- 1Range Cattle Research & Education Center, University of Florida, Ona, FL, United States
- 2Prairie Research Unit, Mississippi State University, Prairie, MS, United States
- 3Department of Animal Science, Texas A&M University, College Station, TX, United States
Maternal nutritional management during gestation appears to modulate fetal development and imprint offspring postnatal health and performance, via altered organ and tissue development and tissue-specific epigenetics. This review highlighted the studies demonstrating how developmental programming could be explored by beef producers to enhance offspring performance (growth, immune function, and reproduction), including altering cow body condition score (BCS) during pregnancy and maternal supplementation of protein and energy, polyunsaturated fatty acids (PUFA), trace minerals, frequency of supplementation, specific amino acids, and vitamins. However, this review also highlighted that programming effects on offspring performance reported in the literature were highly variable and depended on level, duration, timing, and type of nutrient restriction during gestation. It is suggested that maternal BCS gain during gestation, rather than BCS per se, enhances offspring preweaning growth. Opportunities for boosting offspring productive responses through maternal supplementation of protein and energy were identified more consistently for pre- vs. post-weaning phases. Maternal supplementation of specific nutrients (i.e., PUFA, trace minerals, and methionine) demonstrated potential for improving offspring performance, health and carcass characteristics during immunological challenging scenarios. Despite the growing body of evidence in recent years, the complexity of investigating developmental programming in beef cattle production is also growing and potential reasons for current research challenges are highlighted herein. These challenges include: (1) intrinsic difficulty of accurately measuring cow milk production multiple times in cow-calf systems; (2) larger focus on Bos taurus vs. Bos indicus breeds despite the predominance of Bos indicus-influenced beef breeds in tropical/subtropical environments and their specific, and sometimes opposite, physiological and performance outcomes compared to Bos taurus breeds; (3) limited focus on interaction between prenatal and postnatal management; (4) sex-specific outcomes following similar maternal nutrition during gestation; (5) greater focus on nutrient deficiency vs. excess; (6) limited implementation of immunological challenges; and (7) lack of multigeneration and longer periods of offspring evaluation. This review provides multiple evidence that such obstacles need to be overcome in order to significantly advance the scientific knowledge of developmental programming in beef cattle and promote global beef production.
Introduction
Developmental programming is the concept that maternal external stimuli (i.e., maternal nutrition) during critical periods of gestation modulate fetal development leading to long-term consequences on offspring postnatal life (Barker, 1992). Describing all mechanisms underlying developmental programming is beyond the scope of this review and have been previously covered by others (Broadhead et al., 2019; Elolimy et al., 2019; Reynolds et al., 2019; Caton et al., 2020). Briefly, published mechanisms regulating nutritional programming in the beef offspring include altered organ and tissue development (Long et al., 2010) and tissue-specific modifications of transcription factors and gene network (Liu et al., 2020; Diniz et al., 2021a), via methylation (Liu et al., 2020) and post-transcription non-coding microRNA (Moisá et al., 2016). Developmental programming studies analyzing histone modifications in beef progeny are currently lacking. Epigenetic analyses have grown steadily in recent years, but currently, transcriptomics of multiple tissues has been the primary analytical procedure in many beef studies exploring maternal nutritional management during early (Crouse et al., 2019; Liu et al., 2020; Diniz et al., 2021a) and late gestation (Sanglard et al., 2018). For example, maternal energy restriction during early- (Diniz et al., 2021a) and late-gestation (Sanglard et al., 2018; Palmer et al., 2021) altered the transcriptome of numerous nutrient-sensing pathways involved in muscle tissue development and energy metabolism, suggesting a reprogramming of genes in order to adapt the fetus to the altered maternal nutrient availability.
Fetal adaptations to nutritional programming are complex and may lead to either negative or positive consequences on offspring postnatal life. It is suggested that the final outcome to specific in utero nutrition is driven by how maternal nutrition during gestation interacts with the genetic and epigenetic abilities of the embryo to adapt to such nutritional conditions (Caton et al., 2020). Identifying dietary manipulations for pregnant beef females that can improve offspring nutrient utilization efficiency, growth, immune function, and reproduction is crucial to optimize beef production, sustainability, and profitability. This review focuses on numerous applied nutritional strategies for gestating beef cows that can be immediately implemented by beef cattle producers to reprogram postnatal performance of the offspring. More specifically, this review highlights the specific effects of maternal nutrition during first, second, and third trimester of gestation that enhanced postnatal growth, reproduction, and immunocompetence of their beef progeny. For each topic, current research gaps and opportunities for future research are also described below in order to strengthen the existing scientific knowledge and ultimately increase global beef production.
Maternal Malnutrition
Negative energy balance of gestating beef cows is one of the stressors that can be detrimental for placental environment and calf development (Funston et al., 2012) and impact how individuals will respond throughout their lives (Moriel et al., 2016a; Sanglard et al., 2018). Multiple nutritional strategies described herein may directly or indirectly affect cow body condition score (BCS). Cows fed to achieve and sustain BCS of 5.5 (scale of 1–9) during the third trimester of gestation had greater calving and weaning rates and weaned heavier calves compared to cows that maintained a BCS of 4.5 from third trimester of gestation until calving (Bohnert et al., 2013). Cow BCS gain induces positive nutrient flushing and nutrient delivery to the fetus (Wu et al., 2006) and may be used to offset the negative effects of malnutrition during previous gestational periods on fetal development (Long et al., 2009, 2010). Marques et al. (2016a) assigned cows to 1 of 5 nutritional managements during gestation. Cows were managed to maintain a high (HBCS) or low (LBCS) BCS throughout the entire gestation or managed to gain 1.5 units of BCS during the first (BCS1), second (BCS2), and third (BCS3) trimester of gestation and then maintain the resultant BCS until calving. Calving rate, calf birth body weight (BW), cow milk production, weaning rate, and calf age at weaning did not differ among treatments. However, calf weaning BW was greater for calves born from BCS2 and BCS3 vs. HBCS and LBCS cows. Thus, the greater calf preweaning growth of BCS2 and BCS3 cows may be at least partially attributed to cow BCS gain during second and third trimester rather than BCS per se (Marques et al., 2016a).
Energy deficiency (70% of requirements) in primiparous beef cows during the last 100 days of gestation reduced calf BW at birth and weaning by 6.5 and 8.1%, respectively, compared to calves born from cows fed 100% of energy requirements (Corah et al., 1975). In contrast, energy deficiency of similar magnitude (70% of requirements) for a short period (last 40 d of gestation) did not impact calf preweaning growth and post-weaning average daily gain (ADG) during a 45-d preconditioning period (Moriel et al., 2016a). Interestingly, calf birth and weaning BW were not impacted when protein and energy requirements were not met (57 vs. 100% of requirements) during the last 90 days of gestation (Hough et al., 1990). Maternal malnutrition during gestation may also lead to long-lasting effects in carcass characteristics. Protein restriction during early pregnancy had no impact on carcass characteristics of calves compared to no protein restriction (McLean et al., 2018). Contrary, providing diets containing 6% CP crude protein (DM basis) from 132 days of gestation until calving had no impact on offspring backfat thickness and hot carcass weight, but decreased Longissimus muscle area and dressing percentage at slaughter compared to offspring born from cows offered diets containing 12% CP (Maresca et al., 2019). Nutrient-restricted beef cows (70% of energy and protein requirements) from day 45 to 185 of gestation produced offspring with greater adipocyte diameter in subcutaneous, mesenteric, and omental adipose tissues, but reduced muscle fiber diameter, yield grade, and Semitendinosus muscle weight per kg of carcass at slaughter compared to the offspring from cows offered 100% of their energy and protein requirements (Long et al., 2012). Contrary, negative energy status (80% of energy requirements) during second trimester of gestation had no impact on beef offspring carcass weight or dressing percentage but tended to increase 12th rib backfat thickness and USDA yield grade compared to beef calves born from cows that experienced a positive energy status during the second trimester of gestation (Mohrhauser et al., 2015). As demonstrated, the outcomes of nutrient deficiency on offspring growth performance and carcass characteristics are highly variable and likely dependent on slaughter endpoint chosen by each study (i.e., common backfat thickness vs. days on feed) and the level of restriction during gestation (Ramírez et al., 2020). Duration, timing (first, second, and third trimester) and type (energy, protein, or energy + protein) of nutrient restriction also impact calf development differently, which highlights the need for further studies revealing the underlying mechanisms regulating offspring productive responses.
Applied Management Strategies
Protein and Energy Supplementation
Growth and Carcass Quality
Protein and energy supplementation from a wide variety of feedstuffs and forage sources are used to alter cow BCS, either meeting or exceeding fetal nutrient requirements during in utero development. Maternal supplementation of protein and energy during multiple stages of gestation had variable effects on offspring BW at birth (Table 1), with most studies reporting no differences (19 studies) and less studies (14 studies) reporting increased calf birth BW by an average of 3.2 kg (ranging from 0.4 to 10.2 kg) compared to no supplementation. Greater birth BW has been correlated with increasing calving difficulty (Varona et al., 1999). Most studies did not report an increased offspring birth BW following maternal supplementation of protein and energy. It is important to highlight, however, that among the studies reporting increased calf birth BW, very few of them included data regarding calving dystocia and percentage of calves born alive. It is recommended that future studies consider reporting data on calving difficulty to confirm whether maternal gestational nutrition increases the incidence of dystocia.
