01). a,bWithin days, means without a common superscript differ (P ≤ 0.05).
Metabolic Imprinting in Normally Weaned Beef Calves
While EW provides an opportunity to manipulate calf nutrient intake during a critical window of development and increase cow productivity (Arthington and Kalmbacher, 2003; Arthington and Minton, 2004), this strategy may not be a feasible management alternative for many commercial cow-calf systems depending on forage availability, supplement cost, and overall net return (Vendramini et al., 2006). Thus, alternative management strategies to enhance early life nutrition and growth of nursing calves are warranted. For example, post-weaning injections of bovine somatotropin (bST) hastened puberty attainment of replacement beef heifers (Cooke et al., 2013), whereas circulating IGF-1 during juvenile development has been positively associated with puberty attainment (Moriel et al., 2014b). Hence, Piccolo et al. (2018) investigated the impact of pre-weaning injections of bST on growth and reproductive performance of B. taurus × B. indicus beef heifers. Heifers received a s.c. injection of a saline solution or 250 mg of bST every 14 days from 134 to 162 days of age (Piccolo et al., 2018). Piccolo et al. (2018) reported pre-weaning injections of bST decreased age at puberty in heifers by approximately 26 days and hasted puberty attainment and pregnancy rates compared to saline injections, despite similar growth performance and nutritional management from weaning and during the breeding season. Contrariwise, Moriel et al. (2019) reported decreased puberty attainment in Nelore heifers (B indicus) receiving pre-weaning bST injections (25% vs. 40% for bST and saline heifers, respectively). Inconsistencies between the two experiments were attributed to greater baseline plasma IGF-1 concentrations in B. indicus females compared with to B. taurus crosses (Alvarez et al., 2000; Moriel et al., 2019). This likely led to an overstimulation of the somatotropic axis following bST injections in Nelore heifers (Cooke et al., 2020), which was detrimental to reproductive maturation (Moriel et al., 2019), emphasizing the importance of developing metabolic imprinting strategies specific to cattle subspecies.
Another alternative to isolate the effects of metabolic imprinting and EW is to provide supplements to nursing cattle via creep feeding (CF). This practice serves to adapt calves to concentrate supplementation prior to weaning, and it is well-established that BW at weaning may be increased if limited or unlimited CF supplementation is offered to nursing calves (Faulkner et al., 1994; Sexten et al., 2004; Moriel and Arthington, 2013a,b). Creep-fed calves also exhibit greater dry matter intake (Faulkner et al., 1994; Moriel and Arthington, 2013b) and BW gain during the feedlot receiving period (Arthington et al., 2008), which is one of the most critical periods in the beef production cycle when cattle are exposed to a variety of challenges resulting in increased rates of morbidity (Snowder et al., 2006). Therefore, strategies to enhance nutrient intake during this period are warranted to optimize receiving cattle performance (Cooke, 2017). For example, CF calves experience decreased morbidity and mortality during transition to the feedlot (e.g., 7–10 months of age) compared to cohorts receiving no pre-weaning supplementation (Loerch and Fluharty, 1999; Myers et al., 1999; Lardy and Maddock, 2007). Research examining the impacts of CF and finishing performance and carcass traits has been variable. In a two-year study, Lancaster et al. (2007) observed CF calves had greater carcass weights, dressing percentage, and backfat thickness compared to non-supplemented calves in the first year but not the second. Others have also reported no differences in carcass traits comparing calves provided or not CF supplementation (Tarr et al., 1994; Myers et al., 1999; Shike et al., 2007; Gadberry et al., 2012).
The inconsistency in lifelong effects associated with pre-weaning nutrient supply on carcass quality may be due to a variety of confounding factors across experiments including post-weaning management, and composition and intake of nutrients during CF. However, nutrient specific strategies, such as supplemental fat, warranted investigation given the roles endogenous fatty acids play in adipogenesis (Price et al., 2000; Houseknecht et al., 2002). Supplementation with CSSO after weaning has resulted in increased marbling and percent choice carcasses upon slaughter compared with non-supplemented cohorts (Cooke et al., 2011; Mangrum et al., 2016). However, such dietary inputs offered earlier in life may be even more beneficial, given that developmental plasticity declines as animals mature (Lucas, 1998). In a 2 × 2 factorial design, Schubach et al. (2019) investigated the impacts of CSSO supplementation to beef steers at 2 months of age via CF and/or during a 40-day preconditioning period on carcass development and quality. Treatments were formulated to be isocaloric and isolipidic but differing in fatty acid profile. These authors observed steers receiving CSSO via CF supplementation had greater mRNA expression of adipocyte fatty acid binding protein-4, fatty acid synthase (FASN), PPAR-γ, and stearoyl CoA desaturase in muscle samples collected during feedlot finishing compared with cohorts that received prilled saturated fat during CF (Table 2). Expression of these genes did not differ between all calves before shipping to the feedlot, whereas differences were only noted during the feedlot period, when calves were exposed to high-energy diets and lipogenesis is substantial (Pethick et al., 2004; Schubach et al., 2019). However, supplementing CSSO during a 40-day preconditioning period had no impact on muscle gene expression during the feedlot period, whereas providing CSSO during both CF and preconditioning did not yield additive benefits (Schubach et al., 2019). These authors concluded that CSSO supplementation provided via CF, during a period of elevated epigenetic and developmental plasticity (Lucas, 1998), elicited alterations in mRNA expressions of genes associated with adipogenic activities in the muscle, which was suggestive of a metabolic imprinting effect (Du et al., 2010). However, differential gene expression during the feedlot period did not translate into differences in performance or carcass traits upon slaughter, which did not differ between treatments (Table 2) (Schubach et al., 2019). These results are contrary to those reported by Cooke et al. (2011) and Mangrum et al. (2016), whereas the treatments offered in the previous experiments were not isocaloric or isolipidic as utilized by Schubach et al. (2019). Nonetheless, elevated mRNA expression may not be accompanied by equivalent phenotypic responses (Clancy and Brown, 2008; Graugnard et al., 2010), and research is still warranted to investigate the effects of CSSO supplementation to nursing beef cattle on adipogenesis and lipid metabolism (Schubach et al., 2019).
