- 1Pregnancy and Developmental Programming Area of Excellence, Department of Animal Science, Texas A&M University, College Station, TX, United States
- 2Department of Animal Science, University of Tennessee, Knoxville, Knoxville, TN, United States
- 3College of Veterinary Medicine, University of Tennessee, Knoxville, Knoxville, TN, United States
The activity of the immune system in the reproductive tract has been proven to be crucial in the response to uterine diseases, normal reproductive functions, and tolerance to the allogeneic fetus during pregnancy. The objectives of the current study were to (1) evaluate uterine and vaginal cytokine concentrations in postpartum cows undergoing estrus synchronization followed by timed artificial insemination (TAI) and (2) correlate bacterial communities with cytokine concentrations. Postpartum Angus cows (n = 20) were subjected to a 7-Day Co-Synch protocol with pre-synchronization beginning 21 days prior (d −21) to TAI (d 0). Uterine and vaginal flushes were collected on d −21 and −2. Pregnancy was determined by transrectal ultrasound on d 30. Cytokines include interleukin (IL)-1b, IL-6, IL-10, transforming growth factor beta (TGF-β), and immunoglobin A (IgA) and concentrations were determined by commercial ELISA kits. No differences by day or pregnancy status in cytokine concentrations were detected in vaginal samples. No differences by day or pregnancy status in IgA, IL-10, or IL-1b concentrations were detected in uterine samples. Overall TGF-β concentrations in the uterus were greater in resulting pregnant than non-pregnant cows (44.0 ± 13.4 pg/mL vs. 14.7 ± 4.9 pg/mL; P = 0.047). Uterine TGF-β was correlated with the relative abundance of genera Treponema (r = −0.668; P = 0.049) in resulting non-pregnant cows on d −21 and with the relative abundance of genera Ureaplasma (r = 0.901; P = 0.0004) in resulting pregnant cows on d −2. In resulting pregnant animals, a tendency for a strong correlation was detected between d −2 progesterone concentrations and uterine TGF-β concentrations (r = 0.591, P = 0.07). Overall IL-6 concentrations in the uterus were greater in resulting non-pregnant than pregnant cows (198.7 ± 21.8 pg/mL vs. 144.3 ± 16.1 pg/mL; P = 0.045). A correlation was also detected between uterine IL-6 concentrations and the relative abundance of genera Butyrivibrio (r = 0.742; P = 0.022) in resulting non-pregnant cows on d −21. These results suggest possible relationships between different bacterial communities and cytokine concentrations within the uterus of beef cattle prior to TAI that may ultimately affect fertility outcomes.
Introduction
Reproductive efficiency is vital to cow-calf producers to reduce losses and maintain profit. Infertility, defined as the inability to develop and maintain a pregnancy in a defined breeding season, is estimated to cost the beef and dairy industries over $1 billion annually in the United States (Bellows et al., 2002; Pohler et al., 2020). Infertility can be attributed to numerous factors such as genetics, nutrition, body condition, environmental stress, or disease. These factors are particularly challenging for the postpartum cow because of the rapid changes occurring during the uterine involution period, when the uterus returns to a normal, non-pregnant size.
Normally, the placenta will be expelled within 12 h following parturition (Beagley et al., 2010). By 28 days postpartum (DPP; week 4), necrosis of the maternal caruncles within the uterus will occur, and new caruncles will develop (Wagner and William, 1969; Archbald et al., 1972; Sheldon et al., 2008). In addition, cows generally resume ovarian cyclicity and fertility between 28 and 42 DPP (week 4–6; Short et al., 1990). However, if the placenta or necrotic tissues are not properly expelled, inflammation and/or bacterial infections may occur leading to uterine diseases such as metritis or clinical and subclinical endometritis (Azawi, 2008; Sheldon et al., 2008). Most endometritis research has focused on dairy cattle, however the prevalence of subclinical endometritis in postpartum beef cattle can range from 34 to 88% within a herd (Santos et al., 2009; Ricci et al., 2017; Machado Pfeifer et al., 2018). Before 50 DPP (week 7), pregnancy rates are reduced to <40% and after 50 DPP, pregnancy rates greatly improve, even with ~15% of cows still affected with subclinical endometritis (Santos et al., 2009; Ricci et al., 2017; Machado Pfeifer et al., 2018). The presence of pathogenic bacteria from any infection in the uterus is detected by the innate immune system. The production of small proteins called cytokines, which communicate between immune cells, initiate an inflammatory environment and influx of immune cells into the uterus to clear the infection (Wira et al., 2005; Azawi, 2008).
Although the immunological environment of the reproductive tract is most often associated with the response to postpartum uterine diseases, the immune system plays a role in healthy cows for normal reproductive functions and the development and maintenance of pregnancy (Ott, 2019). One common example is the semi-allogeneic fetus, expressing both maternal antigens and foreign paternal antigens, the maternal immune system must tolerate the presence of the fetus to maintain pregnancy (Guleria and Sayegh, 2007). Cytokines serve as the communicators between immune cells present in the reproductive tract to then regulate the local immune environment and stimulate the proper response to the semi-allogeneic fetus before and throughout pregnancy (Ott, 2019). In cattle, the reproductive tract experiences a decrease in mRNA abundance of pro-inflammatory cytokines, such as interleukin (IL)-1b and IL-6, and an increase in anti-inflammatory cytokines, such as IL-10, during the postpartum period and leading up to breeding (Oliveira et al., 2013; Heppelmann et al., 2015). Moreover, anti-inflammatory cytokines, including IL-10 and transforming growth factor beta (TGF-β), steadily increase during early pregnancy and placentation in cattle, respectively (Oliveira and Hansen, 2008, 2009; Vasudevan et al., 2017).
It has been indicated that the immune system has a vital role in the clearance of uterine diseases, normal reproductive functions, and establishment and maintenance of pregnancy; however, few studies have investigated the reproductive tract immunological environment prior to breeding and the subsequent effect on fertility in beef cattle. Based on published data from the current study, the diversity of bacterial communities of the reproductive tract shifts throughout an estrus synchronization protocol (Ault et al., 2019a) and this is likely due to the taxonomic composition of the bacteria in the uterus and vagina (Ault et al., 2019b). Notably, the relative abundance of multiple genera of bacteria differed between resulting pregnant and non-pregnant cows (Ault et al., 2019b). In the current report, the first objective was to evaluate the concentration of both pro- and anti-inflammatory cytokines from uterine and vaginal flush samples throughout an estrus synchronization protocol to determine the potential effects of the immune environment on fertility. An additional objective was then to correlate the relative abundance of bacterial communities with both pro- and anti-inflammatory cytokine concentrations. It was hypothesized that pro- and anti-inflammatory cytokine concentrations will differ between cows that establish a pregnancy vs. cows that are not pregnant 30 days after timed artificial insemination (TAI). Additionally, it was hypothesized that the difference in cytokines is most likely due to relationships with either commensal or pathogenic bacteria.
Materials and Methods
This study was performed under an approved protocol by the Institutional Animal Care and Use Committee of the University of Tennessee, Knoxville.
Experimental Design
Sixty-eight Angus cows, with no clinical signs of disease or reproductive tract abnormalities were an average of 80 ± 2.6 DPP and 4.6 ± 0.57 years old at breeding and subjected to an industry standard estrus synchronization protocol. An intramuscular injection of prostaglandin F2α (PGF2α; Lutalyse, 5 mL; 5 mg/mL) was given 21 days prior (d −21) to TAI. A 7 Day Co-Synch Protocol was implemented beginning 9 days prior to TAI (d −9) with an intramuscular injection of gonadotropin releasing hormone (GnRH; Factrel, 100 mcg). Two days prior to TAI (d −2), an intramuscular injection of PGF2α (Lutalyse, 5 mL; 25 mg/mL) was administered. Controlled internal release devices (CIDR) were not used in the study due to uterine and vaginal flush collection methods. On the day of TAI (d 0), an intramuscular injection of GnRH (Factrel, 100 mcg) was administered followed by artificial insemination by a single technician using a single sire. Ultrasonography was used to determine presence of ovarian structures at each day of the protocol and pregnancy diagnosis 30 days following TAI. Twenty cows (10 pregnant and 10 non-pregnant) were selected for analysis based on the following criteria outlined by Ault et al. (2019a,b): (1) corpus luteum (CL) present on d −21 and −9 with progesterone (P4) >1 ng/mL, (2) response to GnRH on day −9 as assumed by the presence of a CL on day −2, and (3) ovulatory follicle present on day 0 (TAI).