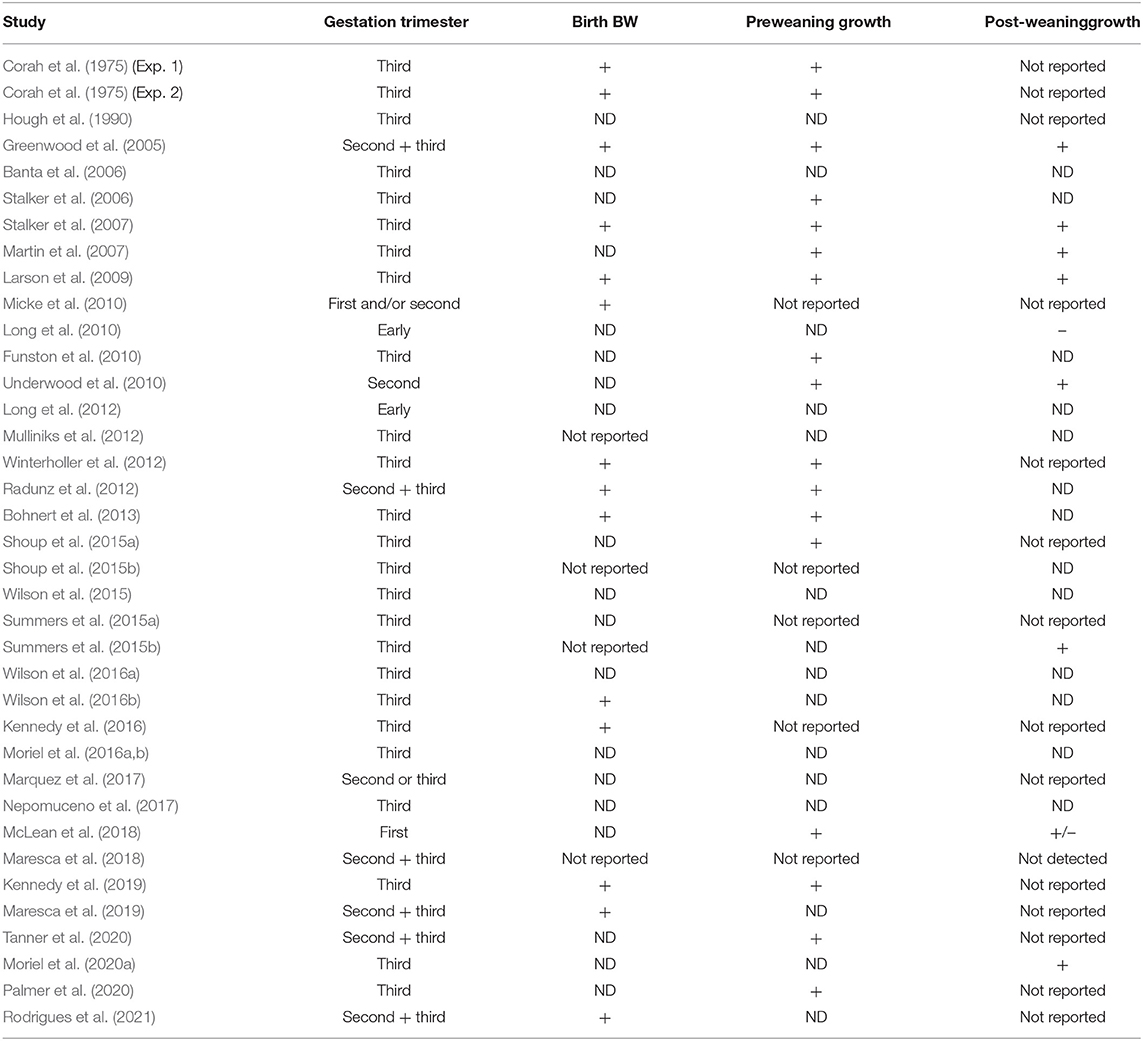
Table 1. Studies (n = 37) reporting positive (+) or negative (–) effects, or no differences (ND; P > 0.10) on beef offspring birth body weight (BW), pre- and post-weaning growth performance following maternal protein and/or energy supplementation during gestation.
Inconsistent results were also observed for calf pre- and post-weaning growth following in utero protein and energy supplementation (Table 1). When cows received supplemental protein and energy during gestation, calf preweaning growth performance increased in 17 studies and did not differ in 15 studies. Calf post-weaning growth increased in 8 studies, did not differ in 13 studies, and decreased in 2 studies. Thus, the calf BW advantage observed at birth and weaning did not consistently carry over during subsequent periods, revealing that calf post-weaning growth was impacted to a lesser extent compared to calf preweaning growth. Cow BCS at the start of the study, different supplementation amount, length, source (grain vs. byproduct), type (energy vs. protein), timing (first, second or third trimester), postnatal malnutrition, prenatal × postnatal nutrition, cow age, and breed, supplement intake behavior, and sire are potential reasons for the inconsistent results summarized in Table 2. Additional research is needed to expand current available data and reveal new mechanisms driving the variable maternal nutrition programming effects on offspring growth.
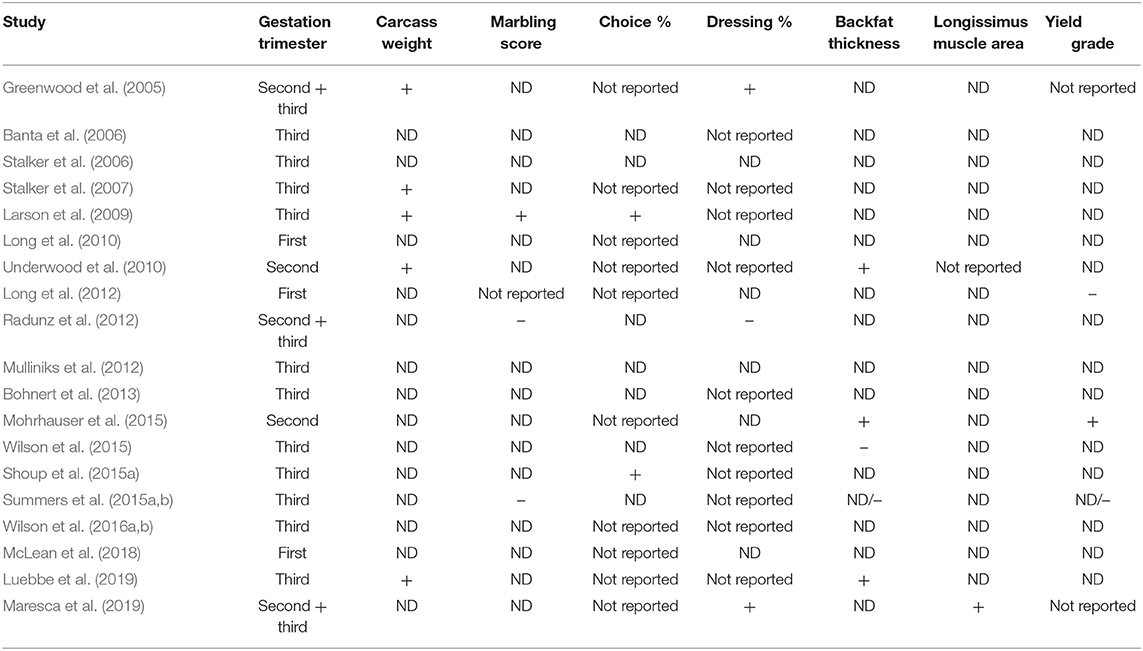
Table 2. Studies (n = 19) reporting positive (+) or negative (–) effects, or no differences (ND; P > 0.10) of maternal protein and/or energy supplementation during gestation on carcass characteristics of beef.
Maternal nutrition during gestation has been shown to alter offspring mRNA expression of genes related to myogenesis and adipogenesis (Sanglard et al., 2018; Liu et al., 2020). However, slightly fewer studies reported any improvement vs. no differences (8 vs. 11 studies, respectively) on carcass characteristics in beef offspring born from cows provided protein and energy supplementation during gestation (Table 2). Differences in feedlot management among all studies, such as days on feed, diet, endpoint at slaughter (days on feed vs. physiological endpoint), and age at slaughter could be potential reasons for the lack of consistent positive long-lasting effects of maternal nutrition on offspring carcass measurements. Despite this inconsistency, Table 2 demonstrates that offspring development and carcass quality could be improved by providing supplemental protein and energy for pregnant beef cows. However, current literature indicates that other factors beyond maternal nutrition during gestation may have a larger role on impacting final offspring performance.
Reproduction
Most reproductive organs initiate their development during early gestation but continue until calving (Cushman and Perry, 2019) and are susceptible to maternal malnutrition (Long et al., 2012). Less emphasis has been placed on the effects of maternal nutrition on subsequent beef progeny reproductive performance despite its potential to increase fertility of the female and male offspring (Cushman and Perry, 2019). Timing of puberty attainment is crucial for optimal heifer reproductive performance (Moriel et al., 2017, 2020b). Heifers that conceive early during their first breeding season have greater lifetime productivity compared to those conceiving later during the breeding season (Cushman et al., 2013). Heifers born from cows offered protein supplementation during late gestation were younger at puberty attainment (Funston et al., 2010) but had similar pregnancy rates compared to cohorts born from non-supplemented cows (Funston et al., 2010; Luebbe et al., 2019). Contrary, maternal supplementation of protein and energy during gestation did not accelerate puberty attainment in other studies (Martin et al., 2007; Cushman et al., 2014; Gunn et al., 2015) but increased overall pregnancy percentages to natural breeding (Martin et al., 2007) and artificial insemination (Gunn et al., 2015), calving percentage (Cushman et al., 2014) and percentage of heifers calving during the first 21 days of the calving season (Martin et al., 2007; Cushman et al., 2014) compared to no maternal supplementation. The results described above suggest that heifer reproductive performance was improved by unknown mechanisms leading to increased fertility rather than earlier puberty attainment. Fertility is related to ovarian characteristics, and ovarian reserve has been used to predict the reproductive performance and lifetime productivity of females (Ireland et al., 2011). Specifically, research has demonstrated heifers born to energy restricted dams had a reduction in antral follicles compared to control cohorts (Sullivan et al., 2009; Mossa et al., 2013). Suboptimal maternal nutrient status also results in disruptions within the neuroendocrine system of the female offspring, leading to altered reproductive function (O'Neil et al., 2019). However, other studies have reported contrasting outcomes to heifer progeny due to long-term exposure to nutrient restriction during gestation (Roberts et al., 2009; Beard et al., 2019). Beard et al. (2019) summarized 46 years of data to determine the effects of precipitation level during key fetal development periods on beef progeny performance. Female progeny gestated during low precipitation throughout gestation were more likely to: (1) remain in the herd and calve after the age of 8 years; (2) produce a calf within the herd after 8 years of age; and (3) produced a greater number of calves while in production compared to average and high precipitation groups. These results indicate that selection of heifers exposed to lower than average precipitation levels in utero may result in increased herd retention and productivity (Beard et al., 2019). Collectively, the findings described herein indicate that maternal nutrition impacts reproductive development of female progeny and the effect of specific maternal nutritional strategies deserves further investigation.
Studies evaluating the effects of maternal gestational nutrition on beef male fertility are rare (Cushman and Perry, 2019). Current data observed altered reproductive organ development (Copping et al., 2018; McCarty et al., 2018), spermatogenesis-related genes (Yang et al., 2014) and reproduction of male progeny (Copping et al., 2018). Cows inserted with melatonin implants during gestation had greater uterine artery blood flow compared to non-implanted cows, explaining the greater body weight at weaning and scrotal circumference of male offspring born from implanted vs. non-implanted beef cows (McCarty et al., 2018). Daily CP supplementation at 0.62 kg/day during periconception period reduced the proportion of seminiferous tubules and blood vessel area at the fetal stage compared to CP supplementation at 1.18 kg/day (Copping et al., 2018). At the adult stage, maternal low protein supplementation reduced blood vessel numbers and semen quality (reduced percentage of normal spermatozoa, sperm density, and motility) and delayed the age at puberty and maturity attainment compared to high protein supplementation (Copping et al., 2018). The in-depth analyses approach of Copping et al. (2018) should be applied during additional gestational periods and different supplementation strategies to expand current knowledge on how developmental programming impacts the reproductive functioning of beef male progeny.