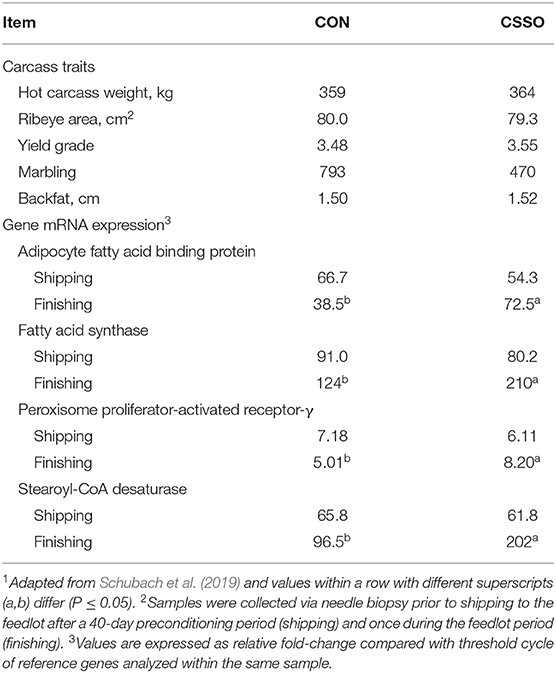
Table 2. Carcass traits and expression of genes in the longissimus muscle associated from beef steers supplemented with Ca salts of soybean oil (CSSO) or prilled saturated fat (CON) from 2 to 4 months of age via creep feeding1,2.
Numerous studies have shown reductions in age at puberty in heifer calves offered a high plane of nutrition early in juvenile development (Shamay et al., 2005; Gasser et al., 2006a; Davis Rincker et al., 2011; Cardoso et al., 2014b; Moriel et al., 2014b) due to advanced conditioning and maturation of the reproductive axis (Kelly et al., 2020). However, these experiments have been conducted using EW, and research exploring the effects of early-life nutritional status while suckling on growth and puberty attainment is limited. Providing nursing beef heifers access to CF supplementation to increase pre-weaning ADG may also affect their future lactation potential. For example, nursing heifers receiving CF supplementation beginning at 4 months (Buskirk et al., 1996a) or 5 months (Buskirk et al., 1996b) of age had decreased milk production as primiparous cows, which was attributed to greater fat accumulation in the mammary gland (Brown et al., 2005). Conversely, Sexten et al. (2004) reported heifers offered CF supplements containing 18% CP had greater milk production 52 and 108 days postpartum compared to heifers fed supplements containing 14% CP, suggesting increasing dietary CP may alleviate the negative effects of accelerated BW gain during the pre-weaning period on mammary gland development. However, pre-weaning body weight gain and weaning body weight was similar between calves born from creep-fed and non-creep fed heifers (Buskirk et al., 1996b; Sexten et al., 2004), suggesting calves are able to compensate for decreased milk production from the dam by increasing forage intake (Sexten et al., 2004).
In an effort to avoid the critical allometric period of mammary gland growth (Buskirk et al., 1996a), and yet still initiate metabolic imprinting events, Reis et al. (2015) provided nursing beef heifers ad libitum access to CF supplementation (MI) or not (CON) from 68 to 118 days of age. These authors reported MI heifers had transient and long-term increases in genes regulating adipocyte development (Reis et al., 2015). More specifically, MI heifers had increased adipocyte mRNA expression of FASN following the imprinting phase and long-term increased mRNA expression of PPAR-γ during the entire experimental period compared to CON heifers (Table 3) (Reis et al., 2015). These authors reported no differences in backfat thickness or subcutaneous adipocyte size and density (Table 3), despite the established role both genes play in adipocyte development (Graugnard et al., 2009; Lim et al., 2011). Furthermore, MI heifers had long-term increased hepatic mRNA expression of IGF-1 (Table 3), whereas plasma IGF-1 concentrations increased only transiently following the imprinting phase. Treatment differences noted for mRNA expression of genes associated with nutrient and lipid metabolism between MI and CON heifers were suggestive of metabolic imprinting effects due to CF supplementation during a critical window of development (Reis et al., 2015). Guggeri et al. (2018) also reported providing CF supplementation to nursing beef heifers beginning at 75 days of age resulted in long-term increases in hepatic and endometrial mRNA expression. More specifically, heifers receiving CF supplementation had greater hepatic mRNA expression of growth hormone receptor and IGF binding protein-3 on day 7 of their estrous cycle, and greater endometrial mRNA expression of IGF-1 on day 16 of their estrous cycle compared to non-supplemented cohorts (Guggeri et al., 2018). Such expression in the endometrium is consistent with ewes carrying larger embryos (Sequeira et al., 2016) and is related to embryo growth and uterine function in dairy cows (Wathes et al., 2011). Therefore, Guggeri et al. (2018) concluded that early life nutritional status programmed the sensitivity of the liver to growth hormone, and resulted in long-term effects on the functioning of the somatotropic axis in the endometrium.