Sample Collection, DNA Extraction, and Sequencing
Uterine and vaginal flushes were collected on d −21 and −2 and used for bacterial DNA extraction and sequencing and to determine cytokine concentrations and pH as previously described by Ault et al. (2019a,b). Briefly, the perineal area was cleaned and disinfected prior to flushing. To obtain vaginal flush samples, 0.9% sterile saline (Vetivex; pH = 5.6) was drawn up into a 60 mL sterile syringe and expelled into the vagina and recovered via vaginal lavage. To obtain uterine flush samples, sterile saline (180 mL) was flushed through a Foley catheter into the uterus. Resulting uterine flush fluid was collected by rectal massage. The pH of the flush samples was measured by UltraBasic pH meter (Denver Instruments, Arvada, CO) and recorded immediately following collection. Samples were snap-frozen in liquid nitrogen and stored at −80°C until analysis. Extensive detail for methods describing bacterial DNA extraction and sequencing has been previously outlined by Ault et al. (2019a,b). Briefly, samples were thawed and vortexed, and 5 mL aliquots were placed in sterile 15 mL tubes and centrifuged (4,696 x g) at 4 °C for 10 min. Supernatant were removed and the resulting pellet was resuspended in 180 μL of enzymatic lysis buffer, vortexed, and incubated for 30 min at 37°C. Following incubation, 25 μL of proteinase K and 200 μL of AL buffer were added, then the sample was vortexed and incubated for 30 min at 56°C, then 200 μL of 100% ethanol was added and the sample was vortexed. Purification of DNA was completed using the QIAGEN DNeasy Blood and Tissue kit (Qiagen, Hilden, Germany) according to manufactured protocol. Extracted DNA quantity and quality, with 260/280 ranging from 1.8 to 2.0, were estimated using AmPure beads and Nanodrop 1000 spectrophotometer (Agencourt, Beverly, MA and ThermoScientific, Wilmington, DE). Library preparation was conducted using PCR targeting the V1 to V3 hypervariable regions of the 16S rRNA bacterial gene and libraries were submitted for sequencing at the United States Meat Animal Research Center (USDA-ARS-USMARC, Clay Center, NE). Vaginal and uterine bacterial community alpha- and beta-diversity were reported by Ault et al. (2019a) and vaginal and uterine bacterial taxonomic composition were reported by Ault et al. (2019b).
Cytokine, Antibody, and Progesterone Assays
Concentrations of interleukin (IL)-1b, IL-6, IL-10, transforming growth factor beta (TGF-β), and immunoglobin A (IgA) in uterine and vaginal flushes were determined by commercially available bovine-specific ELISA kits (MyBioSource, San Diego, CA) and performed according to manufacturer instructions. Standards and samples were run in duplicate and inter- and intra-assay CV were <10%.
Given the lack of differences in bacterial community abundance and diversity in the vaginal samples (Ault et al., 2019a,b), a subset of ten cows (5 pregnant and 5 non-pregnant) were selected for analysis in vaginal samples. Selection criteria included response to the estrus synchronization protocol as outlined previously. Concentrations for IL-1b in vaginal samples were unable to be quantified due to concentrations running below the standard curve. For the uterine samples, the subsets were initially analyzed, and further analysis was completed for TGF-β, IL-6, and IL-1b in all twenty cows (10 pregnant and 10 non-pregnant).
Progesterone RIA was performed according to the previously described protocol (Pohler et al., 2016) using a double-antibody RIA kit (MP Biomedicals, Santa Ana, CA). A standard curve was used to calculate sample concentrations and in-house controls for quality control. Inter- and intra-assay CV were <10%. There were no differences in circulating P4 concentrations between pregnant and non-pregnant cows at d −21 (2.34 ± 0.62 ng/mL vs. 2.20 ± 0.74 ng/mL) or d −2 (6.11 ± 1.81 ng/mL vs. 4.76 ± 1.77 ng/mL) as reported by Ault et al. (2019a).
Statistical Analysis
Resulting IL-6, IL-10, IL-1b, TGF-β, and IgA concentrations were analyzed for normality by SAS Enterprise Guide distribution analysis using a Kolmogorov-Smirnov statistic with P < 0.05 indicating non-normal distribution and P > 0.05 indicating normal distribution of data. IL-6, IL-10, IL-1b, and IgA data had a normal distribution and were analyzed by SAS Enterprise Guide ANOVA with dependent variables of cytokine concentration and independent variables of day or pregnancy status. TGF-β concentrations were non-normally distributed and analyzed by SAS Enterprise Guide Non-parametric ANOVA with dependent variable of TGF-β concentration and independent variables of day or pregnancy status. Correlations were performed in SAS 9.4 using Pearson correlation. A statistical significance was reported at P ≤ 0.05. A tendency was reported at P > 0.05 and ≤ 0.10.
Results
Cytokine and Antibody Concentrations in Vaginal Samples
Concentrations of IgA had no change over time in the vagina of both resulting pregnant and non-pregnant cows (P > 0.05; Figure 1A). Additionally, no differences in IgA concentrations were observed in the vagina between resulting pregnant and non-pregnant cows at d −21 and −2. No differences were detected in IL-10 concentrations in the vagina between resulting pregnant and non-pregnant cows at d −21 and d −2 (data not shown). For TGF-β, no difference between resulting pregnant and non-pregnant cows or between protocol days were detected (P > 0.05; Figure 1B). High variation was detected for IL-6 among cows and no differences were determined significant (P > 0.05) although trends were observed (Figure 1C). IL-6 concentrations tended to be greater in resulting non-pregnant cows compared to pregnant cows at d −21 (515.5 ± 75.2 pg/mL vs. 375.3 ± 262.9 pg/mL) and d −2 (403.1 ± 180.4 pg/mL vs. 289.6 ± 109.7 pg/mL) in the vagina.
