- 1Department of Food Hygiene and Technology, Faculty of Veterinary, University of León, León, Spain
- 2Department of Nutrition and Food Science, Faculty of Medicine, University of Valladolid, Valladolid, Spain
- 3Facultad de Industrias Alimentarias, Universidad Nacional Agraria La Molina, Lima, Peru
- 4Laboratorio de Salud Pública y Salud Ambiental, Facultad de Medicina Veterinaria, Universidad Nacional Mayor de San Marcos, Lima, Peru
Reformulation approaches in the meat industry are required to promote nutritional improvement, health functionality, and reduce environmental impact. A relevant approach among these is to reduce the amount of meat in meat products. Reduced-meat products should maintain or improve the sensory characteristics and nutritive value compared to conventional meat products. Among meat products, heat-treated emulsion-meat sausages are widely consumed and especially suitable for reformulation approaches. Due to its high protein content, with high functionally and biological value, pulse flour has a high potential to be used as meat replacer. Most studies regarding the replacement of meat with pulses have been made on fresh meat preparations where amounts of up to 15% of pulse flour did not negatively affect sensory quality while increased yield and firmness. However, studies using pulse flour in emulsion-type sausages are scarce. Further research is warranted to optimize the reformulation of these meat products using flour pulses. The topics to be addressed are the following: effects of pulse type, pulse pretreatments, such as soaking or germination, pulse flour treatments before incorporation into the meat mix, combination of pulses with other proper ingredients, and heat treatment intensity on the pulse antinutrient inactivation and the technological and edible quality traits of the pulse-containing sausages.
Introduction
A large part of the meat consumed in industrialized countries is previously processed. Versatility, more attractive products, improved cost-effectiveness, and higher shelf life are some of the main features that distinguish meat derivatives from fresh meat. There are many types meat derivatives, which are classified according to whether they are or are not heat treated or whether they are made with whole pieces of meat or minced meat.
Among the last, a relevant type of meat products is that composed of the heat-treated emulsion-type sausages, such as Frankfurters or hot dogs. Heat-treated emulsion-type sausages are made from cooking a homogeneous fine batter, i.e., finely cut meat, fatty tissue, and water, where the particles of meat or fat tissue are not visible, stuffed into casings (Feiner, 2006). The batter consists mainly of immobilized water, dissolved compounds such as common salt, phosphates, or curing agents, protein aggregates, suspended muscle, connective and fat tissue tiny particles, spices particles, and air micro bubbles. The particle size reduction in this type of meat product is carried out by cutting and shearing meat and non-meat ingredients using cutters or colloid mills at temperatures usually lower than 12°C.
Meat is considered a relevant dietary source of a wide range of nutrients, such as high-quality proteins, zinc, iron, selenium, and B12 vitamins (Salter, 2018; Cocking et al., 2020). On the other hand, an excess of meat and meat products in the diet is seem a problem in rich countries due to meat contributing to a diet with high fat, and cholesterol contents, unfavorable fatty acid profile, and the eventual presence of nitrites as additives (Grasso et al., 2014). Evidence from medical research indicated that long-term excessive meat intake, particularly red meat and processed meat, has been associated with increased risk of total mortality due to chronic diseases, such as cardiovascular disease, type 2 diabetes, and certain types of cancer such as colon cancer (De Smet and Vossen, 2016; Domingo and Nadal, 2017; Ekmekcioglu et al., 2018).
Concerns on excessive meat consumption are not only based on human health issues but also on the negative environmental impacts of intensive farming (Kowalski, 2019; Pintado and Delgado-Pando, 2020). Farmland used for livestock feed production, nitrogen emissions, and greenhouse gas emissions has been estimated to be reduced by 20, 40, and 25–40%, respectively, with a 50% reduction in meat and dairy consumption in Europe (Westhoek et al., 2014).
Furthermore, the steady global population growth and industrial development have resulted in an increased demand for food and meat production (Flynn et al., 2019). In the context of achieving global food security, the increasing rate of meat consumption expected for the following years will be contentious to maintain (Hicks et al., 2018). This arises an urgent need for new protein-rich alternatives to animal food in human nutrition (Fasolin et al., 2019). The main problem is that animal protein production is poorly efficient compared to plant protein (Nadathur et al., 2017), which can be estimated by comparing the grams of protein delivered to the wholesale point per unit energy used or per unit total greenhouse gases. About 30% of the global land surface (7% for feed and 23% of pasture) is needed for animal production to meet humans' food needs, while about 7% is used for cropland for human consumption (Stoll-Kleemann and O'Riordan, 2015; Alexander et al., 2016; Salter, 2018).
The health, environmental, and food security problems associated with an excess of meat production and consumption have seemed to be appealing to a segment of Western meat-eaters to adopt specific meat consumption approaches aimed at reducing the amount of meat in their diets (Hicks et al., 2018; Sánchez-Sabaté and Sabaté, 2019). Among them, an emerging approach is to diversify the food types in the diet in order to contribute to more sustainable use of meat and consumption habits, while achieving a high nutritional value. This means a trend toward flexitarian diets (Derbyshire, 2017; Hicks et al., 2018).