Immune Responses
Development of the immune system in cattle begins during early gestation and continues until early postnatal life (Bell et al., 2005). A robust immunocompetence is crucial to cope with health challenges during production practices (Cooke, 2019a). Maternal energy restriction during gestation did not impact immunoglobulin G concentrations in the colostrum (Hough et al., 1990) or serum (Corah et al., 1975; Stalker et al., 2006; Bohnert et al., 2013; Moriel et al., 2016a; Kennedy et al., 2019). In turn, energy restriction of the dam increased calf serum cortisol concentrations at birth (Hough et al., 1990), neonatal mortality and incidence of scours (Corah et al., 1975), calf preweaning mortality (Stalker et al., 2006), incidence of gastrointestinal and bovine respiratory disease (BRD) in the feedlot (Larson et al., 2009), and impaired humoral immunity following vaccination against BRD pathogens (Moriel et al., 2016a, 2020a). Altered transcriptome and organ formation are potential mechanisms leading to poor immune function measurements following gestational maternal malnutrition. During a vaccination challenge, whole blood mRNA expression of multiple immune response-related genes were downregulated in calves born from cows provided 70 vs. 100% of energy requirements during the last 40 days of gestation (Sanglard et al., 2018). These findings imply that even a moderate, short-term energy restriction during gestation may impair prenatal development of the immune system and suppress postnatal humoral immune function in beef calves (Moriel et al., 2016a, 2020a). These data also corroborate with the greater mortality and BRD incidence in calves born from enery-restricted cows previously observed by others (Corah et al., 1975; Stalker et al., 2006; Larson et al., 2009). Muscle growth and immune function are tightly connected. During an immunological challenge, muscle protein is mobilized (Jahoor et al., 1999) and absorbed amino acids are shifted from growth toward hepatic uptake (Reeds and Jahoor, 2001) to meet the increased nutrient demand for the synthesis of acute-phase proteins, immune cells, and gluconeogenic precursors (Waggoner et al., 2009). Furthermore, mRNA expression of multiple immune function-related genes in the muscle and blood were strongly correlated (Sanglard et al., 2018). Thus, calf immunocompetence should be considered a target system for evaluation in combination with muscle tissue growth in future studies evaluating the effects of maternal nutrition during gestation on offspring performance.
Complete Trace Mineral Salt Supplementation
Growth and Carcass Quality
Trace minerals are essential for the development of fetal muscle (Ohashi et al., 2015), nervous, reproductive, and immune systems (Hostetler et al., 2003). The specific effects of individual trace minerals on progeny performance was described by Emon et al. (2020). In this section, we will focus on studies evaluating whether a complete trace mineral supplement or injectable trace mineral mixture impacted progeny performance. The fetus relies solely on maternal supply of trace minerals during gestation and then primarily on colostrum and milk consumption following birth (Emon et al., 2020). Previous research reported similar calf birth BW when supplementing gestating beef cows with inorganic or organic sources of trace minerals (Stanton et al., 2000; Sprinkle et al., 2006; Marques et al., 2016a). Prenatal supplementation of trace minerals impacted colostrum concentrations of trace elements (Emon et al., 2020) and offspring postnatal growth and heath (Marques et al., 2016b; Harvey et al., 2021a,b). Prenatal supplementation of organic trace minerals increased concentrations of Co and Cu in the cotyledons and concentrations of Co, Cu, and Zn in calf liver at the time of birth, as well as calf BW at weaning and hot carcass weights compared to non-supplemented groups (Marques et al., 2016b). Contrary, supplementation of organic vs. inorganic sources during the last 5 months of gestation did not impact Longissimus muscle mRNA expression of adipogenesis- and myogenesis-related genes and calf pre- and post-weaning growth performance (Harvey et al., 2021a,b). Serial injections of trace minerals during gestation decreased calf birth BW, increased milk production (Stokes et al., 2019a), and increased the percentage of carcases graded as Choice or greater (Shao et al., 2020), but did not affect calf BW at weaning, morbidity, and mortality (Stokes et al., 2019a). Further investigations need to better identify scenarios in which trace mineral source and method of supplementation (injectable vs. loose meal trace minerals) during gestation may or may not improve offspring postnatal performance.
Reproduction
Studies evaluating the reproductive performance of the progeny exposed to different prenatal trace mineral supplementation are scarce. Lamb et al. (2008) observed that source of trace mineral supplementation (no mineral vs. organic and inorganic sources) for at least 45 days prior to embryo collection did not significantly alter embryo number or quality in superovulated purebred Angus heifers provided diet that met the requirement of all trace minerals. Recent evidence, however, indicated that supplementing organic-complexed Co, Cu, Mn, and Zn increased the number of culturable oocytes and transferable embryos from in vitro fertilization (Dantas et al., 2019) and also impacted heifer puberty attainment (Harvey et al., 2021b) compared to inorganic sources. Heifers born from cows supplemented with organic-complexed trace minerals during the last 5 months of gestation had similar post-weaning ADG compared to those born from cows supplemented with inorganic trace minerals. Despite the similar growth performance, puberty attainment was accelerated in heifers born to cows offered organic vs. inorganic sources potentially due to improved ovarian development and protection against reactive oxidative species (Harvey et al., 2021b).
Immune Responses
Progeny immune response can also be modulated by maternal trace mineral nutrition during gestation (Marques et al., 2016b). Multiple injections of trace minerals during gestation decreased basal concentrations of lipopolysaccharide (LPS) binding protein during a LPS challenge (Stokes et al., 2019b) but did not impact calf post-weaning morbidity and mortality rates (Stokes et al., 2019a). Contrary, organic trace mineral supplementation increased offspring plasma cortisol concentrations at weaning and vaccination but decreased calf BRD incidence compared to cohorts born from cows provided inorganic trace minerals and no supplementation (Marques et al., 2016b). Upon weaning, calves born to cows supplemented with organic trace minerals for 5 months before calving had greater hepatic mRNA expression of copper-zinc-superoxide dismutase 1, an enzyme that protects cells from oxidative damage by eliminating reactive oxygen species (Tsang et al., 2014), compared to cohorts born to inorganic supplemented cows. Together, these data suggest that maternal trace mineral nutrition can modulate offspring hepatic metabolism and steroidogenesis required to cope with the stress elicited by weaning. During preconditioning, plasma titers against BRD pathogens and BRD incidence did not differ between steers born from cows offered organic vs. inorganic trace mineral supplementation for 5 months prior to calving (Harvey et al., 2021b). Together, these data indicate that calf immunocompetence may be programmed by gestational trace mineral nutrition in some scenarios (Marques et al., 2016b) while having no impact on calf immune response and health in others (Stokes et al., 2019a,b).
Future research should focus on other gestational periods and on the effects of alternative trace mineral sources and trace elements, as well as on the specific effects of each trace mineral element on progeny growth, reproductive, and immune responses. All of these recommendations should be tested in scenarios with or without trace mineral deficiency during gestation to provide economically feasible recommendations of trace mineral supplementation for gestating beef cows.
Polyunsaturated Fatty Acids (PUFA)
Growth and Carcass Quality
Fatty acid supplementation is a method to modulate crucial physiological processes for cow-calf performance or alter fatty acid composition of beef (Hess et al., 2008). Dietary linolenic acid (ω-3) and ω-6 PUFA derivatives are transferred from maternal circulation to the fetus via placenta (Innis, 2005), regulating muscle and adipocyte cell differentiation and development (Mangrum et al., 2016). Supplementing beef cows during late gestation with Ca salts of ω-3 and ω-6 PUFA (Marques et al., 2017) or ω-6 PUFA (Brandão et al., 2020) improved offspring performance compared to supplements containing primarily saturated fatty acids (SFA). Calf BW at birth and weaning and post-weaning ADG during preconditioning were not impacted by maternal supplementation of Ca salts of ω-3 and ω-6 PUFA (Marques et al., 2017) or ω-6 PUFA (Brandão et al., 2020). However, calves born from cows offered PUFA-enriched supplementation had greater feedlot ADG and increased hot carcass weight, marbling and Longissimus muscle area compared to calves from cows supplemented with SFA (Marques et al., 2017; Brandão et al., 2020). In contrast, late gestation supplementation with Ca salts of ω-3 and ω-6 PUFA decreased steer offspring preweaning growth performance (Shao et al., 2021a) and had no major impacts on feedlot body weight and carcass characteristics, despite the improved feedlot G:F compared to saturated fatty acids supplementation (Shao et al., 2021b). Thus, supplementing Ca salts of PUFA to late gestating beef cows led to variable results on beef offspring productive performance.
Reproduction
The supplementation of PUFA immediately following artificial insemination (AI) has been reported to increase ω-6 fatty acid uptake by maternal and embryonic tissues, leading to greater embryonic interferon-tau synthesis, which is essential for maternal recognition of pregnancy, and pregnancy rates to fixed-time AI in B. indicus and B. taurus beef cows (Cooke, 2019b). Subsequent impacts of maternal PUFA supplementation during gestation on progeny reproduction are yet to be investigated.
Immune Responses
Polyunsaturated fatty acids (ω-3 and ω-6) are key components of cell structure and integrity and play crucial roles in immune system development, maturation, and function (Cooke, 2019a). In beef cattle, less calves required antimicrobial treatment against BRD pathogens after feedlot entry when born from beef cows supplemented with Ca salts of ω-6 PUFA (Brandão et al., 2020) but not when supplemented with ω-3 and ω-6 PUFA (Marques et al., 2017). Despite the shorter transportation distance, the incidence of BRD after feedlot entry was similar in both studies (Marques et al., 2017; Brandão et al., 2020). Hence, a possible explanation for the contrasting results includes the profile of PUFA utilized in both studies, given that Brandão et al. (2020) supplemented a source rich in ω-6 PUFA which are known to participate in immune system development, maturation, and function (Cooke, 2019a). Nonetheless, maternal PUFA supplementation during late gestation may be explored to improve calf immunocompetence after feedlot entry. Additional studies are needed to investigate the main mechanisms by which late gestation supplementation of PUFA led to greater growth and immunocompetence, as well as the impacts of maternal PUFA supplementation during subsequent gestation periods on fetal development and postnatal offspring performance.
Additional Nutritional Strategies
Frequency of Supplementation
Several conditions can create a negative nutrient availability for fetal development (Funston et al., 2012), including altered cow metabolic status caused by infrequent concentrate supplementation. Decreasing the frequency of concentrate supplementation reduces labor and feeding costs of beef cattle while modulating blood concentrations of hormones and metabolites (Moriel et al., 2016b, 2020b). Reducing the frequency of wet brewers grains supplementation, from daily to 3 times weekly during the last 60 days of gestation, did not impact cow BW change and reproduction but led to fluctuations in precalving plasma glucose and insulin concentrations of cows and calf plasma concentrations of haptoglobin and cortisol (Moriel et al., 2016b). These data suggest a greater physiological stress in offspring born from cows supplemented infrequently (Moriel et al., 2016b). However, infrequent supplementation during late gestation did not have carryover effects on postnatal calf growth and post-weaning immunity, likely because the fluctuations in hormones and metabolites were lower than expected due to the slower maternal consumption of wet brewers grains. It remains possible that using dry supplements during gestation would lead to faster maternal supplement intake and more drastic metabolic changes.