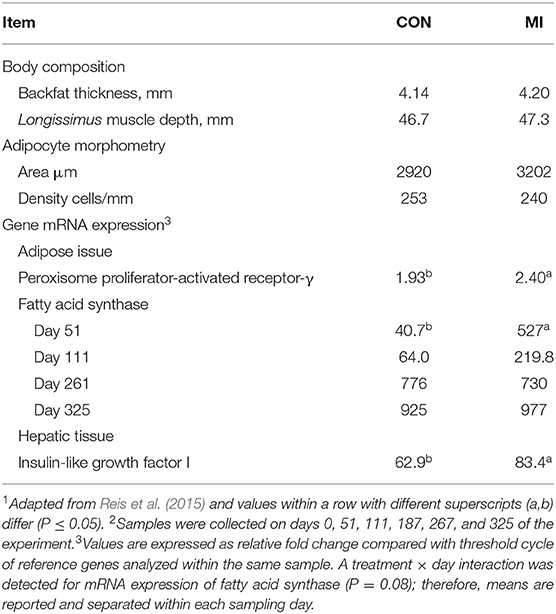
Table 3. Body composition and mRNA expression of genes associated with lipogenesis and growth in beef heifers receiving (MI) or not (CON) a corn-based supplement ad libitum from 2 to 4 months of age via creep feeding1,2.
Reis et al. (2015) noted that puberty attainment did not differ between MI vs. CON heifers, corroborating similar circulating IGF-1 concentrations and body composition (Table 3) given these variables influence advancement of puberty onset (Schillo et al., 1992; Cooke et al., 2008). As mentioned, others have reported hastened puberty attainment in EW heifers receiving a high-concentrate for 70 days (Gasser et al., 2006a) and 90 days (Moriel et al., 2014b); therefore, Reis et al. (2015) concluded that perhaps a longer period of CF supplementation for nursing beef heifers may be required to substantially increase their supplement intake and effectively enhance their reproductive development. Corroborating this rationale, Guggeri et al. (2014) reported that providing CF supplementation to nursing beef heifers from 75 to 158 days of age resulted in accelerated puberty attainment compared to unsupplemented, traditionally weaned cohorts, as well as EW heifers. Collectively, these results demonstrate that providing CF supplementation to nursing heifers during a critical window of development may induce early activation of the reproductive axis.
Conclusions
Metabolic imprinting is associated with a critical window of postnatal developmental plasticity in which nutritional interventions may result in long-term consequences on animal metabolism (Patel and Srinivasan, 2011). Identifying and implementing strategies targeting this period of development offers an opportunity to enhance efficiency and profitability of beef production systems. Early-weaning of beef calves prior to the breeding season is a strategy that may increase reproductive performance of primiparous cows, but simultaneously optimize carcass quality of feeder cattle and reproductive development of replacement females. Providing supplement to nursing calves via CF may be a nutritional alternative to stimulate metabolic imprinting effects, without the need for EW. Collectively, the results from the experiments described herein demonstrate that manipulating nutrient supply during periods of developmental plasticity, such as early postnatal life, exerts long-term consequences on performance and physiology of beef cattle.
Author Contributions
KH, RC, and PM: conceptualization, writing—original draft preparation, writing—review, and editing. RC: supervision. All authors contributed to the article and approved the submitted version.
Conflict of Interest
The handling editor JL declared a past co-authorship with one of the authors PM.
The remaining authors declare that the research was conducted in the absence of any commercial or financial relationships that could be construed as a potential conflict of interest.
Publisher's Note
All claims expressed in this article are solely those of the authors and do not necessarily represent those of their affiliated organizations, or those of the publisher, the editors and the reviewers. Any product that may be evaluated in this article, or claim that may be made by its manufacturer, is not guaranteed or endorsed by the publisher.
Acknowledgments
The authors thank collaborating faculty, staff, and students that have helped conduct research in our laboratory and described in this manuscript.
References
Allen, C. C., Alves, B. R. C., Li, X., Tedeschi, L. O., Zhou, H., Paschal, J. C., et al. (2012). Gene expression in the arcuate nucleus of heifers is affected by controlled intake of high- and low-concentrate diets. J. Anim. Sci. 90, 2222–2232. doi: 10.2527/jas.2011-4684
Alvarez, P., Spicer, L. J., Chase, C. C. Jr., Payton, M. E., Hamilton, T. D., Stewart, R. E., et al. (2000). Ovarian and endocrine characteristics during an estrous cycle in Angus, Brahman, and Senepol cows in a subtropical environment. J. Anim. Sci. 78, 1291–1302. doi: 10.2527/2000.7851291x
Alves, B. R. C., Cardoso, R. C., Doan, R., Zhang, Y., Dindot, S. V., Williams, G. L., et al. (2017). Nutritional programming of accelerated puberty in heifers: alterations in DNA methylation in the arcuate nucleus. Biol. Reprod. 96, 174–184. doi: 10.1095/biolreprod.116.144741
Alves, B. R. C., Cardoso, R. C., Prezotto, L. D., Thorson, J. F., Bedenbaugh, M., Sharpton, S. M., et al. (2015). Elevated body weight gain during the juvenile period alters neuropeptide Y-gonadotropin-releasing hormone circuitry in prepubertal heifers. Biol. Reprod. 92:46. doi: 10.1095/biolreprod.114.124636
Arthington, J. D., and Kalmbacher, R. S. (2003). Effect of early weaning on the performance of three-year-old, first-calf beef heifers and calves reared in the subtropics. J. Anim. Sci. 81, 1136–1141. doi: 10.2527/2003.8151136x
Arthington, J. D., and Minton, J. E. (2004). The effect of early calf weaning on feed intake, growth, and postpartum interval in thin, Brahman-crossbred primiparous cows. Prof. Anim. Sci. 20, 34–38. doi: 10.15232/S1080-7446(15)31269-9
Arthington, J. D., Qiu, X., Cooke, R. F., Vendramini, J. M. B., Araujo, D. B., Chase, C. C. Jr., et al. (2008). Effects of preshipping management on measures of stress and performance of beef steers during feedlot receiving. J. Anim. Sci. 86, 2016–2023. doi: 10.2527/jas.2008-0968