Considering the above-mentioned concerns and consumers' tendencies and regarding the processed meat industry, reformulation strategies are needed in order to obtain either healthier processed meat or reduced-meat products. Approaches to deliver healthier meat products have been aimed at fat, sodium, or nitrite reduction, fatty acid profile modification, and inclusion of calcium, vitamins, and nutritional, functional ingredients such as fiber or phytochemicals (Grasso et al., 2014; Thøgersen and Bertram, 2021). Strategies to deliver reduced meat products have been based on a partial replacement of meat (muscle protein) with vegetal ingredients with high protein content, with the suggested ingredients being grain legumes, cereals, oilseeds, or mushrooms or protein concentrates obtained from them (Asgar et al., 2017).
Reformulation Approaches for Reduced-Meat Heat-Treated Emulsion-Type Sausages
Muscle proteins exert different technological–functional properties, which are crucial for the edible quality of the resulting meat products, i.e., viscoelastic textural characteristics responsible for their chewiness and mouthfeel (Sha and Xiong, 2020). The muscle proteins with higher functionality in meat products are myofibrillar proteins, mainly myosin, and collagen (Asgar et al., 2017). In the making process, thanks to the ionic strength provided by the common salt and other salts such as phosphates and the cutter's mechanical energy, a large amount of the myofibrillar proteins are solubilized. The proteins activated immobilize water, interact among them, and with the hydrocolloids, e.g., starch or carrageenan, which are eventually used as ingredients, and cover and emulsify the small fat particles formed. Moreover, when the sausage is cooked, proteins, and other gelling agents form a high viscoelastic gel (Sikorski, 2001; Sha and Xiong, 2020).
The partial replacement of the muscle proteins by non-meat proteins, such as soy protein, caseinates or gluten, pork skin or gelatin, starches or gums, has been standard in the emulsion-type meat sausage-making process (Santhi et al., 2015). This practice's primary purpose is to reduce the cost of raw materials, being usually the meat the more expensive raw material (Feiner, 2006). According to this author, sausages with non-muscle protein and meat extenders can show an acceptable cost/eating quality ratio; however, if a large amount of meat is replaced, the nutritive value of sausage can be significantly reduced.
The reformulation of emulsion-type sausages toward meat reduction cannot only be directed to reduce the costs of raw materials, but it can also be primary aimed at making sausages healthier. The main approaches used to accomplish this goal include the use of vegetable oils as animal fat replacers or the utilization of phytochemical- or dietary fiber-rich ingredients in the formulations (Weiss et al., 2010; Kaur and Sharma, 2019; Das et al., 2020). Moreover, reformulation can also reduce the meat content and consequently diminish the environmental effect associated with excessive meat production. In this approach, the critical question is to obtain sausages with lower meat content (reduced-meat products), as nutritious as the conventional meat products or even more, and showing sensory characteristics quite similar to those of the conventional meat products in order to avoid rejection by frequent meat consumers (Hoek et al., 2011).
Pulses as Candidate Ingredients to Prepare Reduced-Meat Products
Dry grain legumes or pulses, such as lentils, chickpeas, or beans, are foods produced and consumed in large amounts worldwide and are considered relatively cheap healthy food in most regions (Headey and Alderman, 2019). Furthermore, they can be used for intercropping and cover crops, thus enhancing their production sustainability (Maitra et al., 2021). As for their nutritional value, pulses contain high levels of proteins (20–30%), minerals, B-group vitamins, and dietary fiber, apart from several phytochemicals (Farooq and Boye, 2011). In vitro protein digestibility of pulse flours has been reported to be around 80% (Bessada et al., 2019) and their essential amino acid index ranged 50–65% (Khattab et al., 2009). The protein quality of pulses as regards to the essential amino acid content is relatively high as compared with cereals, however, it is worse than that of meat (Table 1), which is due to a low content in sulfur amino acids and tryptophan, the limiting amino acids in pulse protein (Iqbal et al., 2006; Boye et al., 2010; Grela et al., 2017).
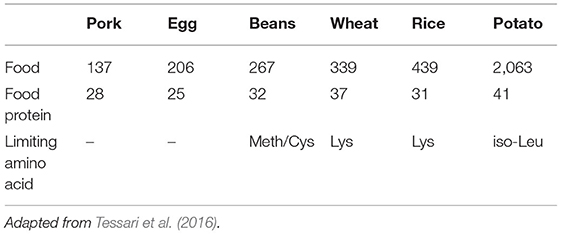
Table 1. Amounts (g) of food or food protein to provide the recommended daily allowance for the corresponding limiting essential amino acid, referred to a 70-kg man, considering a protein content of 21, 11, 12, 11, 7, and 2% for pork, egg, beans, wheat, rice, and potato, respectively.
Nonetheless, when pulses are combined with rice or egg protein, the protein biological value considerably improves (Farooq and Boye, 2011). Pulses also contain a large amount of starch (50–70%), composed of amylose and amylopectin chains. Its high resistance to digestion confers the pulse starch a dietary interest since it acts as dietary fiber (Singh et al., 2017).