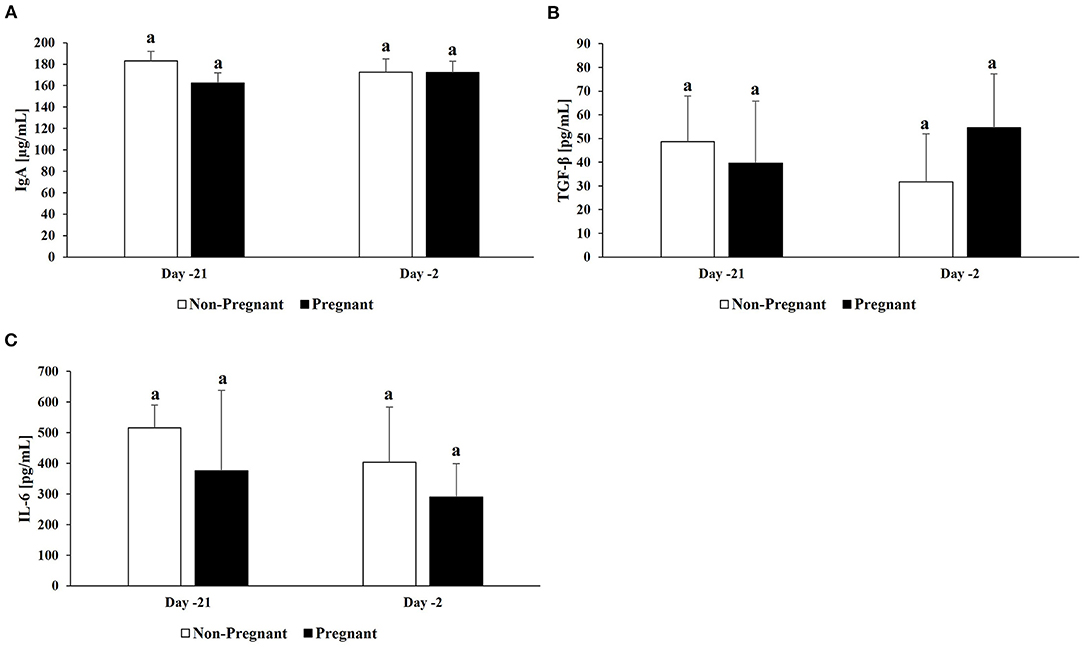
Figure 1. Cytokine concentrations in vaginal samples. For a subset of cows (n =10; n = 5/group), cytokine concentrations were determined in vaginal samples: (A) IgA, (B) TGF-β, and (C) IL-6. Non-pregnant cows are indicated by white bars and pregnant cows by black bars for both d −21 and −2. Different superscripts denotes significant differences (P ≤ 0.05).
Cytokine and Antibody Concentrations in Uterine Samples
No differences were detected in IgA concentrations in the uterus between resulting pregnant and non-pregnant cows at d −21 and −2 (P > 0.05; Figure 2A). No differences were detected in IL-10 concentrations in the uterus between resulting pregnant and non-pregnant cows at d −21 and −2 (P > 0.05; data not shown). Overall TGF-β concentrations in the uterus were greater in resulting pregnant than non-pregnant cows (44.0 ± 13.4 pg/mL vs. 14.7 ± 4.9 pg/mL; P = 0.047). Additionally, uterine TGF-β on both d −21 (46.7 ± 22.0 pg/mL vs. 12.3 ± 7.1 pg/mL) and −2 (41.4 ± 16.7 pg/mL vs. 17.1 ± 7.0 pg/mL) were greater in resulting pregnant than non-pregnant cows (P ≤ 0.05; Figure 2B); however, there was no significant change over time (P > 0.05). Overall IL-6 concentrations in the uterus were greater in resulting non-pregnant than pregnant cows (198.7 ± 21.8 pg/mL vs 144.3 ± 16.1 pg/mL; P = 0.045). Additionally, uterine IL-6 concentrations on d −21 were greater in resulting non-pregnant than pregnant cows (238.1 ± 31.2 pg/mL vs. 133.6 ± 21.8 pg/mL; P = 0.008; Figure 2C), and concentrations decreased in resulting non-pregnant cows from d −21 to −2 (238.1 ± 31.2 pg/mL vs. 159.3 ± 26.2 pg/mL; P = 0.031; Figure 2C). No differences were detected in IL-1b concentrations in the uterus between resulting pregnant and non-pregnant cows at d −21 and −2 (P > 0.05; Figure 2D).
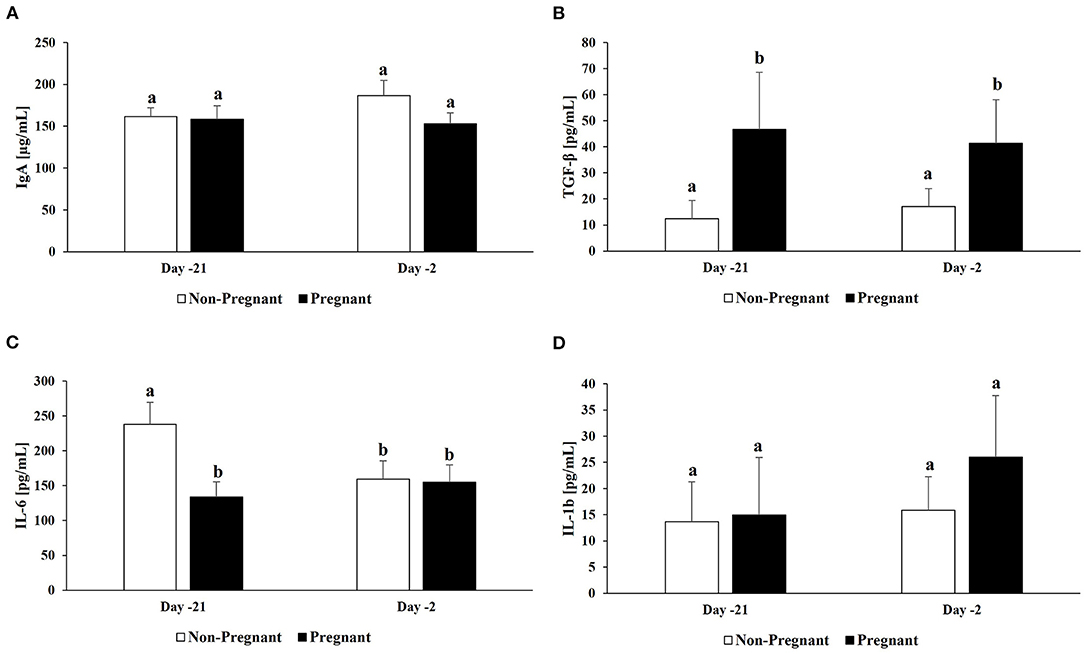
Figure 2. Cytokine concentrations in uterine samples. For a subset of cows (n =10; n = 5/group), cytokine concentrations were determined in uterine samples: (A) IgA. For all cows (n = 20; n = 10/group), cytokines concentrations were determined in uterine samples: (B) TGF-β, (C) IL-6, and (D) IL-1b. Non-pregnant cows are indicated by white bars and pregnant cows by black bars for both d −21 and −2. Different superscripts denotes significant differences (P ≤ 0.05).
Pearson Correlation Coefficients—Phyla
There were no significant correlations between cytokine concentrations (TGF-β, IL-6, IL-1b) and relative abundances of phyla in the uterus for resulting non-pregnant animals on d −21 or −2 (P > 0.05; Supplementary Tables 1, 2). Additionally, there were no significant correlations between cytokine concentrations (TGF-β and IL-1b) and relative abundances of phyla in the uterus for resulting pregnant animals on d −21 (P > 0.05; Supplementary Table 3). However, there was a strong correlation between uterine IL-6 concentrations and the relative abundance of Acidobacteria (r = −0.701, P = 0.024) in resulting pregnant animals on d −21 (Supplementary Table 3). There were no significant correlations between cytokine concentrations (IL-1b) and relative abundances of phyla in the uterus for resulting pregnant animals on d −2 (P > 0.05). There was a strong correlation between uterine TGF-β concentrations and the relative abundance of Tenericutes in resulting pregnant animals on d −2 (r = 0.897, P = 0.0004; Supplementary Table 4). Additionally, there was a correlation between uterine IL-6 concentrations and the relative abundance of Tenericutes in resulting pregnant animals on d −2 (r = 0.678, P = 0.031; Supplementary Table 4).
Pearson Correlation Coefficients—Genera
Correlations between cytokines and the relative abundance of genera >0.1% abundance in the uterus of resulting non-pregnant cows for d −21 are reported in Table 1. There were a few significant correlations between uterine TGF-β concentrations and the relative abundance of Phascolarctobacterium, unclassified genera from the family Clostridiaceae, and Treponema (P ≤ 0.05). Significant correlations were detected between uterine IL-1b concentrations and the relative abundance of Streptococcus and Campylobacter (P ≤ 0.05). Significant correlations were also detected between uterine IL-6 concentrations and the relative abundance of unclassified genera from the order Clostridiales, Butyrivibrio, Mogibacterium, and unclassified genera from the family Coriobacteriaceae (P ≤ 0.05). There was only one significant correlation observed in the uterus of resulting non-pregnant cows for d −2 was between uterine IL-6 concentrations and the relative abundance of unclassified genera from the order Burkholderiales (P ≤ 0.05; Table 2).