Methionine
The periconceptional period is a critical time for the fetus in which placental development and fetal organogenesis occur (Caton et al., 2020). Methyl donors, such as methionine, are crucial for early embryonic development by regulating the synthesis of free radicals and transcriptome (Palmer et al., 2021) via DNA methylation or histone modifications (Crouse et al., 2020). Metabolizable methionine supplementation to lactating beef cows during a 115-day periconception period altered muscle transcriptome (Palmer et al., 2021) and methylome of calves (Liu et al., 2020), programming their postnatal performance (Silva et al., 2021). Cows offered supplemental methionine tended to produce more energy-corrected milk without increasing calf 205-day adjusted weaning BW than a control supplement without methionine inclusion (Silva et al., 2021). However, during a 42-day post-weaning metabolism evaluation, calves born from dams supplemented with methionine had greater ADG and gain:feed than control calves (Silva et al., 2021) corroborating the alterations in muscle transcriptomics and metabolomics (Liu et al., 2020). Methionine calves also tended to have greater apparent total tract NDF and ADF digestibility and lesser plasma glucose concentrations than control calves (Silva et al., 2021).
Maternal supplementation of methionine during the third trimester of gestation altered calf muscle transcriptome (Palmer et al., 2021) but did not impact offspring pre- and post-weaning growth (Clements et al., 2017; Moriel et al., 2020a; Palmer et al., 2020) or post-vaccination measurements of innate and humoral immune functions (Moriel et al., 2020a) compared to supplementation without methionine inclusion. Muscle protein deposition in growing calves does not increase once amino acid supply exceed requirements (Froidmont et al., 2000). Hence, methionine deficiency likely did not occur in the studies described above. Oversupplying methionine during third trimester may have not been sufficient to modulate calf postnatal growth and immune responses. Cattle subspecies, final metabolizable protein intake (combined outcome of forage quality and forage and supplement intake) and lack of methionine deficiency in some grazing scenarios, as well as source, amount, timing and duration of methionine supplementation (and potentially an interaction among all of these factors) are major explanations for these inconsistent results. Further research is necessary to confirm this rationale.
Vitamins
Studies focusing exclusively on vitamin nutrition of pregnant beef cows and its consequences to offspring development are limited. Recent evidence demonstrated the opportunity to modify placental and fetal development via maternal supplementation with vitamins + minerals (VTM) during early gestation. Maternal VTM supplementation increased fetal liver size (but not fetal and gravid uterus size) and altered placental expression of genes related to energy metabolism, hormone synthesis, and nutrient transport (Diniz et al., 2021b), as well as concentrations of amino acids in maternal serum and fetal fluids (Diniz et al., 2021b; Menezes et al., 2021). Further studies exploring productive responses of offspring born from beef cows offered or not a complete vitamin mixture or individual vitamins to meet or exceed vitamin requirements during gestation are needed.
Discussion
The studies reported herein demonstrate an unbalanced focus on maternal protein and energy supplementation compared to individual nutrients (i.e., trace minerals, PUFA, and specific amino acids). Inconsistent results within each topic were also evident. In addition to the explanations already discussed, it remains possible that the impact of maternal nutrition on offspring epigenetics differed widely among those studies, highlighting the need for a greater focus on multiple epigenetic analyses (methylation, histone modifications, and microRNA) to better integrate the transcriptomics data with phenotypic traits in beef cattle. Despite the growing evidence published in recent years, the complexity of investigating developmental programming in beef cattle production also increased and are highlighted below.
Cow Milk Production
One of these challenges is separating the confounding effects of developmental programming and cow milk production to explain the improved calf pre-weaning growth performance. Cow milk production increased in some (Exp. 2 in Corah et al., 1975; Kennedy et al., 2019) but not all studies (Exp. 1 in Corah et al., 1975; Banta et al., 2006; Shoup et al., 2015a; Wilson et al., 2015, 2016a,b; Marques et al., 2016b; Shao et al., 2021b) following improved maternal prepartum nutrition. Although developmental programming effects on calf growth may have occurred regardless of dam milk production, the limitations of the methodology of milk estimation (i.e., weigh-suckle-weigh) and low number of days of milk yield evaluation partially explain the variable milk production data. Weigh-suckle-weigh method consists of weighing calves immediately before and after milk consumption, which combined with gut fill effects, leads to large variations in the calculations of daily milk production and lower likelihood of detecting treatment effects. Due to obvious laborious management limitations (i.e., distance between pastures and weighing facilities), milk production estimates are often evaluated at a single or few time points throughout lactation, and most likely do not accurately determine the total cow milk yield and lactation curve persistence. Therefore, enhanced total milk yield of beef cows providing better nutrition during gestation should not be ruled out in some developmental programming studies. Nonetheless, calf preweaning growth can be enhanced by promoting maternal BCS gain during the second and third trimesters of gestation (Marques et al., 2016a) directly via increased nutrient delivery to the developing fetus (Wu et al., 2006) or possibly via greater total milk yield of cows.
B. indicus vs. B. taurus
Most beef production increase is expected to come from tropical/subtropical regions to meet the rising global beef demand (Cooke et al., 2020). Nearly 45% of beef cows in U.S.A. are located in southern/southeastern states where Bos indicus-influenced cattle predominate (NASS, 2017). Under similar management, B. indicus-influenced cattle displayed different behavior and energy requirements (Cooke et al., 2020), trace mineral metabolism (Ranches et al., 2021), pregnancy loss (Fontes et al., 2019), and opposite physiology and reproduction (Piccolo et al., 2018; Moriel et al., 2019) compared to B. taurus breeds. Most studies described herein utilized B. taurus beef cows to investigate the impact of multiple maternal gestational nutrition on offspring growth. Recently, few studies began addressing this research gap in nutritional programming of B. indicus (Nepomuceno et al., 2017; Costa et al., 2021; Rodrigues et al., 2021) and B. indicus-influenced beef offspring (Micke et al., 2010; Brandão et al., 2020; Copping et al., 2020; Liu et al., 2020; Moriel et al., 2020a; Palmer et al., 2020; Harvey et al., 2021a,b; Silva et al., 2021). Nonetheless, limited research in beef cattle with B. indicus genetics is an excellent opportunity for future investigations.
Prenatal × Postnatal Nutrition
Growing data in beef cattle indicate that maternal nutrition during gestation interacts with early postnatal nutrition to determine the final outcome to offspring performance (McLean et al., 2018; Cardoso et al., 2020; Copping et al., 2020), which partially explains the variable results of maternal nutrition on beef offspring performance. Beef cows were assigned to treatments in a 2 × 2 factorial arrangement and provided either a control (CON) or restricted (RES) protein supplement during prenatal and postnatal periods (McLean et al., 2018). Calf ADG and BW at weaning increased for RES-CON vs. CON-CON calves but decreased for RES-RES vs. CON-RES calves, suggesting that prenatal protein restriction increased efficiency of nutrient utilization in RES-CON calves and increased their growth performance when postnatal nutrition was adequate (McLean et al., 2018). Postnatal nutrition of heifers had greater impacts on age at puberty attainment of beef heifers compared to maternal nutrition. However, heifers born from cows that experienced nutrient restriction during gestation were more sensitive to the detrimental effects of poor postnatal heifer nutrition and growth (Cardoso et al., 2020). It is crucial, therefore, that future studies consider both prenatal and postnatal nutritional environments to interpretate offspring performance exposed to different maternal nutrition during gestation.
Sex-Specific Responses
Long-term beef offspring performance can be affected by maternal gestational nutrition in a sex-specific manner. Protein restriction during the first trimester of gestation increased post-weaning BW of male calves but decreased post-weaning BW and hot carcass weights of females compared to meeting protein requirements during first trimester of gestation (Micke et al., 2010). Sexual dimorphic responses were also observed in circulating concentrations of insulin-like growth factor 1 and thyroid hormones following maternal protein restriction during early gestation (Micke et al., 2010, 2015). Likewise, maternal supplementation of Ca salts of PUFA during late gestation increased feedlot ADG and hot carcass weight in steers but not heifers (Brandão et al., 2020). These results underscore the importance of evaluating which maternal supplementation strategies induce or not sex-dependent programming effects on offspring performance. Such knowledge will enhance beef production efficiency by allowing the strategic implementation of maternal gestational nutrition targeted to promote offspring growth, reproduction, and health, according to calf sex and specific needs of the commercial operation.
Nutrient Restriction vs. Excess
Greater number of studies focused on nutrient restriction vs. excess in beef cattle. Although nutrient deficiency is often experienced at some point during gestation (i.e., cyclic BCS gain and loss of beef cows), periods of nutrient overconsumption also occurs due to seasonal changes to forage nutritive value and herbage mass. In addition, timing of nutrient restriction during gestation can vary widely due to differences in management systems (i.e., fall vs. spring calving seasons). Thus, it is imperative that each supplementation strategy for gestating beef cows is evaluated in situations of nutrient deficiency and excess and different management systems to document its specific effects of progeny performance and identify optimal nutritional strategies for each scenario.
Immunological Challenges
Certain developmental programming effects may not be evident without an external challenge (i.e., immunological challenge). Muscle growth and immunity are strongly connected (Sanglard et al., 2018). Immunological challenges elicit an acute phase response, decreasing feed intake and intensifying protein mobilization to support the immune system, which combined leads to compromised growth (Jahoor et al., 1999). For instance, effects of maternal supplementation of protein and energy during late gestation were detected for calf ADG immediately after a vaccination challenge against BRD pathogens but not during pre-vaccination period (Moriel et al., 2020a). Likewise, maternal supplementation of organic trace minerals did not impact pre-weaning BRD incidence, but significantly reduced BRD incidence during stressful events such as weaning and feedlot entry compared to inorganic trace mineral supplementation (Marques et al., 2016b). Additional studies utilizing comprehensive evaluations during a wide variety of immunological challenges are crucial to unravel the complex developmental programing mechanisms leading to suppressed immunity and increased mortality of beef calves.