Pulses, or proteins extracted from them, have been suggested as ingredients to be considered in reformulation of processed meat. The principal proteins in pulses are albumins and globulins. The firsts are water-soluble and are composed of enzymes, lectins, enzymatic inhibitors. Globulins, also known as reserve proteins, are the most abundant and are soluble in saline solutions (Singhal et al., 2016). These proteins are the main ones responsible for functionality, i.e., water retention, emulsion, gel formation, attributed to pulse proteins (Farooq and Boye, 2011; Toews and Wang, 2013; Shevkani et al., 2017; Jarpa-Parra, 2018). Their functional performance is comparable or even higher to soy protein, which is frequently used in the meat industry.
The pulses' starch forms gels at temperatures between 70 and 90°C (Farooq and Boye, 2011). When pulses are mixed with other ingredients, for making bakery, pasta, food emulsions, or meat products, both proteins and starches establish chemical links and form structures of different degree of firmness depending on the food matrix pH, ionic strength, gelling agent concentrations, fat content, or heating conditions (Sozer et al., 2017).
A relevant problem derived from the utilization of pulse flours as ingredients in processed food is that pulses contain a high amount of natural antinutrient compounds necessary to reduce (Paterson et al., 2017). These can be classified according to their composition in non-proteinaceous and proteinaceous. Among the first type are phytic acid, oxalate, saponins, and cyanogenic glycosides that can interact with food proteins, mineral, vitamins, or carbohydrates. The second group is composed of lectins, agglutinins, trypsin, chymotrypsin, and amylase inhibitors that interact with human enzymes or can agglutinate red blood cells (Sreerama et al., 2012).
Different pulse processing techniques, such as dehulling, soaking, extrusion, micronization, heating, germination, or fermentation, have been found to reduce the content of antinutrient compounds in pulse flours (Kumar et al., 2009; Paterson et al., 2017; Kaspchak et al., 2018). Dehulling mainly decreases the tannin content in seeds, between 68 and 90%, and improves their nutritional quality (Kumar et al., 2009). Soaking is effective in reducing the tannin and phytic acid contents to an extent depending on soaking conditions, i.e., time and temperature combinations, and pulse type (Martín-Cabrejas et al., 2009; Paterson et al., 2017). The reduction tends to be higher when pulses are soaked in salt solutions (Taiwo and Akanbi, 1997). Heat treatment considerably reduces phytic acid and tannin contents and is the most effective process to reduce trypsin inhibitors (Osman, 2007; López-Martínez et al., 2017); however, due to their remarkable heat stability, an intense heating treatment is needed, e.g., boiling or autoclaving, to reduce most of the activity (Chan et al., 2014). Finally, germination and fermentation improve the digestibility and the availability of certain nutrients, i.e., minerals, and reduce the levels of antinutrient compounds, such as phytic acid, tripsin inhibitors, and α-galactosides; however, the reduction of enzyme inhibitors by these processes is lower than boiling or autoclaving (Kumar et al., 2009; Wood and Malcolmson, 2011; Gharachorloo et al., 2013; Paterson et al., 2017).
Research on The Utilization Of Pluses as Meat Replacers in Processed Meat
The research on the utilization of pulse flour as a meat replacer is not new. As a matter of example, Verna et al. (1984) studied the effect of the partial replacement of meat with chickpea flour, based on a protein-to-protein replacement, on the microbial quality of English-type fresh sausages prepared from either mutton, pork, or beef. The technological interest in the use of pulses in the meat industry keeps active. Recent research studies on the reformulation of minced-meat preparations such as burgers, patties, meatballs, and fresh sausages with pulses as meat replacers can be found in the literature (Serdaroglu et al., 2005; Holliday et al., 2011; Ghribi et al., 2018; Argel et al., 2020; Pintado and Delgado-Pando, 2020). In these studies, pulse flour was used at levels between 5 and 50%, with a reduction in the meat content from c.a. 5 to 40%. Results have shown, in general, that the pulse flour at amounts lower than 15% increased the cooking yield and firmness of the meat preparations without exerting, in most cases, a negative effect on the sensory acceptation. Furthermore, it has been found that the effect of flour pulses on the quality of reduced meat preparations not only depends on the flour amount used, and the amount of meat that was replaced, but also on the type of pulse (Holliday et al., 2011).
However, research on the use of pulses in emulsion-type cooked meat products seems to be scarce. Dzudie et al. (2002), Sanjeewaa et al. (2010), and Albarracín et al. (2010) prepared emulsion-type sausages with bean or chick pea flours at levels up to 10%, and a subsequent meat reduction equal to pulse flour added. Furthermore, Tahmasebi et al. (2016) formulated sausages with variable amounts of pigeon pea flour (up to 22%), combined with corn flour, walnut, and sesame paste. A summary of the methodology and results from those studies found are shown in Table 2. In general, the use of pulse flour increased sausage yield and emulsion stability and increased lightness (L*). Regarding texture, the four studies reported increased cohesiveness due to pulse flour and three out of the four higher hardness, although an inverse effect was observed in the other study (Dzudie et al., 2002). Finally, the effect of pulse flour on consumers' acceptance was not consistent, i.e., while Albarracín et al. (2010) reported lower acceptance scores for Frankfurters with pulse flour, (Sanjeewaa et al., 2010) found no negative effect of pulse flour on the sausage flavor.