Barker, D. J., and Clark, P. M. (1997). Fetal undernutrition and disease in later life. Rev. Reprod. 2, 105–112. doi: 10.1530/ror.0.0020105
Bartol, F. F., Wiley, A. A., Miller, D. J., Silva, A. J., Roberts, K. E., Davolt, M. L. P., et al. (2013). lactation biology symposium: lactocrine signaling and developmental programming. J. Anim. Sci. 91, 696–705. doi: 10.2527/jas.2012-5764
Bateson, P., Barker, D., Clutton-Brock, T., Deb, D., D'Udine, B., Foley, R. A., et al. (2004). Developmental plasticity and human health. Nature 430, 419–421. doi: 10.1038/nature02725
Brown, E. G., VandeHaar, M. J., Daniels, K. M., Liesman, J. S., Chapin, L. T., Forrest, J. W., et al. (2005). Effect of increasing energy and protein intake on mammary development in heifer calves. J. Dairy Sci. 88, 595–603. doi: 10.3168/jds.S0022-0302(05)72723-5
Buskirk, D. D., Faulkner, D. B., Hurley, W. L., Kesler, D. J., Ireland, F. A., Nash, T. G., et al. (1996a). Growth, reproductive performance, mammary development, and milk production of beef heifers as influenced by prepubertal dietary energy and administration of bovine somatotropin. J. Anim. Sci. 74, 2649–2662. doi: 10.2527/1996.74112649x
Buskirk, D. D., Faulkner, D. B., and Ireland, F. A. (1996b). Subsequent productivity of beef heifers that received creep feed for 0, 28, 56, or 84 d before weaning. Prof. Anim. Sci. 12, 37–43. doi: 10.15232/S1080-7446(15)32480-3
Canani, R. B., Costanzo, M. D., Leone, L., Bedogni, G., Brambilla, P., Cianfarani, S., et al. (2011). Epigenetic mechanisms elicited by nutrition in early life. Nut Res. Rev. 24, 198–205. doi: 10.1017/S0954422411000102
Cardoso, R. C., Alves, B. R. C., Prezotto, L. D., Thorson, J. F., Tedeschi, L. O., Keisler, D. H., et al. (2014a). Reciprocal changes in leptin and NPY during nutritional acceleration of puberty in heifers. J. Endocrinol. 223, 289–298. doi: 10.1530/JOE-14-0504
Cardoso, R. C., Alves, B. R. C., Prezotto, L. D., Thorson, J. F., Tedeschi, L. O., Keisler, D. H., et al. (2014b). Use of a stair-step compensatory gain nutritional regimen to program the onset of puberty in beef heifers. J. Anim. Sci. 92, 2942–2949. doi: 10.2527/jas.2014-7713
Cianzio, D. S., Topel, D. G., Whitehurst, G. B., Beitz, D. C., and Self, H. L. (1985). Adipose tissue growth and cellularity: changes in bovine adipocyte size and number. J. Anim. Sci. 60, 970–976. doi: 10.2527/jas1985.604970x
Cooke, R. F. (2017). Invited paper: nutritional and management considerations for beef cattle experiencing stress-induced inflammation. Prof. Anim. Sci. 33, 1–11. doi: 10.15232/pas.2016-01573
Cooke, R. F., Arthington, J. D., Araujo, D. B., Lamb, G. C., and Ealy, A. D. (2008). Effects of supplementation frequency on performance, reproductive, and metabolic responses of Brahman-crossbred females. J. Anim. Sci. 86, 2296–2309. doi: 10.2527/jas.2008-0978
Cooke, R. F., Bohnert, D. W., Francisco, C. L., Marques, R. S., Mueller, C. J., and Keisler, D. H. (2013). Effects of bovine somatotropin administration on growth, physiological, and reproductive responses of replacement beef heifers. J. Anim. Sci. 91:2894–2901. doi: 10.2527/jas.2012-6082
Cooke, R. F., Bohnert, D. W., Moriel, P., Hess, B. W., and Mills, R. R. (2011). Effects of polyunsaturated fatty acid supplementation on ruminal in situ forage degradability, performance, and physiological responses of feeder cattle. J. Anim. Sci. 89, 3677–3689. doi: 10.2527/jas.2010-3515
Cooke, R. F., Daigle, C. L., Moriel, P., Smith, S. B., Tedeschi, L. O., and Vendramini, J. M. B. (2020). Cattle adapted to tropical and subtropical environments: social, nutritional, and carcass quality considerations. J. Anim. Sci. 98:skaa014. doi: 10.1093/jas/skaa014
Davis Rincker, L. E., VandeHaar, M. J., Wolf, C. A., Liesman, J. S., Chapin, L. T., and Weber Nielsen, M. S. (2011). Effect of intensified feeding of heifer calves on growth, pubertal age, calving age, milk yield, and economics. J. Dairy Sci. 94, 3554–3567. doi: 10.3168/jds.2010-3923
Day, M. L., and Anderson, L. H. (1998). Current concepts on the control of puberty in cattle. J. Anim. Sci. 76, 1–15. doi: 10.2527/1998.76suppl_31x
Du, M., Tong, J., Zhao, J., Underwood, K. R., Zhu, M., Ford, S. P., et al. (2010). Fetal programming of skeletal muscle development in ruminant animals. J. Anim. Sci. 88, E51–E60. doi: 10.2527/jas.2009-2311
Fall, C. H. D. (2011). Evidence for the intra-uterine programming of adiposity in later life. Ann. Hum. Biol. 38, 410–428. doi: 10.3109/03014460.2011.592513
Faulkner, D. B., Hummel, D. F., Buskirk, D. D., Berger, L. L., Parrett, D. F., and Cmarik, G. F. (1994). Performance and nutrient metabolism by nursing calves supplemented with limited or unlimited corn or soyhulls. J. Anim. Sci. 72, 470–477. doi: 10.2527/1994.722470x
Fenech, M. F. (2010). Dietary reference values of individual micronutrients and nutriomes for genome damage prevention: current status and a road map to the future. Am. J. Clin. Nutr. 91, 1438S–1454S. doi: 10.3945/ajcn.2010.28674D
Food and Agricultural Organization (FAO) (2009). “How to feed the world in 2050,” in Proceedings of the Expert Meeting on How to Feed the World in 2050 (Rome: FAO Headquarters).