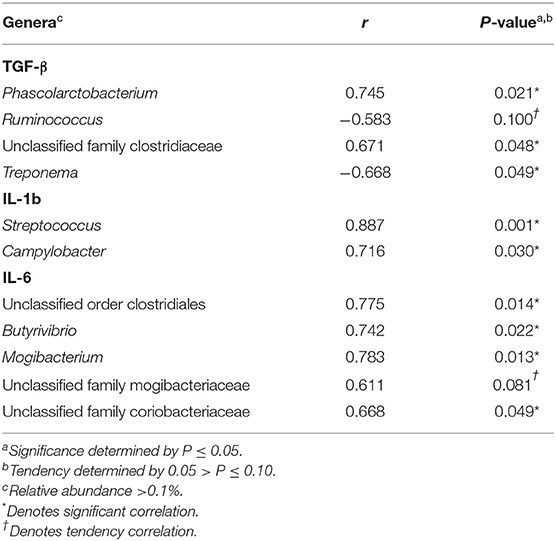
Table 1. Pearson correlation coefficients (r) for TGF-β, IL-1b, and IL-6 and the relative abundance of genera within the uterus of resulting non-pregnant cows for d −21.
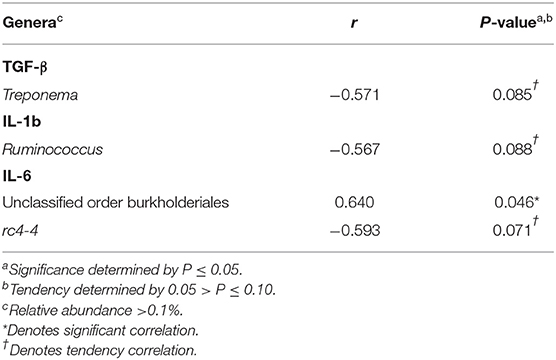
Table 2. Pearson correlation coefficients (r) for TGF-β, IL-1b, and IL-6 and the relative abundance of genera within the uterus of resulting non-pregnant cows for d −2.
There were no significant correlations between cytokines and the relative abundance of genera >0.1% abundance in the uterus of resulting pregnant cows for d −21 (P > 0.05). Correlations between cytokines and the relative abundance of genera >0.1% abundance in the uterus of resulting pregnant cows for d −2 are reported in Table 3. There was a significant correlation between uterine TGF-β concentrations and the relative abundance of Ureaplasma (P ≤ 0.05). Significant correlations were detected between uterine IL-1b concentrations and the relative abundance of unclassified genera from the family Ruminococcaceae, unclassified genera from the order Clostridiales, Helcococcus, and Bacteroides (P ≤ 0.05). A significant correlation was also detected between uterine IL-6 concentrations and the relative abundance Ureaplasma (P ≤ 0.05).
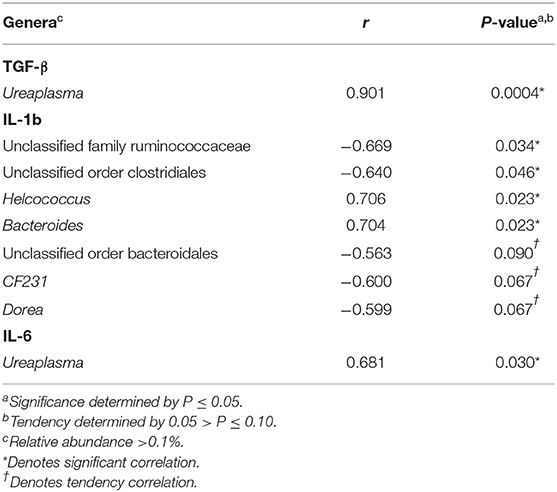
Table 3. Pearson correlation coefficients (r) for TGF-β, IL-1b, and IL-6 and the relative abundance of genera within the uterus of resulting pregnant cows for d −2.
Pearson Correlation Coefficients—Progesterone
Progesterone concentrations were not significantly correlated with uterine TGF-β concentrations in both resulting pregnant and non-pregnant animals on d −21 and d −2 (r = 0.173, P > 0.05; Figure 3A). In resulting pregnant animals, P4 concentrations were moderately correlated with uterine TGF-β concentrations for both days (r = 0.441, P = 0.05; Figure 3B) whereas no significant correlation was detected in resulting non-pregnant animals (P > 0.05). Progesterone concentrations were not significantly correlated with uterine TGF-β concentrations in resulting pregnant cows on d −21 (r = 0.371, P > 0.05; Figure 3C). Finally, a tendency for a strong correlation was detected in resulting pregnant cows between d −2 P4 concentrations and uterine TGF-β concentrations (r = 0.591, P = 0.07; Figure 3D).
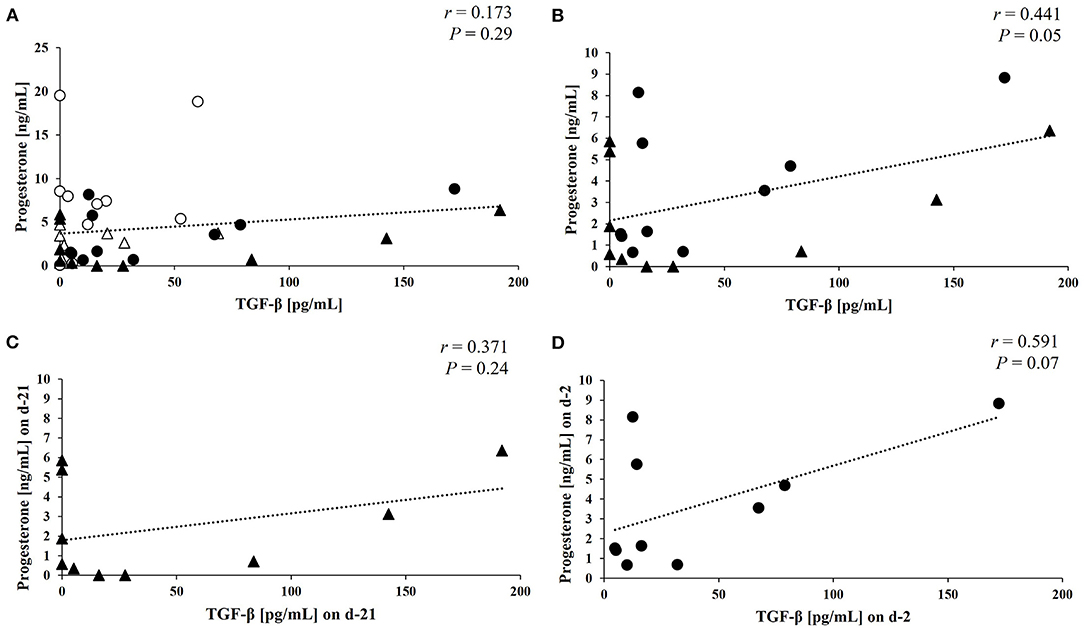
Figure 3. Pearson correlation coefficients between progesterone and TGF-β concentrations. Pearson correlation coefficients between progesterone concentrations (ng/mL) and TGF-β concentrations (pg/mL) in uterine samples: (A) Overall relationship between concentrations for both resulting non-pregnant and pregnant cows. Non-pregnant cows on d −21 are indicated by the open triangles, non-pregnant cows on d −2 are indicated by the open circles, pregnant cows on d −21 are indicated by the black triangles, and pregnant cows on d −2 by the black circles, (B) Relationship between concentrations for pregnant cows, (C) Relationship between concentrations for pregnant cows on d −21, and (D) Relationship between concentrations for pregnant cows on d −2.