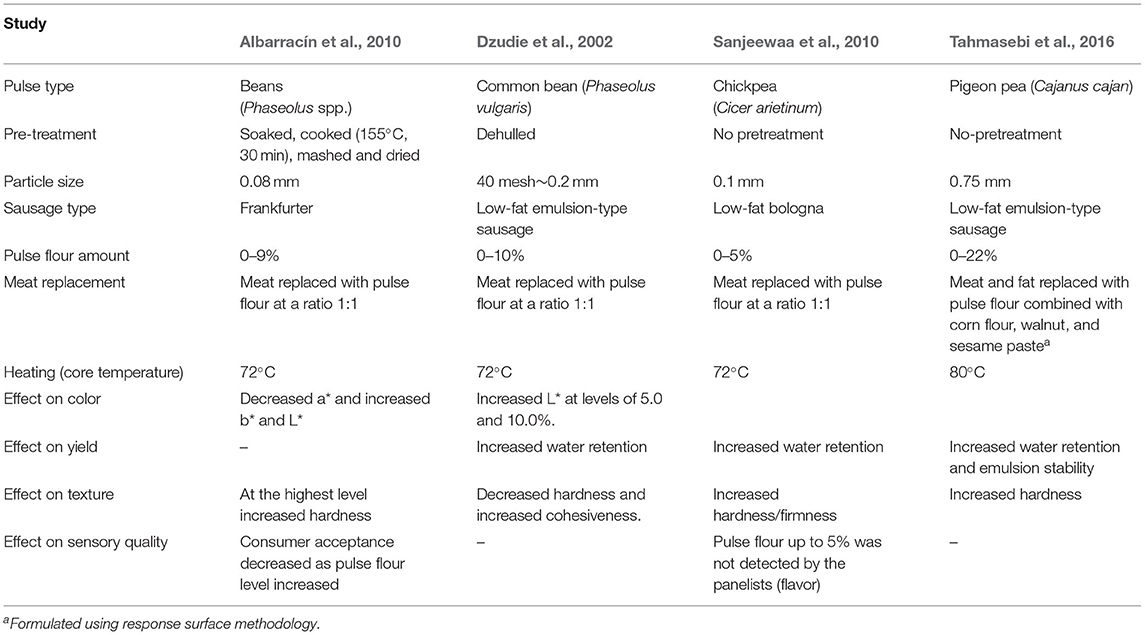
Table 2. Methodology and results from studies testing the effect pulse flours on the quality of emulsion-type sausages.
Reformulation Challenges to Include Pulses in Heat-Treated Emulsion-Type Sausages
Due to the scarcity of scientific information, further research on the reformulation of emulsion-type meat sausages using pulses is deserved, and the lines to explore are many. As relevant research, the effects of different levels of meat (muscle proteins) replacement by pulse flour (pulse proteins) on the sausage quality traits should be studied. In these reformulations, the amounts of protein, water, and starch should be optimized. Furthermore, the incorporation of pulses could be combined with other functional proteins such as collagen or albumin, which could improve the technological functionality and the sausage edible quality and nutritive value. The response surface methodology and mixture design could be used as a valuable tool to select the best formulations (Arteaga et al., 1994). Moreover, the flour of different pulses should be tested, and their performance compared among them.
Previous to the research mentioned above, suitable procedures to prepare the pulse flours and incorporate them in the sausage-making process (pulse flour pre-treatment) should be researched. Pulse flour functional properties such as protein solubility, water absorption, or pasting properties, among others, would depend on pulse pre-treatments such as soaking, dehulling, germination, or heating (Felker et al., 2003; Wood and Malcolmson, 2011; Ribéreau et al., 2018). Furthermore, functional properties of pulse flours as food ingredients are significantly affected by flour particle size; thus the particle size should be appropriate for the product concerned (Wood and Malcolmson, 2011).
Moreover, before adding the pulse flour to the sausage batter, mixing or homogenizing pulse flour in water or saline could improve pulse protein and starch functionality. This statement's rationale regarding proteins is that globulins, the more significant proteins in pulses, are highly soluble in saltwater (Bessada et al., 2019). It might be advantageous to solubilise these proteins before incorporating the pulse flour into the sausage batter.
Regarding pulse antinutrients, it should be relevant to study to what extent pulse or pulse flour pre-treatments, i.e., soaking, germination, toasting, or the heat-treatment intensity of the pulse-containing sausages, would inactivate or remove those compounds. On the other hand, it should be investigated how the pre-treatment, supposing pulses are pre-treated to diminish their antinutrient content (Bessada et al., 2019), could affect pulse proteins' technological functionality and how it could affect the edible sausage yield and texture quality. Furthermore, the increment in the cost of using processed legumes instead of non-treated pulses should also be considered.
The effect of relevant sausage processing conditions such as the amount of phosphates required, the optimum cuterization time or the heating intensity warrant further research. For the latter, it should be considered that gelatinization temperature of pulse starch is higher (70–90°C; Farooq and Boye, 2011) than that of the conventionally used potato starch (about 60°C; Feiner, 2006).
Possible adverse effects of pulse flour on the quality of emulsion-type sausages might be manifested, and, in this case, the defects should be addressed with appropriate approaches. The high content of fiber in pulses might result in a less accepted texture, e.g., excessive hardness or atypical mouthfeel, compared to conventional sausages. In this respect, the use of flours from dehulled pulses instead of wholemeal flour, can be considered to reduce fiber content (Wood and Malcolmson, 2011). Pulse flours could also be accompanied with collagen to improve mouthfeel. In this regard, Hjelm et al. (2019) found the use of collagen protein (1–3 mg/100 g) to ameliorate the negative effect of the inclusion of fiber (rye bran) on Frankfurter texture. Nonetheless, fibre's adverse effect might not be detected since Cofrades et al. (2000) did not found fiber (2% oat fiber) to affect the texture of emulsion-type sausages.