Funston, R. N., Martin, J. L., Larson, D. M., and Roberts, A. J. (2012). Physiology and endocrinology symposium: nutritional aspects of developing replacement heifers. J. Anim. Sci. 90, 1166–1171. doi: 10.2527/jas.2011-4569
Funston, R. N., and Summers, A. F. (2013). Epigenetics: setting up lifetime production of beef cows by managing nutrition. Ann. Rev. Anim. Biosci. 1, 339–363. doi: 10.1146/annurev-animal-031412-103649
Gadberry, M. S., Beck, P. A., Gunter, S. A., Barham, B. L., Whitworth, W. A., and Apple, J. K. (2012). Effect of corn- and soybean hull-based creep feed and backgrounding diets on lifelong performance and carcass traits of calves from pasture and rangeland conditions. Prof. Anim. Sci. 28, 507–518. doi: 10.15232/S1080-7446(15)30399-5
Gaillard, D., Négrel, R., Lagarde, M., and Ailhaud, G. (1989). Requirement and role of arachidonic acid in the differentiation of pre-adipose cells. Biochem. J. 257, 389–397. doi: 10.1042/bj2570389
Gasser, C. L., Behlke, E. J., Grum, D. E., and Day, M. L. (2006a). Effect of timing of feeding a high-concentrate diet on growth and attainment of puberty in early-weaned heifers. J. Anim. Sci. 84:3118–3122. doi: 10.2527/jas.2005-676
Gasser, C. L., Bridges, G. A., Mussard, M. L., Grum, D. E., Kinder, J. E., and Day, M. L. (2006b). Induction of precocious puberty in heifers III: hastened reduction of estradiol negative feedback on secretion of luteinizing hormone. J. Anim. Sci. 84, 2050–2056. doi: 10.2527/jas.2005-638
Gasser, C. L., Burke, C. R., Mussard, M. L., Behlke, E. J., Grum, D. E., Kinder, J. E., et al. (2006c). Induction of precocious puberty in heifers II: advanced ovarian follicular development. J. Anim. Sci. 84, 2042–2049. doi: 10.2527/jas.2005-637
Gasser, C. L., Grum, D. E., Mussard, M. L., Fluharty, F. L., Kinder, J. E., and Day, M. L. (2006d). Induction of precocious puberty in heifers I: enhanced secretion of luteinizing hormone. J. Anim. Sci. 84, 2035–2041. doi: 10.2527/jas.2005-636
Graugnard, D. E., Berger, L. L., Faulkner, D. B., and Loor, J. J. (2010). High-starch diets induce precocious adipogenic gene network up-regulation in longissimus lumborum of early-weaned Angus cattle. Br. J. Nutr. 103, 953–963. doi: 10.1017/S0007114509992789
Graugnard, D. E., Piantoni, P., Bionaz, M., Berger, L. L., Faulkner, D. B., and Loor, J. J. (2009). Adipogenic and energy metabolism gene networks in longissimus lumborum during rapid post-weaning growth in Angus and Angus × Simmental cattle fed high-starch or low-starch diets. BMC Genomics 10, 142. doi: 10.1186/1471-2164-10-142
Guggeri, D., Meikle, A., Carriquiry, M., Montossi, F., De Barbieri, I., and Viñoles, C. (2014). Effect of different management systems on growth, endocrine parameters and puberty in Hereford female calves grazing Campos grassland. Livest. Sci. 167, 455–462. doi: 10.1016/j.livsci.2014.06.026
Guggeri, D. A., Meikle, M., Carriquiry, I. D., Barbieri, F., and Montossi Viñoles, C. (2018). Long-term effect of early nutrition on endocrine parameters and liver and endometrial gene expression of the members of the somatotrophic axis in Hereford heifers. Reprod. Dom. Anim. 53, 930–936. doi: 10.1111/rda.13190
Hochberg, Z., Feil, R., Constancia, M., Fraga, M., Junien, C., Carel, J.-C., et al. (2011). Child health, developmental plasticity, and epigenetic programming. Endocr. Rev. 32, 159–224. doi: 10.1210/er.2009-0039
Houseknecht, K. L., Cole, B. M., and Steele, P. J. (2002). Peroxisome proliferator-activated receptor gamma (PPARγ) and its ligands: a review. Domest. Anim. Endocrinol. 22, 1–23. doi: 10.1016/S0739-7240(01)00117-5
Hu, L., Peng, X., Han, F., Wu, F., Chen, D., Wu, D., et al. (2020). Effects of birth weight and postnatal nutritional restriction on skeletal muscle development, myofiber maturation, and metabolic status of early-weaned piglets. Animals 10:156. doi: 10.3390/ani10010156
Kelly, A. K., Byrne, C., McGee, M., Perry, G. A., Crowe, M. A. H., et al. (2020). Effect of calfhood nutrition on metabolic hormones, gonadotropins, and estradiol concentrations and on reproductive organ development in beef heifer calves. J. Anim. Sci. 98:skaa310. doi: 10.1093/jas/skaa310
Koletzko, B., Brands, B., Poston, L., Godfrey, K., and Demmelmair, H. (2012). Early nutrition programming of long-term health. Proc. Nutr. Soc. 71, 371–378. doi: 10.1017/S0029665112000596
Lancaster, P. A., Corners, J. B., Thompson, L. N., Ellersieck, M. R., Buckner, C. D., and Williams, J. E. (2007). Effects of distillers dried grains with solubles as a protein source in creep feed. 2. Subsequent feedlot performance, carcass measurements, and plasma parameters. Prof. Anim. Sci. 23, 91–103. doi: 10.15232/S1080-7446(15)30949-9
Lardy, G. P., and Maddock, T. D. (2007). Creep feeding nursing beef calves. Vet. Clin. N. Am. Food Anim. Pract. 23, 21–28. doi: 10.1016/j.cvfa.2006.11.002