Discussion
Regulation of immune system responses and the shift between an overall state of anti-inflammatory and pro-inflammatory environments has been previously determined to influence the development and maintenance of pregnancy following fertilization (van Mourik et al., 2009; Fair, 2015; Ott, 2019). One objective of the current study was to evaluate the immunological environment by the presence of vaginal and uterine cytokines prior to breeding and their potential effect on fertility in beef cattle. Results from the current study indicate that cows diagnosed as not pregnant 30 days following TAI had an increased level of IL-6 and decreased TGF-β in the uterus leading up to breeding, directly contrasting cows identified as pregnant. Because IL-6 is classified as a pro-inflammatory cytokine and TGF-β as an anti-inflammatory cytokine, the observed differences between resulting pregnant and non-pregnant cows suggest the uterine immunological environment may affect fertility.
Interleukin-6 is a well-known pro-inflammatory cytokine and that regulates acute phase response and other inflammatory responses (Scheller et al., 2011; Ho et al., 2015). Numerous cytokines, such as IL-6, have been identified and subsequently classified as embryokines which are used by the oviduct and endometrium to regulate development of bovine preimplantation embryos (Tríbulo et al., 2018; Wooldridge and Ealy, 2019; Wooldridge et al., 2019). Toward the end of gestation (final 60 days), IL-6 concentrations are elevated and steadily decrease during the postpartum period (Ishikawa et al., 2004; Herath et al., 2009; Gabler et al., 2010; Heppelmann et al., 2015), and this aligns with normal resolution of endometrial inflammation. In the current study, greater levels of IL-6 prior to TAI were associated with the failure to develop and/or maintain a pregnancy to day 30 in postpartum beef cows. These results are similar to those observed by Herath et al. (2009), in which resulting infertile cows had increased mRNA abundance of IL-6 in the endometrium during the first week postpartum when compared to resulting fertile cows. While IL-6 concentrations were elevated in resulting non-pregnant cows in the current study, the concentrations were similar to those observed in uterine flushes of presumed healthy cows (approximately 100-200 pg/mL) rather than cows diagnosed with subclinical endometritis (~500–600 pg/mL; Brodzki et al., 2015). Therefore, the acute pro-inflammatory state in the uterus prior to breeding could be associated with a uterine environment that is not conducive to pregnancy establishment.
Similar to IL-6, TGF-β is an embryokine and is generally considered an anti-inflammatory cytokine with multifaceted actions including a potent modulator of oviduct and endometrial functions (Godkin and Doré, 1998; Ingman and Robertson, 2002; Neira et al., 2010; Tríbulo et al., 2018). Transforming growth factor β is abundantly found in seminal plasma and is thought to help facilitate induction of maternal immune tolerance to paternal and conceptus antigens and thereby promote implantation success (Robertson et al., 2002). The addition of seminal plasma to bovine endometrial cells and sperm to oviduct epithelial cells in vitro increases mRNA expression of TGF-β (Yousef et al., 2016; Ibrahim et al., 2019), and treatment with TGF-β in the uterus prior to insemination marginally improves conception rates in beef cows with compromised fertility (Odhiambo et al., 2009). T-regulatory (Treg) cells are major contributors to maintaining an anti-inflammatory environment by producing TGF-β which continues to recruit additional T cells to the Treg designation and inhibiting production of pro-inflammatory cytokines (Robertson et al., 2018). Interestingly, Treg cells are not temporally regulated in bovine endometrial cells during the estrous cycle or early pregnancy; however, appear to be upregulated during active placentation in cattle (Oliveira and Hansen, 2008; Oliveira et al., 2013; d 33–34 of gestation). In the current study, greater levels of TGF-β prior to insemination were associated with the successful development and maintenance of pregnancy to day 30 following TAI in postpartum beef cows. The establishment of an anti-inflammatory uterine environment begins during the estrous and menstrual cycle, where the steroid hormones such as progesterone (P4) optimize the reproductive tract environment in preparation for pregnancy establishment (Bauersachs et al., 2008; Schumacher et al., 2014). Specifically, P4 is an important immunomodulator of Treg cell release and TGF-β secretion from mice and human endometrium, respectively (Polli et al., 1996; Kim et al., 2005; Mao et al., 2010). In the current study, there was a strong relationship between TGF-β and P4 concentrations during the estrus synchronization protocol in resulting pregnant cows; however, this relationship was not observed in resulting non-pregnant cows. Therefore, results presented herein suggests an immunomodulatory effect of P4 that potentially increases uterine TGF-β prior to TAI, subsequently resulting in an anti-inflammatory environment that is conducive for successful establishment and maintenance of pregnancy in beef cattle. Future studies may evaluate methods to alter and maintain an anti-inflammatory uterine environment through estrus synchronization to obtain optimal breeding outcomes.
After parturition in cattle, there typically is an upsurge in bacterial inhabitance within the uterus, and the presence of pathogenic bacteria often leads to uterine infection and infertility (Sheldon et al., 2008). Therefore, an additional objective of this study was to correlate the relative abundance of bacterial communities with both pro- and anti-inflammatory cytokine concentrations within the uterus. In resulting non-pregnant cows, there were quite a few relationships between cytokine concentrations and bacteria, most notably though was IL-6 with both Butyrivibrio and unclassified genera from the order Burkbolderiales and TGF-β with Treponema. On d −21 in resulting non-pregnant cows, there was a strong, positive correlation between IL-6 concentrations and Butyrivibrio. The genera Butyrivibrio, in the class Clostridia, is most often associated with ruminal functions such as plant fiber digestion (Palevich et al., 2020). While not generally considered pathogenic, the genera Butyrivibrio has been identified within the uterus of resulting non-pregnant cows in previous studies (Clemmons et al., 2017; Ault et al., 2019b). Additionally, there was a strong, positive correlation between IL-6 concentrations and unclassified genera from the order Burkholderiales on d −2 in resulting non-pregnant cows. Bacterial species within this order have been characterized as pathogenic in humans, causing devastating symptoms in those with immunodeficiencies and cystic fibrosis (Nunvar et al., 2017; Wallner et al., 2019). Clemmons et al. (2017) found that uterine flush samples collected from resulting non-pregnant cows on d −2 also contained unclassified genera from the order Burkholderiales. Similarly, Ault et al. (2019b) found a greater relative abundance of unclassified genera from the order Burkholderiales in uterine samples from resulting non-pregnant compared to pregnant cows on d −2. Unlike the positive correlations between IL-6 and both Butyrivibrio and unclassified genera from the order Burkbolderiales, TGF-β was negatively correlated with Treponema meaning that as Treponema increased then concentrations of TGF-β decreased in the uterus of non-pregnant cows on d −21. The genera Treponema, in the phylum Spirochaetes, has been characterized as pathogenic with Treponema spp. causing syphilitic lesions in the cervix uteri (i.e., lowest region of the uterus) of women (Sykes and Kalan, 1975). Additionally, Treponema spp. is prevalent in infectious bovine foot disease resulting in necrotic lesions and lameness (Bay et al., 2018; Watts et al., 2018; Kontturi et al., 2019). These results demonstrate some pathogenic bacteria have positive relationships with pro-inflammatory cytokines and negative relationships with anti-inflammatory cytokines, which potentially could be affecting fertility.