Furthermore, the effect of pulses in the sausage color might diminish its color acceptance, and it could also exert an eventual negative effect on flavor. Pulses could impart the sausage an undesirable “beany” flavor (Bessada et al., 2019) or promote lipid oxidation due to the action of lipoxidase, which seems to be able to resist the sausage pasteurization heating treatment (Wood and Malcolmson, 2011). On the other hand, pulses such as lentils are rich in polyphenols, thus being a source of natural antioxidants (Munekata et al., 2020), thus improving the oxidative stability of sausages. Besides, the effects of incorporating pulse flour on microbial concentration, microbial growth, and sausage shelf life also deserve to be studied and understood.
In conclusion, the utilization of pulse flours in the formulation of reduced-meat heat-treated emulsion meat sausages without resulting in an adverse effect on the sausage quality seems feasible. Nonetheless, further research is warranted to develop best practices and strategies. This research should aim to prevent the presence of dangerous levels of pulse antinutrients, achieve a high pulse protein functionality and search for suitable combinations of pulse flour with proteins, i.e., collagen or albumin, or spices and condiments to improve the quality of pulse-containing sausages.
Author Contributions
JM: conception, compiling references, writing, drafting, and coordination. IC, SK, and AC: compiling references and writing. BS: conception, compiling references, and writing. DR: funding, conception, writing, and coordination. All authors contributed to the article and approved the submitted version.
Funding
This study is part of the project A2008001m, titled Embutidos funcionales de bajo costo para recuperar deficiencias nutricionales, inmunitarias y económicas caudadas por la pandemia COVID-19 funded by the Vicerrectorado de Investigación y Posgrado, Universidad Nacional Mayor de San Marcos.
Conflict of Interest
The authors declare that the research was conducted in the absence of any commercial or financial relationships that could be construed as a potential conflict of interest.
References
Albarracín, W., Acosta, L. F., and Sánchez, I. C. (2010). Elaboration of a scalded sausage using common bean flour (Phaseolus spp.) as extender. Vitae 17, 264–271.
Alexander, P., Brown, C., Arneth, A., Finnigan, J., and Rounsevell, M. (2016). Human appropriation of land for food: the role of diet. Glob. Environ. Change 41, 88–98. doi: 10.1016/j.gloenvcha.2016.09.005
Argel, N. S., Ranalli, N., Califiano, A. N., and Andrés, S. C. (2020). Influence of partial pork meat replacement by pulse flour on physicochemical and sensory characteristics of low-fat burgers. J. Sci. Food Agric. 100, 3932–3941. doi: 10.1002/jsfa.10436
Arteaga, G. E., Li-Chan, E., Vázquez-Arteaga, M. C., and Nakai, S. (1994). Systematic experimental designs for product formula optimization. Trends Food Sci. Technol. 5, 243–254. doi: 10.1016/0924-2244(94)90017-5
Asgar, M. A., Fazilah, A., Huda, N., Bhat, R., and Karim, A. A. (2017). Nonmeat protein alternatives as meat extenders and meat analogs. Comprehen. Rev. Food Sci. Food Saf. 9, 513–529. doi: 10.1111/j.1541-4337.2010.00124.x
Bessada, M. F., Barreira, J. C. M., and Oliveira, M. B. P. P. (2019). Pulses and food security: dietary protein, digestibility, bioactive and functional properties. Trends Food Sci. Technol. 93, 53–68. doi: 10.1016/j.tifs.2019.08.022
Boye, J., Zare, F., and Pletch, A. (2010). Pulse proteins: processing, characterization, functional properties and applications in food and feed. Food Res. Int. 43, 414–431. doi: 10.1016/j.foodres.2009.09.003
Chan, Y. S., Zhang, Y., Sze, S. C. W., and Ng, T. B. (2014). A thermostable trypsin inhibitor with antiproliferative activity from small pinto beans. J. Enzyme Inhib. Med. Chem. 29, 485–490. doi: 10.3109/14756366.2013.805756
Cocking, C., Walton, J., Kehoe, L., Cashman, K. D., and Flynn, A. (2020). The role of meat in the European diet: current state of knowledge on dietary recommendations, intakes and contribution to energy and nutrient intakes and status. Nutr. Res. Rev. 33. 181–189. doi: 10.1017/S0954422419000295
Cofrades, S., Hughes, E., and Troy, D. (2000). Effects of oat fibre and carrageenan on the texture of frankfurters formulated with low and high fat. Eur. Food Res. Technol. 211, 19–26. doi: 10.1016/s0309-1740(96)00109-x
Das, A. K., Nanda, P. K., Madne, P., Biswas, S., Das, A., Zhang, W., et al. (2020). A comprehensive review on antioxidant dietary fibre enriched meat-based functional foods. Trends Food Sci. Technol. 99, 323–336. doi: 10.1016/j.tifs.2020.03.010
De Smet, S., and Vossen, E. (2016). Meat: the balance between nutrition and health. A review. Meat Sci. 120, 145–156. doi: 10.1016/j.meatsci.2016.04.008
Derbyshire, E. J. (2017). Flexitarian diets and health: a review of the evidence-based literature. Front. Nutr. 3:55. doi: 10.3389/fnut.2016.00055
Domingo, J. L., and Nadal, M. (2017). Carcinogenicity of consumption of red meat and processed meat: a review of scientific news since the IARC decision. Food Chem. Toxicol. 105, 256–261. doi: 10.1016/j.fct.2017.04.028