Longer and Multigeneration Evaluations
Another downfall highlighted herein is that less studies focused on longer vs. shorter periods of offspring evaluations (for example, see Table 2). This approach is sometimes necessary but obviously not optimal as differences in offspring performance might be observed only during longer vs. shorter postnatal evaluation periods (Brandão et al., 2020; Silva et al., 2021). In addition, stressors experienced during gestation may elicit opposite outcomes to offspring performance during shorter vs. longer periods of evaluation. For example, a retrospective study investigated the effects of precipitation during key stages of gestation on progeny performance (Beard et al., 2019). Calves experiencing low precipitation during gestation were lighter at birth and weaning but had greater longevity and percentage of females calving after 8 years of age compared to those experiencing high precipitation levels during gestation (Beard et al., 2019). Thus, it is plausible that some maternal gestational nutrition-induced benefits to offspring performance reported herein may not be long-lasting throughout the remaining productive life of the progeny. This pitfall is even more striking when we consider beef cattle studies investigating the effects of developmental programming in multiple generations (i.e., F1 daughter and F2 granddaughters) as recently reported in dairy cattle (Laporta et al., 2020). Using 10 years of consecutive data collection, Laporta et al. (2020) observed that maternal heat stress during late gestation decreased milk production of daughters during first, second and third lactations, as well as milk production of granddaughters during their first lactation period compared to maternal heat abatement during gestation. Similar effects might also occur in beef cattle production.
Additional Factors
In addition to all factors described above, effects of sire and supplement intake variability among cows utilized by each study likely contributed to the inconsistent results in the literature. In multiple research studies and commercial operations, herds are often placed with multiple sires during the breeding season, and consequently generate confounding effects of sire- vs. maternal gestational diet-induced programming effects. Very few studies have explored the extent to which paternal contributions, rather than maternal gestational diet, explains the observed differences on offspring postnatal performance. Greater insight into paternal contributions to fetal development, epigenetic status and potential offspring programming mechanisms are warranted to address this parental- vs. maternal-programming focus imbalance (Lucas and Watkins, 2017).
The reported effectiveness of supplementation programs on grazing cattle performance has been inconsistent, which may me largely attributed to variation in supplement intake behavior by individual cows (DelCurto et al., 2000; Wyffels et al., 2019). Cattle exhibiting rapid rates of supplement consumption had greater body weight gains and calf weaning weights compared to cohorts slower supplement consumption rates (Wesley et al., 2012). Therefore, it is possible that the inconsistent results observed for maternal supplementation of protein and energy reported herein may be associated with behavioral differences on supplement intake among cows, and its consequent effects on fetal development, programming, and postnatal performance.
Lastly, it is also important to highlight to that multiple studies currently available in the literature were conducted in a single vs. multiple years, and sometimes with a relatively small number of animals in each herd which do not mimic the scenarios/challenges experienced by commercial cow-calf operations. Seasonal variation in wheather conditions and its consequences to animal nutrient rerquirements and forage availability and nutritional composition should be taken into account and likely partially explain the inconsistent results on progeny performance. Multiple years of evaluation would better determine when a specific maternal gestational supplementation strategy is expected to enhance progeny performance. These limitations remain as a concern and should be addressed in future studies.
Conclusions
Maternal nutrition during gestation in beef cattle could be explored by beef producers in order to enhance offspring growth, immune function, and reproduction. Offspring outcomes to previous maternal nutrition are variable and depended primarily on timing, magnitude, and type of nutrient restriction or excess. In addition, less studies continued the offspring evaluation after weaning. Nonetheless, increasing maternal BCS during second and third trimester of gestation, and prepartum maternal supplementation of protein and energy, polyunsaturated fatty acids, and trace minerals positively impacted multiple aspects of beef cattle performance. Maternal supplementation of protein and energy during gestation enhanced offspring growth more consistently during preweaning compared to post-weaning phases. Beef progeny reproduction and immunocompetence were also improved by supplemental protein and energy during gestation compared to non-supplemented groups. Although few studies explored the effects of specific nutrients, such as PUFA, trace minerals, and methionine, existing evidence revealed their positive effects on offspring growth, measurements of innate and humoral immune responses and carcass characteristics. Current research challenges and opportunities identified herein included the need to improve cow milk production evaluations, limited data available for beef cattle breeds with Bos indicus genetic contribution, reduced investigations on the interaction between pre- and postnatal calf nutrition, sex-specific outcomes following similar maternal nutrition, less focus on excess nutrient consumption, and lack of evaluations for multiple generations beyond F1 offspring. Nonetheless, this review provides current nutritional strategies that are easy to implement by beef cattle producers. It also describes current pitfalls in nutritional programming of beef cattle that need to be addressed in order to significantly increased beef cattle production worldwide.
Author Contributions
All authors listed have made a substantial, direct and intellectual contribution to the work, and approved it for publication.
Conflict of Interest
The authors declare that the research was conducted in the absence of any commercial or financial relationships that could be construed as a potential conflict of interest.
The handling editor JL declared a past co-authorship with one of the authors EP at the time of review.
Publisher's Note
All claims expressed in this article are solely those of the authors and do not necessarily represent those of their affiliated organizations, or those of the publisher, the editors and the reviewers. Any product that may be evaluated in this article, or claim that may be made by its manufacturer, is not guaranteed or endorsed by the publisher.
References
Banta, J. P., Lalman, D. L., Owens, F. N., Krehbiel, C. R., and Wettemann, R. P. (2006). Effects of interval-feeding whole sunflower seeds during mid to late gestation on performance of beef cows and their progeny. J. Anim. Sci. 84, 2410–2417. doi: 10.2527/jas.2005-400
Beard, J. K., Silver, G. A., Scholljegerdes, E. J., and Summers, A. F. (2019). The effect of precipitation received during gestation on progeny performance in Bos indicus influenced beef cattle. Transl. Anim. Sci. 3, 256–262. doi: 10.1093/tas/txy139
Bell, A. W., Greenwood, P. L., and Ehrhardt, R. A. (2005). “Regulation of metabolism and growth during prenatal growth,” in Biology of Metabolism in Growing Animals, eds D. G. Burrin and H. J. Mersmann (Edinburgh: Elsevier Limited), 7–31.
Bohnert, D. W., Stalker, L. A., Mills, R. R., Nyman, A., Falck, S. J., and Cooke, R. F. (2013). Late gestation supplementation of beef cows differing in BCS: effects on cow and calf performance. J. Anim. Sci. 91, 5485–5491. doi: 10.2527/jas.2013-6301
Brandão, A. P., Cooke, R. F., Schubach, K. M., Rett, B., Souza, O. A., Schachtschneider, C. L., et al. (2020). Supplementing Ca salts of soybean oil to late-gestating beef cows: impacts on performance and physiological responses of the offspring. J. Anim. Sci. 98, 1–12. doi: 10.1093/jas/skaa278.353
Broadhead, D., Mulliniks, J. T., and Funston, R. N. (2019). Developmental programming in a beef production system. Vet. Clin. Food Anim. 35, 379–390. doi: 10.1016/j.cvfa.2019.02.011
Cardoso, R. C., West, S. N., Maia, T. S., Alves, B. R. C., and Williams, G. L. (2020). Nutritional control of puberty in the bovine female: prenatal and early postnatal regulation of the neuroendocrine system. Domestic Animal Endocrinol. 73:106434. doi: 10.1016/j.domaniend.2020.106434
Caton, J. S., Crouse, M. S., McLean, K. J., Dahlen, C. R., Ward, A. K., Cushman, R. A., et al. (2020). Maternal periconceptual nutrition, early pregnancy, and developmental outcomes in beef cattle. J. Anim. Sci. 98, 1–16. doi: 10.1093/jas/skaa358
Clements, A. R., Ireland, F. A., Freitas, T., Tucker, H., and Shike, D. W. (2017). Effects of supplementing methionine hydroxy analog on beef cow performance, milk production, reproduction, and preweaning calf performance. J. Anim. Sci. 95, 5597–5605. doi: 10.2527/jas2017.1828
Cooke, R. F. (2019a). Effects on animal health and immune function. Vet. Clin. Food Anim. Prac. 35, 331–341. doi: 10.1016/j.cvfa.2019.02.004
Cooke, R. F. (2019b). Early career achievement award: supplementing omega-6 fatty acids to enhance early embryonic development and pregnancy establishment in Bos indicus and B. taurus beef cows. J. Anim. Sci. 97, 485–495. doi: 10.1093/jas/sky414
Cooke, R. F., Daigle, C. L., Moriel, P., Smith, S. B., Tedeschi, L. O., and Vendramini, J. M. B. (2020). Cattle adapted to tropical and subtropical environments: social, nutritional, and carcass quality considerations. J. Anim. Sci. 98, 1–20. doi: 10.1093/jas/skaa014
Copping, K. J., Hernandez-Medrano, J., Hoare, A., Hummitzsch, K., McMillen, I. C., Morrison, J. L., et al. (2020). Maternal periconceptional and first trimester protein restriction in beef heifers: effects on placental parameters and fetal and neonatal calf development. Repro. Fert. Dev. 32, 495–507. doi: 10.1071/RD19017