While the presence of pathogenic bacteria in the uterus generally has a negative effect on fertility, commensal bacteria may contribute to an optimal uterine environment for the establishment of pregnancy. The relative abundance of the phyla Acidobacteria was negatively correlated with IL-6 concentrations in the uterus of resulting pregnant cows on d −21, meaning that as the concentration of IL-6 decreases then the relative abundance of Acidobacteria increases. Ault et al. (2019b) reported that resulting pregnant cows had greater relative abundance Acidobacteria in the uterus on d −21. In addition, Acidobacteria has been identified to be greater in the uterus of healthy vs. metritis diagnosed cows during the first 35 DPP (Chen et al., 2020). Interestingly, on d −2 in resulting pregnant cows, there was a strong, positive correlation between TGF-β and IL-6 concentrations and Ureaplasma. The genera Ureaplasma, in the phylum Tenericutes, has bacterial species that have been previously associated with reproductive disorders (Crane and Hughes, 2018). However, Ault et al. (2019b) observed Ureaplasma genera had the greatest abundance detected in the uterus of pregnant cows at d −2. Similar results also found that Ureaplasma spp. were abundantly found in the postpartum uterus of healthy cows vs. metritis-diagnosed cows (Santos et al., 2011; Jeon et al., 2015). An additional study observed that Ureaplasma and Ruminococcus genera had the greatest abundance detected in the uterus of resulting pregnant heifers at d −3 when compared to resulting non-pregnant heifers (Owens, 2020). Future research is necessary to elucidate the presence of specific bacterial species within a healthy uterine environment.
No differences were detected in uterine IL-1b, IL-10, or IgA concentrations in the current study. Similar to IL-6, IL-1b is a prominent pro-inflammatory cytokine that is greatly associated with endometrial inflammation and uterine disease in cattle (Herath et al., 2009; Gabler et al., 2010; Brodzki et al., 2015; Heppelmann et al., 2015). One mechanism to reduce inflammation is through the production of immunoglobulins, specifically IgA which is predominant in mucosal membranes and prevents pathogenic bacterial infections (Deng et al., 2015; Elluru et al., 2015; Russell et al., 2015). However, given that all cows in the current study did not display any clinical signs of disease or reproductive tract abnormalities, this could explain the lack of differences in IL-1b and IgA concentrations. Interlukein-10 is an anti-inflammatory cytokine and has similar secretion patterns and functions to TGF-β (Ott, 2019); however, it does not appear to be as greatly expressed in the endometrium and oviduct during the estrous cycle when compared to other embryokines such as IL-6 and TGF-β (Tríbulo et al., 2018). This could be one explanation for the lack of observed difference in IL-10 concentrations in the current study. In all vaginal samples, there were no differences detected. This observation could be associated with the absence of bacterial community diversity within the vagina between non-pregnant and pregnant cows (Ault et al., 2019a). Additionally, while there were shifts in bacterial community abundances, they were more pronounced within the uterus rather than the vagina (Ault et al., 2019b).
In conclusion, the current study suggests that immunological factors present in the uterus throughout estrus synchronization leading up to TAI are associated with different uterine bacterial communities and can influence fertility in beef cattle. An anti-inflammatory uterine environment prior to TAI appears to be associated with the successful establishment and maintenance of pregnancy. Future research is necessary to determine factors that can positively affect the uterine immune and microbiota environment and ultimately improve reproductive efficiency.
Data Availability Statement
The datasets presented in this study can be found in online repositories. This data can be found here: https://www.ncbi.nlm.nih.gov/sra/SRP103314.
Ethics Statement
The animal study was reviewed and approved by Institutional Animal Care and Use Committee of the University of Tennessee, Knoxville.
Author Contributions
KP and PM conceived and designed the experiment. RKP, TA-S, and AL wrote, reviewed, and edited the manuscript. RKP, KP, PM, and AL provided experimental and laboratorial resources. TA-S, KP, and PM conducted sample collection. RKP, TA-S, and RRP performed the lab analysis. RKP conducted data analysis and interpretation of results. All authors have read and approved the final manuscript.
Funding
The authors would like to acknowledge support from the Agriculture and Food Research Initiative Competitive Grant No. 2020-67034-31776 from the USDA National Institute of Food and Agriculture, as well as USDA-NIFA Hatch/Multistate Project W3112-TEX07702 Reproductive performance in domestic animals, and a Center of Excellence grant provide by a joint contribution from the state of Tennessee and University of Tennessee.
Conflict of Interest
The authors declare that the research was conducted in the absence of any commercial or financial relationships that could be construed as a potential conflict of interest.
Supplementary Material
The Supplementary Material for this article can be found online at: https://www.frontiersin.org/articles/10.3389/fanim.2021.704714/full#supplementary-material
References
Archbald, L. F., Schultz, R. H., Fahning, M. L., Kurtz, H. J., and Zemjanis, R. (1972). A sequential histological study of the post-partum bovine uterus. Reproduction 29, 133–136. doi: 10.1530/jrf.0.0290133
Ault, T. B., Clemmons, B. A., Reese, S. T., Dantas, F. G., Franco, G. A., Smith, T. P. L., et al. (2019a). Uterine and vaginal bacterial community diversity prior to artificial insemination between pregnant and nonpregnant postpartum cows. J. Anim. Sci. 97, 4298–4304. doi: 10.1093/jas/skz210
Ault, T. B., Clemmons, B. A., Reese, S. T., Dantas, F. G., Franco, G. A., Smith, T. P. L., et al. (2019b). Bacterial taxonomic composition of the postpartum cow uterus and vagina prior to artificial insemination. J. Anim. Sci. 97, 4305–4313. doi: 10.1093/jas/skz212
Azawi, O. I. (2008). Postpartum uterine infection in cattle. Anim. Reprod. Sci. 105, 187–208. doi: 10.1016/j.anireprosci.2008.01.010
Bauersachs, S., Mitko, K., Ulbrich, S. E., Blum, H., and Wolf, E. (2008). Transcriptome studies of bovine endometrium reveal molecular profiles characteristic for specific stages of estrous cycle and early pregnancy. Exp. Clin. Endocrinol. Diabetes 116, 1–14. doi: 10.1055/s-2008-1076714
Bay, V., Griffiths, B., Carter, S., Evans, N. J., Lenzi, L., Bicalho, R. C., et al. (2018). 16S rRNA amplicon sequencing reveals a polymicrobial nature of complicated claw horn disruption lesions and interdigital phlegmon in dairy cattle. Sci. Rep. 8:15529. doi: 10.1038/s41598-018-33993-9
Beagley, J. C., Whitman, K. J., Baptiste, K. E., and Scherzer, J. (2010). Physiology and treatment of retained fetal membranes in cattle. J. Vet. Intern. Med. 24, 261–268. doi: 10.1111/j.1939-1676.2010.0473.x
Bellows, D. S., Ott, S. L., and Bellows, R. A. (2002). Review: cost of reproductive diseases and conditions in cattle. Prof. Anim. Sci. 18, 26–32. doi: 10.15232/S1080-7446(15)31480-7
Brodzki, P., Kostro, K., Krakowski, L., and Marczuk, J. (2015). Inflammatory cytokine and acute phase protein concentrations in the peripheral blood and uterine washings of cows with subclinical endometritis in the late postpartum period. Vet. Res. Commun. 39, 143–149. doi: 10.1007/s11259-015-9635-4
Chen, H., Fu, K., Pang, B., Wang, J., Li, H., Jiang, Z., et al. (2020). Determination of uterine bacterial community in postpartum dairy cows with metritis based on 16S rDNA sequencing. Vet. Anim. Sci. 10:100102. doi: 10.1016/j.vas.2020.100102
Clemmons, B. A., Reese, S. T., Dantas, F. G., Franco, G. S., Smith, T. P. L., Adeyosoye, O. I., et al. (2017). Vaginal and uterine bacterial communities in postpartum lactating cows. Front. Microbiol. 8:1047. doi: 10.3389/fmicb.2017.01047
Crane, M. B., and Hughes, C. A. (2018). Can Ureaplasma diversum be transmitted from donor to recipient through the embryo? Two case reports outlining U. diversum losses in bovine embryo pregnancies. Can. Vet. J. 59, 43–46.