Dzudie, T., Scher, J., and Hardy, J. (2002). Common bean flour as an extender in beef sausages. J. Food Engin. 52, 143–147. doi: 10.1016/S0260-8774(01)00096-6
Ekmekcioglu, C., Wallner, P., Kundi, M., Weisz, U., Haas, W., and Hutter, H. P. (2018). Red meat, diseases, and healthy alternatives: a critical review. Crit. Rev. Food Sci. Nutr. 58, 247–261. doi: 10.1080/10408398.2016.1158148
Farooq, Z., and Boye, J. I. (2011). “Novel food and industrial applications of pulse flours and fractions,” in Pulse Foods Processing, Quality and Nutraceutical Applications, eds B. K. Tiwari, A. Gowen, and B. McKenna (Oxford: Academic Press), 283–324. doi: 10.1016/B978-0-1238-2018-1.00007-0
Fasolin, L. H., Pereira, R. N., Pinheiro, A. C., Martins, J. T., Andrade, C. C. P., Ramos, O. L., et al. (2019). Emergent food proteins – towards sustainability, health and innovation. Food Res. Int. 125:108586. doi: 10.1016/j.foodres.2019.108586
Feiner, G. (2006). Meat Products Handbook: Practical Science and Technology. Cambridge, UK: CRC Press. doi: 10.1533/9781845691721
Felker, P., Grados, N., Cruz, G., and Prokopiuk, D. (2003). Economic assessment of production of flour from Prosopis alba and P. pallida pods for human food applications. J. Arid Environ. 53, 517–528. doi: 10.1006/jare.2002.1064
Flynn, K., Villarreal, B. P., Barranco, A., Belc, N., Björnsdóttir, B., Fusco, V., et al. (2019). An introduction to current food safety needs. Trends Food Sci. Technol. 84, 1–3. doi: 10.1016/j.tifs.2018.09.012
Gharachorloo, M., Ghiassi Tarzi, B., and Baharinia, M. (2013). The effect of germination on phenolic compounds and antioxidant activity of pulses. J. Am. Oil Chem. Soc. 90, 407–411. doi: 10.1007/s11746-012-2170-3
Ghribi, A. M., Amira, A. B., Gafsi, I. M., Lahiani, M., Bejar, M., Triki, M., et al. (2018). Toward the enhancement of sensory profile of sausage “Merguez” with chickpea protein concentrate. Meat Sci. 143, 74–80. doi: 10.1016/j.meatsci.2018.04.025
Grasso, S., Brunton, N. P., Lyng, J. G., Lalor, F., and Monahan, F. J. (2014). Healthy processed meat products - regulatory, reformulation and consumer challenges. Trends Food Sci. Technol. 39, 4–17. doi: 10.1016/j.tifs.2014.06.006
Grela, E. R., Kiczorowska, B., Samolińska, W., Matras, J., Kiczorowski, P., Rybiński, W., et al. (2017). Chemical composition of leguminous seeds: part I—content of basic nutrients, amino acids, phytochemical compounds, and antioxidant activity. Eur. Food Res. Technol. 243, 1385–1395. doi: 10.1007/s00217-017-2849-7
Headey, D. D., and Alderman, H. H. (2019). The relative caloric prices of healthy and unhealthy foods differ systematically across income levels and continents. J. Nutr. 149, 2020–2033. doi: 10.1093/jn/nxz158
Hicks, T. M., Knowles, S. O., and Farouk, M. M. (2018). Global provisioning of red meat for flexitarian diets. Front. Nutr. 5:50. doi: 10.3389/fnut.2018.00050
Hjelm, L., Mielby, L. A., Gregersen, S., Eggers, N., and Bertram, H. C. (2019). Partial substitution of fat with rye bran fibre in Frankfurter sausages – bridging technological and sensory attributes through inclusion of collagenous protein. LWT Food Sci. Technol. 101, 607–617. doi: 10.1016/j.lwt.2018.11.055
Hoek, A., Luning, P. A., Weijzen, P., Engels, W., Kok, F. J., and de Graaf, C. (2011). Replacement of meat by meat substitutes. A survey on person- and product-related factors in consumer acceptance. Appetite 56, 662–673. doi: 10.1016/j.appet.2011.02.001
Holliday, D. L., Sandlin, C., Schott, A., Malekian, F., and Finley, J. W. (2011). Characteristics of meat or sausage patties using pulses as extenders. J. Culin. Sci. Technol. 9, 158–176. doi: 10.1080/15428052.2011.594731
Iqbal, A., Khalil, I. A., Ateeq, N., and Khan, M. S. (2006). Nutritional quality of important food legumes. Food Chem. 97, 331–335. doi: 10.1016/j.foodchem.2005.05.011
Jarpa-Parra, M. (2018). Lentil protein: a review of functional properties and food application. An overview of lentil protein functionality. Int. J. Food Sci. Technol. 53, 892–903. doi: 10.1111/ijfs.13685
Kaspchak, E., Mafra, L. I., and Mafra, M. R. (2018). Effect of heating and ionic strength on the interaction of bovine serum albumin and the antinutrients tannic and phytic acids, and its influence on in vitro protein digestibility. Food Chem. 252, 1–8. doi: 10.1016/j.foodchem.2018.01.089
Kaur, R., and Sharma, M. (2019). Cereal polysaccharides as sources of functional ingredient for reformulation of meat products: a review. J. Funct. Foods 62:103527. doi: 10.1016/j.jff.2019.103527
Khattab, R. Y., Arntfield, S. D., and Nyachoti, C. M. (2009). Nutritional quality of legume seeds as affected by some physical treatments, part 1: protein quality evaluation. LWT Food Sci. Technol. 42, 1107–1112. doi: 10.1016/j.lwt.2009.02.008
Kowalski, J. K. (2019). Sustainability impacts of pulses in meat-analogue food products. Cereal Foods World 64:52. doi: 10.1094/CFW-64-5-0052
Kumar, J. A., Sudhir, K., and Panwar, J. D. S. (2009). Antinutritional factors and their detoxification in pulses – a review. Agric. Rev. 30, 64–70.