Copping, K. J., Ruiz-Diaz, M. D., Rutland, C. S., Mongan, N. P., Callaghan, M. J., McMillen, I. C., et al. (2018). Peri-conception and first trimester diet modifies reproductive development in bulls. Reprod. Fert. Develop. 30, 703–720. doi: 10.1071/RD17102
Corah, L. R., Dunn, T. G., and Kaltenbach, C. C. (1975). Influence of prepartum nutrition on the reproductive performance of beef females and the performance of their progeny. J. Anim. Sci. 41, 819–824. doi: 10.2527/jas1975.413819x
Costa, T. C., Du, M., Nascimento, K. B., Galvão, M. C., Meneses, J. A. M., Schultz, E. B., et al. (2021). Skeletal muscle development in postnatal beef cattle resulting from maternal protein restriction during mid-gestation. Animals 11:860. doi: 10.3390/ani11030860
Crouse, M. S., Caton, J. S., and Ward, A. K. (2020). Micronutrients, one-carbon metabolism, and epigenetics: potential developmental and production outcomes 2020 ASAS-CSAS- WSASAS Virtual Annual Meeting. J. Anim. Sci. 98:390. doi: 10.1093/jas/skaa278.312
Crouse, M. S. J. S, Caton, R. A., Cushman, K. J., McLean, C. R., Dahlen, P. P., Borowicz, L. P., et al. (2019). Moderate nutrient restriction of beef heifers alters expression of genes associated with tissue metabolism, accretion, and function in fetal liver, muscle, and cerebrum by day 50 of gestation. Transl. Anim. Sci. 3, 855–866. doi: 10.1093/tas/txz026
Cushman, R. A., Kill, L. K., Funston, R. N., Mousel, E. M., and Perry, G. A. (2013). Heifer calving date positively influences calf weaning weights through six parturitions. J. Anim. Sci. 91, 4486–4491. doi: 10.2527/jas2013-6465
Cushman, R. A., McNeel, A. K., and Freetly, H. C. (2014). The impact of cow nutrient status during the second and third trimesters on age at puberty, antral follicle count, and fertility of daughters. Livestock Sci. 162, 252–258. doi: 10.1016/j.livsci.2014.01.033
Cushman, R. A., and Perry, G. A. (2019). Developmental programming of fertility in livestock. Vet. Clin. Food Anim. 35, 321–330. doi: 10.1016/j.cvfa.2019.02.003
Dantas, F. G., Reese, S. T., Filho, R. V. O., Carvalho, R. S., Franco, G. A., Abbott, C. R., et al. (2019). Effect of complexed trace minerals on cumulus-oocyte complex recovery and in vitro embryo production in beef cattle. J. Anim. Sci. 97, 1478–1490. doi: 10.1093/jas/skz005
DelCurto, T., Hess, B., Huston, J., and Olson, K. (2000). Optimum supplementation strategies for beef cattle consuming low-quality roughages in the western United States. J. Anim. Sci. 77, 1–16. doi: 10.2527/jas2000.77E-Suppl1v
Diniz, W. J. S., Crouse, M. S., Cushman, R. A., McLean, K. J., Caton, J. S., Dahlen, C. R., et al. (2021a). Cerebrum, liver, and muscle regulatory networks uncover maternal nutrition effects in developmental programming of beef cattle during early pregnancy. Sci. Rep. 11:2271. doi: 10.1038/s41598-021-82156-w
Diniz, W. J. S., Reynolds, L. P., Borowicz, P. P., Ward, A. K., Sedivec, K. K., McCarthy, K. L., et al. (2021b). Maternal vitamin and mineral supplementation and rate of maternal weight gain affects placental expression of energy metabolism and transport-related genes. Genes 12:385. doi: 10.3390/genes12030385
Elolimy, A., Vailati-Riboni, M., Liang, Y., and Loor, J. J. (2019). Cellular mechanisms and epigenetic changes: role of nutrition in livestock. Vet. Clin. North. Am. Food Anim. Prac. 35, 249–263. doi: 10.1016/j.cvfa.2018.12.001
Emon, M. V., Sanford, C., and McCoski, S. (2020). Impacts of bovine trace mineral supplementation on maternal and offspring production and health. Animals 10:2404. doi: 10.3390/ani10122404
Fontes, P. L. P., Oosthuizen, N., Ciriaco, F. M., Sanford, C. D., Canal, L. B., Pohler, K. G., et al. (2019). Impact of fetal vs. maternal contributions of Bos indicus and Bos taurus genetics on embryonic and fetal development. J. Anim. Sci. 97, 1645–1655. doi: 10.1093/jas/skz044
Froidmont, E., Beckers, Y., and Thewis, A. (2000). Determination of the methionine requirement of growing double-muscled Belgian blue bulls with a three-step method. J. Anim. Sci. 78, 233–241. doi: 10.2527/2000.781233x
Funston, R. N., Martin, J. L., Adams, D. C., and Larson, D. M. (2010). Winter grazing system and supplementation of beef cows during late gestation influence heifer progeny. J. Anim. Sci. 88, 4094–4101. doi: 10.2527/jas.2010-3039
Funston, R. N., Summers, A. F., and Roberts, A. J. (2012). Implications of nutritional management for beef cow-calf systems. J. Anim. Sci. 90, 2301–2307. doi: 10.2527/jas.2011-4568
Greenwood, P., Café, L., Hearnshaw, H., and Hennessy, D. (2005). Consequences of nutrition and growth retardation early in life for growth and composition of cattle and eating quality of beef. Recent Adv. Anim. Nutr. Australia. 15, 183–195. Available online at: https://hdl.handle.net/1959.11/10225
Gunn, P. J., Schoonmaker, J. P., Lemenager, R. P., and Bridges, G. A. (2015). Feeding distiller's grains as an energy source to gestating and lactating beef heifers: Impact on female progeny growth, puberty attainment, and reproductive processes. J. Anim. Sci. 93, 746–757. doi: 10.2527/jas.2014-8130
Harvey, K. M., Cooke, R. F., Colombo, E. A., Rett, B., de Sousa, O. A., Harvey, L. M., et al. (2021a). Supplementing organic-complexed or inorganic Co, Cu, Mn, and Zn to beef cows during gestation: physiological and productive response of cows and their offspring until weaning. J. Anim. Sci. 21:95. doi: 10.1093/jas/skab095
Harvey, K. M., Cooke, R. F., Colombo, E. A., Rett, B., de Sousa, O. A., Harvey, L. M., et al. (2021b). Supplementing organic-complexed or inorganic Co, Cu, Mn, and Zn to beef cows during gestation: post-weaning responses of offspring reared as replacement heifers or feeder cattle. J. Anim. Sci. 21:82. doi: 10.1093/jas/skab082
Hess, B. W., Moss, G. E., and Rule, D. C. (2008). A decade of developments in the area of fat supplementation research with beef cattle and sheep. J. Anim. Sci. 86, E188–E204. doi: 10.2527/jas.2007-0546
Hostetler, C. E., Kincaid, R. L., and Mirando, M. A. (2003). The role of essential trace elements in embryonic and fetal development in livestock. Vet. J. 166, 125–139. doi: 10.1016/S1090-0233(02)00310-6
Hough, R. L., McCarthy, F. D., Kent, H. D., Eversole, D. E., and Wahlberg, M. L. (1990). Influence of nutritional restriction during late gestation on production measures and passive immunity in beef cattle. J. Anim. Sci. 68, 2622–2627. doi: 10.2527/1990.6892622x
Innis, S. M. (2005). Essential fatty acid transfer and fetal development. Placenta 26, 70–75. doi: 10.1016/j.placenta.2005.01.005
Ireland, J. J., Smith, G. W., Scheetz, D., Jimenez-Krassel, F., Folger, J. K., Ireland, J. L. H., et al. (2011). Does size matter in females? An overview of the impact of the high variation in the ovarian reserve on ovarian function and fertility, utility of anti-Müllerian hormone as a diagnostic marker for fertility and causes of variation in the ovarian reserve in cattle. Reprod. Fertil. Dev. 23:1. doi: 10.1071/RD10226
Jahoor, F., Wykes, L., Del Rosario, M., Frazer, M., and Reeds, P. J. (1999). Chronic protein undernutrition and an acute inflammatory stimulus elicit different protein kinetic responses in plasma but not in muscle of piglets. J. Nutr. 129, 693–699. doi: 10.1093/jn/129.3.693
Kennedy, V. C., Bauer, M. L., Swanson, K. C., and Vonnahme, K. A. (2016). Supplementation of corn dried distillers grains plus solubles to gestating beef cows fed low-quality forage: I. altered intake behavior, body condition, and reproduction. J. Anim. Sci. 94, 240–247. doi: 10.2527/jas.2015-9615
Kennedy, V. C., Gaspers, J. J., Mordhorst, B. R., Stokka, G. L., Swanson, K. C., Bauer, M. L., et al. (2019). Late gestation supplementation of corn dried distiller's grains plus solubles to beef cows fed a low-quality forage: III. effects on mammary gland blood flow, colostrum and milk production, and calf body weights. J. Anim. Sci. 97, 3337–3347. doi: 10.1093/jas/skz201
Lamb, G. C., Brown, D. R., Larson, J. E., Dahlen, C. R., Dilorenzo, N., Arthington, J. D., et al. (2008). Effect of organic or inorganic trace mineral supplementation on follicular response, ovulation, and embryo pro- duction in superovulated Angus heifers. Anim. Reprod. Sci. 106, 221–231. doi: 10.1016/j.anireprosci.2007.04.007
Laporta, J., Ferreira, F. C., Ouellet, V., Dado-Senn, B., Almeida, A. K., DeVries, A., et al. (2020). Late-gestation heat stress impairs daughter and granddaughter lifetime performance. J. Dairy. Sci. 103, 7555–7568. doi: 10.3168/jds.2020-18154
Larson, D. M., Martin, J. L., Adams, D. C., and Funston, R. N. (2009). Winter grazing system and supplementation during late gestation influence performance of beef cows and steer progeny. J. Anim. Sci. 87, 1147–1155. doi: 10.2527/jas.2008-1323
Liu, L., R., Amorin, P., Moriel, N., DiLorenzo, P., and Lancaster, F. (2020). Differential network analysis of bovine muscle reveals changes in gene coexpression patterns in response to changes in maternal nutrition. BMC Genomics. 21:684. doi: 10.1186/s12864-020-07068-x
Long, N. M., Prado-Cooper, M. J., Krehbiel, C. R., DeSilva, U., and Wettemann, R. P. (2010). Effects of nutrient restriction of bovine dams during early gestation on postnatal growth, carcass and organ characteristics, and gene expression in adipose tissue and muscle. J. Anim. Sci. 88, 3251–3261. doi: 10.2527/jas.2009-2512
Long, N. M., Tousley, C. B., Underwood, K. R., Paisley, S. I., Means, W. J., Hess, B. W., et al. (2012). Effects of early- to mid-gestational undernutrition with or without protein supplementation on offspring growth, carcass characteristics, and adipocyte size in beef cattle. J. Anim. Sci. 90, 197–206. doi: 10.2527/jas.2011-4237
Long, N. M., Vonnahme, K. A., Hess, B. W., Nathanielsz, P. W., and Ford, S. P. (2009). Effects of early gestational undernutrition on fetal growth, organ development, and placentomal composition in the bovine. J. Anim. Sci. 87, 1950–1959. doi: 10.2527/jas.2008-1672
Lucas, E. S., and Watkins, A. J. (2017). “The long-term effects of the periconceptional period on embryo epigenetic profile and phenotype; the paternal role and his contribution, and how males can affect offspring's phenotype/epigenetic profile,” in Periconception in Physiology and Medicine Advances in Experimental Medicine and Biology, eds A. Fazeli and W. V. Holt (Cham: Springer International), 137–154.