Lillycrop, K. A., Phillips, E. S., Torrens, C., Hanson, M. A., Jackson, A. A., and Burdge, G. C. (2008). Feeding pregnant rats a protein-restricted diet persistently alters the methylation of specific cytosines in the hepatic PPARα promoter of the offspring. Br. J. Nutr. 100, 278–282. doi: 10.1017/S0007114507894438
Lillycrop, K. A., Slater-Jefferies, J. L., Hanson, M. A., Godfrey, K. M., Jackson, A. A., and Burdge, G. C. (2007). Induction of altered epigenetic regulation of the hepatic glucocorticoid receptor in the offspring of rats fed a protein-restricted diet during pregnancy suggests that reduced DNA methyltransferase-1 expression is involved in impaired DNA methylation and changes in histone modifications. Br. J. Nutr. 97, 1064–1073. doi: 10.1017/S000711450769196X
Lim, D., Kim, N.-K., Park, H.-S., Lee, S.-H., Cho, Y.-M., Oh, S. J., et al. (2011). Identification of candidate genes related to bovine marbling using protein-protein interaction networks. Int. J. Biol. Sci. 7:992–1002. doi: 10.7150/ijbs.7.992
Ling, C., and Groop, L. (2009). Epigenetics: a molecular link between environmental factors and type 2 diabetes. Diabetes 58, 2718–2725. doi: 10.2337/db09-1003
Loerch, S. C., and Fluharty, F. L. (1999). Physiological changes and digestive capabilities of newly received feedlot cattle. J. Anim. Sci. 77:1113–1119. doi: 10.2527/1999.7751113x
Lucas, A. (1991). Programming by early nutrition in man. Ciba Found. Symp. 156, 38–50. doi: 10.1002/9780470514047.ch4
Lucas, A. (1998). Programming by early nutrition: an experimental approach. J. Nutr. 128, 401S–406S. doi: 10.1093/jn/128.2.401S
MacInstosh, B., Gardiner, P., and McComas, A. (2006). Skeletal Muscle: Form and Function; Human Kinetics, 2nd Edn. Champaign, IL: Human Kinetics. doi: 10.5040/9781492596912
Maddox, L. A. (1965). Nutrient Requirements of the Cow and Calf. College Station, TX: Texas Agricultural Experimental Station, Bulletin No. 1044.
Mangrum, K. S., Tuttle, G., Duckett, S. K., Sell, G. S., Krehbiel, C. R., and Long, N. M. (2016). The effect of supplementing rumen undegradable unsaturated fatty acids on marbling in early-weaned steers. J. Anim. Sci. 94, 833–844. doi: 10.2527/jas.2015-9809
Meyer, D. L., Kerley, M. S., Walker, E. L., Keisler, D. H., Pierce, V. L., Schmidt, T. B., et al. (2005). Growth rate, body composition, and meat tenderness in early vs. traditionally weaned beef calves. J. Anim. Sci. 83:2752–2761. doi: 10.2527/2005.83122752x
Moriel, P., and Arthington, J. D. (2013a). Effects of molasses-based creep-feeding supplementation on growth performance of pre- and post-weaned beef calves. Livest. Sci. 151, 171–178. doi: 10.1016/j.livsci.2012.11.008
Moriel, P., and Arthington, J. D. (2013b). Effects of trace mineral-fortified, limit-fed preweaning supplements on performance of pre- and postweaned beef calves. J. Anim. Sci. 91, 1371–1380. doi: 10.2527/jas.2012-5469
Moriel, P., Cappellozza, B. I., Piccolo, M. B., Cooke, R. F., Miranda, M. F., Batista, L. F. D., et al. (2019). Pre- and post-weaning injections of bovine somatotropin to optimize puberty achievement of Bos indicus beef heifers. Trans. Anim. Sci. 3, 443–455. doi: 10.1093/tas/txy125
Moriel, P., Johnson, S. E., Vendramini, J. M. B., McCann, M. A., Gerrard, D. E., Mercadante, V. R. G., et al. (2014a). Effects of calf weaning age and subsequent management systems on growth performance and carcass characteristics of beef steers. J. Anim. Sci. 92, 3598–3609. doi: 10.2527/jas.2014-7751
Moriel, P., Johnson, S. E., Vendramini, J. M. B., Mercadante, V. R. G., Hersom, M. J., and Arthington, J. D. (2014b). Effects of calf weaning age and subsequent management system on growth and reproductive performance of beef heifers. J. Anim. Sci. 92, 3096–3107. doi: 10.2527/jas.2013-7389
Myers, S. E., Faulkner, D. B., Ireland, F. A., Berger, L. L., and Parrett, D. F. (1999). Production systems comparing early weaning to normal weaning with or without creep feeding for beef steers. J. Anim. Sci. 77, 300–310. doi: 10.2527/1999.772300x
Neibergs, H. L., and Johnson, K. A. (2012). Alpharma beef cattle nutrition symposium: nutrition and the genome. J. Anim. Sci. 90:2308–2316. doi: 10.2527/jas.2011-4582
Oksbjerg, N., Gondret, F., and Vestergaard, M. (2004). Basic principles of muscle development and growth in meat-producing mammals as affected by the insulin-like growth factor (IGF) system. Domest. Anim. Endocrinol. 27, 219–240. doi: 10.1016/j.domaniend.2004.06.007
Ong, K. K., Emmett, P. M., Noble, S., Ness, A., and Dunger, D. B. (2006). Dietary energy intake at the age of 4 months predicts postnatal weight gain and childhood body mass index. Pediatrics 117, e503–e508. doi: 10.1542/peds.2005-1668
Patel, M. S., and Srinivasan, M. (2011). Metabolic programming in the immediate postnatal life. Ann. Nutr. Metab. 58, 18–28. doi: 10.1159/000328040
Perry, G. A. (2016). Factors affecting puberty in replacement beef heifers. Theriogenology 86, 373–378. doi: 10.1016/j.theriogenology.2016.04.051