Deng, Q., Odhiambo, J. F., Farooq, U., Lam, T., Dunn, S. M., and Ametaj, B. N. (2015). Intravaginal lactic acid bacteria modulated local and systemic immune responses and lowered the incidence of uterine infections in periparturient dairy cows. PLoS ONE 10:e0124167. doi: 10.1371/journal.pone.0124167
Elluru, S. R., Kaveri, S. V., and Bayry, J. (2015). The protective role of immunoglobulins in fungal infections and inflammation. Semin. Immunopathol. 37:187–197. doi: 10.1007/s00281-014-0466-0
Fair, T. (2015). The contribution of the maternal immune system to the establishment of pregnancy in cattle. Front. Immunol. 6:7. doi: 10.3389/fimmu.2015.00007
Gabler, C., Fischer, C., Drillich, M., Einspanier, R., and Heuwieser, W. (2010). Time-dependent mRNA expression of selected pro-inflammatory factors in the endometrium of primiparous cows postpartum. Reprod. Biol. Endocrinol. 8:152. doi: 10.1186/1477-7827-8-152
Godkin, J. D., and Doré, J. J. (1998). Transforming growth factor beta and the endometrium. Rev. Reprod. 3, 1–6. doi: 10.1530/ror.0.0030001
Guleria, I., and Sayegh, M. H. (2007). Maternal acceptance of the fetus: true human tolerance. J. Immunol. 178, 3345–3351. doi: 10.4049/jimmunol.178.6.3345
Heppelmann, M., Brömmling, A., Ulbrich, S. E., Weinert, M., Piechotta, M., Wrenzycki, C., et al. (2015). Effect of suppression of postpartum ovulation on endometrial inflammation in dairy cows. Theriogenology 84, 155–162. doi: 10.1016/j.theriogenology.2015.03.003
Herath, S., Lilly, S. T., Santos, N. R., Gilbert, R. O., Goetze, L., Bryant, C. E., et al. (2009). Expression of genes associated with immunity in the endometrium of cattle with disparate postpartum uterine disease and fertility. Reprod. Biol. Endocrinol. 7:55. doi: 10.1186/1477-7827-7-55
Ho, L., Luo, S., and Lai, J. (2015). Biological effects of interleukin 6: Clinical applications in autoimmune diseases and cancers. Biochem. Pharmacol. 97, 16–26. doi: 10.1016/j.bcp.2015.06.009
Ibrahim, L. A., Rizo, J. A., Fontes, P. L. P., Lamb, G. C., and Bromfield, J. J. (2019). Seminal plasma modulates expression of endometrial inflammatory meditators in the bovine. Biol. Reprod. 100, 660–671. doi: 10.1093/biolre/ioy226
Ingman, W. V., and Robertson, S. A. (2002). Defining the actions of transforming growth factor beta in reproduction. BioEssays 24, 904–912. doi: 10.1002/bies.10155
Ishikawa, Y., Nakada, K., Hagiwara, K., Kirisawa, R., Iwai, H., Moriyoshi, M., et al. (2004). Changes in interleukin-6 concentration in peripheral blood of pre- and post-partum dairy cattle and its relationship to postpartum reproductive diseases. J. Vet. Med. Sci. 66, 1403–1408. doi: 10.1292/jvms.66.1403
Jeon, S. J., Vieira-Neto, A., Gobikrushanth, M., Daetz, R., Mingoti, R. D., Parize, A. C., et al. (2015). Uterine microbiota progression from calving until establishment of metritis in dairy cows. Appl. Environ. Microbiol. 81, 6324–6332. doi: 10.1128/AEM.01753-15
Kim, M. R., Park, D. W., Lee, J. H., Choi, D. S., Hwang, K. J., Ryu, H. S., et al. (2005). Progesterone-dependent release of transforming growth factor-beta1 from epithelial cells enhances the endometrial decidualization by turning on the Smad signalling in stromal cells. Mol. Hum. Reprod. 11, 801–808. doi: 10.1093/molehr/gah240
Kontturi, M., Junni, R., Simojoki, H., Malinen, E., Seuna, E., Klitgaard, K., et al. (2019). Bacterial species associated with interdigital phlegmon outbreaks in Finnish dairy herds. BMC Vet. Res. 15:44. doi: 10.1186/s12917-019-1788-x
Machado Pfeifer, L. F., de Souza Andrade, J., Moreira, E. M., Reis da Silva, R., Araújo Neves, P. M., Moreira da Silva, F., et al. (2018). Uterine inflammation and fertility of beef cows subjected to timed AI at different days postpartum. Anim. Reprod. Sci. 197, 268–277. doi: 10.1016/j.anireprosci.2018.08.039
Mao, G., Wang, J., Kang, Y., Tai, P., Wen, J., Zou, Q., et al. (2010). Progesterone increases systemic and local uterine proportions of CD4+CD25+ treg cells during midterm pregnancy in mice. Endocrinology 151, 5477–5488. doi: 10.1210/en.2010-0426
Neira, J. A., Tainturier, D., Peña, M. A., and Martal, J. (2010). Effect of the association of IGF-I, IGF-II, bFGF, TGF-beta1, GM-CSF, and LIF on the development of bovine embryos produced in vitro. Theriogenology 73, 595–604. doi: 10.1016/j.theriogenology.2009.10.015
Nunvar, J., Capek, V., Fiser, K., Fila, L., and Drevinek, P. (2017). What matters in chronic Burkholderia cenocepacia infection in cystic fibrosis: Insights from comparative genomics. PLOS Pathog 13:e1006762. doi: 10.1371/journal.ppat.1006762
Odhiambo, J. F., Poole, D. H., Hughes, L., DeJarnette, J. M., Inskeep, E. K., and Dailey, R. A. (2009). Pregnancy outcome in dairy and beef cattle after artificial insemination and treatment with seminal plasma or transforming growth factor beta-1. Theriogenology 72, 566–571. doi: 10.1016/j.theriogenology.2009.04.013
Oliveira, L. J., and Hansen, P. J. (2008). Deviations in populations of peripheral blood mononuclear cells and endometrial macrophages in the cow during pregnancy. Reproduction 36, 481–490. doi: 10.1530/REP-08-0218
Oliveira, L. J., and Hansen, P. J. (2009). Phenotypic characterization of macrophages in the endometrium of the pregnant cow. Am. J. Reprod. Immunol. 62, 418–426. doi: 10.1111/j.1600-0897.2009.00761.x
Oliveira, L. J., Mansourri-Attia, N., Fahey, A. G., Browne, J., Forde, N., Roche, J. F., et al. (2013). Characterization of the Th profile of the bovine endometrium during the oestrous cycle and early pregnancy. PLoS ONE 8:e75571. doi: 10.1371/journal.pone.0075571
Ott, T. L. (2019). Symposium review: Immunological detection of the bovine conceptus during early pregnancy. J. Dairy. Sci. 102, 3766–3777. doi: 10.3168/jds.2018-15668
Owens, C. E. (2020). Phenotypic and Microbial Influences on Dairy Heifer Fertility and Calf Gut Microbial Development. [Dissertation], Virginia Polytechnic Institute and State University, Blacksburg (VA).