Luebbe, K. M., Stalker, L. A., Klopfenstein, T. J., and Funston, R. N. (2019). Influence of weaning date and late gestation supplementation on beef system productivity I: animal performance. Trans. Anim. Sci. 3, 1492–1501. doi: 10.1093/tas/txz116
Mangrum, K. S., Tuttle, G., Duckett, S. K., Sell, G. S., Krehbiel, C. R., and Long, N. M. (2016). The effect of supplementing rumen undegradable unsaturated fatty acids on marbling in early-weaned steers. J. Anim. Sci. 94, 833–844. doi: 10.2527/jas.2015-9809
Maresca, S., Valiente, S. L., Rodriguez, A. M., Long, N. M., Pavan, E., and Quintans, G. (2018). Effect of protein restriction of bovine dams during late gestation on offspring postnatal growth, glucose-insulin metabolism and IGF-1 concentration. Livestock Sci. 212, 120–126. doi: 10.1016/j.livsci.2018.04.009
Maresca, S., Valiente, S. L., rodriguez, A. M., Testa, L. M., Long, N. M., Quintans, G. I., et al. (2019). The influence of protein restriction during mid- to late gestation on beef offspring growth, carcass characteristic and meat quality. Meat Sci. 153, 103–108. doi: 10.1016/j.meatsci.2019.03.014
Marques, R. S., Cooke, R. F., Rodrigues, M. C., Brandão, A. P., Schubach, K. M., Lippolis, K. D., et al. (2017). Effects of supplementing calcium salts of polyunsaturated fatty acids to late-gestating beef cows on performance and physiological responses of the offspring. J. Anim. Sci. 95, 5347–5357. doi: 10.2527/jas2017.1606
Marques, R. S., Cooke, R. F., Rodrigues, M. C., Cappellozza, B. I., Mills, R. R., Larson, C. K., et al. (2016b). Effects of organic or inorganic cobalt, copper, manganese, and zinc supplementation to late gestating beef cows on productive and physiological responses of the offspring. J. Anim. Sci. 94, 1215–1226. doi: 10.2527/jas.2015-0036
Marques, R. S., Cooke, R. F., Rodrigues, M. C., Moriel, P., and Bohnert, D. W. (2016a). Impacts of cow body condition score during gestation on weaning performance of the offspring. Livestock Sci. 191, 174–178. doi: 10.1016/j.livsci.2016.08.007
Marquez, D., Paulino, M., Rennó, L., Villadiego, F., Ortega, R., Moreno, D., et al. (2017). Supplementation of grazing beef cows during gestation as a strategy to improve skeletal muscle development of the offspring. Animal 11, 2184–2192. doi: 10.1017/S1751731117000982
Martin, J. L., Vonnahme, K. A., Adams, D. C., Lardy, G. P., and Funston, R. N. (2007). Effects of dam nutrition on growth and reproductive performance of heifer calves. J. Anim. Sci. 85, 841–847. doi: 10.2527/jas.2006-337
McCarty, K. J., Owen, M. P. T., Hart, C. G., Thompson, R. C., Burnett, D. D., King, E. H., et al. (2018). Effect of chronic melatonin supplementation during mid to late gestation on maternal uterine artery blood flow and subsequent development of male offspring in beef cattle. J. Anim. Sci. 96, 5100–5111. doi: 10.1093/jas/sky363
McLean, K. J., Boehmer, B. H., Spicer, L. J., and Wettemann, R. P. (2018). The effects of protein supplementation of fall calving beef cows on pre- and postpartum plasma insulin, glucose and IGF-I, and postnatal growth and plasma insulin and IGF-I of calves. J. Anim. Sci. 96, 2629–2639. doi: 10.1093/jas/sky173
Menezes, A. C. B., McCarthy, K. L., Kassetas, C. J., Baumgaertner, F., Kirsch, J. D., Dorsam, S., et al. (2021). Vitamin and mineral supplementation and rate of gain during the first trimester of gestation affect concentrations of amino acids in maternal serum and allantoic fluid of beef heifers. J. Anim. Sci. 99, 1–10. doi: 10.1093/jas/skab024
Micke, G. C., Sullivan, T. M., Gatford, K. L., Owens, J. A., and Perry, V. E. A. (2010). Nutrient intake in the bovine during early and mid-gestation causes sex-specific changes in progeny plasma IGF-1, liveweight, height and carcass traits. Anim. Reprod. Sci. 121, 208–217. doi: 10.1016/j.anireprosci.2010.05.017
Micke, G. C., Sullivan, T. M., Kennaway, D. J., Hernandez-Medrano, J., and Perry, V. E. A. (2015). Maternal endocrine adaptation throughout pregnancy to nutrient manipulation: Consequences for sexually dimorphic programming of thyroid hormones and development of their progeny. Theriogenolgy 83, 604–615. doi: 10.1016/j.theriogenology.2014.10.022
Mohrhauser, D. A., Taylor, A. R., Underwood, K. R., Pritchard, R. H., Wertz-Lutz, A. E., and Blair, A. D. (2015). The influence of maternal energy status during midgestation on beef offspring carcass characteristics and meat quality. J. Anim. Sci. 93, 786–793. doi: 10.2527/jas.2014-8567
Moisá, S. J., Shike, D. W., Shoup, L., and Loor, J. J. (2016). Maternal plane of nutrition during late-gestation and weaning age alter steer calf longissimus muscle adipogenic microrna and target gene expression. Lipids 51, 123–138. doi: 10.1007/s11745-015-4092-y
Moriel, P., Artioli, L. F. A., Piccolo, M. B., Marques, R. S., Poore, M. H., and Cooke, R. F. (2016b). Frequency of wet brewers grains supplementation during late gestation of beef cows and its effects on offspring postnatal growth and immunity. J. Anim. Sci. 94, 2553–2563. doi: 10.2527/jas.2016-0427
Moriel, P., Cappellozza, B. I., Piccolo, M. B., Cooke, R. F., Miranda, M. F., Batista, L. F. D., et al. (2019). Pre- and post-weaning injections of bovine somatotropin to optimize puberty achievement of Bos indicus beef heifers. Transl. Anim. Sci. 3, 443–455. doi: 10.1093/tas/txy125
Moriel, P., Lancaster, P., Lamb, G. C., Vendramini, J. M. B., and Arthington, J. D. (2017). Effects of post-weaning growth rate and puberty induction protocol on reproductive performance of Bos indicus-influenced beef heifers. J. Anim. Sci. 95, 3523–3531. doi: 10.2527/jas.2017.1666
Moriel, P., Palmer, E., Vedovatto, M., Piccolo, M. B., Ranches, J., Silva, H. M., et al. (2020b). Supplementation frequency and amount modulate post-weaning growth and reproductive performance of Bos indicus-influenced beef heifers. J. Anim. Sci. 98, 1–11. doi: 10.1093/jas/skaa236
Moriel, P., Piccolo, M. B., Artioli, L. F. A., Marques, R. S., Poore, M. H., and Cooke, R. F. (2016a). Short-term energy restriction during late gestation of beef cows decreases postweaning calf humoral immune response to vaccination. J. Anim. Sci. 94, 2542–2552. doi: 10.2527/jas.2016-0426
Moriel, P., Vedovatto, M., Palmer, E. A., Oliveira, R. A., Silva, H. M., Ranches, J., et al. (2020a). Maternal supplementation of energy and protein, but not methionine hydroxy analogue, enhanced postnatal growth and response to vaccination in Bos indicus-influenced beef offspring. J. Anim. Sci. 98, 1–12. doi: 10.1093/jas/skaa123
Mossa, F., Carter, F., Walsh, S. W., Kenny, D. A., Smith, G. W., Ireland, J. L. H., et al. (2013). Maternal undernutrition in cows impairs ovarian and cardiovascular systems in their offspring. Biol. Reprod. 88:107235. doi: 10.1095/biolreprod.112.107235
Mulliniks, J. T., Sawyer, J. E., Mathis, C. P., Cox, S. H., and Petersen, M. K. (2012). Winter protein management during late gestation alters range cow and steer progeny performance. J. Anim. Sci. 90, 5099–5106. doi: 10.2527/jas.2012-5535
NASS (2017). National Agricultural Statistics Service. Washington, DC: Agricultural Statistics Board, USDA.
Nepomuceno, D. D., Pires, A. V., Ferraz, M. V. C. Jr., Biehl, M. V., Gonçalves, J. R. S., Moreira, E. M., et al. (2017). Effect of pre-partum dam supplementation, creep-feeding and post- weaning feedlot on age at puberty in Nellore heifers. Livest. Sci. 195, 58–62. doi: 10.1016/j.livsci.2016.11.008
Ohashi, K., Nagata, Y., Wada, E., Zammit, P. S., Shiozuka, M., and Matsuda, R. (2015). Zinc promotes proliferation and activation of myogenic cells via the PI3K/Akt and ERK signaling cascade. Exp. Cell Res. 333, 228–237. doi: 10.1016/j.yexcr.2015.03.003
O'Neil, M. M., West, S. M., Maia, T. S., Cardoso, R. C., and Williams, G. L. (2019). Effects of maternal nutrition on secretion of leptin in the neonatal heifer and interaction of maternal and postnatal nutrition on age at puberty and postpubertal secretion of luteinizing hormone. J. Anim. Sci. 97, 140–141. doi: 10.1093/jas/skz258.287
Palmer, E. A., Peñagaricano, F., Vedovatto, M., Oliveira, R. A., Field, S. L., Laporta, J. et al. (2021). Effects of maternal gestational diet, with or without methionine, on muscle transcriptome of Bos indicus-influenced beef calves following a vaccine-induced immunological challenge. PLOS ONE 17:253810. doi: 10.1371/journal.pone.0253810
Palmer, E. A., Vedovatto, M., Oliveira, R. A., Gouvea, V., Silva, H. M., Vendramini, J. M. B., et al. (2020). Maternal supplement type and methionine hydroxy analogue fortification T effects on performance of BOS indicus-influenced beef cows and their offspring. Livest. Sci. 240:104176. doi: 10.1016/j.livsci.2020.104176
Piccolo, M. B., Arthington, J. D., Silva, G. M., Lamb, G. C., Cooke, R. F., and Moriel, P. (2018). Preweaning injections of bovine ST enhanced reproductive performance of Bos indicus-influenced replacement beef heifers. J. Anim. Sci. 96, 618–631. doi: 10.1093/jas/sky016
Radunz, A. E., Fluharty, F. L., Relling, A. E., Felix, T. L., Shoup, L. M., Zerby, H. N., et al. (2012). Prepartum dietary energy source fed to beef cows: II. effects on progeny postnatal growth, glucose tolerance, and carcass composition. J. Anim. Sci. 90, 4962–4974. doi: 10.2527/jas.2012-5098
Ramírez, M., Testa, L. M., Valiente, S. L., Latorre, M. E., Long, N. M., Rodriguez, A. M., et al. (2020). Maternal energy status during late gestation: effects on growth performance, carcass characteristics and meat quality of steers progeny. Meat Sci. 164:108095. doi: 10.1016/j.meatsci.2020.108095
Ranches, J., Alves, R., Vedovatto, M., Palmer, E. A., Moriel, P., and Arthington, J. D. (2021). Differences in copper and selenium metabolism between Angus (Bos taurus) and Brahman (Bos indicus) cattle. J. Anim. Sci. 99:48. doi: 10.1093/jas/skab048
Reeds, P. J., and Jahoor, F. (2001). The amino acid requirements of disease. Clin. Nutr. 20, 15–22. doi: 10.1054/clnu.2001.0402