Perry, G. A., and Cushman, R. (2013). Effect of age at puberty/conception date on cow longevity. Vet. Clin. N. Am. Food Anim. Pract. 29, 579–590. doi: 10.1016/j.cvfa.2013.07.011
Pethick, D. W., Harper, G. S., and Oddy, V. H. (2004). Growth, development and nutritional manipulation of marbling in cattle: a review. Aust. J. Exp. Agric. 44, 705–715. doi: 10.1071/EA02165
Piccolo, M. B., Arthington, J. D., Silva, G. M., Lamb, G. C., Cooke, R. F., and Moriel, P. (2018). Preweaning injections of bovine ST enhanced reproductive performance of Bos indicus-influenced replacement beef heifers. J. Anim. Sci. 96, 618–631. doi: 10.1093/jas/sky016
Price, P. T., Nelson, C. M., and Clarke, S. D. (2000). Omega-3 polyunsaturated fatty acid regulation of gene expression. Curr. Opin. Lipidol. 11, 3–7. doi: 10.1097/00041433-200002000-00002
Rasby, R. (2007). Early weaning beef calves. Vet. Clin. N. Am. Food Anim. Pract. 23, 29–40. doi: 10.1016/j.cvfa.2007.01.002
Reis, M. M., Cooke, R. F., Cappellozza, B. I., Marques, R. S., Guarnieri Filho, T. A., Rodrigues, M. C., et al. (2015). Creep-feeding to stimulate metabolic imprinting in nursing beef heifers: impacts on heifer growth, reproductive and physiological variables. Animal 9, 1500–1508. doi: 10.1017/S1751731115000828
Robinson, D. L., Café, L. M., and Greenwood, P. L. (2013). Meat science and muscle biology symposium: developmental programming in cattle: consequences for growth, efficiency, carcass, muscle, and beef quality characteristics. J. Anim. Sci. 91, 1428–1442. doi: 10.2527/jas.2012-5799
Rodrigues, H. D., Kinder, J. E., and Fitzpatrick, L. A. (2002). Estradiol regulation of luteinizing hormone secretion in heifers of two breed types that reach puberty at different ages. Biol. Reprod. 66, 603–609. doi: 10.1095/biolreprod66.3.603
Scheffler, J. M., McCann, M. A., Greiner, S. P., Jiang, H., Hanigan, M. D., Bridges, G. A., et al. (2014). Early metabolic imprinting events increase marbling scores in fed cattle. J. Anim. Sci. 92, 320–324. doi: 10.2527/jas.2012-6209
Schillo, K. K., Hall, J. B., and Hileman, S. M. (1992). Effects of nutrition and season on the onset of puberty in the beef heifer. J. Anim. Sci. 70, 3994–4005. doi: 10.2527/1992.70123994x
Schoonmaker, J. P., Cecava, M. J., Faulkner, D. B., Fluharty, F. L., Zerby, H. N., and Loerch, S. C. (2003). Effect of source of energy and rate of growth on performance, carcass characteristics, ruminal fermentation, and serum glucose and insulin of early-weaned steers. J. Anim. Sci. 81:843–855. doi: 10.2527/2003.814843x
Schubach, K. M., Cooke, R. F., Brandão, A. P., de Sousa, O. A., Schumaher, T. F., Jump, D. B., et al. (2019). Supplementing calcium salts of soybean oil to beef steers early in life to enhance carcass development and quality. J. Anim. Sci. 97, 4182–4192. doi: 10.1093/jas/skz272
Sequeira, M., Pain, S. J., de Brun, V., Meikle, A., Kenyon, P. R., and Blair, H. T. (2016). Gestation-related gene expression and protein localization in endometrial tissue of Suffolk and Cheviot ewes at gestation Day 19, after transfer of Suffolk or Cheviot embryos. Theriogenology 86, 1557–1565. doi: 10.1016/j.theriogenology.2016.05.015
Sexten, W. J., Faulkner, D. B., and Ireland, F. A. (2004). Influence of creep feeding and protein level on growth and maternal performance of replacement beef heifers. Prof. Anim. Sci. 20, 211–217. doi: 10.15232/S1080-7446(15)31303-6
Shamay, A., Werner, D., Moallem, U., Barash, H., and Bruckental, I. (2005). Effect of nursing management and skeletal size at weaning on puberty, skeletal growth rate, and milk production during first lactation of dairy heifers. J. Dairy Sci. 88, 1460–1469. doi: 10.3168/jds.S0022-0302(05)72814-9
Shike, D. W., Faulkner, D. B., Cecava, M. J., Parrett, D. F., and Ireland, F. A. (2007). Effects of weaning age, creep feeding, and type of creep on steer performance, carcass traits, and economics. Prof. Anim. Sci. 23, 325–332. doi: 10.15232/S1080-7446(15)30985-2
Sinclair, K. D., Allegrucci, C., Singh, R., Gardner, D. S., Sebastian, S., Bispham, J., et al. (2007). DNA methylation, insulin resistance, and blood pressure in offspring determined by maternal periconceptional B vitamin and methionine status. Proc. Natl. Acad. Sci. 104, 19351–19356. doi: 10.1073/pnas.0707258104
Snowder, G. D., Van Vleck, L. D., Cundiff, L. V., and Bennett, G. L. (2006). Bovine respiratory disease in feedlot cattle: environmental, genetic, and economic factors. J. Anim. Sci. 84, 1999–2008. doi: 10.2527/jas.2006-046
Soberon, F., and Van Amburgh, M. E. (2013). Lactation biology symposium: the effect of nutrient intake from milk or milk replacer of preweaned dairy calves on lactation milk yield as adults: a meta-analysis of current data. J. Anim. Sci. 91, 706–712. doi: 10.2527/jas.2012-5834
Spicer, L. J., and Echternkamp, S. E. (1995). The ovarian insulin and insulin-like growth factor system with an emphasis on domestic animals. Domest. Anim. Endocrinol. 12, 223–245. doi: 10.1016/0739-7240(95)00021-6