Palevich, N., Kelly, W. J., Leahy, S. C., Denman, S., Altermann, E., Rakonjac, J., et al. (2020). Comparative genomics of rumen Butyrivibrio spp. uncovers a continuum of polysaccharide-degrading capabilities. Appl. Environ. Microbiol. 86, e01993–e01919. doi: 10.1128/AEM.01993-19
Pohler, K. G., Pereira, M. H. C., Lopes, F. R., Lawrence, J. C., Keisler, D. H., Smith, M. F., et al. (2016). Circulating concentrations of bovine pregnancy associated glycoproteins and late embryonic mortality in lactating dairy herds. J. Dairy Sci. 99, 1584–1594. doi: 10.3168/jds.2015-10192
Pohler, K. G., Reese, S. T., Franco, G. A., Oliveira Filho, R. V., Paiva, R., Fernandez, L., et al. (2020). New approaches to diagnose and target reproductive failure in cattle. Anim. Reprod. 17:e20200057. doi: 10.1590/1984-3143-ar2020-0057
Polli, V., Bulktti, C., Galassi, A., Borini, A., Ciotti, P. M., Seracchioli, R., et al. (1996). Transforming growth factor-β1 in the human endometrium. Gynecol. Endocrinol. 10, 297–302. doi: 10.3109/09513599609012815
Ricci, A., Bonizzi, G., Sarasso, G., Gallo, S., Dondo, A., Zoppi, S., et al. (2017). Subclinical endometritis in beef cattle in early and late postpartum: Cytology, bacteriology, haptoglobin and test strip efficiency to evaluate the evolution of the disease. Theriogenology 94, 86–93. doi: 10.1016/j.theriogenology.2017.02.006
Robertson, S. A., Care, A. S., and Moldenhauer, L. M. (2018). Regulatory T cells in embryo implantation and the immune response to pregnancy. J. Clin. Investig. 128, 4224–4235. doi: 10.1172/JCI122182
Robertson, S. A., Ingman, W. V., O'Leary, S., Sharkey, D. J., and Tremellen, K. P. (2002). Transforming growth factor β - a mediator of immune deviation in seminal plasma. J. Reprod. Immunol. 57, 109–128. doi: 10.1016/S0165-0378(02)00015-3
Russell, M. W., Kilian, M., Mantis, N. J., and Corthésy, B. (2015). “Biological activities of IgA,” in Mucosal Immunology, 4th Edn, ed. J. Mesticky (Boston, MA: Elsevier Academic Press), 429–454. doi: 10.1016/B978-0-12-415847-4.00021-5
Santos, N. R., Lamb, G. C., Brown, D. R., and Gilbert, R. O. (2009). Postpartum endometrial cytology in beef cows. Theriogenology 71, 739–745. doi: 10.1016/j.theriogenology.2008.09.043
Santos, T. M. A., Gilbert, R. O., and Bicalho, R. C. (2011). Metagenomic analysis of the uterine bacterial microbiota in healthy and metritic postpartum dairy cows. J. Dairy Sci. 94, 291–302. doi: 10.3168/jds.2010-3668
Scheller, J., Chalaris, A., Schmidt-Arras, D., and Rose-John, S. (2011). The pro- and anti-inflammatory properties of the cytokine interleukin 6. Biochim. Biophys. Acta 1813, 878–888. doi: 10.1016/j.bbamcr.2011.01.034
Schumacher, A., Costa, S., and Zenclussen, A. C. (2014). Endocrine factors modulating immune responses in pregnancy. Front. Immunol. 5:196. doi: 10.3389/fimmu.2014.00196
Sheldon, I. M., Williams, E. J., Miller, A. N. A., Nash, D. M., and Herath, S. (2008). Uterine diseases in cattle after parturition. Vet. J. 176, 115–121. doi: 10.1016/j.tvjl.2007.12.031
Short, R. E., Bellows, R. A., Staigmiller, R. B., Berardinelli, J. G., and Custer, E. E. (1990). Physiological mechanisms controlling anestrus and infertility in postpartum beef cattle. J. Anim. Sci. 68, 799–816. doi: 10.2527/1990.683799x
Sykes, J. A., and Kalan, J. (1975). Intracellular Treponema pallidum in cells of a syphilitic lesion of the uterine cervix. Am. J. Obstet. Gynecol. 122, 361–367. doi: 10.1016/0002-9378(75)90185-4
Tríbulo, P., Siqueira, L. G. B., Oliveira, L. J., Scheffler, T., and Hansen, P. J. (2018). Identification of potential embryokines in the bovine reproductive tract. J. Dairy Sci. 101, 690–704. doi: 10.3168/jds.2017-13221
van Mourik, M. S., Macklon, N. S., and Heijnen, C. J. (2009). Embryonic implantation: cytokines, adhesion molecules, and immune cells in establishing an implantation environment. J. Leukoc. Biol. 85, 4–19. doi: 10.1189/jlb.0708395
Vasudevan, S., Kamat, M. M., Walusimbi, S. S., Pate, J. L., and Ott, T. L. (2017). Effects of early pregnancy on uterine lymphocytes and endometrial expression of immune-regulatory molecules in dairy heifers. Biol. Reprod. 97, 104–118. doi: 10.1093/biolre/iox061
Wagner, W. C., and William, H. (1969). Reproductive Physiology of the Postpartum Cow. Reproduction 18, 493–500. doi: 10.1530/jrf.0.0180493
Wallner, A., King, E., Ngonkeu, E. L. M., Moulin, L., and Béna, G. (2019). Genomic analyses of Burkholderia cenocepacia reveal multiple species with differential host-adaptation to plants and humans. BMC Genomics 20:803. doi: 10.1186/s12864-019-6186-z
Watts, K. M., Fodor, C., Beninger, C., Lahiri, P., Arrazuria, R., De Buck, J., et al. (2018). A differential innate immune response in active and chronic stages of bovine infectious digital dermatitis. Front. Microbiol. 9:1586. doi: 10.3389/fmicb.2018.01586
Wira, C. R., Grant-Tschudy, K. S., and Crane-Godreau, M. A. (2005). Epithelial cells in the female reproductive tract: a central role as sentinels of immune protection. Am. J. Reprod. Immunol. 53, 65–76. doi: 10.1111/j.1600-0897.2004.00248.x
Wooldridge, L. K., and Ealy, A. D. (2019). Interleukin-6 increases inner cell mass numbers in bovine embryos. BMC Dev. Biol. 19:2. doi: 10.1186/s12861-019-0182-z
Wooldridge, L. K., Johnson, S. E., Cockrum, R. R., and Ealy, A. D. (2019). Interleukin-6 requires JAK to stimulate inner cell mass expansion in bovine embryos. Reproduction 158, 303–314. doi: 10.1530/REP-19-0286
Yousef, M. S., Marey, M. A., Hambruch, N., Hayakawa, H., Shimizu, T., Hussien, H. A., et al. (2016). Sperm binding to oviduct epithelial cells enhances TGFB1 and IL10 expressions in epithelial cells as well as neutrophils in vitro: prostaglandin E2 as a main regulator of anti-inflammatory response in the bovine oviduct. PLoS ONE 11:e0162309. doi: 10.1371/journal.pone.0162309
Keywords: beef cow, fertility, cytokines, reproductive microbiome, uterus
Citation: Poole RK, Ault-Seay TB, Payton RR, Myer PR, Lear AS and Pohler KG (2021) Evaluation of Reproductive Tract Cytokines in Post-partum Beef Cows Relating to Reproductive Microbiota and Fertility Outcomes. Front. Anim. Sci. 2:704714. doi: 10.3389/fanim.2021.704714
Received: 03 May 2021; Accepted: 31 May 2021;
Published: 24 June 2021.
Edited by:
Edward Narayan, The University of Queensland, AustraliaReviewed by:
Jamie Larson, Mississippi State University, United StatesA. Menchaca, Fundacion IRAUy, Uruguay
Copyright © 2021 Poole, Ault-Seay, Payton, Myer, Lear and Pohler. This is an open-access article distributed under the terms of the Creative Commons Attribution License (CC BY). The use, distribution or reproduction in other forums is permitted, provided the original author(s) and the copyright owner(s) are credited and that the original publication in this journal is cited, in accordance with accepted academic practice. No use, distribution or reproduction is permitted which does not comply with these terms.
*Correspondence: Ky G. Pohler, a3BvaGxlckB0YW11LmVkdQ==
†These authors have contributed equally to this work and share first authorship