Reynolds, L. P., Borowics, P. P., Caton, J. S., Crouse, M. S., Dahlen, C. R., and Ward, A. K. (2019). Developmental programming of fetal growth and development. Vet. Clin. Nort. Am. Food Anim. Prac. 35, 229–247. doi: 10.1016/j.cvfa.2019.02.006
Roberts, A. J., Geary, T. W., Grings, E. E., Waterman, R. C., and MacNeil, M. D. (2009). Reproductive performance of heifers offered ad libitum or restricted access to feed for a one hundred forty-day period after weaning. J. Anim. Sci. 87, 3043–3052. doi: 10.2527/jas.2008-1476
Rodrigues, L. M., Schoonmaker, J. P., Resende, F. D., Siqueira, G. R., Neto, O. R. M., Gionbelli, M. P., et al. (2021). Effects of protein supplementation on Nellore cows' reproductive performance, growth, myogenesis, lipogenesis and intestine development of the progeny. Anim. Prod. Sci. 61, 371–380. doi: 10.1071/AN20498
Sanglard, L. P., Nascimento, M., Moriel, P., Sommer, J., Ashwell, M., Poore, M. H., et al. (2018). Impact of energy restriction during late gestation on the muscle and blood transcriptome of beef calves after preconditioning. BMC Genom. 19:702. doi: 10.1186/s12864-018-5089-8
Shao, T., Brattain, R. S., and Shike, D. W. (2020). Effects of maternal supplementation with an injectable trace mineral containing copper, manganese, zinc, and selenium on subsequent steer finishing phase performance and carcass characteristics. Animals. 10:2226. doi: 10.3390/ani10122226
Shao, T., Ireland, F., McCann, J. C., and Shike, D. W. (2021a). Effects of supplements differing in fatty acid profile to late gestational beef cows on cow performance, calf growth performance, and mRNA expression of genes associated with myogenesis and adipogenesis. J. Anim. Sci. Biotech. 12:647. doi: 10.1186/s40104-021-00588-w
Shao, T., McCann, J. C., and Shike, D. W. (2021b). Effects of supplements differing in fatty acid profile to late gestational beef cows on steer progeny finishing phase growth performance, carcass characteristics, and mrna expression of myogenic and adipogenic genes. Animals 11:1904. doi: 10.3390/ani11071904
Shoup, L. M., Kloth, A. C., Wilson, T. B., González-Peña, D., Ireland, F. A., Rodriguez-Zas, S., et al. (2015a). Prepartum supplement level and age at weaning: I. effects on pre- and postpartum beef cow performance and calf performance through weaning. J. Anim. Sci. 93, 4926–4935. doi: 10.2527/jas.2014-8564
Shoup, L. M., Kloth, A. C., Wilson, T. B., González-Peña, D., Ireland, F. A., Rodriguez-Zas, S., et al. (2015b). Beef cow prepartum supplement level and age at weaning: II. effects of developmental programming on performance and carcass composition of steer progeny. J. Anim. Sci. 93, 4936–4947. doi: 10.2527/jas.2014-8565
Silva, G. M., Chalk, C. D., Ranches, J., Schulmeister, T. M., Henry, D. D., DiLorenzo, N., et al. (2021). Effect of rumen-protected methionine supplementation to beef cows during the periconception period on performance of cows, calves, and subsequent offspring. Animal 15:100055. doi: 10.1016/j.animal.2020.100055
Sprinkle, J. E., Cuneo, S. P., Frederick, H. M., Enns, R. M., Schafer, D. W., Carstens, G. E., et al. (2006). Effects of a long- acting, trace mineral, reticulorumen bolus on range cow productivity and trace mineral profiles. J. Anim. Sci. 84, 1439–1453. doi: 10.2527/2006.8461439x
Stalker, L. A., Adams, D. C., Klopfenstein, T. J., Fuez, D. M., and Funston, R. N. (2006). Effects of pre- and postpartum nutrition on re- production in spring calving cows and calf feedlot performance. J. Anim. Sci. 84, 2582–2589. doi: 10.2527/jas.2005-640
Stalker, L. A., Ciminski, L. A., Adams, D. C., Klopfenstein, T. J., and Clark, R. T. (2007). Effects of weaning date and prepartum protein supplementation on cow performance and calf growth. Rangeland Ecol. Manage. 60, 578–587. doi: 10.2111/06-082R1.1
Stanton, T. L., Whittier, J. C., Geary, T. W., Kimberling, C. V., and Johnson, A. B. (2000). Effects of Trace mineral supplementation on cow-calf performance, reproduction, and immune function. Prof. Anim. Sci. 16, 121–127. doi: 10.15232/S1080-7446(15)31674-0
Stokes, R. S., Ireland, F. A., and Shike, D. W. (2019a). Influence of repeated trace mineral injections during gestation on beef heifer and subsequent calf performance. Transl. Anim. Sci. 3, 493–503. doi: 10.1093/tas/txy105
Stokes, R. S., Volk, M. J., Ireland, F., and Shike, D. W. (2019b). Effects of maternal supplementation with an injectable trace mineral on subsequent calf performance and inflammatory response. J. Anim. Sci. 97, 4475–4481. doi: 10.1093/jas/skz305
Sullivan, T. M., Micke, G. C., Perkins, N., Martin, G. B., Wallace, C. R., Gatford, K. L., et al. (2009). Dietary protein during gestation affects maternal insulin-like growth factor, insulin-like growth factor binding protein, leptin concentrations, and fetal growth in heifers. J. Anim. Sci. 87, 3304–3316. doi: 10.2527/jas.2008-1753
Summers, A. F., Blair, A. D., and Funston, R. N. (2015b). Impact of supplemental protein source offered to primiparous heifers during gestation on II. progeny performance and carcass characteristics. J. Anim. Sci. 93, 1871–1880. doi: 10.2527/jas.2014-8297
Summers, A. F., Meyer, T. L., and Funston, R. N. (2015a). Impact of supplemental protein source offered to primiparous heifers during gestation on I. average daily gain, feed intake, calf birth body weight, and rebreeding in pregnant beef heifers. J. Anim. Sci. 93, 1865–1870. doi: 10.2527/jas.2014-8296
Tanner, A. R., Bauer, M. L., Kennedy, V. C., Keomanivong, F. E., Kirsch, J. D., Reynolds, L. P., et al. (2020). Influence of corn supplementation to beef cows during mid- to late-gestation: maternal feed intake, body condition, plasma metabolites, and calf growth. Livestock Sci. 240:104142. doi: 10.1016/j.livsci.2020.104142
Tsang, C. K., Lui, T., Thomas, J., Zhang, Y., and Zheng, X. F. S. (2014). Superoxide dismutase 1 acts as a nuclear transcription factor to regulate oxidative stress resistance. Nat. Commun. 5:446. doi: 10.1038/ncomms4446
Underwood, K. R., Tong, J. F., Price, P. L., Roberts, A. J., Grings, E. E., Hess, B. W., et al. (2010). Nutrition during mid to late gestation affects growth, adipose tissue deposition, and tenderness in cross-bred beef steers. Meat Sci. 86, 588–593. doi: 10.1016/j.meatsci.2010.04.008
Varona, L., Misztal, I., and Bertrand, J. K. (1999). Threshold-linear versus linear-linear analysis of birth weight and calving ease using an animal model: I. Variance component estimation. J. Anim. Sci. 77, 1994–2002. doi: 10.2527/1999.7781994x
Waggoner, J. W., Löest, C. A., Mathis, C. P., Hallford, D. M., and Petersen, M. K. (2009). Effects of rumen-protected methionine supplementation and bacterial lipopolysaccharide infusion on nitrogen metabolism and hormonal responses of growing beef steers. J. Anim. Sci. 87, 681–692. doi: 10.2527/jas.2008-1068
Wesley, R. L., Cibils, A. F., Mulliniks, J. T., Pollak, E. R., Petersen, M. K., and Fredrickson, E. L. (2012). An assessment of behavioural syndromes in rangeland-raised beef cattle. Appl. Anim. Behav. Sci. 139, 183–194. doi: 10.1016/j.applanim.2012.04.005
Wilson, T. B., Faulkner, D. B., and Shike, D. W. (2016b). Influence of prepartum dietary energy on beef cow performance and calf growth and carcass characteristics. Livestock Sci. 184, 21–27. doi: 10.1016/j.livsci.2015.12.004
Wilson, T. B., Long, N. M., Faulkner, D. B., and Shike, D. W. (2016a). Influence of excessive dietary protein intake during late gestation on drylot beef cow performance and progeny growth, carcass characteristics, and plasma glucose and insulin concentrations. J. Anim. Sci. 94, 2035–2046. doi: 10.2527/jas.2015-0224
Wilson, T. B., Schroeder, A. R., Ireland, F. A., Faulkner, D. B., and Shike, D. W. (2015). Effects of late gestation distillers grains supplementation on fall-calving beef cow performance and steer calf growth and carcass characteristics. J. Anim. Sci. 93, 4843–4851. doi: 10.2527/jas.2015-9228
Winterholler, S. J., McMurphy, C. P., Mourer, G. L., Krehbiel, C. R., Horn, G. W., and Lalman, D. L. (2012). Supplementation of dried distillers grains with solubles to beef cows consuming low-quality forage during late gestation and early lactation. J. Anim. Sci. 90, 2014–2025. doi: 10.2527/jas.2011-4152
Wu, G., Bazer, F. W., Wallace, J. M., and Spencer, T. E. (2006). Board-invited review: intrauterine growth retardation: implications for the animal sciences. J. Anim. Sci. 84, 2316–2337. doi: 10.2527/jas.2006-156
Wyffels, S. A., McClain, T. P., Dafoe, J. M., Parsons, C. T., Boss, D. L., and DelCurto, T. (2019). An evaluation of the relationship between supplement intake behavior, performance, and grazing behavior by beef cattle grazing northern mixed-grass rangelands. Transl. Anim. Sci. 3, 1802–1806. doi: 10.1093/tas/txz103
Keywords: beef, developmental programing, growth, immune response, reproduction, gestation
Citation: Moriel P, Palmer EA, Harvey KM and Cooke RF (2021) Improving Beef Progeny Performance Through Developmental Programming. Front. Anim. Sci. 2:728635. doi: 10.3389/fanim.2021.728635
Received: 21 June 2021; Accepted: 24 September 2021;
Published: 20 October 2021.
Edited by:
Jimena Laporta, University of Wisconsin-Madison, United StatesReviewed by:
Travis Mulliniks, University of Nebraska-Lincoln, United StatesDaniel Shike, University of Illinois at Urbana-Champaign, United States
Copyright © 2021 Moriel, Palmer, Harvey and Cooke. This is an open-access article distributed under the terms of the Creative Commons Attribution License (CC BY). The use, distribution or reproduction in other forums is permitted, provided the original author(s) and the copyright owner(s) are credited and that the original publication in this journal is cited, in accordance with accepted academic practice. No use, distribution or reproduction is permitted which does not comply with these terms.
*Correspondence: Philipe Moriel, cG1vcmllbCYjeDAwMDQwO3VmbC5lZHU=