López-Martínez, L. X., Leyva-López, N., Gutiérrez-Grijalva, E. P., and Heredia, J. B. (2017). Effect of cooking and germination on bioactive compounds in pulses and their health benefits. J. Funct. Foods 38, 624–634. doi: 10.1016/j.jff.2017.03.002
Maitra, S., Hossain, A., Brestic, M., Skalicky, M., Ondrisik, P., Gitari, H., et al. (2021). Intercropping—a low input agricultural strategy for food and environmental security. Agronomy 11:343. doi: 10.3390/agronomy11020343
Martín-Cabrejas, M. A., Aguilera, Y., Pedrosa, M. M., Cuadrado, C., Hernández, T., Díaz, S., et al. (2009). The impact of dehydration process on antinutrients and protein digestibility of some legume flours. Food Chem. 114, 1063–1068. doi: 10.1016/j.foodchem.2008.10.070
Munekata, P. E. S., Gullón, B., Pateiro, M., Tomasevic, I., Domínguez, R., and Lorenzo, J. M. (2020). Natural antioxidants from seeds and their application in meat products. Antioxidants 9:815. doi: 10.3390/antiox9090815
Nadathur, S. R., Wanasundara, J. P. D., and Scanlin, L. (2017). “Proteins in the diet: challenges in feeding the global population,” in Sustainable Protein Sources, eds S. R. Nadathur, J. P. D. Wanasundara, and L. Scanlin (London, UK: Academic Press), 1–19. doi: 10.1016/B978-0-12-802778-3.00001-9
Osman, M. A. (2007). Effect of different processing methods, on nutrient composition, antinutritional factor, and invitro protein digestibility of Dolichos Lablab bean [Lablab purpuresus (L) Sweet]. Pak. J. Nutr. 6, 299–303. doi: 10.3923/pjn.2007.299.303
Paterson, C. A., Curran, J., and Der, T. (2017). Effect of processing on antinutrient compounds in pulses. Cereal Chem. 94, 2–10. doi: 10.1094/CCHEM-05-16-0144-FI
Pintado, T., and Delgado-Pando, G. (2020). Towards more sustainable meat products: extenders as a way of reducing meat content. Foods 9:1044. doi: 10.3390/foods9081044
Ribéreau, S., Aryee, A. N. A., Tanvier, S., Han, J., and Boye, J. I. (2018). Composition, digestibility, and functional properties of yellow pea as affected by processing. J Food Process Preserv. 42:e13375. doi: 10.1111/jfpp.13375
Salter, A. M. (2018). The effects of meat consumption on global health. Rev. Sci. Tech. 37, 47–55. doi: 10.20506/rst.37.1.2739
Sánchez-Sabaté, R., and Sabaté, J. (2019). Consumer attitudes towards environmental concerns of meat consumption: a systematic review. Int. J. Environ. Res. Public Health 16:1220. doi: 10.3390/ijerph16071220
Sanjeewaa, T. W. G., Wanasundara, J. P. D., Pietrasika, Z., and Shanda, P. J. (2010). Characterization of chickpea (Cicer arietinum L.) flours and application in low-fat pork bologna as a model system. Food Res. Int. 43, 617–623. doi: 10.1016/j.foodres.2009.07.024
Santhi, D., Kalaikannan, A., and Sureshkumar, S. (2015). Factors influencing meat emulsion properties and product texture: a review. Crit. Rev Food Sci Nutr. 57, 2021–2027. doi: 10.1080/10408398.2013.858027
Serdaroglu, M., Yildiz-Turp, G., and Abordímov, K. (2005). Quality of low-fat meatballs containing Legume flours as extenders. Meat Sci. 70, 99–105. doi: 10.1016/j.meatsci.2004.12.015
Sha, L., and Xiong, Y. L. (2020). Plant protein-based alternatives of reconstructed meat: science, technology, and challenges. Trends Food Sci. Technol. 102, 51–61. doi: 10.1016/j.tifs.2020.05.022
Shevkani, K., Singh, N., Chen, Y., Kaur, A., and Yu, L. (2017). Pulse proteins: secondary structure, functionality and applications. J. Food Sci. Technol. 56, 2787–2798. doi: 10.1007/s13197-019-03723-8
Sikorski, Z. E. (2001). “Functional properties of proteins in food systems,” in Chemical and Functional Properties of Food Proteins, ed Z. E. Sikorski (Boca Raton, FL: CRC Press), 113–132. doi: 10.1201/9781482279047
Singh, B., Singh, J. P., Shevkani, K., Singh, N., and Kaur, A. (2017). Bioactive constituents in pulses and their health benefits. J. Food Sci. Technol. 54, 858–870. doi: 10.1007/s13197-016-2391-9