Srinivasan, M., Mitrani, P., Sadhanandan, G., Dodds, C., Shbeir-ElDika, S., Thamotharan, S., et al. (2008). A high-carbohydrate diet in the immediate postnatal life of rats induces adaptations predisposing to adult-onset obesity. J. Endocrinol. 197, 565–574. doi: 10.1677/JOE-08-0021
Srinivasan, M., and Patel, M. S. (2008). Metabolic programming in the immediate postnatal period. rends in Endocrinol. Metab. 19, 146–152. doi: 10.1016/j.tem.2007.12.001
Stickland, N. C. (1978). A quantitative study of muscle development in the bovine foetus (Bos indicus). Anat. Histol. Embryol. 7, 193–205. doi: 10.1111/j.1439-0264.1978.tb00795.x
Tarr, S. L., Faulkner, D. B., Buskirk, D. D., Ireland, F. A., Parrett, D. F., and Berger, L. L. (1994). The value of creep feeding during the last 84, 56, or 28 days prior to weaning on growth performance of nursing calves grazing endophyte-infected tall fescue. J. Anim. Sci. 72:1084–1094. doi: 10.2527/1994.7251084x
Thoennes, S. R., Tate, P. L., Price, T. M., and Kilgore, M. W. (2000). Differential transcriptional activation of peroxisome proliferator-activated receptor gamma by omega-3 and omega-6 fatty acids in MCF-7 cells. Mol. Cell Endocrinol. 160, 67–73. doi: 10.1016/S0303-7207(99)00254-3
Tipton, J. E., Lewis, L. K., Ricks, R. E., Maresca, S., Lopez Valiente, S., and Long, N. M. (2020). The effects of age at weaning and length of lipid supplementation on growth, metabolites, and marbling of young steers. Animals 10:1819. doi: 10.3390/ani10101819
USDA (1997). United States standards for grades of carcass beef . Washington, DC: Agricultural Marketing Service, USDA.
Vendramini, J. M. B., Sollenberger, L. E., Dubeux, J. C. B., Interrante, S. M., Stewart, R. L., and Arthington, J. D. (2006). Concentrate supplementation effects on forage characteristics and performance of early weaned calves grazing rye–ryegrass pastures. Crop Sci. 46, 1595–1600. doi: 10.2135/cropsci2005.11-0419
Waterland, R. A., and Garza, C. (1999). Potential mechanisms of metabolic imprinting that lead to chronic disease. Am. J. Clin. Nutr. 69, 179–197. doi: 10.1093/ajcn/69.2.179
Waterman, R. C., Geary, T. W., Paterson, J. A., and Lipsey, R. J. (2012a). Early weaning in Northern Great Plains beef cattle production systems: II. development of replacement heifers weaned at 80 or 215d of age. Livest. Sci. 148, 36–45. doi: 10.1016/j.livsci.2012.04.020
Waterman, R. C., Geary, T. W., Paterson, J. A., Lipsey, R. J., Shafer, W. R., Berger, L. L., et al. (2012b). Early weaning in Northern Great Plains beef cattle production systems: III. Steer weaning, finishing and carcass characteristics. Livest. Sci. 148, 282–290. doi: 10.1016/j.livsci.2012.06.024
Wathes, D. C., Cheng, Z., Fenwick, M. A., Fitzpatrick, R., and Patton, J. (2011). Influence of energy balance on the somatotrophic axis and matrix metalloproteinase expression in the endometrium of the postpartum dairy cow. Reproduction 141, 269–281. doi: 10.1530/REP-10-0177
Winick, M., and Noble, A. (1966). Cellular response in rats during malnutrition at various ages. J. Nutr. 89, 300–306. doi: 10.1093/jn/89.3.300
Wu, G., Bazer, F. W., Wallace, J. M., and Spencer, T. E. (2006). Board-invited review: intrauterine growth retardation: implications for the animal sciences. J. Anim. Sci. 84, 2316–2337. doi: 10.2527/jas.2006-156
Xu, H. E., Lambert, M. H., Montana, V. G., Parks, D. J., Blanchard, S. G., Brown, P. J., et al. (1999). Molecular recognition of fatty acids by peroxisome proliferator–activated receptors. Mol. Cell 3, 397–403. doi: 10.1016/S1097-2765(00)80467-0
Yan, X., Zhu, M.-J., Dodson, M. V., and Du, M. (2013). Developmental programming of fetal skeletal muscle and adipose tissue development. J. Genomics 1, 29–38. doi: 10.7150/jgen.3930
Keywords: beef cattle, early weaning, metabolic imprinting, production, supplementation
Citation: Harvey KM, Cooke RF and Moriel P (2021) Impacts of Nutritional Management During Early Postnatal Life on Long-Term Physiological and Productive Responses of Beef Cattle. Front. Anim. Sci. 2:730356. doi: 10.3389/fanim.2021.730356
Received: 24 June 2021; Accepted: 26 August 2021;
Published: 16 September 2021.
Edited by:
Jimena Laporta, University of Wisconsin-Madison, United StatesReviewed by:
M. Sofia Ortega, University of Missouri, United StatesPedro Fontes, University of Georgia, United States
Copyright © 2021 Harvey, Cooke and Moriel. This is an open-access article distributed under the terms of the Creative Commons Attribution License (CC BY). The use, distribution or reproduction in other forums is permitted, provided the original author(s) and the copyright owner(s) are credited and that the original publication in this journal is cited, in accordance with accepted academic practice. No use, distribution or reproduction is permitted which does not comply with these terms.
*Correspondence: Reinaldo F. Cooke, reinaldocooke@tamu.edu