Singhal, A., Karaca, A. C., Tyler, R., and Nickerson, M. (2016). “Pulse proteins: from processing to structure-function relationships,” in Grain Legumes, ed. A. K. Goyal (London, UK: IntechOpen), 55–78. doi: 10.5772/64020
Sozer, N., Holopainen-Mantila, U., and Poutanen, K. (2017). Traditional and new food uses of pulses. Cereal Chem. 94, 66–73. doi: 10.1094/CCHEM-04-16-0082-FI
Sreerama, Y. N., Sashikala, V. B., and Pratape, V. M. (2012). Phenolic compounds in cowpea and horse gram flours in comparison to chickpea flour: evaluation of their antioxidant and enzyme inhibitory properties associated with hyperglycemia and hypertension. Food Chem. 133, 156–162. doi: 10.1016/j.foodchem.2012.01.011
Stoll-Kleemann, S., and O'Riordan, T. (2015). The sustainability challenges of our meat and dairy diets. Environ. Sci. Policy Sustain. Dev. 57, 34–48. doi: 10.1080/00139157.2015.1025644
Tahmasebi, M., Labbafi, M., Emam-Djomeh, Z., and Yarmand, M. S. (2016). Manufacturing the novel sausages with reduced quantity of meat and fat: the product development, formulation optimization, emulsion stability and textural characterization. LWT Food Sci. Technol. 68, 76–84. doi: 10.1016/j.lwt.2015.12.011
Taiwo, K. A., and Akanbi, O. C. (1997). The effects of soaking and cooking time on the cooking properties of two cowpea varieties. J. Food Eng. 33, 337–346. doi: 10.1016/S0260-8774(97)87278-0
Tessari, P., Lante, A., and Mosca, G. (2016). Essential amino acids: master regulators of nutrition and environmental footprint? Sci. Rep. 6:26074. doi: 10.1038/srep26074
Thøgersen, B., and Bertram, H. C. (2021). Reformulation of processed meat to attenuate potential harmful effects in the gastrointestinal tract – a review of current knowledge and evidence of health prospects. Trends Food Sci. Technol. 108, 111–118. doi: 10.1016/j.tifs.2020.12.015
Toews, R., and Wang, N. (2013). Physicochemical and functional properties of protein concentrates from pulses. Food Res. Int. 52, 445–451. doi: 10.1016/j.foodres.2012.12.009
Verna, M. M., Ledward, D. A., and Lawrie, R. A. (1984). Utilization of chickpea flour in sausages. Meat Sci. 11, 109–121.
Weiss, J., Gibis, M., Schuh, V., and Saminen, H. (2010). Advances in ingredient and processing systems for meat and meat products. Meat Sci. 86, 196–213. doi: 10.1016/j.meatsci.2010.05.008
Westhoek, H., Lesschen, J. P., Rood, T., Wagner, S., De Marco, A., Murphy-Bokern, D., et al. (2014). Food choices, health and environment: effects of cutting Europe's meat and dairy intake. Glob. Environ. Chang. 26, 196–205. doi: 10.1016/j.gloenvcha.2014.02.004
Keywords: dry-grain legumes, reformulation, extenders, Frankfurters, meat reduction
Citation: Mateo J, Caro I, Kasiayan S, Salvá BK, Carhuallanqui A and Ramos DD (2021) Potential of Pulse Flours as Partial Meat Replacers in Heat-Treated Emulsion-Type Meat Sausages. Front. Anim. Sci. 2:693086. doi: 10.3389/fanim.2021.693086
Received: 09 April 2021; Accepted: 16 June 2021;
Published: 09 July 2021.
Edited by:
Ruben Dominguez, Centro Tecnologico de la Carne, SpainReviewed by:
Jose Angel Pérez-Alvarez, Miguel Hernández University of Elche, SpainCamila Paglarini, Federal University of Mato Grosso, Brazil
Copyright © 2021 Mateo, Caro, Kasiayan, Salvá, Carhuallanqui and Ramos. This is an open-access article distributed under the terms of the Creative Commons Attribution License (CC BY). The use, distribution or reproduction in other forums is permitted, provided the original author(s) and the copyright owner(s) are credited and that the original publication in this journal is cited, in accordance with accepted academic practice. No use, distribution or reproduction is permitted which does not comply with these terms.
*Correspondence: Javier Mateo, am1hdG8mI3gwMDA0MDt1bmlsZW9uLmVz